- 1Fujian Provincial Key Laboratory of Plant Functional Biology, College of Life Sciences, Fujian Agriculture and Forestry University, Fuzhou, China
- 2Key Laboratory of Ministry of Education for Genetics, Breeding and Multiple Utilization of Crops, College of Agriculture, Fujian Agriculture and Forestry University, Fuzhou, China
Phosphoinositide 3-kinase (PI3K) plays a vital role in plant response to abiotic stress. However, the role of PI3K in plant immunity is largely unknown. This study showed that PI3K enhanced Arabidopsis resistance to Pseudomonas syringae pv tomato DC3000 (Pst DC3000) and Pst DC3000 (avrRpt2). Overexpression of AtVPS34 promoted stomatal closure while PI3K inhibitors blocked that after spray inoculation. Additionally, gene expression of AtVPS34 was increased upon infection by Pst DC3000 (avrRpt2), and SA upregulated AtVPS34 gene expression in this process. Furthermore, overexpression of AtVPS34 enhanced PR gene expression after syringe infiltration with Pst DC3000 (avrRpt2), while PI3K inhibitors inhibited that. The production of hydrogen peroxide and the expression of gene encoding antioxidant enzyme were both enhanced in AtVPS34 overexpressing lines after spray inoculation or syringe infiltration with Pst DC3000 (avrRpt2). Collectively, these results unraveled a novel and broad role of PI3K in plant immunity which promoted stomatal closure and PR gene expression possibly via regulating ROS production.
Introduction
Plant defense involves two overlapping tiers of responses, PAMP-triggered immunity (PTI) and effector-triggered immunity (ETI) (Jones and Dangl, 2006). PTI involves distinct well-characterized physiological mechanisms, such as stomata closure to limit pathogen entry, reactive oxygen species (ROS) production, the biosynthesis of antimicrobial metabolites and proteins such as pathogenesis-related (PR) proteins, defense hormones such as salicylic acid (SA), jasmonic acid (JA). In contrast to PTI, ETI induces stronger and long-lasting responses, which is frequently accompanied by programmed cell death, a process known as the hypersensitive response (HR), leading to pathogen resistance. Autophagy operates negative feedback loop modulating SA signaling to suppress the process of HR (Yoshimoto et al., 2009). Phosphoinositide 3-kinase (PI3K) as a key regulator of lipid signal has been reported to be involved in autophagy. PI3K is associated with BECN1/ATG6 to construct the PI3K type III complex, contributing to activation of autophagy (Abeliovich and Klionsky, 2001). In mammalian cells, there are three types of PI3K with distinct substrate specificities. In cells of the mammalian immune system, PI3K is activated by receptors for antigen, cytokines, costimulatory molecules, and so on. PI3K signaling regulates immune cell proliferation, survival, differentiation, chemotaxis, phagocytosis, degranulation, and respiratory burst (Fruman and Cantley, 2002). However, type III PI3K (VPS34), the only type of PI3K identified in plants, phosphorylates the D-3 position of phosphoinositides to generate phosphatidylinositol-3-phosphate (PI3P) (Bunney et al., 2000). In Arabidopsis, PI3K is encoded by a single-copy gene, AtVPS34 (Lee et al., 2008). Given its evolutionarily conserved family of signal transducing enzymes, it is worth revealing the role of PI3K/VPS34 in plant immunity.
Actually, phosphoinositide signaling plays a vital role in plant immunity. The abundant types and metabolizing enzymes of phosphoinositide allowing rapid and reversible interconversion between them provide a highly dynamic and powerful system to coordinate membrane reorganization, vesicle trafficking and cytoskeleton rearrangements as well as signaling pathways determining cell fate (Payrastre et al., 2012). Meanwhile, pathogens have evolved many different strategies to subvert the phosphoinositide metabolism and express phosphoinositide binding effectors (Payrastre et al., 2012). In the process of oomycetes infection, effector Avh5 could interacts with PI3P, which promotes host cell entry (Kale et al., 2010). After internalization, PI3P assists bacterial toxins to escape via several mechanisms including retrograde translocation from the ER and transit of partially unfolded proteins directly across membranes (Bhattacharjee et al., 2012). However, PI3K also seems to play a positive role in immune response. PI3K/VPS34 functions not only to limit the spread of TMV-induced HR PCD, but also to inhibit virus replication and/or movement (Liu et al., 2005). In addition, PI3P activates the p40phox subunit of NADPH oxidase that forms part of the human innate immune response (Ellson et al., 2006). Therefore, the role of PI3K in immune response is complex. Despite many reports, the role of PI3K is still not entirely clear besides its role in HR. For example, PI3K/AtVPS34 functions in stomatal closure in plant stress response (Jung et al., 2002; Park et al., 2003; Choi et al., 2008; Liu et al., 2016), indicating a possible role of PI3K/AtVPS34 in stomatal immunity.
In this study, two types of bacteria, Pseudomonas syringae pv Tomato DC3000 (Pst DC3000) and avirulent Pst DC3000 (avrRpt2), were used. To examine the novel role of PI3K in plant immunity, AtVPS34 overexpressing Arabidopsis and two PI3K inhibitors, LY294002 (LY) and wortmannin (WM), were used as previously described (Liu et al., 2016). LY, which is derived from the flavonoid quercetin, competes with ATP and binds to Lys residues in the ATP-binding pocket of PI3Ks (Walker et al., 2000). WM, a fungal metabolite, dose-dependently targets PI3K and PI4K (Takáč et al., 2012). Given the key role of PI3K in stomatal movement, stomatal aperture was determined after spray inoculation. The effect of PI3K on plant immunity after syringe infiltration was also examined by phenotype analysis, PR gene expression and ROS signaling. In conclusion, we revealed a new role of PI3K during bacterial infection in Arabidopsis.
Materials and Methods
Plant Material
Seedling of wild-type (ecotype Columbia) Arabidopsis (Arabidopsis thaliana), transgenic PR1pro::GUS Arabidopsis (Sun et al., 2012), 35Spro::YFP Arabidopsis and 35Spro::AtVP34-YFP Arabidopsis were sterilized and grown in soil as described previously (Liu et al., 2016). AtVPS34 was cloned into the pHB-YFP vector containing CaMV35S promoter to generate 35Spro::AtVPS34-YFP plasmid (Liu et al., 2016). The determination of transgenic Arabidopsis was shown in Figure S1.
Chemicals
Commercial chemicals were used at the following concentrations: 30 μM LY (Beyotime), 10 μM WM (Beyotime), 10 μM abscisic acid (ABA, Beyotime), 1 mM salicylic acid (SA, Sigma), 1 mM methyl jasmonate (MeJA, Sigma), 20 μM brassinolide (BL, Sigma), 100 μM indol-yl-3-acetic acid (IAA, Sigma), and 10 μM gibberellin (GA, Sigma). The treatment of phytohormone was performed according to the previous study (Yi et al., 2014; Yuan et al., 2017).
Pathogen Growth and Inoculation
Bacterial infections were performed with three-week-old Arabidopsis. Pst DC3000 (avrRpt2) and Pst DC3000 was cultured at 30°C in LB medium supplemented with appropriate antibiotics (Melotto et al., 2006; Hung et al., 2014). Overnight log-phase cultures were cultured by centrifugation, washed with 10 mM MgCl2, and then diluted to a final optical density at 600 nm (OD600) of 0.01. The bacterial suspensions were infiltrated from the abaxial side into a leaf using a 1 ml syringe without needle.
For stomatal immunity assay, 3-week-old Arabidopsis were uniformly spray-inoculated with Pst DC3000 suspension (OD600 = 0.05) in 10 mM MgCl2 with 0.02% Silwet L-77 (Su et al., 2017).
Bacterial Growth Assay
The harvested leaves were surface sterilized (30 s in 70% ethanol, then 30 s in sterile distilled water) for spray inoculation (Zipfel et al., 2004). Three leaf discs (5 mm diameter) containing the sites syringe-infiltrated or spray-inoculated with bacteria were excised from each leaf and collected in three groups, each of which contained six discs each from a different leaf (Alvarez et al., 1998). Leaf discs were ground in 10 mM MgCl2, then thoroughly vortex-mixed and diluted 1:10 serially. Samples were finally plated on King's B medium with appropriate antibiotics. Colonies were counted after incubation at 30°C.
Assessment of Response of Stomata to Treatments
To assure that most stomata were open before beginning experiments, we kept plants under light (100 μE/m2/s) for at least 3 h. Full expanded young leaves were immersed in water or bacterial suspension (108 cfu/ml in water). 1 and 3 hours after spray inoculation, epidermis of three leaves was peeled off and immediately observed by a microscope. The width and length of the stomatal aperture were measured using the software ImageJ. All stomatal aperture results reported here were from blind experiments in which genotypes and treatments were unknown to the experimenters who measured stomatal responses until the completion of experiments (Melotto et al., 2006).
RNA Extraction and RT-PCR Analyses
Total RNA was extracted using RNAiso Plus kit (Takara, cat. no. 9108) according to the manufacturer's specifications. cDNA synthesis was carried out using GoScript™ reverse transcription system (Promega, cat. no. A5000).
The transcript levels of AtVPS34, AtPR1, AtPR5, AtCAT1, AtCAT2, AtAPX1, AtCSD1, and AtMSD1 genes were analyzed by quantitative RT-PCR. The gene-specific primers were shown in Table 1. AtActin2 gene was amplified as a quantitative control.
Histochemical GUS Staining
Approximately 3-week-old transgenic PR1pro::GUS Arabidopsis was syringe-infiltrated with Pst DC3000 (avrRpt2), 0, 12, and 24 h after infiltration, histochemical detection of GUS enzyme activity was performed as described by β-Galactosidase Reporter Gene Staining Kit (Solarbio, cat. no. G3060).
Measurements of H2O2 Content
1 and 3 h after infection, syringe-infiltrated and spray-inoculated leaves were collected, then the content of H2O2 produced in leaf tissues was measured by Hydrogen Peroxide Assay Kit (Beyotime, cat. no. S0038).
YFP Fluorescence Analysis
All microscopic observations were performed using a confocal laser-scanning microscope (Leica SP8). 35Spro::AtVP34-YFP-1 Arabidopsis was pretreated with 1 mM SA or not, then syringe-infiltrated with Pst DC3000 (avrRpt2). YFP fluorescence was examined one day after infiltration (YFP: excitation 514 nm, emission 525–550 nm).
Western Blot and Coomassie Staining of Proteins
35Spro::AtVP34-YFP-1 Arabidopsis was pretreated with 1 mM SA or not, then syringe-infiltrated with Pst DC3000 (avrRpt2). One day after infiltration with Pst DC3000 (avrRpt2), the infected leaves were collected. The total protein were extracted from leaf samples. Proteins extracts were separated on a 12% (w/v) SDS-PAGE and transferred onto PVDF membranes, then subjected to immunodetection with GFP polyclonal antibody (Beyotime, AG279). The antigen-antibody complex was visualized with anti-rabbit secondary antibody and enhanced chemiluminescence. Coomassie staining of the large subunit of Rubisco was used as a loading control. All the experiments took three independent repetitions.
Statistical Analysis
All assays were performed independently for a minimum of three biological replications. Data are represented as mean ± SD. Statistical analysis was performed with the Student’s paired t test.
Results
PI3K Functioned in Plant Immunity During Pst DC3000 (AvrRpt2) and Pst DC3000 Infection
To assess the role of PI3K in plant immunity, a previous constructed Arabidopsis overexpressing AtVPS34 was used (Liu et al., 2016). 35Spro::AtVP34-YFP lines showed more resistant compared with 35Spro::YFP Arabidopsis when syringe-infiltrated or spray-inoculated with Pst DC3000 (avrRpt2) (Figures 1A, C) and Pst DC3000 (Figures S2A, C). To further examine the role of PI3K in Arabidopsis resistance against bacteria invasion, two PI3K inhibitors LY and wortmannin (WM) was used. The specificity of LY and WM in inhibiting plant PI3K was shown in previous studies (Takáč et al., 2012; Leprince et al., 2015). Arabidopsis was pretreated with 30 μM LY or 10 μM WM for 24 h, then syringe-infiltrated or spray-inoculated with Pst DC3000 (avrRpt2) and Pst DC3000. More susceptible phenotype was observed on leaves after spraying with PI3K inhibitors compared with that in control (Figures 1B, D and Figures S2B, D). These results suggested that PI3K was involved in plant immunity against Pst DC3000 and Pst DC3000 (avrRpt2) invasion.
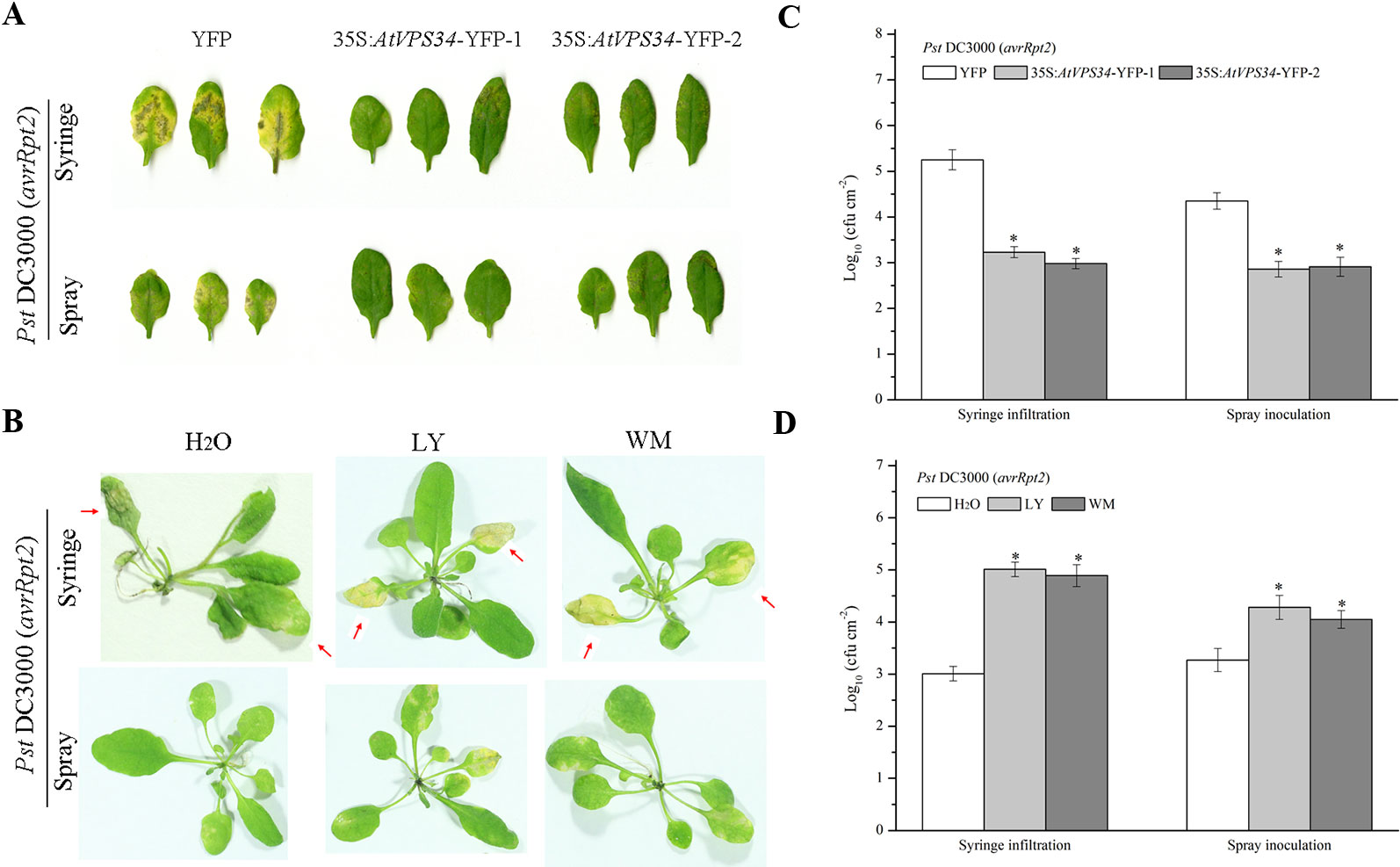
Figure 1 PI3K functioned in plant immunity against Pst DC3000 (avrRpt2). (A) 35Spro::AtVP34-YFP Arabidopsis and 35Spro::YFP Arabidopsis were spray-inoculated or syringe-infiltrated with Pst DC3000 (avrRpt2) for 3 days, Lesion phenotypes in Arabidopsis leaves was taken. Pictures represent typical examples. And the corresponding bacterial growth quantification of spray-inoculated or syringe-infiltrated leaves was shown in (C). (B) WT Arabidopsis was pretreated either with 30 μM LY or 10 μM WM or not for 1 day, then syringe-infiltrated with Pst DC3000 (avrRpt2) for 1 day or spray-inoculated with Pst DC3000 (avrRpt2) for 2 days. Lesion phenotypes in Arabidopsis leaves was taken. The red arrow indicated the leaves infiltrated. Pictures represent typical examples. The corresponding bacterial growth quantification of spray-inoculated or syringe-infiltrated leaves was shown in (D). Each bar is the mean ± SD of three biological replications. Asterisks (*) indicates significant difference by t test from 35Spro::YFP Arabidopsis or no PI3K inhibitors treatment (P < 0.05; Student's t test). PI3K, phosphoinositide 3-kinase; LY, LY294002; WM, wortmannin.
PI3K Functioned in Stomatal Immunity During Pst DC3000 (AvrRpt2) and Pst DC3000 Infection
Stomata are the first line of defense that prevents bacterial infection (Melotto et al., 2017). In previous study, PI3K was proved to participate in regulation of stomatal movement (Liu et al., 2005; Kale et al., 2010; Bhattacharjee et al., 2012). For clarify the possible role of PI3K in stomatal immunity, 35Spro::YFP Arabidopsis and 35Spro::AtVP34-YFP Arabidopsis were exposed to light for at least 3 h to enlarge the stomatal aperture, and then sprayed Arabidopsis leaves with Pst DC3000 (avrRpt2) or Pst DC30000. Stomatal aperture was determined 1 and 3 h after spray inoculation. It was shown that Pst DC3000 (avrRpt2) and Pst DC3000 induced stomatal closure at 1 hour and recovered at 3 h after spray inoculation. Additionally, no significant difference of stomatal aperture was found between 35Spro::AtVP34-YFP lines and 35Spro::YFP Arabidopsis with water treatment (Figure 2A). However, stomatal closure was enhanced in 35Spro::YFP Arabidopsis lines compared with that in 35Spro::YFP Arabidopsis, when spray-inoculated with Pst DC3000 (avrRpt2) or Pst DC3000 (Figure 2A and Figure S3A). And PI3K inhibitors blocked stomatal closure in stomatal immunity (Figure 2B and Figure S3B). These results indicated that PI3K was involved in stomatal immunity against Pst DC3000 (avrRpt2) and Pst DC3000 infection.
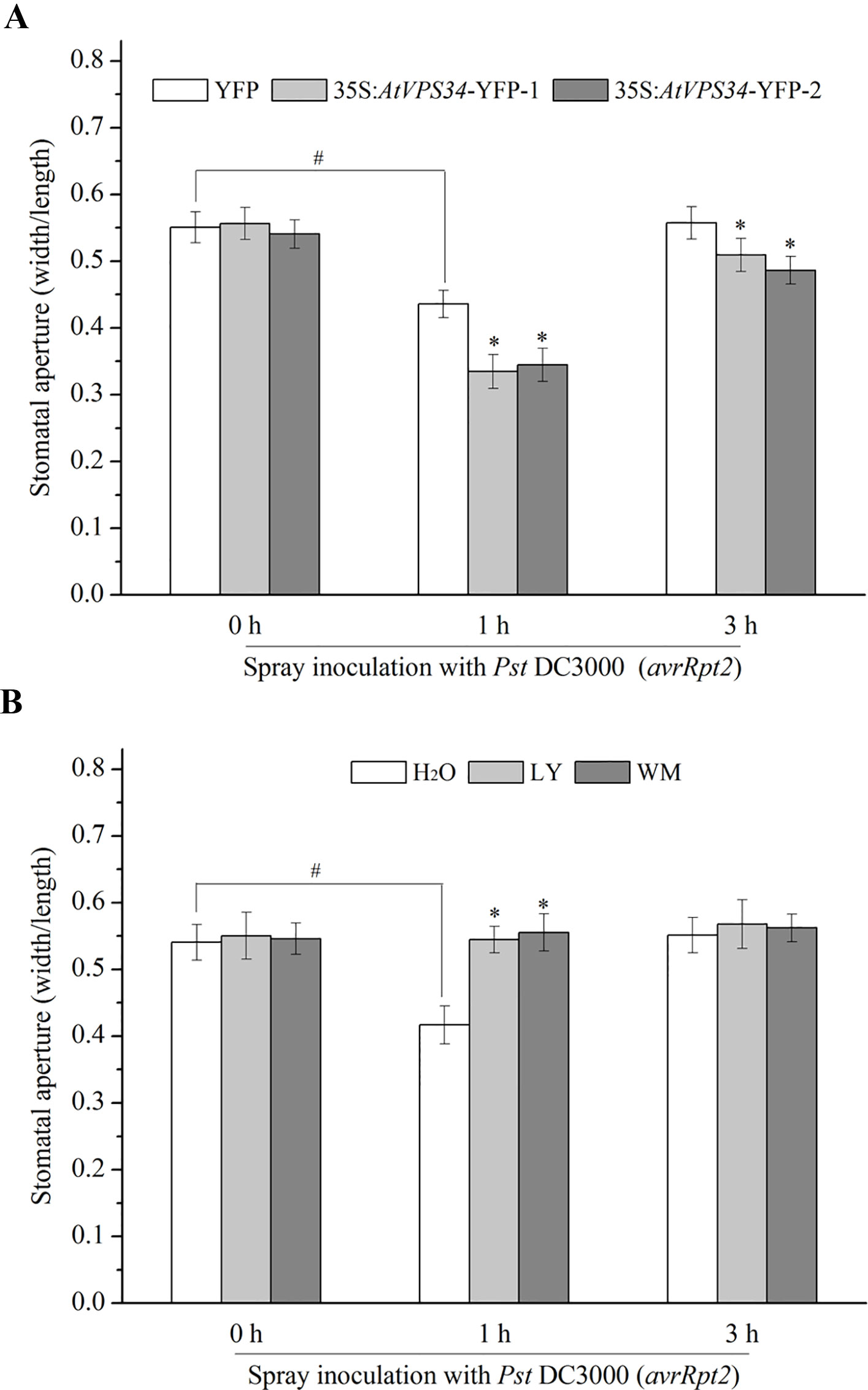
Figure 2 PI3K functioned in stomatal immunity against Pst DC3000 (avrRpt2). (A) 35Spro::AtVP34-YFP Arabidopsis and 35Spro::YFP Arabidopsis were taken in light for at least 3 h, (B) WT Arabidopsis was pretreated either with 30 μM LY or 10 μM WM or not for 24 h, then transport the plant under light for at least 3 h. The full expanded young leaves were immersed in water or Pst DC3000 (avrRpt2) suspension (108 cfu/ml in water). 1 and 3 h after spray inoculation, epidermis of three leaves was peeled off and immediately observed under a microscope. The stomatal aperture was represented as the ratio of width to length. Each bar is the mean ± SD of three biological replications (n > 30). Asterisks (*) indicate statistically significant differences from control in the indicated times (P < 0.05; Student's t test). Hash marks (#) indicate statistically significant differences between indicated samples (P < 0.05; Student's t test). PI3K, phosphoinositide 3-kinase; LY, LY294002; WM, wortmannin.
Effect of Exogenous Phytohormone Supply on AtVPS34 Expression When Syringe-Infiltrated With Pst DC3000 (AvrRpt2)
Phytohormones play a vital role in plant resistance against bacterial invasion. Previous studies revealed that PI3K functioned as a common platform for multi-hormone signaling to trigger intracellular response (Hirsch et al., 2007). To investigate the link PI3K and phytohormone in plant immunity, Arabidopsis leaves were pretreated either with water, 10 μM ABA, 1 mM SA, 1 mM MeJA, 20 μM BL, 100 μM IAA or 10 μM GA for 24 h, then syringe-infiltrated with Pst DC3000 (avrRpt2). Gene expression of AtVPS34 was strongly induced after syringe inoculation with avirulent Pst DC3000 (avrRpt2) for 12 h compared with that in water treatment. Although the supply of exogenous phytohormone such as SA, MeJA, ABA, and GA promoted AtVPS34 gene expression when syringe-infiltrated with water, only SA significantly increased AtVPS34 gene expression while other phytohormones suppressed it after syringe inoculation with avirulent Pst DC3000 (avrRpt2) compared with that in no phytohormone treatment (Figure 3A).
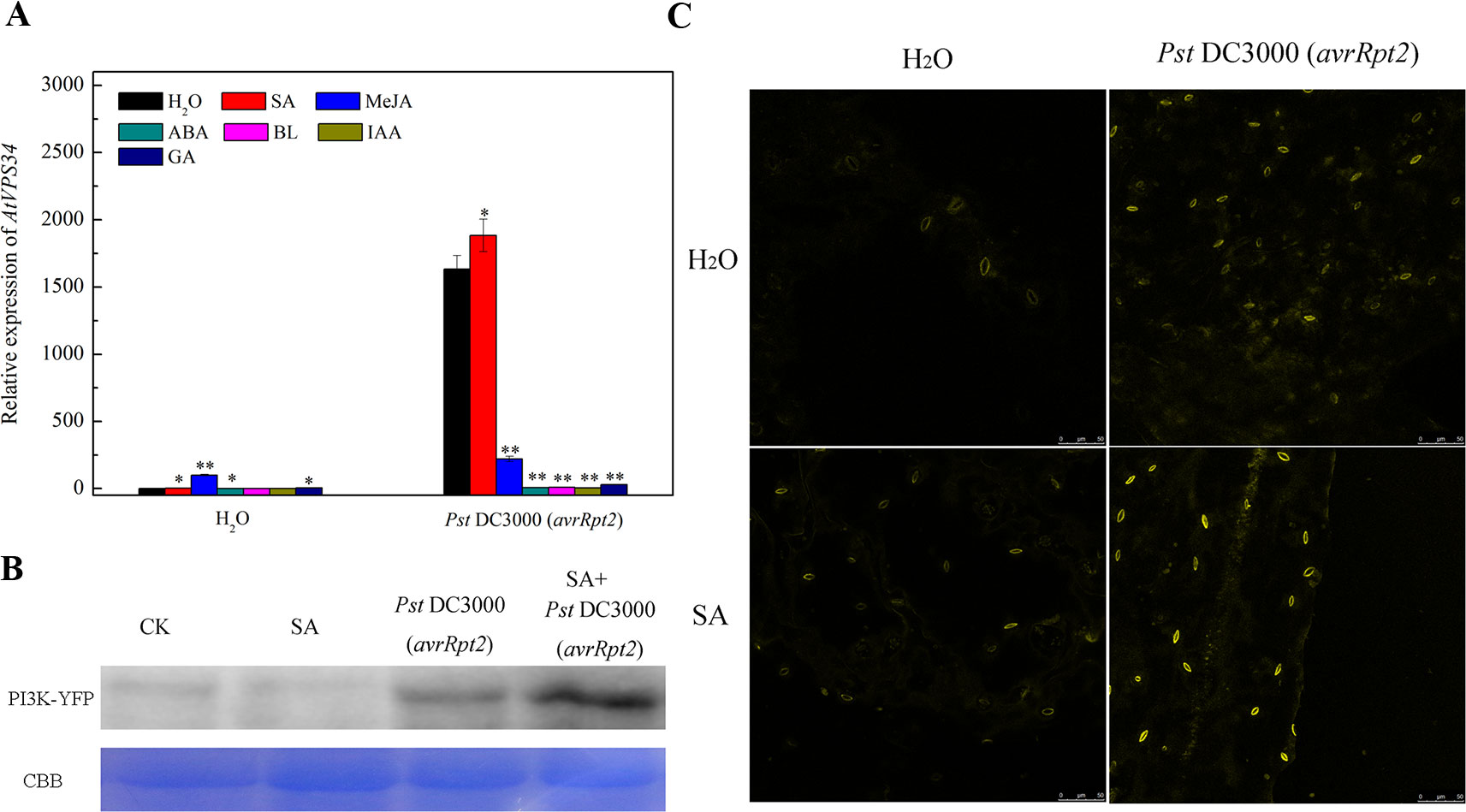
Figure 3 The effect of exogenous phytohormone supply on AtVPS34 gene expression against Pst DC3000 (avrRpt2). (A) three-week-old Arabidopsis leaves were pretreated either with water (H2O) or 10 μM abscisic acid (ABA) or 1 mM salicylic acid (SA) or 1 mM methyl jasmonate (MeJA) or 20 μM brassinolide (BL) or 100 μM indol-yl-3-acetic acid (IAA) or 10 μM gibberellin (GA) for 1 day, then syringe-infiltrated with Pst DC3000 (avrRpt2). 12 h after infiltration, RNA was extracted from leaves, AtVPS34 gene expression was examined by qPCR. AtActin2 was used as an internal control. Each bar is the mean ± SD of three biological replications. Asterisks (*) indicate a significant difference from the treatment with water at *P < 0.05 or **P < 0.01 by t-test. (B) and (C) 35Spro::AtVP34-YFP-1 Arabidopsis was pretreated with 1 mM SA or not, then syringe-infiltrated with Pst DC3000 (avrRpt2). Western blots showing PI3K levels 1 day after infiltration (B). Coomassie staining of the large subunit of Rubisco was used as a loading control. CBB, Coomassie brilliant blue staining. (C) YFP fluorescence was also examined one day after infiltration. Results shown are representative. PI3K, phosphoinositide 3-kinase.
To further investigate the role of SA on PI3K signaling activation in response to bacterial infection, 35Spro::AtVP34-YFP-1 Arabidopsis was pretreated with 1 mM SA or not, then syringe-infiltrated with Pst DC3000 (avrRpt2). The expression of PI3K was examined by western blot (Figure 3B) and fluorescent analysis (Figure 3C) 1 day after infiltration. The expression of PI3K was enhanced after Pst DC3000 (avrRpt2) infiltration, and exogenous SA further increased PI3K expression in bacterial invasion. These results indicated that SA induce PI3K signaling in plant resistance against Pst DC3000 (avrRpt2) invasion.
PI3K Enhanced Pathogenesis Related Genes Expression in Plant Resistance Against Pst DC3000 (AvrRpt2) Invasion
Pathogenesis related (PR) PR1 and PR5 genes are late marker genes for Arabidopsis defense response (van Loon et al., 2006). Transgenic PR1pro::GUS Arabidopsis plants were used to examine PR1 gene expression. In the group without PI3K inhibitors pretreatment, syringe infiltration with Pst DC3000 (avrRpt2) induced GUS signal at 12 h after infiltration and GUS signal was enhanced at 24 h after infiltration. In contrast, PI3K inhibitors inhibited PR1 gene expression after syringe infiltration with Pst DC3000 (avrRpt2) in the first 24 h (Figure 4A). Moreover, similar results were found for PR1 gene expression by RT-qPCR analysis (Figure 4B).
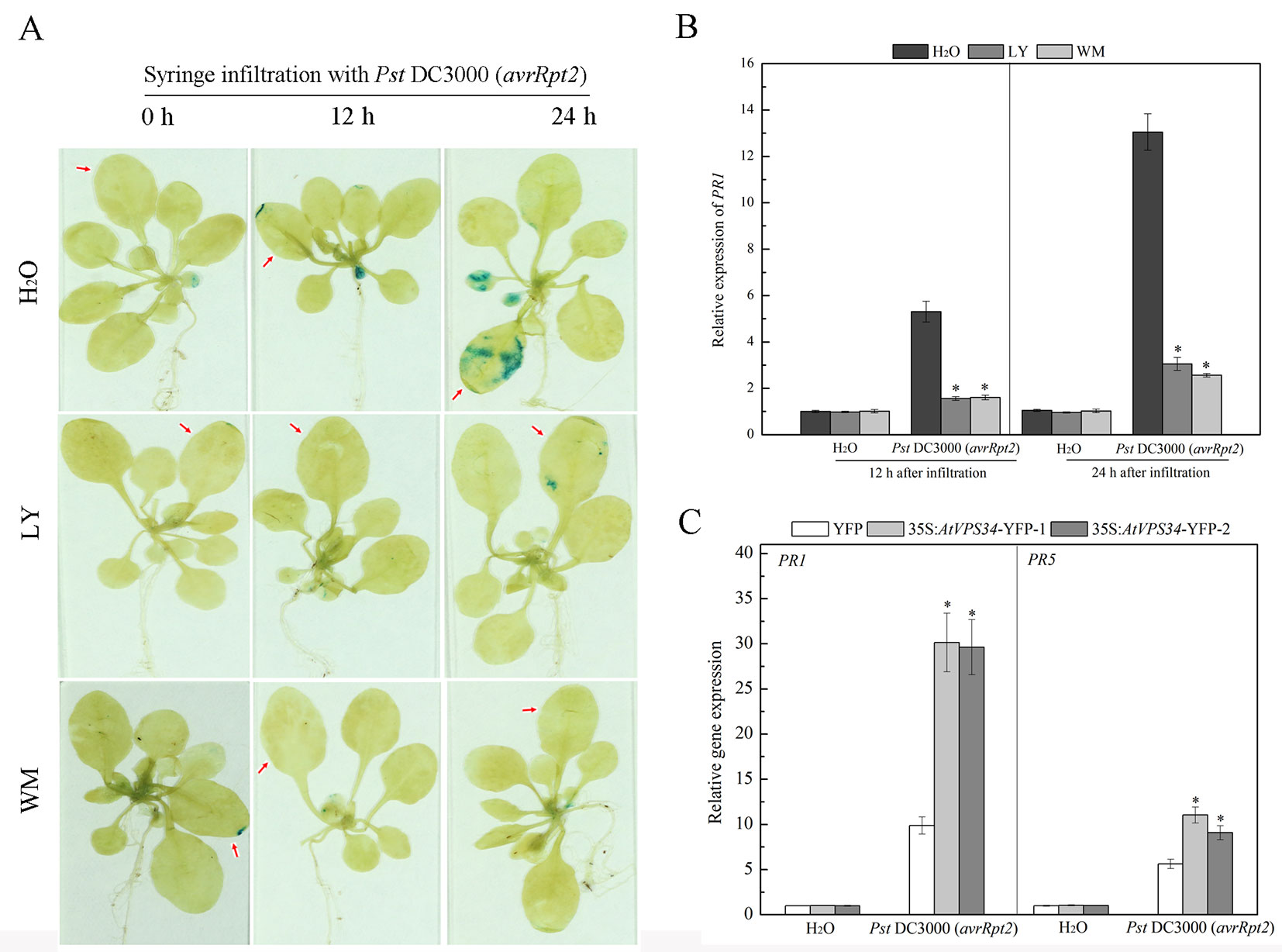
Figure 4 PI3K enhanced pathogen-related genes expression in resistance against Pst DC3000 (avrRpt2) invasion. (A) Approximately 3-week-old transgenic PR1pro::GUS Arabidopsis was syringe-infiltrated with Pst DC3000 (avrRpt2), 12 and 24 h after inoculation, histochemical detection of GUS enzyme activity was performed. The red arrow indicated the leaves infiltrated. Results shown are representative. (B) WT Arabidopsis was pretreated either with 30 μM LY or 10 μM WM or not for 24 h, then syringe-infiltrated with Pst DC3000 (avrRpt2) for 12 h or 24 h, AtPR1 gene expression was examined by qPCR. Each bar is the mean ± SD of three biological replications. Asterisks (*) indicate statistically significant differences from the treatment with water (P < 0.05; Student's t test). (C) 35Spro::AtVP34-YFP Arabidopsis and 35Spro::YFP Arabidopsis were syringe-infiltrated with Pst DC3000 (avrRpt2) for 24 h, gene expression of AtPR1 and AtPR5 was determined by qPCR. Each bar is the mean ± SD of three biological replications. Asterisks (*) indicate statistically significant differences from 35Spro::YFP Arabidopsis (P < 0.05; Student's t test). PI3K, phosphoinositide 3-kinase; LY, LY294002; WM, wortmannin.
PR genes expression was also examined in AtVPS34 overexpressing Arabidopsis under bacterial infection. As shown in Figure 4C, overexpression of AtVPS34 enhanced PR1 and PR5 gene expression compared with that in 35Spro::YFP Arabidopsis after syringe infiltration with Pst DC3000 (avrRpt2). These results indicated that PI3K had a positive role in transcription response of PR genes in plant resistance against Pst DC3000 (avrRpt2) invasion.
PI3K Induced ROS Signaling in Plant Resistance Against Pst DC3000 (AvrRpt2) Invasion
Previous studies have revealed that ROS plays a vital role in plant immunity (Lambeth, 2004) and PI3K regulates ROS production in various physiological events and stress responses (Lee et al., 2010). Therefore, 1 and 3 h after bacteria invasion, syringe-infiltrated and spray-inoculated leaves were collected. As shown in Figure 5, the content of hydrogen peroxide was significantly increased at 1 h after spray-inoculation and declined at 3 h after spray-inoculation in 35Spro::YFP Arabidopsis, while syringe infiltration with Pst DC3000 (avrRpt2) induced a progressive increase of hydrogen peroxide. However, overexpression of AtVPS34 further upregulated the content of hydrogen peroxide compared with that in 35Spro::YFP Arabidopsis after bacterial invasion.
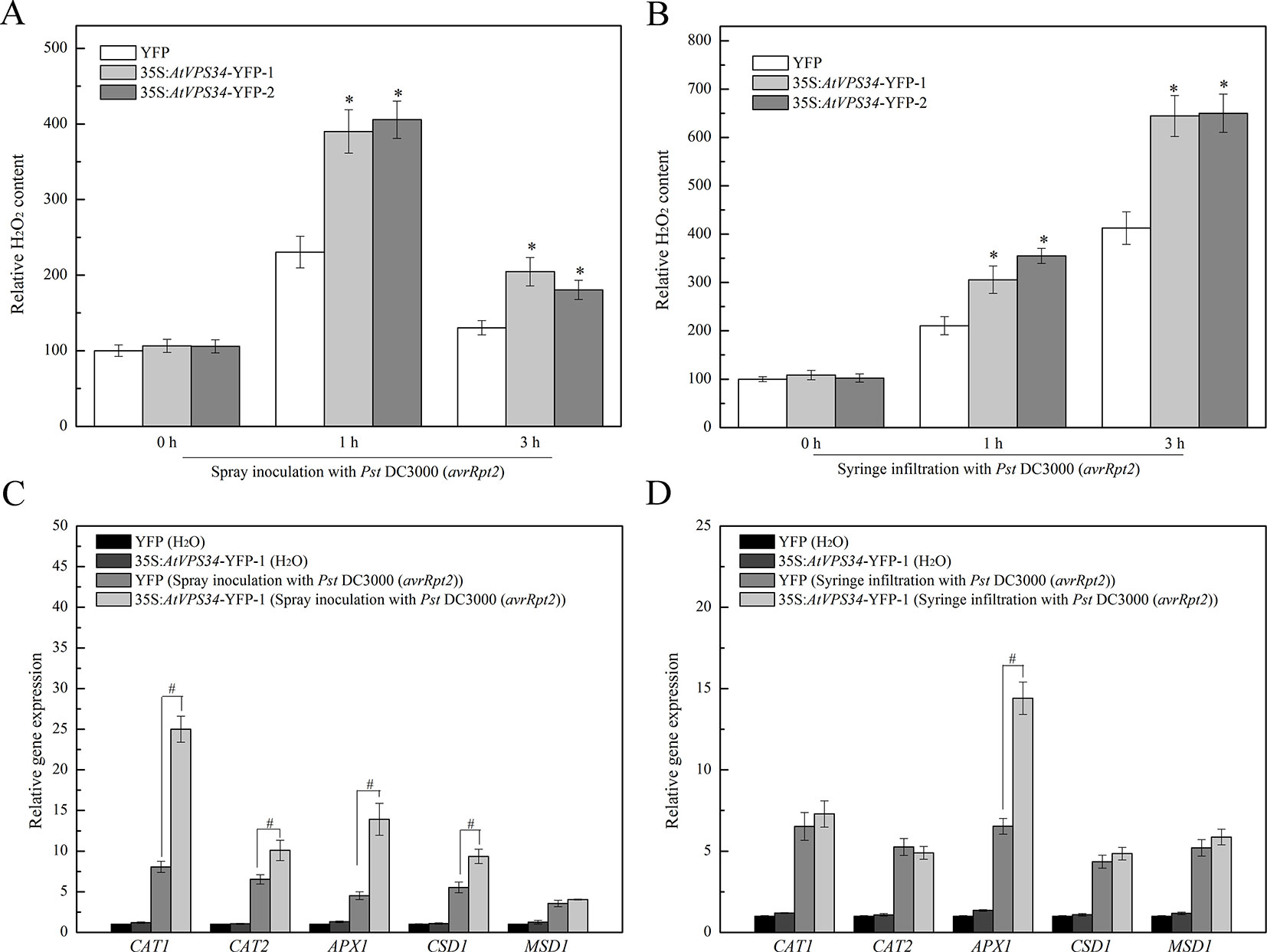
Figure 5 PI3K induced ROS signaling in Arabidopsis resistance against Pst DC3000 (avrRpt2) invasion. 1 and 3 h after invasion, the spray-inoculated (A) and syringe-infiltrated (B) leaves of 35Spro::AtVP34-YFP Arabidopsis and 35Spro::YFP Arabidopsis were collected, the H2O2 content was assayed using a colorimetric hydrogen peroxide assay kit from Beyotime. (C, D) 35Spro::AtVP34-YFP Arabidopsis and 35Spro::YFP Arabidopsis were spray-inoculated or syringe-infiltrated with Pst DC3000 (avrRpt2) for 24 h, then CAT1, CAT2, APX1, CSD1, and MSD1 genes expression were determined by qPCR. Each bar is the mean ± SD of three biological replications. Hash marks (#) indicate statistically significant differences between indicated samples (P < 0.05; Student's t test). PI3K, phosphoinositide 3-kinase.
Additionally, to further reveal the key role of PI3K in ROS signaling, the expression of genes encoding antioxidant enzymes was also examined. Most of genes encoding antioxidant enzymes were upregulated after spray inoculation with Pst DC3000 (avrRpt2) (Figure 5C), and gene expression of APX1 was increased after syringe infiltration with Pst DC3000 (avrRpt2) (Figure 5D). It seems that PI3K was involved in plant immunity via promoting ROS production.
Discussion
In this study, we investigated the role of PI3K in plant immunity against Pst DC3000 (avrRpt2). Overexpression of AtVPS34 enhanced plant resistance against Pst DC3000 and Pst DC3000 (avrRpt2) invasion. Further analysis showed that stomatal immunity was enhanced by the overexpression of AtVPS34. In addition, exogenous SA treatment enhanced AtVPS34 expression and PR gene expression was significantly induced by the overexpression of AtVPS34 after syringe infiltration with Pst DC3000 (avrRpt2). Moreover, the content of hydrogen peroxide and the expression of genes encoding antioxidant enzyme were increased when inoculated with Pst DC3000 (avrRpt2) regardless of syringe or spray. These results indicated a novel role of PI3K in plant immunity.
Plant effective immune responses are activated through various phytohormones signaling such as jasmonic acid, ethylene, Abscisic acid, auxin, gibberellins, cytokinin brassinolide (An and Mou, 2011). Most phytohormones have been reported to trigger PI3K signaling. In seed germination, PI3K is a positive regulator of GA signaling (Liu et al., 2012). And ABA and Auxin-induced ROS production also requires the activation of PI3K (Park et al., 2003; Joo et al., 2005). Moreover, PI3K is involved in MeJA-induced leaf senescence (Hung et al., 2006; Hung and Kao, 2007; Liu et al., 2016). Increasing evidences indicated that PI3K functioned as a common platform for multi-hormone signaling to trigger intracellular response (Hirsch et al., 2007). For network regulation of phytohormone in plant immunity, PI3K seems to play a complex role in plant-pathogen interactions. We speculated that the timing of infection might be a crucial element in the regulatory role of PI3K on pathogen defense.
The role of ABA in plant immunity is complex. In Arabidopsis, ABA-regulated stomatal closure is a key element of pre-invasion SA-regulated innate immunity to Pseudomonas syringae (Melotto et al., 2006). COR counteracts PAMP-induced stomatal closure downstream of ABA (Xie et al., 1998). And our previous study showed that one unknown upstream signaling of PI3K initiated the antagonistic effect on JA signaling (Liu et al., 2016). Although gene expression of AtPI3K was inhibited by ABA upon syringe-infiltrated with Pst DC3000 (avrRpt2) (Figure 1), we cannot rule out the possibility that ABA regulated the PI3K signaling in stomatal immunity. Moreover, guard cell ABA could activate ROS-generating NADPH oxidases (Kwak et al., 2006), which are also necessary for ROS production during pathogen defense (Torres and Dangl, 2005). However, the exact role of ABA on PI3K signaling should be determined by future.
Stomata functions as innate immunity gates to actively prevent bacterial entry in plant immunity (Melotto et al., 2006). If Pst DC3000 fails to enter leaf tissues after spray-inoculation, it dies quickly (Xin and He, 2013). In eukaryocyte, the activation of PI3K signaling is tightly associated with primary metabolism. Upon glucose stimulation, PI3K regulated V-ATPase activation (Sautin et al., 2005). PI(3)P production by VPS34 is stimulated by amino acid (Byfield et al., 2005; Yoon et al., 2016). And previous study also revealed a significant change of primary metabolites in bacterial invasion. Fructose showed more than a threefold increase at 30 min, and most amino acids showed a decrease at 180 min after pathogen infection (Pang et al., 2018). Therefore, we speculated that PI3K signaling might be activated by sugar and amino acid signaling in the early stage of bacterial invasion. Once the accumulation of sugar and amino acid was decreased, PI3K signaling was diminished. Thus, we found the overexpression of PI3K recovered to almost the same level as control plants in stomatal reopening. However, the enhanced closure of stomata induced by PI3K in the early stage of bacterial invasion is still very important for plant defense. For one thing, PI3K signaling senses the danger of potential bacterial invasion more effectively and take a more effective restriction of bacterial entry through the epidermis. For another, this stomatal regulation might be necessary for the priming induction (Ramirez-Prado et al., 2018). Primed plants are in a heightened state of defense and produce a stronger defensive response when challenged (Singh et al., 2012).
Our results revealed ROS content was enhanced by overexpression of AtVPS34 after syringe infiltration or spray inoculation with Pst DC3000 (avrRpt2). ROS production is thought to be directly toxic to pathogens in animal immunity (Lambeth, 2004), and restricts pathogen entry by triggering stomatal closure (Su et al., 2017). It seems that PI3K regulates stomatal immunity by promoting ROS accumulation. There is multiple pathways for ROS production in guard cells (Song et al., 2014). Our former study revealed that PI3K could regulate the activity of NADPH oxidase in seed germination (Liu et al., 2012). And upon perception of PAMPs, NADPH oxidase RbohD could be activated by plasma-associated kinase BIK1 to induce ROS production (Su et al., 2017). We speculated that NADPH oxidase was one of the important sources of ROS regulated by PI3K. ROS burst triggers an activation of MAPKs signaling and an increase in the concentration of cytosolic calcium, leading to activation of ion channels and modification of cellular turgor, thus closure of the stomatal pores (Balmant et al., 2016). Moreover, cytoskeleton plays a vital role in Arabidopsis guard cell architecture, thus pathogen manipulation of actin within the stomata might be implicated in having a role in immune subversion (Porter and Day, 2015). Growing evidence showed that PI3K and its production PI3P modulate actin filament reorganization (Choi et al., 2008; Li et al., 2008). Thus, PI3K might regulate stomatal immunity by modulating the dynamic of cytoskeleton. Of course, all these speculations should be investigated in future studies.
Once bacteria enter leaf tissues, SA possibly enhances PI3K signaling. Our experiment showed that AtVPS34 was expressed in leaf epidermal and guard cells, especially in guard cell. And exogenous SA further enhanced PI3K expression in stomata after syringe infiltration. It seems that PI3K-mediated guard cell signaling regulates not only stomatal immunity, but also other type of plant defense. Previous study showed ETI is an accelerated and amplified PTI response, resulting in disease resistance and usually an HR PCD at the infection site (Jones and Dangl, 2006). Plant autophagy operated negative feedback loop modulating SA signaling to negatively regulate immunity-related PCD (Yoshimoto et al., 2009). Therefore, SA is hyperaccumulated in L-methionine sulfoximine-induced cell death in NbPI3K-RNAi plant (Sumida et al., 2017). However, AvrRpt2-triggered HR PCD may be independent of autophagy and may require other cell death processes (Hofius et al., 2009). Thus, the negative feedback of PI3K on the SA production might not be existed in avirulent Pst DC3000 (AvrRpt2) infected Arabidopsis. Nevertheless, the effect of PI3K on the ROS production is important. Therefore, enhancement the ROS content and expression of genes encoding antioxidant enzyme by overexpression of AtVPS34 in the process of Pst DC3000 (avrRpt2) infection (avrRpt2) (Figure 4) might result in initiation of PR1 and PR5 gene expression. Plant immunity to avirulent pathogen is usually associated with subcellular membrane dynamics, such as fusion between the vacuolar and plasma membranes (Hatsugai et al., 2018). And previous study has been revealed that YFP-2xFYVE, a fluorescent PI3P-specific biosensor, strongly labelled the vacuolar membrane in leaf epidermal and guard cells (Vermeer et al., 2006). Therefore, it is interesting to investigate the role of PI3K on membrane traffic in plant resistance to avirulent bacteria invasion in the future.
In this study, previously unknown role of PI3K in bacterial resistance was unraveled. Our results showed that PI3K promotes the process of stomatal immunity and play a positive role in SA-induced immunity possibly via the regulation of ROS production. We found that PI3K could play a positive role in Arabidopsis against avirulent Pst DC3000 (avrRpt2) and Pst DC3000 infection. Given a key role of PI3K in plant immunity, we propose that analysis of the effect of PI3K on cytoskeleton dynamic and ROS production may provide novel important information for the control of bacterial disease.
Data Availability Statement
All datasets generated for this study are included in the article/Supplementary Material.
Author Contributions
HZ and JL designed the research. HZ, XL, XZ, NQ, KX, WY, YZ and JL performed research. HZ, YS, RZ and JL contributed analytic and computational tools. HZ, XL, XZ, NQ, KX, WY, YZ, YS, RZ and JL analyzed data. HZ, RZ and JL wrote the paper. All authors read and approved the final manuscript.
Funding
This research was supported by the China Postdoctoral Science Foundation (2019M652233), the Natural Science Foundation of Fujian Province of China (2016J01102; 2017J01425), the Young and Middle-aged Teachers Education Scientific Research Project of Fujian Provincial Department of Education (JAT160163; JAT160176), the Fujian Agriculture and Forestry University (Xjq201617), and the School International Science and Technology Cooperation and Exchange Project (KXB16009A).
Conflict of Interest
The authors declare that the research was conducted in the absence of any commercial or financial relationships that could be construed as a potential conflict of interest.
Supplementary Material
The Supplementary Material for this article can be found online at: https://www.frontiersin.org/articles/10.3389/fpls.2019.01740/full#supplementary-material
References
Abeliovich, H., Klionsky, D. J. (2001). Autophagy in yeast: mechanistic insights and physiological function. Microbiol. Mol. Biol. Rev. 65, 463–479. doi: 10.1128/MMBR.65.3.463-479.2001
Alvarez, M. E., Pennell, R. I., Meijer, P. J., Ishikawa, A., Dixon, R. A., Lamb, C. (1998). Reactive oxygen intermediates mediate a systemic signal network in the establishment of plant immunity. Cell 82, 773–784. doi: 10.1016/S0092-8674(00)81405-1
An, C. F., Mou, Z. L. (2011). Salicylic acid and its function in plant immunity. J. Integr. Plant Biol. 53, 412–428. doi: 10.1111/j.1744-7909.2011.01043.x
Balmant, K. M., Zhang, T., Chen, S. (2016). Protein phosphorylation and redox modification in stomatal guard cells. Front. Physiol. 7, 26. doi: 10.3389/fphys.2016.00026
Bhattacharjee, S., Stahelin, R. V., Speicher, K. D., Speicher, D. W., Haldar, K. (2012). Endoplasmic reticulum PI(3)P lipid binding targets malaria proteins to the host cell. Cell 148, 201–212. doi: 10.1016/j.cell.2011.10.051
Bunney, T. D., Watkins, P. A., Beven, A. F., Shaw, P. J., Hernandez, L. E., Lomonossoff, G. P., et al. (2000). Association of phosphatidylinositol 3-kinase with nuclear transcription sites in higher plants. Plant Cell 12, 1679–1688. doi: 10.1105/tpc.12.9.1679
Byfield, M. P., Murray, J. T., Backer, J. M. (2005). hVps34 is a nutrient-regulated lipid kinase required for activation of p70 S6 kinase. J. Biol. Chem. 280, 33076–33082. doi: 10.1074/jbc.M507201200
Choi, Y., Lee, Y., Jeon, B. W., Staiger, C. J., Lee, Y. (2008). Phosphatidylinositol 3- and 4-phosphate modulate actin filament reorganization in guard cells of day flower. Plant Cell Environ. 31, 366–377. doi: 10.1111/j.1365-3040.2007.01769.x
Ellson, C., Davidson, K., Anderson, K., Stephens, L. R., Hawkins, P. T. (2006). PtdIns3P binding to the PX domain of p40phox is a physiological signal in NADPH oxidase activation. EMBO J. 25, 4468–4478. doi: 10.1038/sj.emboj.7601346
Fruman, D. A., Cantley, L. C. (2002). Phosphoinositide 3-kinase in immunological systems. Semin. Immunol. 14, 7–18. doi: 10.1006/smim.2001.0337
Hatsugai, N., Nakatsuji, A., Unten, O., Ogasawara, K., Kondo, M., Nishimura, M., et al. (2018). Involvement of adapter protein complex 4 in hypersensitive cell death induced by avirulent bacteria. Plant Physiol. 176, 1824–1834. doi: 10.1104/pp.17.01610
Hirsch, E., Costa, C., Ciraolo, E. (2007). Phosphoinositide 3-kinases as a common platform for multi-hormone signaling. J. Endocrinol. 194, 243–256. doi: 10.1677/JOE-07-0097
Hofius, D., Schultz-Larsen, T., Joensen, J., Tsitsigiannis, D. I., Petersen, N. H., Mattsson, O., et al. (2009). Autophagic components contribute to hypersensitive cell death in Arabidopsis. Cell 137, 773–783. doi: 10.1016/j.cell.2009.02.036
Hung, K. T., Kao, C. H. (2007). The participation of hydrogen peroxide in methyl jasmonate-induced NH4+ accumulation in rice leaves. J. Plant Physiol. 164, 1469–1479. doi: 10.1016/j.jplph.2006.10.005
Hung, K. T., Hsu, Y. T., Kao, C. H. (2006). Hydrogen peroxide is involved in methyl jasmonate-induced senescence of rice leaves. Physiol. Plant 127, 293–303. doi: 10.1111/j.1399-3054.2006.00662.x
Hung, C. Y., Aspesi, P., Jr., Hunter, M. R., Lomax, A. W., Perera, I. Y. (2014). Phosphoinositide-signaling is one component of a robust plant defense response. Front. Plant Sci. 5, 267. doi: 10.3389/fpls.2014.00267
Jones, J. D. G., Dangl, J. L. (2006). The plant immune system. Nature 444, 323. doi: 10.1038/nature05286
Joo, J. H., Yoo, H. J., Hwang, I., Lee, J. S., Nam, K. H., Bae, Y. S. (2005). Auxin-induced reactive oxygen species production requires the activation of phosphatidylinositol 3-kinase. FEBS Lett. 579, 1243–1248. doi: 10.1016/j.febslet.2005.01.018
Jung, J. Y., Kim, Y. W., Kwak, J. M., Hwang, J. U., Young, J., Schroeder, J. I., et al. (2002). Phosphatidylinositol 3- and 4-Phosphate are required for normal stomatal movements. Plan Cell 14, 2399–, 2412.doi: 10.1105/tpc.004143
Kale, S. D., Gu, B., Capelluto, D. G. S., Dou, D. L., Feldman, E., Rumore, A., et al. (2010). External lipid PI3P mediates entry of eukaryotic pathogen effectors into plant and animal host cells. Cell 142, 284–295. doi: 10.1016/j.cell.2010.06.008
Kwak, J. M., Nguyen, V., Schroeder, J. I. (2006). The role of reactive oxygen species in hormonal responses. Plant Physiol. 141, 323–329. doi: 10.1104/pp.106.079004
Lambeth, J. D. (2004). NOX enzymes and the biology of reactive oxygen. Nat. Rev. Immunol. 4, 181. doi: 10.1038/nri1312
Lee, Y., Kim, E. S., Choi, Y., Hwang, I., Staiger, C. J., Chung, Y. Y., et al. (2008). The Arabidopsis phosphatidylinositol 3-kinase is important for pollen development. Plant Physiol. 147, 1886–1897. doi: 10.1104/pp.108.121590
Lee, Y., Munnik, T., Lee, Y. (2010). “Plant phosphatidylinositol 3-kinase,” in Lipid signaling in plants (Berlin, Heidelberg: Springer).
Leprince, A. S., Magalhaes, N., De Vos, D., Bordenave, M., Crilat, E., Clément, G., et al. (2015). Involvement of phosphatidylinositol 3-kinase in the regulation of proline catabolism in Arabidopsis thaliana. Front. Plant Sci. 5, 772.doi: 10.3389/fpls.2014.00772
Li, L., Saga, N., Mikami, K. (2008). Phosphatidylinositol 3-kinase activity and asymmetrical accumulation of F-actin are necessary for establishment of cell polarity in the early development of monospores from the marine red alga Porphyra yezoensis. J. Exp. Bot. 59, 3575–3586. doi: 10.1093/jxb/ern207
Liu, J., Zhou, J., Xing, D. (2012). Phosphatidylinositol 3-Kinase plays a vital role in regulation of rice seed vigor via altering NADPH Oxidase activity. PloS One 7, e33817. doi: 10.1371/journal.pone.0033817
Liu, J., Ji, Y. B., Zhou, J., Xing, D. (2016). Phosphatidylinositol 3-Kinase promotes V-ATPase activation and vacuolar acidification and delays methyl jasmonate-Induced leaf senescence. Plant Physiol. 170, 1714–1731. doi: 10.1104/pp.15.00744
Liu, Y. L., Schiff, M., Czymmek, K., Tallóczy, Z., Levine, B., Dinesh-Kumar, S. P. (2005). Autophagy regulates programmed cell death during the plant innate immune response. Cell 121, 567–577. doi: 10.1016/j.cell.2005.03.007
Melotto, M., Underwood, W., Koczan, J., Nomura, K., He, S. Y. (2006). Plant stomata funvtion in innate immunity against bacterial invasion. Cell 126, 969–980. doi: 10.1016/j.cell.2006.06.054
Melotto, M., Zhang, L., Oblessuc, P. R., He, S. Y. (2017). Stomatal defense a decade later. Plant Physiol. 174, 561–571. doi: 10.1104/pp.16.01853
Pang, Q., Zhang, T., Wang, Y., Kong, W., Guan, Q., Yan, X., et al. (2018). Metabolomics of early stage plant cell–microbe interaction using stable isotope labeling. Front. Plant Sci. 9, 760. doi: 10.3389/fpls.2018.00760
Park, K. Y., Jung, J. Y., Park, J., Hwang, J. U., Kim, Y. W., Hwang, I., et al. (2003). A role for phosphatidylinositol 3-Phosphate in abscisic acid-induced reactive oxygen species generation in guard cells. Plant Physiol. 132, 92–98. doi: 10.1104/pp.102.016964
Payrastre, B., Gaits-Iacovoni, F., Ansonetti, S. P., Tronchère, H. (2012). “Phosphoinositides and cellular pathogens,” in Phosphoinositides II: the diverse biological functions. Eds. Balla, T., Wymann, M., York, J. D. (Dordrecht: Springer).
Porter, K., Day, B. (2015). From filaments to function: the role of the plant actin cytoskeleton in pathogen perception, signaling and immunity. J. Integr. Plant Biol. 58, 299–311. doi: 10.1111/jipb.12445
Ramirez-Prado, J. S., Abulfaraj, A. A., Rayapuram, N., Benhamed, M., Hirt, H. (2018). Plant immunity: from signaling to epigenetic control of defense. Trends Plant Sci. 23, 833–844. doi: 10.1016/j.tplants.2018.06.004
Sautin, Y. Y., Lu, M., Gaugler, A., Zhang, L., Gluck, S. L. (2005). Phosphatidylinositol 3-kinase-mediated effects of glucose on vacuolar H+-ATPase assembly, translocation, and acidification of intracellular compartments in renal epithelial cells. Mol. Cell. Biol. 25, 575–589. doi: 10.1128/MCB.25.2.575-589.2005
Singh, P., Kuo, Y. C., Mishra, S., Tsai, C. H., Chien, C. C., Chen, C. W., et al. (2012). The lectin receptor kinase-VI. 2 is required for priming and positively regulates Arabidopsis pattern-triggered immunity. Plant Cell 24, 1256–1270. doi: 10.1105/tpc.112.095778
Song, Y., Miao, Y., Song, C. P. (2014). Behind the scenes: the roles of reactive oxygen species in guard cells. New Phytol. 201, 1121–1140. doi: 10.1111/nph.12565
Su, J., Zhang, M., Zhang, L., Sun, T., Liu, Y., Lukowitz, W., et al. (2017). Regulation of stomatal immunity by interdependent functions of a pathogen-responsive MPK3/MPK6 cascade and abscisic acid. Plant Cell 29, 526–542. doi: 10.1105/tpc.16.00577
Sumida, S., Ito, M., Galis, I., Nakatani, H., Shinya, T., Ohnishi, K., et al. (2017). Phosphoinositide 3-kinase participates in L-methionine sulfoximine-induced cell death via salicylic acid mediated signaling in Nicotiana benthamiana. J. Plant Physiol. 218, 167–170. doi: 10.1016/j.jplph.2017.07.016
Sun, A. Z., Nie, S. J., Xing, D. (2012). Nitric oxide-mediated maintenance of redox homeostasis contributes to NPR1-dependent plant innate immunity triggered by lipopolysaccharides. Plant Physiol. 160, 1081–1096. doi: 10.1104/pp.112.201798
Takáč, T., Pechan, T., Samajová, O., Ovečka, M., Richter, H., Eck, C., et al. (2012). Wortmannin treatment induces changes in Arabidopsis root proteome and post-Golgi compartments. J. Proteome. Res. 11, 3127–3142. doi: 10.1021/pr201111n
Torres, M. A., Dangl, J. L. (2005). Functions of the respiratory burst oxidase in biotic interactions, abiotic stress and development. Curr. Opin. Plant Biol. 8, 397–403. doi: 10.1016/j.pbi.2005.05.014
van Loon, L. C., Rep, M., Pieterse, C. M. (2006). Significance of inducible defense-related proteins in infected plants. Annu. Rev. Phytopathol. 44, 135–162. doi: 10.1146/annurev.phyto.44.070505.143425
Vermeer, J. E., van Leeuwen, W., Tobeña-Santamaria, R., Laxalt, A. M., Jones, D. R., Divecha, N., et al. (2006). Visualization of PtdIns3P dynamics in living plant cells. Plant J. 47, 687–700. doi: 10.1111/j.1365-313X.2006.02830.x
Walker, E. H., Pacold, M. E., Perisic, O., Stephens, L., Hawkins, P. T., Wymann, M. P., et al. (2000). Structural determinants of phosphoinositide 3-kinase inhibition by wortmannin, LY294002, quercetin, myricetin, and staurosporine. Mol. Cell. 6, 909–919. doi: 10.1016/S1097-2765(05)00089-4
Xie, D. X., Feys, B. F., Ames, J. S., Nieto-Rostro, M., Turner, J. G. (1998). COI1: an Arabidopsis gene required for jasmonate-regulated defense and fertility. Science 280, 1091–1094. doi: 10.1126/science.280.5366.1091
Xin, X. F., He, S. Y. (2013). Pseudomonas syringae pv. tomato DC3000: a model pathogen for probing disease susceptibility and hormone signaling in plants. Annu. Rev. Phytopathol. 51, 473–498. doi: 10.1146/annurev-phyto-082712-102321
Yi, S. Y., Shirasu, K., Moon, J. S., Lee, S. G., Kwon, S. Y. (2014). The activated SA and JA signaling pathways have an influence on flg22-triggered oxidative burst and callose deposition. PloS One 9, e88951. doi: 10.1371/journal.pone.0088951
Yoon, M. S., Son, K., Arauz, E., Han, J. M., Kim, S., Chen, J. (2016). Leucyl-tRNA synthetase activates Vps34 in amino acid-sensing mTORC1 signaling. Cell Rep. 16, 1510–1517. doi: 10.1016/j.celrep.2016.07.008
Yoshimoto, K., Jikumaru, Y., Kamiya, Y., Kusano, M., Consonni, C., Panstruga, R., et al. (2009). Autophagy negatively regulates cell death by controlling NPR1-dependent salicylic acid signaling during senescence and the innate immune response in Arabidopsis. Plant Cell 21, 2914–2927. doi: 10.1105/tpc.109.068635
Yuan, H. M., Liu, W. C., Lu, Y. T. (2017). CATALASE2 coordinates SA-mediated repression of both auxin accumulation and JA biosynthesis in plant defenses. Cell Host Microbe 21, 143–155. doi: 10.1016/j.chom.2017.01.007
Keywords: phosphoinositide 3-kinase, stomatal immunity, reactive oxygen species, phytohormone, PR gene1
Citation: Zhang H, Liu X, Zhang X, Qin N, Xu K, Yin W, Zheng Y, Song Y, Zeng R and Liu J (2020) Phosphoinositide 3-Kinase Promotes Oxidative Burst, Stomatal Closure and Plant Immunity in Bacterial Invasion. Front. Plant Sci. 10:1740. doi: 10.3389/fpls.2019.01740
Received: 28 June 2019; Accepted: 11 December 2019;
Published: 24 January 2020.
Edited by:
Keiko Yoshioka, University of Toronto, CanadaReviewed by:
Hong-Gu Kang, Texas State University, United StatesTong Zhang, Pacific Northwest National Laboratory, United States
Copyright © 2020 Zhang, Liu, Zhang, Qin, Xu, Yin, Zheng, Song, Zeng and Liu. This is an open-access article distributed under the terms of the Creative Commons Attribution License (CC BY). The use, distribution or reproduction in other forums is permitted, provided the original author(s) and the copyright owner(s) are credited and that the original publication in this journal is cited, in accordance with accepted academic practice. No use, distribution or reproduction is permitted which does not comply with these terms.
*Correspondence: Rensen Zeng, cnN6ZW5nQHNjYXUuZWR1LmNu; Jian Liu, bGl1amlhbl90b255QDE2My5jb20=