- Agroécologie, AgroSup Dijon, INRA, Univ. Bourgogne Franche-Comté, Dijon, France
Higher temperatures induced by the on-going climate change are a major cause of yield reduction in legumes. Pea (Pisum sativum L.) is an important annual legume crop grown in temperate regions for its high seed nitrogen (N) concentration. In addition to yield, seed N amount at harvest is a crucial characteristic because pea seeds are a source of protein in animal and human nutrition. However, there is little knowledge on the impacts of high temperatures on plant N partitioning determining seed N amount. Therefore, this study investigates the response of seed dry matter and N fluxes at the whole-plant level (plant N uptake, partitioning in vegetative organs, remobilization, and accumulation in seeds) to a range of air temperature (from 18.4 to 33.2°C) during the seed-filling-period. As pea is a legume crop, plants relying on two different N nutrition pathways were grown in glasshouse: N2-fixing plants or NO3−-assimilating plants. Labeled nitrate (15NO3−) and intra-plant N budgets were used to quantify N fluxes. High temperatures decreased seed-filling duration (by 0.8 day per °C), seed dry-matter and N accumulation rates (respectively by 0.8 and 0.032 mg seed−1 day−1 per °C), and N remobilization from vegetative organs to seeds (by 0.053 mg seed−1 day−1 per °C). Plant N2-fixation decreased with temperatures, while plant NO3− assimilation increased. However, the additional plant N uptake in NO3−-assimilating plants was never allocated to seeds and a significant quantity of N was still available at maturity in vegetative organs, whatever the plant N nutrition pathway. Thus, we concluded that seed N accumulation under high temperatures is sink limited related to a shorter seed-filling duration and a reduced seed dry-matter accumulation rate. Consequently, sustaining seed sink demand and preserving photosynthetic capacity of stressed plants during the seed-filling period should be promising strategies to promote N allocation to seeds from vegetative parts and thus to maintain crop N production under exacerbated abiotic constraints in field due to the on-going climate change.
Introduction
Temperature is one of the main environmental factors explaining the variations in seed yield and quality in annual crop plants, especially legumes (Wheeler et al., 2000; Peng et al., 2004; Schlenker and Roberts, 2009; Asseng et al., 2011; Sita et al., 2017). The observed global increase in temperature (1.0°C of global warming above pre-industrial levels) is projected to continue by 0.2°C per decade due to past and ongoing emissions (including greenhouse gases) (IPCC, 2018). High temperatures are thus expected to be more frequent during the reproductive period of crops in temperate climate. They are already a major cause of the recent yields stagnation and projected decline due to the climatic change in Europe (Brisson et al., 2010; Supit et al., 2012; Trnka et al., 2012).
Pea (Pisum sativum L.) is an important annual legume crop grown in temperate regions for its high seed nitrogen (N) concentration. Including legumes in rotations leads to environmental benefits thanks to their unique capacity to acquire N via atmospheric N2 symbiotic fixation (Jensen and Hauggaard-Nielsen, 2003; Nemecek et al., 2008; Siddique et al., 2012). However, to extend the pea crop area in Europe, pea yield and seed protein concentration should be increased as well as their stability, especially in fluctuating climatic conditions (Siddique et al., 2012; Vadez et al., 2012).
Nitrogen yield (product of the yield and the seed N concentration) is a crucial characteristic at harvest in pea because seeds are a source of protein in animal and human nutrition. During the reproductive phase, N partitioning is the key process involved in the modulation of N yield. In most grain crops and, above all, in legumes, newly acquired N is generally low and insufficient to fulfill the high N demand of seeds, consequently endogenous N previously accumulated in vegetative parts is exported to seeds (Sinclair and Wit, 1976; Salon et al., 2001; Malagoli et al., 2005; Schiltz et al., 2005; Kichey et al., 2007; Barraclough et al., 2014). This remobilized N derives from the proteolysis of essential leaf proteins involved in photosynthesis, mostly Rubisco (Gregersen et al., 2008; Masclaux-Daubresse et al., 2008). The resulting decrease in leaf photosynthetic capacity may thus limit yield by shortening the duration of the seed-filling period (Sinclair and Horie, 1989; Munier-Jolain et al., 2008; Bueckert et al., 2015). Nitrogen remobilization not only affects yield, but also N yield since N remobilized from vegetative parts is the major contributor to seed N in most grain crops (Malagoli et al., 2005; Schiltz et al., 2005; Kichey et al., 2007; Araujo et al., 2012).
High temperatures affect plant phenology and carbon metabolism through various processes such as hastening reproductive development (Badeck et al., 2004; Barnabas et al., 2008; Bueckert et al., 2015; Sita et al., 2017), reducing photosynthesis (Guilioni et al., 2003; Kirschbaum, 2004; Sage and Kubien, 2007; Pimentel et al., 2013; Tacarindua et al., 2013), and reducing seed set (Guilioni et al., 2003; Djanaguiraman et al., 2013; Edreira and Otegui, 2013; Tacarindua et al., 2013; Bueckert et al., 2015). Conversely, impacts of high temperatures on assimilate partitioning remain unclear, especially concerning their effect on N remobilization to filling seeds. Some authors reported a decrease in N remobilization from vegetative parts to filling grain in response to heat stress in wheat (Triticum aestivum L.) (Tahir and Nakata, 2005) and in rice (Oryza sativa) (Ito et al., 2009). On the contrary, other authors suggest that high temperatures increase N remobilization from vegetative organs to seeds causing an acceleration of senescence (Spiertz, 1977; Morison and Lawlor, 1999; Masclaux-Daubresse et al., 2008; Zhao et al., 2011; Wu et al., 2012). Moreover, high temperatures may also affect N uptake of legumes (mainly acquired via N2 fixation), but unfortunately little is known about temperate legume crops (Bordeleau and Prevost, 1994).
Further investigations are thus needed to improve the understanding of the effect of high temperatures on N assimilate partitioning during the seed-filling period and to quantify the impact on seed N yield in legumes. For this purpose, the present study therefore assessed the response of seed dry matter and N fluxes at the whole-plant level (seed N accumulation, N remobilization, plant N uptake, and N amount variation in vegetative organs) to contrasting temperature ranging from permissive to heat stress during the seed-filling period of pea (Guilioni et al., 2003). We compared N2-fixing and NO3−-assimilating plants, the first being more representative of field conditions while the later allow the use of a 15NO3−-labeled nutrient solution to assess N fluxes.
Materials and Methods
Plant Material and Growth Conditions
Three different glasshouse experiments (Exp. 1, 2, and 3) were conducted. One single line of spring dry pea (cv. Baccara) has been used, all plants were genetically identical. Baccara characteristics are described in Bourion et al. (2002a; 2002b). Pea seeds were sown in 5 L pots at a density of eight plants per pot. Pots were filled with a 1:1 (v/v) mixture of sterilized attapulgite and clay balls (diameter 2–6 mm) in Exp. 1 and 3 or with a mixture of 1/6 vermiculite, 1/3 siliceous sand, and 1/2 clay balls (diameter 2–6 mm) in Exp. 2. After seedling establishment the plants were thinned to the four most homogeneous per pot. Plant N nutrition relied exclusively on NO3− assimilation in Exp. 1 and 2 due to the high nitrate availability of the nutrient solution (14 meq NO3−, P, K, and micronutrients; Table S1). In Exp. 3, pea plant N nutrition relied exclusively on N2 fixation due to a nutrient solution without nitrate (0 meq NO3−, P, K, and micronutrients; Table S1) and an inoculation. Seedlings were inoculated with 1ml of Rhizobacterium leguminosarum bv. Vicieae, strain P221 (MIAE01212, 108 bacteria per plant), the strain usually used in the laboratory because of its good efficiency in particular with cv. Baccara (Voisin et al., 2013).
Photosynthetic active radiation was provided to the plants by day light and mercury lamps (MACS 400 W; Mazda, Dijon, France) with a 14-h day length. The air temperature was recorded every 5 min in order to calculate the mean daily temperature. Prior to the different temperature treatments, the glasshouse temperature was maintained at a day/night temperature of 24/16°C.
Temperature Treatments During the Seed-Filling Period
The experiments aimed at testing the effect of temperature during the seed-filling period. As peas are indeterminate plants with a sequential flowering up the stem leading to a wide heterogeneity of seed developmental stages, the temperature treatments started at the beginning of seed filling of the last reproductive node (BSL) and ended when seed physiological maturity was reached at the whole-plant level, as described by Larmure et al. (2005).
At BSL, different sets of pots were randomly transferred to glasshouses maintained at the different day/night temperatures until plant physiological maturity. The air temperature treatments tested in Exp. 1, 2, and 3 ranged approximately from 20/16°C to 35/31°C day/night (Table 1). In Exp. 1 and 2 monitoring NO3−-assimilating plants, respectively four and three day/night temperatures were chosen in order to form a range of seven temperatures. In Exp. 3 monitoring N2-fixing plants, four day/night temperatures were chosen in order to form a temperature range similar to that tested for -assimilating plants.
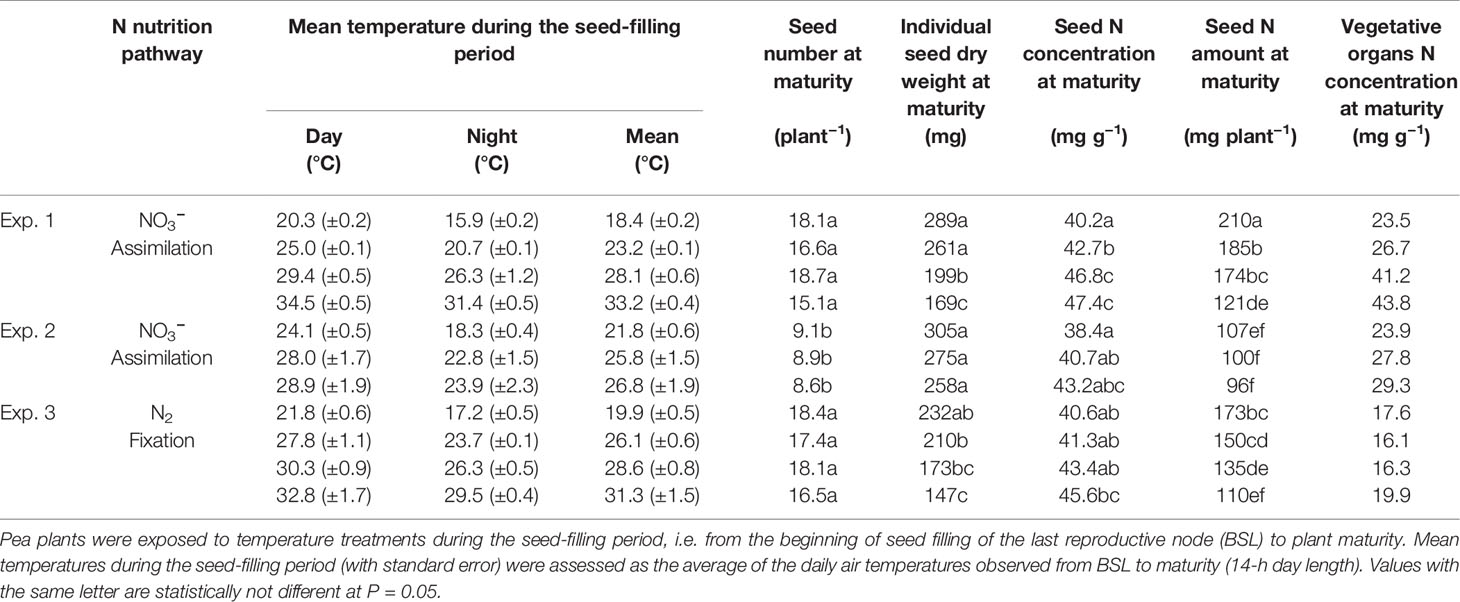
Table1 Glasshouse experiments characteristics and seed number, individual seed dry weight, seed N concentration and amount, and vegetative organs N concentration at maturity.
The temperatures were modified gradually during two acclimatization days to reach the temperature objectives of each treatment. All temperature treatments are described in Table 1 including the average of mean air temperatures actually observed in the glasshouses (ranging from 18.4 to 33.2°C). Plants were maintained at the maximum soil water capacity by providing non-limiting water availability with an automatic watering system.
Plant Sampling and Measurements
Prior to the different temperature treatments, seed water concentration was destructively measured at each node twice a week to assess the date of BSL.
For each temperature treatment, randomly chosen pots were harvested: (1) at the beginning of the temperature treatment, (2) during the temperature treatment, and (3) after plant physiological maturity (three pots per treatment for Exp. 1 or five pots per treatment for Exp. 2 and 3). At each sampling date, seeds, leaves, stems, pod walls, and roots were collected separately. Dry matters, seed number, and water concentration were determined as described by Larmure et al. (2005).
In Exp. 1 and 2, total N concentrations and 15N enrichments were determined using a dual inlet mass spectrometer coupled with a CHN analyzer (Sercon, ANCA-GSL-2020). In Exp. 3, total N concentrations were determined with an elemental analyser (Carlo Erba).
Determination of N Fluxes
Nitrogen fluxes (seed N accumulation, endogenous-N remobilization, plant exogenous-N uptake, and N amount variation in vegetative organs) were expressed in mg seed−1 day−1. This unit is adequate to depict N partitioning to seeds in plants, because the individual seed N accumulation rate depends on N available per seed (N from endogenous-remobilization and exogenous sources) (Lhuillier-Soundélé et al., 1999; Larmure and Munier-Jolain, 2004). Moreover, this unit allows to compare N fluxes in plants differing in seed number and vegetative parts biomass.
Plant 15N Labeling and Calculation of N Fluxes for -Assimilating Plants
15N labeling sessions with NO3−-assimilating plants (Exp. 1 and 2) were used to distinguish the remobilization of endogenous-14N stored before labeling from the exogenous-15N uptake supplied by 15NO3− nutritive solution with 5% 15N APE (atom percent excess) enrichment. Successive 3-day labeling sessions were conducted during the temperature treatments as described by Schiltz et al. (2005). Homogenous groups of six pots for Exp. 1 or 10 pots in Exp. 2 were constituted and randomly used for the each labeling session. The first labeling session began at the end of the two acclimatization days. In Exp. 1, three successive labeling sessions were conducted for all temperature treatments, except for the warmest treatment permitting only two labeling sessions due to an earlier physiological maturity. In Exp. 2, two successive labeling sessions were conducted for all temperature treatments. At the beginning of each session, unlabeled control pots were harvested (three pots for Exp. 1 or five pots for Exp. 2). During the session, labeled pots were supplied during three days with the 15NO3− nutritive solution and harvested (three pots for Exp. 1 or five pots for Exp. 2).
For -labeled assimilating plants, N fluxes were assessed using the data of the labeling sessions. Rates of plant N uptake, seed N accumulation, endogenous-N remobilization to filling seeds, and variation of N amount in each vegetative organ during a labeling session were calculated using the total N concentrations and the 15N enrichment of the labeling nutrient solution (5 %) as described by Schiltz et al. (2005). Each N flux value represents the mean value of the two or the three 3-day labeling sessions. Values resulted from the measurement of three (Exp. 1) or five (Exp. 2) biological replicates, each consisting of one pot with four plants.
Calculation of N Fluxes for N2-Fixing Plants
For unlabeled N2-fixing plants (Exp. 3), rates of plant N uptake, seed N accumulation, and variation of N amount in each vegetative organ were assessed as the linear regressions coefficients of each variable (plant N, seed N, and vegetative organ N amounts, respectively) v. time (expressed in days). Values resulted from the measurement of five biological replicates, each consisting of one pot with four plants. Endogenous-N remobilized to filling seeds could not be determined in Exp. 3 using unlabeled N2-fixing plants.
Statistical Analysis
The experiments were conducted with completely randomized design with three (Exp. 1) or five (Exp. 2 and 3) biological replications. Each biological replication consisting of one pot with four plants (one single line, cv. Baccara). Data were analyzed using SigmaPlot® 12 (Systat Software, Inc.). All data obtained were subjected to analysis of variance. Differences at P ≤ 0.05 were considered significant.
Results
Seed Number, Individual Seed Dry Weight, Seed N Amount, and Seed N Concentration At Maturity
Seed number per plant at maturity was not significantly different among temperature treatments within an experiment (Table 1). Seed N amount at maturity and individual seed dry weight decreased in response to increasing temperatures in all three experiments (Table 1). On the contrary, seed N concentration increased with the increase in temperature in all experiments (Table 1). These changes of seed characteristics at maturity were significant for Exp. 1 and 3, that explored a wider range of mean daily air temperature during the seed-filling period than Exp. 2 (Table 1).
Seed number per plant at maturity was significantly different between experiments: it was lower in Exp. 2 than in Exp. 1 and 3 (Table 1), as was total seed dry matter (Table S2). And thus, seed N amount at maturity was also lower in Exp. 2 than in Exp. 1 and 3 (Table 1).
Response of Seed Dry Matter Accumulation and Seed N Accumulation to the Increase in Temperature
Individual seed dry matter accumulation during the seed-filling period decreased linearly with increasing temperature for both NO3−-assimilating and N2-fixing plants (data from the three experiments gathered) by 19.6 mg seed−1 per °C, from 227.8 mg seed−1at 18.4°C to 26.5 mg seed−1 at 33.2°C (R2 = 0.95) (Figure 1A). Individual seed dry matter accumulation was assessed as the product of the seed-filling duration and the rate of seed dry matter accumulation during the temperature treatments. Both variables significantly decreased with increasing temperature for the three experiments and for both plant N nutrition pathways (Figures 2A, B). The seed-filling duration was reduced progressively by 0.8 day for each additional °C (Figure 2A). Similarly, the rate of seed dry matter accumulation decreased progressively by 0.8 mg seed−1 day−1 per °C from 19.8 mg seed−1 day−1 at 18.4°C to 5 mg seed−1 day−1 at 33.2°C (Figure 2B).
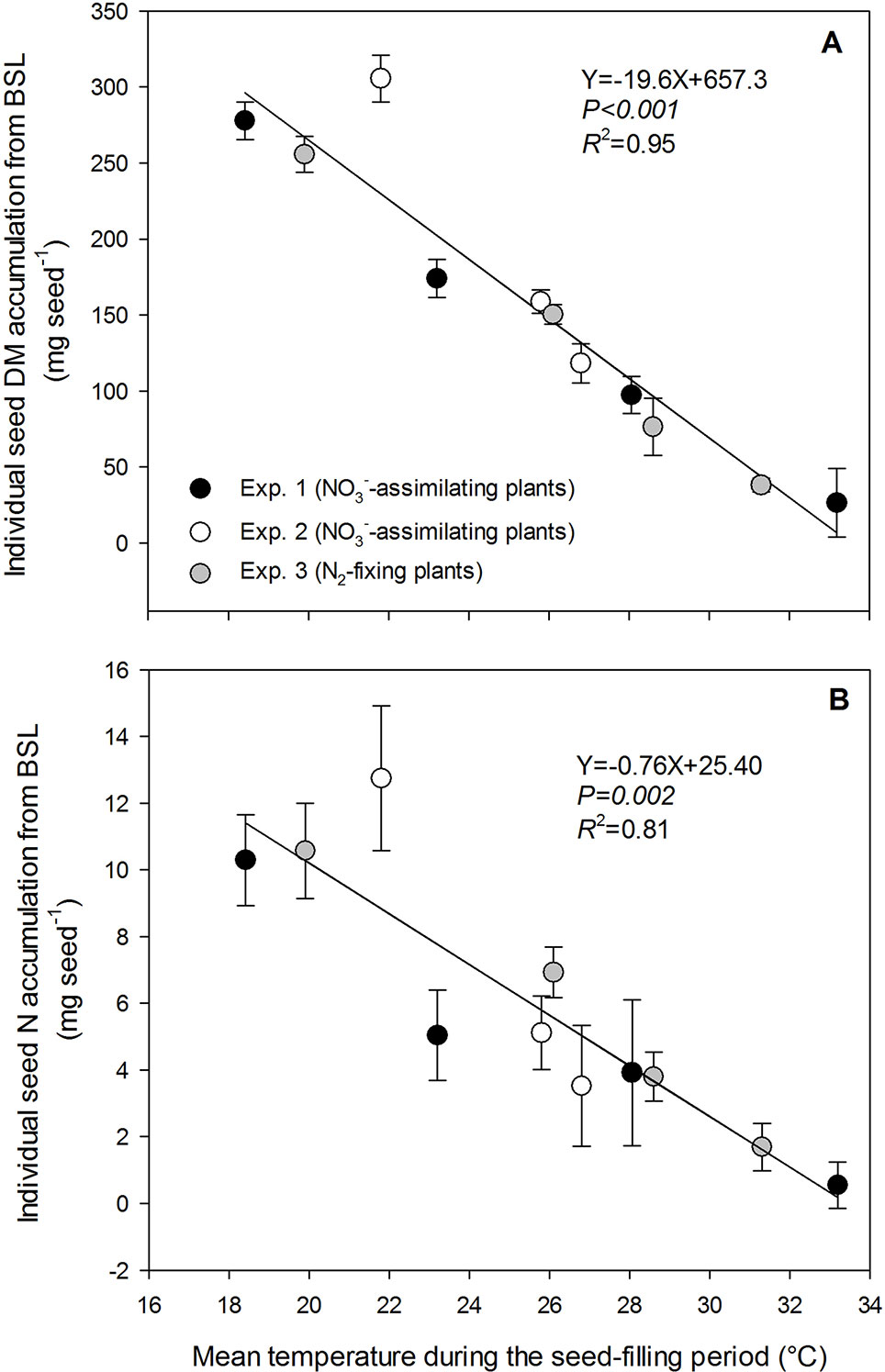
Figure 1 Decrease in individual seed dry matter accumulation (A) and individual seed nitrogen accumulation (B) with increasing temperature of the treatments during the seed-filling period. Pea plants were exposed to temperature treatments from the beginning of seed filling of the last reproductive node (BSL) to plant maturity. The vertical bars represent SE (when larger than symbol). The data were fitted with a linear regression.
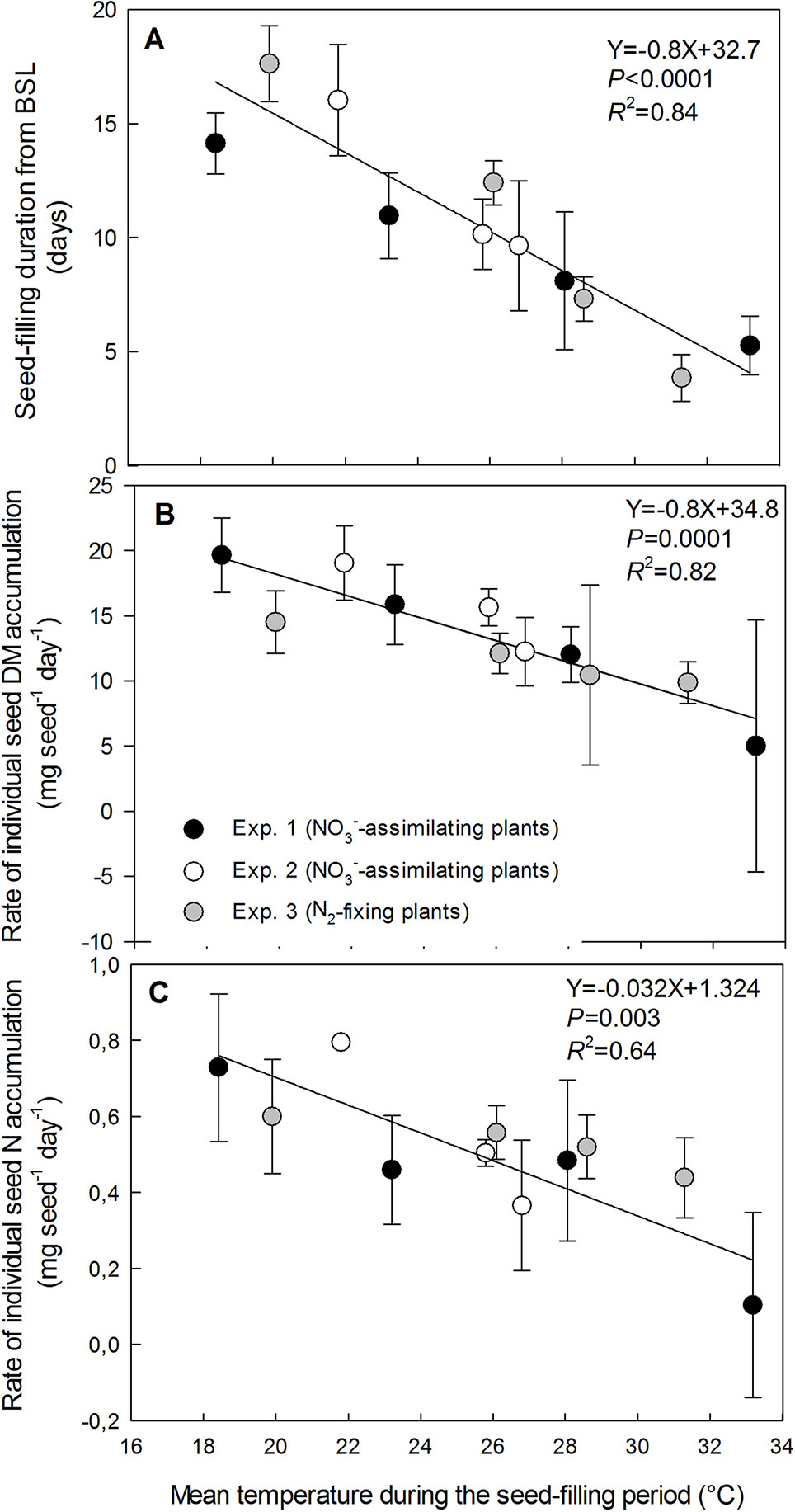
Figure 2 Decrease in seed-filling duration (A), the rate of individual seed dry-matter accumulation (B) and the rate of individual seed nitrogen-accumulation (C) with increasing temperature of the treatments during the seed-filling period. The vertical bars represent SE (when larger than symbol). The data were fitted with a linear regression.
Individual seed N accumulation during the temperature treatments decreased linearly with increasing temperature for both NO3−-assimilating and N2-fixing plants (data from the three experiments gathered) by 0.76 mg seed−1 per °C from 10.3 mg N seed−1at 18.4°C to 0.55 mg N seed−1 at 33.2°C (R2 = 0.81) (Figure 1B). Seed N accumulation was assessed as the product of the seed-filling duration and the rate of seed N accumulation during the temperature treatments. Both variables significantly decreased with increasing temperature from 18.4°C to 33.2°C, for the three experiments and both plant N nutrition pathways (Figures 2A, C). The rate of seed N accumulation decreased progressively by 0.032 mg seed−1 day−1 per °C from 0.73 mg seed−1 day−1 at 18.4°C to 0.10 mg seed−1 day−1 at 33.2°C (Figure 2C).
Effect of High Temperatures on the Remobilization of Endogenous-N to Filling Seeds by NO3−-Assimilating Plants
Endogenous-N remobilization to filling seeds was measured on labeled NO3−-assimilating plants in Exp. 1 and 2. The contribution of remobilized N to the rate of seed N accumulation exceeded 82 % in both experiments with NO3−-assimilating plants (Exp. 1 and 2) for all temperatures (Figures 2B and 3). The temperature increase dramatically decreased the rate of N remobilization to filling seeds from 0.71 mg seed−1 day−1 at 18.4°C to 0 at 33.2°C (Figure 3). The detrimental effect of increasing temperature suggests a full stop of N remobilization at a temperature around 33°C (intersection of regression and X-axis in Figure 3).
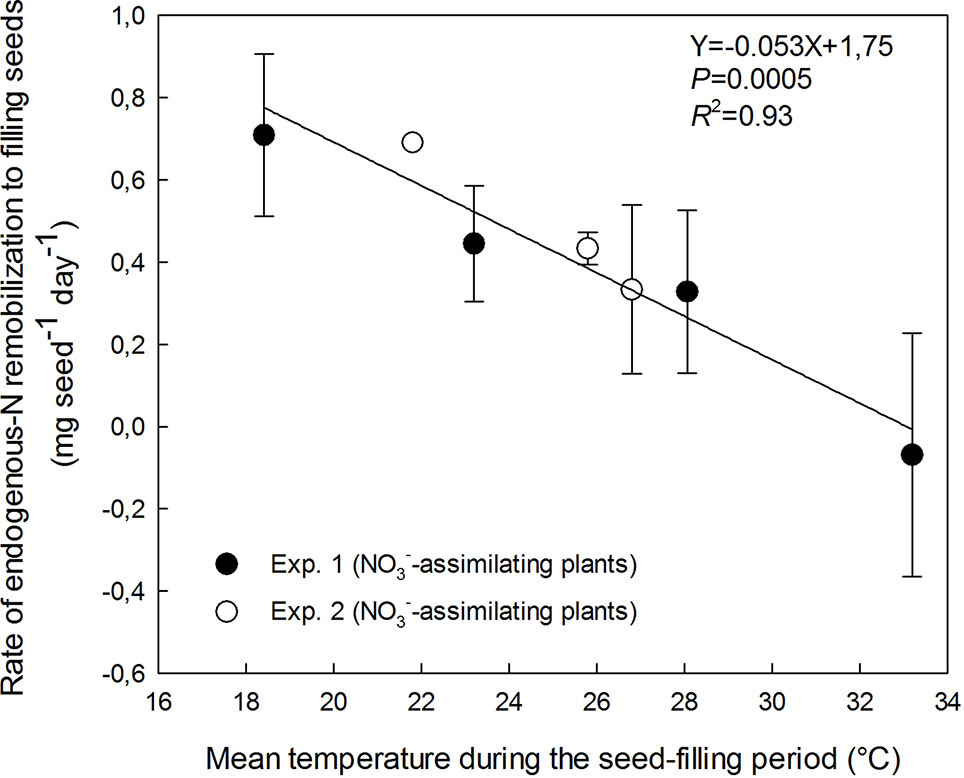
Figure 3 Decrease in the rate of endogenous-N remobilization from vegetative parts (roots, pod walls, stems, and leaves) to filling seeds with increasing temperature of the treatments during the seed-filling period. The vertical bars represent SE (when larger than symbol). The data were fitted with a linear regression.
Effects of High Temperatures on the Plant N Uptake by NO3−-Assimilating and N2-Fixing Plants
The rate of plant N uptake during the seed-filling varied between 0.11 and 0.64 mg seed−1 day−1 whatever the plant nutrition pathway. The variation range of the plant N uptake rate for N2-fixing plants was included in the variation range for NO3−-assimilating plants.
The rate of plant N uptake relying exclusively on NO3− assimilation significantly increased from 0.11 mg seed−1 day−1 at 18.4°C to 0.64 mg seed−1 day−1 at 33.2°C (Figure 4A). Plant N uptake was not significantly modified by the small range of increasing temperature from 21.8 to 26.8°C in Exp. 2, while it increased linearly with increasing temperature from 18.4 to 33.2°C in Exp. 1 (Figure 4A).
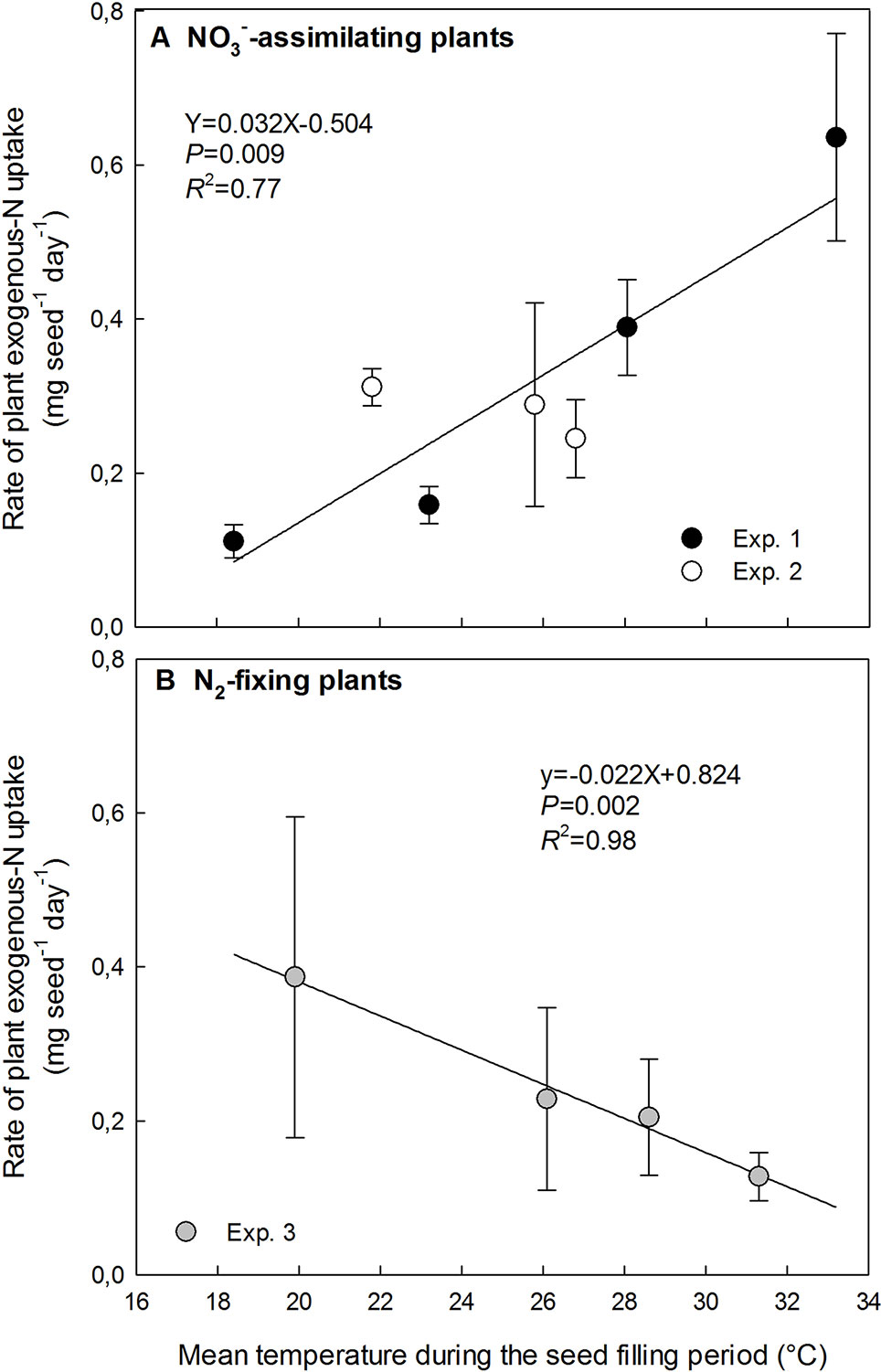
Figure 4 Opposite responses to temperature increase of exogenous-N uptake rate in plants during the seed-filling period for NO3−-assimilating plants (A) and N2-fixing plants (B). The vertical bars represent SE. The data were fitted with a linear regression.
Conversely, for N2-fixing plants in Exp. 3 the temperature increase significantly decreased the rate of N uptake in plants following a linear relationship from 0.39 mg seed−1 day−1 at 19.9°C to 0.13 mg seed−1 day−1 at 31.3°C (Figure 4B).
Effects of High Temperatures on the Variation of the N Amount Within the Different Vegetative Organs During the Seed-Filling Period of NO3−-Assimilating and N2-Fixing Plants
A net export of N represents a decrease in the N amount of a vegetative organ during the temperature treatment application through the seed-filling period, while a net import represents an increase in the N amount (Figure 5).
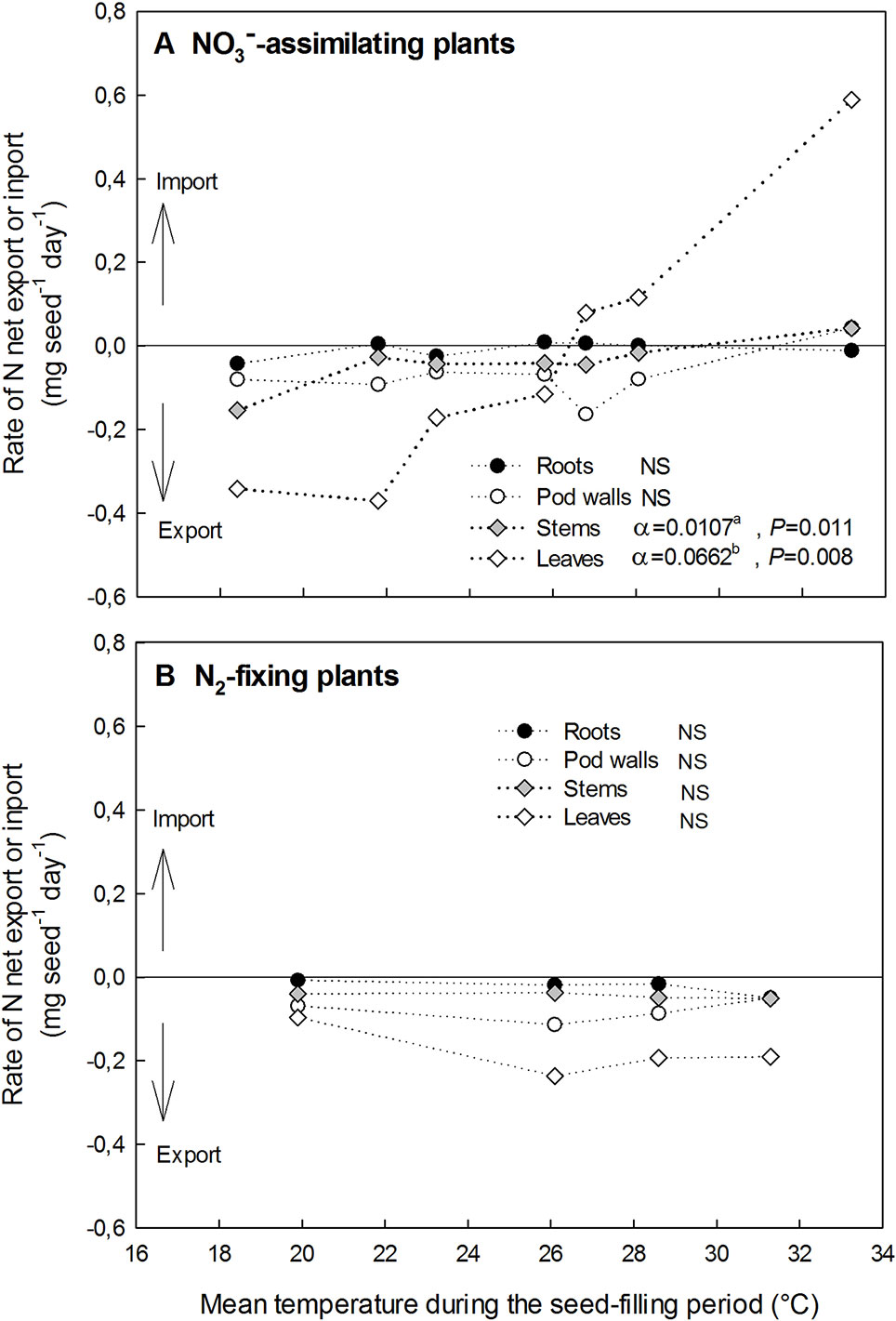
Figure 5 Impacts of the temperature increase on the rate of net N export or import in vegetative organs (roots, pod walls, stems, and leaves) during the seed-filling period for NO3−-assimilating plants (A) and N2-fixing plants (B). α represents the linear regressions correlation coefficient of the net N export (or import) in each vegetative organ v. temperature. NS, not significantly different from 0 (P < 0.05). Values with different letters are statistically different at P = 0.05.
Considering NO3−-assimilating plants (Exp. 1 and 2), the effect of temperature on rates of the N amount variation during the seed-filling period was significant only in leaves and to a lesser extent in stems (Figure 5A). In the leaves, N fluxes switched from N export to N import approximately above 26.3°C (Figure 5A). At the lowest temperature (18.4°C) leaves and stems respectively exported 0.34 and 0.15 mg seed−1 day−1, while at the highest temperature (33.2°C) leaves and stems respectively imported 0.59 and 0.04 mg seed−1 day−1 (Figure 5A). Thus, the rate of the N amount variation during the seed-filling period in leaves was by far the most responsive to temperature among vegetative organs in NO3−-assimilating plants (Figure 5A).
Considering N2-fixing plants (Exp. 3), all vegetative organs presented a net export of N whatever the temperature (Figure 5B). The temperature increase (from 19.9 to 31.3 °C) had no significant effect on the rate of the N export whatever the vegetative organ of N2-fixing plants (Figure 5B).
At maturity, N concentrations of vegetative organs (roots, pod walls, stems, and leaves) were above 16 mg g−1, for both NO3−-assimilating and N2-fixing plants and whatever the temperature treatment (Table 1).
Discussion
The present study quantifies and explains, for the first time, the effects of high temperatures on N partitioning to filling seeds in pea, an annual legume crop. Plants differing in seed number between experiments allow us to assess trends representative of various field conditions. The wide range of mean air temperature explored (from 18.4 to 33.2°C) is representative of the present and future climatic conditions expected in field during the seed-filling period of most annual crops in Western Europe (June-July): mean monthly temperatures above 18°C and an increase in the frequency, intensity, and duration of heat waves (Christensen et al., 2007; Vliet et al., 2012; Xu et al., 2012). This temperature range was similar for the two plant N nutrition pathways tested: 19.9 to 31.3°C for NO3−-assimilating plants allowing to measure endogenous fluxes and 18.4 to 33.2°C for N2-fixing plants, more representative of field conditions. Temperature treatments started when all seeds had begun to fill. At this stage, pea plants had no longer the possibility to adjust the number of seed sinks to assimilate availability as earlier in their development (Ney et al., 1993). Indeed, seed number per plant at maturity was equal for all temperature treatments within an experiment.
Decrease in Seed Dry Matter and N Accumulation With Increasing High Temperature, Resulting Effects on Seed N Concentration and N Yield
The rate of individual seed dry matter accumulation and seed-filling duration in pea were reduced by 0.8 mg seed−1 day−1 and 0.8 days, respectively, for each additional °C of mean temperature from 18.4 to 33.2°C. Therefore, individual seed weight decreased with increasing temperature. These results are consistent with previous reports of a reduction in seed weight at high temperatures due to a decrease in the rate of seed fill and an abbreviated seed-filling duration (Singletary et al., 1994; Kim et al., 2011; Bueckert et al., 2015).
Our study demonstrates that seed N accumulation was also reduced by 0.76 mg seed−1 day−1 for each additional °C of mean temperature from 18.4 to 33.2°C, for both NO3−-assimilating and N2-fixing plants. Results showed that, whatever the plant N nutrition pathway, the decrease of seed N accumulation with increasing temperature was due to the reduction of both the rate of individual seed N accumulation and the seed-filling duration. The rate of individual seed N accumulation progressively decreased by 0.032 mg seed−1 day−1 for each additional °C temperature from 18.4 to 33.2°C. Therefore the amount of N accumulated in seeds significantly decreased with increasing temperatures.
Seed N concentration at maturity is the ratio of seed N and seed dry matter accumulation rates during the seed-filling period. Our results demonstrate that the decrease of the individual seed N rate with increasing high temperatures was lower than that of the individual seed dry matter rate (0.032 and 0.8 mg seed−1, respectively). Thus seed N concentration increased with increasing high temperatures. This result is consistent with previous reports of higher seed N concentration when temperatures rise during the seed-filling period (Karjalainen and Kortet, 1987; Tashiro and Wardlaw, 1991; Wardlaw and Wrigley, 1994; Larmure et al., 2005; Farooq et al., 2018).
In Europe, the current and projected warming rate in summer (June to August) is between 4.5 and 6.8°C/century, higher than for other seasons (Rowell, 2005; Xu et al., 2012; Terray and Boe, 2013). Consequently, the on-going climate warming has caused and will continue to cause severe seed N yield losses in pea without adaptation strategies. From our study, it can be expected that at the field scale, seed N yield in pea could decrease by 1.8 gN m−2 for each additional °C of mean temperature during the seed-filling period, considering 2,400 seed m−2. From the perspective of French pea production, it represents more than 13 % loss of recent seed N yield (~13.8 gN m−2 calculated with the mean yield and seed N concentration from 2013 to 2017: respectively 3.83 t·m−2 and 36.2 mgN·g−1 ; UNIP and ARVALIS, 2013, 2014; Terres Inovia and Terres Univia, 2015, 2016, 2017). Our study enables the identification of plant mechanisms involved in these seed N yield losses in order to provide levers for improving varieties tolerating heat stress.
Nitrogen Sources Availability Does Not Explain the Decrease in Seed N Amount With Increasing High Temperature
Nitrogen for pea seeds comes from two sources: current plant N uptake and N remobilization from vegetative organs (Lhuillier-Soundélé et al., 1999; Schiltz et al., 2005). Nitrogen availability from plant sources is known to determine seed N accumulation (Lhuillier-Soundélé et al., 1999; Martre et al., 2003; Larmure and Munier-Jolain, 2004; Kinugasa et al., 2012) . However, our results contradict the possibility of a decrease in seed N accumulation at high temperatures resulting of a limitation in N supply.
Indeed, plant NO3− assimilation provides higher N availability under high temperatures (with non-limiting water availability) as plant N uptake of NO3−-assimilating plants significantly increased with increasing temperature by 0.032 mg seed−1 day−1 for each additional °C temperature. NO3− assimilation may have been enhanced by the increase in plant transpiration with increasing temperature under our no-limiting water conditions, because the transport of water and N solutes from roots to shoots is driven by the evaporative loss of water (Salon et al., 2011). Indeed, the transpiration of well-watered plants is expected to increase by 1–5% for each additional °C temperature between 5 and 35°C (Kirschbaum, 2004). Contrary to NO3− assimilation, plant N2 fixation was reduced under high temperatures: plant N uptake of N2-fixing plants decreased with increasing temperature by 0.022 mg seed−1 day−1 for each additional °C temperature. High temperatures may decrease N2-fixation efficiency by affecting nitrogenase activity and/or nodule longevity (Bordeleau and Prevost, 1994; Hungria and Vargas, 2000), as no nodule production occurs during the seed-filling period of N2-fixing plants (Voisin et al., 2003; Bourion et al., 2007).
Despite the opposite effect of increasing temperature on plant N uptake acquired via N2 fixation or NO3− assimilation, a lot of N was still available at maturity in vegetative organs (leaves, stems, pod walls, and roots), whatever the plant N nutrition pathway and the temperature treatment. Concentrations of vegetative organs at maturity were all above 16 mg g−1, much higher than the threshold of non-remobilizable N concentration (Larmure and Munier-Jolain, 2004). This result suggests that the shorter duration of seed-filling at high temperature was not due to a reduction of photosynthetic activity caused by N remobilization from vegetative organs to seeds. Indeed, the present study using 15NO3−-labeled N source clearly demonstrates a gradual limitation of the rate of endogenous-N remobilization from vegetative organs to filling seeds above 18.4°C. N remobilization was nevertheless the major contributor to the N filling of pea seeds whatever the temperature, consistently with the previous observations at non-stressing temperatures in oilseed rape (Brassica napus) and in pea (Malagoli et al., 2005; Schiltz et al., 2005).
Sink Strength Determines Plant N Fluxes to Filling Seeds Under Heat Stress Conditions
Our results demonstrate a sink limitation of seed N accumulation by high temperatures (from 18.4 to 33.2°C). Actually, additional plant N uptake in NO3−-assimilating plants at high temperature provided by the xylem was never allocated to seeds but stored in leaves and to a lesser extent in stems. This findings are in line with the observation that the majority of seeds N intake is attributable to phloem (Pate and Hocking, 1978). This hypothesis of sink limitation at high temperature is consistent with (1) the shorter duration of seed-filling with increasing temperature observed in our study, that leads to a progressive premature reduction of seed sink; (2) the decrease of the individual seed dry matter accumulation rate with increasing temperature that reduces seed sink; and (3) previous studies reporting a decrease in photoassimilates translocation to filling seeds at high temperatures due to reduced sink activity rather than source activity (Ito et al., 2009; Suwa et al., 2010; Kim et al., 2011). Early loss of individual seed sink activity at high temperature may result from a reduction of the activity of starch synthesis-related enzymes in the seed (Ito et al., 2009; Suwa et al., 2010; Yamakawa and Hakata, 2010; Kim et al., 2011). At high temperature, synthesis of hemicelluloses, cellulose, and starch in grain declines while sucrose accumulates (Ito et al., 2009; Suwa et al., 2010; Yamakawa and Hakata, 2010). While increasing temperatures might impede phloem transport, they also might hasten the preferential unloading of carbon (C) along the stem to meet local increasing respiratory demand (Atkin and Tjoelker, 2003; Sevanto, 2014). The resulting enrichment in N relative to C in the phloem sap reaching the seeds would explain its higher N concentration (Layzell and Larue, 1982).
Definition of Plant Senescence Under Heat Stress and Strategies to Develop Cultivars Adapted to Higher Temperatures Due to Climate Change
The original results of our study throw a new light on the regulation of N remobilization and definition of senescence in plants submitted to abiotic stress, such as heat-stress. At moderate temperatures senescence is linked to N remobilization to filling seeds, a mechanism to compensate the limitation of N uptake by roots (Hebbar et al., 2014). On the other hand, this research established that the heat-induced senescence (noticeable through the reduction of seed-filling duration) is surprisingly not associated with an acceleration of N nutrient remobilization to filling seeds. Under high temperature, shorter duration of seed-filling with increasing temperature may more likely result from alterations in various photosynthetic attributes and carbon budget than from plant N resources remobilization to cope with the heat stress (Wahid et al., 2007; Mathur et al., 2014).
Our results demonstrate that seed N yield processes are and will continue to be very frequently sink-limited by high temperatures during the seed-filling period in the warming climate context. It is worth noting that under the current and future climate change context, the increased frequency of early heat waves are and will be often associated to water deficit in field, resulting from either decreased precipitation and/or increased evaporation (Dai, 2013; Sehgal et al., 2018). The combined effects of water deficit and heat-stress on crops are more severe (Sehgal et al., 2018). Both abiotic constraints were previously reported to enhance assimilate remobilization from source to sink (Pic et al., 2002; Sehgal et al., 2018). On the contrary, our study using labeled nitrate demonstrates that N assimilate remobilization was reduced and most likely sink-limited under heat stress. Consequently, sustaining seed sink demand and preserving photosynthetic attributes of stressed plants during the seed-filling period should be promising strategies to maintain crop N production under exacerbated combined heat and water-deficit stresses in field due to the on-going climate change. Such improvements may especially require further investigations in order to elucidate how sink activity could be modulated at high temperature and water deficit. While water deficit can be mitigated by irrigation (Bueckert et al., 2015), few cultural practices are available to leverage high temperatures stress. A better understanding of mechanisms controlling C and N allocation to sinks, are required to build robust sustainable practices.
Data Availability Statement
The datasets generated for this study are available on request to the corresponding author.
Author Contributions
AL and NM-J designed the study. AL collected and analyzed the data. AL and NM-J interpreted the results. Both authors contributed to manuscript writing.
Funding
This research was supported by INRA, AgroSupDijon and a grant of UNIP (Union Nationale Interprofessionnelle des Plantes Riches en Protéines).
Conflict of Interest
The authors declare that the research was conducted in the absence of any commercial or financial relationships that could be construed as a potential conflict of interest.
Acknowledgments
The authors are grateful to Vincent Durey, Christian Jeudy, Patrick Mathey, Carole Reibel, Valentine Pelissier, Eric Pimet and Anne-Lise Santoni for their excellent technical assistance, and the greenhouse staff at INRA Dijon for managing the experiments. This research was supported by INRA, AgroSupDijon and a grant of UNIP (Union Nationale Interprofessionnelle des Plantes Riches en Protéines). The experimental protocol and the manuscript were greatly improved thanks to the valuable comments of colleagues from the UMR 1347 Agroécologie: Nathalie Colbach, Christian Jeudy, Delphine Moreau, Marion Prudent, Christophe Salon, Aude Tixier and Anne-Sophie Voisin.
Supplementary Material
The Supplementary Material for this article can be found online at: https://www.frontiersin.org/articles/10.3389/fpls.2019.01608/full#supplementary-material
References
Araujo, A. P., Del Pin, B., Teixeira, M. G. (2012). Nitrogen and phosphorus in senescent leaves of field-grown common bean cultivars and their contribution to crop nutrient budget. Field Crops Res. 127, 35–43. doi: 10.1016/j.fcr.2011.11.009
Asseng, S., Foster, I., Turner, N. C. (2011). The impact of temperature variability on wheat yields. Global Change Biol. 17, 997–1012. doi: 10.1111/j.1365-2486.2010.02262.x
Atkin, O. K., Tjoelker, M. G. (2003). Thermal acclimation and the dynamic response of plant respiration to temperature. Trends Plant Sci. 8, 343–351. doi: 10.1016/S1360-1385(03)00136-5
Badeck, F. W., Bondeau, A., Bottcher, K., Doktor, D., Lucht, W., Schaber, J., et al. (2004). Responses of spring phenology to climate change. New Phytol. 162, 295–309. doi: 10.1111/j.1469-8137.2004.01059.x
Barnabas, B., Jager, K., Feher, A. (2008). The effect of drought and heat stress on reproductive processes in cereals. Plant Cell Environ. 31, 11–38. doi: 10.1111/j.1365-3040.2007.01727.x
Barraclough, P. B., Lopez-Bellido, R., Hawkesford, M. J. (2014). Genotypic variation in the uptake, partitioning and remobilisation of nitrogen during grain-filling in wheat. Field Crops Res. 156, 242–248. doi: 10.1016/j.fcr.2013.10.004
Bordeleau, L. M., Prevost, D. (1994). Nodulation and nitrogen fixation in extreme environments. Plant Soil 161, 115–125. doi: 10.1007/BF02183092
Bourion, V., Fouilloux, G., Le Signor, C., Lejeune-Henaut, I. (2002a). Genetic studies of selection criteria for productive and stable peas. Euphytica 127, 261–273. doi: 10.1023/A:1019995822353
Bourion, V., Duparque, M., Lejeune-Henaut, I., Munier-Jolain, N. G. (2002b). Criteria for selecting productive and stable pea cultivars. Euphytica 126, 391–399. doi: 10.1093/aob/mcm147
Bourion, V., Laguerre, G., Depret, G., Voisin, A. S., Salon, C., Duc, G. (2007). Genetic variability in nodulation and root growth affects nitrogen fixation and accumulation in pea. Ann. Bot. 100, 589–598. doi: 10.1016/j.fcr.2010.07.012
Brisson, N., Gate, P., Gouache, D., Charmet, G., Oury, F. X., Huard, F. (2010). Why are wheat yields stagnating in Europe? A comprehensive data analysis for France France Field Crops Res. 119, 201–212. doi: 10.4141/cjps-2014-342
Bueckert, R. A., Wagenhoffer, S., Hnatowich, G., Warkentin, T. D. (2015). Effect of heat and precipitation on pea yield and reproductive performance in the field. Can. J. Plant Sci. 95, 629–639. doi: 10.4141/cjps-2014-342
Christensen, J. H., Hewitson, B., Busuioc, A., Chen, A., Gao, X., Held, I., et al. (2007). “Regional Climate Projections,” in Climate Change 2007: The Physical Science Basis. Contribution of Working Group I to the Fourth Assessment Report of the Intergovernmental Panel on Climate Change. Eds. Solomon, S., Qin, D., Manning, M., Chen, Z., Marquis, M., Averyt, K. B., Tignor, M., Miller, H. L. (Cambridge, United Kingdom and New York, NY, USA: Cambridge University Press). doi: 10.1111/jac.12005
Dai, A. G. (2013). Increasing drought under global warming in observations and models. Nat. Clim. Change 3, 52–58. doi: 10.1016/j.fcr.2012.11.009
Djanaguiraman, M., Prasad, P. V. V., Boyle, D. L., Schapaugh, W. T. (2013). Soybean pollen anatomy, viability and pod set under high temperature stress. J. Agron. Crop Sci. 199, 171–177. doi: 10.1021/acs.jafc.8b02924
Edreira, J. I. R., Otegui, M. E. (2013). Heat stress in temperate and tropical maize hybrids: a novel approach for assessing sources of kernel loss in field conditions. Field Crops Res. 142, 58–67. doi: 10.1111/j.1438-8677.2008.00114.x
Farooq, M., Hussain, M., Usman, M., Farooq, S., Alghamdi, S. S., Siddique, K. H. M. (2018). Impact of abiotic stresses on grain composition and quality in food legumes. J. Agric. Food Chem. 66, 8887–8897. doi: 10.1071/FP03105
Gregersen, P. L., Holm, P. B., Krupinska, K. (2008). Leaf senescence and nutrient remobilisation in barley and wheat. Plant Biol. 10, 37–49. doi: 10.1007/s11104-013-2012-6
Guilioni, L., Wery, J., Lecoeur, J. (2003). High temperature and water deficit may reduce seed number in field pea purely by decreasing plant growth rate. Funct. Plant Biol. 30, 1151–1164. doi: 10.1016/S0378-4290(99)00084-2
Hebbar, K. B., Rane, J., Ramana, S., Panwar, N. R., Ajay, S., Rao, A. S., et al. (2014). Natural variation in the regulation of leaf senescence and relation to N and root traits in wheat. Plant Soil 378, 99–112. doi: 10.1007/s11104-013-2012-6
Hungria, M., Vargas, M. A. T. (2000). Environmental factors affecting N 2 fixation in grain legumes in the tropics, with an emphasis on Brazil. Field Crops Res. 65, 151–164. doi: 10.1111/j.1439-037X.2009.00376.x
IPCC. (2018). Summary for Policymakers. In: Global Warming of 1.5°C. An IPCC Special Report on the impacts of global warming of 1.5°C above pre-industrial levels and related global greenhouse gas emission pathways, in the context of strengthening the global response to the threat of climate change, sustainable development, and efforts to eradicate poverty. Eds. Masson-Delmotte, V., Zhai, P., Pörtner, H.-O., Roberts, D., Skea, J., Shukla, P. R., Pirani, A., Moufouma-Okia, W., Péan, C., Pidcock, R., Connors, S., Matthews, J. B. R., Chen, Y., Zhou, X., Gomis, M. I., Lonnoy, E., Maycock, T., Tignor, M., Waterfield, T. (Geneva, Switzerland: World Meteorological Organization), 32 pp. doi: 10.1023/A:1024189029226. Available at: www.ipcc.ch
Ito, S., Hara, T., Kawanami, Y., Watanabe, T., Thiraporn, K., Ohtake, N., et al. (2009). Carbon and nitrogen transport during grain filling in rice under high-temperature conditions. J. Agron. Crop Sci. 195, 368–376. doi: 10.23986/afsci.72238
Jensen, E. S., Hauggaard-Nielsen, H. (2003). How can increased use of biological N2 fixation in agriculture benefit the environment? Plant Soil 252, 177–186. doi: 10.1016/j.fcr.2007.01.002
Karjalainen, R., Kortet, S. (1987). Environmental and genetic variation in protein content of peas under northern growing conditions and breeding implications. J. Agric. Sci. In Finland 59, 1–9. doi: 10.1016/j.fcr.2011.03.014
Kichey, T., Hirel, B., Heumez, E., Dubois, F., Gouis, J. L. (2007). In winter wheat (Triticum aestivum L.), post-anthesis nitrogen uptake and remobilisation to the grain correlates with agronomic traits and nitrogen physiological markers. Field Crops Res. 102, 22–32. doi: 10.1007/s10265-011-0439-5
Kim, J., Shon, J., Lee, C., Yang, W., Yoon, Y., Yang, W., et al. (2011). Relationship between grain filling duration and leaf senescence of temperate rice under high temperature. Field Crops Res. 122, 207–213. doi: 10.1055/s-2004-820883
Kinugasa, T., Sato, T., Oikawa, S., Hirose, T. (2012). Demand and supply of N in seed production of soybean (Glycine max) at different N fertilization levels after flowering. J. Plant Res. 125, 275–281. doi: 10.1016/S0378-4290(03)00158-8
Kirschbaum, M. U. F. (2004). Direct and indirect climate change effects on photosynthesis and transpiration. Plant Biol. 6, 242–253. doi: 10.1071/FP05154
Larmure, A., Munier-Jolain, N. G. (2004). A crop model component simulating N partitioning during seed filling in pea. Field Crops Res. 85, 135–148. doi: 10.1104/pp.70.51290
Larmure, A., Salon, C., Munier-Jolain, N. G. (2005). How does temperature affect C and N allocation to the seeds during the seed-filling period in pea? Effect Seed Nitrogen Concentration Funct. Plant Biol. 32, 1009–1017. doi: 10.2135/cropsci1999.3961741x
Layzell, D. B., Larue, T. A. (1982). Modeling c and n transport to developing soybean fruits. Plant Physiol. 70, 1290–1298. doi: 10.1093/aob/mci091
Lhuillier-Soundélé, A., Munier-Jolain, N. G., Ney, B. (1999). Influence of nitrogen availability on seed nitrogen accumulation in pea. Crop Sci. 39, 1741–1748. doi: 10.1104/pp.103.030585
Malagoli, P., Laine, P., Rossato, L., Ourry, A. (2005). Dynamics of nitrogen uptake and mobilization in field-grown winter oilseed rape (Brassica napus) from stem extension to harvest: I. Global N flows between vegetative and reproductive tissues in relation to leaf fall and their residual N. Ann. Bot. 95, 853–861. doi: 10.1111/j.1438-8677.2008.00097.x
Martre, P., Porter, J. R., Jamieson, P. D., Triboi, E. (2003). Modeling grain nitrogen accumulation and protein composition to understand the sink/source regulations of nitrogen remobilization for wheat. Plant Physiol. 133, 1959–1967. doi: 10.1016/j.jphotobiol.2014.01.010
Masclaux-Daubresse, C., Reisdorf-Cren, M., Orsel, M. (2008). Leaf nitrogen remobilisation for plant development and grain filling. Plant Biol. 10, 23–36. doi: 10.1046/j.1365-3040.1999.00443.x
Mathur, S., Agrawal, D., Jajoo, A. (2014). Photosynthesis: response to high temperature stress. J. Photochem. Photobiol. B-Biol. 137, 116–126. doi: 10.1016/j.crvi.2008.07.020
Morison, J. I. L., Lawlor, D. W. (1999). Interactions between increasing CO 2 concentration and temperature on plant growth. Plant Cell Environ. 22, 659–682. doi: 10.1016/j.eja.2007.11.004
Munier-Jolain, N., Larmure, A., Salon, C. (2008). Determinism of carbon and nitrogen reserve accumulation in legume seeds. Comptes Rendus Biol. 331, 780–787. doi: 10.2135/cropsci1993.0011183X003300020010x
Nemecek, T., Dubois, G., Casta, P., Charles, R., Pahl, H. (2008). Environmental impacts of introducing grain legumes into European crop rotations. Eur. J. Agron. 28, 380–393. doi: 10.1093/oxfordjournals.aob.a085530
Ney, B., Duthion, C., Fontaine, E. (1993). Timing of reproductive abortions in relation to cell division, water content, and growth of pea seeds. Crop Sci. 33, 267–270. doi: 10.1073/pnas.0403720101
Pate, J. S., Hocking, P. J. (1978). Phloem and xylem transport in the supply of minerals to a developing legume (Lupinus albus L.) fruit. Ann. Bot. 42, 911–921. doi: 10.1104/pp.128.1.236
Peng, S., Huang, J., Sheehy, J. E., Laza, R. C., Visperas, R. M., Zhong, X. H., et al. (2004). Rice yields decline with higher night temperature from global warming, in: Proceedings of the National Academy of Sciences of the United States of America, Vol. 101. pp. 9971–9975. doi: 10.1016/j.envexpbot.2013.03.005
Pic, E., de la Serve, B. T., Tardieu, F., Turc, O. (2002). Leaf senescence induced by mild water deficit follows the same sequence of macroscopic, biochemical, and molecular events as monocarpic senescence in pea. Plant Physiol. 128, 236–246. doi: 10.1007/s00382-005-0068-6
Pimentel, C., Ribeiro, R. V., Machado, E. C., dos Santos, M. G., de Oliveira, R. F. (2013). In vivo temperature limitations of photosynthesis in Phaseolus vulgaris L. Environ. Exp. Bot. 91, 84–89. doi: 10.1111/j.1365-3040.2007.01682.x
Rowell, D. P. (2005). A scenario of European climate change for the late twenty-first century: seasonal means and interannual variability. Climate Dyn. 25, 837–849. doi: 10.1007/s00382-005-0068-6
Sage, R. F., Kubien, D. S. (2007). The temperature response of C 3 and C 4 photosynthesis. Plant Cell Environ. 30, 1086–1106. doi: 10.1051/agro:2001143
Salon, C., Munier-Jolain, N. G., Duc, G., Voisin, A. S., Grandgirard, D., Larmure, A., et al. (2001). Grain legume seed filling in relation to nitrogen acquisition: a review and prospects with particular reference to pea. Agronomie 21, 539–552. doi: 10.1073/pnas.0906865106
Salon, C., Avice, J. C., Larmure, A., Ourry, A., Prudent, P., Voisin, A. S. (2011). “Plant N fluxes and modulation by nitrogen, heat and water stresses: a review based on comparison of legumes and non legume plants,” in Abiotic Stress in Plants - Mechanisms and Adaptations. Ed. Venkateswarlu, A.S.A.B., 79–118. http://www.intechweb.org/,. doi: 10.1104/pp.104.056713
Schiltz, S., Munier-Jolain, N., Jeudy, C., Burstin, J., Salon, C. (2005). Dynamics of exogenous nitrogen partitioning and nitrogen remobilization from vegetative organs in pea revealed by 15N in vivo labeling throughout seed filling. Plant Physiol. 137, 1463–1473. doi: 10.3389/fpls.2018.01705
Schlenker, W., Roberts, M. J. (2009). Nonlinear temperature effects indicate severe damages to U.S. crop yields under climate change, in: Proceedings of the National Academy of Sciences of the United States of America, Vol. 106. pp. 15594–15598. doi: 10.1007/s13593-011-0021-5
Sehgal, A., Sita, K., Siddique, K. H. M., Kumar, R., Bhogireddy, S., Varshney, R. K., et al. (2018). Drought or/and heat-stress effects on seed filling in food crops: impacts on functional biochemistry, seed yields, and nutritional quality. Front. Plant Sci. 9, 1705. doi: 10.2135/cropsci1989.0011183X002900010023x
Sevanto, S. (2014). Phloem transport and drought. J. Exp. Bot. 65, 1751–1759. doi: 10.2134/agronj1976.00021962006800020021x
Siddique, K. H. M., Johansen, C., Turner, N. C., Jeuffroy, M. H., Hashem, A., Sakar, D., et al. (2012). Innovations in agronomy for food legumes. A review. Agron. Sustain. Dev. 32, 45–64. doi: 10.1071/PP9940829
Sinclair, T. R., Horie, T. (1989). Leaf nitrogen, photosynthesis, and crop radiation use efficiency: a review. Crop Sci. 29, 90–98. doi: 10.3389/fpls.2017.01658
Sinclair, T. R., DeWitt, C. T. (1976). Analysis of carbon and nitrogen limitations to soybean yield. Agron. J. 68, 319–324. doi: 10.2134/agronj1976.00021962006800020021x
Singletary, G. W., Banisadr, R., Keeling, P. L. (1994). Heat stress during grain filling in maize: effects on carbohydrate storage and metabolism. Aust. J. Plant Physiol. 21, 829–841. doi: 10.1016/j.agrformet.2012.05.005
Sita, K., Sehgal, A., HanumanthaRao, B., Nair, R. M., Prasad, P. V. V., Kumar, S., et al. (2017). Food legumes and rising temperatures: effects, adaptive functional mechanisms specific to reproductive growth stage and strategies to improve heat tolerance. Front. Plant Sci. 8, 1658. doi: 10.1016/j.plaphy.2009.12.010
Spiertz, J. H. J. (1977). The influence of temperature and light intensity on grain growth in relation to the carbohydrate and nitrogen economy of the wheat plant. Netherlands J. Agric. Sci. 25, 182–197. doi: 10.1016/j.fcr.2013.07.021
Supit, I., van Diepen, C. A., de Wit, A. J. W., Wolf, J., Kabat, P., Baruth, B., et al. (2012). Assessing climate change effects on European crop yields using the crop growth monitoring system and a weather generator. Agric. For. Meteorol. 164, 96–111. doi: 10.1111/j.1439-037X.2004.00127.x
Suwa, R., Hakata, H., Hara, H., El-Shemy, H. A., Adu-Gyamfi, J. J., Nguyen Tran, N., et al. (2010). High temperature effects on photosynthate partitioning and sugar metabolism during ear expansion in maize (Zea mays L.) genotypes. Plant Physiol. Biochem. 48, 124–130. doi: 10.1071/PP9910259
Tacarindua, C. R. P., Shiraiwa, T., Homma, K., Kumagai, E., Sameshima, R. (2013). The effects of increased temperature on crop growth and yield of soybean grown in a temperature gradient chamber. Field Crops Res. 154, 74–81. doi: 10.1016/j.crte.2013.02.003
Tahir, I. S. A., Nakata, N. (2005). Remobilization of nitrogen and carbohydrate from stems of bread wheat in response to heat stress during grain filling. J. Agron. Crop Sci. 191, 106–115. doi: 10.1111/j.1439-037X.2004.00127.x
Tashiro, T., Wardlaw, I. F. (1991). The effect of high temperature on the accumulation of dry matter, carbon and nitrogen in the kernel of rice. Aust. J. Plant Physiol. 18, 259–265. doi: 10.1071/PP9910259
Terray, L., Boe, J. (2013). Quantifying 21st-century France climate change and related uncertainties. Comptes Rendus Geosci. 345, 136–149. doi: 10.1016/j.crte.2013.02.003
Terres Inovia, T. U. (2015). Qualité des graines : Pois récolte 2015. www.terresinovia.fr. doi: 10.1016/j.agrformet.2012.05.020
Terres Inovia, T. U. (2016). Qualité des graines : Pois récolte 2015. www.terresinovia.fr.
Terres Inovia, T. U. (2017). Qualité des graines : Pois récolte 2017. www.terresinovia.fr.
Trnka, M., Brazdil, R., Olesen, J. E., Eitzinger, J., Zahradnicek, P., Kocmankova, E., et al. (2012). Could the changes in regional crop yields be a pointer of climatic change? Agric. For. Meteorol. 166, 62–71. doi: 10.1007/s13593-011-0020-6
UNIP, A. (2013). Récolte 2013 : Qualité des pois. www.unip.fr www.arvalisinstitutduvegetal.fr. doi: 10.1007/s10584-011-0131-8
UNIP, A. (2014). Récolte 2014 : Qualité des pois. www.unip.fr www.arvalisinstitutduvegetal.fr. doi: 10.1007/s13593-013-0146-9
Vadez, V., Berger, J. D., Warkentin, T., Asseng, S., Pasala, R., Rao, K. P. C., et al. (2012). Adaptation of grain legumes to climate change: a review. Agron. Sustain. Dev. 32, 31–44. doi: 10.1093/jxb/erg290
Vliet, M.T.H.V., Blenkinsop, S., Burton, A., Harpham, C., Broers, H. P., Fowler, H. J. (2012). A multi-model ensemble of downscaled spatial climate change scenarios for the Dommel catchment, Western Europe. Clim. Change 111, 249–277. doi: 10.1016/j.envexpbot.2007.05.011
Voisin, A. S., Salon, C., Jeudy, C., Warembourg, F. R. (2003). Symbiotic N 2 fixation activity in relation to C economy of Pisum sativum L. as a function of plant phenology. J. Exp. Bot. 54, 2733–2744. doi: 10.1016/S0167-8809(00)00224-3
Voisin, A. S., Cazenave, A. B., Duc, G., Salon, C. (2013). Pea nodule gradients explain C nutrition and depressed growth phenotype of hypernodulating mutants. Agron. Sustain. Dev. 33, 829–838. doi: 10.1071/PP9940695
Wahid, A., Gelani, S., Ashraf, M., Foolad, M. R. (2007). Heat tolerance in plants: an overview. Environ. Exp. Bot. 61, 199–223. doi: 10.1111/jipb.12005
Wardlaw, I. F., Wrigley, C. W. (1994). Heat tolerance in temperate cereals - An overview. Aust. J. Plant Physiol. 21, 695–703. doi: 10.1071/pp9940695
Wheeler, T. R., Craufurd, P. Q., Ellis, R. H., Porter, J. R., Prasad, P. V. V. (2000), 159–167. Temperature variability and the yield of annual crops. Special issue: Food and forestry: global change and global challenges. Selected papers from the GCTE Focus 3 Conference, Reading, UK, September 1999., pp. doi: 10.1093/pcp/pcq034
Wu, X. Y., Kuai, B. K., Jia, J. Z., Jing, H. C. (2012). Regulation of Leaf Senescence and Crop Genetic Improvement. J. Integr. Plant Biol. 54, 936–952. doi: 10.1016/j.envexpbot.2010.09.005
Xu, Y., Castel, T., Richard, Y., Cuccia, C., Bois, B. (2012). Burgundy regional climate change and its potential impact on grapevines. Climate Dyn. 39, 1–14. doi: 10.1007/s00382-011-1284-x
Yamakawa, H., Hakata, M. (2010). Atlas of rice grain filling-related metabolism under high temperature: joint analysis of metabolome and transcriptome demonstrated inhibition of starch accumulation and induction of amino acid accumulation. Plant Cell Physiol. 51, 795–809. doi: 10.1093/pcp/pcq034
Keywords: high temperatures, Pisum sativum L, Seed N amount, N partitioning, 15N labeling, seed-filling, plant N uptake
Citation: Larmure A and Munier-Jolain NG (2019) High Temperatures During the Seed-Filling Period Decrease Seed Nitrogen Amount in Pea (Pisum sativum L.): Evidence for a Sink Limitation. Front. Plant Sci. 10:1608. doi: 10.3389/fpls.2019.01608
Received: 29 March 2019; Accepted: 15 November 2019;
Published: 20 December 2019.
Edited by:
Penelope Mary Smith, La Trobe University, AustraliaReviewed by:
Eva Stoger, University of Natural Resources and Life Sciences Vienna, AustriaPetr Smýkal, Palacký University Olomouc, Czechia
Copyright © 2019 Larmure and Munier-Jolain. This is an open-access article distributed under the terms of the Creative Commons Attribution License (CC BY). The use, distribution or reproduction in other forums is permitted, provided the original author(s) and the copyright owner(s) are credited and that the original publication in this journal is cited, in accordance with accepted academic practice. No use, distribution or reproduction is permitted which does not comply with these terms.
*Correspondence: Annabelle Larmure, annabelle.larmure@agrosupdijon.fr