- Department of Biomolecular Engineering, Graduate School of Engineering, Tohoku University, Sendai, Japan
Essential elements taken up from the soil and distributed throughout the whole plant play diverse roles in different tissues. Cations and anions contribute to maintenance of intracellular osmolarity and the formation of membrane potential, while nitrate, ammonium, and sulfate are incorporated into amino acids and other organic compounds. In contrast to these ion species, calcium concentrations are usually kept low in the cytosol and calcium displays unique behavior as a cytosolic signaling molecule. Various environmental stresses stimulate increases in the cytosolic calcium concentration, leading to activation of calcium-regulated protein kinases and downstream signaling pathways. In this review, we summarize the stress responsive regulation of nutrient uptake and balancing by two types of calcium-regulated phosphorylation systems: CPK and CBL-CIPK. CPK is a family of protein kinases activated by calcium. CBL is a group of calcium sensor proteins that interact with CIPK kinases, which phosphorylate their downstream targets. In Arabidopsis, quite a few ion transport systems are regulated by CPKs or CBL-CIPK complexes, including channels/transporters that mediate transport of potassium (KAT1, KAT2, GORK, AKT1, AKT2, HAK5, SPIK), sodium (SOS1), ammonium (AMT1;1, AMT1;2), nitrate and chloride (SLAC1, SLAH2, SLAH3, NRT1.1, NRT2.4, NRT2.5), and proton (AHA2, V-ATPase). CPKs and CBL-CIPKs also play a role in C/N nutrient response and in acquisition of magnesium and iron. This functional regulation by calcium-dependent phosphorylation systems ensures the growth of plants and enables them to acquire tolerance against various environmental stresses. Calcium serves as the key factor for the regulation of membrane transport systems.
Introduction
Plants require various ions as essential nutrients, which are taken up from the soil and distributed throughout the whole plant (Welch, 1995; Merchant, 2010; Grusak et al., 2016). Each of these nutrients, once they are transferred to their destination within plant tissues via corresponding transporters/ion channels, plays diverse and critical roles in maintaining plant growth. Potassium, nitrate, and chloride contribute to maintenance of intracellular osmolarity, enabling control of cell turgor pressure which is crucial for cell expansion, stomatal movement, and pollen tube growth (Kroeger et al., 2011; Saito and Uozumi, 2019). Nitrate, ammonium, sulfate, and phosphorus are metabolized to produce various proteins and organic compounds (Leustek and Saito, 1999; López-Arredondo et al., 2013; López-Arredondo et al., 2014). Metal ions such as iron (Balk and Schaedler, 2014), manganese (Schmidt et al., 2016; Schmidt and Husted, 2019), magnesium (Gerendás and Führs, 2013), zinc (Broadley et al., 2007) and molybdenum (Mendel, 2013) work as essential cofactors for enzyme activity.
Among these essential nutrient ions, calcium exhibits some unique behaviors. In contrast to other macronutrient ions, such as potassium, for which the cellular concentration is normally in the range of 80 to 100 mM, calcium concentrations are usually relatively low and kept around 0.1 μM in the cytosol (Bush, 1995; Walker et al., 1996; Sanders et al., 1999; Hepler, 2005). However, when plants are exposed to environmental stresses such as drought, saline soil, pathogens, wounding, or nutrient deficiency, a rapid increase of the cytosolic calcium concentration occurs, either as a result of Ca2+ import via plasma membrane ion channels or Ca2+ release from intracellular calcium stores (Steinhorst and Kudla, 2013b; Zhu, 2016; Manishankar et al., 2018; Toyota et al., 2018). This leads to activation of calcium-regulated protein kinases, initiation of downstream phosphorylation signaling, and finally, achievement of stress resistance resulting from an activation of stress responsive genes or adjustment of ion channel activity. Ca2+-regulated proteins which play a key role in this phosphorylation process can be divided into three major groups: Calcium dependent protein kinases (CPK), CPK-related protein kinases (CRK), and Calcineurin-B like proteins (CBL). CPK is a family of Ser/Thr kinases containing a calcium binding site (EF hand) in their C-terminal region. Binding of Ca2+ to the EF hand stimulates a conformational change, thus allowing autophosphorylation of the kinase (Hashimoto and Kudla, 2011; Schulz et al., 2013). There are 34 CPK members in the Arabidopsis genome, and over half of these have been functionally characterized (Kudla et al., 2010; Boudsocq and Sheen, 2013; Shi et al., 2018; Saito and Uozumi, 2019). CRKs, on the other hand, were recently shown to be able to phosphorylate Tyr residues (Nemoto et al., 2015). The function of only two of eight CRK members in Arabidopsis has been analyzed so far (Rigó et al., 2013; Baba et al., 2018). CBL differs from the other two groups with regard to CBL itself being a Ca2+ sensor protein but not a kinase. Ca2+-bound and activated CBL interacts with another group of kinases called CBL-interacting protein kinases (CIPK), thereby enhancing CIPK autophosphorylation and recruitment to their target proteins (Batistic et al., 2008; Batistič and Kudla, 2009; Mao et al., 2016). Ten members of CBL and 26 members of CIPK exist in the Arabidopsis genome, each has a unique expression and subcellular localization profile. Together they form a specific interaction network, allowing regulation of genes and ion channels in various locations (Mahajan et al., 2006; Steinhorst and Kudla, 2013b; Manik et al., 2015; Manishankar et al., 2018; Saito and Uozumi, 2019). In this review, we focus on the stress responsive regulation of nutrient uptake and balancing by CPK and CBL-CIPK.
Calcium-Dependent Import of Potassium and Anions—Regulator of Intracellular Osmolarity
Potassium (K+) is the most abundant ion in plant cells. As a soluble ion, it plays a critical role in adjusting cellular osmolarity, membrane electric potential, or intracellular pH (Almeida et al., 2017; Ragel et al., 2019). These processes are important for the regulation of cell expansion, which is a prerequisite for plant growth and stomatal movement. Other ion species that contribute to this regulation are nitrate (NO3−) and chloride (Cl−). These anions work synergistically with K+ in the regulation of guard cell turgor pressure, and ultimately the control of stomatal aperture. K+, NO3−, and Cl− fluxes across the plasma membrane of pollen tubes are also essential for its growth (Mouline et al., 2002; Wu et al., 2011; Gutermuth et al., 2013; Liu et al., 2016).
Early studies proposed a correlation between cytosolic calcium and the uptake of potassium in a variety of plant species (Hirata and Mitsui, 1965; Johansen et al., 1968; Rains and Floyd, 1970). Indeed, it has been reported that K+ deficiency induces rapid Ca2+ increase in Arabidopsis roots (Behera et al., 2016). In Arabidopsis root cells, K+ uptake from the soil and export to the xylem are orchestrated by several types of transporters. Main contributors to root K+ uptake are the Shaker-type K+ channel AKT1 and the KT/KUP/HAK type transporter HAK5 (Pyo et al., 2010; Rubio et al., 2010; Alemán et al., 2011). The activity of these two K+ transport systems depends on CBL1 (or CBL9) and CIPK23 (Xu et al., 2006; Lee et al., 2007; Ragel et al., 2015). When cytosolic Ca2+ increases, activated CBL1/9 interacts with and recruits CIPK23 to the plasma membrane, enabling it to activate AKT1 and HAK5. Another CBL member, CBL10, is capable of CIPK-independent negative regulation of AKT1 activity, suggesting a role in maintaining balance of K+ uptake (Ren et al., 2013). CIPK9, most likely paired with CBL2 or CBL3, also regulates K+ homeostasis under low K+ conditions via phosphorylation of a yet unknown target (Pandey et al., 2007a; Liu et al., 2012b; Singh et al., 2018). In addition, members of the cyclic-nucleotide gated channel family CNGC3, CNGC10, and CNGC13 (Kaplan et al., 2007; Caballero et al., 2012; Ragel et al., 2019), and the cation-proton antiporter CHX13 (Zhao et al., 2008) have also been reported to mediate K+ flux into root cell. Activity of these CNGCs might be regulated by Ca2+-activated calmodulin binding and resulting blocking of the cyclic-nucleotide binding domain (Kaplan et al., 2007; DeFalco et al., 2016; Pan et al., 2019). K+ uptake by AKT1 and HAK5 is also conserved in rice, although systems corresponding to CNGCs and CHX remain to be identified in rice (Ragel et al., 2019). Increase of the K+ concentration in root stellar cell enables drive of K+ into the xylem mediated by the Shaker-type K+ efflux channel SKOR, followed by translocation of K+ to the shoot (Liu et al., 2006; Ragel et al., 2019).
In contrast to its role connected to potassium, the role of calcium as a second messenger for the nitrate response was only recently discovered (Riveras et al., 2015). Nitrate uptake and distribution throughout the plant is mainly mediated by members of the nitrate transporter (NRT) or nitrate transporter 1/peptide transporter (NPF) family (Léran et al., 2014). In Arabidopsis roots, NRT2.1/2.2/2.4/2.5 and NPF2.3/4.6/6.3 are responsible for NO3− uptake and translocation (Taochy et al., 2015; Noguero and Lacombe, 2016; Xuan et al., 2017; Zhao et al., 2018a). Among these transporters, NPF6.3, also known as NRT1.1 or CHL1, is well studied and considered a major contributor to NO3− transport (Léran et al., 2013; Leran et al., 2015; Undurraga et al., 2017). NPF6.3 is characterized as a dual affinity bidirectional NO3− transporter. This unique transporter switches its affinity from low-affinity to high-affinity mode by dimerization, which is controlled by phosphorylation of Thr101 by CBL1/9-CIPK23 (Liu and Tsay, 2003; Ho et al., 2009; Parker and Newstead, 2014; Sun et al., 2014). Two other members of CBL-CIPK are also involved in regulation of NRT/NPF. CIPK8 plays a role in the nitrate response by influencing the expression level of several nitrate-responsive genes including NPF6.3 and NRT2.1 (Hu et al., 2009). CBL7, on the other hand, was shown to regulate the expression levels of NRT2.4 and NRT2.5 (Ma et al., 2015). Other than NRT/NPF, two homologues of the guard cell S-type anion channel SLAC1, SLAH2 and SLAH3, are also suggested to mediate xylem loading of NO3− (Maierhofer et al., 2014a; Maierhofer et al., 2014b). Activation of these two SLAHs is also dependent on CBL1/9-CIPK23, and in addition, several members of the CPK family, such as CPK21 (Maierhofer et al., 2014a; Maierhofer et al., 2014b; Yao et al., 2017). Some members of the NRT/NPF or SLAH family are capable of transporting other ion species as well. NRT1.5/NPF7.3 can mediate K+ and NO3− loading into the xylem, working synergistically with SKOR to maintain K+/NO3− balance in root and shoot (Lin et al., 2008; Drechsler et al., 2015; Li et al., 2017b). Another NPF member, NPF2.4, is responsible for Cl− loading into the xylem (Li et al., 2016). SLAH1, a silent channel subunit expressed together with SLAH3 in xylem-pole pericycle cells, mediates root to shoot Cl− translocation by forming a heteromer with SLAH3 (Cubero-Font et al., 2016; Qiu et al., 2016).
Once imported into the xylem, K+ travels long-distance from root to shoot to be exported into appropriate aerial tissues. In addition, K+ can be transported from green cells into the phloem to be returned back to the roots. Detailed mechanism of this root-shoot translocation of K+ still remains ambiguous, although transporters which affect the shoot/root ratio of K+ might contribute, such as KUP7 (Han et al., 2016) and OsHAK16 from rice (Feng et al., 2019). Likewise, the identity of the transporters responsible for the retrieval of anions from the xylem remains unclear too, albeit several transporters such as NRT1.8/NPF7.2 (Li et al., 2010; Fan et al., 2017; Zhang et al., 2018) and Cation/Chloride Cotransporters (CCCs) (Li et al., 2017a) have been suggested.
Regulation of Cell Expansion and Movement
One of the key roles of K+, NO3−, and Cl− in aerial parts of plants is regulation of stomatal aperture. Stomatal movement occurs through change of osmolarity concomitantly with ion flow (mainly K+) across the guard cell membrane. A number of guard cell-expressed transporters contribute to this regulation; KAT1, KAT2, AKT1, AKT2, NPF6.3, and H+-ATPases such as AHA2 for stomatal opening (Szyroki et al., 2001; Guo et al., 2003; Saito and Uozumi, 2019), and SLAC1, SLAH3, GORK, and ALMT12 for stomatal closing (Hosy et al., 2003; Vahisalu et al., 2008; Meyer et al., 2010; Geiger et al., 2011; Saito and Uozumi, 2019). Stomata, being the site of water loss via transpiration and entrance of pathogens, are regulated by specific signal transduction pathways that ensure rapid closure in response to drought or pathogen attack. This signaling is mediated by an increase in guard cell cytosolic Ca2+ concentration and the resulting regulation of transporters by activated CPKs or CBL-CIPKs (Pandey et al., 2007b; Munemasa et al., 2015; Saito and Uozumi, 2019). Ca2+-activated CPK3, CPK6, CPK21, CPK23 (Geiger et al., 2010; Geiger et al., 2011; Scherzer et al., 2012), and CBL1/9-CIPK23 (Maierhofer et al., 2014a) are capable of eliciting anion efflux through SLAC1 and SLAH3. In addition, CBL5-CIPK11 can also activate SLAC1 (Saito et al., 2018). Following this anion efflux, K+ is driven out from guard cells via the Shaker K+ efflux channel GORK, causing turgor pressure decrease and cell shrinkage, leading to stomatal closure. Moreover, GORK itself, either directly or indirectly, is activated by CPK21 (van Kleeff et al., 2018), CPK33 (Corratgé-Faillie et al., 2017), and CBL1-CIPK5 (Förster et al., 2019). In addition, Ca2+ also triggers attenuation of stomatal opening. CIPK11 (although its interacting CBL remains undetermined) has been reported to inhibit AHA2 activity (Fuglsang et al., 2007; Yang et al., 2010), and CPK13 reduces K+ influx mediated by the Shaker K+ channels KAT1 and KAT2 (Ronzier et al., 2014). Additionally, CBL2/3 and CIPK9/17 were reported to regulate stomatal movement via control of vacuolar morphology (Song et al., 2018), possibly achieved by phosphorylation of the vacuolar localized transporters like K+/H+ antiporter NHX (Barragán et al., 2012; Andres et al., 2014), two pore K+ channel TPK1 (Gobert et al., 2007) and V-ATPase (Ratajczak, 2000; Eisenach and De Angeli, 2017). It is noteworthy that cbl2 cbl3 double mutation in Arabidopsis results in reduced activity of V-ATPase (Tang et al., 2012).
Calcium is also well recognized as a predominant regulator of pollen germination and pollen tube elongation in a wide range of plant species (Steinhorst and Kudla, 2013a; Zheng et al., 2019). Control of cell volume through Ca2+-dependent regulation of ion channels plays a crucial role in pollen tube growth. So far, CPKs and CBL-CIPKs reported to function in pollen tubes are CPK2/11/17/20/24/34, CBL1/2/3/9, and CIPK12/19 (Myers et al., 2009; Mähs et al., 2013; Zhou et al., 2015). CPK11, together with CPK24, modulates the activity of the pollen-expressed plasma membrane K+ influx channel SPIK, which is required for pollen germination (Mouline et al., 2002; Zhao et al., 2013). Pollen tubes also require an anion gradient at the tube tip, which was shown to be maintained by SLAH3 and its activator CPK2 and CPK20 (Gutermuth et al., 2013). CBL2/3-CIPK12 participate in pollen germination and tube growth by controlling vacuole morphology via regulation of a yet to be identified tonoplast protein (Steinhorst et al., 2015).
Sodium and Calcium—Resistance Against Salinity Stress
Sodium (Na+) is widely considered the major cause of salt stress damage, osmotic stress, as well as K+ deficiency due to their chemical and structural similarity (Benito et al., 2014). Uptake of Na+ is likely mediated by non-selective cation channels and considered accidental (Demidchik and Tester, 2002; Keisham et al., 2018). In order to protect leaves and reproductive tissues, plants have developed a sophisticated system for sequestering Na+ or sending it back to the soil. The salt overly sensitive (SOS) pathway, a major salt resistance mechanism, is initiated by rapid intracellular Ca2+ increase in response to salt treatment (Tester and Davenport, 2003; Köster et al., 2018), suggested to be achieved by opening of Ca2+ influx channels by Na+-activated GIPC sphingolipids (Jiang et al., 2019). In this pathway, CIPK24 (SOS2), in combination with Ca2+-activated CBL4 (SOS3), phosphorylates Na+ efflux/H+ influx antiporter SOS1 to remove Na+ from cells (Qiu et al., 2002; Shi et al., 2002). Alternatively, CBL1-CIPK24 might also mediate this process (Kolukisaoglu, 2004; Manik et al., 2015). Tonoplast localized CBL10 is another CBL required for salt tolerance, presumably by activating a Na+/H+ antiporter together with CIPK24, allowing compartmentalization of Na+ into the vacuole (Kim et al., 2007; Manik et al., 2015). Guard cell K+ efflux channel GORK is also expressed in roots. Sudden salt stress induces membrane depolarization and cytosolic Ca2+ increase in root cell, which activates GORK via a mechanism mentioned earlier in this review. Activated GORK mediates K+ efflux from root cells, which alongside with H+ efflux by AHA2, repolarizes membrane potential and restores Ca2+ homeostasis (van Kleeff et al., 2018).
Calcium Controlling Ammonium Uptake Level
Plants absorb two kinds of nitrogen species from the soil, nitrate (NO3−) and ammonium (NH4+). NO3− import and translocation by multiple NRT/NPF and SLAH transporters and regulation of these by Ca2+ were described earlier in this review. NH4+ uptake, on the other hand, is mediated by ammonium transporters (AMT). While NH4+ is beneficial as an alternative nitrogen source, high levels of NH4+ can be toxic, and therefore its cellular level must be strictly controlled (Britto and Kronzucker, 2002; Zheng et al., 2015). Two members of the AMT family, AMT1;1 and AMT1;2, were shown to be inhibited by the CBL1-CIPK23 complex, also known as an activator of root-expressed AKT1, HAK5, and SLAH2/3 (Loqué and Von Wirén, 2004; Straub et al., 2017). Thus, it is likely that CBL1-CIPK23 plays a key role in maintaining ion homeostasis in root cells and in preventing the toxic effects of NH4+ (Britto and Kronzucker, 2002; Zheng et al., 2015). Another element that controls the NO3−/NH4+ balance is the transcription factor NLP7, which was recently shown to induce up-regulation of NPF6.3 transcripts in the presence of NH4+ (Zhao et al., 2018b). NLP7 is phosphoregulated by CPK10/30/32, which are activated by NO3−-dependent elevation of intracellular Ca2+ (Liu et al., 2017).
Possible Role of Calcium in Balancing of Energy Source
Essential nutrients translocated through vascular tissues are not only limited to ions but also include organic compounds such as amino acids and sugars (Fischer et al., 1998; Liu et al., 2012a). Sugars are transported through phloem in the form of sucrose and distributed throughout the plant (Liu et al., 2012a). Loading of sucrose from the phloem to the apoplast requires activity of the shaker K+ channel AKT2 (Shabala, 2003; Dreyer et al., 2017; Ragel et al., 2019). AKT2, usually weakly-rectified, can be converted into a non-rectifying K+ channel via phosphorylation, thereby enabling K+ efflux and phloem membrane repolarization and the consequent retrieval of sucrose (Deeken et al., 2002; Michard et al., 2005a; Michard et al., 2005b; Gajdanowicz et al., 2011; Sandmann et al., 2011; Saito et al., 2017). Though the kinase responsible for this phosphorylation remains to be identified, it must be noted that AKT2 activity can be enhanced by the CBL4-CIPK6 complex in a Ca2+-dependent but phosphorylation-independent manner (Held et al., 2011).
The efficiency of cellular energy use is optimized by carbon/nitrogen (C/N) balance, and therefore its maintenance is of great significance for growth and development of plants (Coruzz and Bush, 2001; Zheng, 2009; Maekawa et al., 2014). In a recent study, three members of CIPK, CIPK7/12/14, were identified as key regulators of the C/N-nutrient response, achieved through their phosphorylation of ubiquitin ligase ATL31 (Yasuda et al., 2014; Yasuda et al., 2017).
Additionally, although most of the carbon compounds are derived from photosynthesis, plants respond to externally supplied sugars as well. These exogenous sugars, in addition to their use as energy source, show hormone-like behavior, working in parallel with some of the ABA-responsive genes (Rolland and Sheen, 2005; Yamada et al., 2011; Singh et al., 2014; Williams et al., 2014; Yuan et al., 2014). Among the calcium-regulated phosphorylation modules, CBL1 (Li et al., 2013) and CIPK14 (Yan et al., 2014) were found to positively regulate the response to glucose by an yet unidentified mechanism.
Uptake Regulation of Metal Ions and Toxins
Metal ions such as magnesium (Mg2+), iron (Fe), zinc (Zn2+), and manganese (Mn2+) work as cofactors of numerous enzymes and are therefore indispensable for plant growth. Several Ca2+-regulated phosphorylation components also participate in maintaining homeostasis of these ions. Iron deficiency was reported to elicit an increase of Ca2+ in Arabidopsis roots. This induces CBL1/9-CIPK23 to enhance Ferric chelate reductase (FRO) activity, which is required for converting Fe3+ in the soil into the transported form, Fe2+, thereby substantially regulating iron acquisition (Tian et al., 2016). CIPK23 alongside with CBL2/3 and CIPK3/9, is also required for modulation of plant growth under high Mg2+ condition, likely mediated by Mg2+ compartmentalization to the vacuole (Mogami et al., 2015; Tang et al., 2015). Additionally, Zn2+ and Mn2+ levels were found to be reduced in cipk23 mutant plants, suggesting some unidentified regulatory system of metal acquisition involving CIPK23 (Tian et al., 2016). On the other hand, some CPKs and CBL-CIPKs are involved in uptake of toxic ions. For instance, CPK31 was reported to regulate uptake of non-essential and toxic arsenite (As3+) (Ji et al., 2017), and in rice, several members of the CPK family exhibited increased phosphorylation in response to cadmium (Cd2+) application (Zhong et al., 2017).
Conclusions
In this minireview we have summarized the Ca2+-regulated uptake, storage, and translocation of nutrient ions, and possible role of Ca2+ in energy source balancing (Figure 1, Table 1). Most of these regulatory mechanisms are initiated by a rise of the cytosolic Ca2+ level in response to stress or nutrient depletion, and ultimately lead to resistance against unfavorable conditions. Thus, full understanding of the Ca2+-dependent phosphorylation machinery would be a vital step for optimizing plant growth and reproduction.
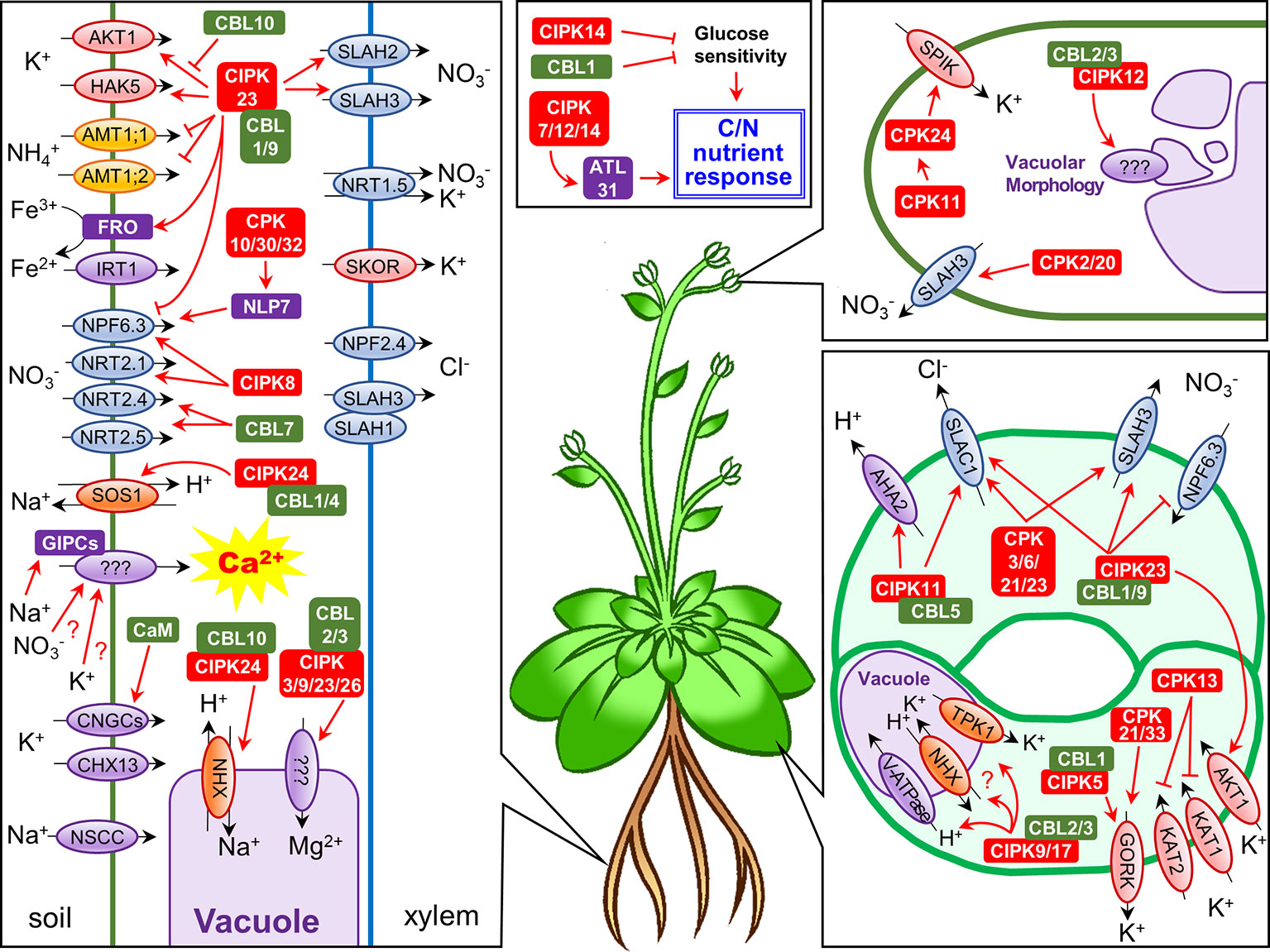
Figure 1 Schematic representation of Ca2+-regulated nutrient uptake and translocation in Arabidopsis thaliana. Each panel shows ion channel/transporter regulation by Ca2+-dependent phosphorylation systems in roots, guard cells, and pollen tubes, or the roles of CBLs/CIPKs in C/N nutrient response, respectively. Abbreviations: NSCC, non-selective cation channel; IRT1, iron transporter 1; CaM, calmodulin.
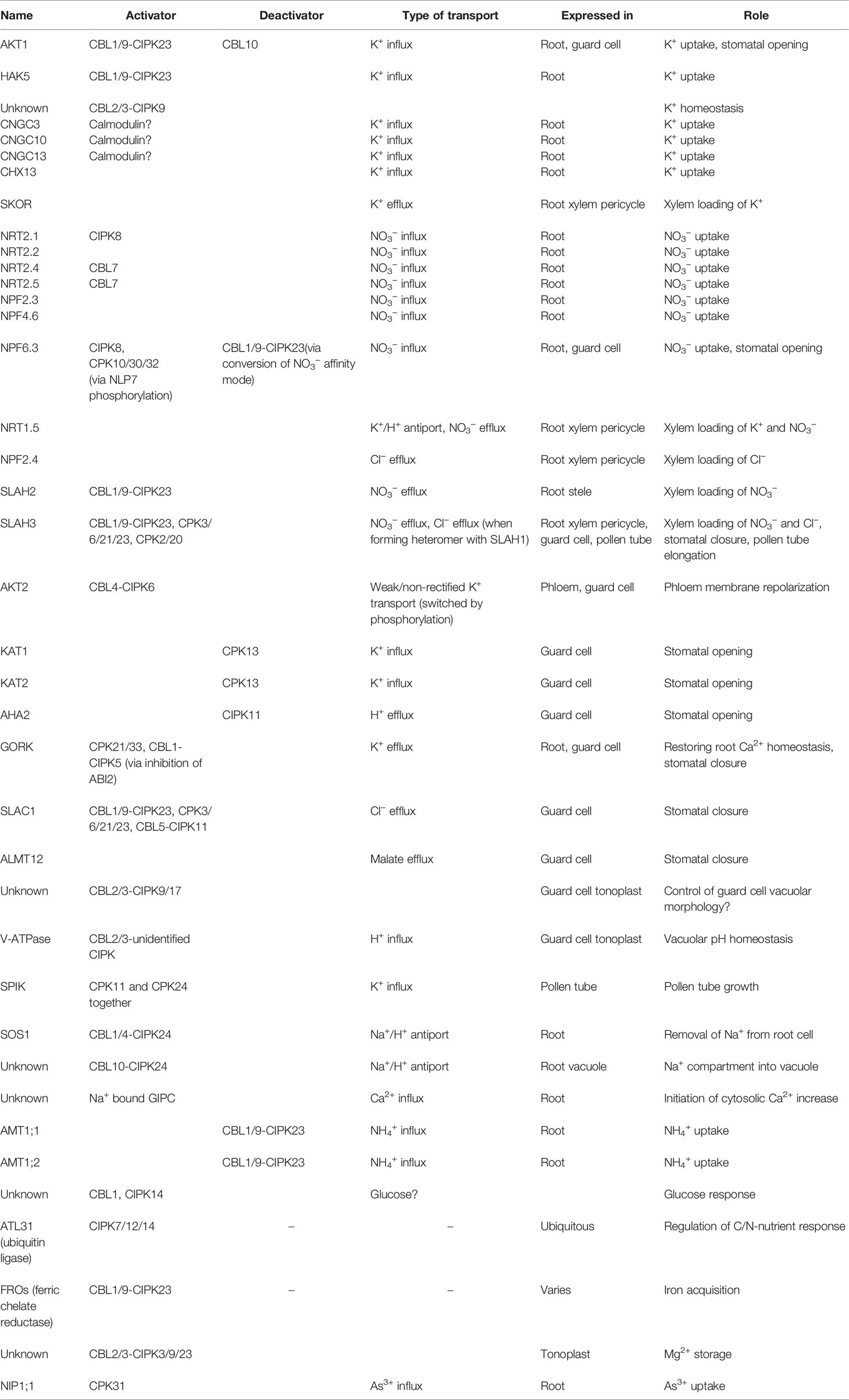
Table 1 Summary of ion channels/transporters and other Ca2+-regulated proteins reviewed in this article.
Author Contributions
Conceptualization, original draft preparation, review, and editing were done by SS, and NU contributed to the article by funding acquisition.
Funding
This work was supported by JSPS KAKENHI Grant Number (16H06558, 18H03762, 19H02880, 19K21140, and 19K22264).
Conflict of Interest
The authors declare that the research was conducted in the absence of any commercial or financial relationships that could be construed as a potential conflict of interest.
Acknowledgments
We thank Anke Reinders for critical reading of the manuscript.
References
Alemán, F., Nieves-Cordones, M., Martínez, V., Rubio, F. (2011). Root K+ acquisition in plants: the Arabidopsis thaliana model. Plant Cell Physiol. 52, 1603–1612. doi: 10.1093/pcp/pcr096
Almeida, D. M., Margarida Oliveira, M., Saibo, N. J. M. (2017). Regulation of Na+ and K+ homeostasis in plants: towards improved salt stress tolerance in crop plants. Genet. Mol. Biol. 40, 326–345. doi: 10.1590/1678-4685-gmb-2016-0106
Andres, Z., Perez-Hormaeche, J., Leidi, E. O., Schlucking, K., Steinhorst, L., McLachlan, D. H., et al. (2014). Control of vacuolar dynamics and regulation of stomatal aperture by tonoplast potassium uptake. Proc. Natl. Acad. Sci. 111, E1806–E1814. doi: 10.1073/pnas.1320421111
Baba, A. I., Rigó, G., Ayaydin, F., Rehman, A. U., Andrási, N., Zsigmond, L., et al. (2018). Functional analysis of the Arabidopsis thaliana CDPK-related kinase family: AtCRK1 regulates responses to continuous light. Int. J. Mol. Sci. 19, 1–21. doi: 10.3390/ijms19051282
Balk, J., Schaedler, T. A. (2014). Iron cofactor assembly in plants. Annu. Rev. Plant Biol. 65, 125–153. doi: 10.1146/annurev-arplant-050213-035759
Barragán, V., Leidi, E. O., Andrés, Z., Rubio, L., de Luca, A., Fernández, J. A., et al. (2012). Ion exchangers NHX1 and NHX2 mediate active potassium uptake into vacuoles to regulate cell turgor and stomatal function in Arabidopsis. Plant Cell 24, 1127–1142. doi: 10.1105/tpc.111.095273
Batistič, O., Kudla, J. (2009). Plant calcineurin B-like proteins and their interacting protein kinases. Biochim. Biophys. Acta Mol. Cell Res. 1793, 985–992. doi: 10.1016/j.bbamcr.2008.10.006
Batistic, O., Sorek, N., Schültke, S., Yalovsky, S., Kudla, J. (2008). Dual fatty acyl modification determines the localization and plasma membrane targeting of CBL/CIPK Ca2+ signaling complexes in Arabidopsis. Plant Cell 20, 1346–1362. doi: 10.1105/tpc.108.058123
Behera, S., Long, Y., Schmitz-Thom, I., Wang, X.-P., Zhang, C., Li, H., et al. (2016). Two spatially and temporally distinct Ca2+ signals convey Arabidopsis thaliana responses to K+ deficiency. New Phytol. 213, 739–750. doi: 10.1111/nph.14145
Benito, B., Haro, R., Amtmann, A., Cuin, T. A., Dreyer, I. (2014). The twins K+ and Na+ in plants. J. Plant Physiol. 171, 723–731. doi: 10.1016/j.jplph.2013.10.014
Boudsocq, M., Sheen, J. (2013). CDPKs in immune and stress signaling. Trends Plant Sci. 18, 30–40. doi: 10.1016/j.tplants.2012.08.008
Britto, D. T., Kronzucker, H. J. (2002). NH4+ toxicity in higher plants: a critical review. J. Plant Physiol. 159, 567–584. doi: 10.1078/0176-1617-0774
Broadley, M. R., White, P. J., Hammond, J. P., Zelko, I., Lux, A. (2007). Zinc in plants: Tansley review. New Phytol. 173, 677–702. doi: 10.1111/j.1469-8137.2007.01996.x
Bush, D. S. (1995). Calcium regulation in plant cells and its role in signaling. Annu. Rev. Plant Physiol. Plant Mol. Biol. 46, 95–122. doi: 10.1146/annurev.pp.46.060195.000523
Caballero, F., Botella, M. A., Rubio, L., Fernández, J. A., Martínez, V., Rubio, F. (2012). A Ca2+-sensitive system mediates low-affinity K+ uptake in the absence of AKT1 in Arabidopsis plants. Plant Cell Physiol. 53, 2047–2059. doi: 10.1093/pcp/pcs140
Corratgé-Faillie, C., Ronzier, E., Sanchez, F., Prado, K., Kim, J.-H., Lanciano, S., et al. (2017). The Arabidopsis guard cell outward potassium channel GORK is regulated by CPK33. FEBS Lett., 591, 1982–1992. doi: 10.1002/1873-3468.12687
Coruzz, G., Bush, D. R. (2001). Nitrogen and carbon nutrient and metabolite signaling in plants. Plant Physiol. 125, 61–64. doi: 10.1104/pp.125.1.61
Cubero-Font, P., Maierhofer, T., Jaslan, J., Rosales, M. A., Espartero, J., Díaz-Rueda, P., et al. (2016). Silent S-type anion channel subunit SLAH1 gates SLAH3 open for chloride root-to-shoot translocation. Curr. Biol., 26, 1–8. doi: 10.1016/j.cub.2016.06.045
Deeken, R., Geiger, D., Fromm, J., Koroleva, O., Ache, P., Langenfeld-Heyser, R., et al. (2002). Loss of the AKT2/3 potassium channel affects sugar loading into the phloem of Arabidopsis. Planta 216, 334–344. doi: 10.1007/s00425-002-0895-1
DeFalco, T. A., Marshall, C. B., Munro, K., Kang, H. G., Moeder, W., Ikura, M., et al. (2016). Multiple calmodulin-binding sites positively and negatively regulate Arabidopsis CYCLIC NUCLEOTIDE-GATED CHANNEL12. Plant Cell 28, 1738–1751. doi: 10.1105/tpc.15.00870
Demidchik, V., Tester, M. (2002). Sodium fluxes through nonselective cation channels in the plasma membrane of protoplasts from Arabidopsis roots. Plant Physiol. 128, 379–387. doi: 10.1104/pp.010524
Drechsler, N., Zheng, Y., Bohner, A., Nobmann, B., von Wirén, N., Kunze, R., et al. (2015). Nitrate-dependent control of shoot K homeostasis by NPF7.3/NRT1.5 and SKOR in Arabidopsis. Plant Physiol. 169, 01152.2015. doi: 10.1104/pp.15.01152
Dreyer, I., Gomez-porras, J. L., Riedelsberger, J. (2017). The potassium battery: a mobile energy source for transport processes in plant vascular tissues. New Phytol. 216, 1049–1053. doi: 10.1111/nph.14667
Eisenach, C., De Angeli, A. (2017). Ion transport at the vacuole during stomatal movements. Plant Physiol. 174, 520–530. doi: 10.1104/pp.17.00130
Fan, X., Naz, M., Fan, X., Xuan, W., Miller, A. J., Xu, G. (2017). Plant nitrate transporters: from gene function to application. J. Exp. Bot. 68, 2463–2475. doi: 10.1093/jxb/erx011
Feng, H., Tang, Q., Cai, J., Xu, B., Xu, G., Yu, L. (2019). Rice OsHAK16 functions in potassium uptake and translocation in shoot, maintaining potassium homeostasis and salt tolerance. Planta 250, 549–561. doi: 10.1007/s00425-019-03194-3
Fischer, W. N., André, B., Rentsch, D., Krolkiewicz, S., Tegeder, M., Breitkreuz, K., et al. (1998). Amino acid transport in plants. Trends Plant Sci. 3, 188–195. doi: 10.1016/S1360-1385(98)01231-X
Förster, S., Schmidt, L. K., Kopic, E., Anschütz, U., Huang, S., Schlücking, K., et al. (2019). Wounding-induced stomatal closure requires jasmonate-mediated activation of GORK K+ channels by a Ca2+ sensor-kinase CBL1-CIPK5 complex. Dev. Cell 48, 87–99.e6. doi: 10.1016/j.devcel.2018.11.014
Fuglsang, A. T., Guo, Y., Cuin, T. A., Qiu, Q., Song, C., Kristiansen, K. A., et al. (2007). Arabidopsis protein kinase PKS5 inhibits the plasma membrane H+-ATPase by preventing interaction with 14-3-3 protein. Plant Cell 19, 1617–1634. doi: 10.1105/tpc.105.035626
Gajdanowicz, P., Michard, E., Sandmann, M., Rocha, M., Corrêa, L. G. G., Ramírez-Aguilar, S. J., et al. (2011). Potassium K+ gradients serve as a mobile energy source in plant vascular tissues. Proc. Natl. Acad. Sci. U. S. A. 108, 864–869. doi: 10.1073/pnas.1009777108
Geiger, D., Scherzer, S., Mumm, P., Marten, I., Ache, P., Matschi, S., et al. (2010). Guard cell anion channel SLAC1 is regulated by CDPK protein kinases with distinct Ca2+ affinities. Proc. Natl. Acad. Sci. U. S. A. 107, 8023–8028. doi: 10.1073/pnas.0912030107
Geiger, D., Maierhofer, T., Al-Rasheid, K. A. S., Scherzer, S., Mumm, P., Liese, A., et al. (2011). Stomatal closure by fast abscisic acid signaling is mediated by the guard cell anion channel SLAH3 and the receptor RCAR1. Sci. Signal. 4, ra32. doi: 10.1126/scisignal.2001346
Gerendás, J., Führs, H. (2013). The significance of magnesium for crop quality. Plant Soil 368, 101–128. doi: 10.1007/s11104-012-1555-2
Gobert, A., Isayenkov, S., Voelker, C., Czempinski, K., Maathuis, F. J. M. (2007). The two-pore channel TPK1 gene encodes the vacuolar K+ conductance and plays a role in K+ homeostasis. Proc. Natl. Acad. Sci. 104, 10726–10731. doi: 10.1073/pnas.0702595104
Grusak, M. A., Broadley, M. R., White, P. J. (2016). Plant macro- and micronutrient minerals. eLS, 1–6. doi: 10.1002/9780470015902.a0001306.pub2
Guo, F.-Q., Young, J., Crawford, N. M. (2003). The nitrate transporter AtNRT1.1 (CHL1) functions in stomatal opening and contributes to drought susceptibility in Arabidopsis. Plant Cell 15, 107–117. doi: 10.1105/tpc.006312
Gutermuth, T., Lassig, R., Portes, M., Maierhofer, T., Romeis, T., Borst, J., et al. (2013). Pollen tube growth regulation by free anions depends on the interaction between the anion channel SLAH3 and calcium-dependent protein kinases CPK2 and CPK20. Plant Cell 25, 4525–4543. doi: 10.1105/tpc.113.118463
Han, M., Wu, W., Wu, W. H., Wang, Y. (2016). Potassium transporter KUP7 is involved in K+ acquisition and translocation in Arabidopsis root under K+-limited conditions. Mol. Plant 9, 437–446. doi: 10.1016/j.molp.2016.01.012
Hashimoto, K., Kudla, J. (2011). Calcium decoding mechanisms in plants. Biochimie 93, 2054–2059. doi: 10.1016/j.biochi.2011.05.019
Held, K., Pascaud, F., Eckert, C., Gajdanowicz, P., Hashimoto, K., Corratgé-Faillie, C., et al. (2011). Calcium-dependent modulation and plasma membrane targeting of the AKT2 potassium channel by the CBL4/CIPK6 calcium sensor/protein kinase complex. Cell Res. 21, 1116–1130. doi: 10.1038/cr.2011.50
Hepler, P. K. (2005). Calcium: a central regulator of plant growth and development. Plant Cell 17, 2142–2155. doi: 10.1105/tpc.105.032508
Hirata, H., Mitsui, S. (1965). Role of calcium in potassium uptake by plant roots. Plant Cell Physiol. 6, 699–709. doi: 10.1093/oxfordjournals.pcp.a079142
Ho, C. H., Lin, S. H., Hu, H. C., Tsay, Y. F. (2009). CHL1 functions as a nitrate sensor in plants. Cell 138, 1184–1194. doi: 10.1016/j.cell.2009.07.004
Hosy, E., Vavasseur, A., Mouline, K., Dreyer, I., Gaymard, F., Porée, F., et al. (2003). The Arabidopsis outward K+ channel GORK is involved in regulation of stomatal movements and plant transpiration. Proc. Natl. Acad. Sci. U. S. A. 100, 5549–5554. doi: 10.1073/pnas.0733970100
Hu, H. C., Wang, Y. Y., Tsay, Y. F. (2009). AtCIPK8, a CBL-interacting protein kinase, regulates the low-affinity phase of the primary nitrate response. Plant J. 57, 264–278. doi: 10.1111/j.1365-313X.2008.03685.x
Ji, R., Zhou, L., Liu, J., Wang, Y., Yang, L., Zheng, Q., et al. (2017). Calcium-dependent protein kinase CPK31 interacts with arsenic transporter AtNIP1;1 and regulates arsenite uptake in Arabidopsis thaliana. PloS One 12, 1–20. doi: 10.1371/journal.pone.0173681
Jiang, Z., Zhou, X., Tao, M., Yuan, F., Liu, L., Wu, F., et al. (2019). Plant cell-surface GIPC sphingolipids sense salt to trigger Ca2+ influx. Nature 572, 341–346. doi: 10.1038/s41586-019-1449-z
Johansen, C., Edwards, D. G., Loneragan, J. F. (1968). Interaction between potassium and calcium in their absorption by intact barley plants. Plant Physiol. 43, 1722–1726. doi: 10.1104/pp.43.10.1722
Kaplan, B., Sherman, T., Fromm, H. (2007). Cyclic nucleotide-gated channels in plants. FEBS Lett. 581, 2237–2246. doi: 10.1016/j.febslet.2007.02.017
Keisham, M., Mukherjee, S., Bhatla, S. C. (2018). Mechanisms of sodium transport in plants—progresses and challenges. Int. J. Mol. Sci. 19, e647. doi: 10.3390/ijms19030647
Kim, B. G., Waadt, R., Cheong, Y. H., Pandey, G. K., Dominguez-Solis, J. R., Schültke, S., et al. (2007). The calcium sensor CBL10 mediates salt tolerance by regulating ion homeostasis in Arabidopsis. Plant J. 52, 473–484. doi: 10.1111/j.1365-313X.2007.03249.x
Kolukisaoglu, U. (2004). Calcium sensors and their interacting protein kinases: genomics of the Arabidopsis and rice CBL-CIPK signaling networks. Plant Physiol. 134, 43–58. doi: 10.1104/pp.103.033068
Köster, P., Wallrad, L., Edel, K. H., Faisal, M., Alatar, A. A., Kudla, J. (2018). The battle of two ions: Ca2+ signalling against Na+ stress. Plant Biol., 1, 39–48. doi: 10.1111/plb.12704
Kroeger, J. H., Zerzour, R., Geitmann, A. (2011). Regulator or driving force? The role of turgor pressure in oscillatory plant cell growth. PloS One 6, e18549. doi: 10.1371/journal.pone.0018549
Kudla, J., Batistic, O., Hashimoto, K. (2010). Calcium signals: the lead currency of plant information processing. Plant Cell 22, 541–563. doi: 10.1105/tpc.109.072686
Lee, S. C., Lan, W.-Z., Kim, B.-G., Li, L., Cheong, Y. H., Pandey, G. K., et al. (2007). A protein phosphorylation/dephosphorylation network regulates a plant potassium channel. Proc. Natl. Acad. Sci. U. S. A. 104, 15959–15964. doi: 10.1073/pnas.0707912104
Leran, S., Edel, K. H., Pervent, M., Hashimoto, K., Corratge-Faillie, C., Offenborn, J. N., et al. (2015). Nitrate sensing and uptake in Arabidopsis are enhanced by ABI2, a phosphatase inactivated by the stress hormone abscisic acid. Sci. Signal. 8, ra43. doi: 10.1126/scisignal.aaa4829
Léran, S., Muños, S., Brachet, C., Tillard, P., Gojon, A., Lacombe, B. (2013). Arabidopsis NRT1.1 is a bidirectional transporter involved in root-to-shoot nitrate translocation. Mol. Plant 6, 1984–1987. doi: 10.1093/mp/sst068
Léran, S., Varala, K., Boyer, J. C., Chiurazzi, M., Crawford, N., Daniel-Vedele, F., et al. (2014). A unified nomenclature of nitrate transporter 1/peptide transporter family members in plants. Trends Plant Sci. 19, 5–9. doi: 10.1016/j.tplants.2013.08.008
Leustek, T., Saito, K. (1999). Sulfate transport and assimilation in plants. Plant Physiol. 120, 637–643. doi: 10.1104/pp.120.3.637
López-Arredondo, D. L., Leyva-González, M. A., Alatorre-Cobos, F., Herrera-Estrella, L. (2013). Biotechnology of nutrient uptake and assimilation in plants. Int. J. Dev. Biol. 57, 595–610. doi: 10.1387/ijdb.130268lh
López-Arredondo, D. L., Leyva-González, M. A., González-Morales, S. I., López-Bucio, J., Herrera-Estrella, L. (2014). Phosphate nutrition: improving low-phosphate tolerance in crops. Annu. Rev. Plant Biol. 65, 95–123. doi: 10.1146/annurev-arplant-050213-035949
Li, J. Y., Fu, Y. L., Pike, S. M., Bao, J., Tian, W., Zhang, Y., et al. (2010). The Arabidopsis nitrate transporter NRT1.8 functions in nitrate removal from the xylem sap and mediates cadmium tolerance. Plant Cell 22, 1633–1646. doi: 10.1105/tpc.110.075242
Li, Z. Y., Xu, Z. S., Chen, Y., He, G. Y., Yang, G. X., Chen, M., et al. (2013). A novel role for Arabidopsis CBL1 in affecting plant responses to glucose and gibberellin during germination and seedling development. PloS One 8. doi: 10.1371/journal.pone.0056412
Li, B., Byrt, C., Qiu, J., Baumann, U., Hrmova, M., Evrard, A., et al. (2016). Identification of a stelar-localized transport protein that facilitates root-to-shoot transfer of chloride in Arabidopsis. Plant Physiol. 170, 1014–1029. doi: 10.1104/pp.15.01163
Li, B., Tester, M., Gilliham, M. (2017a). Chloride on the move. Trends Plant Sci. 22, 236–248. doi: 10.1016/j.tplants.2016.12.004
Li, H., Yu, M., Du, X.-Q., Wang, Z.-F., Wu, W.-H., Quintero, F. J., et al. (2017b). NRT1.5/NPF7.3 functions as a proton-coupled H+/K+ antiporter for K+ loading into the xylem in Arabidopsis. Plant Cell 29, tpc.00972.2016. doi: 10.1105/tpc.16.00972
Lin, S.-H., Kuo, H.-F., Canivenc, G., Lin, C.-S., Lepetit, M., Hsu, P.-K., et al. (2008). Mutation of the Arabidopsis NRT1.5 nitrate transporter causes defective root-to-shoot nitrate transport. Plant Cell Online 20, 2514–2528. doi: 10.1105/tpc.108.060244
Liu, K. H., Tsay, Y. F. (2003). Switching between the two action modes of the dual-affinity nitrate transporter CHL1 by phosphorylation. EMBO J. 22, 1005–1013. doi: 10.1093/emboj/cdg118
Liu, K., Li, L., Luan, S. (2006). Intracellular K+ sensing of SKOR, a Shaker-type K+ channel from Arabidopsis. Plant J. 46, 260–268. doi: 10.1111/j.1365-313X.2006.02689.x
Liu, D. D., Chao, W. M., Turgeon, R. (2012a). Transport of sucrose, not hexose, in the phloem. J. Exp. Bot. 63, 4315–4320. doi: 10.1093/jxb/ers127
Liu, L.-L., Ren, H.-M., Chen, L.-Q., Wang, Y., Wu, W.-H. (2012b). A protein kinase CIPK9 interacts with calcium sensor CBL3 and regulates K+ homeostasis under low-K+ stress in Arabidopsis. Plant Physiol. 161, 266–277. doi: 10.1104/pp.112.206896
Liu, L., Zheng, C., Kuang, B., Wei, L., Yan, L., Wang, T. (2016). Receptor-like kinase RUPO interacts with potassium transporters to regulate pollen tube growth and integrity in rice. PloS Genet. 12, 1–23. doi: 10.1371/journal.pgen.1006085
Liu, K. H., Niu, Y., Konishi, M., Wu, Y., Du, H., Sun Chung, H., et al. (2017). Discovery of nitrate-CPK-NLP signalling in central nutrient-growth networks. Nature 545, 311–316. doi: 10.1038/nature22077
Loqué, D., Von Wirén, N. (2004). Regulatory levels for the transport of ammonium in plant roots. J. Exp. Bot. 55, 1293–1305. doi: 10.1093/jxb/erh147
Mähs, A., Steinhorst, L., Han, J. P., Shen, L. K., Wang, Y., Kudla, J. (2013). The calcineurin B-like Ca2+ sensors CBL1 and CBL9 function in pollen germination and pollen tube growth in Arabidopsis. Mol. Plant 6, 1149–1162. doi: 10.1093/mp/sst095
Ma, Q., Tang, R. J., Zheng, X. J., Wang, S. M., Luan, S. (2015). The calcium sensor CBL7 modulates plant responses to low nitrate in Arabidopsis. Biochem. Biophys. Res. Commun. 468, 59–65. doi: 10.1016/j.bbrc.2015.10.164
Maekawa, S., Inada, N., Yasuda, S., Fukao, Y., Fujiwara, M., Sato, T., et al. (2014). The carbon/nitrogen regulator ARABIDOPSIS TOXICOS EN LEVADURA31 controls papilla formation in response to powdery mildew fungi penetration by interacting with SYNTAXIN OF PLANTS121 in Arabidopsis. Plant Physiol. 164, 879–887. doi: 10.1104/pp.113.230995
Mahajan, S., Sopory, S. K., Tuteja, N. (2006). CBL-CIPK paradigm: role in calcium and stress signaling in plants. Proc. Indian Natl. Sci. Acad. 78, 63–78. https://insa.nic.in/Default.aspx.
Maierhofer, T., Diekmann, M., Offenborn, J. N., Lind, C., Bauer, H., Hashimoto, K., et al. (2014a). Site- and kinase-specific phosphorylation-mediated activation of SLAC1, a guard cell anion channel stimulated by abscisic acid. Sci. Signal. 7, ra86. doi: 10.1126/scisignal.2005703
Maierhofer, T., Lind, C., Hüttl, S., Scherzer, S., Papenfuß, M., Simon, J., et al. (2014b). A single-pore residue renders the Arabidopsis root anion channel SLAH2 highly nitrate selective. Plant Cell 3, 1–15. doi: 10.1105/tpc.114.125849
Manik, S. M. N., Shi, S., Mao, J., Dong, L., Su, Y., Wang, Q., et al. (2015). The calcium sensor CBL-CIPK is involved in plant's response to abiotic stresses. Int. J. Genomics 2015, 1–10. doi: 10.1155/2015/493191
Manishankar, P., Wang, N., Köster, P., Alatar, A. A., Kudla, J. (2018). Calcium signaling during salt stress and in the regulation of ion homeostasis. J. Exp. Bot. 69, 4215–4226. doi: 10.1093/jxb/ery201
Mao, J., Manik, S. M. N., Shi, S., Chao, J., Jin, Y., Wang, Q., et al. (2016). Mechanisms and physiological roles of the CBL-CIPK networking system in Arabidopsis thaliana. Genes (Basel) 7, 1–15. doi: 10.3390/genes7090062
Mendel, R. R. (2013). The molybdenum cofactor. J. Biol. Chem. 288, 13165–13172. doi: 10.1074/jbc.R113.455311
Merchant, S. S. (2010). The elements of plant micronutrients. Plant Physiol. 154, 512–515. doi: 10.1104/pp.110.161810
Meyer, S., Mumm, P., Imes, D., Endler, A., Weder, B., Al-Rasheid, K. A. S., et al. (2010). AtALMT12 represents an R-type anion channel required for stomatal movement in Arabidopsis guard cells. Plant J. 63, 1054–1062. doi: 10.1111/j.1365-313X.2010.04302.x
Michard, E., Dreyer, I., Lacombe, B., Sentenac, H., Thibaud, J. B. (2005a). Inward rectification of the AKT2 channel abolished by voltage-dependent phosphorylation. Plant J. 44, 783–797. doi: 10.1111/j.1365-313X.2005.02566.x
Michard, E., Lacombe, B., Porée, F., Mueller-Roeber, B., Sentenac, H., Thibaud, J.-B., et al. (2005b). A unique voltage sensor sensitizes the potassium channel AKT2 to phosphoregulation. J. Gen. Physiol. 126, 605–617. doi: 10.1085/jgp.200509413
Mogami, J., Fujita, Y., Yoshida, T., Tsukiori, Y., Nakagami, H., Nomura, Y., et al. (2015). Two distinct families of protein kinases are required for plant growth under high external Mg2+ concentrations in Arabidopsis. Plant Physiol. 167, 1039–1057. doi: 10.1104/pp.114.249870
Mouline, K., Véry, A. A., Gaymard, F., Boucherez, J., Pilot, G., Devic, M., et al. (2002). Pollen tube development and competitive ability are impaired by disruption of a Shaker K+ channel in Arabidopsis. Genes Dev. 16, 339–350. doi: 10.1101/gad.213902
Munemasa, S., Hauser, F., Park, J., Waadt, R., Brandt, B., Schroeder, J. I. (2015). Mechanisms of abscisic acid-mediated control of stomatal aperture. Curr. Opin. Plant Biol. 28, 154–162. doi: 10.1016/j.pbi.2015.10.010
Myers, C., Romanowsky, S. M., Barron, Y. D., Garg, S., Azuse, C. L., Curran, A., et al. (2009). Calcium-dependent protein kinases regulate polarized tip growth in pollen tubes. Plant J. 59, 528–539. doi: 10.1111/j.1365-313X.2009.03894.x
Nemoto, K., Takemori, N., Seki, M., Shinozaki, K., Sawasaki, T. (2015). Members of the plant CRK superfamily are capable of trans- and autophosphorylation of tyrosine residues. J. Biol. Chem. 290, 16665–16677. doi: 10.1074/jbc.M114.617274
Noguero, M., Lacombe, B. (2016). Transporters involved in root nitrate uptake and sensing by Arabidopsis. Front. Plant Sci. 7, 1–7. doi: 10.3389/fpls.2016.01391
Pan, Y., Chai, X., Gao, Q., Zhou, L., Zhang, S., Li, L., et al. (2019). Dynamic interactions of plant CNGC subunits and calmodulins drive oscillatory Ca2+ channel activities. Dev. Cell. 48, 710–725. doi: 10.1016/j.devcel.2018.12.025
Pandey, G. K., Cheong, Y. H., Kim, B. G., Grant, J. J., Li, L., Luan, S. (2007a). CIPK9: a calcium sensor-interacting protein kinase required for low-potassium tolerance in Arabidopsis. Cell Res. 17, 411–421. doi: 10.1038/cr.2007.39
Pandey, S., Zhang, W., Assmann, S. M. (2007b). Roles of ion channels and transporters in guard cell signal transduction. FEBS Lett. 581, 2325–2336. doi: 10.1016/j.febslet.2007.04.008
Parker, J. L., Newstead, S. (2014). Molecular basis of nitrate uptake by the plant nitrate transporter NRT1.1. Nature 507, 68–72. doi: 10.1038/nature13116
Pyo, Y. J., Gierth, M., Schroeder, J. I., Cho, M. H. (2010). High-affinity K+ transport in Arabidopsis: AtHAK5 and AKT1 are vital for seedling establishment and postgermination growth under low-potassium conditions. Plant Physiol. 153, 863–875. doi: 10.1104/pp.110.154369
Qiu, Q.-S., Guo, Y., Dietrich, M. A., Schumaker, K. S., Zhu, J.-K. (2002). Regulation of SOS1, a plasma membrane Na+/H+ exchanger in Arabidopsis thaliana, by SOS2 and SOS3. Proc. Natl. Acad. Sci. U. S. A. 99, 8436–8441. doi: 10.1073/pnas.122224699
Qiu, J., Henderson, S. W., Tester, M., Roy, S. J., Gilliham, M. (2016). SLAH1, a homologue of the slow type anion channel SLAC1, modulates shoot Cl– accumulation and salt tolerance in Arabidopsis thaliana. J. Exp. Bot. 67, 4495–4505. doi: 10.1093/jxb/erw237
Ragel, P., Ródenas, R., García-Martín, E., Andrés, Z., Villalta, I., Nieves-Cordones, M., et al. (2015). CIPK23 regulates HAK5-mediated high-affinity K+ uptake in Arabidopsis roots. Plant Physiol. 169, 01401.2015. doi: 10.1104/pp.15.01401
Ragel, P., Raddatz, N., Leidi, E. O., Quintero, F. J., Pardo, J. M. (2019). Regulation of K+ nutrition in plants. Front. Plant Sci. 10, 281. doi: 10.3389/fpls.2019.00281
Rains, W. D., Floyd, A. R. (1970). Influence of calcium on sodium and potassium absorption by fresh and aged bean stem slices. Plant Physiol. 46, 93–98. doi: 10.1104/pp.46.1.93
Ratajczak, R. (2000). Structure, function and regulation of the plant vacuolar H+translocating ATPase. Biochim. Biophys. Acta Biomembr. 1465, 17–36. doi: 10.1016/S0005-2736(00)00129-2
Ren, X. L., Qi, G. N., Feng, H. Q., Zhao, S., Zhao, S. S., Wang, Y., et al. (2013). Calcineurin B-like protein CBL10 directly interacts with AKT1 and modulates K+ homeostasis in Arabidopsis. Plant J. 74, 258–266. doi: 10.1111/tpj.12123
Rigó, G., Ayaydin, F., Tietz, O., Zsigmond, L., Kovács, H., Páy, A., et al. (2013). Inactivation of plasma membrane-localized CDPK-RELATED KINASE5 decelerates PIN2 exocytosis and root gravitropic response in Arabidopsis. Plant Cell 25, 1592–1608. doi: 10.1105/tpc.113.110452
Riveras, E., Alvarez, J. M., Vidal, E. A., Oses, C., Vega, A., Gutiérrez, R. A. (2015). The calcium ion is a second messenger in the nitrate signaling pathway of Arabidopsis. Plant Physiol. 169, 1397–1404. doi: 10.1104/pp.15.00961
Rolland, F., Sheen, J. (2005). Sugar sensing and signalling networks in plants. Biochem. Soc Trans. 33, 269–271. doi: 10.1042/BST0330269
Ronzier, E., Corratgé-Faillie, C., Sanchez, F., Prado, K., Brière, C., Leonhardt, N., et al. (2014). CPK13, a noncanonical Ca2+-dependent protein kinase, specifically inhibits KAT2 and KAT1 Shaker K+ channels and reduces stomatal opening. Plant Physiol. 166, 314–326. doi: 10.1104/pp.114.240226
Rubio, F., Alemán, F., Nieves-Cordones, M., Martínez, V. (2010). Studies on Arabidopsis athak5, atakt1 double mutants disclose the range of concentrations at which AtHAK5, AtAKT1 and unknown systems mediate K+ uptake. Physiol. Plant. 139, 220–228. doi: 10.1111/j.1399-3054.2010.01354.x
Saito, S., Uozumi, N. (2019). Guard cell membrane anion transport systems and their regulatory components: an elaborate mechanism controlling stress-induced stomatal closure. Plants 8, 9. doi: 10.3390/plants8010009
Saito, S., Hoshi, N., Zulkifli, L., Widyastuti, S., Goshima, S., Dreyer, I., et al. (2017). Identification of regions responsible for the function of the plant K+ channels KAT1 and AKT2 in Saccharomyces cerevisiae and Xenopus laevis oocytes. Channels 11, 510–516. doi: 10.1080/19336950.2017.1372066
Saito, S., Hamamoto, S., Moriya, K., Matsuura, A., Sato, Y., Muto, J., et al. (2018). N -myristoylation and S-acylation are common modifications of Ca2+-regulated Arabidopsis kinases and are required for activation of the SLAC1 anion channel. New Phytol. 218, 1504–1521. doi: 10.1111/nph.15053
Sanders, D., Brownlee, C., Harper, J. F. (1999). Communicating with calcium. Plant Cell 11, 691–706. doi: 10.1105/tpc.11.4.691
Sandmann, M., Skłodowski, K., Gajdanowicz, P., Michard, E., Rocha, M., Gomez-Porras, J. L., et al. (2011). The K+ battery-regulating Arabidopsis K+ channel AKT2 is under the control of multiple post-translational steps. Plant Signal. Behav. 6, 558–562. doi: 10.4161/psb.6.4.14908
Scherzer, S., Maierhofer, T., Al-Rasheid, K. A. S., Geiger, D., Hedrich, R. (2012). Multiple calcium-dependent kinases modulate ABA-activated guard cell anion channels. Mol. Plant 5, 1409–1412. doi: 10.1093/mp/sss084
Schmidt, S. B., Husted, S. (2019). The biochemical properties of manganese in plants. Plants 8, 381. doi: 10.3390/plants8100381
Schmidt, S. B., Jensen, P. E., Husted, S. (2016). Manganese deficiency in plants: the impact on photosystem II. Trends Plant Sci. 21, 622–632. doi: 10.1016/j.tplants.2016.03.001
Schulz, P., Herde, M., Romeis, T. (2013). Calcium-dependent protein kinases: hubs in plant stress signaling and development. Plant Physiol. 163, 523–530. doi: 10.1104/pp.113.222539
Shabala, S. (2003). Regulation of potassium transport in leaves: from molecular to tissue level. Ann. Bot. 92, 627–634. doi: 10.1093/aob/mcg191
Shi, H., Quintero, F. J., Pardo, J. M., Zhu, J. K. (2002). The putative plasma membrane Na+/H+ antiporter SOS1 controls long-distance Na+transport in plants. Plant Cell 14, 465–477. doi: 10.1105/tpc.010371
Shi, S., Li, S., Asim, M., Mao, J., Xu, D., Ullah, Z., et al. (2018). The Arabidopsis calcium-dependent protein kinases (CDPKs) and their roles in plant growth regulation and abiotic stress responses. Int. J. Mol. Sci. 19, e1900. doi: 10.3390/ijms19071900
Singh, M., Gupta, A., Laxmi, A. (2014). Glucose control of root growth direction in Arabidopsis thaliana. J. Exp. Bot. 65, 2981–2993. doi: 10.1093/jxb/eru146
Singh, A., Yadav, A. K., Kaur, K., Sanyal, S. K., Jha, S. K., Fernandes, J. L., et al. (2018). A protein phosphatase 2C, AP2C1, interacts with and negatively regulates the function of CIPK9 under potassium-deficient conditions in Arabidopsis. J. Exp. Bot. 69, 4003–4015. doi: 10.1093/jxb/ery182
Song, S.-J., Feng, Q.-N., Li, C., Li, E., Liu, Q., Kang, H., et al. (2018). A tonoplast-associated calcium-signaling module dampens ABA signaling during stomatal movement. Plant Physiol. 177, 00377.2018. doi: 10.1104/pp.18.00377
Steinhorst, L., Kudla, J. (2013a). Calcium—a central regulator of pollen germination and tube growth. Biochim. Biophys. Acta Mol. Cell Res. 1833, 1573–1581. doi: 10.1016/j.bbamcr.2012.10.009
Steinhorst, L., Kudla, J. (2013b). Calcium and reactive oxygen species rule the waves of signaling. Plant Physiol. 163, 471–485. doi: 10.1104/pp.113.222950
Steinhorst, L., Mähs, A., Ischebeck, T., Zhang, C., Zhang, X., Arendt, S., et al. (2015). Vacuolar CBL-CIPK12 Ca2+-sensor-kinase complexes are required for polarized pollen tube growth. Curr. Biol. 25, 1475–1482. doi: 10.1016/j.cub.2015.03.053
Straub, T., Ludewig, U., Neuhaeuser, B. (2017). The kinase CIPK23 inhibits ammonium transport in Arabidopsis thaliana. Plant Cell, 29, 409–422. doi: 10.1105/tpc.16.00806
Sun, J., Bankston, J. R., Payandeh, J., Hinds, T. R., Zagotta, W. N., Zheng, N. (2014). Crystal structure of the plant dual-affinity nitrate transporter NRT1.1. Nature 507, 73–77. doi: 10.1038/nature13074
Szyroki, A., Ivashikina, N., Dietrich, P., Roelfsema, M. R. G., Ache, P., Reintanz, B., et al. (2001). KAT1 is not essential for stomatal opening. Proc. Natl. Acad. Sci. 98, 2917–2921. doi: 10.1073/pnas.051616698
Tang, R.-J., Liu, H., Yang, Y., Yang, L., Gao, X.-S., Garcia, V. J., et al. (2012). Tonoplast calcium sensors CBL2 and CBL3 control plant growth and ion homeostasis through regulating V-ATPase activity in Arabidopsis. Cell Res. 22, 1650–1665. doi: 10.1038/cr.2012.161
Tang, R.-J., Zhao, F.-G., Garcia, V. J., Kleist, T. J., Yang, L., Zhang, H.-X., et al. (2015). Tonoplast CBL–CIPK calcium signaling network regulates magnesium homeostasis in Arabidopsis. Proc. Natl. Acad. Sci. 112 (10), 3134–3139. doi: 10.1073/pnas.1420944112
Taochy, C., Gaillard, I., Ipotesi, E., Oomen, R., Leonhardt, N., Zimmermann, S., et al. (2015). The Arabidopsis root stele transporter NPF2.3 contributes to nitrate translocation to shoots under salt stress. Plant J. 83, 466–479. doi: 10.1111/tpj.12901
Tester, M., Davenport, R. (2003). Na+ tolerance and Na+ transport in higher plants. Ann. Bot. 91, 503–527. doi: 10.1093/aob/mcg058
Tian, Q., Zhang, X., Yang, A., Wang, T., Zhang, W. H. (2016). CIPK23 is involved in iron acquisition of Arabidopsis by affecting ferric chelate reductase activity. Plant Sci. 246, 70–79. doi: 10.1016/j.plantsci.2016.01.010
Toyota, M., Spencer, D., Sawai-toyota, S., Jiaqi, W., Zhang, T., Abraham, K. J., et al. (2018). Glutamate triggers long-distance, calcium-based plant defense signaling. Science (80-.), 1112–1115. doi: 10.1126/science.aat7744
Undurraga, S. F., Ibarra-Henríquez, C., Fredes, I., Álvarez, J. M., Gutiérrez, R. A. (2017). Nitrate signaling and early responses in Arabidopsis roots. J. Exp. Bot. 68, 2541–2551. doi: 10.1093/jxb/erx041
Vahisalu, T., Kollist, H., Wang, Y.-F., Nishimura, N., Chan, W.-Y., Valerio, G., et al. (2008). SLAC1 is required for plant guard cell S-type anion channel function in stomatal signalling. Nature 452, 487–491. doi: 10.1038/nature06608
van Kleeff, P. J. M., Gao, J., Mol, S., Zwart, N., Zhang, H., Li, K. W., et al. (2018). The Arabidopsis GORK K+-channel is phosphorylated by calcium-dependent protein kinase 21 (CPK21), which in turn is activated by 14-3-3 proteins. Plant Physiol. Biochem. 125, 219–231. doi: 10.1016/j.plaphy.2018.02.013
Walker, D. J., Leigh, R. A., Miller, A. J. (1996). Potassium homeostasis in vacuolate plant cells. Proc. Natl. Acad. Sci. U. S. A. 93, 10510–10514. doi: 10.1073/pnas.93.19.10510
Welch, R. M. (1995). Micronutrient nutrition of plants. CRC Crit. Rev. Plant Sci. 14, 49–82. doi: 10.1080/07352689509701922
Williams, S. P., Rangarajan, P., Donahue, J. L., Hess, J. E., Gillaspy, G. E. (2014). Regulation of sucrose non-fermenting related kinase 1 genes in Arabidopsis thaliana. Front. Plant Sci. 5, 1–13. doi: 10.3389/fpls.2014.00324
Wu, J. Y., Jin, C., Zhang, S. L. (2011). Potassium flux in the pollen tubes was essential in plant sexual reproduction. Plant Signal. Behav. 6, 898–900. doi: 10.4161/psb.6.6.15322
Xu, J., Li, H. D., Chen, L. Q., Wang, Y., Liu, L. L., He, L., et al. (2006). A protein kinase, interacting with two calcineurin B-like proteins, regulates K+ transporter AKT1 in Arabidopsis. Cell 125, 1347–1360. doi: 10.1016/j.cell.2006.06.011
Xuan, W., Beeckman, T., Xu, G. (2017). Plant nitrogen nutrition: sensing and signaling. Curr. Opin. Plant Biol. 39, 57–65. doi: 10.1016/j.pbi.2017.05.010
Yamada, K., Kanai, M., Osakabe, Y., Ohiraki, H., Shinozaki, K., Yamaguchi-Shinozaki, K. (2011). Monosaccharide absorption activity of Arabidopsis roots depends on expression profiles of transporter genes under high salinity conditions. J. Biol. Chem. 286, 43577–43586. doi: 10.1074/jbc.M111.269712
Yan, J., Niu, F., Liu, W. Z., Zhang, H., Wang, B., Yang, B., et al. (2014). Arabidopsis CIPK14 positively regulates glucose response. Biochem. Biophys. Res. Commun. 450, 1679–1683. doi: 10.1016/j.bbrc.2014.07.064
Yang, Y., Qin, Y., Xie, C., Zhao, F., Zhao, J., Liu, D., et al. (2010). The Arabidopsis chaperone J3 regulates the plasma membrane H+-ATPase through interaction with the PKS5 kinase. Plant Cell 22, 1313–1332. doi: 10.1105/tpc.109.069609
Yao, F. Y., Qi, G. N., Hussain, J. (2017). Investigation of the regulation mechanism of Arabidopsis thaliana anion channel SLAH2. Turk. J. Bot. 41, 543–551. doi: 10.3906/bot-1702-23
Yasuda, S., Sato, T., Maekawa, S., Aoyama, S., Fukao, Y., Yamaguchi, J. (2014). Phosphorylation of arabidopsis ubiquitin ligase ATL31 is critical for plant carbon/nitrogen nutrient balance response and controls the stability of 14-3-3 proteins. J. Biol. Chem. 289, 15179–15193. doi: 10.1074/jbc.M113.533133
Yasuda, S., Aoyama, S., Hasegawa, Y., Sato, T., Yamaguchi, J. (2017). Arabidopsis CBL-interacting protein kinases regulate carbon/nitrogen-nutrient response by phosphorylating ubiquitin ligase ATL31. Mol. Plant 10, 605–618. doi: 10.1016/j.molp.2017.01.005
Yuan, T.-T., Xu, H.-H., Zhang, K.-X., Guo, T.-T., Lu, Y.-T. (2014). Glucose inhibits root meristem growth via ABA INSENSITIVE 5, which represses PIN1 accumulation and auxin activity in Arabidopsis. Plant Cell Environ. 37, 1338–1350. doi: 10.1111/pce.12233
Zhang, G. B., Meng, S., Gong, J. M. (2018). The expected and unexpected roles of nitrate transporters in plant abiotic stress resistance and their regulation. Int. J. Mol. Sci. 19, 1–15. doi: 10.3390/ijms19113535
Zhao, J., Cheng, N. H., Motes, C. M., Blancaflor, E. B., Moore, M., Gonzales, N., et al. (2008). AtCHX13 is a plasma membrane K+ transporter. Plant Physiol. 148, 796–807. doi: 10.1104/pp.108.124248
Zhao, L.-N., Shen, L.-K., Zhang, W.-Z., Zhang, W., Wang, Y., Wu, W.-H. (2013). Ca2+-dependent protein kinase11 and 24 modulate the activity of the inward rectifying K+ channels in Arabidopsis pollen tubes. Plant Cell 25, 649–661. doi: 10.1105/tpc.112.103184
Zhao, L., Liu, F., Crawford, N. M., Wang, Y. (2018a). Molecular regulation of nitrate responses in plants. Int. J. Mol. Sci. 19, e2039. doi: 10.3390/ijms19072039
Zhao, L., Zhang, W., Yang, Y., Li, Z., Li, N., Qi, S., et al. (2018b). The Arabidopsis NLP7 gene regulates nitrate signaling via NRT1.1-dependent pathway in the presence of ammonium. Sci. Rep. 8, 1–13. doi: 10.1038/s41598-018-20038-4
Zheng, X., He, K., Kleist, T., Chen, F., Luan, S. (2015). Anion channel SLAH3 functions in nitrate-dependent alleviation of ammonium toxicity in Arabidopsis. Plant Cell Environ. 38, 474–486. doi: 10.1111/pce.12389
Zheng, R. H., de Su, S., Xiao, H., Tian, H. Q. (2019). Calcium: a critical factor in pollen germination and tube elongation. Int. J. Mol. Sci. 20, e420. doi: 10.3390/ijms20020420
Zheng, Z.-L. (2009). Carbon and nitrogen nutrient balance signaling in plants. Plant Signal. Behav. 4, 584–591. doi: 10.4161/psb.4.7.8540
Zhong, M., Li, S., Huang, F., Qiu, J., Zhang, J., Sheng, Z., et al. (2017). The phosphoproteomic response of rice seedlings to cadmium stress. Int. J. Mol. Sci. 18, e2055. doi: 10.3390/ijms18102055
Zhou, L., Lan, W., Chen, B., Fang, W., Luan, S. (2015). A calcium sensor-regulated protein kinase, CALCINEURIN B-LIKE PROTEIN-INTERACTING PROTEIN KINASE19, is required for pollen tube growth and polarity. Plant Physiol. 167, 1351–1360. doi: 10.1104/pp.114.256065
Keywords: nutrition, calcium, membrane transport, Arabidopsis thaliana, ion homeostasis
Citation: Saito S and Uozumi N (2020) Calcium-Regulated Phosphorylation Systems Controlling Uptake and Balance of Plant Nutrients. Front. Plant Sci. 11:44. doi: 10.3389/fpls.2020.00044
Received: 05 November 2019; Accepted: 14 January 2020;
Published: 11 February 2020.
Edited by:
Francisco Rubio, Spanish National Research Council, SpainReviewed by:
Isabelle Chérel, Institut National de la Recherche Agronomique (INRA), FranceIngo Dreyer, University of Talca, Chile
Copyright © 2020 Saito and Uozumi. This is an open-access article distributed under the terms of the Creative Commons Attribution License (CC BY). The use, distribution or reproduction in other forums is permitted, provided the original author(s) and the copyright owner(s) are credited and that the original publication in this journal is cited, in accordance with accepted academic practice. No use, distribution or reproduction is permitted which does not comply with these terms.
*Correspondence: Shunya Saito, c2h1bnlhLnNhaXRvQHRvaG9rdS5hYy5qcA==; Nobuyuki Uozumi, dW96dW1pQHRvaG9rdS5hYy5qcA==