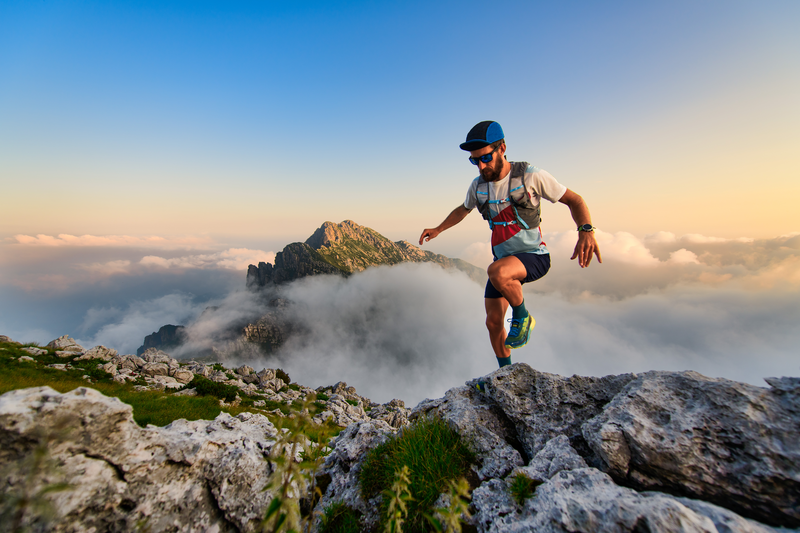
94% of researchers rate our articles as excellent or good
Learn more about the work of our research integrity team to safeguard the quality of each article we publish.
Find out more
ORIGINAL RESEARCH article
Front. Plant Sci. , 14 May 2020
Sec. Plant Systematics and Evolution
Volume 11 - 2020 | https://doi.org/10.3389/fpls.2020.00572
This article is part of the Research Topic Origins and Domestication of the Grape View all 8 articles
Grapevine (Vitis vinifera) is one of the most important fruit species in the Classical Mediterranean world. It is thought to have been domesticated 6,000–8,000 years ago in the Near East. However, the domestication of its wild relative into wine grapes or table grapes remains largely unknown. In this study, we analyzed 30 table grapes, 30 wine grapes, 30 dual-purpose grape accessions, as well as 30 wild relatives (Vitis vinifera ssp. sylvestris). The phenotypic comparison showed striking differences in berry weight, acidity and the content of aroma. Based on a total of 7,522,958 single-nucleotide polymorphisms, we identified several significant selective sweep regions for table and wine grapes. Besides the well-known sex-determination locus on chromosome 2, the other four highest signals shared by table and wine grapes could not be linked to the known QTLs. The identification of these genomic regions under selection sweep may reveal agronomically important traits that have been selected during grape domestication. This information not only sheds light on the mechanisms of adaptions and diversification, but also guide the genetic improvement in breeding programs.
Approximately 13,000 years ago, human began to domesticate and selectively breed crops (Smith, 2001; Diamond, 2002). During this process, wild species were genetically selected on certain traits that are advantageous for human as sources of food or materials. The most remarkable evolutionary transitions are enlarged seed and fruit sizes (Frary et al., 2000), seed dispersal, loss of dormancy, and flowering and ripening time (Cockram et al., 2007). Meantime, the notably diversification in plant architecture (Clark et al., 2004), adaptation and quality can also be observed. In addition to phenotypes, plant domestication also leaves genetic signatures on both population structure and genetic diversity of the domesticated species (Doebley et al., 2006; Abbo et al., 2014). Understanding how plants are affected during the domestication process, not only can shed light on the mechanisms of adaptions and diversification (Diamond, 2002) but also will guide the genetic improvement in breeding programs.
As one of the oldest domesticated crop plants, grapevine (Vitis vinifera L.) is now economically the most important cultivated fruit crop in the world (Alston and Sambucci, 2019). Since domesticated from its wild progenitor, Vitis vinifera ssp. sylvestris, grape was already a major fruit crop in the Mediterranean area. Moreover, the archeological record suggests that grape has been a source of food and wine 6000–8000 years ago in the Near East (McGovern et al., 2017; McGovern, 2019). Till now, several significant morphological shifts as a result of domestication have been reported, such as larger berry and bunch sizes (Cabezas et al., 2006), higher sugar content, increased variation in berry color (This et al., 2007) and a shift from dioecy to a hermaphroditic mating system (Carmona et al., 2007). Genes contributing to the morphological shifts during domestication and improvement of grapevines have been identified in several resequencing and genetic studies (Costantini et al., 2008; Fechter et al., 2012; Picq et al., 2014; Chen et al., 2015; Correa et al., 2016; Liang et al., 2019).
Generally, commercially cultivated grapes can be classified as either table or wine grapes based on their intended method of consumption: table grapes or wine grapes. Though almost all of them belong to the same species (V. vinifera), significant differences between table and wine grapes were brought about through selective breeding. Table grapes tend to have large, seedless fruit with relatively thin skin, while wine grapes are smaller, usually seeded and have relatively thick skins. At the time of harvest wine grapes tend to be very sweet (approximately 24% sugar by weight), while table grapes have usually around 15% sugar by weight. In addition, wine grapes are often small with concentrated flavors, whereas table grapes are large, bursting with a lot more water.
Genetic diversity among V. vinifera varieties has been studied. Simple sequence repeats were used by Bowers et al. (1999) and more recently, a chip containing 9,000 SNPs was applied on 950 vinifera and 59 sylvestris accessions by Myles et al. (2011). Their results suggested that grape domestication led to a mild reduction of genetic diversity, indicating that grape is a reasonable perennial model for studying the accumulation of deleterious variation in the absence of a pronounced bottleneck. In addition, whole-genome sequencing (WGS) was used in more recent studies to assess structural variation among grape varieties (Cardone et al., 2016; Xu et al., 2016). Di Genova et al. (2014) identified a large number of structural variants and SNPs by comparing genome of “Sultanina” with the reference genome of a nearly homozygous genotype of cultivar “Pinot noir” (PN40024), which are table and wine cultivar, respectively. Moreover, Migicovsky et al. (2017) combined phenotypic data and genome-wide polymorphism data of 580 table and wine grape accessions, and identified some large effect loci controlling phenotypic traits that have been targeted during domestication and breeding, such as hermaphroditism, lighter skin color and muscat aroma.
However, there has been little research performed to identify the positive selection signals between table and wine grapes during selective breeding. To this end, we randomly selected 30 wild accessions (V. vinifera ssp. sylvestris) and 90 domesticated grape accessions (V. vinifera) from a previous resequencing study (Liang et al., 2019). The domesticated grapes include 30 each in three sub-groups: wine, table and dual-purpose grapes. Using the available SNPs, we present phenotypic and genetic difference as well as selection signals during the domestication of wine and table grapes.
A set of 90 V. vinifera accessions including 30 wine grapes, 30 table grapes and 30 dual-purpose grapes which can be used for both fresh consuming and wine production, as well as 30 wild sylvestris accessions were randomly selected from the samples of a previous work (Liang et al., 2019), in which we re-sequenced 472 Vitis accessions on an Illumina HiSeq 4000 sequencer at Novogene-Beijing. The raw sequencing data of these 120 accessions were obtained from NCBI database1 under BioProject PRJNA393611, totaling 728 Gb. Detailed information of these samples, including SRA ID, cultivar name, country of origin, ploidy level, breeding parents, etc. were listed in Supplementary Table 1. Utilization of all accessions were simultaneously checked in VIVC database2 and by experienced researchers.
Liang et al. (2019) reported in their study a total of 24 traits for the cultivated accessions at the ripening stage. We obtained the corresponding phenotypic data for these 90 selected accessions from this study. Three phenotypes (the amount of benzaldehyde, phenylethyl alcohol and 6-methyl-5-hepten-2-one) were discarded due to high proportion of missing value. In the end, a total of 21 traits were reserved in this study (Supplementary Table 2).
Cleaned paired-end resequencing reads were mapped to the Vitis vinifera cv. PN40024 reference genome (Ensembl Plants Release-31, Jaillon et al., 2007) using BWA software (Version: 0.7.10-r789; Li and Durbin, 2009) with the default parameters. To convert mapping results into the BAM format and filter the unmapped and non-unique reads, SAMtools software (Version: 1.3.1; Li et al., 2009) was used. Duplicated reads were filtered with the Picard software (Version: 2.1.1; picard.sourceforge.net). After BWA alignment, the reads around INDELs were realigned by Genome Analysis Toolkit software (GATK, version 3.3-0-g37228af; McKenna et al., 2010) in two steps. In the first step, the RealignerTargetCreator function was used to identify regions where realignment was needed, then the IndelRealigner function was used to realign the regions to produce a realigned BAM file for each accession. Following the best practice workflow recommended by GATK, the variation detection was conducted. In brief, the variants were called for each accession using the GATK HaplotypeCaller function (Emanuelli et al., 2013). A joint genotyping step for comprehensive variations union was performed on the gVCF files. In the filtering step, the SNP filter parameter was set as “QD < 5.0 | | MQ < 540.0 | | FS > 60.0 | | SOR > 3.0 | | MQRankSum < −10.0 | | ReadPosRankSum < −8.0 | | QUAL < 30”. SNPs with MAF < 0.05 were further removed for phylogenetic tree structure, LD decay, PCA and population structure analysis. Making use of the ANNOVAR software (Version: 2015-12-14; Wang et al., 2010), SNPs annotation was performed according to the grapevine reference genome. The coverage of each accession against each chromosome was counted according to the aligned BAM files using SAMtools. SNP density and total genetic diversity across each chromosome were counted within a 100 kb sliding window by VCFtools software (v0.1.13; Danecek et al., 2011).
The whole-genome SNPs were used to construct the Maximum likelihood (ML) phylogenetic tree with 100 bootstrap using SNPhylo software (Version: 20140701; Clark et al., 2007). The iTOL3 (Letunic and Bork, 2019) tool was applied to color the phylogenetic tree. SNPs in linkage disequilibrium (LD) was filtered by PLINK software (Version v1.90b3.38; Purcell et al., 2007) with a window size of 50 SNPs (advancing 5 SNPs at a time) and an r2 threshold set to 0.5. Principal component analysis (PCA) was performed with the Genome-wide Complex Trait Analysis software (GCTA, version: 1.25.3; Yang et al., 2009, 2011), and the first three eigenvectors were plotted. To analyze the population structure, the ADMIXTURE program (Version: 1.3; Alexander et al., 2009) with a block-relaxation algorithm was applied. The convergence of individuals was explored by predefining the number of genetic clusters K from 2 to 4 and running the cross-validation error (CV) procedure. To calculate the linkage disequilibrium (LD), the PopLDdecay (Version: v3.314) software was employed and the pairwise r2 values within and between different chromosomes were calculated. For each sub-group, the LD was calculated using the corresponding SNP pairs.
To detect selective sweeps, SweeD software (Version 3.3.1; Sheehan et al., 2013) was utilized based on the composite likelihood ratio (CLR) test to detect signatures of domestication in the table and wine accessions, respectively. To investigate the selection signals across the whole genome, we also calculated the population divergence statistic (FST) and population nucleotide diversity (π, pairwise nucleotide variation as a measure of variability). A 100 kb sliding window with 10 kb step was applied to quantify FST and π by using VCFtools software (v0.1.13; Danecek et al., 2011). Sliding windows with both of the top 5% values were picked as candidate selective signals.
In our study, a total of 120 grape accessions, including 90 domesticated grape accessions (30 each in wine, table and dual-purpose grapes) and 30 of its wild relative (V. vinifera ssp. sylvestris), were randomly selected. The geographic distributions of these accessions were from total of 23 countries, including China, France, Japan, the United States, Italy, etc. (Supplementary Table 1). When comparing the country of origin between each sub-group, a correlation between geography and utilization can be found. In our datasets, there were 28 table grape accessions with geographic data, of which 20 (71.4%) were Eastern table grapes but only 8 were Western (28.6%). In comparison, 84.6% (22) of the wine table accessions were Western and only 15.4% (4) were Eastern wine grapes. While for dual purpose accessions, less difference was observed between the proportion of Eastern (42.9%) and Western (57.1%). As an attractive trait for consumers, skin color showed different proportion in the three sub-groups. Among 30 table grape accessions, the majority were in white (11) and red (16) while only 1 and 2 were in pink and black, respectively. Dual-purpose grapes also had four colors, but more than half (17) were in white and 2, 3, and 8 were in pink, red and black, respectively. As for the wine grapes, there was no pink or red accession but 1 gray-skinned accession, and white and black skin-colored accessions almost had the equal number (12 and 13, respectively). Additionally, almost all of the cultivated accessions had hermaphrodite flowers except TA-334, which was a female accession.
Moreover, phenotypic data of 21 traits on 90 cultivated grape accessions (V. vinifera) were collected (Supplementary Table 2 and Supplementary Figure 1). All of these cultivated accessions were planted in the Vitis germplasm repository at the Institute of Botany of Chinese Academy of Sciences in Beijing and harvested at the ripening stage, which was determined by the browning seeds and stable sugar content. Berry weight can be an index referring to the berry size. Though there was a small berry accession (TA-261, whose average berry weight was 1.64 g), table grapes had an average berry weight (5.80 g) significantly larger than that of wine grapes (2.27 g). The berry weight of dual-purpose grapes was in between. Additionally, wine grapes tended to have rounder berry shape as the ratio of length/width was closer to 1. The table grape accession TA-268 had the biggest number of 1.698. Among 3 sub-groups, the average seed number seemed similar, but table and dual-purpose grapes did have seedless accessions. In addition, significant differences were also observed in the acid levels among table grapes (3.48 ± 0.72 mg/ml for tartaric acid, 1.49 ± 0.68 mg/ml for malic acid and 4.97 ± 1.10 mg/ml for total acid), dual-purpose grapes (4.32 ± 1.13 mg/ml for tartaric acid, 1.87 ± 0.97 mg/ml for malic acid and 6.19 ± 1.54 mg/ml for total acid) and wine grapes (4.74 ± 0.70 mg/ml for tartaric acid, 2.29 ± 0.89 mg/ml for malic acid and 7.03 ± 0.85 mg/ml for total acid), showing a higher acidity of wine grape. However, among three sub-groups the less significant difference in sugar level was unexpectedly observed. The similar range of sugar level was surprisingly detected between 0 and 8.42 mg/ml for sucrose and 47.36 and 211.83 mg/ml for total sugar. When comparing the brix concentration detected during 2015 and 2017, wine grapes were found to have higher variance. For instance, TA-291, its brix content was 23.41° in 2015 and 20.10° in 2017, but in 2016 this value was only 13.48°. TA-236 was another example, it had 10.85° brix in 2016 but 18.60° in 2017. Moreover, extreme low cases of brix were also found in wine grapes, such TA-242 (7.80° in 2015 and 7.50° in 2017) and TA-183 (9.99° in 2017). For aroma, uptrends could also be observed in the content of hexanal, 2-hexenal, nonanal and (E)-2-hexen-1-ol, indicating their essential role in high quality winemaking.
When considering the breeding history that referred to the parent-offspring relationship in our accessions, we found that at least one parent had the same usage as the offspring, i.e. breeding parents (at least one) should be the same grape type as the offspring or be dual-purpose. Additionally, several accessions were siblings sharing the same breeding parents, and particularly noteworthy was that even the same parents could breed offspring of different types (usage). For instance, Cabernet Suntory and Suntory Blanc are both bred from the cross between Koshu Sanjaku and Cabernet Sauvignon, but they were dual-purpose and wine grape, respectively. The same situation was found in Aishenmeigui (dual-purpose grape) and Zaomanao (table grape), whose parents are dual-purpose grapes Muscat Hamburg and Jingzaojing. Together, these observations could further evidence that grape is a highly heterozygous species with complex genetic background and quantitative agronomic traits.
The sequencing data of 120 grape accessions (4.85 Gb reads) have an average coverage depth of 16.2X for each accession (Supplementary Table 3). After mapping against the V. vinifera reference genome (Jaillon et al., 2007), the average mapping rate was 98.17% with the genome coverage above 80% across all chromosomes for the majority of accessions (Supplementary Table 3). After a basic filtering criterion (minor allele frequency >0.05 and missing rate <40%, see section “Materials and Methods”), a total of 7,522,958 single-nucleotide polymorphisms (SNPs) were identified on 19 chromosomes (Supplementary Figure 2). Among 19 chromosomes, the largest number of SNPs (543,458) were identified on chromosome 14 with a moderate SNP density of 18 SNPs/kb while both of the lowest SNPs number (305,687) and also the lowest SNP density (∼16 SNPs/kb) were identified on chromosome 2. Additionally, chromosome 15 and 12 were two chromosomes with the densest SNP distribution with the density of approximately 19 SNPs/kb.
In accordance with expectations, PCA showed substantial genetic diversity among major grapevine categories. 11.7, 4.9, and 3.9% of total genetic variance were explained by the first three principal components, respectively (Figures 1A,B). PC3 separated wild Eurasian accessions (WEU, V. vinifera subsp. sylvestris) from the cultivated grape accessions (table, wine and dual-purpose grapes, Figure 1B), supporting the fact that the latter three sub-groups shared more similarity in the genetic background than wild Eurasian accessions. This finding was further supported by the result of model-based analyses of population admixture (Figure 1C) and phylogeny analysis (Figure 2). PC1 evidently separated WEU, table grapes, wine grapes and dual-purpose grapes whereas PC2 set two WEU and one dual-purpose accessions apart from other accessions (Figure 1A). The differentiation between these grapevine categories was also consistent with the population admixture graph (Figure 1C, K = 4). In the admixture plot at K = 4 (Figure 1C), it was apparent that comparing to the table grapes, the majority of wine grapes received more genetic contributions from WEU, whereas table and dual-purpose grapes shared more similar genetic background and this result was in line with the ML phylogenetic tree (Figure 2). Even though most of domesticated grapevine accessions were closely clustered in the PCA graphs, they showed clear pattern of high genetic heterogeneity as evidenced by the population admixture analyses (Figure 1C, K = 4).
Figure 1. Population structure analyses of all accessions. (A,B) PCA analysis of all accessions in this study. (C) Population admixture of all Vitis accessions. Each color represents one ancestral population. Each accession is represented by a vertical bar, and the length of each colored segment in each vertical bar represents the proportion contributed by ancestral populations. WNA, Wine, and Table represent wild European grapevine, wine grape accessions, and table grape accessions, respectively.
Figure 2. Maximum Likelihood phylogenetic tree of all accessions inferred from whole-genome SNPs. Red, blue, green, and orange represent wild European grapevines (WEU), wine grapes, table grapes and dual-purpose grapevines which can be used for both fresh consuming and wine production, respectively. Bootstrap values are indicated by blue circles.
Linkage disequilibrium (LD) was evaluated in wild European and each domesticated sub-group using large-scale genetic markers (Figure 3). For wild European grapevines the LD decay reached half of the maximum average r2 at a distance of 3 kb while all three sub-groups of domesticated grapevines had similar LD around 350 bp, being concordant to the previous study (Marrano et al., 2018; Liang et al., 2019). Comparing with the domesticated grapevine accessions, the wild European species had relatively slower decay of LD, but it is important to realize that this difference may narrow with a more diverse wild European Vitis population. The LD correlates to the number of recombination events along generations, thus lower or higher LD within subgroups may allow to testify different group histories (Laucou et al., 2018). However, the calculation of LD is sensitive to the population size and the detected regions (Nicolas et al., 2016). In this study, the LD had not significant difference between three sub-groups of cultivated grapes. Thus, any conclusion of group history or possible causal relation of LD could not be taken.
The potential selective signals in the table and wine grapevines were investigated by identifying the regions (∼1 kb in length) that both scored in the top 5% of the Fst and CLR analysis (Figure 4, Supplementary Figures 3, 4 and Supplementary Tables 4, 5). 2,119 selective sweep regions were both detected in table and wine grapes, which harbored 450 and 491 candidate genes, respectively, and 109 overlapped genes (Supplementary Tables 4–6). For table grape accessions, the highest signal 1237.24 was found on chromosome 16 at position 6,961,106 bp, and the most selective signals were detected on chromosome 2 (358 signals) while chromosome 4 had the least signal number of 19. For wine grapes, 300 selective signals were identified on chromosome 13 but only 22 on chromosome 15, with the highest signal reached to 1317.4 on chromosome 10 at 12,113,058 bp. In addition to the 2 highest signals on chromosome 10 and 16, both table and wine grapevines shared some other high signal at the same position, including chromosome 1 (15,167,366 bp), chromosome 13 (13,996,633) and chromosome 2 (4,918,924 bp). It was worth mentioning that the signal on chromosome 2 at 4.92 Mb was within the fine-mapped 143 kb regions (4.91–5.05 Mb) believed to harbor the causal flower sex locus (Fechter et al., 2012; Picq et al., 2014). Meanwhile, some special signal for different grape accessions indicated they had undergone a different selection pressure, such as table grape unique signals on chromosome 18 at 18,447,039 bp and 21,205,860 bp and wine grape unique signal on chromosome 11 at 17,260,626 bp. Annotation of genes in selective sweep regions identified two (VIT_12s0034g00310 and VIT_18s0075g00100) and three (VIT_04s0043g00970, VIT_09s0002g06180, VIT_19s0015g01260) disease resistance related genes specific for table and wine, respectively, indicating their undergoing of the different breeding process for disease resistance. Additionally, genes VIT_16s0013g01070 and VIT_16s0013g01080 on the flanking sides of the highest signal on chromosome 16 were annotated as ethylene-responsive transcription factor ERF105 and ERF104, respectively. In Arabidopsis, ERF104 and ERF105 were found to play important role in signaling response, plant immunity and plant response to abiotic stresses (Mase et al., 2013; Meng et al., 2013; Vogel et al., 2014; Müller and Munné-Bosch, 2015; Cao et al., 2019). By inference, this positive selected region may relate to the stress tolerance of grapevine. Unfortunately, there were more candidate genes at or close those high signal positions had unclear molecular function, thus the proper trait selected during the domestication could not be deduced. GO enrichment of the 341 table grape unique and 382 wine grape unique domesticated candidate genes showed significant difference in functional representation in the GO categories (Figure 5), whereas table grapes had most of genes annotated to biological process and wine grapes had most related to catalytic activity, which plays a vital role during the synthesis of aroma components and winemaking.
Figure 4. Global View of Candidate Domesticated Regions in the table and wine grapes. Regions with both XP-CLR values and p ratios in the top 5% were regarded as having domestication signals. The admixture patterns of all accessions are presented in Figure 1C. The sources of all the samples are provided in Supplementary Table 1.
Figure 5. GO enrichment of the candidate selective sweep genes from the CLR analysis. (A) Venn plot of the GO terms shared by table and wine grapes. Bubble plots of enriched GO terms for (B) the table grapes (n = 30) and (C) wine grapes (n = 30). The size of the bubble represents the number of genes in the corresponding GO category. The color of the bubble shows the corresponding P-value. Rich factor shows the percentage of enriched genes out of the total number in the GO category.
In this research work, we collected 30 accessions each in table grapes, wine grapes, dual-purpose grapes and wild relative (V. vinifera ssp. sylvestris). Given that most table grape accessions were from the East and most wine accessions were from the West, we found a correlation between usage and geography, which is likely to be influenced by religions (Migicovsky et al., 2017). In East, the consumption of alcohol has been prohibited among Muslim countries for over a millennium, grape breeding has thus more focused on the development of table grapes with attractive traits like large berry size and colorful skin (This et al., 2006). Conversely, as a dominant religion in Western country, Christianity does not prohibit alcohol and grapes have been selected to produce the high-quality wine.
Differ from wine grapes which are pressed and fermented prior to consumption, table grapes are consumed directly. Therefore, their desirability depends largely on a visual assessment by consumers. As a result, most table grapes have large and colorful berries. Our results also confirmed that table grapes, even the dual-purpose grape accessions, had larger and colorful berries than wine grapes, indicating that these characteristics may have been targeted by table grape breeders. Smaller berries, by contrast, often have more better properties for vinification hence may be more desirable and suitable for winemaking (Gil et al., 2015). In this study, there had three table grape accessions and four dual-purpose accessions produce no seed, indicating that seedlessness becomes another preferable trait for fresh fruit consumption and probably for raisin production. Acidity also comes in to play when comparing these two grapes that higher acid levels were detected in wine grapes comparing with table grape, which was consistent with the previous work (Migicovsky et al., 2017). Sweetness is another characteristic where these two grape types should differ drastically. Wine grapes are commonly harvested at around 22–30 percent sugar whereas table grapes at around 15–20% sugar. This high sugar content is preferred because sugar is converted by yeast into alcohol by the process of fermentation. The greater concentration of sugars in grape berries there is, the greater potential alcohol level will be produced. For this reason, sugar content is a much more important factor for wine grapes. Some winemakers and viticulturists will continually sample grapes for a higher potential alcohol level and even delay harvesting time until grapes have a sufficiently high sugar concentration (Bird, 2011; Robinson and Harding, 2015). However, in our study, sugar content of grapevine, especially of wine grapes, showed a less reliable results. For instance, Cabernet Franc (i.e. TA-183), is usually harvested above 20° Brix (Smart et al., 1990; Gaudillère et al., 2002), but had only 9.99° Brix in 2017. This huge difference was possibly due to the unusual high temperatures in Beijing. In long terms, the effects of temperature on grapevine have been recognized. It influences plant physiology, berry composition and ultimately wine characteristics (Jones et al., 2005; Bonada and Sadras, 2015). Although the basic climatic conditions for grape growing are easily satisfied, high temperatures may make it difficult to consistently fulfill criteria required for grape quality without adjusting variety or changing management practices (Martínez-Lüscher et al., 2016). Consequently, the content of sugars and probably other phenolic compounds show very different accumulation patterns throughout grape development.
The genetic structure of grapevine, which can be largely understood as one large complex pedigree, is the result of a limited number of crosses among elite cultivars (Myles et al., 2011; Bacilieri et al., 2013). In this study, we randomly selected 90 cultivated grape accessions (30 each for different usages) for the identification of selective sweep, but common breeding parents were found between several accessions, some of which were even in the different sub-groups. This relatedness of genetic background may reduce the identification scope, but on the other hand had more precise detection of the selected signals. Due to the limited number (30) of each genetic pool, we are unable to provide any conclusion of group history with the current dataset, but still, the rapid LD decay in cultivated grapevine, is far more quickly than that in rice (Mather et al., 2007; Huang et al., 2012), soybean (Zhou et al., 2015), and Arabidopsis (Kim et al., 2007).
Positive selection acts on beneficial alleles, increase their frequency in the population and leaves signature over time in the genome. The analysis of large genomic data allows the detection of the molecular patterns of advantageous mutations that have been selected and fixed during domestication and breeding. In this study, an SFS-based method (SweeD) with the composite likelihood ratio (CLR) test was applied in table and wine grape accessions, and each had 2119 positively selected signals, covering 450 candidate genes and 491 candidate genes, respectively. Among them, a signal on chromosome 2 at the position of 4.91 Mb was shared by both groups, where the sex-determining locus had been fine-mapped (Fechter et al., 2012; Picq et al., 2014). As one of the oldest cultivated fruits, the most discriminating characteristic between the cultivated V. vinifera ssp. vinifera and the wild-form V. vinifera ssp. sylvestris is their sexual system. Flowers of cultivated grapes are mainly hermaphroditic, whereas all wild Vitis species, including the ancestor of V. vinifera, are dioecious. This key transition enables self-pollination and subsequent clonal propagation without the need for pollinators (Palumbo et al., 2019).
Due to the advancement of high-throughput sequencing technology (Alaimo et al., 2017), a huge number of QTLs underlying important phenotypic traits have been explored, such as the MADs-Box gene VviAGL11 on chromosome 18 which codes seedlessness (Mejia et al., 2011; Royo et al., 2018), VvMybA1 on chromosome 2 that affects grape skin color, and several other traits like berry firmness (Carreño et al., 2014; Correa et al., 2016) and resistance (van Heerden et al., 2014; Pap et al., 2016; Zyprian et al., 2016; Zendler et al., 2017; Hou et al., 2018). However, the highest 4 positive selection signals shared by both table and wine grape, which were chromosome 1: 15,167,366 bp, chromosome 10: 12,113,058 bp, chromosome 13: 13,996,633 bp and chromosome 16, 6,961,106 bp, were not linked to those QTLs. Nevertheless, two flanking genes at 6,961,106 bp on chromosome 16 were annotated as ERF104 and ERF105, respectively, which were involved in the immunity and stress response in Arabidopsis. Thus, we may deduce that the candidate genes at or close at these positions could be very important genes related to the traits selected during the domestication process, such as stress response.
During domestication, the selection is often aimed at some specific phenotype, where the internal mechanism is to select genes that have a direct relationship with the preferable traits. In this study, we have identified several genomic regions under positive selection that may have been artificially selected during the process of grapevine domestication. The detection of these significant selection regions can lead to the candidate genes that perform the corresponding functions and therefore would have great significance to understand the evolution of grapevine (Adam-Blondon et al., 2005; Lamoureux et al., 2006). Economically speaking, the improvement of wine quality and environmental stress resistance during domestication by genetically artificial screening has been making great benefits to both of the wine industry and fresh fruit market.
The WGRS data set generated and analyzed in the current study is available from NCBI under the BioProject accession PRJNA393611.
LK collected the samples and performed the experiments. SD and SW completed the data analysis. XD and MT edited and modified the manuscript. All authors read and approved the manuscript.
This work was supported by the grants from the Jiangsu University (19JDG039) and Yunnan provincial key programs of the Yunnan Eco-friendly Food International Cooperation Research Center project under grant 2019ZG00908.
SW, SD, and XD are employees of the Nowbio Biotechnology Company and they declare that Nowbio Biotechnology Company plays no role in the funding, design, analysis, and publication of this manuscript.
The remaining authors declare that the research was conducted in the absence of any commercial or financial relationships that could be construed as a potential conflict of interest.
We thank all the individuals who have helped us in this study.
The Supplementary Material for this article can be found online at: https://www.frontiersin.org/articles/10.3389/fpls.2020.00572/full#supplementary-material
PCA, principle component analysis; QTL, quantitative trait locus.
Abbo, S., Pin Pinhasi, R., Gopher, A., Saranga, Y., Ofner, I., and Peleg, Z. (2014). Plant domestication versus crop evolution: a conceptual framework for cereals and grain legumes. Trends Plant Sci. 19, 351–360. doi: 10.1016/j.tplants.2013.12.002
Adam-Blondon, A. F., Bernole, A., Faes, G., Lamoureux, D., Pateyron, S., Grando, M. S., et al. (2005). Construction and characterization of BAC libraries from major grapevine cultivars. Theor. Appl. Genet. 110, 1363–1371. doi: 10.1007/s00122-005-1924-9
Alaimo, S., Marceca, G. P., Giugno, R., Ferro, A., and Pulvirenti, A. (2017). Current knowledge and computational techniques for grapevine meta-omics analysis. Front. Plant Sci. 8:2241. doi: 10.3389/fpls.2017.02241
Alexander, D. H., Novembre, J., and Lange, K. (2009). Fast model-based estimation of ancestry in unrelated individuals. Genome Res. 19, 1655–1664. doi: 10.1101/gr.094052.109
Alston, J. M., and Sambucci, O. (2019). “Grapes in the world economy,” in The Grape Genome, eds D. Cantu and W. Walker (Berlin: Springer), 1–24. doi: 10.1007/978-3-030-18601-2_1
Bacilieri, R., Lacombe, T., Le Cunff, L., Di Vecchi-Staraz, M., Laucou, V., Genna, B., et al. (2013). Genetic structure in cultivated grapevines is linked to geography and human selection. BMC Plant Biol. 13:25. doi: 10.1186/1471-2229-13-25
Bird, D. (2011). Understanding Wine Technology: The Science of Wine Explained. Board and Bench Publishing.
Bonada, M., and Sadras, V. O. (2015). Review: critical appraisal of methods to investigate the effect of temperature on grapevine berry composition. Aust. J. Grape Wine Res. 21, 1–17. doi: 10.1111/ajgw.12102
Bowers, J., Boursiquot, J.-M., This, P., Chu, K., Johansson, H., and Meredith, C. (1999). Historical genetics-the parentage of chardonnay, gamay, and other wine grapes of northeastern france. Science 285, 1562–1565. doi: 10.1126/science.285.5433.1562
Cabezas, J. A., Cervera, M. T., Ruiz-García, L., Carreno, J., and Martínez-Zapater, J. M. (2006). A genetic analysis of seed and berry weight in grapevine. Genome 49, 1572–1585. doi: 10.1139/g06-122
Cao, F. Y., Khan, M., Taniguchi, M., Mirmiran, A., Moeder, W., Lumba, S., et al. (2019). A host–pathogen interactome uncovers phytopathogenic strategies to manipulate plant ABA responses. Plant J. 100, 187–198. doi: 10.1111/tpj.14425
Cardone, M. F., D’Addabbo, P., Alkan, C., Bergamini, C., Catacchio, C. R., Anaclerio, F., et al. (2016). Inter-varietal structural variation in grapevine genomes. Plant J. 88, 648–661. doi: 10.1111/tpj.13274
Carmona, M. J., Cubas, P., Calonje, M., and Martinez-Zapater, J. M. (2007). Flowering transition in grapevine (Vitis vinifera L.). Botany 85, 701–711. doi: 10.1139/B07-059
Carreño, I., Cabezas, J. A., Martínez-Mora, C., Arroyo-García, R., Cenis, J. L., Martínez-Zapater, J. M., et al. (2014). Quantitative genetic analysis of berry firmness in table grape (Vitis vinifera L.). Tree Genet. Genomes 11:818. doi: 10.1007/s11295-014-0818-x
Chen, J., Wang, N., Fang, L. C., Liang, Z. C., Li, S. H., and Wu, B. H. (2015). Construction of a high-density genetic map and QTLs mapping for sugars and acids in grape berries. BMC Plant Biol. 15:28. doi: 10.1186/s12870-015-0428-2
Clark, R. M., Linton, E., Messing, J., and Doebley, J. F. (2004). Pattern of diversity in the genomic region near the maize domestication gene tb1. Proc. Natl. Acad. Sci. U.S.A. 101, 700–707. doi: 10.1073/pnas.2237049100
Clark, R. M., Schweikert, G., Toomajian, C., Ossowski, S., Zeller, G., Shinn, P., et al. (2007). Common sequence polymorphisms shaping genetic diversity in Arabidopsis thaliana. Science 317, 338–342. doi: 10.1126/science.1138632
Cockram, J., Jones, H., Leigh, F. J., O’Sullivan, D., Powell, W., Laurie, D. A., et al. (2007). Control of flowering time in temperate cereals: genes, domestication, and sustainable productivity. J. Exp. Bot. 58, 1231–1244. doi: 10.1093/jxb/erm042
Correa, J., Mamani, M., Munoz-Espinoza, C., Gonzalez-Aguero, M., Defilippi, B. G., Campos-Vargas, R., et al. (2016). New Stable QTLs for Berry Firmness in Table Grapes. Am. J. Enol. Viticult. 67, 212–217. doi: 10.5344/ajev.2015.15049
Costantini, L., Battilana, J., Lamaj, F., Fanizza, G., and Grando, M. S. (2008). Berry and phenology-related traits in grapevine (Vitis vinifera L.): from quantitative trait loci to underlying genes. BMC Plant Biol. 8:38. doi: 10.1186/1471-2229-8-38
Danecek, P., Auton, A., Abecasis, G., Albers, C. A., Banks, E., DePristo, M. A., et al. (2011). The variant call format and VCFtools. Bioinformatics 27, 2156–2158. doi: 10.1093/bioinformatics/btr330
Di Genova, A., Almeida, A. M., Munñoz-Espinoza, C., Vizoso, P., Travisany, D., Moraga, C., et al. (2014). Whole genome comparison between table and wine grapes reveals a comprehensive catalog of structural variants. BMC Plant Biol. 14:7. doi: 10.1186/1471-2229-14-7
Diamond, J. (2002). Evolution, consequences and future of plant and animal domestication. Nature 418, 700–707. doi: 10.1038/nature01019
Doebley, J. F., Gaut, B. S., and Smith, B. D. (2006). The molecular genetics of crop domestication. Cell 127, 1309–1321. doi: 10.1016/j.cell.2006.12.006
Emanuelli, F., Lorenzi, S., Grzeskowiak, L., Catalano, V., Stefanini, M., Troggio, M., et al. (2013). Genetic diversity and population structure assessed by SSR and SNP markers in a large germplasm collection of grape. BMC Plant Biol. 13:39. doi: 10.1186/1471-2229-13-39
Fechter, I., Hausmann, L., Daum, M., Sorensen, T. R., Viehover, P., Weisshaar, B., et al. (2012). Candidate genes within a 143 kb region of the flower sex locus in Vitis. Mol. Genet. Genomics 287, 247–259. doi: 10.1007/s00438-012-0674-z
Frary, A., Nesbitt, T. C., Grandillo, S., Knaap, E., Cong, B., Liu, J., et al. (2000). fw2.2: a quantitative trait locus key to the evolution of tomato fruit size. Science 289, 85–88. doi: 10.1126/science.289.5476.85
Gaudillère, J. P., Van Leeuwen, C., and Ollat, N. (2002). Carbon isotope composition of sugars in grapevine, an integrated indicator of vineyard water status. J. Exp. Bot. 53, 757–763. doi: 10.1093/jexbot/53.369.757
Gil, M., Pascual, O., Gómez-Alonso, S., García-Romero, E., Hermosín-Gutiérrez, I., Zamora, F., et al. (2015). Influence of berry size on red wine colour and composition. Austr. J. Grape Wine Res. 21, 200–212. doi: 10.1111/ajgw.12123
Hou, L., Zhang, G., Zhao, F., Zhu, D., Fan, X., Zhang, Z., et al. (2018). VvBAP1 is involved in cold tolerance in Vitis vinifera L. Front. Plant Sci. 9:726. doi: 10.3389/fpls.2018.00726
Huang, X., Kurata, N., Wang, Z. X., Wang, A., Zhao, Q., Zhao, Y., et al. (2012). A map of rice genome variation reveals the origin of cultivated rice. Nature 490, 497–501. doi: 10.1038/nature11532
Jaillon, O., Aury, J. M., Noel, B., Policriti, A., Clepet, C., Casagrande, A., et al. (2007). The grapevine genome sequence suggests ancestral hexaploidization in major angiosperm phyla. Nature 449, 463–467. doi: 10.1038/nature06148
Jones, G. V., White, M. A., Cooper, O. R., and Storchmann, K. (2005). Climate change and global wine quality. Clim. Change 73, 319–343. doi: 10.1007/s10584-005-4704-2
Kim, S., et al. (2007). Recombination and linkage disequilibrium in Arabidopsis thaliana. Nat. Genet. 39, 1151–1155. doi: 10.1038/ng2115
Lamoureux, D., Bernole, A., Le Clainche, I., Tual, S., Thareau, V., Paillard, S., et al. (2006). Anchoring of a large set of markers onto a BAC library for the development of a draft physical map of the grapevine genome. Theor. Appl. Genet. 113, 344–356. doi: 10.1007/s00122-006-0301-7
Laucou, V., Launay, A., Bacilieri, R., Lacombe, T., Adam-Blondon, A. F., Berard, A., et al. (2018). Extended diversity analysis of cultivated grapevine Vitis vinifera with 10K genome-wide SNPs. PLoS ONE 13:e0192540. doi: 10.1371/journal.pone.0192540
Letunic, I., and Bork, P. (2019). Interactive Tree Of Life (iTOL) v4: recent updates and new developments. Nucleic Acids Res. 47, W256–W259. doi: 10.1093/nar/gkz239
Li, H., and Durbin, R. (2009). Fast and accurate short read alignment with burrows-wheeler transform. Bioinformatics 25, 1754–1760. doi: 10.1093/bioinformatics/btp324
Li, H., Handsaker, B., Wysoker, A., Fennell, T., Ruan, J., Homer, N., et al. (2009). The sequence alignment/map format and SAMtools. Bioinformatics 25, 2078–2079. doi: 10.1093/bioinformatics/btp352
Liang, Z., Duan, S., Sheng, J., Zhu, S., Ni, X., Shao, J., et al. (2019). Whole-genome resequencing of 472 Vitis accessions for grapevine diversity and demographic history analyses. Nat. Commun. 10:1190. doi: 10.1038/s41467-019-09135-8
Marrano, A., Micheletti, D., Lorenzi, S., Neale, D., and Grando, M. S. (2018). Genomic signatures of different adaptations to environmental stimuli between wild and cultivated Vitis vinifera L. Hortic. Res. 5, 1–12. doi: 10.1038/s41438-018-0041-2
Martínez-Lüscher, J., Kizildeniz, T., Vučetić, V., Dai, Z., Luedeling, E., et al. (2016). Sensitivity of grapevine phenology to water availability, temperature and CO2 concentration. Front. Environ Sci. 4:48. doi: 10.3389/fenvs.2016.00048
Mase, K., Ishihama, N., Mori, H., Takahashi, H., Kaminaka, H., Kodama, M., et al. (2013). Ethylene-responsive AP2/ERF transcription factor MACD1 participates in phytotoxin-triggered programmed cell death. Mol. Plant Microbe Interact. 26, 868–879. doi: 10.1094/MPMI-10-12-0253-R
Mather, K. A., Caicedo, A. L., Polato, N. R., Olsen, K. M., McCouch, S., and Purugganan, M. D. (2007). The extent of linkage disequilibrium in rice (Oryza sativa L.). Genetics 177, 2223–2232. doi: 10.1534/genetics.107.079616
McGovern, P., Jalabadze, M., Batiuk, S., Callahan, M. P., Smith, K. E., Hall, G. R., et al. (2017). Early neolithic wine of Georgia in the South Caucasus. Proc. Natl. Acad. Sci. U.S.A. 114, E10309–E10318. doi: 10.1073/pnas.1714728114
McGovern, P. E. (2019). Ancient Wine: The Search for the Origins of Viniculture. Princeton, NJ: Princeton University Press.
McKenna, A., Hanna, M., Banks, E., Sivachenko, A., Cibulskis, K., Kernytsky, A., et al. (2010). The genome analysis toolkit: a mapreduce framework for analyzing next-generation DNA sequencing data. Genome Res. 20, 1297–1303. doi: 10.1101/gr.107524.110
Mejia, N., Soto, B., Guerrero, M., Casanueva, X., Houel, C., de los Angeles Miccono, M., et al. (2011). Molecular, genetic and transcriptional evidence for a role of VvAGL11 in stenospermocarpic seedlessness in grapevine. BMC Plant Biol. 11:57. doi: 10.1186/1471-2229-11-57
Meng, X., Xu, J., He, Y., Yang, K. Y., Mordorski, B., Liu, Y., et al. (2013). Phosphorylation of an ERF transcription factor by Arabidopsis MPK3/MPK6 regulates plant defense gene induction and fungal resistance. Plant Cell 25, 1126–1142. doi: 10.1105/tpc.112.109074
Migicovsky, Z., Sawler, J., Gardner, K. M., Aradhya, M. K., Prins, B. H., Schwaninger, H. R., et al. (2017). Patterns of genomic and phenomic diversity in wine and table grapes. Hortic. Res. 4:17035. doi: 10.1038/hortres.2017.35
Müller, M., and Munné-Bosch, S. (2015). Ethylene response factors: a key regulatory hub in hormone and stress signaling. Plant Physiol. 169, 32–41. doi: 10.1104/pp.15.00677
Myles, S., Boyko, A. R., Owens, C. L., Brown, P. J., Grassi, F., Aradhya, M. K., et al. (2011). Genetic structure and domestication history of the grape. Proc. Natl. Acad. Sci. U.S.A. 108, 3530–3535. doi: 10.1073/pnas.1009363108
Nicolas, S. D., Péros, J. P., Lacombe, T., Launay, A., Le Paslier, M. C., Bérard, A., et al. (2016). Genetic diversity, linkage disequilibrium and power of a large grapevine (Vitis vinifera L) diversity panel newly designed for association studies. BMC Plant Biol. 16:74. doi: 10.1186/s12870-016-0754-z
Palumbo, F., Vannozzi, A., Magon, G., Lucchin, M., and Barcaccia, G. (2019). Genomics of flower identity in grapevine (Vitis vinifera L.). Front. Plant Sci. 10:316. doi: 10.3389/fpls.2019.00316
Pap, D., Riaz, S., Dry, I. B., Jermakow, A., Tenscher, A. C., Cantu, D., et al. (2016). Identification of two novel powdery mildew resistance loci, Ren6 and Ren7, from the wild Chinese grape species Vitis piasezkii. BMC Plant Biol. 16:170. doi: 10.1186/s12870-016-0855-8
Picq, S., Santoni, S., Lacombe, T., Latreille, M., Weber, A., Ardisson, M., et al. (2014). A small XY chromosomal region explains sex determination in wild dioecious V. vinifera and the reversal to hermaphroditism in domesticated grapevines. BMC Plant Biol. 14:229. doi: 10.1186/s12870-014-0229-z
Purcell, S., Neale, B., Todd-Brown, K., Thomas, L., Ferreira, M. A., Bender, D., et al. (2007). PLINK: a tool set for whole-genome association and population-based linkage analyses. Am. J. Hum. Genet. 81, 559–575. doi: 10.1086/519795
Robinson, J., and Harding, J. (2015). The Oxford Companion to Wine. Washington, DC: American Chemical Society.
Royo, C., Torres-Perez, R., Mauri, N., Diestro, N., Cabezas, J. A., Marchal, C., et al. (2018). The major origin of seedless grapes is associated with a missense mutation in the MADS-box gene VviAGL11. Plant Physiol. 177, 1234–1253. doi: 10.1104/pp.18.00259
Sheehan, S., Harris, K., and Song, Y. S. (2013). Estimating variable effective population sizes from multiple genomes: a sequentially markov conditional sampling distribution approach. Genetics 194, 647–662. doi: 10.1534/genetics.112.149096
Smart, R., Dick, J., Gravett, I., and Fisher, B. (1990). Canopy management to improve grape yield and wine quality – principles and practices. S. Afr. J. Enol. Viticult. 11, 1–17. doi: 10.21548/11-1-2232
Smith, B. D. (2001). Documenting plant domestication- The consilience of biological and archaeological approaches. Proc. Natl. Acad. Sci. U.S.A. 98, 1324–1326. doi: 10.1073/pnas.98.4.1324
This, P., Lacombe, T., Cadle-Davidson, M., and Owens, C. L. (2007). Wine grape (Vitis vinifera L.) color associates with allelic variation in the domestication gene VvmybA1. Theor. Appl. Genet. 114, 723–730. doi: 10.1007/s00122-006-0472-2
This, P., Lacombe, T., and Thomas, M. R. (2006). Historical origins and genetic diversity of wine grapes. Trends Genet. 22, 511–519. doi: 10.1016/j.tig.2006.07.008
van Heerden, C. J., Burger, P., Vermeulen, A., and Prins, R. (2014). Detection of downy and powdery mildew resistance QTL in a ‘Regent’ RedGlobe’ population. Euphytica 200, 281–295.
Vogel, M. O., Moore, M., König, K., Pecher, P., Alsharafa, K., Lee, J., et al. (2014). Fast retrograde signaling in response to high light involves metabolite export, MITOGEN-ACTIVATED PROTEIN KINASE6, and AP2/ERF transcription factors in Arabidopsis. Plant Cell 26, 1151–1165. doi: 10.1105/tpc.113.121061
Wang, K., Li, M., and Hakonarson, H. (2010). ANNOVAR: functional annotation of genetic variants from high-throughput sequencing data. Nucleic Acids Res. 38:e164. doi: 10.1093/nar/gkq603
Xu, Y., Gao, Z., Tao, J., Jiang, W., Zhang, S., Wang, Q., et al. (2016). Genome-Wide detection of SNP and SV variations to reveal early ripening-related genes in grape. PLoS ONE 11:e0147749. doi: 10.1371/journal.pone.0147749
Yang, C., Wang, Y., Liang, Z., Fan, P., Wu, B., Yang, L., et al. (2009). Volatiles of grape berries evaluated at the germplasm level by headspace-SPME with GC–MS. Food Chem. 114, 1106–1114. doi: 10.1016/j.foodchem.2008.10.061
Yang, J., Lee, S. H., Goddard, M. E., and Visscher, P. M. (2011). GCTA: a tool for genome-wide complex trait analysis. Am. J. Hum. Genet. 88, 76–82. doi: 10.1016/j.ajhg.2010.11.011
Zendler, D., Schneider, P., Töpfer, R., and Zyprian, E. (2017). Fine mapping of Ren3 reveals two loci mediating hypersensitive response against Erysiphe necator in grapevine. Euphytica 213:68. doi: 10.1007/s10681-017-1857-9
Zhou, Z., Jiang, Y., Wang, Z., Gou, Z., Lyu, J., Li, W., et al. (2015). Resequencing 302 wild and cultivated accessions identifies genes related to domestication and improvement in soybean. Nat. Biotechnol. 33, 408–414. doi: 10.1038/nbt.3096
Keywords: Vitis vinifera L., next-generation sequencing, QTLs, domestication signal, SNPs, selective sweep
Citation: Kui L, Tang M, Duan S, Wang S and Dong X (2020) Identification of Selective Sweeps in the Domesticated Table and Wine Grape (Vitis vinifera L.). Front. Plant Sci. 11:572. doi: 10.3389/fpls.2020.00572
Received: 06 November 2019; Accepted: 17 April 2020;
Published: 14 May 2020.
Edited by:
Rosa Arroyo-Garcia, Instituto Nacional de Investigación y Tecnología Agraria y Alimentaria (INIA), SpainReviewed by:
Patricio Hinrichsen, INIA, ChileCopyright © 2020 Kui, Tang, Duan, Wang and Dong. This is an open-access article distributed under the terms of the Creative Commons Attribution License (CC BY). The use, distribution or reproduction in other forums is permitted, provided the original author(s) and the copyright owner(s) are credited and that the original publication in this journal is cited, in accordance with accepted academic practice. No use, distribution or reproduction is permitted which does not comply with these terms.
*Correspondence: Xiao Dong, anVzdGR4QDEyNi5jb20=
Disclaimer: All claims expressed in this article are solely those of the authors and do not necessarily represent those of their affiliated organizations, or those of the publisher, the editors and the reviewers. Any product that may be evaluated in this article or claim that may be made by its manufacturer is not guaranteed or endorsed by the publisher.
Research integrity at Frontiers
Learn more about the work of our research integrity team to safeguard the quality of each article we publish.