- 1Institute of Experimental Botany, Czech Academy of Sciences, Centre of the Region Hana for Biotechnological and Agricultural Research, Olomouc, Czechia
- 2Banana Breeding, International Institute of Tropical Agriculture, Kampala, Uganda
- 3Bioversity International, Banana Genetic Resources, Heverlee, Belgium
- 4Division of Crop Biotechnics, Laboratory of Tropical Crop Improvement, Katholieke Universiteit Leuven, Leuven, Belgium
- 5Banana Breeding, International Institute of Tropical Agriculture, Arusha, Tanzania
Oligo painting FISH was established to identify all chromosomes in banana (Musa spp.) and to anchor pseudomolecules of reference genome sequence of Musa acuminata spp. malaccensis “DH Pahang” to individual chromosomes in situ. A total of 19 chromosome/chromosome-arm specific oligo painting probes were developed and were shown to be suitable for molecular cytogenetic studies in genus Musa. For the first time, molecular karyotypes of diploid M. acuminata spp. malaccensis (A genome), M. balbisiana (B genome), and M. schizocarpa (S genome) from the Eumusa section of Musa, which contributed to the evolution of edible banana cultivars, were established. This was achieved after a combined use of oligo painting probes and a set of previously developed banana cytogenetic markers. The density of oligo painting probes was sufficient to study chromosomal rearrangements on mitotic as well as on meiotic pachytene chromosomes. This advance will enable comparative FISH mapping and identification of chromosomal translocations which accompanied genome evolution and speciation in the family Musaceae.
Introduction
Bananas (Musa spp.) are grown in tropical and subtropical regions of South East Asia, Africa and South America (Häkkinen, 2013; Janssens et al., 2016). They are one of the world’s major fruit crops, a staple and important export commodity for millions of people living mainly in developing countries. Despite the importance and breeding efforts (Ortiz and Swennen, 2014; Brown et al., 2017), little is known about banana genome structure, organization and evolution at chromosomal level across the whole Musaceae family.
The genus Musa comprises about 75 species and numerous cultivated edible clones. Based on a set of morphological descriptors (IPGRI-INIBAP/CIRAD, 1996) and basic chromosome number (x), the genus Musa has traditionally been divided into four sections: Eumusa (x = 11), Rhodochlamys (x = 11), Australimusa (x = 10), and Callimusa (x = 9, 10) (Cheesman, 1947). Argent (1976) created a separate section Ingentimusa which contains a single species Musa ingens with the lowest basic chromosome number (x = 7). However, genotyping using molecular markers revealed close relationship of M. ingens with other species of sections Callimusa and Australimusa (Li et al., 2010). Most of the modern edible banana clones originated within section Eumusa after intra- and inter-specific crosses between two wild diploid species M. acuminata (donor of A genome) and M. balbisiana (donor of B genome). In some cases, diploid M. schizocarpa (S genome) contributed to the evolution of edible clones, mainly after cross-breeding with diploid M. acuminata (Carreel et al., 1994; Čížková et al., 2013; Němečková et al., 2018). The spontaneous intra- and inter-specific crosses gave arise to seed sterile diploid (AA, AB) and triploid (AAA, AAB, or ABB) edible banana cultivars. Although tetraploid clones (AAAB, AABB) that originated spontaneously are known (Simmonds and Shepherd, 1955; Simmonds, 1956), currently cultivated tetraploid bananas were obtained in the breeding programs.
Species of genus Musa have a relatively small genome, ranging from 550 to 750 Mbp/1C (Doležel et al., 1994; Lysák et al., 1999; Asif et al., 2001; Kamaté et al., 2001; Bartoš et al., 2005; Čížková et al., 2015) and until now it was possible to identify only a few chromosomes in their karyotypes. The attempts were hampered by the relatively high number of chromosomes, their small size at mitotic metaphase (1–2 µm) and morphological similarity (Doleželová et al., 1998; Osuji et al., 1998; D’Hont et al., 2000). Chromosome banding, which was found informative in plant species with large and repeat-rich genomes, including wheat and rye (Gill and Kimber, 1977; Gill et al., 1991), did not result in diagnostic chromosome banding patterns in Musa, similar to many other plant species (Greilhuber, 1977; Schubert et al., 2001).
The application of fluorescence in situ hybridization (FISH), usually done with probes for DNA repeats with chromosome-specific distribution, provided a powerful approach to identify chromosomes in a range of plant species and study chromosome structural changes (e.g., Liu et al., 2011; Danilova et al., 2014; Amosova et al., 2017; Hou et al., 2018). Unfortunately, its use in Musa was hampered by the lack of suitable probes (Doleželová et al., 1998; Osuji et al., 1998; Valárik et al., 2002; Hřibová et al., 2007; Čížková et al., 2013). Until now, only NOR-bearing satellite chromosome, two chromosomes with clusters of tandem repeats CL18 and CL33, and two chromosomes bearing 5S rDNA loci can be cytogenetically identified in M. acuminata and M. balbisiana (Čížková et al., 2013). In M. schizocarpa, one chromosome pair bearing NOR and two chromosome pairs bearing tandem repeats CL18 and CL33 and other four chromosome pairs with 5S rDNA loci can be identified cytogenetically (Čížková et al., 2013). Even the mining of the reference genome sequence of M. acuminata “DH Pahang” (D’Hont et al., 2012) did not result in identification of sequences suitable as FISH probes useful for unambiguous identification of all Musa chromosomes and their anchoring to the genome sequence.
A method for chromosome painting, which allows fluorescent labeling of whole chromosomes, was developed in the late 1980s. This advance revolutionized human cytogenetics and found numerous applications in animal cytogenetics (e.g., Speicher et al., 1996; Cremer and Cremer, 2001; Ferguson-Smith and Trifonov, 2007). The original method was based on FISH with whole chromosome probes obtained from chromosomes isolated by flow cytometric sorting or microdissection. This was the reason why the method failed in plants where a majority of DNA repeats is distributed across the whole genome and only a minority of sequences are unique and chromosome-specific (Schubert et al., 2001). A solution was to use pools of chromosome-specific BAC (Bacterial Artificial Chromosome) clones (Lysák et al., 2001). However, the development of chromosome BAC pools requires whole genome sequence obtained after clone by clone (BAC by BAC) sequencing to identify single or low copy BAC clones useful for painting. Thus, the method is suitable for species with small genomes and containing low amounts of DNA repeats. Till now, painting using chromosome-specific BAC pools was used in dicotyledonous species with small nuclear genomes— Arabidopsis and its closely related species (e.g., Lysák et al., 2001; Mandáková and Lysák, 2008; Mandáková et al., 2013) as well as in monocot Brachypodium distachyon (Idziak et al., 2014). The attempts to use BAC FISH in banana were not successful due to the lack of a larger number of BAC clones containing single or low copy sequences (Hřibová et al., 2008).
The recent progress in the production of reference genome sequences and in technologies for DNA synthesis provided an alternative opportunity for affordable preparation of whole chromosome probes (chromosome paints) for FISH. The method called oligo painting FISH (Han et al., 2015) is based on in silico identification of large numbers of short (usually 45–50 bp) and unique (single copy) sequences in pseudomolecules of individual chromosomes, or their parts, synthesis of oligonucleotides, and their fluorescent labeling. A pool of synthesized and fluorescently labeled oligonucleotides is then used as a probe for FISH. Thus, the oligo painting FISH provides an opportunity to identify individual chromosomes and chromosome regions in Musa, perform comparative chromosome analysis and characterize chromosomal rearrangements (Qu et al., 2017; Braz et al., 2018; Xin et al., 2018; Jiang, 2019).
The present study fills the important gap in molecular cytogenetics of Musa. The application of oligo painting FISH described here allows anchoring genome sequence to chromosomes in situ and unambiguous identification of all Musa chromosomes after development of molecular karyotypes by a combined use of oligo painting probes and existing cytogenetic landmarks. Molecular karyotypes are described and compared for the three main genomes of Eumusa section—M. acuminata ssp. malaccensis, M. balbisiana, and M. schizocarpa, which contributed to the evolution of many edible banana clones.
Materials and Methods
Plant Material and Preparations of Chromosome Spreads
Representatives of three species from the section Eumusa were obtained as in vitro rooted plants from the International Musa Transit Centre (ITC, Bioversity International, Leuven, Belgium). In vitro plants were transferred to garden soil and maintained in a heated greenhouse. Table 1 lists the accessions used in this study. Male buds of M. acuminata “Pahang” and M. balbisiana “Tani” were obtained from the research station of the International Institute of Tropical Agriculture in Sendusu, Uganda.
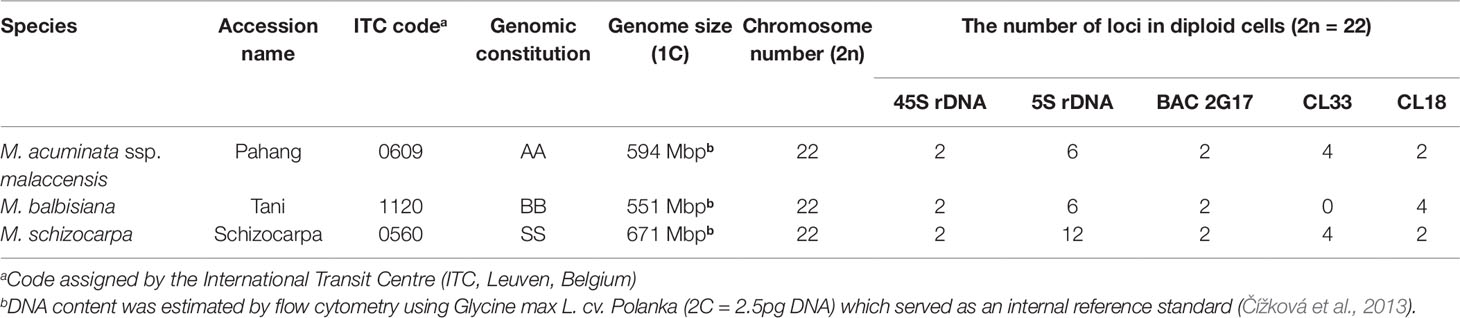
Table 1 List of analyzed accessions, their genomic constitution, genome size, and the number of loci identified on mitotic metaphase chromosomes (data from Čížková et al., 2013).
Actively growing root tips (∼1 cm long) were collected into 50-mM phosphate buffer (pH 7.0) containing 0.2% (v/v) β-mercaptoethanol, pre-treated in 0.05% (w/v) 8-hydroxyquinoline for three hours at room temperature, fixed in 3:1 ethanol:acetic acid fixative overnight, and stored in 70% ethanol. Preparation of protoplast suspensions was performed according to Doležel et al. (1998). Briefly, after digesting root tip segments in a mixture of 2% (w/v) cellulase and 2% (w/v) pectinase in 75-mM KCl and 7.5-mM EDTA (pH 4) for 90 min at 30°C, the suspension of resulting protoplasts was filtered through a 150-µm nylon mesh, pelleted, and washed in 70% ethanol. For further use, the protoplast suspension was stored in 70% ethanol at −20°C. Mitotic metaphase chromosome spreads were prepared by dropping method according to Doležel et al. (1998), the slides were postfixed in 4% (v/v) formaldehyde solution in 2x SSC solution and used for FISH.
Preparation of pachytene chromosome spreads was performed according to Mandáková and Lysák (2008), with minor modifications. Male flowers were fixed in 3:1 ethanol:acetic acid fixative overnight and stored in 70% ethanol at −20°C. Anthers were incubated in 0.3% (w/v) mix of cellulase, cytohelicase, and pectolyase (Sigma Aldrich, Darmstadt, Germany) for 30 min at 37°C. After the incubation in the enzyme mixture, the anthers were dissected in a drop of 60% (v/v) acetic acid on a microscopic slide and spread on the slide placed on a metal hot plate (50°C) after adding 60% (v/v) acetic acid for 25 s. The preparations were fixed in 3:1 ethanol:acetic acid fixative, air-dried, and used for FISH.
Identification of Specific Oligomers and Labeling of the Oligo Probes
Oligomers specific for individual chromosome arms were identified in the reference genome sequence of M. acuminata “DH Pahang” v.2 (Martin et al., 2016) using Chorus pipeline (Han et al., 2015). Sets of 20,000 oligomers (45-mers) per one library were synthesized by Arbor Biosciences (Ann Arbor, Michigan, USA). Labeled oligomer probes were prepared according to Han et al. (2015). Briefly, the oligomer libraries were amplified using emulsion PCR (Murgha et al., 2014), where F primer contained T7 RNA polymerase promoter. The emulsified PCR product was washed with water-saturated diethyl ether and ethyl acetate and purified with QIAquick PCR purification kit (Qiagen, Hilden, Germany). The product (480 ng DNA) was used for T7 in vitro transcription with MEGAshortscript T7 Kit (ThermoFisher Scientific/Invitrogen, Waltham, Massachusetts, USA) at 37°C for 4 h. The RNA product was purified using RNeasy Mini Kit (Qiagen) and 42 µg of RNA was reverse-transcribed with either digoxigenin-, biotin-, or CY5-labeled R primer (Eurofins Genomics, Ebersberg, Germany) using Superscript II Reverse Transcriptase and SUPERase-In RNase inhibitor (ThermoFisher Scientific/Invitrogen). The RNA : DNA hybrids were cleaned with Quick-RNA MiniPrep Kit (Zymo Research, Freiburg im Breisgau, Germany) and hydrolyzed with RNase H (New England Biolabs, Ipswich, Massachusetts, USA) and finally with RNase A (ThermoFisher Scientific/Invitrogen). The products were purified with Quick-RNA MiniPrep Kit (Zymo Research) and eluted with nuclease-free water to obtain single-stranded labeled oligomers, which were used as FISH probes.
Preparation of Other Cytogenetic Markers for FISH
Probes specific for ribosomal DNA sequences were prepared by labeling Radka1 (part of 26S rRNA gene) and Radka2 (contains 5S rRNA gene and non-transcribed spacer) DNA clones (Valárik et al., 2002) with biotin-16-dUTP (Roche Applied Science, Penzberg, Germany) or aminoallyl-dUTP-CY5 (Jena Biosciences, Jena, Germany) by PCR using T3 (forward) and T7 (reverse) primers (Invitrogen). Probes for tandem repeats CL18 and CL33 (Hřibová et al., 2010) were amplified using specific primers and labeled with aminoallyl-dUTP-CY5 or fluorescein-12-dUTP (Jena Biosciences, Jena, Germany) by PCR according to Čížková et al. (2013). Single copy BAC clone 2G17 (Hřibová et al., 2008) was labeled by digoxigenin-11-dUTP nick translation following manufacturer’s recommendation (Roche Applied Science, Penzberg, Germany).
Fluorescence In Situ Hybridization and Image Analysis
Hybridization mix (30 µl) containing 50% (v/v) formamide, 10% (w/v) dextran sulfate in 2x SSC and 10 ng/µl of labeled probe was added onto slide and denatured for 3 min at 80°C. Hybridization was carried out overnight at 37°C. The sites of hybridization of digoxigenin- and biotin-labeled probes were detected using anti-digoxigenin-FITC (Roche Applied Science) and streptavidin-Cy3 (ThermoFisher Scientific/Invitrogen), respectively. Chromosomes were counterstained with DAPI and mounted in VECTASHIELD Antifade Mounting Medium (Vector Laboratories, Burlingame, CA, USA). The slides were examined with Axio Imager Z.2 Zeiss microscope (Zeiss, Oberkochen, Germany) equipped with Cool Cube 1 camera (Metasystems, Altlussheim, Germany) and appropriate optical filters. The capture of fluorescence signals, merging the layers, and measurement of chromosome length were performed with ISIS software 5.4.7 (Metasystems), the final image adjustment and creation of idiograms were done in Adobe Photoshop CS5.
Results
Development of Chromosome Painting Probes and In Situ Hybridization
In order to produce chromosome arm-specific painting probes, unique k- mers were identified according to Han et al. (2015) in the reference genome sequence of the doubled haploid banana (M. acuminata “DH Pahang”; Martin et al., 2016) and analyzed with the Chorus program (https://github.com/forrestzhang/Chorus). While eight pseudomolecules corresponded to metacentric chromosomes, pseudomolecules 1, 2, and 10 appeared to be acrocentric with peri-centromeric region occupying an entire chromosome arm. The density of unique oligomers was lower in peri-centromeric regions in all pseudomolecules (Supplementary Figure S1) and these regions were excluded from the selection of oligomers for painting probes. The number of unique oligomers ranged from 79,896 to 127,835 for pseudomolecules 2 and 6, respectively. Sets of 20,000 45-mers specific to individual chromosome arms were then selected in Chorus, synthesized as so called immortal libraries and labeled directly by Cy5 or indirectly by biotin or digoxigenin as described in Materials and Methods. Oligomer libraries were designed to achieve a density of 0.9 to 2.1 oligomers per 1-kb chromosome sequence (Supplementary Table S1). To confirm that it is not possible to paint peri-centromeric regions with low oligomer densities and large gaps between low copy oligomers, a painting probe was prepared from peri-centromeric region of pseudomolecule 3. In total, 8,317 oligomers spanning this region (∼10.5 Mb long) ensured an average density of ∼0.8 oligomers/kb.
First, the painting probes were hybridized to mitotic metaphase chromosomes spreads of M. acuminata ssp. malaccensis (A genome)—the genotype from which the Musa reference genome sequence was developed. FISH with the painting probes resulted in visible signals covering chromosome arms along their lengths (Figures 1A–F). This observation confirmed that the probes had the expected parameters. Moreover, because the painting highlighted individual chromosome arms, it was possible to anchor pseudomolecules to individual chromosome arms. This work revealed that in the assembly, pseudomolecules 1, 6, 7 start with long arms and end with short arms, i.e., they are oriented inversely to the way karyotypes are presented, where the short arm of the chromosome is on top and the long arm on the bottom.
Following this, the painting probes were used for FISH in M. balbisiana (B genome) and M. schizocarpa (S genome) (Figures 1B, G, H). Comparison of chromosome and/or chromosome-arm painting in M. acuminata ssp. malaccensis and M. schizocarpa did not reveal any large chromosome translocations differentiating both species. On the other hand, a large translocation of the long arm of chromosome 3 to long (painted) arm of chromosome 1 was found in M. balbisiana (Figure 1B).
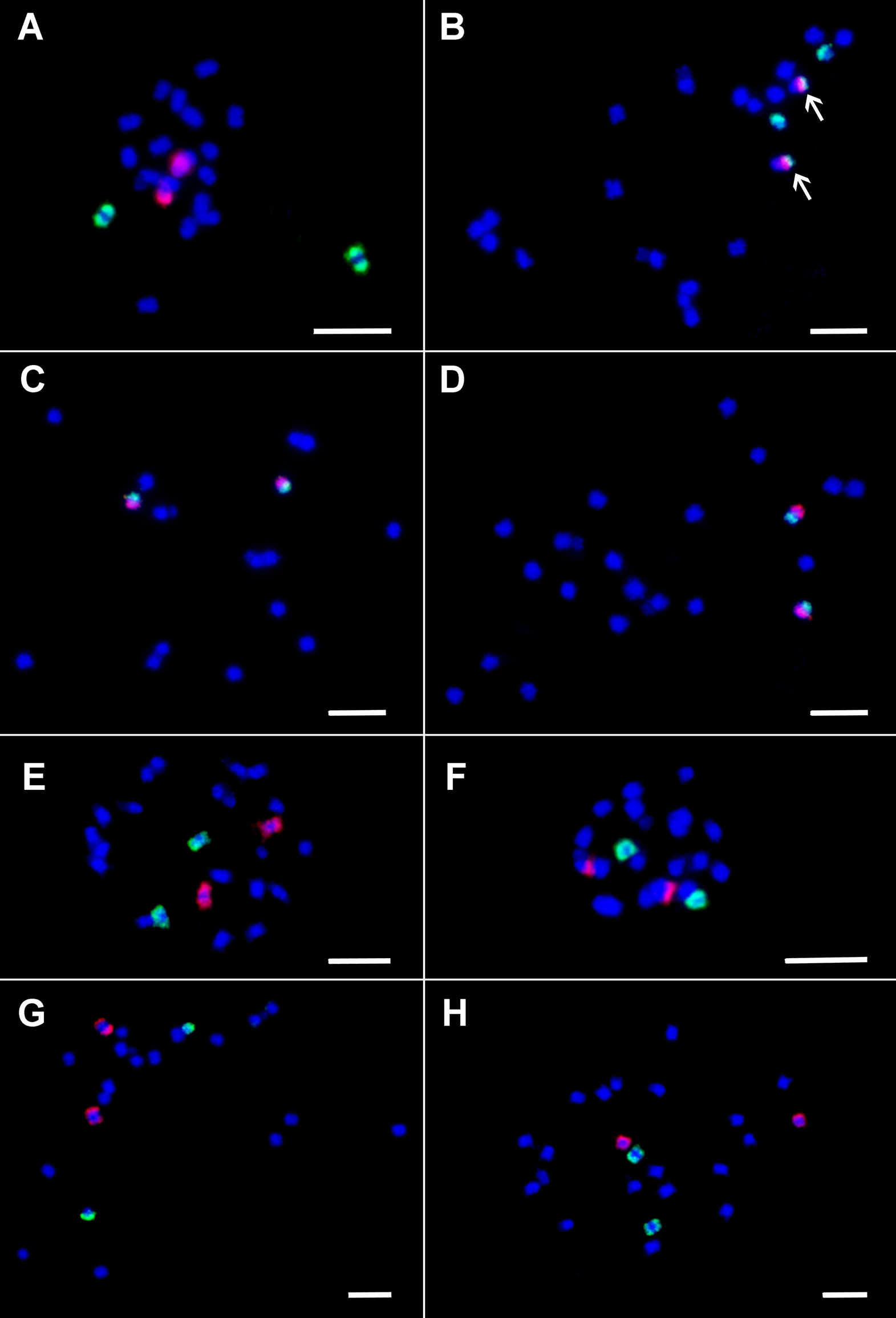
Figure 1 Oligo painting FISH on mitotic metaphase plates of three species of Musa. (A) M. acuminata ssp. malaccensis “Pahang” (2n = 22, AA; chromosome 1 in red, chromosome 3 in green). (B) M. balbisiana “Tani” (2n = 22, BB; chromosome 1 in red, chromosome 3 in green). (C) M. acuminata ssp. malaccensis “Pahang” (2n = 22, AA; short arm of chromosome 4 in green, its long arm in red). (D) M. acuminata ssp. malaccensis “Pahang” (2n = 22, AA; short arm of chromosome 5 in red, its long arm in green). (E) M. acuminata ssp. malaccensis “Pahang” (2n = 22, AA; chromosome 6 in red, chromosome 7 in green. (F) M. acuminata ssp. malaccensis “Pahang” (2n = 22, AA; chromosome 10 in red, chromosome 11 in green. (G) Musa schizocarpa “Schizocarpa” (2n = 2x = 22, SS; chromosome 8 in red, chromosome 2 in green). (H) Musa schizocarpa “Schizocarpa” (2n = 2x = 22, SS; chromosome 11 in red, chromosome 9 in green). Chromosomes were counterstained with DAPI (blue). Arrows point to the region of chromosome 3 translocated to chromosome 1 in M. balbisiana. Bars = 5 µm.
The small size of condensed mitotic metaphase chromosomes reduces the longitudinal resolution of chromosome painting and hence a chance to discover small structural rearrangements. An alternative is to perform chromosome painting with meiotic pachytene chromosomes (Figure 2) which are approximately fifty times longer. When hybridized to pachytene chromosome spreads of M. acuminata ssp. malaccensis, painting probes provided visible signals and the opportunity to analyze chromosome structure in more detail. This experiment showed that banana chromosomes do not contain large blocks of heterochromatin in distal and subtelomeric regions (Figure 2). Taking the advantage of higher spatial resolution, the set of oligo painting probes developed in this work will be suitable to visualize meiotic processes such as crossing over and synapsis. Following this, pachytene chromosome spreads of M. acuminata ssp. malaccensis were used to evaluate the signal of peri-centromeric painting probe designed for chromosome 3. FISH with the probe did not result in a continuous signal along the whole region. Instead, discontinuous signals, with signal-free gaps along most of the (peri-)centromeric region of chromosome 3 (Figure 2B), were observed. Based on this observation, painting probes were not designed for (peri-)centromeric regions of the remaining ten banana chromosomes.
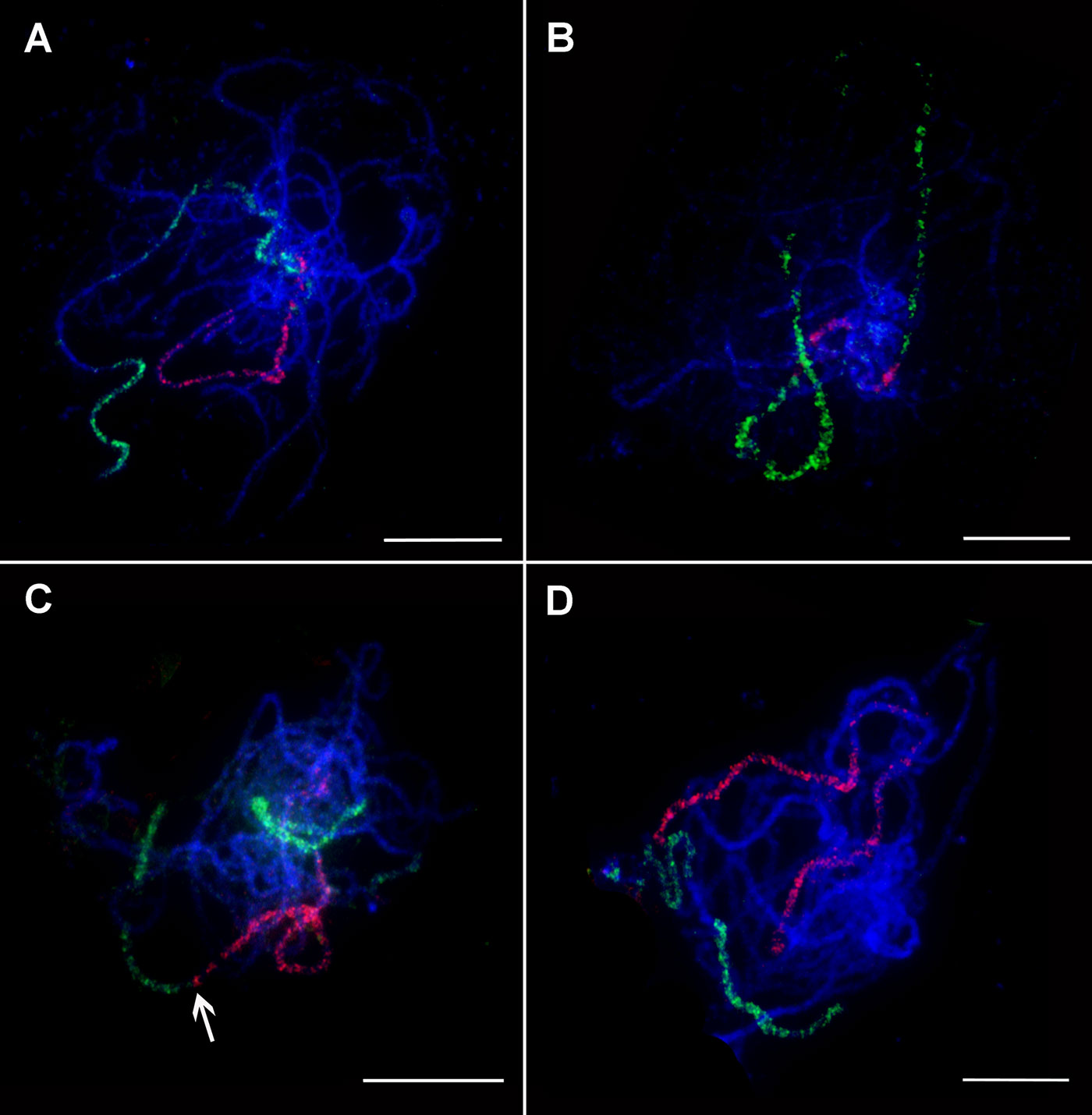
Figure 2 Oligo painting FISH on meiotic pachytene chromosome spreads of Musa. (A) M. acuminata ssp. malaccensis “Pahang” (2n = 22, AA; chromosome 1 in red, chromosome 4 in green). (B) M. acuminata ssp. malaccensis “Pahang” (2n = 22, AA; (peri-)centromeric region in red, chromosome 3 in green). (C) M. balbisiana “Tani” (2n = 22, BB; chromosome 1 in red, chromosome 3 in green). (D) M. balbisiana “Tani” (2n = 22, BB; chromosome 5 in red, chromosome 11 in green). Chromosomes were counterstained with DAPI (blue). Arrows point to the region translocated from chromosome 3 to chromosome 1 in M. balbisiana. Bars = 10 µm.
Integration of Cytogenetic Landmarks and Oligopaints
In order to utilize the existing probes for FISH in Musa and develop a highly informative toolbox to characterize Musa chromosome structure, the existing cytogenetic landmarks were integrated with the painting probes.
45S rRNA genes mapped to secondary constriction located on non-painted arm of chromosome 10 in all three Musa species. The probe for 5S rRNA genes localized to different chromosome regions and on different chromosomes in the three Musa species studied. In M. acuminata ssp. malaccensis, six signals of 5S rDNA were observed on mitotic metaphase plates and were localized in sub-telomeric region of chromosome 1 and long arm of chromosome 8, and in peri-centromeric region on short arm of chromosome 3. Six hybridization signals with 5S rDNA probe were observed also in mitotic metaphase plate of M. balbisiana. Two pairs of strong signals were localized in sub-telomeric region of chromosome 2 (non-painted arm) and in peri-centromeric region of the long arm of chromosome 3. Additional weak signal was observed in peri-centromeric region of the long arm of chromosome 6 (Figures 3 and 4). In M. schizocarpa, three pairs of strong signals and three pairs of weaker signals were observed after FISH with 5S rDNA probe on mitotic metaphase plate. Sub-telomeric region of chromosome 1 (non-painted arm) and peri-centromeric region of short arm of chromosome 3 and long arm of chromosome 4 contained strong signals of 5S rDNA. Additional weaker signals of 5S rDNA probe were observed in peri-centromeric regions of short arm of chromosome 8 and short arm of chromosome 11, as well as on the non-painted arm of chromosome 10 (Figures 3 and 4).
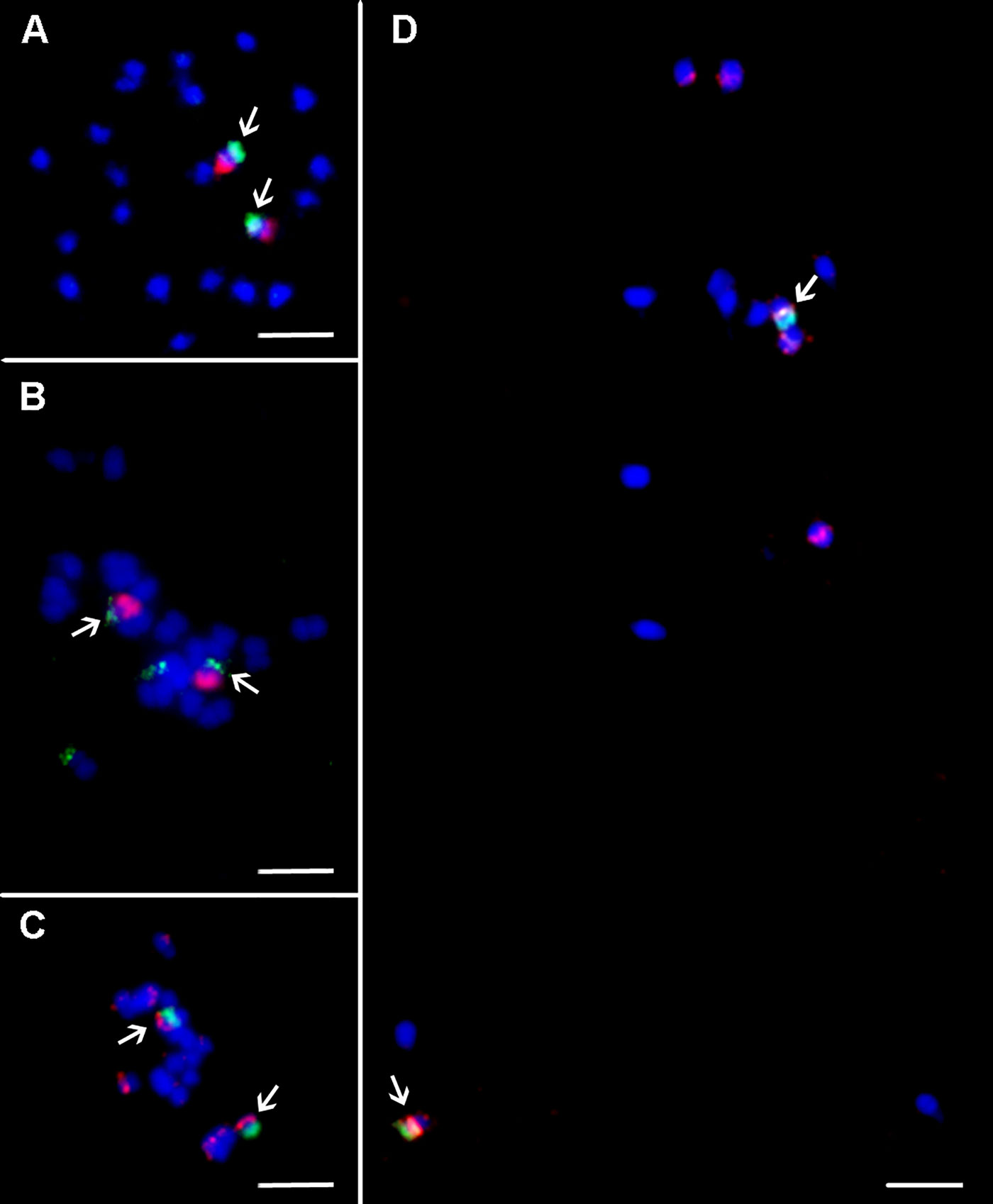
Figure 3 Integration of oligo painting FISH and existing cytogenetic markers on mitotic metaphase plates of Musa. (A) M. acuminata ssp. malaccensis “Pahang” (2n = 22, AA; chromosome 1 in red, BAC clone 2G17 in green). (B) M. schizocarpa “Schizocarpa” (2n = 22, SS; chromosome 2 in red, tandem repeat CL33 in green). (C) M. schizocarpa “Schizocarpa” (2n = 22, SS; short arm of chromosome 4 in green, 5S rRNA in red—two loci are localized on long arm of chromosome 4). (D) M. acuminata ssp. malaccensis “Pahang” (2n = 22, AA; 5S rRNA in red, short arm of chromosome 3 in green bears 5S rRNA). Chromosomes were counterstained with DAPI (blue). Bars = 5 µm. Arrows indicate colocalization of oligo painting FISH probes with existing cytogenetic markers.
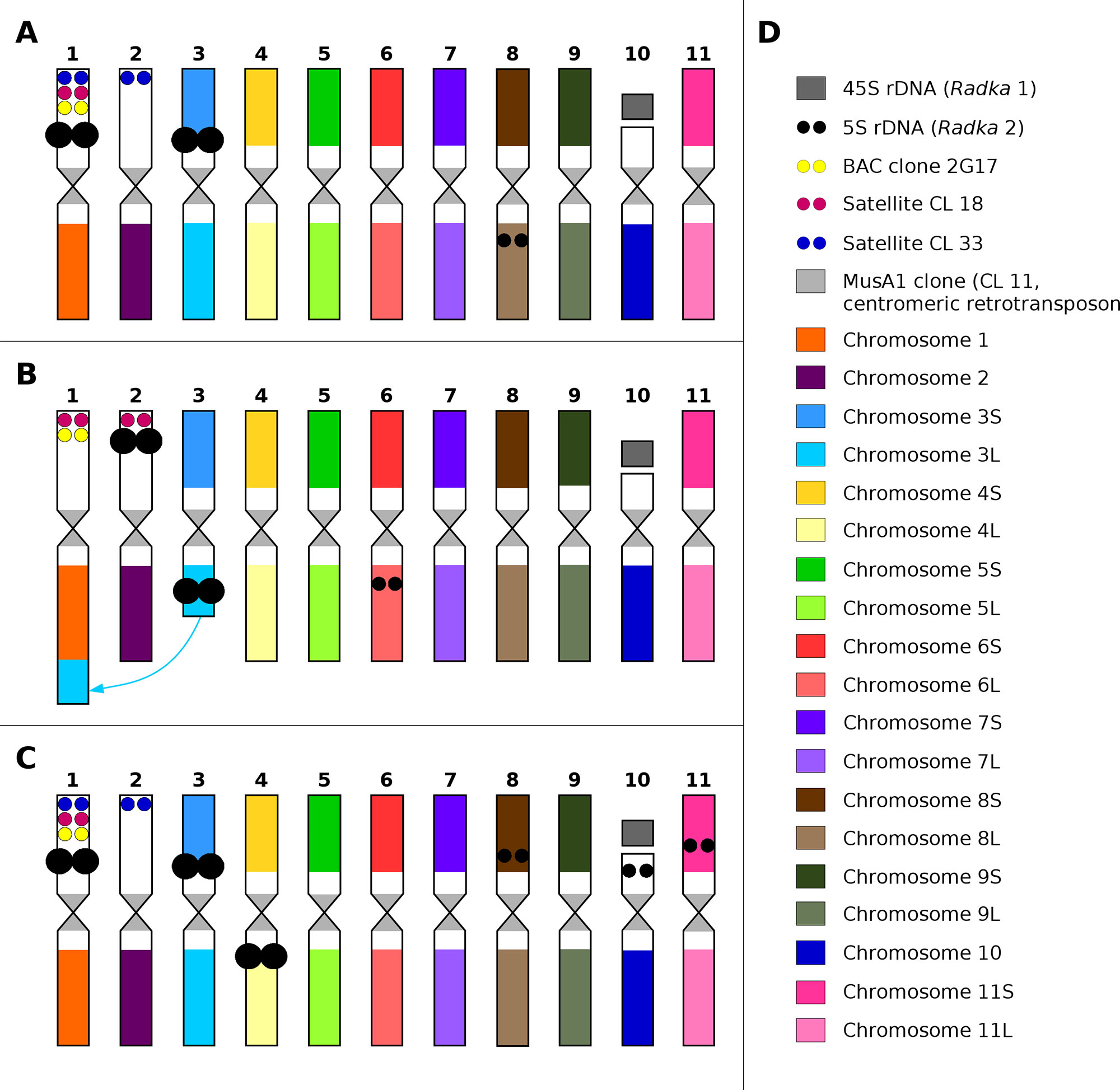
Figure 4 Idiograms of three diploid species of Eumusa section of Musa. (A) Musa acuminata ssp. malaccensis “Pahang” (genome A) ITC 0609. (B) Musa balbisiana “Tani” (genome B) ITC 1120. (C) Musa schizocarpa “Schizocarpa” (genome S) ITC 0560. (D) multicolored scheme.
Tandem organized repeats CL18, CL33, and BAC clone 2G17 were localized on non-painted arm of chromosome 1 in all three Musa species, except satellite CL33, which was not detected on any chromosome in M. balbisiana. In contrast, additional signal of tandem repeat CL33 located on non-painted arm of chromosome 2 was observed in M. acuminata ssp. malaccensis and in M. schizocarpa (Figures 3 and 4). Finally, additional signal of tandem repeat CL18 was observed in M. balbisiana on the non-painted arm of chromosome 2.
Discussion
Until recently, chromosome painting could be used only in plants whose genomes were sequenced clone by clone (The Arabidopsis Genome Initiative, 2000; The International Brachypodium Initiative, 2010) and chromosome painting was achieved by FISH with pools of single copy BAC clones that covered entire chromosomes. Importantly, chromosome paints developed in one species could be used in related species, providing a powerful approach for comparative karyotype analysis and for tracing karyotype changes during the evolution and speciation (e.g., Lysák et al., 2001; Idziak et al., 2014). Unfortunately, this painting method cannot be used in species with large genomes due to the prevalence of repetitive DNA and in species not closely related to those for which painting using BAC pools was developed.
The progress in DNA sequencing technology and assembly algorithms resulted in a shift from the clone by clone sequencing to shotgun sequencing and a majority of plant genomes has been sequenced in this way (Hamilton and Buell, 2012; Zimin et al., 2017; Belser et al., 2018). The availability of reference genome sequences and the affordable cost of synthesizing short oligonucleotides offered a direct way to develop chromosome paints (Han et al., 2015). Here, thousands of short single copy sequences are identified, bulk synthesized, fluorescently labeled and used as probes for FISH (Han et al., 2015). Pools of labeled oligonucleotide probes were found suitable for FISH on somatic metaphase chromosomes, meiotic pachytene chromosomes and interphase nuclei (Han et al., 2015; Filiault et al., 2018; Xin et al., 2018; Albert et al., 2019; Jiang, 2019). Successful applications of oligo painting FISH include construction of molecular cytogenetic karyotypes (Braz et al., 2018; Qu et al., 2017; Meng et al., 2018), identification of large chromosomal rearrangements, analysis of chromosome pairing in meiosis (Han et al., 2015; He et al., 2018; Albert et al., 2019), as well as the visualization of the arrangement of chromosomes in 3D space of interphase nuclei (Albert et al., 2019).
Despite the availability of a reference genome sequence of M. acuminata ssp. malaccensis (D’Hont et al., 2012; Martin et al., 2016) and resequencing of more than 120 accessions of Musa (Dupouy et al., 2019), DNA pseudomolecules have not been anchored to individual chromosomes and molecular karyotype of Musa has not been developed to date. In many plant species, tandem organized repeats serve as useful probes for FISH to identify individual chromosomes and their regions (Hřibová et al., 2007; Badaeva et al., 2015; Koo et al., 2016; Křivánková et al., 2017; Said et al., 2018). The nuclear genome of Musa species is relatively small (1C ∼ 500–750 Mb; Doležel et al., 1994; Lysák et al., 1999; Asif et al., 2001; Kamaté et al., 2001; Bartoš et al., 2005; Čížková et al., 2015) and until now, only a few tandem organized repeats and rDNA sequences were successfully used as cytogenetic landmarks (Balint-Kurti et al., 2000; Valárik et al., 2002; Hřibová et al., 2007; Hřibová et al., 2010; Čížková et al., 2013; Novák et al., 2014). Moreover, only one BAC clone has been used as a cytogenetic marker in Musa (Hřibová et al., 2008) and only four BAC clones were localized on pachytene chromosomes (De Capdeville et al., 2009). Thus, the attempts to use of BAC clones for anchoring pseudomolecules to chromosomes in banana as has been done in other species (Jiang et al., 1995; Lapitan et al., 1997; Kim et al., 2002; Idziak et al., 2014), were not successful.
Unlike the previous approaches, chromosome painting using pools of single copy oligomers offers the opportunity to establish a molecular karyotype of Musa, making it possible to identify individual chromosomes, follow their behavior during somatic cell cycle and meiosis, perform comparative karyotype analysis, and identify structural chromosome changes. FISH with oligo painting probes developed in this work resulted in visible hybridization signals along chromosomal arms on condensed mitotic metaphase chromosomes (Figure 1) as well as on less condensed pachytene chromosomes (Figure 2) confirming their usefulness as painting probes in Musa. Only small regions on pachytene chromosomes were free of painting signals. This could be either due to the presence of heterochromatin blocks, or due to gaps in the genome sequence (Figure 2). In contrast to chromosome arms, peri-centromeric regions were not labeled. These regions contain large gaps in the genome sequence and large proportion of repetitive DNA sequences in peri-centromeric regions (Hřibová et al., 2010; Neumann et al., 2011; D’Hont et al., 2012; Martin et al., 2016).
We demonstrate that chromosome/chromosome-arm specific oligo painting libraries designed for M. acuminata ssp. malaccensis can be used for cytogenetic analysis of related species M. balbisiana and M. schizocarpa, which played an important role in the evolution of many edible banana clones (Carreel et al., 1994; D’Hont et al., 2012; Davey et al., 2013; Čížková et al., 2013). This observation provided an opportunity for comparative karyotype analysis and identification of putative chromosome translocations. In our study, we observed translocation of long arm of chromosome 3 to long arm of chromosome 1 in M. balbisiana (B genome) (Figures 1B and 2C). This observation confirms the result of Baurens et al. (2019), which were obtained after anchoring a dense genetic map of M. balbisiana “Pisang Klutuk Wulung” to M. acuminata ssp. malaccensis reference genome sequence (Martin et al., 2016). The authors estimated the size of the translocated region of long arm of chromosome 3 to be ∼8 Mb, confirming the sensitivity of oligo chromosome painting.
Co-localization of chromosome painting probes with cytogenetic markers developed earlier for Musa (Valárik et al., 2002; Hřibová et al., 2010; Čížková et al., 2013) offered an opportunity to create molecular karyotypes suitable for comparative analysis. The presence of 5S rRNA genes on non-collinear chromosomes in the A, B, and S genomes of Musa as described here indicates small chromosomal rearrangements which occurred during Musa speciation. On the other hand, the location of tandem organized repeats CL18, CL33, and BAC clone 2G17 on collinear chromosome arms in all three species indicates their structural homology of the chromosome arms. These observations imply that chromosomes containing a particular DNA sequence, e.g., 5S rDNA, cannot be considered as collinear. This shows a potential weakness of comparative karyotype analysis of using only a few cytogenetic markers (Fukui et al., 1994; Murata et al., 1997).
Tandem organized repeats CL18 and CL33 (Hřibová et al., 2010) were located together with 5S rRNA genes on short arms of chromosomes 1 and 2, which lacked oligopainting signals. Genome sequence of M. acuminata ssp. malaccensis includes three pseudomolecules which are represented by two large regions differing in DNA repeat composition and in density of unique oligomers (Supplementary Figure 1, Martin et al., 2016). The constitution of banana pseudomolecules 1, 2, and 10 indicates that they cover only one chromosome arm and a peri-centromeric region. Painting probes created for the three pseudomolecules localized to only one chromosomal arm. One of the pseudomolecules is collinear with acrocentric chromosome 10 and bears 45S rRNA locus on its short arm. The two remaining pseudomolecules represent chromosomes 1 and 2, which seem to be meta or sub-metacentric thus could miss a large sequence region. These observations indicate that these genomic regions were not completely assembled and are missing due to the presence of a large number of various tandem organized sequences.
The improved version of M. acuminata “DH Pahang” reference genome sequence represents 450.7 Mbp which corresponds to ∼81% of its nuclear genome size estimated by flow cytometry (Čížková et al., 2013). In addition, the reference genome sequence contains a total of 56.6-Mbp sequences, which were not anchored to the 11 pseudomolecules. The most plausible explanation why these sequences were not included in pseudomolecules is that they represent heterochromatin regions, which are difficult to sequence. However, relatively high number of unique oligomers in unanchored scaffolds as observed in this work (Supplementary Figure 1) indicates that the unanchored part of the reference genome sequence contains low copy sequences from euchromatic regions. Thus, these regions were probably not anchored due to the absence of DNA markers, or they were too short to be anchored using Bionano optical mapping. The use of long-read sequencing technologies such as Oxford Nanopore in combination with optical mapping (Belser et al., 2018) should further improve the current assembly and shed light on the difficult parts of M. acuminata ssp. malaccensis genome.
Conclusions
In this work, chromosome painting probes were developed for banana (Musa spp.) and used to establish molecular karyotypes for three species of Musa that were the parents of a majority of cultivated edible banana clones. This advance made it possible to anchor reference genome sequence of banana, Musa acuminata spp. malaccensis to individual chromosomes. The study also demonstrates the potential of oligo painting FISH for comparative karyotype analysis and identification of structural chromosome changes that accompanied the evolution and speciation in the genus Musa.
Data Availability Statement
All datasets generated for this study are included in the article/Supplementary Material.
Author Contributions
EH and JD conceived the experiments. DŠ, AN, and MK conducted the study and processed the data. BU and RS provided the banana materials. DŠ and EH wrote the manuscript. EH, JD, and DŠ discussed the results and contributed to manuscript writing. All authors have read and approved the final manuscript.
Conflict of Interest
The authors declare that the research was conducted in the absence of any commercial or financial relationships that could be construed as a potential conflict of interest.
Acknowledgments
We thank Dr. Ines van den Houwe for providing the plant material and Ms. Radka Tušková for excellent technical assistance. This work was supported by the Czech Science Foundation (award No. 19-20303S). The computing was supported by the National Grid Infrastructure MetaCentrum (grant No. LM2010005 under the program Projects of Large Infrastructure for Research, Development, and Innovations). The authors thank all donors who supported this work through their contributions to the CGIAR Fund (http://www.cgiar.org/who-we-are/cgiar-fund/fund-donors-2/), and in particular to the CGIAR Research Program Roots, Tubers and Bananas (RTB-CRP).
Supplementary Material
The Supplementary Material for this article can be found online at: https://www.frontiersin.org/articles/10.3389/fpls.2019.01503/full#supplementary-material
Supplementary Figure S1 | Oligomer coverage of 11 pseudomolecules (labeled A– K) and concatenated unanchored scaffolds (L) in the reference genome of M. acuminata‘DH Pahang’ (Martin et al., 2016). The oligomers (45 bp) were designed using the Chorus program (Han et al., 2015) and are depicted in black. Position and coverage of tandem repeats CL18 (pink) and CL33 (green) are also shown.
References
Albert, P. S., Zhang, T., Semrau, K., Rouillard, J. M., Kao, Y. H., Wang, C. R. (2019). Whole-chromosome paints in maize reveal rearrangements, nuclear domains, and chromosomal relationships. Proc. Natl. Acad. Sci. U. S. A. 116 (5), 1679–1685. doi: 10.1073/pnas.1813957116
Amosova, A. V., Bolsheva, N. L., Zoshchuk, S. A., Twardovska, M. O., Yurkevich, O. Y., Andreev, I. O., et al. (2017). (A) Comparative molecular cytogenetic characterization of seven Deschampsia (Poaceae) species. PloS One 12 (4), e0175760. doi: 10.1371/journal.pone.0175760
Argent, G. C. G. (1976). The wild bananas of Papua New Guinea. Notes R. Bot. Gard. Edinburgh. 35 (1), 77–114.
Asif, M. J., Mak, C., Othman, R. Y. (2001). Characterization of indigenous Musa species based on flow cytometric analysis of ploidy and nuclear DNA content. Caryologia. 54 (2), 161–168. doi: 10.1080/00087114.2001.10589223
Badaeva, E. D., Amosova, A. V., Goncharov, N. P., Macas, J., Ruban, A. S., Grechishnikova, I. V., et al. (2015). A set of cytogenetic markers allows the precise identification of all A-genome chromosomes in diploid and polyploid wheat. Cytogenet. Genome Res. 146 (1), 71–79. doi: 10.1159/000433458
Balint-Kurti, P., Clendennen, S., Doleželová, M., Valárik, M., Doležel, J., Beetham, P. R., et al. (2000). Identification and chromosomal localization of the monkey retrotransposon in Musa sp. Mol. Gen. Genet. 263 (6), 908–915. doi: 10.1007/s004380000265
Bartoš, J., Alkhimova, O., Doleželová, M., De Langhe, E., Doležel, J. (2005). Nuclear genome size and genomic distribution of ribosomal DNA in Musa and Ensete (Musaceae): taxonomic implications. Cytogenet. Genome Res. 109, 50–57. doi: 10.1159/000082381
Baurens, F. C., Martin, G., Hervouet, C., Salmon, F., Yohomé, D., Ricci, S. (2019). Recombination and large structural variations shape interspecific edible bananas genomes. Mol. Biol. Evol. 36 (1), 97–111. doi: 10.1093/molbev/msy199
Belser, C., Istace, B., Denis, E., Dubarry, M., Baurens, F. C., Falentin, C. (2018). Chromosome-scale assemblies of plant genomes using nanopore long reads and optical maps. Nat. Plants. 4 (11), 879–887. doi: 10.1038/s41477-018-0289-4
Braz, G. T., He, L., Zhao, H., Zhang, T., Semrau, K., Rouillard, J. M. (2018). Comparative oligo-FISH mapping: An efficient and powerful methodology to reveal karyotypic and chromosomal evolution. Genetics. 208 (2), 513–523. doi: 10.1534/genetics.117.300344
Brown, A., Tumuhimbise, R., Amah, D., Uwimana, B., Nyine, M., Mduma, H. (2017). The genetic improvement of bananas and plantains (Musa spp.) In Genetic Improvement of Tropical Crops Vol. pp. Campos, HCaligari, PDS. Cham: Springer, 219–240.
Carreel, F., Fauré, S., González de León, D., Lagoda, P. J. L., Perrier, X., Bakry, F. (1994). Evaluation of the genetic diversity in diploid bananas (Musa sp.). Genet. Sel. Evol. 26 (Suppl 1), 125–136. doi: 10.1051/gse:19940709
Cheesman, E. E. (1947). Classification of the bananas. The genus Ensete Horan and the genus Musa L. Kew. Bull. 2 (2), 97–117. doi: 10.2307/4109206
Čížková, J., Hřibová, E., Humplíková, L., Christelová, P., Suchánková, P., Doležel, J. (2013). Molecular analysis and genomic organization of major DNA satellites in banana (Musa spp.). PloS One 8, e54808. doi: 10.1371/journal.pone.0054808
Čížková, J., Hřibová, E., Christelová, P., Van den Houwe, I., Häkkinen, M., Roux, N., et al. (2015). Molecular and cytogenetic characterization of wild Musa species. PLoS One 10:e0134096. doi: 10.1371/journal.pone.0134096
Cremer, T., Cremer, S. (2001). (B) Chromosome territories, nuclear architecture and gene regulation in mammalian cells.. Nat. Rev. Genet. 2 (4), 292–301. doi: 10.1038/35066075
D’Hont, A., Paget-Goy, A., Escoute, J., Carreel, F. (2000). The interspecific genome structure of cultivated banana, Musa spp. revealed by genomic DNA in situ hybridization. Theor. Appl. Genet. 100, 177–183. doi: 10.1007/s001220050024
D’Hont, A., Denoeud, F., Aury, J. M., Baurens, F. C., Carreel, F., Garsmeur, O., et al. (2012). The banana (Musa acuminata) genome and the evolution of monocotyledonous plants. Nature 488, 213–217. doi: 10.1038/nature11241
Danilova, T. V., Friebe, B., Gill, B. S. (2014). Development of wheat single gene FISH map for analyzing homoeologous relationship and chromosomal rearrangements within the triticeae. Theor. Appl. Genet. 127 (3), 715–730. doi: 10.1007/s00122-013-2253-z
Davey, M. W., Gudimella, R., Harikrishna, J. A., Sin, L. W., Khalid, N., Keulemans, J. (2013). A draft Musa balbisiana genome sequence for molecular genetics in polyploid, inter- and intra-specific Musa hybrids. BMC Genomics 14, 683. doi: 10.1186/1471-2164-14-683
De Capdeville, G., Souza Junior, M. T., Szinay, D., Diniz, L. E. C., Wijnker, E., Swennen, R., et al. (2009). The potential of high-resolution BAC-FISH in banana breeding. Euphytica 166, 431–443. doi: 10.1007/s10681-008-9830-2
Doležel, J., Doleželová, M., Novák, F. J. (1994). Flow cytometric estimation of nuclear DNA amount in diploid bananas (Musa acuminata and Musa balbisiana). Biol. Plant 36, 351–357. doi: 10.1007/BF02920930
Doležel, J., Doleželová, M., Roux, N., Van den houwe, I. (1998). A novel method to prepare slides for high resolution chromosome studies in Musa spp. Infomusa 7, 3–4.
Doleželová, M., Valárik, M., Swennen, R., Horry, J. P., Doležel, J. (1998). Physical mapping of the 18S-25S and 5S ribosomal RNA genes in diploid bananas. Biol. Plant 41, 497–505. doi: 10.1023/A:1001880030275
Dupouy, M., Baurens, F. C., Derouault, P., Hervouet, C., Cardi, C., Cruaud, C., et al. (2019). Two large reciprocal translocations characterized in the disease resistance-rich burmannica genetic group of Musa acuminata. Ann. Bot. XX, 1–11. doi: 10.1093/aob/mcz078
Ferguson-Smith, M. A., Trifonov, V. (2007). Mammalian karyotype evolution. Nat. Rev. Genet. 8 (12), 950–962. doi: 10.1038/nrg2199
Filiault, D. L., Ballerini, E. S., Mandáková, T., Aköz, G., Derieg, N. J., Schmutz, J., et al. (2018). The Aquilegia genome provides insight into adaptive radiation and reveals an extraordinarily polymorphic chromosome with a unique history. eLife 7, e36426. doi: 10.7554/eLife.36426
Fukui, K., Kamisugi, Y., Sakai, F. (1994). Physical mapping of 5S rDNA loci by direct-cloned biotinylated probes in barley chromosomes. Genome. 37 (1), 105–111. doi: 10.1139/g94-013
Gill, B. S., Kimber, G. (1977). Recognition of translocations and alien chromosome transfers in wheat by the Giemsa C-banding technique. Crop Sci. 17, 264–266. doi: 10.2135/cropsci1977.0011183X001700020008x
Gill, B. S., Friebe, B., Endo, T. R. (1991). Standard karyotype and nomenclature system for description of chromosome bands and structural aberrations in wheat (Triticum aestivum). Genome 34, 830–839. doi: 10.1139/g91-128
Greilhuber, J. (1977). Why plant chromosomes do not show G-bands. Theor. Appl. Genet. 50 (3), 121–124. doi: 10.1007/BF00276805
Häkkinen, M. (2013). Reappraisal of sectional taxonomy in Musa (Musaceae). Taxon. 62 (4), 809–813. doi: 10.12705/624.3
Hamilton, J. P., Buell, C. R. (2012). Advances in plant genome sequencing. Plant J. 70 (1), 177–190. doi: 10.1111/j.1365-313X.2012.04894.x
Han, Y., Zhang, T., Thammapichai, P., Weng, Y., Jiang, J. (2015). Chromosome-specific painting in Cucumis species using bulked oligonucleotides. Genetics. 200, 771–779. doi: 10.1534/genetics.115.177642
He, L., Braz, G. T., Torres, G. A., Jiang, J. M. (2018). Chromosome painting in meiosis reveals pairing of specific chromosomes in polyploid Solanum species. Chromosoma 127, 505–513. doi: 10.1007/s00412-018-0682-9
Hou, L., Xu, M., Zhang, T., Xu, Z., Wang, W., Zhang, J., et al. (2018). BMC Plant Biol. 18 (1), 110. doi: 10.1186/s12870-018-1325-2
Hřibová, E., Doleželová, M., Town, C. D., Macas, J., Doležel, J. (2007). Isolation and characterization of the highly repeated fraction of the banana genome. Cytogenet. Genome Res. 119 (3-4), 268–274. doi: 10.1159/000112073
Hřibová, E., Doleželová, M., Doležel, J. (2008). Localization of BAC clones on mitotic chromosomes of Musa acuminata using fluorescence in situ hybridization. Biol. Plant 52, 445–452. doi: 10.1007/s10535-008-0089-1
Hřibová, E., Neumann, P., Matsumoto, T., Roux, N., Macas, J., Doležel, J. (2010). Repetitive part of the banana (Musa acuminata) genome investigated by low-depth 454 sequencing. BMC Plant Biol. 10, 204. doi: 10.1186/1471-2229-10-204
Idziak, D., Hazuka, I., Poliwczak, B., Wiszynska, A., Wolny, E., Hasterok, R. (2014). Insight into the karyotype evolution of Brachypodium species using comparative chromosome barcoding. PloS One 9 (3), e93503. doi: 10.1371/journal.pone.0093503
International Plant Genetic Resources Institute-International Network for the Improvement of Banana and Plantain/Centre de Coopération internationale en recherche agronomique pour le développement [IPGRI-INIBAP/CIRAD]International Plant Genetic Resources Institute-International Network for the Improvement of Banana and Plantain/Centre de Coopération internationale en recherche agronomique pour le développement [IPGRI-INIBAP/CIRAD] (1996). Description for Banana (Musa spp.). Int. Network for the Improvement of Banana and Plantain, Montpellier, France; Centre de coopération int. en recherche agronomique pour le développement, Montpellier, France; International Plant Genetic Resources Institute Press, Rome.
Janssens, S. B., Vandelook, F., De Langhe, E., Verstraete, B., Smets, E., Van den Houwe, I., et al. (2016). Evolutionary dynamics and biogeography of Musaceae reveal a correlation between the diversification of the banana family and the geological and climatic history of Southeast Asia. New Phytol. 210 (4), 1453–1465. doi: 10.1111/nph.13856
Jiang, J., Gill, B. S., Wang, G. L., Ronald, P. C., Ward, D. C. (1995). Metaphase and interphase fluorescence in situ hybridization mapping of the rice genome with bacterial artificial chromosomes. Proc. Natl. Acad. Sci. U. S. A. 92 (10), 4487–4491. doi: 10.1073/pnas.92.10.4487
Jiang, J. (2019). Fluorescence in situ hybridization in plants: recent developments and future applications. Chromosom. Res. 27 (3), 153–165. doi: 10.1007/s10577-019-09607-z
Křivánková, A., Kopecký, D., Stočes, Š., Doležel, J., Hřibová, E. (2017). Repetitive DNA: a versatile tool for karyotyping in Festuca pratensis Huds. Cytogenet. Genome Res. 151 (2), 96–105. doi: 10.1159/000462915
Kamaté, K., Brown, S., Durand, P., Bureau, J. M., De Nay, D., Trinh, T. H. (2001). Nuclear DNA content and base composition in 28 taxa of Musa. Genome. 44, 622–627. doi: 10.1139/g01-058
Kim, J. S., Childs, K. L., Islam-Faridi, M. N., Menz, M. A., Klein, R. R., Klein, P. E. (2002). Integrated karyotyping of sorghum by in situ hybridization of landed BACs. Genome. 45, 402–412. doi: 10.1139/g01-141
Koo, D. H., Zhao, H., Jiang, J. (2016). Chromatin-associated transcripts of tandemly repetitive DNA sequences revealed by RNA-FISH. Chromosome Res. 24 (4), 467–480. doi: 10.1007/s10577-016-9537-5
Lapitan, N. L. V., Brown, S. E., Kennard, W., Stephens, J. L., Knudson, D. L. (1997). FISH physical mapping with barley BAC clones. Plant J. 11, 149–156. doi: 10.1046/j.1365313X.1997.11010149.x
Li, L. F., Häkkinen, M., Yuan, Y. M., Hao, G., Ge, X. J. (2010). Molecular phylogeny and systematics of the banana family (Musaceae) inferred from multiple nuclear and chloroplast DNA fragments, with a special reference to the genus Musa. Mol. Phylogenet. Evol. 57 (1), 1–10. doi: 10.1016/j.ympev.2010.06.021
Liu, W., Rouse, M., Friebe, B., Jin, Y., Gill, B., Pumphrey, M. O. (2011). Discovery and molecular mapping of a new gene conferring resistance to stem rust, Sr53, derived from Aegilops geniculata and characterization of spontaneous translocation stocks with reduced alien chromatin. Chromosome Res. 19 (5), 669–682. doi: 10.1007/s10577-011-9226-3
Lysák, M. A., Doleželová, M., Horry, J. P., Swennen, R., Doležel, J. (1999). Flow cytometric analysis of nuclear DNA content in Musa. Theor. Appl. Genet. 98 (8), 1344–1350. doi: 10.1007/s001220051201
Lysák, M. A., Fransz, P. F., Ali, H. B. M., Schubert, I. (2001). Chromosome painting in A. thaliana. Plant J. 28, 689–697. doi: 10.1046/j.1365-313x.2001.01194.x
Mandáková, T., Lysák, M. A. (2008). Chromosomal phylogeny and karyotype evolution in x = 7 crucifer species (Brassicaceae). Plant Cell 20, 2559–2570. doi: 10.1105/tpc.108.062166
Mandáková, T., Marhold, K., Lysák, M. A. (2013). The widespread crucifer species Cardamine flexulosa is an allotetraploid with a conserved subgenomic structure. New Phytol. 201, 982–992. doi: 10.1111/nph.12567
Martin, G., Baurens, F. C., Droc, G., Rouard, M., Cenci, A., Kilian, A., et al. (2016). Improvement of the banana “Musa acuminata“ reference sequence using NGS data and semi-automated bioinformatics methods. BMC Genomics 17, 1–12. doi: 10.1186/s12864-016-2579-4
Meng, Z., Zhang, Z. L., Yan, T. Y., Lin, Q. F., Wang, Y., Huang, W. Y., et al. (2018). Comprehensively characterizing the cytological features of Saccharum spontaneum by the development of a complete set of chromosome-specific oligo probes. Front. Plant Sci. 9, 1624. doi: 10.3389/fpls.2018.01624
Murata, M., Heslop-Harrison, J. S., Motoyoshi, F. (1997). Physical mapping of the 5S ribosomal RNA genes in Arabidopsis thaliana by multi-color fluorescence in situ hybridization with cosmid clones. Plant J. 12 (1), 31–37. doi: 10.1046/j.1365-313X.1997.12010031.x
Murgha, Y. E., Rouillard, J. M., Gulari, E. (2014). Methods for the preparation of large quantities of complex single-stranded oligonucleotide libraries. PloS One 9, e94752. doi: 10.1371/journal.pone.0094752
Němečková, A., Christelová, P., Čížková, J., Nyine, M., Van den houwe, I., Svačina, R., et al. (2018). Molecular and cytogenetic study of East African Highland Banana. Front. Plant Sci. 9, 1371. doi: 10.3389/fpls.2018.01371
Neumann, P., Navrátilová, A., Koblížková, A., Kejnovský, E., Hřibová, E., Hobza, R., et al. (2011). Plant cytogenetic perspective. Mob. DNA 2 (1), 4. doi: 10.1186/1759-8753-2-4
Novák, P., Hřibová, E., Neumann, P., Koblížková, A., Doležel, J., Macas, J. (2014). Genome-wide analysis of repeat diversity across the family Musaceae. PloS One 9 (6), e98918. doi: 10.1371/journal.pone.0098918
Ortiz, R., Swennen, R. (2014). From crossbreeding to biotechnology-facilitated improvement of banana and plantain. Biotechnol. Adv. 32, 158–169. doi: 10.1016/j.biotechadv.2013.09.010
Osuji, J. O., Crouch, J., Harrison, G., Heslop-Harrison, J. S. (1998). Molecular cytogenetics of Musa species, cultivars and hybrids: location of 18S-5.8S-25S and 5S rDNA and telomere-like sequences. Ann. Bot. 82, 243–248. doi: 10.1006/anbo.1998.0674
Qu, M., Li, K., Han, Y., Chen, L., Li, Z., Han, Y. (2017). Integrated karyotyping of woodland strawberry (Fragaria vesca) with oligopaint FISH probes. Cytogenet. Genome Res. 153, 158–164. doi: 10.1159/000485283
Said, M., Hřibová, E., Danilova, T. V., Karafiátová, M., Čížková, J., Friebe, B., et al. (2018). The Agropyron cristatum karyotype, chromosome structure and cross-genome homoeology as revealed by fluorescence in situ hybridization with tandem repeats and wheat single-gene probes. Theor. Appl. Genet. 131 (10), 2213–2227. doi: 10.1007/s00122-018-3148-9
Schubert, I., Fransz, P. F., Fuchs, J., De Jong, J. H. (2001). Chromosome painting in plants. Methods Cell Sci. 23, 57–69. doi: 10.1023/A:1013137415093
Simmonds, N. W., Shepherd, K. (1955). The taxonomy and origins of the cultivated bananas. J. Linn. Soc. Bot. 55, 302–312. doi: 10.1111/j.1095-8339.1955.tb00015.x
Simmonds, N. W. (1956). Botanical results of the banana collecting expeditions, 1954-5. Kew Bull. 11, 463–489. doi: 10.2307/4109131
Speicher, M. R., Ballard, S. G., Ward, D. C. (1996). Karyotyping human chromosomes by combinatorial multi-fluor FISH. Nat. Genet. 12 (4), 368–375. doi: 10.1038/ng0496-368
The Arabidopsis Genome Initiative. (2000). Analysis of the genome sequence of the flowering plant Arabidopsis thaliana. Nature 408, 6814, 796–815. doi: 10.1038/35048692
The International Brachypodium Initiative (2010). Genome sequencing and analysis of the model grass Brachypodium distachyon. Nature 463 (7282), 763–768. doi: 10.1038/nature08747
Valárik, M., Šimková, H., Hřibová, E., Šafář, J., Doleželová, M., Doležel, J. (2002). Isolation, characterization and chromosome localization of repetitive DNA sequences in bananas (Musa spp.). Chromosome Res. 10 (2), 89–100. doi: 10.1023/A:1014945730035
Xin, H., Zhang, T., Han, Y., Wu, Y., Shi, J., Xi, M., et al. (2018). Chromosome painting and comparative physical mapping of the sex chromosomes in Populus tomentosa and Populus deltoides. Chromosoma. 127, 313–321. doi: 10.1007/s00412-018-0664-y
Keywords: banana, chromosome identification, fluorescence in situ hybridization, molecular karyotype, Musa, oligo painting FISH
Citation: Šimoníková D, Němečková A, Karafiátová M, Uwimana B, Swennen R, Doležel J and Hřibová E (2019) Chromosome Painting Facilitates Anchoring Reference Genome Sequence to Chromosomes In Situ and Integrated Karyotyping in Banana (Musa Spp.). Front. Plant Sci. 10:1503. doi: 10.3389/fpls.2019.01503
Received: 29 July 2019; Accepted: 29 October 2019;
Published: 20 November 2019.
Edited by:
Martin A. Lysak, Masaryk University, CzechiaReviewed by:
Alexander Betekhtin, University of Silesia of Katowice, PolandJiming Jiang, University of Wisconsin-Madison, United States
Copyright © 2019 Šimoníková, Němečková, Karafiátová, Uwimana, Swennen, Doležel and Hřibová. This is an open-access article distributed under the terms of the Creative Commons Attribution License (CC BY). The use, distribution or reproduction in other forums is permitted, provided the original author(s) and the copyright owner(s) are credited and that the original publication in this journal is cited, in accordance with accepted academic practice. No use, distribution or reproduction is permitted which does not comply with these terms.
*Correspondence: Eva Hřibová, hribova@ueb.cas.cz