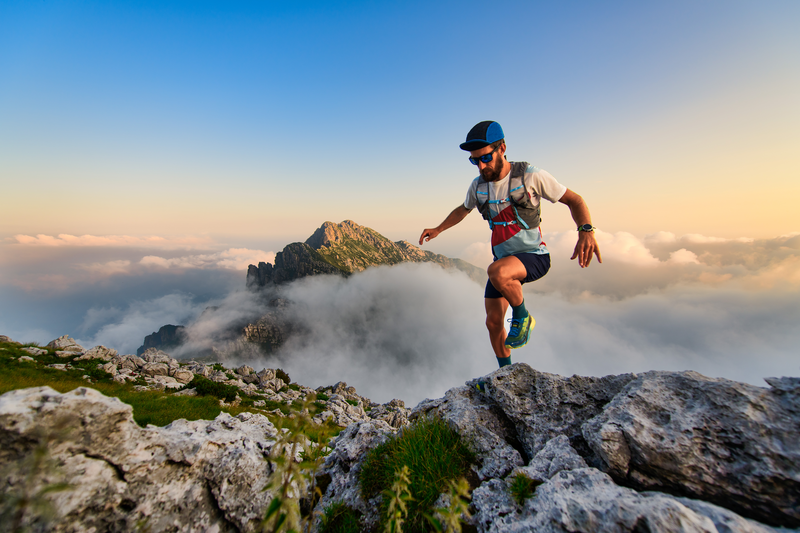
95% of researchers rate our articles as excellent or good
Learn more about the work of our research integrity team to safeguard the quality of each article we publish.
Find out more
REVIEW article
Front. Plant Sci. , 06 March 2020
Sec. Plant Breeding
Volume 11 - 2020 | https://doi.org/10.3389/fpls.2020.00252
This article is part of the Research Topic Introgression Breeding in Cultivated Plants View all 15 articles
Breeding progress in most crops has relied heavily on the exploitation of variation within the species’ primary gene pool, a process which is destined to fail once the supply of novel variants has been exhausted. Accessing a crop’s secondary gene pool, as represented by its wild relatives, has the potential to greatly expand the supply of usable genetic variation. The crop in which this approach has been most strongly championed is bread wheat (Triticum aestivum), a species which is particularly tolerant of the introduction of chromosomal segments of exotic origin thanks to the genetic buffering afforded by its polyploid status. While the process of introgression can be in itself cumbersome, a larger problem is that linkage drag and/or imperfect complementation frequently impose a yield and/or quality penalty, which explains the reluctance of breeders to introduce such materials into their breeding populations. Thanks to the development of novel strategies to induce introgression and of genomic tools to facilitate the selection of desirable genotypes, introgression breeding is returning as a mainstream activity, at least in wheat. Accessing variation present in progenitor species has even been able to drive genetic advance in grain yield. The current resurgence of interest in introgression breeding can be expected to result in an increased deployment of exotic genes in commercial wheat cultivars.
The process of crop domestication, in which selection is applied to a population of wild plants, inevitably introduces a major genetic bottleneck (Simmonds, 1976). After many centuries of farmer-based selection, the late 19th century ushered in the hugely successful era of scientific plant breeding, a process which is predicated on creating novel combinations of the genetic variants represented in the crop’s primary gene pool (Borlaug, 1983). While continuing to deliver genetic advances in many crops, the evidence now suggests that a yield plateau has been reached in certain crops, leading to concerns that the still growing global demand for plant-based products (food, feed, fiber, and industrial products such as starch and oil) will not be easily met without innovations being made in breeding technology (Tester and Langridge, 2010). First and foremost is the need therefore to expand the genetic base of the most intensely bred crop species, notably maize, rice, and wheat.
The secondary gene pool of a crop species is populated by its wild (as well as, in some cases, its cultivated) relatives. Accessing this gene pool typically requires ab initio the creation of a wide hybrid between the domesticate and one of its related species. Reducing the representation of the exotic parent’s genome is most straight-forwardly achieved by backcrossing the hybrid to the domesticate (Anderson and Hubricht, 1938; Anderson, 1949), resulting in so-called “introgression” materials. While creating a viable wide hybrid is generally considered to require human intervention, wide hybrids clearly occur in the wild, as witnessed by the evolution of many natural allopolyploids, such as tetraploid and hexaploid wheat, oat, cotton, sugarcane, canola, coffee, and tobacco (Ribeiro-Carvalho et al., 2004; Dvorak et al., 2006; Udall and Wendel, 2006; Soltis et al., 2009). Introgression has made some significant contributions to crop improvement: an example is the introduction into rice of a gene conditioning male sterility gene from its wild relative Oryza rufipogon, which has facilitated the production of plentiful and affordable F1 hybrid grain, and thereby led to the widespread exploitation of heterosis (Yuan, 1966). In another example, a chromosome comprising a segment of a wheat chromosome fused to one from rye (Secale cereale) by the early 1990s was present in almost one half of the bread wheat cultivars bred in 17 countries (Rabinovich, 1998). Polyploid species such as wheat tend to be better able than diploid ones to tolerate the presence of exotic chromatin due to the buffering provided by the presence of multiple homeologs (Sears, 1956; Dubcovsky and Dvorak, 2007).
Bread wheat represents a case model for introgression breeding. The species belongs to the Triticeae tribe which houses over 500 species, including several other important crop species [macaroni wheat (T. durum), barley (Hordeum vulgare), and rye] and fodder species (Wang et al., 1994; Yen et al., 2005). The genome of each members of the tribe comprises a combination of 26 related, but distinct genomes (Liu et al., 2017a). The first reported wide hybrids made between Triticeae species date back to an attempt to cross bread wheat with rye by Wilson (1876). The literature describing so-called “chromosome engineering” (Sears, 1972) is dominated by experiments conducted using bread wheat as the recipient. Wide hybrids involving Triticeae species have been used to understand a number of basic phenomena, such as the loss of centromeric histone H3 which accompanies chromosome elimination (Sanei et al., 2011), the formation of unreduced gametes underlying spontaneous chromosome doubling (Hao et al., 2014) and the restriction of chromosome pairing in allopolypoids to homologs (Sears, 1976).
Many papers have been published on the theme of introgression in wheat, and some useful reviews have been assembled by Sears (1981), Friebe et al. (1996), Kole (2011), and Mason (2017). The aim here was therefore not to provide another review of wheat introgression, but rather to discuss how some recently developed breeding strategies are contributing to a resurgence of interest in introgression breeding. Although the focus of the review is very much concentrated on wheat, many of these issues will be equally applicable to other crop species.
The gene pool concept proposed by Harlan and de Wet (1971) rests on a classification of the ease/difficulty of generating viable sexual hybrids, which is the sine qua non of introgression. In their scheme, a crop species’ near relatives fall into either the primary (GP-1), secondary (GP-2) or tertiary (GP-3) gene pools. Membership of the three gene pools in Triticeae is determined largely by the species’ genomic constitution (Jiang et al., 1993; Feuillet et al., 2008). In the case of common wheat as the recipient species, we here re-consider Triticeae species as one of four types based on the genome constitution and the ease/difficulty of introgression breeding.
GP-1 species share the same three genomes (B, A, and D) which make up the bread wheat genome. These species, such as T. spelta and T. macha, are fully sexually compatible with bread wheat. As a result, introgression is achievable through conventional crossing and selection, since recombination between the chromosomes of the recipient bread wheat and the donor relative is effectively unrestricted. Some back-crossing is usually required to restore the bread wheat genetic background.
GP-2 species share only some of the bread wheat genomes. Two prominent examples are T. turgidum, a group of tetraploid wheats of genomic constitution BA and Aegilops tauschii (goatgrass), the donor of bread wheat’s D genome. Like GP-1 species, these are generally readily crossable with wheat, although because of their unbalanced chromosome constitution, the resulting hybrids are typically only poorly fertile. Nevertheless, introgression is relatively straight-forward as it can be affected by homologous recombination. These species are of particular value as a genetic resource for bread wheat improvement. As a result, there has been a concerted, worldwide effort to mine variation from these GP-2 species (particularly Ae. tauschii) by creating synthetic hexaploid wheats (SHWs), which replicate the natural wide cross responsible for the speciation of hexaploid wheat (Mujeeb-Kazi et al., 1996).
GP-3 species share no homologous genomes with bread wheat; they thus include the majority of the Triticeae species, including the two domesticates rye (R genome) and barley (H genome). For these species, not only are wide crosses less easy to develop (generally requiring embryo rescue because the hybrid endosperm fails to develop), but also introgression has to rely on inducing either homeologous recombination or a chromosome breakage-fusion event.
GP-2/3 species feature at least one genome present in bread wheat, alongside at least one which is homeologous. Examples include Ae. cylindrica (CD) and Ae. ventricosa (DN) and the large number of synthetic wheat × rye amphiploids (BAR and BADR), referred to as triticales. Here, introgression is possible via homologous recombination, provided that the target gene resides within a chromosome belonging to the homologous genome. Otherwise, as for the GP-3 species, introgression has to rely on inducing either homeologous recombination or a chromosome breakage-fusion event.
In addition, it has been proposed to add a fourth gene pool (GP-4) to acknowledge the potential of transgenesis (Suslow et al., 2002; Kumar and Rustgi, 2014) and somatic hybridization (Xia et al., 2003) to introduce genes without any requirement for the prior formation of a sexual hybrid. While wheat’s GP-4 in principle harbors every living organism, from microbe to mammal, it also includes a number of related species, notably sorghum (Sorghum bicolor), Job’s tears (Coix lacryma-jobi), and Cogon grass (Imperata cylindrica) (Liu et al., 2014); while it is possible to culture in vitro immature hybrid embryos formed when wheat is pollinated by these species and to regenerate viable plants, the non-wheat chromosomes are rapidly eliminated during the zygote’s early cell divisions, so that the regenerants are effectively wheat haploids. Note in passing that access to transgenic crops is limited in many countries, meaning that GP-4 represents at best a theoretical resource, at least for the present.
While a substantial research investment has been made into creating introgression materials, their impact on wheat improvement has been relatively modest. The major reason for this outcome is that many of genotypes have proven to be defective in terms of plant type, grain yield and/or grain quality, reflecting a combination of linkage drag and an inadequate level of genetic complementation.
When a target gene is introduced, whether this is achieved using recombination or chromosome breakage/fusion, it will inevitably be accompanied by other genes linked to it on the introgressed segment; some of these genes may have deleterious consequences on the plant’s agronomy, productivity or grain quality. The ideal therefore is to engineer a transfer which involves as short an introgressed segment as possible. Linkage drag can potentially be overcome where the introgression has been achieved by homologous recombination, since the length of the segment can in principle simply be reduced by inducing further rounds of recombination enabled by a program of backcrossing. Linkage drag is more difficult to negate in materials which have been generated as a result of a homeologous recombination event, because no further recombination will occur once the wild type restriction over the pairing of homeologs has been restored (Sears, 1976).
The potential for inadequate genetic complementation becomes increasingly likely where the donor species is only distantly related to the recipient. In this case, the donor and recipient species have been isolated from one another over such a long period that they will have diverged substantially at the genetic level. As a result, the gene content of the introgressed exotic segment and the wheat segment which has been replaced may not be the same, leading to a progeny in which the introgressed genes cannot fully complement those present on the replaced wheat chromosome segment. This situation can lead to deleterious effects on the plant’s agronomic performance (Birchler and Veitia, 2012). While genetically unbalanced genotypes can be informative for the purpose of genetic analysis, they are seldom of value in the context of varietal improvement.
The genetic background in which an exotic transfer has been engineered can also discourage the breeder take-up of introgression materials. Much of the research effort in bread wheat has been focused on the Sichuan province landrace Chinese Spring (CS), because of its choice for the development of the aneuploid and mutant stocks required for chromosome engineering (Sears and Miller, 1985). This choice has had some unfortunate consequences, since the phenotype of CS is deficient with respect to several key quantitative traits, and has proven difficult to correct (Liu et al., 2018). Nevertheless, a substantial body of germplasm harboring introgression products has been developed by various programs, although so far their impact on wheat improvement has been minimal, given that breeders are unwilling to break up the constellation of favorable alleles which they so laboriously assembled through many years of crossing and selection. Some recent advances in introgression methodology and the development of genomic resources have the potential to overcome some of the problems associated with the exploitation of introgression materials.
Unpaired meiotic chromosomes (univalents) have a tendency to spontaneously break at their centromere during anaphase I to form two fragments. This process can give rise to a Robertsonian translocation (RobT) where two different chromosomes simultaneously break in the same cell, since chromosomal fragments readily fuse with one another (Robertson, 1916; Sears, 1952). Balancing or compensating RobTs (cRobTs), in which a chromosome arm becomes fused to the opposite arm of its homeolog, represent a key intermediate in the process of inducing introgression in the situation where homologous recombination is not feasible. A small number of cRobTs have indeed had a considerable impact on wheat improvement; the most notable examples are the wheat–rye translocation 1BL.1RS, in which the short arm of wheat chromosome 1B has been replaced by the short arm of rye chromosome 1R (Mettin et al., 1973; Zeller, 1973), and the 6AL.6VS translocation, in which the long arm of wheat chromosome 6A has become fused to the short arm of Dasypyrum villosum chromosome 6V. The former cRobT was ubiquitous among high yielding CIMMYT and European wheats during the closing years of the 20th century, while the latter one, which carries a gene determining resistance to the foliar pathogen Blumeria graminis (powdery mildew), is present in many current Chinese cultivars (Cao et al., 2011).
Constructing a wheat plant in which two chromosomes are present as monosomics is a relatively straight-forward procedure given the range of cytogenetic stocks which have been assembled (Davies et al., 1985; Lukaszewski, 1993, 1997; Friebe et al., 2005). A list of some successful inductions is presented in Table 1. The principle of the double monosomic method is based on the certainty that, thanks to the suppression of homeolog pairing, the two monosomes will remain unpaired at meiotic metaphase I (giving rise to a meiotic constitution of 20′′ + 2′); selfed progeny of such a plant are subsequently screened, using a combination of genetic markers and karyotyping, to detect de novo cRobT products. Both marker and karyotyping technology have benefited from progress in DNA analysis, the former taking the form of DNA-based markers such as microsatellites and single nucleotide polymorphisms (SNPs), and the latter exploiting either genomic or fluorescence in situ hybridization (GISH and FISH). Double monosomics are most easily generated by crossing a whole chromosome substitution with a euploid plant (Figure 1): for instance, Ardalani et al. (2016) crossed a 6Eb(6D) substitution with the cultivar “Roushan,” and among 80 F2 segregants of the resulting 20′′ + 6D′ + 6Eb′ plant, it was possible to identify, using a PCR-based screen, a plant carrying a 6DL.6EbS cRobT. Similarly, Hao et al. (2018) only needed to screen 69 segregants to uncover a 6AL.6RS cRobT. A second approach begins with a cross between an established monosomic line (20′′ + 1′) and an addition line (21′′ + 1′′), as exemplified by Danilova et al. (2018); in this case, each of the three stocks monosomic for a group 7 homeolog (20′′ + 7A′, 20′′ + 7B′ and 20′′ + 7D′) were crossed with a line carrying a disomic dose of barley chromosome 7H (21′′ + 7H′′); selection among the resulting F1s was then made for plants having a somatic chromosome number of 42, which were expected to be of meiotic constitution 20′′ + 7A/B/D′ + 7H′. A marker-based screen of the progeny of these selections was successful in identifying all six potential cRobTs involving each arm of the barley chromosome and its opposite wheat arm, and these were confirmed using GISH analysis.
Figure 1. The double monosomic method use to induce cRobTs. A euploid cultivar is crossed with a whole chromosome substitution line in which an exotic chromosome has replaced its wheat homeolog. The resulting F1 hybrid carries two chromosomes which remain as univalents at meiosis metaphase I, giving the opportunity for a joint breakage/fusion event at anaphase I to create a cRobT.
While Sears (1956) showed that it was possible to transfer a segment of an Ae. umbellulata chromosome carrying a gene conditioning leaf rust resistance into wheat by irradiation with X-rays, chromosome engineering was greatly accelerated following the discovery that the suppression of meiotic pairing between wheat homeologs is under relatively simple genetic control. The most important locus is Ph1, mapping to chromosome arm 5BL (Okamoto, 1957; Riley and Chapman, 1958), and now known to have formed as a result of the insertion of a segment of subtelomeric heterochromatin within a cluster of cdc2-related genes (Griffiths et al., 2006). Deleting the Ph2 locus (mapping to chromosome arm 3DS) has an intermediate effect on pairing suppression (Mello-Sampayo, 1971; Sutton et al., 2003), while a large effect locus has recently been mapped to chromosome arm 3AL by Fan et al. (2019). A number of minor effect loci were identified by Sears (1976). Importantly for chromosome engineering technology, the suppression of homoelog pairing imposed by both Ph1 and Ph2 extends beyond wheat itself, and thus can be exploited to induce recombination between a number of wheat chromosomes and their homelogs from related species (Riley et al., 1968; Koebner and Shepherd, 1985, 1986, 1987; Ceoloni et al., 1992).
Since large translocations are prone to be deleterious because of linkage drag and/or inadequate genetic complementation, reducing their size, while maintaining the presence of the gene(s) targeted for introgression, is a desirable goal (Moore et al., 1993). Given that the success rate in inducing introgressions is quite low, and that conventional cytological methods are not capable of distinguishing between large and small introgression segments, pioneering attempts to achieve this goal were largely unsuccessful. Recent advances with respect to both chromosome manipulation and particularly in genetic marker technology, however, are changing this picture (Winfield et al., 2016; King et al., 2017, 2019; Koo et al., 2017). A list of successful examples is presented in Table 2. In a recent case example, an attempt to shorten the 6AL.6VS translocation has been described by Lukaszewski and Cowger (2017). The target gene on the Ha. villosa chromosome arm is Pm21, which protects against infection by B. graminis (Cao et al., 2011). To reduce the length of 6VS chromatin present, the cRobT was introduced into a background deficient for Ph1 to generate plants of constitution 19′′ + 5B′′ (ph1b) + 6AL.6VS′ + 6A′. A marker-based screen of 997 progeny allowed for the identification of 29 new translocations involving combinations of segments from chromosome arms 6AS and 6VS. The critical step was then to cross a plant containing a translocation comprising the terminal segment of 6VS (including Pm21) with one (also including Pm21) which carried a translocation comprising the proximal end of 6VS; since the two translocations shared a small fragment 6VS, it was then possible to exploit homologous recombination to generate a chromosome harboring a small interstitial segment of 6VS (including Pm21) inserted into the sub-terminal region of 6AS (Figure 2).
Figure 2. The introgression of a small fragment of Ha. villosa chromosome 6V into a distal site of wheat chromosome arm 6AS. Two independent introgression lines were inter-crossed: one carries the terminal portion of chromosome arm 6VS and the other a proximal segment, while both carry Pm21, which lies in the short common interstitial segment. As a result of a homologous recombination event in the F1 hybrid, segregants are generated which carry only the short interstitial segment [Figure redrawn from Lukaszewski and Cowger (2017)].
Most of the introgression experiments reported in the literature have sought to transfer a single gene trait, most prominently resistance to disease. Most breeders’ traits – notably grain yield – are, however, under polygenic control. The “omnigenic model” has been developed as a means of accounting for the observation that despite the inheritance of certain human diseases being due to the effect of many genomically dispersed genes, some of these genes encode products having no obvious connection to the expression of the disease (Boyle et al., 2017). The model implies that gene regulatory networks are sufficiently interconnected with one another such that all genes expressed in disease-relevant cells are liable to affect the functions of core disease-related genes and that most of the heritability can be explained by effects on genes outside core pathways. An equivalent situation probably exists with respect to the genetic determination of yield, which is clearly complex given the large number of quantitative trait loci which have been identified as contributing to the trait. A consequence of the hexaploid status of bread wheat is that interactions between homeologous genes are likely an important component of the overall genetic determination of yield-related traits (Flood et al., 1992; Yang et al., 2017). A further consequence is that the triplication of many genes in bread wheat, arising from the presence of three homeologs, provides a buffering effect, such that allelic variation at one of the copies may result in just a minor phenotypic effect (Dubcovsky and Dvorak, 2007). Thus, although variation at a single locus may well have an insubstantial effect on grain yield, combining a number of novel introgression segments into a single genotype has the potential to generate a significant positive effect. This possibility has been validated by the experience with SHWs, pursued originally by CIMMYT in the last decade of the 20th century, and later much expanded, both at CIMMYT and elsewhere. It has become clear that introgressing multiple segments from an SHW parent can significantly enhance the yield potential of wheat (Hoisington et al., 1999; Coghlan, 2006; Warburton et al., 2006; Dreisigacker et al., 2008; Trethowan and Mujeeb-Kazi, 2008; Yang et al., 2009; Li et al., 2018; Hao et al., 2019; Zhang et al., 2019a). The Chinese cultivar Chuanmai 42, for example, which has as one of its parents the CIMMYT SHW Syn769 (T. durum cv. Decoy 1/Ae. tauschii 188), recorded a yield of more than 6 t/ha in Sichuan regional trials, out-performing the commercial check variety by 20% over two consecutive years (Yang et al., 2009). It has been estimated that farmers using this cultivar would gain an extra 0.5–0.8 t/ha of grain (Li et al., 2014b). The recently released cultivars Shumai 580, Shumai 969, and Shumai 830 (Hao et al., 2019) were all bred from an SHW derived from the cross T. turgidum AS2255/Ae. tauschii AS60. Shumai 580 out-performed the best local check cultivar by 56% (equivalent to ∼2 t/ha) in a yield trial in Yunnan province, while Shumai 969 has remained the highest yielding cultivar in Sichuan trials over the past decade. The yield per spike achieved in Sichuan province by Shumai 830 has been consistently higher than any other commercial cultivar’s.
A characterization of the introgressions carried by the three Shumai cultivars, as assessed at the sequence level by Hao et al. (2019), has shown that the ratio of the SHW parent’s DNA retained lay in the range 12.4–15.0%. This outcome coincides well with the predicted ratio of 12.5% based on the assumption that the breeding strategy employed did not discriminate against SHW alleles (except for those responsible for visible deleterious traits such as shattering, lateness and tallness). The genomes of the three Shumai cultivars featured a unique set of introgressions from SHW-L1: alleles from the B genome of the SHW genome were prominent in Shumai 580, those from the D genome in Shumai 969 and those from the A genome in Shumai 830. Although SHWs themselves tend to be poor agronomic performers, an analysis of a recombinant inbred population formed from a cross between SHW-L1 and an elite cultivar has shown that nearly a half (40/86) of the positive alleles at loci affecting yield were inherited from the SHW parent (Hao et al., 2019). Similar phenomenon has also been observed in other plant systems such as tomato (de Vicente and Tanksley, 1993). In addition to these quantitative trait loci, the Shumai cultivars inherited SHW alleles at Ppd-A1, Vrn-A1, Vrn-B1, TaTEF, GPC-2, TaGASR7, TaGW2, TaCKX6, and TaSus1, all of which are genes having a known impact on productivity (Nadolska-Orczyk et al., 2017). A similar representation of SHW alleles in materials selected from advanced backcross populations has been reported by Huang et al. (2003, 2004). The take home message is that while SHWs in themselves cannot compete with elite cultivars, they do represent a valuable source of beneficial alleles, so there is every reason to include them in bread wheat improvement programs.
In addition, SHW is different from bread wheat since its newly synthetic process may introduce transcriptome shock (Li et al., 2014a, 2015). Some marked differences have been noted between the transcriptomes of SHWs and conventional cultivars (Hao et al., 2017; Ramírez-González et al., 2018), resulting in the proposition that aspects of regulatory control differ (Li et al., 2015). Thus, besides the clear contribution of DNA sequence polymorphism, SHWs probably also deliver variation at the RNA level.
The successful use of unadapted germplasm requires the introduction of genes determining the target trait(s) and selection against those determining deleterious traits. It has been conventionally held for a long time that using unadapted germplasm as a parent in a breeding program is inherently risky, since the size of breeding population required to breed these deleterious traits out may be uneconomically large. The indications are, however, that the efficiency of introgression breeding can be improved by adopting more focused strategies for both crossing and selection. The advanced backcross quantitative trait locus approach proposed by Tanksley and Nelson (1996) provides a method to retain favorable alleles inherited from the exotic donor parent while returning the background to that of the elite recipient. The concept has been applied to introgression programs in tomato (Tanksley et al., 1996), rice (Xiao et al., 1998), and bread wheat (Huang et al., 2003, 2004; Liu et al., 2006). However, in commercial practice, it has become evident that the necessity for extensive backcrossing has been exaggerated. Of 46 SHW-based cultivars released in 15 countries in the period 2003–2017, only four have been based on backcrossing to an elite cultivar (Li et al., 2018). Rather, the experience has been that top-crossing is a more effective approach, perhaps because of the unlikelihood that any single elite cultivar has the genetic content to neutralize the many defects present in an SHW, whereas including two or more elite parents increases the chance of success.
In the authors’ hands, an effective strategy has proven to be one based on a double top cross (DTC), followed by two phases of selection (2PS) (Hao et al., 2019; Figure 3). The scheme, summarized as SHW-L1/B//C///D (where B through D indicate three independent elite cultivars), implies that at its end the population’s predicted mean proportion of nuclear DNA inherited from SHW-L1 will be 12.5%. During the F2 and F3 generations, the population size was reduced by selecting against tough glumes, late maturity, tall stature and yellow rust susceptibility, while selection from the F4 onward was directed at yield. The program has already led to the release of three cultivars: Shumai 580 and Shumai 969 both emerged from rather small populations (∼100–200 F2 and ∼100–200 F3 plants), whereas the selection of Shumai 830 required a more conventional population size, because one of its parental lines was a less well established cultivar. A fourth release (Shumai 114) is imminent. Overall, the indication is that the DTC-2PS strategy can be highly effective for introgressing material from an SHW.
Figure 3. The double top-cross (DTC) and two phase selection (2PS) strategies deployed to manage introgression into elite germplasm from an SHW. The DTC populations retain on average 12.5% of the nuclear genome of the SHW parent. The aim of the first selection phase, carried out in the F2 and F3 generations, is to eliminate serious agronomic defects, while that of the second phase (applied from the F4 generation onward) is to improve yield. Shumai 969 and Shumai 830 were both bred using this strategy.
The effectiveness of the DTC-2PS strategy relies on a number of factors. Firstly, it assumes that the SHW donor harbors plentiful genetic variation, allowing introgression to potentially contribute to the improvement of extant traits or even the creation of novel ones. It has been suggested that inheritance of the rapid grain filling trait exhibited by SHW-L1 partially underlies the improved yield potential of the three Shumai cultivars (Hao et al., 2019). Secondly, the DTC scheme combines allelic variation from more than one elite cultivar, providing a wider pool of alleles for generating novel combinations: each of the elite cultivars has its specific constellation of favorable alleles available to interact with the donor’s genome. Thirdly, the early generations are used to select for major genes controlling the important domestication traits, leaving behind a much smaller population for the breeder to handle the issue of yield advance.
As demonstrated by Li et al. (2020), the DTC-2PS strategy has also been successfully deployed to improve the genetic background of a line harboring a 6AL.6RS wheat/rye translocation, valued for the presence of Pm56 (Hao et al., 2018). Two genetic backgrounds provided the starting material: one was CS and the other the Sichuan province landrace Kaixian-luohanmai. The strategy is quite general, so could readily be used to widen the genetic base of any crop species. It is of particular relevance to allopolyploid species where the progenitor parents are known (for example in cotton, 4x Brassica spp. and groundnut), since the domesticate has become genetically isolated from the wild progenitor species and its genetic base has been narrowed as a result of domestication and subsequent breeding (Stebbins, 1950; Buckler et al., 2001). The expectation is that introgression from exotic germplasm will feature strongly in future crop improvement programs.
MH and DL contributed to the conception and design of the study and wrote the first draft of the manuscript. All authors contributed to the manuscript revision, read, and approved the submitted version.
This work was financially supported by the National Natural Science Foundation of China (91935303, 31671689, and 31671682), the Chinese Government National Key Research and Development Program (2016YFD0102000), the Sichuan Provincial Agricultural Department Innovative Research Team (wheat-10), and the Sichuan Province Science and Technology Department Crops Breeding Project (2016NYZ0030).
The authors declare that the research was conducted in the absence of any commercial or financial relationships that could be construed as a potential conflict of interest.
Anderson, E., and Hubricht, L. (1938). Hybridization in Tradescantia. III. The evidence for introgressive hybridization. Am. J. Bot. 25, 396–402. doi: 10.1002/j.1537-2197.1938.tb09237.x
Anugrahwati, D. R. (2009). Isolation of new 1DS/1RS wheat–rye recombinations. J. Crop Agro. 2, 60–69. doi: 10.1007/BF00226788
Ardalani, S., Mirzaghaderi, G., and Badakhshan, H. (2016). A robertsonian translocation from Thinopyrum bessarabicum into bread wheat confers high iron and zinc contents. Plant Breed. 135, 286–290. doi: 10.1111/pbr.12359
Ayala-Navarrete, L., Bariana, H. S., Singh, R. P., Gibson, J. M., Mechanicos, A. A., and Larkin, P. J. (2007). Trigenomic chromosomes by recombination of Thinopyrum intermedium and Th. ponticum translocations in wheat. Theor. Appl. Genet. 116, 63–75. doi: 10.1007/s00122-007-0647-5
Birchler, J. A., and Veitia, R. A. (2012). Gene balance hypothesis: connecting issues of dosage sensitivity across biological disciplines. Proc. Natl Acad Sci. U.S.A. 109, 14746–14753. doi: 10.1073/pnas.1207726109
Borlaug, N. E. (1983). Contributions of conventional plant breeding to food production. Science 219, 689–693. doi: 10.1126/science.219.4585.689
Boyle, E. A., Li, Y. I., and Pritchard, J. K. (2017). An expanded view of complex traits: from polygenic to omnigenic. Cell 169, 1177–1186. doi: 10.1016/j.cell.2017.05.038
Buckler, E., Thornsberry, J., and Kresovich, S. (2001). Molecular diversity, structure and domestication of grasses. Genet. Res. 77, 213–218.
Cainong, J. C., Bockus, W. W., Feng, Y., Chen, P., Qi, L., Sehgal, S. K., et al. (2015). Chromosome engineering, mapping, and transferring of resistance to Fusarium head blight disease from Elymus tsukushiensis into wheat. Theor. Appl. Genet. 128, 1019–1027. doi: 10.1007/s00122-015-2485-1
Cao, A., Xing, L., Wang, X., Yang, X., Wang, W., Sun, Y., et al. (2011). Serine/threonine kinase gene Stpk-V, a key member of powdery mildew resistance gene Pm21, confers powdery mildew resistance in wheat. Proc. Natl. Acad. Sci. U.S.A. 108, 7727–7732. doi: 10.1073/pnas.1016981108
Ceoloni, C., Signore, G. D., Ercoli, L., and Donini, P. (1992). Locating the alien chromatin segment in common wheat-Aegilops longissima mildew resistant transfers. Hereditas 116, 239–245. doi: 10.1111/j.1601-5223.1992.tb00148.x
Dai, K., Zhao, R., Shi, M., Xiao, J., Yu, Z., Jia, Q., et al. (2019). Dissection and cytological mapping of chromosome arm 4VS by the development of wheat-Haynaldia villosa structural aberration library. Theor. Appl. Genet. 133, 217–226. doi: 10.1007/s00122-019-03452-8
Danilova, T. V., Friebe, B., Gill, B. S., Poland, J., and Jackson, E. (2018). Development of a complete set of wheat–barley group-7 Robertsonian translocation chromosomes conferring an increased content of β-glucan. Theor. Appl. Genet. 131, 377–388. doi: 10.1007/s00122-017-3008-z
Danilova, T. V., Poland, J., and Friebe, B. (2019). Production of a complete set of wheat–barley group-7 chromosome recombinants with increased grain β-glucan content. Theor. Appl. Genet. 132, 3129–3141. doi: 10.1007/s00122-019-03411-3
Danilova, T. V., Zhang, G., Liu, W., Friebe, B., and Gill, B. S. (2017). Homoeologous recombination-based transfer and molecular cytogenetic mapping of a wheat streak mosaic virus and Triticum mosaic virus resistance gene Wsm3 from Thinopyrum intermedium to wheat. Theor. Appl. Genet. 130, 549–556. doi: 10.1007/s00122-016-2834-8
Davies, P. A., Pallotta, M. A., and Driscoll, C. J. (1985). Centric fusion between nonhomologous rye chromosomes in wheat. Can. J. Genet.Cytol. 27, 627–632. doi: 10.1139/g85-094
de Vicente, M. C., and Tanksley, S. D. (1993). QTL analysis of transgressive segregation in an interspecific tomato cross. Genetics 134, 585–596.
Dreisigacker, S., Kishii, M., Lage, J., and Warburton, M. (2008). Use of synthetic hexaploid wheat to increase diversity for CIMMYT bread wheat improvement. Aust. J. Agric. Res. 59, 413–420.
Dubcovsky, J., and Dvorak, J. (2007). Genome plasticity a key factor in the success of polyploid wheat under domestication. Science 316, 1862–1866. doi: 10.1126/science.1143986
Dubcovsky, J., Lukaszewski, A. J., Echaide, M., Antonelli, E. F., and Porter, D. R. (1998). Molecular characterization of two Triticum speltoides interstitial translocations carrying leaf rust and greenbug resistance genes. Crop Sci. 38, 1655–1660. doi: 10.2135/cropsci1998.0011183X003800060040x
Dvorak, J., Akhunov, E. D., Akhunov, A. R., Deal, K. R., and Luo, M. C. (2006). Molecular characterization of a diagnostic DNA marker for domesticated tetraploid wheat provides evidence for gene flow from wild tetraploid wheat to hexaploid wheat. Mol. Biol. Evol. 23, 1386–1396. doi: 10.1093/molbev/msl004
Fan, C., Luo, J., Zhang, S., Liu, M., Li, Q., Li, Y., et al. (2019). Genetic mapping of a major QTL promoting homoeologous chromosome pairing in a wheat landrace. Theor. Appl. Genet. 132, 2155–2166. doi: 10.1007/s00122-019-03344-x
Feuillet, C., Langridge, P., and Waugh, R. (2008). Cereal breeding takes a walk on the wild side. Trends Genet. 24, 24–32. doi: 10.1016/j.tig.2007.11.001
Flood, R. G., Lagudah, E. S., and Halloran, G. M. (1992). Expression of vernalization requirement and spikelet number in synthetic hexaploid wheats and their Triticum tauschii and tetraploid wheat parents. Ann. Bot. 69, 213–217. doi: 10.1093/oxfordjournals.aob.a088332
Friebe, B., Jiang, J., Raupp, W. J., McIntosh, R. A., and Gill, B. S. (1996). Characterization of wheat-alien translocations conferring resistance to diseases and pests: current status. Euphytica 91, 59–87. doi: 10.1007/bf00035277
Friebe, B., Zhang, P., Linc, G., and Gill, B. S. (2005). Robertsonian translocations in wheat arise by centric misdivision of univalents at anaphase I and rejoining of broken centromeres during interkinesis of meiosis II. Cytogenet. Genome Res. 109, 293–297. doi: 10.1159/000082412
Griffiths, S., Sharp, R., Foote, T. N., Bertin, I., Wanous, M., Reader, S., et al. (2006). Molecular characterization of Ph1 as a major chromosome pairing locus in polyploid wheat. Nature 439, 749–752. doi: 10.1038/nature04434
Hao, M., Li, A., Shi, T., Luo, J., Zhang, L., Zhang, X., et al. (2017). The abundance of homoeologue transcripts is disrupted by hybridization and is partially restored by genome doubling in synthetic hexaploid wheat. BMC Genomics 18:149. doi: 10.1186/s12864-017-3558-0
Hao, M., Liu, M., Luo, J., Fan, C., Yi, Y., Zhang, L., et al. (2018). Introgression of powdery mildew resistance gene Pm56 on rye chromosome arm 6RS into wheat. Front. Plant Sci. 9:1040. doi: 10.3389/fpls.2018.01040
Hao, M., Luo, J., Zeng, D., Zhang, L., Ning, S., Yuan, Z., et al. (2014). QTug. sau-3B is a major quantitative trait locus for wheat hexaploidization. G3: Genes Genom. Genet 4, 1943–1953. doi: 10.1534/g3.114.013078
Hao, M., Zhang, L., Zhao, L., Dai, S., Li, A., Yang, W., et al. (2019). A breeding strategy targeting the secondary gene pool of bread wheat: introgression from a synthetic hexaploid wheat. Theor. Appl. Genet. 132, 2285–2294. doi: 10.1007/s00122-019-03354-9
Harlan, J. R., and de Wet, J. M. J. (1971). Towards a rational classification of cultivated plants. Taxon 20, 509–517. doi: 10.2307/1218252
Hoisington, D., Khairallah, M., Reeves, T., Ribaut, J. M., Skovmand, B., Taba, S., et al. (1999). Plant genetic resources: what can they contribute toward increased crop productivity? Proc. Natl. Acad. Sci. U.S.A. 96, 5937–5943. doi: 10.1073/pnas.96.11.5937
Huang, X. Q., Coster, H., Ganal, M. W., and Roder, M. S. (2003). Advanced backcross QTL analysis for the identification of quantitative trait loci alleles from wild relatives of wheat (Triticum aestivum L.). Theor. Appl. Genet. 106, 1379–1389. doi: 10.1007/s00122-002-1179-7
Huang, X. Q., Kempf, H., Ganal, M. W., and Roder, M. S. (2004). Advanced backcross QTL analysis in progenies derived from a cross between a German elite winter wheat variety and a synthetic wheat (Triticum aestivum L.). Theor. Appl. Genet. 109, 933–943. doi: 10.1007/s00122-004-1708-7
Jiang, J., Friebe, B., and Gill, B. S. (1993). Recent advances in alien gene transfer in wheat. Euphytica 73, 199–212. doi: 10.1038/hdy.2012.116
King, J., Grewal, S., Yang, C., Hubbart, S., Scholefield, D., Ashling, S., et al. (2017). A step change in the transfer of interspecific variation into wheat from Amblyopyrum muticum. Plant Biot. J. 15, 217–226. doi: 10.1111/pbi.12606
King, J., Newell, C., Grewal, S., Hubbart-Edwards, S., Yang, C., Scholefield, D., et al. (2019). Development of stable homozygous wheat/Amblyopyrum muticum (Aegilops mutica) introgression lines and their cytogenetic and molecular characterization. Front. Plant Sci. 10:34. doi: 10.3389/fpls.2019.00034
Koebner, R. M. D., and Shepherd, K. W. (1985). Induction of recombination between rye chromosome 1RL and wheat chromosomes. Theor. Appl. Genet. 71, 208–215. doi: 10.1007/BF00252057
Koebner, R. M. D., and Shepherd, K. W. (1986). Controlled introgression to wheat of genes from rye chromosome arm 1RS by induction of allosyndesis. Theor. Appl. Genet. 73, 197–208. doi: 10.1007/BF00289275
Koebner, R. M. D., and Shepherd, K. W. (1987). Allosyndetic recombination between a chromosome of Aegilops umbellulata and wheat chromosomes. Heredity 59, 33–45. doi: 10.1038/hdy.1987.94
Kole, C. (2011). Wild crop Relatives: Genomic and Breeding Resources. Cereals. Berlin: Springer-Verlag.
Koo, D. H., Liu, W., Friebe, B., and Gill, B. S. (2017). Homoeologous recombination in the presence of Ph1 gene in wheat. Chromosoma 126, 531–540. doi: 10.1007/s00412-016-0622-5
Kumar, N., and Rustgi, S. (2014). “Agronomically relevant traits transferred to major crop plants by alien introgressions,” in Alien Gene Transfer in Crop Plants, Vol. 1, eds A. Pratap and J. Kumar (New York, NY: Springer), 211–245. doi: 10.1007/978-1-4614-8585-8_9
Li, A., Liu, D., Wu, J., Zhao, X., Hao, M., Geng, S., et al. (2014a). mRNA and small RNA transcriptomes reveal insights into dynamic homoeolog regulation of allopolyploid heterosis in nascent hexaploid wheat. Plant Cell 26, 1878–1900. doi: 10.1105/tpc.114.124388
Li, A., Liu, D., Yang, W., Kishii, M., and Mao, L. (2018). Synthetic hexaploid wheat: yesterday, today, and tomorrow. Engineering 4, 552–558. doi: 10.1016/j.eng.2018.07.001
Li, A. L., Geng, S. F., Zhang, L. Q., Liu, D. C., and Mao, L. (2015). Making the bread: insights from newly synthesized allohexaploid wheat. Mol. Plant 8, 847–859. doi: 10.1016/j.molp.2015.02.016
Li, H., Dong, Z., Ma, C., Tian, X., Qi, Z., Wu, N., et al. (2019). Physical mapping of stem rust resistance gene Sr52 from Dasypyrum villosum based on ph1b-Induced homoeologous recombination. Int. J. Mol. Sci. 20:4887. doi: 10.3390/ijms20194887
Li, J., Wan, H. S., and Yang, W. Y. (2014b). Synthetic hexaploid wheat enhances variation and adaptive evolution of bread wheat in breeding processes. J. Syst. Evol. 52, 735–742. doi: 10.1111/jse.12110
Li, Q. C., Huang, L., Li, Y. Z., Fan, C. L., Xie, D., Zhao, L. B., et al. (2020). Genetic stability of 6RS/6AL translocation chromosome and its transmission through gametes. Acta Agronomica Sinica 46, 513–519. doi: 10.3724/SP.J.1006.2020.91051
Liu, C., Qi, L., Liu, W., Zhao, W., Wilson, J., Friebe, B., et al. (2011a). Development of a set of compensating Triticum aestivum–Dasypyrum villosum robertsonian translocation lines. Genome 54, 836–844. doi: 10.1139/g11-051
Liu, D., Zhang, H., Zhang, L., Yuan, Z., Hao, M., and Zheng, Y. (2014). “Distant hybridization: a tool for interspecific manipulation of chromosomes,” in Alien Gene Transfer in Crop Plants, Vol. 1, eds A. Pratap and J. Kumar (New York, NY: Springer), 25–42. doi: 10.1007/978-1-4614-8585-8_2
Liu, D., Zhang, L., Hao, M., Ning, S., Yuan, Z., Dai, S., et al. (2018). Wheat breeding in the hometown of Chinese Spring. Crop J. 6, 82–90. doi: 10.1016/j.cj.2017.08.009
Liu, D. C., Hao, M., Li, A. L., Zhang, L. Q., Zheng, Y. L., and Mao, L. (2017a). “Allopolyploidy and interspecific hybridization for wheat improvement,” in Polyploidy and Hybridization for Crop Improvement, ed. A. S. Mason (Cambridge: CRC Press), 27–52.
Liu, S., Zhou, R., Dong, Y., Li, P., and Jia, J. (2006). Development, utilization of introgression lines using a synthetic wheat as donor. Theor. Appl. Genet. 112, 1360–1373. doi: 10.1007/s00122-006-0238-x
Liu, W., Danilova, T. V., Rouse, M. N., Bowden, R. L., Friebe, B., Gill, B. S., et al. (2013). Development and characterization of a compensating wheat-Thinopyrum intermedium robertsonian translocation with Sr44 resistance to stem rust (Ug99). Theor. Appl. Genet. 126, 1167–1177. doi: 10.1007/s00122-013-2044-6
Liu, W., Jin, Y., Rouse, M., Friebe, B., Gill, B., and Pumphrey, M. O. (2011b). Development and characterization of wheat-Ae. searsii robertsonian translocations and a recombinant chromosome conferring resistance to stem rust. Theor. Appl. Genet. 122, 1537–1545. doi: 10.1007/s00122-011-1553-4
Liu, W., Koo, D. H., Xia, Q., Li, C., Bai, F., Song, Y., et al. (2017b). Homoeologous recombination-based transfer and molecular cytogenetic mapping of powdery mildew-resistant gene Pm57 from Aegilops searsii into wheat. Theor. Appl. Genet. 130, 841–848. doi: 10.1007/s00122-017-2855-y
Liu, W., Seifers, D. L., Qi, L. L., Friebe, B., and Gill, B. S. (2011c). A compensating wheat-Thinopyrum intermedium robertsonian translocation conferring resistance to wheat streak mosaic virus and Triticum mosaic virus. Crop Sci. 51, 2382–2390. doi: 10.1007/s00122-016-2834-8
Lukaszewski, A. J. (1993). Reconstruction in wheat of complete chromosomes 1B and 1R from the 1RS.1BL translocation of ‘Kavkaz’ origin. Genome 36, 821–824. doi: 10.1139/g93-109
Lukaszewski, A. J. (1997). Further manipulation by centric misdivision of the 1RS.1BL translocation in wheat. Euphytica 94, 257–261.
Lukaszewski, A. J. (2000). Manipulation of the 1RS.1BL translocation in wheat by induced homoeologous recombination. Crop Sci. 40, 216–225. doi: 10.2135/cropsci2000.401216x
Lukaszewski, A. J., and Cowger, C. (2017). Re-Engineering of the Pm21 transfer from Haynaldia villosa to bread wheat by induced homoeologous recombination. Crop Sci. 57, 2590–2594. doi: 10.2135/cropsci2017.03.0192
Lukaszewski, A. J., Rybka, K., Korzun, V., Malyshev, S. V., Lapinski, B., and Whitkus, R. (2004). Genetic and physical mapping of homoeologous recombination points involving wheat chromosome 2B and rye chromosome 2R. Genome 47, 36–45. doi: 10.1139/g03-089
Marais, G. F., Badenhorst, P. E., Eksteen, A., and Pretorius, Z. A. (2010). Reduction of Aegilops sharonensis chromatin associated with resistance genes Lr56 and Yr38 in wheat. Euphytica 171, 15–22. doi: 10.1007/s10681-009-9973-9
Mello-Sampayo, T. (1971). Genetic regulation of meiotic chromosome pairing by chromosome 3D of Triticum aestivum. Nat. New Biol. 230, 22–23. doi: 10.1038/newbio230022a0
Mettin, D., Bluthner, W. D., and Schlegel, G. (1973). “Additional evidence of spontaneous 1B/1R wheat-rye substitutions and translocations,” in Proceedings of the 4th International Wheat Genetetics Symposium (Columbia, MO: Agricultural Experiment Station), 179–184.
Moore, G., Abbo, S., Cheung, W., Foote, T., Gale, M., Koebner, R., et al. (1993). Key features of cereal genome organization as revealed by the use of cytosine methylation-sensitive restriction endonucleases. Genomics 15, 472–482. doi: 10.1006/geno.1993.1097
Mujeeb-Kazi, A., Rosas, V., and Roldan, S. (1996). Conservation of the genetic variation of Triticum tauschii (Coss.) Schmalh. (Aegilops squarrosa auct. non L.) in synthetic hexaploid wheats (T. turgidum L. s. lat. × T. tauschii; 2n = 6x = 42, AABBDD) and its potential utilization for wheat improvement. Genet. Resour. Crop Evolut. 43, 129–134. doi: 10.1007/bf00126756
Nadolska-Orczyk, A., Rajchel, I. K., Orczyk, W., and Gasparis, S. (2017). Major genes determining yield-related traits in wheat and barley. Theor. Appl. Genet. 130, 1081–1098. doi: 10.1007/s00122-017-2880-x
Niu, Z., Klindworth, D. L., Friesen, T. L., Chao, S., Jin, Y., Cai, X., et al. (2011). Targeted introgression of a wheat stem rust resistance gene by DNA marker-assisted chromosome engineering. Genetics 187, 1011–1021. doi: 10.1534/genetics.110.123588
Qi, L. L., Pumphrey, M. O., Friebe, B., Zhang, P., Qian, C., Bowden, R. L., et al. (2011). A novel Robertsonian translocation event leads to transfer of a stem rust resistance gene (Sr52) effective against race Ug99 from Dasypyrum villosum into bread wheat. Theor. Appl. Genet. 123, 159–167. doi: 10.1007/s00122-011-1574-z
Rabinovich, S. V. (1998). “Important of wheat-rye translocation for breeding modern cultivars of Triticum aestivum L,” in Wheat: Prospects for Global Improvement, eds H. J. Braun, F. Altay, W. E. Kronstad, S. P. S. Beniwal, and A. McNAB (Dordrecht: Kluwer Academic), 401–418. doi: 10.1007/978-94-011-4896-2_55
Rahmatov, M., Rouse, M. N., Nirmala, J., Danilova, T., Friebe, B., Steffenson, B. J., et al. (2016). A new 2DS.2RL Robertsonian translocation transfers stem rust resistance gene Sr59 into wheat. Theor. Appl. Genet. 129, 1383–1392. doi: 10.1007/s00122-016-2710-6
Ramírez-González, R. H., Borrill, P., Lang, D., Harrington, S. A., Brinton, J., Venturini, L., et al. (2018). The transcriptional landscape of polyploid wheat. Science 361:eaar6089. doi: 10.1126/science.aar6089
Rey, M. D., Calderón, M. C., and Prieto, P. (2015). The use of the ph1b mutant to induce recombination between the chromosomes of wheat and barley. Front. Plant Sci. 6:160. doi: 10.3389/fpls.2015.00160
Ribeiro-Carvalho, C., Guedes-Pinto, H., Igrejas, G., Stephenson, P., Schwarzacher, T., and Heslop-Harrison, J. S. (2004). High levels of genetic diversity throughout the range of the Portuguese wheat landrace ‘Barbela’. Ann. Bot. 94, 699–705. doi: 10.1093/aob/mch194
Riley, R., and Chapman, V. (1958). Genetic control of the cytologically diploid behaviour of hexaploid wheat. Nature 182, 713–715. doi: 10.1038/182713a0
Riley, R., Chapman, V., and Johnson, R. (1968). Introduction of yellow rust resistance of Aegilops comosa into wheat by genetically induced homoeologous recombination. Nature 217, 383–384. doi: 10.1038/217383a0
Robertson, W. M. R. B. (1916). Chromosome studies. I. Taxonomic relationships shown in the chromosomes of Tettegidae and Acrididiae: V-shaped chromosomes and their significance in Acrididiae, Locustidae and Grillidae: chromosomes and variation. J. Morphol. 27, 179–331.
Sanei, M., Pickering, R., Kumke, K., Nasuda, S., and Houben, A. (2011). Loss of centromeric histone H3 (CENH3) from centromeres precedes uniparental chromosome elimination in interspecific barley hybrids. Proc. Natl. Acad. Sci. U.S.A. 108, 13373–13374. doi: 10.1073/pnas.1103190108
Sears, E. R. (1952). Misdivision of univalents in common wheat. Chromosoma 4, 535–550. doi: 10.1007/bf00325789
Sears, E. R. (1956). The transfer of leaf rust resistance from Aegilops umbellulata to wheat. Brookhaven Symp. Biol. 9, 1–22.
Sears, E. R. (1972). “Chromosome engineering in wheat,” in Proceedings of the 4th Stadler Genetics Symposium (Columbia, MO: University of Missouri), 23–38.
Sears, E. R. (1976). Genetic control of chromosome pairing in wheat. Ann. Rev. Genet. 10, 31–51. doi: 10.1146/annurev.ge.10.120176.000335
Sears, E. R. (1981). “Transfer of alien genetic material to wheat,” in Wheat science-today and tomorrow, eds L. T. Evans and W. J. Peacock (Cambridge: Cambridge University Press), 75–89.
Sears, E. R., and Miller, T. E. (1985). The history of Chinese Spring wheat. Cer. Res. Commun. 13, 261–263.
Soltis, D. E., Albert, V. A., Leebens-Mack, J., Bell, C. D., Paterson, A. H., Zheng, C., et al. (2009). Polyploidy and angiosperm diversification. Am. J. Bot. 96, 336–348. doi: 10.3732/ajb.0800079
Stebbins, G. L. Jr. (1950). Variation and Evolution in Plants. New York, NY: Columbia University Press.
Suslow, T., Thomas, B., and Bradford, K. (2002). Biotechnology Provides New Tools for Plant Breeding. Oakland, CA: UCANR Publications.
Sutton, T., Whitford, R., Baumann, U., Dong, C. M., Able, J. A., and Langridge, P. (2003). The Ph2 pairing homoeologous locus of wheat (Triticum aestivum): identification of candidate meiotic genes using a comparative genetics approach. Plant J. 36, 443–456. doi: 10.1046/j.1365-313x.2003.01891.x
Tanaka, H., Nabeuchi, C., Kurogaki, M., Garg, M., Saito, M., Ishikawa, G., et al. (2017). A novel compensating wheat–Thinopyrum elongatum robertsonian translocation line with a positive effect on flour quality. Breed. Sci. 67, 509–517. doi: 10.1270/jsbbs.17058
Tanksley, S. D., Grandillo, S., Fulton, T. M., Zamir, D., Eshed, Y., Petiard, V., et al. (1996). Advanced backcross QTL analysis in a cross between an elite processing line of tomato and its wild relative L. pimpinellifolium. Theor. Appl. Genet. 92, 213–224. doi: 10.1007/BF00223378
Tanksley, S. D., and Nelson, J. C. (1996). Advanced backcross QTL analysis: a method for the simultaneous discovery and transfer of valuable QTLs from unadapted germplasm into elite breeding lines. Theor. Appl. Genet. 92, 191–203. doi: 10.1007/BF00223376
Tester, M., and Langridge, P. (2010). Breeding technologies to increase crop production in a changing world. Science 327, 818–822. doi: 10.1126/science.1183700
Trethowan, R. M., and Mujeeb-Kazi, A. (2008). A Novel germplasm resources for improving environmental stress tolerance of hexaploid wheat. Crop Sci. 48, 1255–1265. doi: 10.2135/cropsci2007.08.0477
Türkösi, E., Darko, E., Rakszegi, M., Molnár, I., Molnár-Láng, M., and Cseh, A. (2018). Development of a new 7BS.7HL winter wheat-winter barley robertsonian translocation line conferring increased salt tolerance and (1,3; 1,4)-β-D-glucan content. PLoS One 13:e0206248. doi: 10.1371/journal.pone.0206248
Wang, R. R. C., Bothmer, R. V., Dvorak, J., Fedak, G., Linde-Laursen, I., and Muramatsu, M. (1994). “Genome symbols in the Triticeae (Poaceae),” in Proceedings of the 2nd International Triticeae Symposium, eds R. R. C. Wang, K. B. Jensen, and C. Janssi (Logan, UT: Utah State University), 29–34.
Warburton, M. L., Crossa, J., Franco, J., Kazi, M., Trethowan, R., Rajaram, S., et al. (2006). Bringing wild relatives back into the family: recovering genetic diversity in CIMMYT improved wheat germplasm. Euphytica 149, 289–301. doi: 10.1007/s10681-005-9077-0
Winfield, M. O., Allen, A. M., Burridge, A. J., Barker, G. L., Benbow, H. R., Wilkinson, P. A., et al. (2016). High-density SNP genotyping array for hexaploid wheat and its secondary and tertiary gene pool. Plant Biot. J. 14, 1195–1206. doi: 10.1111/pbi.12485
Xia, G., Xiang, F., Zhou, A., Wang, H., and Chen, H. (2003). Asymmetric somatic hybridization between wheat (Triticum aestivum L.) and Agropyron elongatum (Host) Nevishi. Theor. Appl. Genet. 107, 299–305. doi: 10.1007/s00122-003-1247-7
Xiao, J., Li, J., Grandillo, S., Ahn, S. N., Yuan, L., Tanksley, S. D., et al. (1998). Identification of trait-improving quantitative trait loci alleles from a wild rice relative. Oryza Rufipogon Genet. 150, 899–909.
Yang, W., Liu, D., Li, j, Zhang, L., Wei, H., Hu, X., et al. (2009). Synthetic hexaploid wheat and its utilization for wheat genetic improvement in China. J. Genet. Genomics 36, 539–546. doi: 10.1016/s1673-8527(08)60145-9
Yang, W., Lou, X., Li, J., Pu, M., Mirbahar, A. A., Liu, D., et al. (2017). Cloning and functional analysis of MADS-box genes, TaAG-A and TaAG-B, from a wheat K-type cytoplasmic male sterile line. Front. Plant Sci. 8:1081. doi: 10.3389/fpls.2017.01081
Yen, C., Yang, J. L., and Yen, Y. (2005). Hitoshi Kihara, Askell Löve, and the modern genetic concept of the genera in the tribe Triticeae (Poaceae). Acta Phytotaxon. Sin. 43, 82–93.
Yuan, L. P. (1966). A preliminary report on male sterility in rice, Oryza sativa L. Sci. Bull. 4, 32–34.
Zeller, F. J. (1973). “1B/1R wheat-rye chromosome substitutions and translocations,” in Proceedings of the 4th International Wheat Genetics Symposium (Columbia, MO: Agricultural Experiment Station), 209–221.
Zhang, C., Huang, L., Zhang, H., Hao, Q., Lyu, B., Wang, M., et al. (2019a). An ancestral NB-LRR with duplicated 3′UTRs confers stripe rust resistance in wheat and barley. Nat. Commun. 10:4023. doi: 10.1038/s41467-019-11872-9
Zhang, Q., Li, Q., Wang, X., Wang, H., Lang, S., Wang, Y., et al. (2005). Development and characterization of a Triticum aestivum-Haynaldia villosa translocation line T4VS⋅4DL conferring resistance to wheat spindle streak mosaic virus. Euphytica 145, 317–320. doi: 10.1007/s10681-005-1743-8
Zhang, W., Cao, Y., Zhang, M., Zhu, X., Ren, S., Long, Y., et al. (2017). Meiotic homoeologous recombination-based alien gene introgression in the genomics era of wheat. Crop Sci. 57, 1189–1198. doi: 10.2135/cropsci2016.09.0819
Zhang, W., Lukaszewski, A. J., Kolmer, J., Soria, M. A., Goyal, S., and Dubcovsky, J. (2005). Molecular characterization of durum and common wheat recombinant lines carrying leaf rust resistance (Lr19) and yellow pigment (Y) genes from Lophopyrum ponticum. Theor. Appl. Genet. 111, 573–582. doi: 10.1007/s00122-005-2048-y
Zhang, W., Zhu, X., Zhang, M., Chao, S., Xu, S., and Cai, X. (2018). Meiotic homoeologous recombination-based mapping of wheat chromosome 2B and its homoeologues in Aegilops speltoides and Thinopyrum elongatum. Theor. Appl. Genet. 131, 2381–2395. doi: 10.1007/s00122-018-3160-0
Zhang, W., Zhu, X., Zhang, M., Shi, G., Liu, Z., and Cai, X. (2019b). Chromosome engineering-mediated introgression and molecular mapping of novel Aegilops speltoides-derived resistance genes for tan spot and Septoria nodorum blotch diseases in wheat. Theor. Appl. Genet. 132, 2605. doi: 10.1007/s00122-019-03374-5
Zhao, R., Wang, H., Xiao, J., Bie, T., Cheng, S., Jia, Q., et al. (2013). Induction of 4VS chromosome recombinants using the CS ph1b mutant and mapping of the wheat yellow mosaic virus resistance gene from Haynaldia villosa. Theor. Appl. Genet. 126, 2921–2930. doi: 10.1007/s00122-013-2181-y
Zhao, W., Qi, L., Gao, X., Zhang, G., Dong, J., Chen, Q., et al. (2010). Development and characterization of two new Triticum aestivum–Dasypyrum villosum robertsonian translocation lines T1DS⋅1V#3L and T1DL⋅1V#3S and their effect on grain quality. Euphytica 175, 343–350. doi: 10.1007/s10681-010-0177-0
Keywords: alien introgression, marker, synthetic wheat, Triticum aestivum, Triticeae
Citation: Hao M, Zhang L, Ning S, Huang L, Yuan Z, Wu B, Yan Z, Dai S, Jiang B, Zheng Y and Liu D (2020) The Resurgence of Introgression Breeding, as Exemplified in Wheat Improvement. Front. Plant Sci. 11:252. doi: 10.3389/fpls.2020.00252
Received: 06 January 2020; Accepted: 18 February 2020;
Published: 06 March 2020.
Edited by:
Pietro Gramazio, University of Tsukuba, JapanReviewed by:
Mark Owen Winfield, University of Bristol, United KingdomCopyright © 2020 Hao, Zhang, Ning, Huang, Yuan, Wu, Yan, Dai, Jiang, Zheng and Liu. This is an open-access article distributed under the terms of the Creative Commons Attribution License (CC BY). The use, distribution or reproduction in other forums is permitted, provided the original author(s) and the copyright owner(s) are credited and that the original publication in this journal is cited, in accordance with accepted academic practice. No use, distribution or reproduction is permitted which does not comply with these terms.
*Correspondence: Dengcai Liu, ZGNsaXU3QHNpY2F1LmVkdS5jbg==; ZGNsaXU3QHlhaG9vLmNvbQ==
Disclaimer: All claims expressed in this article are solely those of the authors and do not necessarily represent those of their affiliated organizations, or those of the publisher, the editors and the reviewers. Any product that may be evaluated in this article or claim that may be made by its manufacturer is not guaranteed or endorsed by the publisher.
Research integrity at Frontiers
Learn more about the work of our research integrity team to safeguard the quality of each article we publish.