- 1Key Laboratory of Humid Subtropical Eco-geographical Process of Ministry of Education, Fujian Normal University, Fuzhou, China
- 2Long-Term Research Station of Alpine Forest Ecosystems, Key Laboratory of Ecological Forestry Engineering, Institute of Ecology and Forestry, Sichuan Agricultural University, Chengdu, China
- 3Ecological Security and Protection Key Laboratory of Sichuan Province, Mianyang Normal University, Mianyang, China
Plant litter is one of the main sources of soil humus, but which can also promote primary humus degradation by increasing microbial activity due to the higher availability of energy released, resulting in a confusing relationship between litterfall and soil humus. Therefore, an in situ incubation experiment was carried out in three subalpine forests (coniferous, mixed and broadleaved forests) on the eastern Qinghai-Tibetan Plateau. We set up two treatments. One that allowed litterfall to enter the soil normally and the other prevented litterfall to enter the soil. Soils were sampled in October (the end of the growing season), January (the onset of the freezing season), March (the end of the freezing season), and May (the start of the growing season) from May 2017 to May 2018. By assessing the litterfall production, the content of total extracted humus, humic acid (HA) and fulvic acid (FA) in the topsoil (0–20 cm) in each incubation period, we determined the impact of litterfall on the content of humus extracted from the soil during the freezing and the growing season. Over 1-year incubation, soil total extracted humus and HA showed considerable decreases in the treatment of retained litterfall in the mixed forest but not in the coniferous or broadleaved forests. Moreover, litterfall significantly reduced the contents of soil total extracted humus and HA during the growing season in all three forests, while only reduced soil HA content in the broadleaved forest in the freezing season. The relationship between litterfall and soil extracted humic substances was greatly regulated by the seasonal dynamics of litter types and litter production in all forest types. The larger the amount of litterfall was, the more litterfall could promote the reduction of soil extracted humic substances. Compared with a single type of broadleaf or needle litter, mixed litterfall could promote a higher degradation of soil humic substances. However, broadleaf litter might lead to much greater decreases in soil humic substance than needle litter because it is more decomposable. These results indicate that the effect of litterfall on soil humic substances are mainly regulated by litter types and litter production. Moreover, the effects of litterfall on soil humic substances are more significant during the growing season than winter. This suggests that the longer growing season and a shorter winter caused by ongoing global warming may alter the relationships between litterfall and extracted humic substances, further disrupting the carbon balance of forest ecosystems in the subalpine forests.
Introduction
Humus is the main component of soil organic matter (Kogel-Knabner, 2000) and is important for soil fertility and nutrient cycling (Ponge, 1999; Ono et al., 2011; Chertov and Komarov, 2013). As a basic carrier of nutrients and carbon, forest litterfall plays an essential role in the formation of soil humus (Stevenson, 1994; Prescott et al., 2000; Neumann et al., 2018). Different kinds of litterfall have different chemical compositions and qualities that might determine the development processes of humus in soil (Wei et al., 2018; Zanella et al., 2018a, b). However, there is evidence that shows that the incorporation of fresh organic matter into soil can increase microbial activity due to the higher availability of energy released, then exacerbate soil humus mineralization as named “priming effect” (Broadbent and Nakashima, 1974; Wu et al., 1993; Liljeroth et al., 1994). As such, it is still unclear whether the input of litterfall promotes the synthesis or degradation of soil humic substances. Moreover, both climatic factors (Prescott et al., 2000; Ponge, 2013) and forest litter type (Langenbruch et al., 2012) significantly affect litter humification and soil humus development, which further complicates the relationship between litterfall and soil humic substances.
Litterfall production often varies seasonally (Ma and Wang, 1993; Yang et al., 2017). Different litterfall production has different effects on soil humic substance content. Increasing fresh litterfall inputs increases the amount of CO2 released from the soil, indicating that there is a positive correlation between the decomposition of soil organic matter and the amount of litterfall (Jenkinson, 1977; Leff et al., 2012; Xu et al., 2013). Many studies have also shown that the relationship between soil organic matter and nutrient availability is related to the litter quality (e.g., C/N ratio) (Kuzyakov, 2002; Cheng, 2009). When the C/N ratio of twig litter is high (Yang et al., 2007), microorganisms are more likely to mine N from soil organic matter and thus increase its decomposition (Kuzyakov, 2010). Studies also found that the relative proportions of different litterfall components change with time, resulting in the accumulation or decomposition of soil humic substances content (Fontaine et al., 2003; Kuzyakov, 2010). Litterfall is often dominated by foliar litter during the growing period and by twigs in winter. Foliar litter, which is rich in liable components and nutrients, is more likely to stimulate microbial activity and further promote soil humification and “old humus” degradation (Gonet and Debska, 1998). In contrast, the addition of twig litter, which is rich in refractory substances such as lignin, could stay more readily in soil as the components of soil humus than easily decomposable compounds (Fontaine et al., 2003; Kuzyakov, 2010). Therefore, the effects of litterfall on soil humus content may vary at different seasons. In addition, global warming not only increases litter production (Delucia, 1999) but also increases the litter decomposition rate (Chapin and Shaver, 1996; McHale et al., 1998), which complicates the relationship between litterfall and soil humic substances content.
Extracted humic substances are mainly divided into humic acid (HA) and fulvic acid (FA) (Abakumov et al., 2013; Kõlli and Rannik, 2018); these substances are of great significance to soil fertility, nutrient cycling, and the sustainability of ecosystem productivity (Komarov et al., 2016). FAs are a group of substances that are more active than HAs due to their small molecular weight, aromaticity, and stronger acidity (Wen, 1984). However, FA is easily lost through physical leaching by rainfall and snowmelt water (Elliott, 2013; Ni et al., 2015). High temperatures and year-round low temperatures will prevent the accumulation of soil HA (Wen, 1984). Studies have shown that climate, substrate quality, and the soil environment determine whether the formation of humic substances is dominated by HA or FA (Stevenson, 1994) due to their special properties of acid solubility and alkali solubility (Prescott et al., 2000; Ponge and Chevalier, 2006). However, there are still many unclear factors regarding the formation and transformation of humic substances.
As an important ecosystem in southwestern China (Yang et al., 2005), the subalpine forests in western Sichuan play an important role in the regional and national economy as well as in regulating the climate and conserving water and soil (Zhang et al., 2004; Sun et al., 2005; Wu et al., 2010). The accumulation of soil humic substances is essential for soil fertility and maintaining the stability of subalpine forest ecosystems, but it is closely related to litterfall. However, frequent freeze-thaw cycles and long-term low temperatures in winter can cause physical damage and chemical changes in litterfall further inhibiting the accumulation of humic substances by providing a fast-turn-over substrate for living microorganisms (Deng et al., 2010; Wetterstedt et al., 2010), and freeze-thaw cycles can even destroy the structure of newly formed humus (Dou, 2010) in subalpine forests. These processes could offset the contribution of litter to soil humus. Therefore, the effects of litter on the content of soil humus in subalpine forests remain uncertain.
We hypothesized that retained litter may decrease soil humic substances content, but this effect may be regulated by litter types and litter production in different periods. To test this hypothesis, we selected three representative forests (including coniferous, mixed and broadleaved forests) that dominate the subalpine forests on the eastern Tibetan Plateau, and check the effects of litter input on soil humic substances content as affected by seasonal freeze-thaw from May 2017 to May 2018. Our objective is to assess the accumulation or loss of soil humic substances with and without litter inputs.
Materials and Methods
Study Site
The study site was located in the Wanglang National Nature Reserve (103°55′−104°10′ E, 32°49′−33°02′ N, 2540–2600 m), Pingwu County, Sichuan Province, China. The mean annual temperature ranges from 2.5 to 2.9 °C, and the maximum and minimum temperatures are 26°C (July) and −18°C (January), respectively. The annual mean precipitation is approximately 826 mm, with most falling between May and August. The winter normally extends from late October to late April (Yang et al., 2004). The soil type at the experimental sites is dark brown forest soil according to the Chinese soil genetic classification (Gong et al., 2007) and is classified as a type of Cambisol in the world reference base for soil resources [FAO, 2006 (2007)].
The geological characteristics, dominant arboreal species and representative shrubs of the sites are shown in Table 1.
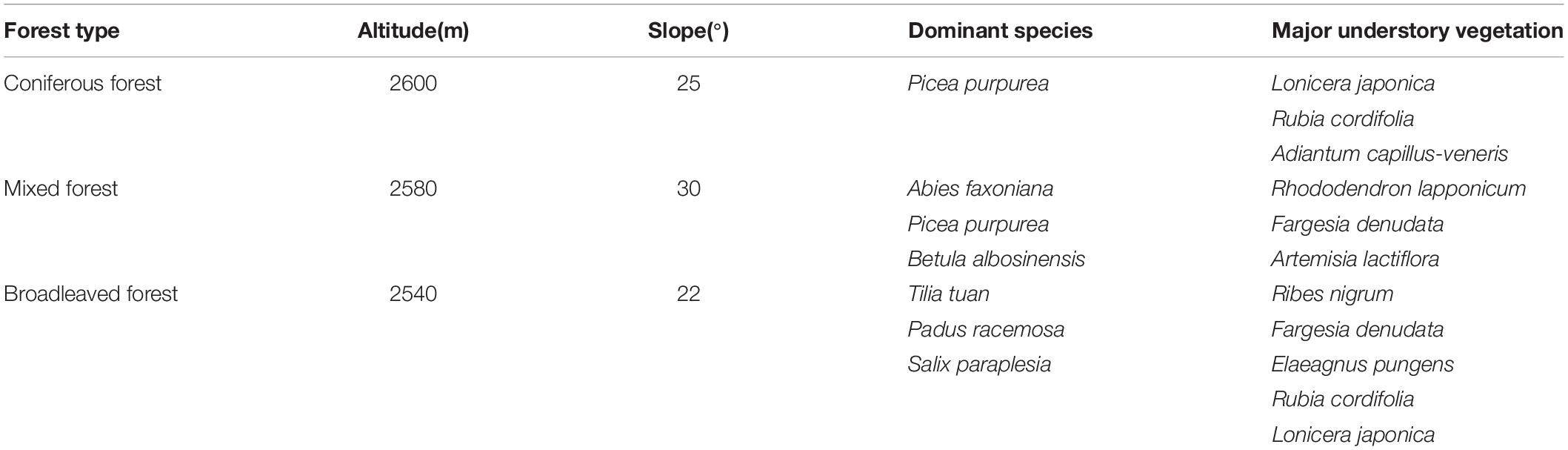
Table 1. Altitude, slope, dominant arboreal species and representative shrubs of the coniferous forest, mixed forest and broadleaved forest in the study site.
Experimental Design
This study was conducted in coniferous, mixed and broadleaved forests with similar elevations and age structures (Table 1). Three plots were established in each forest type at similar altitude, slope and aspect. In May 2017, we set twelve in situ incubation boxes with lengths of 70 cm, widths of 51 cm and heights of 43 cm in each plot, a total of 108 boxes (12 × 3 plots × 3 sites = 108). We dug to about 50 cm depth, removed litterfall, stones, impurities, etc., from the soil and then added the soil to the corresponding in situ incubation boxes. Small holes were drilled in the bottoms of the boxes to ensure that water flow could permeate without removing soil. We set eleven boxes as the litterfall removal boxes and one box as the permanent litterfall input box in each plot. For the permanent litterfall input boxes, no interception net was set above the box. This allowed continuous accumulation of natural input of litter. The litterfall removal boxes meant that the boxes did not accept input of litter. In the litterfall removal boxes, nylon net was fixed 50 cm above the soil surface with brackets. This net captured all falling litterfall and prevented it from reaching the soil. We collected all the litterfall on the nylon net on each sampling date. The daily mean air temperature, air humidity and soil temperature were measured to quantitatively assess the environmental factors. The air temperature and humidity at the three study sites were measured using air temperature and humidity recorders (LITE5032P-RH, Fourtec-Fourier Technologies, Israel) and shown in Figure 1. Button thermometers (iButton DS1923-F5, Maxim/Dallas Semiconductor, Sunnyvale, United States) were randomly buried in the boxes at the three study sites to record the soil temperature.
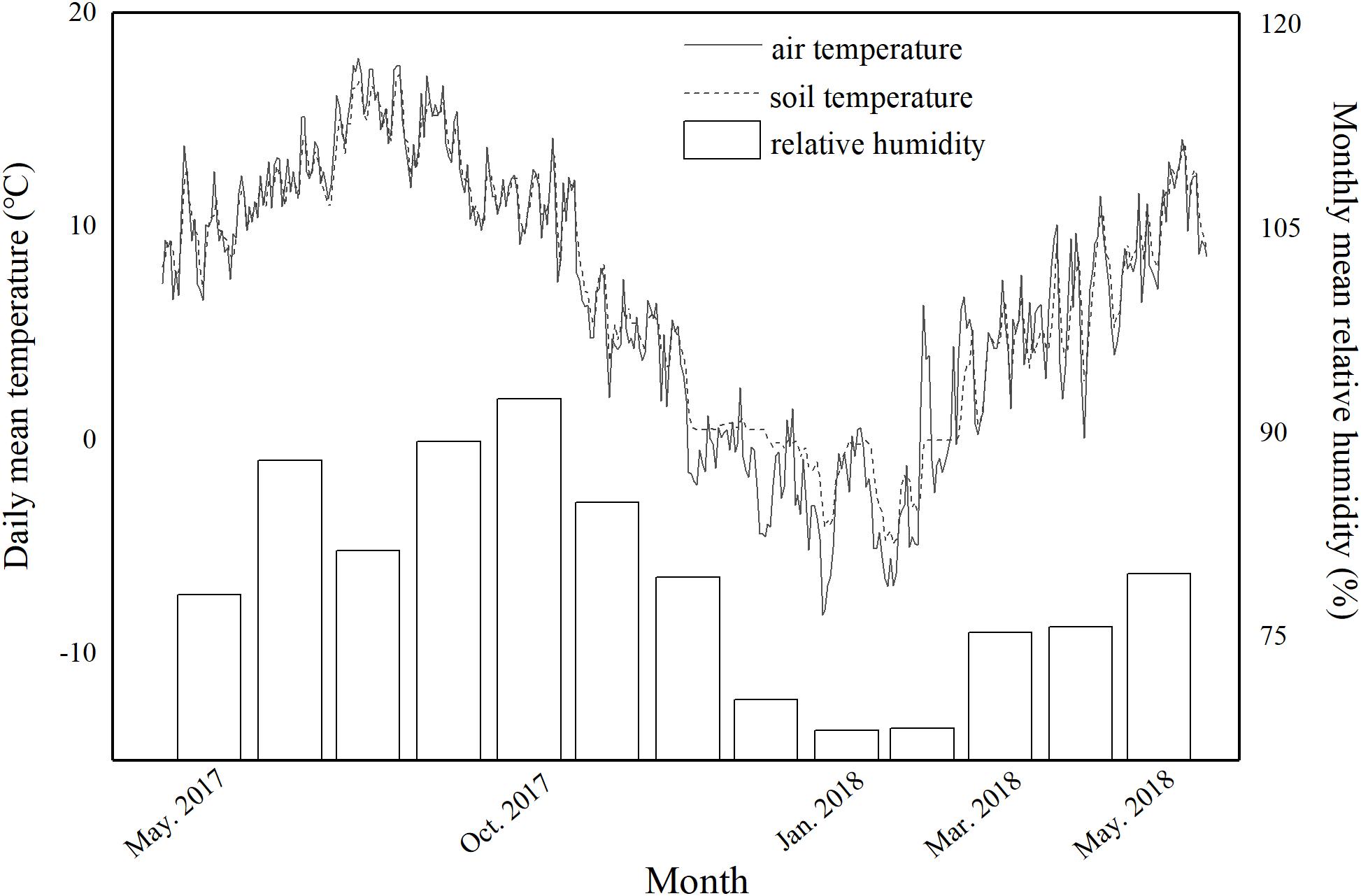
Figure 1. Daily mean temperature of the soil and air and the monthly mean relative humidity of the air in a subalpine forest on the eastern Tibetan Plateau from May 11, 2017 to May 30, 2018.
Based on temperature detection data and previous studies conducted by our group (Wu et al., 2010), the period between two adjacent sampling dates were named the growing season (May 11, 2017–October 29, 2017), early freezing season (October 29, 2017–January 16, 2018), deep freezing season (January 16, 2018–March 24, 2018), and early growing season (March 24, 2018–May 30, 2018).
Sample Collection and Treatment
From May 2017 to May 2018, samples of soil and litterfall were collected at the end of the growing season (October), the onset of the freezing season (January), the end of the freezing season (March), and the start of the growing season (May). First, all the litterfall on the nylon net of the litter removal boxes was collected on each sampling date. All the litterfall from the same plot was evenly mixed and brought back to laboratory to calculate the average litterfall production (Figure 2). Second, the nylon net was removed from one randomly selected litterfall removal box in each plot on each sampling date to allow that box to re-receive litterfall from that time onward. Third, separate soil samples were collected from all permanent litterfall input boxes in every plot on each sampling date. Finally, we collected soil samples from the boxes that were just removed from the nylon net on the sampling date. When collecting the soil samples, the upper litterfall was removed, and the topsoil (0–20 cm, the average depth of soil organic layer in the forest) was collected randomly and mixed uniformity in each in situ incubation box. All litter materials and soil samples were air-dried at room temperature for 1 week. Then, the litter materials were classified as needle leaf, broad leaf, twig litter, flower or fruit litter, and any unrecognized residue was classified as other (including unidentifiable plant residues and animal waste). All soil samples were ground and passed through a 0.25 mm sieve in preparation for the extraction of humic substances.
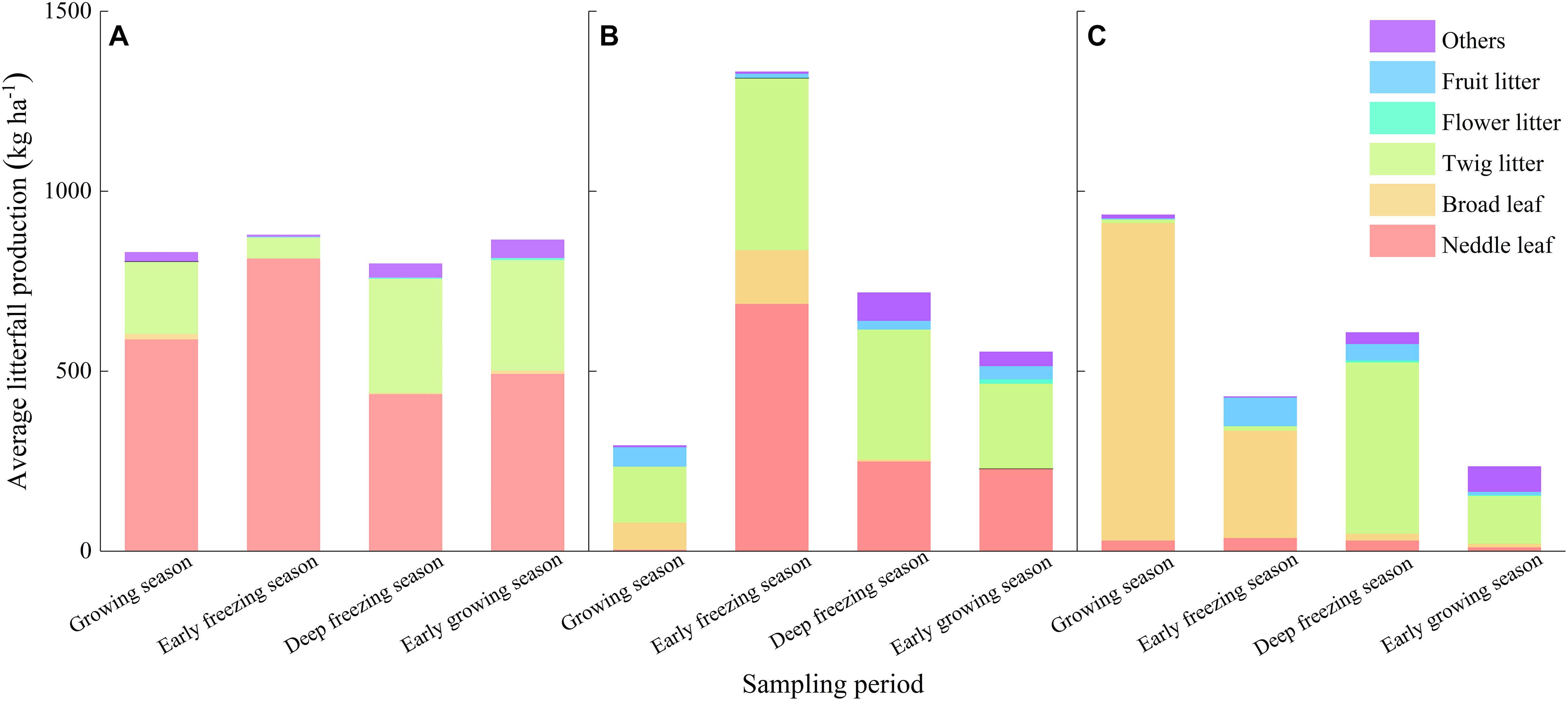
Figure 2. Average production of different litter components in the three forests in the different sampling periods on the eastern Tibetan Plateau: (A) coniferous forest, (B) mixed forest, and (C) broadleaved forest.
Sample Analysis
The extraction and separation methods of soil extractable total humus, HA and FA were as follows. The air-dried samples (0.500 g) were shaken with a mixed solution of 100 mL of 0.1 mol L–1 NaOH+0.1 mol L–1 Na4P2O7 for 10 min and heated at 100°C in boiling water for 1 h (Adani and Ricca, 2004; Wang et al., 2010). The extracted liquid was filtered, and the filtrate was used to analyze the soil extractable total humus. Simultaneously, 20 mL of extracted liquid was collected, and 0.5 mol L–1 H2SO4 was used to separate the HA and FA fractions at 80°C. Then, the HA was dissolved with a hot 0.05 mol L–1 NaOH solution (Kumada et al., 1967). The humus and HA fractions were passed through a 0.45 μm filter and then analyzed for the concentrations of humus and HA using a total organic carbon (TOC) analyzer (vario TOC cube/vario TOC select, Elementar Analysensysteme GmbH, Hanau, Germany).
Calculations and Statistical Analysis
The concentrations of humus and HA were analyzed, and the concentration of FA was calculated as follows (Gigliotti et al., 1999):
where Chumus, CHA and CFA are the contents of total extracted humus, HA, and FA, respectively.
The HA/FA ratios were calculated from the current measured HA and FA contents on each sampling date (Abakumov et al., 2013).
The daily average temperature, number of freeze-thaw cycles were calculated for the different sampling periods based on the temperature data. Freeze-thaw cycles were defines as periods where the temperature was above or below freezing for 3 h until it changed to below or above freezing again (Konestabo et al., 2007).
An analysis of variance (ANOVA) was used to test for significant (P < 0.05) differences in the contents of soil total extracted humus, HA and FA and the HA/FA ratios between the litter retained and removed plots at each period. Correlations between the contents of total extracted humus, HA and FA and the HA/FA ratios and environmental factors under different litter treatments were analyzed by Pearson correlation analysis. The above analyses were performed using SPSS 20.0 (IBM SPSS Statistics Inc., Chicago, IL, United States), and the figures were drawn with Origin Pro9.0 (OriginLab, Northampton, MA, United States).
Results
Total Extracted Humus
The litterfall input of subalpine forests significantly affected the content of soil total extracted humus (F = 27.35, P < 0.01, Table 2), and there were significant differences among forest types and incubation periods (Table 2). Over the 1-year incubation, litterfall significantly decreased the soil total extracted humus content in the mixed forest (Figure 3B), while there were non-significant effects in the coniferous and broadleaved forests (Figures 3A,C). Continuous litterfall input reduced the soil total extracted humus content by 17.5% in the mixed forest. However, litterfall significantly decreased the content of soil total extracted humus in all three forests during the early growing season and the growing season rather than in the other periods (Figure 3). The inhibitory effects of litterfall on the soil total extracted humus in the early growing season was 20.4% for broadleaved forest, 17.5% for coniferous forest, and 6.5% for mixed forest. In contrast, litterfall reduced the soil total extracted humus by 34.5, 31.2 and 6.1% in the growing season in the mixed forest, broadleaved forest, and coniferous forest, respectively.
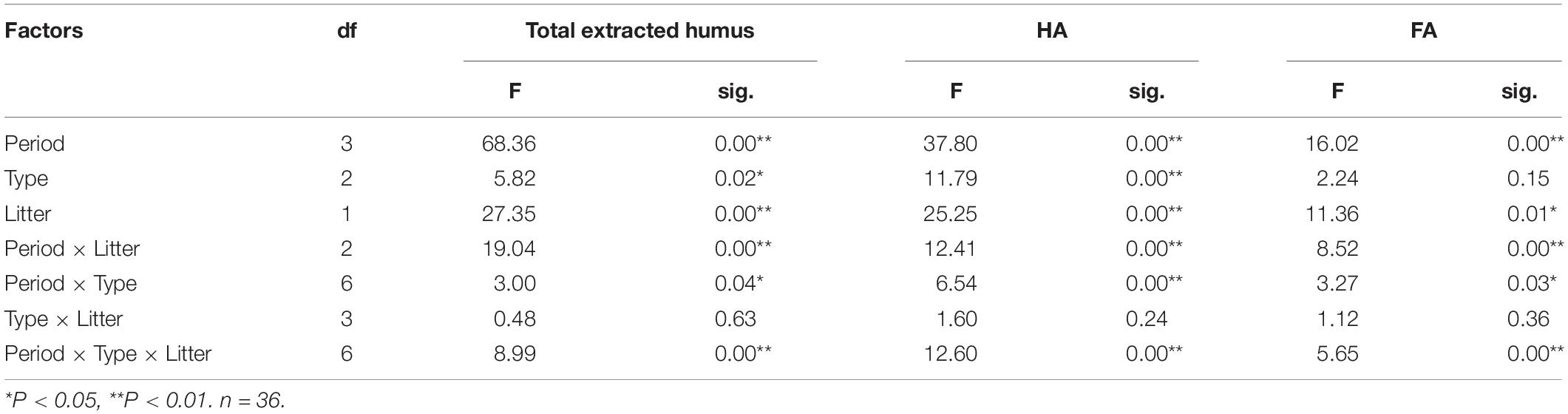
Table 2. Repeated measures ANOVA results for the effects of incubation period, forest type, litter, and their interactions on soil total extracted humus, humic acid, and fulvic acid.
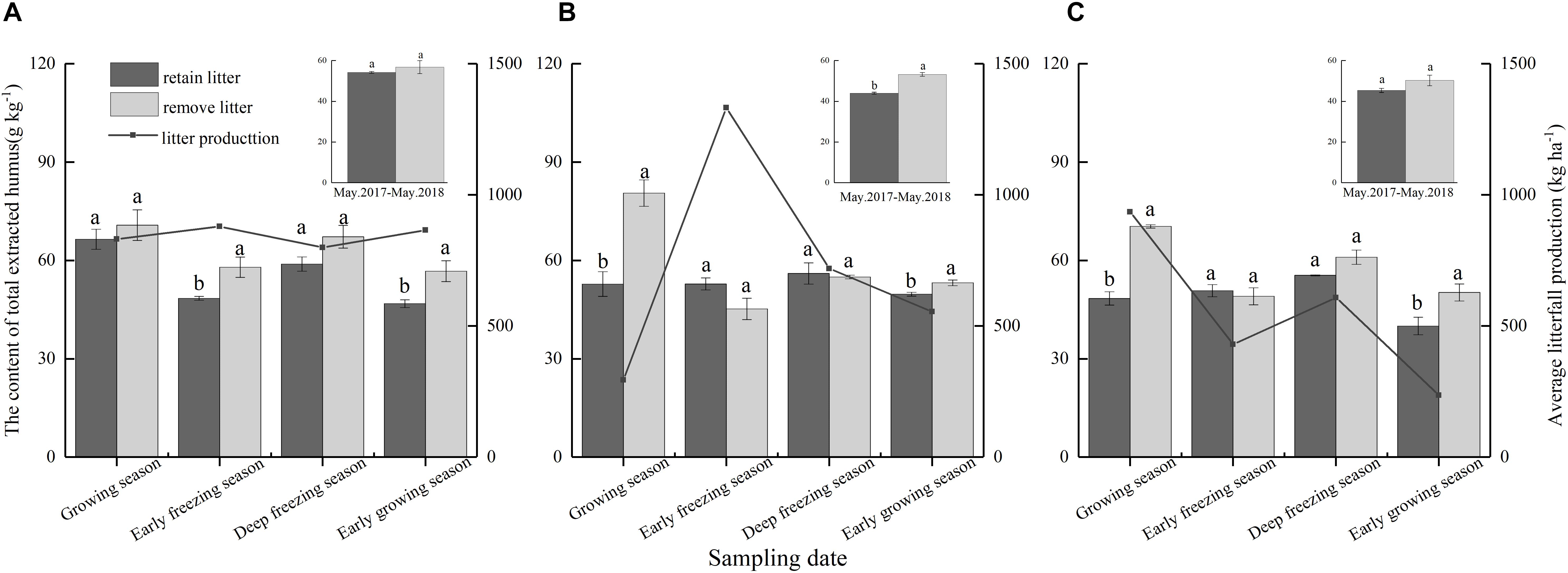
Figure 3. Content of soil total extracted humus was affected by litter removal and average litterfall production at different periods in the (A) coniferous forest, (B) mixed forest, and (C) broadleaved forest. The histograms show the averaged values over 1 year. The lines show the averaged litterfall production in each forest. The presented content values are the means of 3 observations; the error bars represent standard errors. Lowercase letters represent significant differences in the contents of total extracted humus between the different litterfall treatments.
Humic Acid
Similar to the total extracted humus, litterfall significantly affected the HA content in the soil (F = 25.25, P < 0.01, Table 2), and there were significant differences between different forests and seasons (Table 2). The content of HA in the retained litterfall soil was considerably decreased by 26.3% in the mixed forest but not in the coniferous and broadleaved forests after the 1-year incubation. Litter input significantly reduced by 24.8% soil HA content in the coniferous forest during the early growing season (Figure 4A). Litter input also significantly reduced the soil HA content in the mixed forest during the growing season and the early growing season, with a reduction of 36.3 and 7.4%, respectively (Figure 4B). Moreover, litterfall significantly decreased the soil HA content of the broadleaved forest in the growing season and deep freezing season, resulting in a 40.1 and 11.4% reduction, respectively (Figure 4C).
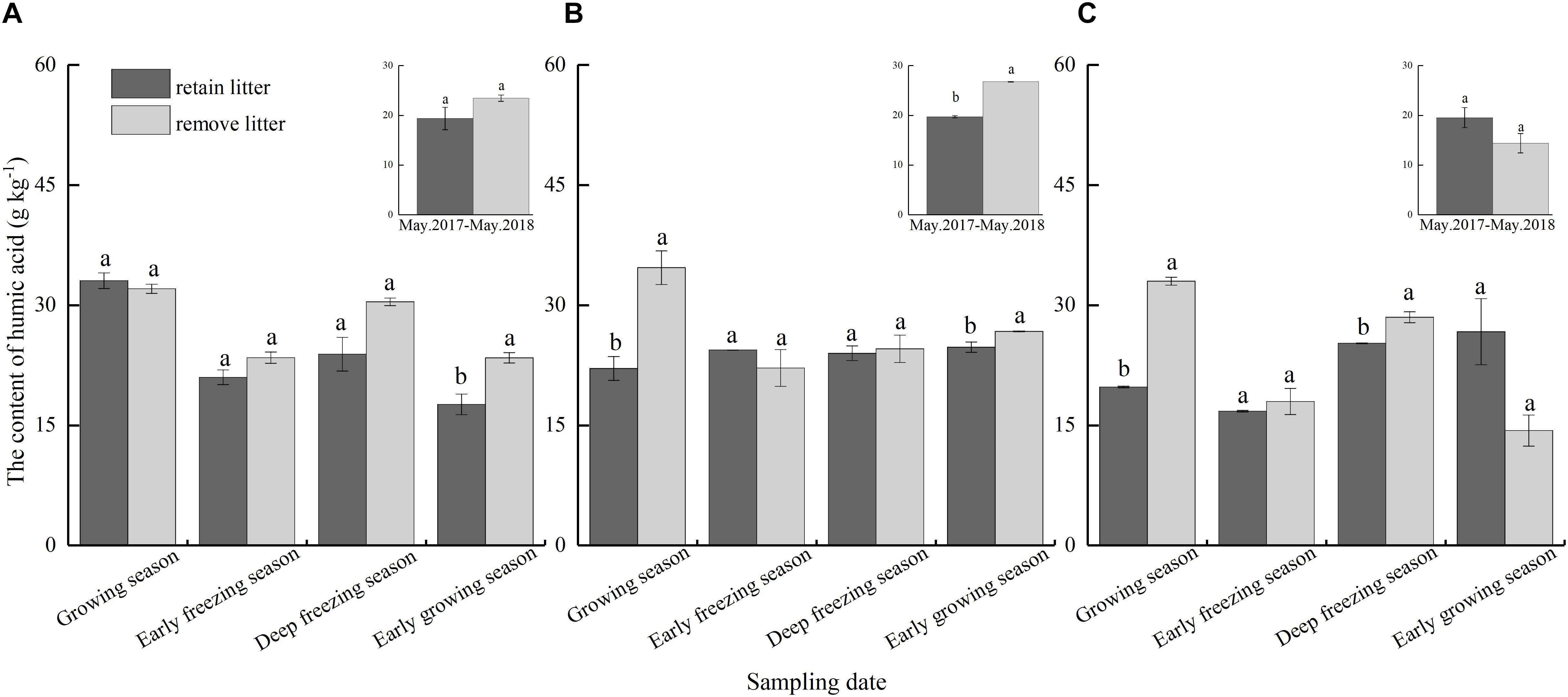
Figure 4. Content of soil humic acid as affected by litter removal at different periods in the (A) coniferous forest, (B) mixed forest, and (C) broadleaved forest. The histograms show the averaged values over 1 year. The presented content values are the means of 3 observations; the error bars represent standard errors. Lowercase letters represent significant differences in the contents of total extracted humus between the different litterfall treatments.
Fulvic Acid
The results showed that litterfall significantly affected the content of soil FA (F = 11.36, P < 0.05, Table 2), and there were significant differences between different seasons (Table 2). The continuous input of litter for 1 year had no significant effect on the content of FA in these three forests (Figure 5). In addition, seasonal litterfall input significantly reduced the content of soil FA in the broadleaved forest only during the early growing season and the growing season (Figure 5C), with a reduction of 63.0 and 23.4%, respectively; there were no significant effects in the other periods and forests.
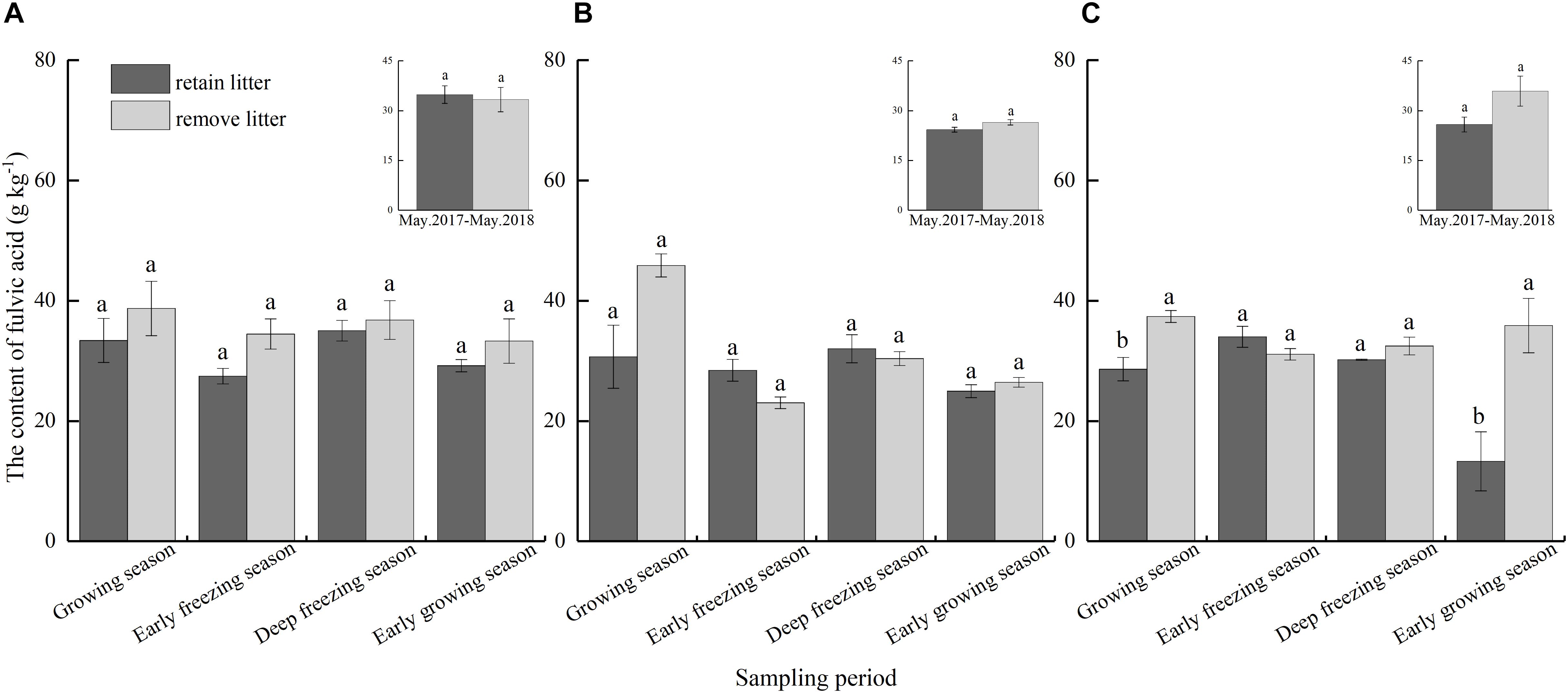
Figure 5. Content of soil fulvic acid as affected by litter removal at different periods in the (A) coniferous forest, (B) mixed forest, and (C) broadleaved forest. The histograms show the averaged values over 1 year. The presented content values are the means of 3 observations; the error bars represent standard errors. Lowercase letters represent significant differences in the contents of total extracted humus between the different litterfall treatments.
HA/FA Ratios
The effects of continuous litterfall input for 1 year on the HA/FA ratios of the three types of forest differed. The HA/FA ratio of the mixed forest (Figure 6B) was reduced significantly, although non-significant effects were detected in the coniferous and broadleaved forests (Figures 6A,C). Seasonal litterfall significantly reduced the HA/FA ratio of the broadleaved forest in the growing season and increased the ratio during the early growing season. Litterfall did not have statistically significant effect on the soil HA/FA ratios in the coniferous and mixed forests in all periods, and the values were always less than 1 (Figures 6A,B).
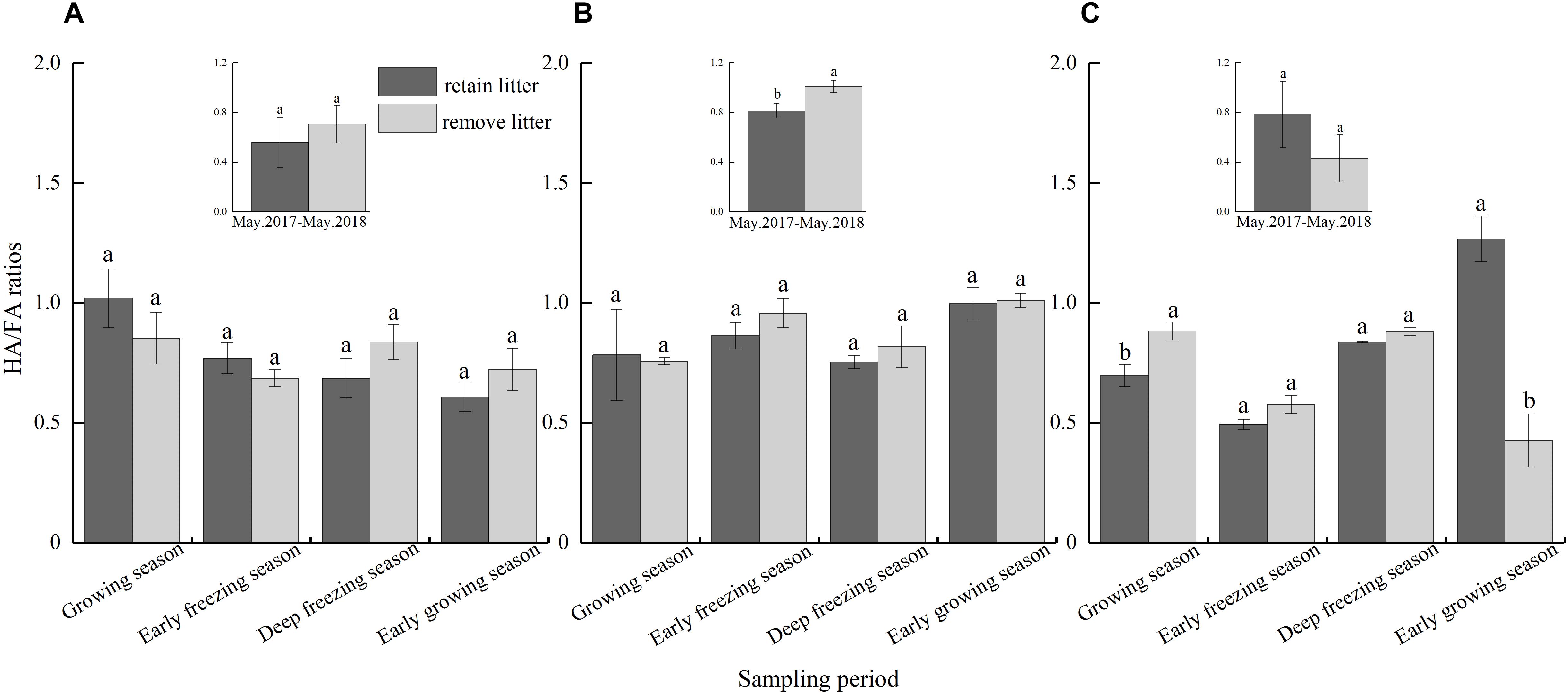
Figure 6. The HA/FA ratios as affected by litter removal at different periods in the (A) coniferous forest, (B) mixed forest, and (C) broadleaved forest. The histograms show the averaged values over 1 year. The presented content values are the means of 3 observations; the error bars represent standard errors. Lowercase letters represent significant differences in the contents of total extracted humus between the different litterfall treatments.
Correlations Between the Extracted Soil Humic Substances and the Environment
The analysis showed that litterfall changed the relationships between the soil humic substances and the selected environmental factors. There was no significant correlation between the soil humic substances and the environmental factors in the litter retained plots in all three forests (Table 3). However, the content of humic substances in the removed litterfall soil was significantly positively correlated with the relative humidity, daily mean temperature, positive accumulated temperature and negative accumulated temperature but was negatively correlated with the number of freeze-thaw cycles in all studied forest types (Table 3).
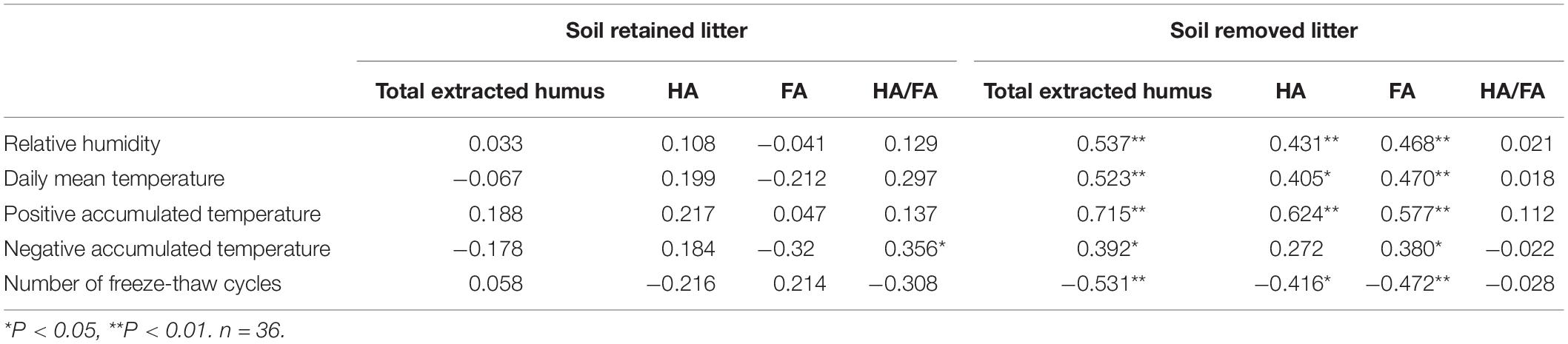
Table 3. Correlation analyses between the total extracted humus, HA, FA, HA/FA ratios and environmental factors with different litterfall treatments.
Discussion
Litterfall transfers approximately one third of the global annual C uptake (approximately 18 Pg⋅C⋅year–1) to soil surface (Grace, 2004; Malhi et al., 2011), although the amount of this C that is sequestered in soils is not fully understood. Humic substances in the soil are in a dynamic process of continuous decomposition and synthesis (Stevenson, 1994), and the amount depends on the relative amounts of the formation and degradation of humic substances (Dou et al., 2007). Our results partly supported our hypothesis that retained litter may decrease soil humic substances content, but this effect may be regulated by litter types and litter production in different periods. Our results show that litterfall promoted a reduction in soil humic substances in the mixed forest but had insignificant effect in the coniferous forest and broadleaved forest. In addition, seasonal litterfall input significantly promoted a reduction in soil total extracted humus in the three forests during the growing season. This finding suggests that the effects of litterfall on soil humic substances were related to the litterfall types. Moreover, a greater amount of seasonal litterfall corresponded to a greater reduction in soil total extracted humus.
Total Extracted Humus
Over 1 year continuous litterfall input, we found that the soil total extracted humus in the mixed forest decreased significantly, indicating that the degradation rate was higher than the synthesis rate in the 1-year incubation. However, litterfall did not have a significant effect on soil extracted humus in coniferous or broadleaved forest. The formation and degradation of humic substances mainly depend on the action of microorganisms (Cotrufo et al., 2013). Litterfall input increases the available carbon source of soil microorganisms (Nadelhoffer et al., 2004), which provides important energy for decomposer activities and stimulates the decomposition or mineralization of soil humic substances that originally existed in the soil (Kuzyakov, 2010; Cotrufo et al., 2013). Moreover, the decomposition rate of mixed forest litterfall has been demonstrated to be faster than that of single litter type (Briones and Ineson, 1996; Hooper, 1998; Chapman and Koch, 2007), and it can enrich the microbial community structure and provide more nutrients for soil microbes to promote their activity (Nadelhoffer et al., 2004), thereby promoting the reduction of soil humic substances. The input of litterfall also provides raw materials for the synthesis of humic substances and promotes their synthesis. Single litter type, such as needle leaves in the coniferous forest and broad leaves in broadleaved forest, decompose slowly and accumulate more refractory substances (Ponge, 2013), which can promote the synthesis of humus and balance the decomposition of humic substances to a certain extent (Gregorich et al., 1996). These results indicate that the effect of litterfall on the soil humic substances is closely related to the litterfall types (Wei et al., 2018).
In the present study, the total extracted humus in the three forests was decreased because of litterfall during the early growing season and the growing season. During the growing season, the greater the amount of litterfall was, the greater the reduction amount of soil total extracted humus, suggesting that the degradation of humus was affected by the amount of litterfall. However, the amount of litterfall during the early growing season occurred in the order of coniferous forest > mixed forest > broadleaved forest (Figure 2), but the amount of reduction occurred in the order of broadleaved forest > coniferous forest > mixed forest (Figure 3). This result may be because the needle litter contained less simple and soluble substrates (Berg, 2000) and was high in C/N, cellulose and lignin (Dai et al., 2001), which have difficulty decomposing and form humus easily (Taylor, 1989; Cotrufo and Ineson, 1995).
Humic Acid, Fulvic Acid and HA/FA Ratios
Soil HA and FA are the main components of soil humic substances, but these two acids have different stability and formation processes. We found that soil HA content and HA/FA ratio significantly decreased in mixed forests after 1 year of continuous litterfall input, but FA content was not affected by litter input. Moreover, the reduction in the soil HA content of mixed forest was more than total extracted humus, which may be due to the conversion of HA and FA (Stevenson, 1994) or the priority synthesis of FA in the synthesis of humus (Dou et al., 2007; Ni et al., 2014, 2016). The effect of seasonal litterfall input on the soil HA was similar to that of the total extracted humus (Figure 4). However, seasonal litterfall significantly reduced the content of HA only in the broadleaved forest during the growing season and early growing season and had no significant effect in the coniferous and mixed forests (Figure 5), which may be related to the litterfall quality and components. The litterfall of the coniferous and mixed forests contained needle litter (Figures 2A,B), which contained terpenoids and phenolic substances (Wu et al., 1993; Shen and Bartha, 1997), and was more likely to cause acidic environments during decomposition (Yang et al., 2007). Acidic environments are more conducive to the synthesis of FA (Dou et al., 2010), and therefore the reductions in soil FA in the coniferous and mixed forests were non-significant. The HA/FA ratios of these forests were less than 1 (Figure 6), suggesting that the synthesis rate of FA was always higher than that of HA. Litterfall had no significant effect on the HA/FA ratios in the coniferous and mixed forests among the different periods, while litterfall significantly decreased the ratio in the broadleaved forest in the early growing season. This result might be due to the fact that litterfall promoted the conversion between HA and FA (Stevenson, 1994). Furthermore, litterfall had no significant effect on the content of soil humic substances in winter. This finding may be because low temperatures and soil freezing inhibited soil microbial activity (Bokhorst et al., 2013) and hindered the physiological metabolism of the microorganisms involved in the formation and degradation of humic substances (Cotrufo et al., 2013).
Litterfall promoted a reduction in soil humic substances mainly in the growing season. Moreover, seasonal litterfall input had a more significant effect on the soil humic substances than continuous litterfall input. The seasonal litterfall input mainly relied on the rapid input of available carbon sources to promote soil microbial activity (Kuzyakov, 2010). After the rapid consumption of soluble carbon sources, continuous litterfall input was mainly dependent on the decomposition of components that are not easily decomposed, such as cellulose and lignin, to provide nutrients to the soil (Kumada et al., 1967). Therefore, we suspected that the input of easily decomposable substances mainly promoted the decomposition of soil humic substances while the input of substances that had difficultly decomposing mainly promoted the synthesis of humus (Fontaine et al., 2003). However, the decomposition of substances that have difficultly decomposing and the synthesis of humic substances are slow processes; therefore, they were not shown in this study.
Correlations Between the Extracted Soil Humic Substances and the Environment
A significant difference was observed between the content of humic substances in the removed litter soil and the environment, although few significant correlations were observed in the retained litter soil (Table 3). This result indicates that litterfall protected or buffered the soil humic substances, making them less susceptible to climatic factors. Furthermore, the content of humic substances in the removed-litter soil might be regulated by biological factors, such as microbial activity. Furthermore, the content of humic substances was significantly positively correlated with the relative humidity, daily mean temperature, positive accumulated temperature and negative accumulated temperature but negatively correlated with the number of freeze-thaw cycles (Table 3). This finding indicates that an increase in temperature affected the content of soil humic substances, and the frequent freeze-thaw cycles in winter may inhibit the formation of humus and even destroy newly formed humus to cause degradation (Dou, 2010). Therefore, under the background of global warming, litterfall can weaken the effects of frequent freeze-thaw cycles (Sahin et al., 2008) and temperature increases (Easterling et al., 1997) on the content of soil humic substances and play a protective role for soil humus.
Conclusion
Litterfall significantly decreased the soil humic substances and HA content of three forests during the growing season but showed insignificant effects in freezing season, implying that a longer growing season and shorter winter caused by global warming may promote the degradation of soil humic substances and the potential loss of soil organic matter. We also found that the relationship between litterfall and soil humus is related to the amount of litterfall during 1-year incubation, displaying a greater increase in the amount of litterfall corresponding to a greater decrease in the content of soil humic substances. Furthermore, a lack of litterfall could increase the sensitivity of soil humic substances to environmental factors. These results showed that, the less litterfall input in a short term, the more conducive to the accumulation of soil humic substances, but the effect of long-term litterfall input on soil humic substances content required further research. This study provided some basic evidence for understanding plant-soil interactions in the subalpine forests.
Data Availability Statement
The raw data supporting the conclusions of this manuscript will be made available by the authors, without undue reservation, to any qualified researcher.
Author Contributions
LZ and FW conceived the study, designed the experiments, and supervised the whole study. XW, YY, YS, ZC, and YD performed the experiments. XW, LZ, and FW wrote the manuscript.
Funding
This work was supported by the National Nature Science Foundation of China (31700542, 31622018, 31670526, and 31570445) and the Fok Ying-Tong Education Foundation (161101).
Conflict of Interest
The authors declare that the research was conducted in the absence of any commercial or financial relationships that could be construed as a potential conflict of interest.
Acknowledgments
We wish to thank the Wanglang National Nature Reserve for their support and all the people who helped with the fieldwork over the course of the experiments. We thank Jiaping Yang for assisting in preparing the figures.
References
Abakumov, E. V., Cajthaml, T., Brus, J., and Frouz, J. (2013). Humus accumulation, humification, and humic acid composition in soils of two post-mining chronosequences after coal mining. J. Soils Sediments 13, 491–500. doi: 10.1007/s11368-012-0579-9
Adani, F., and Ricca, G. (2004). The contribution of alkali soluble (humic acid-like) and unhydrolyzed-alkali soluble (core-humic acid-like) fractions extracted from maize plant to the formation of soil humic acid. Chemosphere 56, 13–22. doi: 10.1016/j.chemosphere.2004.01.040
Berg, B. (2000). Litter decomposition and organic matter turnover in northern forest soils. For. Ecol. Manage. 133, 13–22. doi: 10.1016/s0378-1127(99)00294-7
Bokhorst, S., Metcalfe, D., and Wardle, D. A. (2013). Reduction in snow depth negatively affects decomposers but impact on decomposition rates is substrate dependent. Soil Biol. Biochem. 62, 157–164. doi: 10.1016/j.soilbio.2013.03.016
Briones, M. J. I., and Ineson, P. (1996). Decomposition of eucalyptus leaves in litter mixtures. Soil Biol. Biochem. 28, 1381–1388. doi: 10.1016/S0038-0717(96)00158-7
Broadbent, F. E., and Nakashima, T. (1974). Mineralization of carbon and nitrogen in soil amended with carbon-13 and nitrogen-15 labeled plant material. Soil Sci. Soc. Am. J. 38, 313–315. doi: 10.2136/sssaj1974.03615995003800020029x
Chapin, F. S. I., and Shaver, G. R. (1996). Physiological and growth responses of arctic plants to a field experiment simulating climatic change. Ecology 77, 822–840. doi: 10.2307/2265504
Chapman, S. K., and Koch, G. W. (2007). What type of diversity yields synergy during mixed litter decomposition in a natural forest ecosystem? Plant Soil 299, 153–162. doi: 10.1007/s11104-007-9372-8
Cheng, W. X. (2009). Rhizosphere priming effect: its functional relationships with microbial turnover, evapotranspiration, and C–N budgets. Soil Biol. Biochem. 41, 1795–1801. doi: 10.1016/j.soilbio.2008.04.018
Chertov, O. G., and Komarov, A. S. (2013). Theoretical approaches to modelling the dynamics of soil organic matter. Eurasian Soil Sci. 46, 845–853. doi: 10.1134/S1064229313080012
Cotrufo, M. F., and Ineson, P. (1995). Effects of enhanced atmospheric CO2 and nutrient supply on the quality and subsequent decomposition of fine roots of Betula pendula Roth. and Picea sitchensis (Bong.) Carr. Plant Soil 170, 267–277. doi: 10.1007/bf00010479
Cotrufo, M. F., Wallenstein, M. D., Boot, C. M., Denef, K., and Paul, E. (2013). The Microbial Efficiency-Matrix Stabilization (MEMS) framework integrates plant litter decomposition with soil organic matter stabilization: do labile plant inputs form stable soil organic matter? Glob. Change Biol. 19, 988–995. doi: 10.1111/gcb.12113
Dai, L. M., Xu, Z. B., Zhang, Y. J., and Chen, H. (2001). Study on decomposition rate and fall of Pinus koraiensis needle. Acta Ecol. Sin. 21, 1296–1300. doi: 10.3321/j.issn:1000-0933.2001.08.013
Delucia, E. H. (1999). Net primary production of a forest ecosystem with experimental CO2 enrichment. Science 284, 1177–1179. doi: 10.1126/science.284.5417.1177
Deng, R. J., Yang, W. Q., and Wu, F. Z. (2010). Changes in litter quality of subalpine forests during one freeze-thaw season. Acta Ecol. Sin. 30, 830–835.
Dou, S., Tardy, Y., Zhang, J. J., Kai, L., Yu, S. Q., Ping, L. F., et al. (2010). Thermo dynamic stability of humic acid and fulvic acid in soil and its driving factors. Acta Pedol. Sin. 47, 71–76. doi: 10.11766/trxb200804300111
Dou, S., Yu, S. Q., and Zhang, J. J. (2007). Effects of carbon dioxide concentration on humus formation in corn stalk decomposition. Acta Pedol. Sin. 44, 458–446. doi: 10.3321/j.issn:0564-3929.2007.03.012
Easterling, D. R., Horton, B., and Jones, P. D. (1997). Maximum and Minimum Temperature Trends for the Globe. Science 277, 364–367. doi: 10.1126/science.277.5324.364
Elliott, J. (2013). Evaluating the potential contribution of vegetation as a nutrient source in snowmelt runoff. Can. J. Soil Sci. 93, 435–443. doi: 10.4141/cjss2012-050
Fontaine, S., Mariotti, A., and Abbadie, L. (2003). The priming effect of organic matter: a question of microbial competition? Soil Biol. Biochem. 35, 837–843. doi: 10.1016/s0038-0717(03)00123-8
Gigliotti, G., Businelli, D., and Giusquiani, P. L. (1999). Composition changes of soil humus after massive application of urban waste compost: a comparison between FT-IR spectroscopy and humification parameters. Nutr. Cycl. Agroecosyst. 55, 23–28. doi: 10.1023/a:1009829008018
Gonet, S. S., and Debska, B. (1998). Properties of humic acids developed during humification process of post-harvest plant residues. Environ. Int. 24, 603–608. doi: 10.1016/S0160-4120(98)00034-8
Gong, Z. T., Zhang, G. L., and Chen, Z. C. (2007). Pedgenesis and Soil Taxonomy. Beijing: Science Press.
Grace, J. (2004). Understanding and managing the global carbon cycle. J. Ecol. 92, 189–202. doi: 10.1111/j.0022-0477.2004.00874.x
Gregorich, E. G., Ellert, H. H., Drury, C. F., and Liang, B. C. (1996). Fertilization effects on soil organic matter turnover and corn residue C storage. Soil Sci. Soc. Am. J. 60, 472–476. doi: 10.2136/sssaj1996.03615995006000020019x
Hooper, D. U. (1998). The role of complementarity and competition in ecosystem responses to variation in plant diversity. Ecology 79, 704–719. doi: 10.2307/176964
Jenkinson, D. S. (1977). Studies on the decomposition of plant material in soil.: the effect of rate of addition. J. Soil Sci. 28, 417–423. doi: 10.1111/j.1365-2389.1977.tb02249.x
Kogel-Knabner, I. (2000). Analytical approaches for characterizing soil organic matter. Org. Geochem. 31, 609–625. doi: 10.1016/S0146-6380(00)00042-5
Kõlli, R., and Rannik, K. (2018). Matching Estonian humus cover types’ (pro humus forms’) and soils’ classifications. Appl. Soil Ecol. 123, 627–631. doi: 10.1016/j.apsoil.2017.09.038
Komarov, A., Chertov, O., Bykhovets, S., Shaw, C., Nadporozhskaya, M., Frolov, P., et al. (2016). Romul-Hum model of soil organic matter formation coupled with soil biota activity. I. Problem formulation, model description, and testing. Ecol. Model. 345, 113–124. doi: 10.1016/j.ecolmodel.2016.08.007
Konestabo, H. S., Michelsen, A., and Holmstrup, M. (2007). Responses of springtail and mite populations to prolonged periods of soil freeze-thaw cycles in a sub-artic ecosystem. Appl. Soil Ecol. 36, 136–146. doi: 10.1016/j.apsoil.2007.01.003
Kumada, K., Sato, O., Ohsumi, Y., and Ohta, S. (1967). Humus composition of mountain soils in Central Japan with special reference to the distribution of P type humic acid. Soil Sci. Plant Nutr. 13, 151–158. doi: 10.1080/00380768.1967.10431990
Kuzyakov, Y. (2002). Review: factors affecting rhizosphere priming effects. J. Plant Nutr. Soil Sci. 165, 382–396.
Kuzyakov, Y. (2010). Priming effects: interactions between living and dead organic matter. Soil Biol. Biochem. 42, 1363–1371. doi: 10.1016/j.soilbio.2010.04.003
Langenbruch, C., Helfrich, M., and Flessa, H. (2012). Effects of beech (Fagus sylvatica), ash (Fraxinus excelsior) and lime (Tiliaspec.) on soil chemical properties in a mixed deciduous forest. Plant Soil 352, 389–403. doi: 10.1007/s11104-011-1004-7
Leff, J. W., Wieder, W. R., Taylor, P. G., Townsend, A. R., Nemergut, D. R., Stuart, G. A., et al. (2012). Experimental litterfall manipulation drives large and rapid changes in soil carbon cycling in a wet tropical forest. Glob. Change Biol. 18, 2969–2979. doi: 10.1111/j.1365-2486.2012.02749.x
Liljeroth, E., Kuikman, E., and Van Veen, J. A. (1994). Carbon translocation to the rhizosphere of maize and wheat and influence on the turnover of native soil organic matter at different soil nitrogen levels. Plant Soil 161, 231–240. doi: 10.1007/bf00046394
Ma, Z. G., and Wang, J. X. (1993). A study on the dynamics of forest litter in the habitat of Giant Panda. Acta Phytoecol Geobot Sin. 17, 61–69.
Malhi, Y., Doughty, D., and Galbraith, D. (2011). The allocation of ecosystem net primary productivity in tropical forests. Philos. Trans. R. Soc. B-Biol. Sci. 366, 3225–3245. doi: 10.1098/rstb.2011.0062
McHale, P. J., Mitchell, M. J., and Bowles, F. P. (1998). Soil warming in a northern hardwood forest: trace gas fluxes and leaf litter decomposition. Can. J. For. Res. 28, 1365–1372. doi: 10.1139/x98-118
Nadelhoffer, K. J., Colman, B. P., Currie, W. S., Magill, A., and Aber, J. D. (2004). Decadal-scale fates of 15N tracers added to oak and pine stands under ambient and elevated N inputs at the Harvard Forest (USA). For. Ecol. Manage. 196, 89–107. doi: 10.1016/j.foreco.2004.03.014
Neumann, M., Ukonmaanaho, L., Johnson, J., Benham, S., Vesterdal, L., Novotnı, R., et al. (2018). Quantifying carbon and nutrient input from litterfall in European forests using field observations and modelling. Glob. Biogeochem. Cycle 32, 784–798. doi: 10.1029/2017gb005825
Ni, X. Y., Yang, W. Q., Li, H., Xu, L. Y., He, J., and Wu, F. Z. (2014). Effects of snowpack on early foliar litter humification during winter in a subalpine forest of western Sichuan. Acta Phytophysiol. Sin. 38, 540–549. doi: 10.3724/SP.J.1258.2014.00050
Ni, X. Y., Yang, W. Q., Tan, B., He, J., Xu, L. Y., Li, H., et al. (2015). Accelerated foliar litter humification in forest gaps: dual feedbacks of carbon sequestration during winter and the growing season in an alpine forest. Geoderma 24, 136–144. doi: 10.1016/j.geoderma.2014.11.018
Ni, X. Y., Yang, W. Q., Tan, B., Li, H., He, J., Xu, L. Y., et al. (2016). Forest gaps slow the sequestration of soil organic matter: a humification experiment with six foliar litters in an alpine forest. Sci. Rep. 6:19744. doi: 10.1038/srep19744
Ono, K., Hiradate, S., Morita, S., Ohse, K., and Hirai, K. (2011). Humification processes of needle litters on forest floors in Japanese cedar (Cryptomeria japonica) and Hinoki cypress (Chamaecyparis obtusa) plantations in Japan. Plant Soil 338, 171–181. doi: 10.1007/s11104-010-0397-z
Ponge, J. F. (1999). “Interaction between soil fauna and their environment,” in Going Underground. Ecological Studies in Forest Soils, eds N. Rastin and J. Bauhus (Trivandrum: Research Signpost), 45–76. doi: 10.13140/2.1.1819.6165
Ponge, J. F. (2013). Plant–soil feedbacks mediated by humus forms: a review. Soil Biol. Biochem. 57, 1048–1060. doi: 10.1016/j.soilbio.2012.07.019
Ponge, J. F., and Chevalier, R. (2006). Humus Index as an indicator of forest stand and soil properties. For. Ecol. Manage. 233, 165–175. doi: 10.1016/j.foreco.2006.06.022
Prescott, C. E., Maynard, D. G., and Laiho, R. (2000). Humus in northern forests: friend or foe? For. Ecol. Manag. 133, 23–36. doi: 10.1016/s0378-1127(99)00295-9
Sahin, U., Angin, I., and Kiziloglu, F. M. (2008). Effect of freezing and thawing processes on some physical properties of saline–sodic soils mixed with sewage sludge or fly ash. Soil Tillage Res. 99, 254–260. doi: 10.1016/j.still.2008.03.001
Shen, J., and Bartha, R. (1997). Priming effect of glucose polymers in soil-based biodegradation tests. Soil Biol. Biochem. 29, 1195–1198. doi: 10.1016/S0038-0717(97)00031-X
Stevenson, F. J. (1994). Humus chemistry: genesis, composition, reactions. Soil Sci. 135, 129–130. doi: 10.1097/00010694-198302000-00014
Sun, G., Wu, N., and Luo, P. (2005). Soil N pools and transformation rates under different land uses in a subalpine forest-grassland ecotone. Pedosphere 15, 52–58. doi: 10.1007/s10705-004-5083-1
Taylor, B. R. (1989). Nitrogen and lignin Content as predictors of litter decay rates: a microcosm test. Ecology 70, 97–104. doi: 10.2307/1938416
Wang, H., Hong, Y. T., Lin, Q. H., Hong, B., Zhu, Y. X., Wang, Y., et al. (2010). Response of humification degree to monsoon climate during the Holocene from the Hongyuan peat bog, eastern Tibetan Plateau. Paleogeogr. Paleoclimatol. Paleoecol. 286, 171–177. doi: 10.1016/j.palaeo.2009.12.015
Wei, X. Y., Yang, W. Q., Zhang, L., Tan, B., Chen, Y., Dong, Y. L., et al. (2018). Effects of litter addition on soil humification during freeze-thaw cycles in a subalpine forest. Acta Ecol. Sin. 38, 6521–6529. doi: 10.5846/stxb201802060311
Wen, Q. X. (1984). Composition, formation and decomposition of soil organic matter. Soils 16, 121–129. doi: 10.13758/j.cnki.tr.1984.04.001
Wetterstedt, J. A. M., Persson, T., and Agren, G. I. (2010). Temperature sensitivity and substrate quality in soil organic matter decomposition: results of an incubation study with three substrates. Glob. Change Biol. 16, 1806–1819. doi: 10.1111/j.1365-2486.2009.02112.x
Wu, F. Z., Yang, W. Q., Zhang, J., and Deng, R. J. (2010). Litter decomposition in two subalpine forests during the freeze-thaw season. Acta Oecol. Int. J. Ecol. 36, 135–140. doi: 10.1016/j.actao.2009.11.002
Wu, J., Brookes, P. C., and Jenkinson, D. S. (1993). Formation and destruction of microbial biomass during the decomposition of glucose and ryegrass in soil. Soil Biol. Biochem. 25, 1435–1441. doi: 10.1016/0038-0717(93)90058-j
Xu, S., Liu, L., and Sayer, E. J. (2013). Variability of above-ground litter inputs alters soil physicochemical and biological processes: a meta-analysis of litterfall-manipulation experiments. Biogeosciences 10, 7423–7433. doi: 10.5194/bg-10-7423-2013
Yang, J. P., Liao, R., Yang, W. Q., Tan, B., Fu, C. K., Zhang, Y., et al. (2017). Litter production and its dynamic pattern in a dark coniferous forest in the alpine gorge region. Chin. J. Appl. Environ. Biol. 23, 0745–0752. doi: 10.3724/SP.J.1145.2017.03020
Yang, W. Q., Deng, R. J., and Zhang, J. (2007). Forest litter decomposition and its responses to global climate change. Chin. J. Appl. Ecol. 18, 2889–2895. doi: 10.1360/yc-007-1324
Yang, W. Q., Wang, K. Y., Kellomäki, S., and Gong, H. D. (2005). Litter dynamics of three subalpine forests in Western Sichuan. Pedosphere 5, 653–659. doi: 10.1002/jpln.200521793
Yang, W. Q., Wang, K. Y., Kellomäki, S., and Xiao, L. (2004). Wet canopy evaporation rate of three stands in Western Sichuan. China. J. Mountain Sci. 1, 166–174. doi: 10.1007/bf02919338
Zanella, A., Berg, B., Ponge, J. F., and Kemmers, R. H. (2018a). Humusica 1, article 2: essential basesfunctional considerations. Appl. Soil Ecol. 122, 22–41. doi: 10.1016/j.apsoil.2017.07.010
Zanella, A., Ponge, J. F., and Matteodo, M. (2018b). Humusica 1, article 7: terrestrial humus systems and forms-field practice and sampling problems. Appl. Soil Ecol. 122, 92–102. doi: 10.1016/j.apsoil.2017.05.028
Keywords: litterfall, humic substances, humic acid, fulvic acid, soil organic matter, subalpine forest
Citation: Wei X, Yang Y, Shen Y, Chen Z, Dong Y, Wu F and Zhang L (2020) Effects of Litterfall on the Accumulation of Extracted Soil Humic Substances in Subalpine Forests. Front. Plant Sci. 11:254. doi: 10.3389/fpls.2020.00254
Received: 25 April 2019; Accepted: 18 February 2020;
Published: 05 March 2020.
Edited by:
Sanna Sevanto, Los Alamos National Laboratory (DOE), United StatesReviewed by:
Manuel J. Macía, Autonomous University of Madrid, SpainHua Qin, Zhejiang Agriculture and Forestry University, China
Copyright © 2020 Wei, Yang, Shen, Chen, Dong, Wu and Zhang. This is an open-access article distributed under the terms of the Creative Commons Attribution License (CC BY). The use, distribution or reproduction in other forums is permitted, provided the original author(s) and the copyright owner(s) are credited and that the original publication in this journal is cited, in accordance with accepted academic practice. No use, distribution or reproduction is permitted which does not comply with these terms.
*Correspondence: Fuzhong Wu, d3VmemNoaW5hQDE2My5jb20=; Li Zhang, emhhbmdsaTE5ODMwMTE2QGhvdG1haWwuY29t