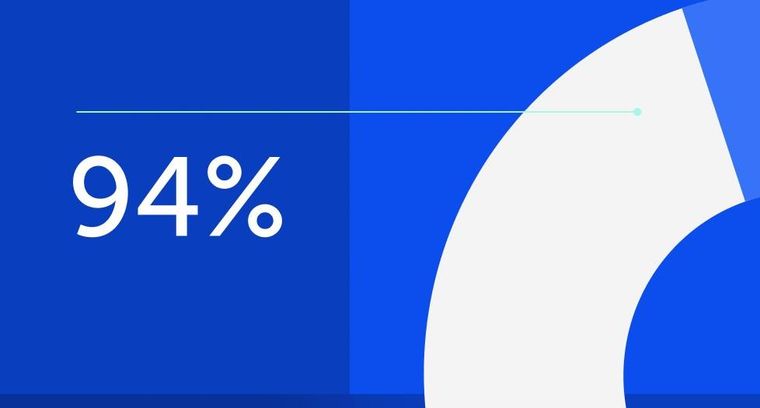
94% of researchers rate our articles as excellent or good
Learn more about the work of our research integrity team to safeguard the quality of each article we publish.
Find out more
ORIGINAL RESEARCH article
Front. Plant Sci., 26 November 2019
Sec. Plant Systematics and Evolution
Volume 10 - 2019 | https://doi.org/10.3389/fpls.2019.01506
This article is part of the Research TopicOrigins and Domestication of the GrapeView all 8 articles
Grapevine (Vitis vinifera ssp. sativa) is a perennial crop especially important for wine and fruit production. The species is highly polymorphic with thousands of different varieties selected by farmers and clonally propagated. However, it is still debated whether grapevine domestication from its wild ancestor (V. vinifera ssp. sylvestris) has been a single event or rather it occurred on multiple occasions during the diffusion of its cultivation across the Mediterranean. Located in the center of the Basin, Sicily is its largest island and has served as a hotspot for all civilizations that have crossed the Mediterranean throughout history. Hundreds of unique grapevine cultivars are still cultivated in Sicily and its surrounding minor islands, though most of them are menaced by extinction. Wild grapevine is also present with isolated populations thriving along riverbanks. With the aim to evaluate the phylogenetic relationships among Sicilian varieties, and to assess the possible contribution of indigenous wild populations to the genetic makeup of cultivated grapevine, we analyzed 170 domestic cultivars and 125 wild plants, collected from 10 different populations, with 23 SSR markers. We also compared our data with published dataset from Eurasia. Results show that Sicilian wild populations are related to the cultivated Sicilian and Italian germplasm, suggesting events of introgression and/or domestication of local varieties.
Grapevine (Vitis vinifera L.) is one of the most widespread and economically important perennial crops on the planet. It was estimated that in 2016 the world vineyard area was 7.4 million hectares, with a production of 76 million tons of fresh grapes and 269 million hectoliters (mhl) of wine (http://www.oiv.int/). The cultivation of the domesticated grape (V. vinifera L. subsp. sativa (DC.) Hegi) is believed to have started at least 7,000–8,000 years ago from its wild progenitor (V. vinifera L. subsp. sylvestris (Gmel.) Hegi (McGovern, 2003). Archaeological and historical studies evidenced that the primary center of domestication of the grapevine is located between the Near East (Zohary et al., 1996) and the Transcaucasian region (Olmo, 1976), then the grapevine spread around the Mediterranean, following the main civilizations (Carthaginians, Etruscans, Phoenicians, Greeks, and Romans) (McGovern, 2003). During its spreading across the Western Mediterranean regions, the grapevine increased its genetic variability due to the contribution of multiple genetic pools and progressive human selection (Bacilieri et al., 2013). Different studies support the presence of secondary domestication centers, where spontaneous hybridizations among cultivated forms and local wild plants, or direct selection, generated the pattern of the modern Western European cultivars (Grassi et al., 2003; Arroyo-García et al., 2006; Myles et al., 2011; De Andrés et al., 2012; Riaz et al., 2018). Nowadays, more than 6,000 cultivated varieties are recorded (Lacombe et al., 2013; in OIV, 2017). The genotypes of the cultivated vine are highly heterozygous and most of the modern cultivars are hermaphrodite, self-fertile, and easily crossed (This et al., 2006). Several authors reported a high genetic diversity within the subsp. sativa, although it was recently demonstrated that such variability is included within a complex network of close pedigree relationships, derived by crosses among elite cultivars (Myles et al., 2011).
The domestic and the wild vine can be distinguished by morphological differences concerning leaves, flowers, and fruits, although in most cases the distinction of wild grape is hampered by the gene flow between the two subspecies (Di Vecchi-Staraz et al., 2009). The wild grapevine is a dioecious liana that grows in northern Africa, Europe, and the Near and Middle East, in areas between 30° and 50° north latitude. In central and eastern Europe, it thrives in mixed deciduous forests in correspondence with warmer (southern exposure) and humid (valleys of the Rhine, the Loire, the Rhone, the Danube, etc.) microclimates, while in the Mediterranean region it mainly participates in the riparian woodlands (pure or mixed populations dominated by poplars, willows, elms, ash trees, alders in areas with shallow water; pioneer shrub communities with tamarisks and oleanders along the middle-terminal section of the streams; mixed stands with holm and downy oak; shrubby mantle assemblages). At present time wild grapevine has become rather rare due to several forms of human disturbance, such as habitat destruction and fragmentation, silvicultural practices, diffusion of pathogens (e.g., oïdium, phylloxera, mildew, and viruses), improper management of natural environment, and hybridization with domestic forms (Arrigo and Arnold, 2007; Zecca et al., 2010; Garfi et al., 2013; Pacifico et al., 2016; Arnold et al., 2017). Gene flow between wild and cultivated grapevines was confirmed in several countries such as Spain (Arroyo-García et al., 2006; De Andrés et al., 2012), Italy (Zecca et al., 2010) and Georgia (Ekhvaia et al., 2014). In the last years, molecular methods based on the use of microsatellite (SSR) (This et al., 2004; Grassi et al., 2008; Carimi et al., 2011; Lacombe et al., 2013; Emanuelli et al., 2013) and, more recently, on single-nucleotide polymorphism (SNP) markers (Salmaso et al., 2004; Myles et al., 2011; Emanuelli et al., 2013; Laucou et al., 2018; De Lorenzis et al., 2019), as well as on genome sequencing (Zhou et al., 2017) allowed not only to improve the discrimination between wild and cultivated populations, but also to study the relationships among different cultivated varieties and wild accessions.
In Italy, grapevine cultivation is reported since the second half of the 2nd millennium BCE, starting from the Southern regions and then moving northward in the second part of the 1st millennium (Hopf, 1991; Forni, 2012). However, the recent discovery of a large storage jar containing tartaric acid could date back to the Copper Age (early 4th–3rd millennium BCE) the origin of winemaking in Sicily (Tanasi et al., 2017). Sicily and its satellite islets host a rich vascular flora and due to its central position in the Mediterranean, the island has played and still plays a key role in connecting both plant and human populations of neighboring Mediterranean countries. Among plant species V. vinifera subsp. sylvestris is also present in the region with isolated populations mainly thriving along riverbanks (Garfì et al., 2013). Moreover, the island boasts a very ancient and rich tradition of viticulture practices and more than 70 different cultivars have been found in mainland Sicily (Carimi et al., 2010; Carimi et al., 2011). In addition, the Sicilian minor islets have recently emerged as a hotspot of genetic diversity for grapevine. Genetic analyses of this germplasm showed that at least 75 different genetic profiles are present in the Aeolian and Pelagie archipelagos, and the isles of Pantelleria and Ustica. Most of these genetic profiles (39) were not listed in national and international grapevine databases (Gristina et al., 2017). Such notable variety may have originated from domestication of wild autochthonous grapevines as well as from introduction of domesticated varieties from different regions during various historical periods.
In order to provide meaningful insights into grapevine evolution and domestication in the Mediterranean Basin, in this work, we compared the unique plant material constituted by the relict populations of Sicilian wild grapevine to the cultivated local germplasm, as well as to grapevine accessions from Western Europe and Central Asia. To evaluate the phylogenetic relationships among Sicilian varieties, and to assess the possible contribution of indigenous wild populations to the genetic makeup of cultivated grapevines, we analyzed with 23 nuclear SSR markers 170 local cultivars (V. vinifera spp. sativa), from the main Island and surrounding archipelagos, and 125 wild plants (V. vinifera spp. sylvestris) collected from 10 different Sicilian populations.
The list of cultivated and wild accessions analyzed in this study includes all the cultivars that had already been described as traditionally cultivated in Sicily and its minor islands (Carimi et al., 2010; Carimi et al., 2011; Gristina et al., 2017) and all the natural populations identified so far (Garfì et al., 2013), plus other cultivated and wild accessions collected in subsequent surveys.
Most part of the grapevine cultivars and the wild germplasm collected in the field is conserved in the germplasm repository for perennial plants by the Institute of Biosciences and BioResources of the National Research Council of Italy (CNR-IBBR) located in Collesano district (province of Palermo), Italy (37°59'19.9"N, 13°54'55.8"E, 80 m a.s.l.).
Wild Vitis germplasm was collected during several surveys between 2007 and 2016 in the main mountainous and protected areas of Sicily (Figure 1). Considering the morphologic resemblance of wild and cultivated grapevines, in order to reduce as much as possible the risk of collecting plants deriving from naturalized grapevine cultivars or rootstocks, the sampling strategy for V. sylvestris was based on the main differentiating reference traits used to distinguish wild grapevines from domesticated ones (Olmo, 1976; Garfì et al., 2013). Morphological data acquired in situ were integrated by 3–5 years of ampelographic studies carried out on grafted plants at the CNR-IBBR germplasm repository. In total, we collected 131 plants (Supplementary Table S1) from 10 different populations (Supplementary Table S2). Following molecular screening, four clones (3076 = 3074-P6, 3045 = 3058 = 3059 P4, 3139 = 3140-P10) and two feral forms (3109-P8, 3143-P10) were excluded from further analysis (final wild samples analyzed = 125).
Cultivated germplasm was collected between 2006 and 2017 directly from old vineyards. The cultivated accessions were selected following the indications of farmers and labeled for subsequent analysis and plant propagation. In total, 104 accessions were collected from Sicily and 66 from the surrounding minor islands (Table 1).
Table 1 List of cultivated and wild accessions of Vitis vinifera (295) grouped into groups based on their geographic origin and analyzed by 23 SSR markers. The number of samples for each group is presented in brackets.
Total genomic DNA was extracted from young leaves or inner wood of young cuttings. Tissues were ground into fine powder with liquid nitrogen and stored at −80°C until use. The extraction was carried out following the CTAB method (Doyle and Doyle, 1987). DNA was diluted in water to a final concentration of 10 ng/µl, and its quality assessed by spectrophotometric measurements.
Samples were analyzed at 23 SSR loci (Simple Sequence Repeat), i.e., VVS2 (Thomas and Scott, 1993), VVMD5, VVMD6, VVMD7, VVMD17, VVMD21, VVMD24, VVMD25, VVMD27, VVMD28, VVMD32 (Bowers et al., 1996; Bowers et al., 1999), VrZAG62, VrZAG79 (Sefc et al., 1999), VMC1b11 (Zyprian and Töpfer, 2005), VMC4f3.1 (Di Gaspero et al., 2000), VVIb01, VVIh54, VVIn16, VVIn73, VVIp31, VVIp60, VVIq52, and VVIv67 (Merdinoglu et al., 2005). Forward primers were labeled with one of four fluorescent dyes: 6-FAM, ATTO550, ATTO565, or Yakima Yellow. SSRs were grouped in six multiplex pools, each comprising three or four SSRs marked by different dyes, and characterized by similar annealing temperatures (Supplementary Table S3). Twenty-ng DNA per sample were amplified in 96 wells plates by using either the MyTaq HS (Bioline) or the DreamTaq HS (ThermoFisher) DNA polymerases with the following conditions: 15 min at 95°C (Taq activation step), followed by seven cycles consisting of 30 s at 94°C (denaturation), 90 s at the appropriate annealing temperature (Supplementary Table S3; touch-down step, with temperature decreasing by 1°C each cycle), 1 min at 72°C (extension). Additional 25 cycles with the same conditions maintained the final annealing temperature constant (Supplementary Table S3). Finally, the final PCR step was set for 30 min at 60°C. PCR products were size-separated by capillary electrophoresis performed on a genetic analyzer (ABI Data analysis Prism3130, Applied Biosystems, Inc.) by an external service (Eurofins Genomics, Germany). Electropherograms were visually verified by Gene Mapper v. 5.0 software. Allele size was estimated by comparing the fragment peaks with the internal size standard, using the default method for size calling with SSR and the expected repeat size. To correct for amplification shifts among different PCRs, SSR profiles were normalized by including in each amplification run the DNA of standard cultivars Pinot Noir, Sauvignon Blanc, and Zibibbo. For comparison with the published dataset from Riaz et al. (2018), we used a subset of common core of 17 SSRs, excluding VrZAG62, VrZAG69, VVS2, VVMD5, VVMD17, and VVMD6 from our profiles. For normalization among datasets, we used two common varieties, namely Sangiovese (present with the synonym Minutidda in our dataset) and Zibibbo.
To identify ferals, i.e., wild accessions with at least one cultivated parent, we performed a parentage analysis between Sicilian sylvestris and cultivated accessions using Bayes' theorem with the R/Solomon package (Christie et al., 2013). The identified ferals (2) were removed from the set of genotypes on which the analyses were performed.
Several diversity parameters were estimated using GenAlEx 6.5 (Peakall and Smouse, 2012): the number of alleles per locus (Na), the number of effective alleles per locus (Ne), the observed (Ho) and expected (He) heterozygosity (Nei, 1978; Nei, 1987), and the fixation index (F). At population level, pairwise Nei's genetic distances and Fst value were calculated. Inbreeding coefficients Fis and Fit were calculated using Arlequin ver. 3.5.2.2 (Excoffier and Lischer, 2010).
The NJ (Neighbor-Joining) phylogenetic tree was designed by using R/ggtree package (Yu et al., 2017) with Nei's distance. The bootstrap analysis was performed based on 1,000 resamplings.
Genetic relationships among the studied genotypes were investigated by Discriminant Analysis of Principal Components (DAPC). DAPC, implemented in the R/adegenet (Jombart, 2008), was performed to infer population subdivision of the analyzed collection, regardless of the geographic origin. The number of principal components (PCs) retained was evaluated using the cross-validation procedure.
Principal coordinates analysis (PCoA) was performed by GenAlEx 6.5 via Covariance matrix with data standardization.
To identify the number of genetic groups in the wild populations and to investigate their relationships with domesticated cultivars we used the software STRUCTURE version 2.3.4 (Pritchard et al., 2000) that employs a model-based Bayesian clustering method. The estimate of the most likely number of genetic groups (Ks) was performed following Pritchard and Wen (2003) and the simulation study by Evanno et al. (2005), which proposed an ad hoc statistic, DELTA K. For each K, 20 independent runs (100,000 burn-in, 1,000,000 Marchov Chain Monte Carlo) were carried out. All runs were performed with the admixture model. The 20 runs were averaged using the software CLUMPP (CLUster Matching and Permutation Program; Jakobsson and Rosenberg, 2007), and shown in histograms using the program Distruct (Rosenberg, 2004). For the hierarchical analysis, samples showing an ancestry value lower than 0.80 to any cluster were removed. The remaining subsets, one for each cluster, were independently subject to a second round of STRUCTURE analysis, as in Emanuelli et al. (2013), following the procedure described above.
One of the most obvious traits distinguishing sativa vs. sylvestris subspecies is the flower structure, since wild grapevine is dioecious whereas flowers of V. vinifera subs. sativa are usually hermaphroditic. In order to verify that plants collected in putative wild populations showed the dioecious phenotype, we analyzed flower morphology either during collection, or in subsequent years for those plants that had been transferred in the germplasm repository. Supplementary Table S1 indicates the flower morphology for each plant. As expected, all the 170 cultivated plants had hermaphroditic flowers. Among the wild plants, we could assign a gender only to 122 out of 131 plants (93%), since in nine plants flowers and fruits were not evident at the collection time, and the scions did not survive grafting in the germplasm repository. Among the remainders, 68 plants were clearly females and 54 males, thus allowing us to exclude hermaphrodite plants that are usually considered feral or naturalized forms.
The Sicilian wild and cultivated germplasm was first screened to identify clones and ferals. The four clones and two ferals identified by parentage analysis were then removed from our dataset.
The genetic profiles of the 170 cultivated and 125 wild accessions at 23 nuclear SSR loci are shown in Supplementary Table S4, and their statistics in Table 2. The total number of alleles (Na) was 314, with a mean value per locus of 13.7. The marker VVIn73 showed the lowest values of Na, effective alleles (Ne), observed and expected heterozygosity (Ho and He), whereas the marker VVMD28 the highest values, with the exception of Ho, where the maximum was present in VrZag62. The F value ranged from −0.056 (VMC1b11) to 0.403 (VVMD17), with a mean value of 0.093.
Table 2 Genetic diversity indices calculated for 295 distinct Sicilian genotypes belonging to sativa and sylvestris accessions.
Genetic diversity analysis at population level shows that the number of alleles (Na) was similar between the cultivated pool (11.8) and the wild pool (10.8 as average), with wild populations ranging from 2.8 (P3, P4) to 7.0 (P9) (Table 3). For the number of effective alleles (Ne), the lowest value was in P4. In the cultivated pool, the observed and expected heterozygosity (Ho and He) were similar (0.697 and 0.741, respectively). The fixation index (F) and the inbreeding coefficient (Fis) were close to zero (0.067 and 0.025, respectively). In wild populations, P1 showed a marked positive F value (0.143), while in the other populations F was negative or close to zero, with P4 showing the lowest value (−0.567). Similarly Fis was strongly negative in P4 showing the lowest Fis value (−0.715).
Table 3 Genetic diversity estimates for wild populations and cultivated grapevines accessions analyzed from Sicily.
The pairwise Nei's genetic distances and Fst values for all the wild populations and the cultivated pool is shown in Table 4. Nei's genetic distance ranged from 0.926 (P4-P5) to 0.083 (P8-cultivated). Fst values confirmed the pattern, with the highest value 0.324 for the pair P5-P4 and the lowest value 0.025 for cultivated-P8. Comparing the wild accessions altogether with the cultivated pool, Nei's genetic distance was 0.147, Fst 0.042 (p < 0.001), Fis 0.02667 (p < 0.001) and Fit 0.07406 (p < 0.001).
Table 4 Estimates of pairwise Fst values (below the diagonal) and Unbiased Nei's genetic distance (above the diagonal) within overall wild and cultivated Sicilian accessions.
The genetic diversity of wild and cultivated Sicilian grapevines was first assessed by DAPC analysis of the SSR profiles (Figure 2A). The cultivated samples formed a compact cluster in the upper right part of the graph, whereas the wild samples were scattered along the left and the lower sides of the axes. Populations 4 and 5 were the most divergent along the y and x axes, respectively. P4 formed a separate pool, neither related to the other wild populations nor to the cultivated cluster; P5 was less homogeneous and it was clearly connected to other wild populations, yet it stood the furthest apart from the cultivated pool. Interestingly, three samples from wild populations, from P1, P6, and P8, lied amidst the cluster of sativa, possibly indicating cases of genetic introgression. Conversely, few cultivated samples fell close to sylvestris pools. These cultivars were: Bracaù, Lorisi, Mantonico B, and Tintorè (Supplementary Dataset S1).
Figure 2 Analyses of Sicilian sativa and sylvestris germplasm. Discriminant Analysis of Principal Components (DAPC) (A); Principal coordinates analysis (PCoA) (B); first round of STRUCTURE (C) with percentage (pies) for each cluster and population (D); second round of STRUCTURE for cluster A (E) and cluster B (F).
Samples were also discriminated by PCoA analysis, that is based on genetic distances (Figure 2B). The distribution pattern closely resembled the one originated by DAPC, with P4 forming an isolated pool, and the cultivated samples clustering on one side of the main axis. Interestingly, the PCoA confirmed that individual plants from P1, P6, and P8 were admixed within the cultivated cluster.
A third independent analysis of the genetic diversity in Sicilian wild and cultivated germplasm was performed by using STRUCTURE. With this method, the most likely sorting indicated two genetic clusters, A and B (Figure 2C, Supplementary Dataset S1). Almost all the cultivated plants (98%) belonged to the cluster B (Figure 2D). Of these, most of them (95%) had an ancestry value higher than 0.65, and 86% higher than 0.80, indicating a strong link to this cluster (Supplementary Dataset S1). Cluster B also included all samples from wild population P4, all of them with ancestry values higher than 0.80, and few individuals from P1, P6, and P8. Cluster A included the majority (76%) of wild plants. Looking in detail the ancestry values of each population, P3, P5, P7, and P10 had all individuals belonging to cluster A. The remaining populations, with the exception of the above mentioned P4, showed variable degrees of association to cluster A (ranging from 95% of P9 to 54% of P8). The association strength to cluster A was high for all wild populations, with ancestry values higher than 0.80 in most cases. The exceptions were P4, as discussed above, and P8, that was equally mixed between the two clusters, and showed low ancestry values (<0.65) in most cases. Interestingly, the cluster A also included four cultivated plants (2% of all cultivated). These varieties are Austina bianca, Bracaù, Giugnatica, and Mantonico B. However, the ancestry values were lower than 0.65 for all these plants, indicating a weak association to the cluster.
Since STRUCTURE did not differentiate among the wild populations, except P4, and between P4 and the cultivated pool, we performed a hierarchical STRUCTURE analysis on the outcome of the first round. In the second round, samples from cluster A split into four subclusters (Figure 2E, Supplementary Dataset S1). Only two populations, P3 and P10, were equally split between two subclusters. Each remaining population belonged exclusively (P2, P5, P6, P7, P8) or predominantly (90% P1; 94% P9) to single subclusters. For cluster B, the second round of STRUCTURE revealed five subclusters (Figure 2F, Supplementary Dataset S1). While the cultivated accessions and the few wild individuals of P1, P6, P8, and P9 (that in the first round grouped in cluster B) showed a very mixed pattern among the five subclusters, all individuals from P4 strongly grouped together in a private subcluster (ancestry value > 0.80 in all cases; Supplementary Dataset S1).
Finally, genetic distances among the Sicilian samples were also visualized in a phylogenetic tree (Supplementary Figure S1). The tree confirmed that most of sylvestris populations formed compact branches, indicating that individuals within a population were closely related with each other. The exception was P8 and a small part of P1, P6, and P9, whose individuals were interspersed among the cultivated samples.
Recently, Riaz et al. (2018) analyzed a large set of cultivated and wild grapevine accessions from across the Mediterranean basin and Central Asia by 20 nuclear SSRs. In order to frame the genetic structure of the Sicilian germplasm within the geographical distribution of the species, we compared the profiles of 17 SSRs, that represented a common set in the two datasets. The genetic parameters for the markers analyzed are shown in Table 5. Overall, ranges and mean values of each parameter were similar to those of the Sicilian germplasm. In the wider survey, we observed a higher number of alleles (Na and Ne), indicating an increased polymorphism in the largest dataset, as expected, especially since Central Asian populations are characterized by high genetic diversity (Riaz et al., 2018).
Table 5 Genetic diversity indices calculated for 1,673 genotypes from Europe to Asia belonging to sativa and sylvestris accessions.
Since the dataset by Riaz et al. (2018) contains 289 Italian wild accessions, we first compared our Sicilian wild samples against this subset. PCoA analysis showed that the two pools were clearly separated, and that the Sicilian samples were characterized by higher diversity along the second axis (Supplementary Figure S2). Therefore, the Sicilian wild dataset was not redundant with the Italian dataset, and it could be compared with all the other samples.
The DAPC analysis of all the cultivated and wild accessions from the Mediterranean and Central Asia, including the Sicilian populations, showed a triangle-shaped distribution (Figure 3A). The center of the triangle was populated by the wild samples from Croatia and the cultivated accessions from all the regions. The exceptions were Italy and Sicily, which clustered in the lowest vertex, together with all the Sicilian wild populations. The upper vertex included the sylvestris samples from Western Countries (Spain, France, Italy), whereas the rightmost vertex included the Eastern sylvestris populations (Armenia, Azerbaijan, Georgia). Similarly, the PCoA graph differentiated the Eastern from the Western wild samples. However, all the cultivated samples, including the Sicilian and the Italian, and the wild Sicilian grouped together in this analysis (Figure 3B).
Figure 3 Analyses of Sicilian, Mediterranean and Central Asian sativa and sylvestris germplasm. Discriminant Analysis of Principal Components (DAPC) (A); Principal coordinates analysis (PCoA) (B); first round of STRUCTURE (C) with percentage (pies) for each cluster and population (D); Second round of STRUCTURE for cluster B (E) with percentage (pies) for each subcluster and population (F).
We then analyzed all data by STRUCTURE. The addition of the Sicilian germplasm did not vary the most likely number of clusters compared to the original analyses by Riaz et al. (2018), with K = 3 (Figure 3C). As in Riaz et al. (2018), one cluster (B) dominated all the cultivated samples, regardless of their origin (Figure 3D). A second cluster (C) characterized Asian wild samples. The third cluster (A) was the most abundant for almost all the wild Spanish, French, and Italian samples. The Croatian and Georgian wild samples were especially mixed between cluster A-B and B-C, respectively. A similar situation was observed for the Sicilian wild samples: the major component was cluster B, but many samples showed an important contribution of cluster A. When the major contribution for each sample was considered, 90% of Sicilian wild samples were dominated by cluster B, and 10% by cluster A. Among the 12 samples belonging to cluster A, seven had an ancestry value higher than 0.65, and three exceeded 0.80 (Supplementary Dataset S2). When we looked at the distribution within each Sicilian population, only P5 had a large amount of samples (6 out of 9, 67%) belonging to cluster A; six populations (P3, P4, P6, P7, P8, P10) clustered entirely in cluster B; the remaining populations had a few samples in cluster A. All the cultivated Sicilian samples showed cluster B as major component. Nevertheless, Bracaù also had 22% association with cluster A, Austina bianca 19%, and Giugnatica 18%. For all the other samples, the ancestry value for cluster B was higher than 0.80.
Finally, we run a second round of STRUCTURE on the samples closely associated to cluster B, which included most of the Sicilian germplasm and the sativa accessions from all the regions. We identified three subclusters (Figures 3E, F, Supplementary Dataset S2). Subcluster BA included most of the Eastern sativa (Georgia 98%; Turkmenistan 83%; Pakistan 70%) and the residual Italian, Croatian, and Georgian sylvestris that were grouped in cluster B in the first round. Subcluster BB included most of the Sicilian germplasm, both sylvestris (98%) and sativa (94%), and more than half of the Italian sativa accessions (58%). Subcluster BC included almost all the Spanish and French cultivated accessions (98% and 93%, respectively).
For millennia, grapevine cultivation has been central in the culture and economy of many regions across the Mediterranean Basin and Middle East, with thousands of different varieties selected. Yet, the exact dynamics of grapevine domestication remains elusive, and subject of a passionate debate among scholars of different fields. In the last decades, molecular techniques have expanded our ability to dig into the genetic signatures left along the history of crossing and migrations. Recent studies have generated genetic profiles for hundreds of cultivated and wild grapevine accessions, though the geographical origin of the sampled material is generally unequal, with Eastern and Southern populations highly underrepresented. In this study, we compared the genetic structure of wild and cultivated grapevine germplasm from Sicily, a region that has been so far overlooked. Due to its size and its central position in the Mediterranean, bridging between Europe and Africa, Sicily has always played a key role in the migration routes crossing the Mediterranean basin, both for natural dispersion of species and during human migrations. The recent discovery of a jar containing wine residues dates back the winemaking culture in Sicily to the 4th–3rd millennium BCE, i.e., at approximately the same time of the oldest wine traces found in the Armenia, and about 2,000 years later than the oldest evidence of wine production in Georgia, considered the primary domestication center for grapevine (Areshian et al., 2012; Tanasi et al., 2017; McGovern et al., 2017). For all these reasons, Sicily might have potentially played a major role in the domestication history of grapevine.
We first focused on the comparison between cultivated and wild populations within the Sicilian germplasm. The DAPC and the PCoA distinguished the cultivated from the wild germplasm along the main axis, reflecting major genetic distances between the two groups. Wild samples were especially scattered, indicating a high degree of diversity, probably due to the genetic isolation of most of the populations. The exception was the wild population P4, which separated from the cultivated pool only along the secondary axis, and was not connected with the other wild populations. STRUCTURE confirmed the major divergence between cultivated and wild samples. However, P4 initially grouped together with the cultivated pool, validating the affinity suggested by DAPC and PCoA. Only a second round of STRUCTURE separated P4 from sativa, matching the segregation observed along the secondary axis of DAPC and PCoA. These results, together with the Fst, and Nei values, that were highest in the pairwise comparisons of P4 with the other wild groups, suggest that P4 is very different from the other Sicilian wild populations, as confirmed by the Fis value, and that is related to the cultivated accessions. We had already noticed the peculiar genetic makeup of P4 before, though using only six SSR markers (Garfì et al., 2013). It was speculated that the isolation and the unique ecological context of P4 (i.e., a scree-type instead than the usual riparian habitat, Supplementary Table S2) likely affected its propagation strategy, relying more on specialized faunal dispersion of seeds than on hydraulic dynamics. In this study, we also observed that P4 was the population showing the highest excess of heterozygosity (F and Fis index), suggesting that it might suffer from genetic introgression from external sources, for example by fertilization of female sylvestris plants with pollen from cultivated plants growing nearby, as prompted by the presence of abandoned fields in the surroundings. Intriguingly, all 18 individuals sampled in P4 turned out to be females (Supplementary Table S1), a pattern that cannot be explained by random sampling in a natural population of this size. Moreover, we excluded that P4 was a clonal population, spreading by vegetative propagation or apomixy, since genetic analysis revealed that only two plants were clones. All the other individuals were genetically different, though closely related to each other, as evident in the DAPC, PCoA, the second round of STRUCTURE and phylogenetic tree. A possible explanation would be that P4 derives from old dioecious varieties, an uncommon feature in modern cultivated plants, or from hybrids V. vinifera x Vitis spp., during the early attempts to transfer phylloxera resistance traits to European grapes. That would explain the dioecious phenotype and the affinity with cultivated varieties. We also have to consider that SSRs are neutral molecular markers, whereas the expression of phenotypic traits can be influenced by environmental conditions. Therefore, it is possible that natural settings (forest or riverbank environment, as opposed to agricultural environment) can favor the expression of a more sylvestris-like morphology, while more sativa-type traits are masked. Finally, during our collection surveys, we deliberately sought plants possessing all the typical sylvestris features, such as a dioecious flower, ignoring those with hermaphroditic flowers, that could randomly appear at each generation in some individuals if a population maintains a significant sativa contribution. Yet, P4 forms a compact cluster in all the analyses we have performed, therefore the putative introgression events must have occurred in a common ancestor of all current individuals. Alternatively, P4 might represent the residues of an ancient sylvestris population that contributed to the genetic structure of many modern Sicilian and Italian cultivars, as discussed below.
Stronger evidence of introgression from the sativa pool characterizes P8, since this population largely mixed with the cultivated pool in all our analyses. Moreover, the STRUCTURE ancestry values of most accessions from P8 were weak, indicating mixed profiles between the sativa and sylvestris clusters. Confirming this hypothesis, the pairwise genetic distance between P8 and the cultivated pool was very low, and individuals from P8 were dispersed among sativa accessions in the phylogenetic tree. Population P8 is located in the Anapo Valley at Pantalica, a site with a large Neolithic necropolis that is known to be actively inhabited since ancient times, and that is currently surrounded by cultivated fields. To a minor extent, we also observed evidence of introgression in single individuals of P1 and P6, since a few samples clustered together with the sativa group in the DAPC, PCoA, STRUCTURE, and the phylogenetic tree. The genetic isolation of these populations was also confirmed by the positive inbreeding coefficient value (Fis). We suspect introgression with cultivated germplasm also for P3, P5, P7, P10 given that the observed heterozygosity (Ho) values are higher than the expected (He). Nevertheless, the negative Fis value showed by the latter populations, indicating an excess of heterozygosity, could be also due to the following different factors: the small population size, overdominant selection favoring heterozygote survival (heterosis) self-incompatibility system effect, proportion of asexual reproduction and effect of clonal reproduction on the number of heterozygotes (asexuality effect) (Stoeckel et al., 2006). All the other sylvestris samples are more isolated, showed different cluster distribution compared to the sativa pool and grouped together in the phylogenetic tree, suggesting that their genetic connection with the cultivated pool was weaker. In particular, P5 was the population less related to the sativa group, and more similar to the sylvestris germplasm from Italy, France, and Spain.
Taking advantage of the extensive study by Riaz et al. (2018), we compared the Sicilian germplasm to cultivated and wild accessions from Western Europe and Central Asia. The dataset used by Riaz et al. (2018) comprises a large number of Italian sylvestris (289 accessions). The exact geographical origin of each accession is not specified in the paper, yet we ascertained that Riaz et al.'s dataset does not comprise any Sicilian sample (De Lorenzis, personal communication), as opposed to the larger collection from which the wild Italian samples from Riaz et al. derived (Biagini et al., 2014). Accordingly, we did not find any clone between our dataset and the one from Riaz et al. (2018). Moreover, DAPC and PCoA showed that the Sicilian sylvestris samples were very distantly related to the other wild Italian populations, including also those from the neighboring region Calabria, suggesting that the Sicilian wild populations are genetically isolated from the rest of Italy.
By using the combined dataset, the DACP, PCoA, and STRUCTURE analyses confirmed what observed by these authors, with three main clusters discriminating: i) a Western sylvestris pool, ii) an Eastern sylvestris pool, and iii) the cultivated germplasm, regardless of their origin. The latter cluster also contained the wild Croatian samples. The samples falling in the transition zones among these clusters might suggest events of gene flow between wild populations and the cultivated germplasm in these regions, as previously reported from several investigations (Arroyo-García et al., 2006; Myles et al., 2011; De Andrés et al., 2012; Riaz et al., 2018). In addition to these three main pools, the DAPC also showed a fourth cluster which included most of the Sicilian sylvestris plants and all the Sicilian and Italian cultivated samples, suggesting that Sicilian and Italian cultivars are closely related, as expected due to the close geographical proximity, the deep historical connections between the two areas, and the intense commercial exchanges. Moreover, the Sicilian and Italian cultivars were more related with each other, and with the Sicilian sylvestris, than with other cultivars worldwide, suggesting events of genetic isolation and/or local secondary domestication, with introgression of genetic material from the Sicilian wild germplasm (possibly, from populations related to current P4) into the cultivated Italian pool. The latter hypothesis is consistent with the assumption that grapevine cultivation in Italy spread from the Southern regions northward since the second part of the 1st millennium BCE (Hopf, 1991; Forni, 2012).
The two-step analysis through STRUCTURE provided some additional information. In the first round of STRUCTURE, the cultivated Sicilian germplasm clustered together with most of the other sativa accessions; on the contrary, the wild populations showed a mixed distribution, clustering in part with the cultivated accessions and in part with the Western sylvestris pool, a situation similar to what observed in Croatia and, as for the Eastern cluster, in Georgia and Armenia. The different results obtained from STRUCTURE in the analysis of the Sicilian germplasm alone (where the cultivated pool clearly differed from the wild populations) and the wide scale analysis, might depend on the number of SSR markers used (23 in the first analysis and 17 in the second) and on the larger genetic diversity present in the world dataset, that may hinder the smaller differences within the Sicilian accessions. However, the second round of STRUCTURE clearly distinguished a cluster including nearly all the Sicilian germplasm, both sylvestris and sativa, and more than half of the Italian cultivated accessions, thus confirming the affinity among these groups, already observed in the DAPC analysis. The hierarchical STRUCTURE also separated the cultivated accessions from other regions of the world in two additional clusters. One included almost all samples from Western Europe (Spain and France); a third cluster included the Eastern sativa accessions (Georgia, Pakistan, Turkmenistan) plus sylvestris form Georgia, Croatia and Italy. Interestingly, about half of the Italian (42%) cultivated accessions also showed affinity for this cluster. Therefore, the Italian cultivars are very different from the rest of Western Europe, and appear as mix between the Eastern group and the Sicilian pool. Accordingly, the list of Italian sativa accessions grouping together with the Sicilian germplasm consists predominantly (15 out of 18) of cultivars from Southern regions (Aglianico, Aglianicone, Catarratto Foglia tonda, Frappato, Grillo, Magliocco, Malvasia, Malvasia del Lazio, Malvasia nera di Brindisi, Montonico, Nerello cappuccio, Primitivo, Sangiovese, Sciaccarello, Zibibbo) with the exception of three cultivars that are from Northern Italian regions (Glera, Ribolla gialla, and Schiava lombarda), pointing to a close relationship of this group with the Sicilian germplasm. Conversely, the remaining Italian cultivars, which show affinity with the Eastern pool, are varieties mostly cultivated in the Northern regions (Albarola, Barbera, Brugnola, Butascera, Croatina bianca, Croatina int. corto, Luglienga bianca, Marzemino, Merlina, Moradella di Montalto, Rossara, Rossola, Schiava grossa, Sirica).
The close relationship between the Sicilian sylvestris and the Sicilian and Italian sativa pools, observed in the wide DAPC, PCoA, and STRUCTURE analyses, is intriguing and can be explained by two different hypotheses. First, it is possible that many Sicilian wild populations suffer from introgression of sativa germplasm. This scenario is plausible, considering many different factors, such as the relatively small extension of the island, its millennial history of exploitation, the ancient reduction of its original forest cover, the importance of viticulture in the local economy with extensive fields, and the diffusion of recent diseases threatening the natural populations (Pacifico et al., 2016). We especially found strong evidence for this situation in population P8. Alternatively, it is possible that the current wild Sicilian populations are phylogenetically related to a sylvestris group that has not been identified yet, or is even extinct, and that contributed to the early domestication of grapevine. In that case, the residual current Sicilian wild populations maintain a close link to the cultivated germplasm or even directly contributed to the development of some local sativa varieties. For its genetic homogeneity, separation from the other sylvestris populations and its relation to the cultivated Sicilian and Italian pool, P4 represents an intriguing candidate.
In agreement with this hypothesis, our analyses indicated a few Sicilian cultivated varieties as closely related to the Sicilian wild germplasm, namely Austina bianca, Bracaù, Giugnatica, Lorisi, Mantonico, and Tintorè. Unfortunately, we could retrieve very little historical information for these varieties. Austina bianca is a white grape variety cultivated in the province of Palermo, it was traditionally used for table and wine production. Bracaù, also known as Grecaù, is not mentioned in ancient literature. It is a black berry vine grown in the province of Catania, traditionally used for wine production (Carimi et al., 2010). Giugnatica is a red table grape grown in the Aeolian archipelago. It is considered an early grape that ripens in June. The first citation of Lorisi dates back to the beginning of the nineteenth century. Geremia (1836) mentioned Lorisi, also known as Visparu (Geremia, 1839) in a review of wine varieties found in the vineyards of Etna valley, province of Catania. It was used to make good quality sweet white wines and for the production of raisins. In the second half of the nineteenth century, Caruso (1869) mentions two forms of Lorisi (white and black berry) grown in the area of Cefalù, province of Palermo. Mantonico, existing as white and black berry versions, has been described in Sicily under different names: Muntonicu, Montonico nero femminino, Mantonicu niuru fimmineddu, Montonico nero and Mantonicu niuru for the black berry version, and Montonico bianco, Mantonicu vrancu, Mantonicu masculu for the white berry version. The first citations of this vine dates back to the early 1500s (Venuti, 1516), and later on it was also mentioned by Cupani (1696), Sestini (1812) and Minà Palumbo (1891). In the Aeolian archipelago, where our accession was collected, the red grape form is considered a traditional local variety and is used to produce sweet wines (Gristina et al., 2017). The Tintorè grape, of unknown origin, was found in the province of Agrigento and used to darken the wine. There is no historical information on this grape variety in Sicily. Our results show that Sicilian wild populations are related to the cultivated Sicilian and Italian germplasm, suggesting events of introgression and/or domestication of local varieties. It is thus intriguing to speculate that these ancient Sicilian varieties may derive from local sylvestris germplasm.
The comparison of the genetic structure of Sicilian sylvestris populations with the cultivated local germplasm and the grapevine accessions across Western Europe and Central Asia confirms the genetic separation between the Western and Eastern sylvestris pools, and their connections with the cultivated germplasm. The Sicilian wild populations appeared closely related to the local cultivated germplasm, probably due to gene flow between the two pools, for either hybridization or early events of introgression of the sylvestris germplasm into sativa accessions. Considering the archeological evidences that point to Sicily among the oldest centers in grapevine cultivation (Copper Age, early 4th–3rd millennium BCE), it is plausible that the genetic affinity among current Sicilian sylvestris and sativa germplasms derives from early domestication events occurred in this region. The data set and the results presented here, in a region of primary interest for understanding domestication, migration, and expansion of grape around the Mediterranean basin, may contribute to facilitate future investigations to further unravel the phylogenetic history and population dynamics of grapevine.
All datasets for this study are included in the article/ Supplementary Files.
RM and FC conceived and supervised the project. RM, AG, LA, AM, GG and FC contributed to collect plant materials. RM, DC and FB performed the genetic characterization of the plant material. RM, AG and IF analysed the data. RM, AG, IF, and FC analysed and interpreted data. RM, AG, DP and FC wrote the first draft. All authors made a substantial, direct and intellectual contribution to this work. All of the author approve the final version of the manuscript.
This research was supported by Regione Siciliana (PSR Sicilia 2007–2013, Sottomisura 214/2A-Preservazione della biodiversità: Centri pubblici di conservazione, Grant No. 94750767637).
The authors declare that the research was conducted in the absence of any commercial or financial relationships that could be construed as a potential conflict of interest.
We are grateful to Mr. Giacomo and Alessandro Mannone of 'Mannone Nurseries' in Petrosino (district of Trapani) for helping us to the identification of main Sicilian grapevines and for supplying nursery plants.
The Supplementary Material for this article can be found online at: https://www.frontiersin.org/articles/10.3389/fpls.2019.01506/full#supplementary-material
Areshian, G. E., Gasparyan, B., Avetisyan, P. S., Pinhasi, R., Wilkinson, K., Smith, A., et al. (2012). The chalcolithic of the Near East and south-eastern Europe: discoveries and new perspectives from the cave complex Areni-1, Armenia. Antiquity 86, 115–130. doi: 10.1017/s0003598x00062499
Arnold, C., Bachmann, O., Schnitzler, A. (2017). Insights into the Vitis complex in the Danube floodplain (Austria). Ecol. Evol. 7, 7796–7806. doi: 10.1002/ece3.3187
Arrigo., N., Arnold, C. (2007). Naturalised rootstocks in Europe and consequences to native wild grapevine. PloS One 2, e521. doi: 10.1371/journal.pone.0000521
Arroyo-García, R., Ruíz-García, L., Bolling, L., Ocete, R., López, M. A., Arnold, C. et al. (2006). Multiple origins of cultivated grapevine (Vitis vinifera L. ssp. sativa) based on chloroplast DNA polymorphisms. Mol. Ecol. 15, 3707–3714. doi: 10.1111/j.1365-294X.2006.03049.x
Bacilieri, R., Lacombe, T., Le Cunff, L., Di Vecchi-Staraz, M., Laucou, V., Genna, B., et al. (2013). Genetic structure in cultivated grapevines is linked to geography and human selection. BMC. Plant Biol. 13, 25. doi: 10.1186/1471-2229-13-25
Biagini, B., De Lorenzis, G., Imazio, S., Failla, O., Scienza, A. (2014). Italian wild grapevine (Vitis vinifera L. subsp. sylvestris) population: insights into eco-geographical aspects and genetic structure. Tree Genet. Genomes 10 (5), 1369–1385. doi: 10.1007/s11295-014-0767-4
Bowers, J. E., Dangl, G. S., Vignani, R., Meredith, C. P. (1996). Isolation and characterization of new polymorphic simple sequence repeat loci in grape (Vitis vinifera L.). Genome 39, 628–633. doi: 10.1139/g96-080
Bowers, J. E., Dangl, G. S., Meredith, C. P. (1999). Development and characterization of additional microsatellite DNA markers for grape. Am. J. Enol. Viticult. 50, 243–246.
Carimi, F., Mercati, F., Abbate, L., Sunseri, F. (2010). Microsatellite analyses for evaluation of genetic diversity among Sicilian grapevine cultivars. Genet. Resour. Crop Ev. 57 (5), 703–719. doi: 10.1007/s10722-009-9506-3
Carimi, F., Mercati, F., De Michele, R., Fiore, M. C., Riccardi, P., Sunseri, F. (2011). Intra-varietal genetic diversity of the grapevine (Vitis vinifera L.) cultivar 'Nero d'Avola' as revealed by microsatellite markers. Genet. Resour. Crop Ev. 58 (7), 967–975. doi: 10.1007/s10722-011-9731-4
Caruso, G. (1869). Trattato di viticoltura e vinificazione. ovvero il presente e l'avvenire enologico dell'Italia meridionale. Stamperia di Giovanni Lorsnaider: Palermo.
Christie, M. R., Tennessen, J. A., Blouin, M. S. (2013). Bayesian parentage analysis with systematic accountability of genotyping error, missing data and false matching. Bioinformatics 29 (6), 725–732. doi: 10.1093/bioinformatics/btt039
Cupani, F. (1696). Hortus Catholicus seù illustrissimi, and excellentissimi principis Catholicae. Neapoli: Ed. Benzi.
De Andrés, M. T., Benito, A., Pérez-Rivera, G., Ocete, R., Lopez, M. A., Gaforio, L. et al. (2012). Genetic diversity of wild grapevine populations in Spain and their genetic relationships with cultivated grapevines. Mol. Ecol. 21 (4), 800–816. doi: 10.1111/j.1365-294X.2011.05395.x
De Lorenzis, G., Mercati, F., Bergamini, C., Cardone, M. F., Lupini, A., Mauceri, A. et al. (2019). SNP genotyping elucidates the genetic diversity of Magna Graecia grapevine germplasm and its historical origin and dissemination. BMC Plant Biol. 19 (1), 7. doi: 10.1186/s12870-018-1576-y
Di Gaspero, G., Peterluner, E., Testolin, R., Edwards, K. J., Cipriani, G. (2000). Conservation of microsatellite loci within the genus Vitis. Theor. Appl. Genet. 101, 301–308. doi: 10.1007/s001220051483
Di Vecchi-Staraz, M., Laucou, V., Bruno, G., Lacombe, T., Gerber, S., Bourse, T., et al. (2009). Low level of pollen-mediated gene flow from cultivated to wild grapevine: consequences for the evolution of the endangered subspecies Vitis vinifera L. subsp. silvestris. J. Hered. 100, 66–75. doi: 10.1093/jhered/esn084
Doyle, J. J., Doyle, J. L. (1987). A rapid DNA isolation procedure from small quantities of fresh leaf tissue. Phytochem. Bull. 19, 11–15.
Ekhvaia, J., Gurushidze, M., Blattner, F. R., Akhalkatsi, M. (2014). Genetic diversity of Vitis vinifera in Georgia: relationships between local cultivars and wild grapevine, V. vinifera L. subsp. sylvestris. Genet. Resour. Crop Ev. 61, 1507–1521. doi: 10.1007/s10722-014-0125-2
Emanuelli, F., Lorenzi, S., Grzeskowiak, L., Catalano, V., Stefanini, M., Troggio, M., et al. (2013). Genetic diversity and population structure assessed by SSR and SNP markers in a large germplasm collection of grape. BMC Plant Biol. 13, 39. doi: 10.1186/1471-2229-13-39
Evanno, G., Regnaut, S., Goudet, J. (2005). Detecting the number of clusters of individuals using the software structure: A simulation study. Mol. Ecol. 14, 2611–2620. doi: 10.1111/j.1365-294x.2005.02553.x
Excoffier, L., Lischer, H. E. (2010). Arlequin suite ver 3.5: A new series of programs to perform population genetics analyses under Linux and Windows. Mol. Ecol. Resour. 10, 564–567. doi: 10.1111/j.1755-0998.2010.02847.x
Forni, G. (2012). "The origin of "old world" viticulture," in Caucasus and Northern Black Sea region ampelography. Eds. Maghradze, L., Rustioni, A., Scienza, J., Turok, O., Failla, D. Vitis, special issue. (VITIS, The Journal of Grapevine Research.), 27–38.
Garfì, G., Mercati, F., Fontana, I., Collesano, G., Pasta, S., Vendramin, G. G., et al. (2013). Habitat features and genetic integrity of wild grapevine Vitis vinifera L. subsp. sylvestris (C.C. Gmel.) Hegi populations: a case study from Sicily. Flora 208, 538–548. doi: 10.1016/j.flora.2013.08.005
Geremia, G. (1836). Vertunno Etneo ovvero Stafulegrafia, storia delle varietà delle uve che trovasi nel d'intorno dell'Etna. Atti. dell'Accademia Gioenia. Catania. 11, 313–340.
Geremia, G. (1839). Vertunno Etneo ovvero Stafulegrafia, storia delle varietà delle uve che trovasi nel d'intorno dell'Etna. Atti. dell'Accademia Gioenia. Catania. 14, 3–5557–68.
Grassi, F., Labra, M., Imazio, S., Spada, A., Sgorbati, S., Scienza, A., et al. (2003). Evidence of a secondary grapevine domestication centre detected by SSR analysis. Theor. Appl. Genet. 107, 1315–1320. doi: 10.1007/s00122-003-1321-1
Grassi, F., Mattia, F. D., Zecca, G., Sala, F., Labra, M. (2008). Historical isolation and Quaternary range expansion of divergent lineages in wild grapevine. Biol. J. Linn. Soc. 95, 611–619. doi: 10.1111/j.1095-8312.2008.01081.x
Gristina, A. S., De Michele, R., Garfì, G., La Mantia, T., Fontana, I., Spinelli, P., et al. (2017). Urgent need for preservation of grapevine (Vitis vinifera L. subsp. vinifera) germplasm from small circum-Sicilian islands as revealed by SSR markers and traditional use investigations. Genet. Resour. Crop Ev. 64 (6), 1395–1415. doi: 10.1007/s10722-016-0444-6
Hopf, M. (1991). “South and Southwest Europe”, in Progress in old word palaeoethnobotany. Eds. Van Zeist, K., Wasylikowa, K. E., Behre, W. (Balkema: Rotterdam-Brookfield), 241–277.
Jakobsson, M., Rosenberg, N. A. (2007). CLUMPP: a cluster matching and permutation program for dealing with label switching and multimodality in analysis of population structure. Bioinformatics 23, 1801–1806. doi: 10.1093/bioinformatics/btm233
Jombart, T. (2008). adegenet: a R package for the multivariate analysis of genetic markers. Bioinformatics 24 (11), 1403–1405. doi: 10.1093/bioinformatics/btn129
Lacombe, T., Boursiquot, J. M., Laucou, V., Di Vecchi-Staraz, M., Peros, J. M., This, P. (2013). Large-scale parentage analysis in an extended set of grapevine cultivars (Vitis vinifera L.). Theor. Appl. Genet. 126, 401–414. doi: 10.1007/s00122-012-1988-2
Laucou, V., Launay, A., Bacilieri, R., Lacombe, T., Adam-Blondon, A. F., Bérard, A., et al. (2018). Extended diversity analysis of cultivated grapevine Vitis vinifera with 10K genome-wide SNPs. PloS One 13 (2), e0192540. doi: 10.1371/journal.pone.0192540
McGovern, P., Jalabadze, M., Batiuk, S., Callahan, M. P., Smith, K. E., Hall, G. R. (2017). Early Neolithic wine of Georgia in the South Caucasus. Proc. Natl. Acad. Sci. 114 (48), E10309–E10318. doi: 10.1073/PNAS.1714728114
McGovern, P. E. (2003). Ancient wine: the search for the origins of viniculture. Princeton: Princeton University Press.
Merdinoglu, D., Butterlin, G., Bevilacqua, L., Chiquet, V., Adam-Blondon., A.F., Decroocq, S. (2005). Development and characterization of a large set of micro satellite markers in grapevine (Vitis vinifera L.) suitable for multiplex PCR. Mol. Breed. 15, 349–366. doi: 10.1007/s11032-004-7651-0
Minà Palumbo, F. (1891). Viticoltura Sicula. Cenni storici. Atti e Rendiconti Acc. Zelanti. Acireale. vol. III, 71–82. Acireale, Italy: Vincenzo Micale.
Myles, S., Boyko, A. R., Owens, C. L., Brown, P. J., Grassi, F., Aradhyag, M. K., et al. (2011). Genetic structure and domestication history of the grape. Proc. Natl. Acad. Sci. 108, 3530–3535. doi: 10.1073/pnas.1009363108
Nei, M. (1978). Estimation of average heterozygosity and genetic distance from a small number of individuals. Genetics 89, 583–590.
OIV. (2017). Vitiviniculture Situation. OIV Statistical Report on World Vitiviniculture. Paris: International Organisation of Vine and Wine. www.oiv.int/.
Olmo, H. P. (1976). “Grapes,” in Evolution of crop plants. Eds. Simmounds, N. W. (London: Longman), 294–298.
Pacifico, D., Stigliano, E., Sposito, L., Spinelli, P., Garfì, G., Gristina., A. S., et al. (2016). Survey of viral infections in spontaneous grapevines from natural environments in Sicily. Eur. J. Plant Pathol. 145, 189–197. doi: 10.1007/s10658-015-0785-6
Peakall, R., Smouse, P. E. (2012). GenAlEx 6.5: genetic analysis in Excel. Population genetic software for teaching and research–an update. Bioinformatics 28, 2537–2539. doi: 10.1093/bioinformatics/bts460
Pritchard, J. K., Wen, W. (2003). Documentation for STRUCTURE Software: Version 2. Chicago: University of Chicago Press.
Pritchard, J. K., Stephens, M., Donnelly, P. (2000). Inference of population structure using multilocus genotype data. Genetics 155, 945–959.
Riaz, S., De Lorenzis, G., Velasco, D., Koehmstedt, A., Maghradze, D., Bobokashvili, Z., et al. (2018). Genetic diversity analysis of cultivated and wild grapevine (Vitis vinifera L.) accessions around the Mediterranean basin and Central Asia. BMC Plant Biol. 18, 137. doi: 10.1186/s12870-018-1351-0
Rosenberg, N. A. (2004). DISTRUCT: a program for the graphical display of population structure. Mol. Ecol. Notes 4, 137–138. doi: 10.1046/j.1471-8286.2003.00566.x
Salmaso, M., Faes, G., Segala, C., Stefanini, M., Salakhutdinov, L., Zyprian, E., et al. (2004). Genome diversity and gene haplotypes in the grapevine (Vitis vinifera L.), as revealed by single nucleotide polymorphisms. Mol. Breed. 14, 385–395. doi: 10.1007/s11032-004-0261-z
Sefc, K. M., Regner, F., Turetschek, E., Glössl, J., Steinkellner, H. (1999). Identification of microsatellite sequences in Vitis riparia and their applicability for genotyping of different Vitis species. Genome 42, 367–373.
Sestini, D. (1812). Dei vini in generale della Sicilia. Firenze, Manuscript 11c: Accademia dei Gorgofili.
Stoeckel, S., Grange, J., Fernández-Manjarres, J. F., Bilger, I., Frascaria-Lacoste, N., Mariette, S. (2006). Heterozygote excess in a self-incompatible and partially clonal forest tree species — Prunus avium L. Mol. Ecol. 5, 2109–2118. doi: 10.1111/j.1365-294X.2006.02926.x
Tanasi, D., Greco, E., Di Tullio, V., Capitani, D., Gullì, D., Ciliberto, E. (2017). 1H-1H NMR 2D-TOCSY, ATR FT-IR and SEM-EDX for the identification of organic residues on Sicilian prehistoric pottery. Microchem. J. 135, 140–147. doi: 10.1016/j.microc.2017.08.010
This, P., Jung, A., Boccacci, P., Borrego, J., Costantini, L., Crespan, M., et al. (2004). Development of a common set of standard varieties and standardized method of scoring microsatellites markers for the analysis of grapevine genetic resources. Theor. Appl. Genet. 109, 1448–1458. doi: 10.1007/s00122-004-1760-3
This, P., Lacombe, T., Thomas, M. R. (2006). Historical origins and genetic diversity of wine grapes. Trends Genet. 22, 511–519. doi: 10.1016/j.tig.2006.07.008
Thomas, M. R., Scott, N. S. (1993). Microsatellite repeats in grapevine reveal DNA polymorphisms when analyzed as sequence tagged sites (STSs). Theor. Appl. Genet. 86, 985–990. doi: 10.1007/bf00211051
Yu, G., Smith, D. K., Zhu, H., Guan, Y., Lam, T. T.-Y. (2017). ggtree: an r package for visualization and annotation of phylogenetic trees with their covariates and other associated data. Methods Ecol. Evol. 8, 28–36. doi: 10.1111/2041-210x.12628
Zecca, G., De Mattia, F., Loviou, G., Labra, M., Sala, F., Grassi, F. (2010). Molecular grapevine: Sylvestris, hybrids or cultivars that escaped from vineyards? Molecular evidence in Sardinia. Plant Biol. 12, 155–162 doi: 10.1111/j.1438-8677.2009.00226.x
Zhou, Y., Massonnet, M., Sanjak, J. S., Cantu, D., Gaut, B. S. (2017). Evolutionary genomics of grape (Vitis vinifera ssp. vinifera) domestication. Proc. Natl. Acad. Sci. 114 (44), 201709257. doi: org/10.1073/pnas.1709257114
Zohary, D. (1996). "Domestication of the Grapevine Vitis vinifera L. in the Near East," in The origins and ancient history of wine. Eds. McGovern, S., Fleming, S., Katz, P. (New York: Gordon and Breach), 23–30.
Keywords: grapevine, Vitis vinifera subsp. sativa, Vitis vinifera subsp. sylvestris, domestication, SSR
Citation: De Michele R, La Bella F, Gristina AS, Fontana I, Pacifico D, Garfi G, Motisi A, Crucitti D, Abbate L and Carimi F (2019) Phylogenetic Relationship Among Wild and Cultivated Grapevine in Sicily: A Hotspot in the Middle of the Mediterranean Basin. Front. Plant Sci. 10:1506. doi: 10.3389/fpls.2019.01506
Received: 29 June 2019; Accepted: 29 October 2019;
Published: 26 November 2019.
Edited by:
Rosa Arroyo-Garcia, Instituto Nacional de Investigación y Tecnología Agraria y Alimentaria (INIA), SpainReviewed by:
Roberto Bacilieri, Institut National de la Recherche Agronomique Centre Montpellier, FranceCopyright © 2019 De Michele, La Bella, Gristina, Fontana, Pacifico, Garfi, Motisi, Crucitti, Abbate and Carimi. This is an open-access article distributed under the terms of the Creative Commons Attribution License (CC BY). The use, distribution or reproduction in other forums is permitted, provided the original author(s) and the copyright owner(s) are credited and that the original publication in this journal is cited, in accordance with accepted academic practice. No use, distribution or reproduction is permitted which does not comply with these terms.
*Correspondence: Roberto De Michele, cm9iZXJ0by5kZW1pY2hlbGVAY25yLml0; Francesco Carimi, ZnJhbmNlc2NvLmNhcmltaUBpYmJyLmNuci5pdA==
†Present Address: Roberto De Michele, Institute of Bioscience and Bioresource (CNR), Palermo, Italy
Francesco Carimi, Institute of Bioscience and Bioresource (CNR), Palermo, Italy
‡These authors have contributed equally to this work
Disclaimer: All claims expressed in this article are solely those of the authors and do not necessarily represent those of their affiliated organizations, or those of the publisher, the editors and the reviewers. Any product that may be evaluated in this article or claim that may be made by its manufacturer is not guaranteed or endorsed by the publisher.
Research integrity at Frontiers
Learn more about the work of our research integrity team to safeguard the quality of each article we publish.