- 1Department of Plant Sciences, University of Cambridge, Cambridge, United Kingdom
- 2Divisions of Plant Science and Biochemistry, University of Missouri, Columbia, MO, United States
- 3Université Clermont Auvergne, INRA, PIAF, Clermont-Ferrand, France
Adenosine 5′-triphosphate (ATP) is an important extracellular signaling agent, operating in growth regulation, stomatal conductance, and wound response. With the first receptor for extracellular ATP now identified in plants (P2K1/DORN1) and a plasma membrane NADPH oxidase revealed as its target, the search continues for the components of the signaling cascades they command. The Arabidopsis root elongation zone epidermal plasma membrane has recently been shown to contain cation transport pathways (channel conductances) that operate downstream of P2K1 and could contribute to extracellular ATP (eATP) signaling. Here, patch clamp electrophysiology has been used to delineate two further conductances from the root elongation zone epidermal plasma membrane that respond to eATP, including one that would permit chloride transport. This perspective addresses how these conductances compare to those previously characterized in roots and how they might operate together to enable early events in eATP signaling, including elevation of cytosolic-free calcium as a second messenger. The role of the reactive oxygen species (ROS) that could arise from eATP’s activation of NADPH oxidases is considered in a qualitative model that also considers the regulation of plasma membrane potential by the concerted action of the various cation and anion conductances. The molecular identities of the channel conductances in eATP signaling remain enigmatic but may yet be found in the multigene families of glutamate receptor-like channels, cyclic nucleotide-gated channels, annexins, and aluminum-activated malate transporters.
Introduction
Adenosine 5′-triphosphate (ATP) is well known as an essential cellular energy source. However, the recognition of ATP as an extracellular signaling agent in plants is becoming more widespread (Clark and Roux, 2018). Extracellular ATP (eATP) has been shown to modulate growth and development, particularly of pollen and root hairs (Roux and Steinebrunner, 2007; Clark et al., 2010; Wu et al., 2018). It is abundant at the apex of growing roots and root hairs in a range of plants (Kim et al., 2006) and is involved in root gravitropism and root curling (Tang et al., 2003; Yang et al., 2015). eATP can also regulate stomatal movement (Clark et al., 2011; Hao et al., 2012; Wang et al., 2014; Chen et al., 2017). Activation of plant stress responses by eATP, notably wounding responses, may be through second messengers such as nitric oxide, reactive oxygen species (ROS), and cytosolic-free calcium ([Ca2+]cyt) (Demidchik et al., 2003a; Song et al., 2006; Foresi et al., 2007; Torres et al., 2008; Wu et al., 2008; Demidchik et al., 2009; Choi et al., 2014). A key advance in the field comes from the identification of the first angiosperm eATP receptor, P2K1 (DORN1, does not respond to nucleotides 1), in Arabidopsis thaliana. The P2K1 nomenclature is preferred since this aligns the plant work with the greater body of animal literature focused on the P2X and P2Y families of purinergic receptors. The P2K1 plasma membrane (PM) receptor kinase commands increases in ROS and [Ca2+]cyt by eATP that operate in seedling wound transcriptional response and regulation of stomatal aperture (Choi et al., 2014; Chen et al., 2017).
Ion fluxes across the PM are likely to be critical components of early eATP signal cascades, particularly in the generation of a [Ca2+]cyt signal. The majority of research to date on eATP-induced ion fluxes has been on root cells, which have proven to be sensitive and experimentally tractable. eATP has been found to depolarize (i.e., make more positive) the PM potential of growing Arabidopsis root hairs (Lew and Dearnaley, 2000), indicating cation influx/anion efflux. It has also been observed to affect root PM Ca2+, K+, and Na+ fluxes (Dark et al., 2011; Demidchik et al., 2011; Lang et al., 2014; Zhao et al., 2016). Moreover, the Ca2+ and K+ fluxes in response to eATP vary spatially along the root (measured using an extracellular, self-referencing ion-selective microelectrode; Dark et al., 2011; Demidchik et al., 2011). The Arabidopsis elongation zone epidermis proved more sensitive to eATP than the mature zone, also sustaining greater net Ca2+ influx and K+ efflux (Dark et al., 2011; Demidchik et al., 2011). Such Ca2+ influx across the PM could relate to eATP-induced [Ca2+]cyt increase as a second messenger. eATP has now been shown to elevate root [Ca2+]cyt, measured using the luminometric reporter aequorin and fluorescence resonance energy transfer (FRET)-based reporters such as YC3.6 (Demidchik et al., 2003a; Demidchik et al., 2009; Tanaka et al., 2010; Loro et al., 2012; Behera et al., 2018). Blocking putative PM Ca2+ influx channel proteins with lanthanides or chelating extracellular Ca2+ can prevent eATP-induced [Ca2+]cyt elevation (Demidchik et al., 2003a; Demidchik et al., 2009; Behera et al., 2018), implicating such passive transporters in the generation of the [Ca2+]cyt signal.
Patch clamp electrophysiology has been applied successfully to resolve eATP-activated PM Ca2+ influx channels in Arabidopsis root cells. Mature epidermal cells have a hyperpolarization activated calcium channel (HACC) conductance that is further activated by eATP (Demidchik et al., 2009). Similar HACC conductances activated by eATP have since been identified at the guard cell and pollen PM (Wang et al., 2014; Wu et al., 2018). In root epidermis, the HACC may lie downstream of the PM RBOHC NADPH oxidase isoform. This HACC may contribute to the net Ca2+ influx reported for this root zone (Demidchik et al., 2009; Shang et al., 2009; Dark et al., 2011; Demidchik et al., 2011). Patch clamping has also implicated the heterotrimeric G protein α subunit in eATP activation of the PM HACC conductance of apical root cells (Zhu et al., 2017). Furthermore, patch clamping of elongation zone epidermal PM has revealed a small HACC-like conductance (which also permits K+ influx) and a K+ efflux conductance (in 44 out of 113 protoplasts) that not only are activated by eATP but also lie downstream of P2K1 (Wang et al., 2018). The K+ efflux pathway resembles a depolarization-activated nonselective cation channel conductance (NSCC; Wang et al., 2018). It is feasible that these could contribute to the Ca2+ influx and K+ efflux evoked by eATP in the elongation zone epidermis (Demidchik et al., 2009; Dark et al., 2011; Demidchik et al., 2011). Thus, so far, little is known about the regulation of plant PM channels by eATP. Based on further patch-clamp studies here of PM conductances from the root elongation zone epidermis, early ionic events in response to eATP (narrowed down to the level of ion channel conductance) are revealed in this perspective.
Diverse Conductances in the Plasma Membrane of Arabidopsis Root Epidermis
A range of Ca2+ channels, K+ channels, NSCC, and anion channels have been identified previously in Arabidopsis root epidermal PM through patch clamping (e.g., Demidchik et al., 2002; Foreman et al., 2003; Pilot et al., 2003; Diatloff et al., 2004; Demidchik et al., 2007; Demidchik et al., 2009; Hedrich et al., 2012; Laohavisit et al., 2012; Demidchik et al., 2014; Makavitskaya et al., 2018). Using the same experimental conditions as our previous study (which identified the eATP-activated small HACC-like and K+ efflux conductances; Wang et al., 2018), 26 out of 113 protoplasts from the elongation zone epidermis were found to have a large time-dependent HACC conductance (Véry and Davies, 2000) under control conditions, which was accompanied by an instantaneous outward current at depolarized voltages (Figure 1A). eATP increased HACC currents rapidly (within a minute) after treatment, and activation lasted for at least 10 min (Figure 1A). This was a similar time course to the eATP-activated HACC from mature epidermal protoplasts, in which activation persisted for up to 20 min (Demidchik et al., 2009). NaCl (600 µM, the control for the Na-ATP salt) did not cause HACC activation (Figure S1). eATP-induced HACC inward currents were blocked by the lanthanide cation channel blocker Gd3+, indicating cation permeability (Figure S2A). Qualitatively, the eATP-activated HACC resembled those found in Arabidopsis root tip cell PM, Vicia faba guard cell PM, and tobacco pollen PM (Wang et al., 2014; Zhu et al., 2017; Wu et al., 2018).
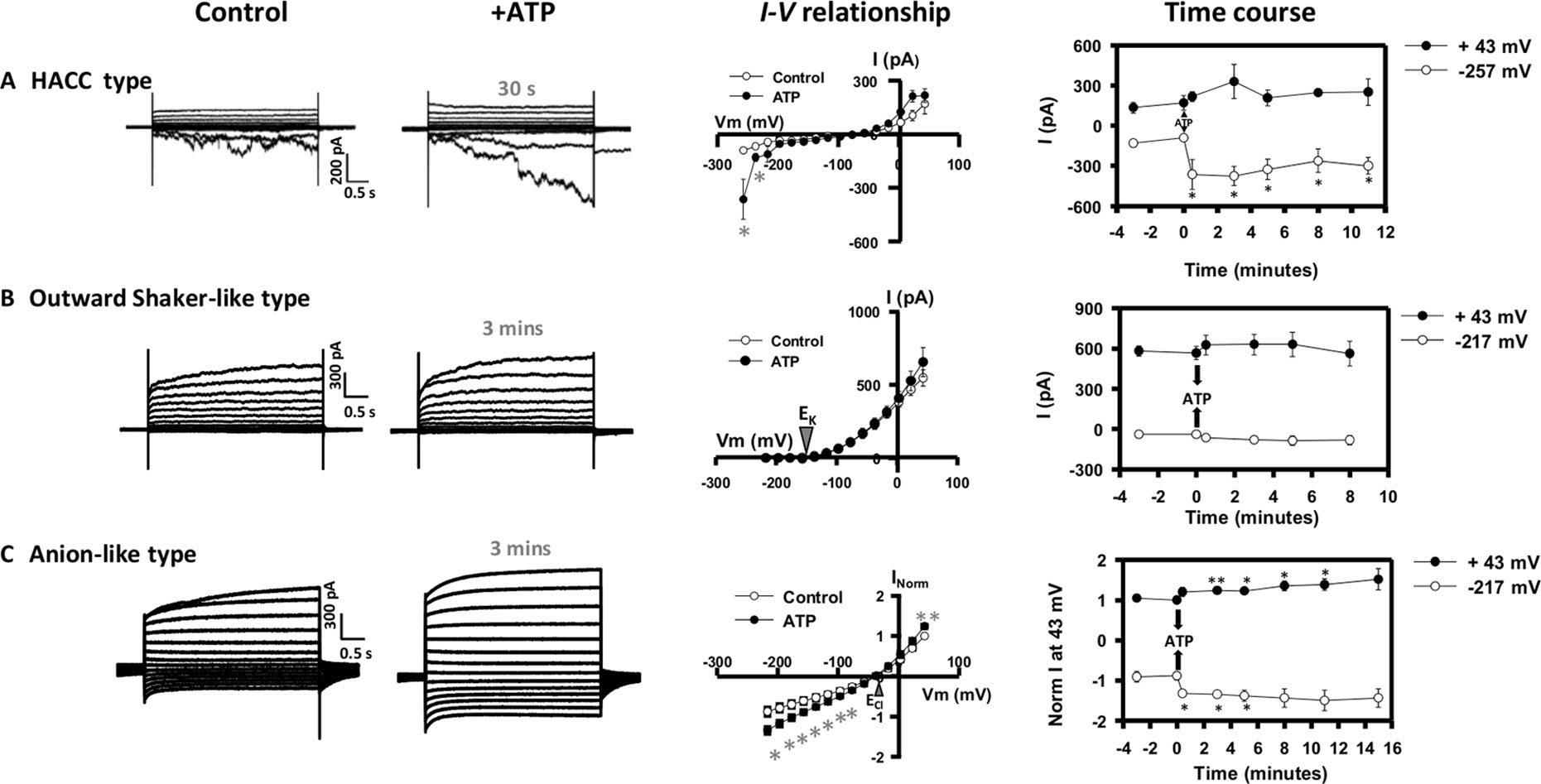
Figure 1 Effect of extracellular adenosine 5′-triphosphate (eATP) on diverse plasma membrane (PM) conductances from the root elongation zone epidermis. Protoplasts were isolated and used in whole-cell patch clamp recordings as described previously (Wang et al., 2018). Origin of the protoplasts was confirmed with the N9093 epidermal-specific green fluorescent protein (GFP) reporter line (Diatloff et al., 2004). This configuration measures populations of channels. Plasma membrane potential was held at −137 mV prior to a stepwise voltage protocol of 20 mV increments. Whole-cell currents were recorded in a bath solution containing (mM) 20 CaCl2, 0.1 KCl, and 5 MES-Tris, at pH 5.6. Pipette solution comprised (mM) 40 K-gluconate, 10 KCl, 0.4 CaCl2, 1 BAPTA, and 2 MES-Tris, at pH 7.2. Osmolarity of both solutions was adjusted to 280–290 mOsm with D-sorbitol. Representative current traces of (A) the hyperpolarization activated calcium channel (HACC) conductance, (B) the outward Shaker-like conductance, and (C) the anion conductance under control and eATP conditions (300 µM) are shown in the left panel. Corresponding mean I–V relationships for control (○) and eATP (•) treatments are shown in the central panel with time of treatment indicated. The right panel presents the time course of eATP-activated outward currents at +43 mV (•) and inward currents at −257 mV/−217 mV (○) for each type of conductance. Data are mean ± SE (n = 4 in A, 5 in B, and 4 in C). Negative current is net cation influx or anion efflux. Positive current is net cation efflux or anion influx. Asterisks denote significant difference from control. *p < 0.05, **p < 0.01 (Student’s t-test).
Protoplasts (11 out of 113) also presented a conductance dominated by a non-linear outward current that activated around the equilibrium potential for K+ [EK annotated on the current voltage (I–V) graph in Figure 1B]. This resembled previously characterized Shaker outward K+ channel conductances (Gaymard et al., 1998; Ache et al., 2000; Hosy et al., 2003; Li et al., 2016) and would mediate K+ efflux from the cytosol. Similar to the plant Shaker outward K+ channels reported so far (Gaymard et al., 1998; Ache et al., 2000; Hosy et al., 2003; Li et al., 2016; Wang et al., 2019), this conductance was inhibited by external application of the classical K+ channel blocker, tetraethylammonium (TEA) (Figure S2B). This Shaker-like outward conductance was not significantly affected by eATP (Figure 1B). This distinguishes the conductance from the eATP-activated NSCC K+ efflux conductance found by Wang et al. (2018). Additionally, the time constant of activation at 23 mV (185.3 ± SE 22.7; n = 6) of the Shaker-like outward conductance is twofold slower than the NSCC outward conductance, suggesting that they are distinct conductances.
An anion conductance was evident in 12 protoplasts. This reversed close to ECl (Figure 1C), indicating an anion (Cl−) permeability. The I–V relationships for control and eATP trials of the individual protoplasts tested are shown in Figure S3. There was variation in the magnitude of current, and statistical analysis of the eATP effect was after normalization (Maierhofer et al., 2014). Qualitatively, this conductance resembles a root epidermal PM conductance that permits ascorbate efflux (Makavitskaya et al., 2018), and the mild deactivation at negative voltages resembles that of the wheat Al3+-activated ALMT1 anion channel (Zhang et al., 2008). Anion fluxes (especially Cl− fluxes) in eATP signaling are poorly documented, possibly due to the methodological limitations of using self-referencing ion-selective electrodes (Shabala et al., 2013; Pottosin et al., 2018). This anion conductance would permit anion efflux at hyperpolarized voltage and anion influx at depolarized voltage. Anion influx responded rapidly (within a minute) to eATP, while efflux was significantly increased after 3 min and was significant for several minutes after (Figure 1C). The eATP-activated conductance was insensitive to Gd3+ (Figure S2C), further supporting its identity as an anion conductance. This conductance may be relevant to the effects of eATP on membrane voltage. Overall, of the 113 protoplasts studied, the most frequently occurring conductances were the small HACC-like conductance (which also permits K+ influx) and the K+ efflux conductance reported by Wang et al. (2018). The remaining 20 protoplasts of the 113 that were not described here did not display a clear conductance type.
Multiple Conductances Could Operate in Root Epidermal eATP Signaling
Combining this new knowledge of eATP-activated root epidermal conductances with findings from previous studies (Véry and Davies, 2000; Demidchik et al., 2003a; Demidchik et al., 2003b; Demidchik et al., 2007; Demidchik et al., 2009; Shang et al., 2009; Demidchik et al., 2011; Tavares et al., 2011; Choi et al., 2014; Wilkins et al., 2016; Chen et al., 2017; Rodrigues et al., 2017; Gutermuth et al., 2018; Pottosin et al., 2018; Wang et al., 2018) allows generation of a hypothetical and qualitative model of the early steps in eATP signaling in Arabidopsis epidermis (Figure 2). This presumes that the conductances found to be activated by eATP here and by Wang et al. (2018) would all be present in one cell, despite the varying frequency of occurrence in patched protoplasts. Those frequencies may reflect different levels of cellular maturity at the point of release or perhaps even the PM state (pump state, K+ state, or depolarized state; Tyerman et al., 2001) at the initiation of patching. In this model, eATP is expected to modulate the root epidermal PM potential through the regulation of these ion conductances. eATP recognition is postulated to be by the PM receptor P2K1 (Choi et al., 2014; Wang et al., 2018). This could possibly phosphorylate the channels involved here, with the HACC as a prime target. However, in guard cells, P2K1 phosphorylates the respiratory burst oxidase protein D (RBOHD) NADPH oxidase, resulting in elevated production of ROS (Chen et al., 2017). This could also occur in the root epidermis (perhaps even with the RBOHC isoform; Demidchik et al., 2009) as eATP can increase root epidermal cytosolic ROS (mainly H2O2) within seconds in an RBOH-dependent manner, which in turn activates downstream [Ca2+]cyt signaling (Demidchik et al., 2009; Demidchik et al., 2011). It is envisaged that extracellular H2O2 (as a downstream product of RBOH activity) could enter the cytosol through PM aquaporins, in common with guard cells (Rodrigues et al., 2017). Due to the fast activation found here of the HACC conductance upon eATP addition (Figure 1A), this HACC may therefore be directly or indirectly responsive to ROS (Figure 2). Which ROS and at which membrane face? Activation of elongation zone epidermal HACC by extracellular H2O2 has been found, but the time course of activation was not reported (Demidchik et al., 2007). Entry of H2O2 into the cytosol could also produce intracellular hydroxyl radicals [formed through a Cu+ catalyst in the Fenton reaction (Richards et al., 2015) to activate Ca2+ influx (Rodrigo-Moreno et al., 2013)]. HACC activation in this cell type by extracellular hydroxyl radicals occurs in a few minutes (Foreman et al., 2003) and also occurs in mature epidermis (time course not reported; Laohavisit et al., 2012). All scenarios assume that ROS could be generated under patch clamp conditions. Supporting this, eATP activation of the mature epidermis HACC in patch clamp was lost in the rbohc loss-of-function mutant and prevented in wild type by the reductant dithiothreitol, suggesting that ROS production is possible (Demidchik et al., 2009). Also, activation of guard cell PM HACC by eATP was prevented by DPI (diphenyleneiodonium), an inhibitor of flavoproteins including NADPH oxidases, placing the HACC downstream of such enzymes (Wang et al., 2014).
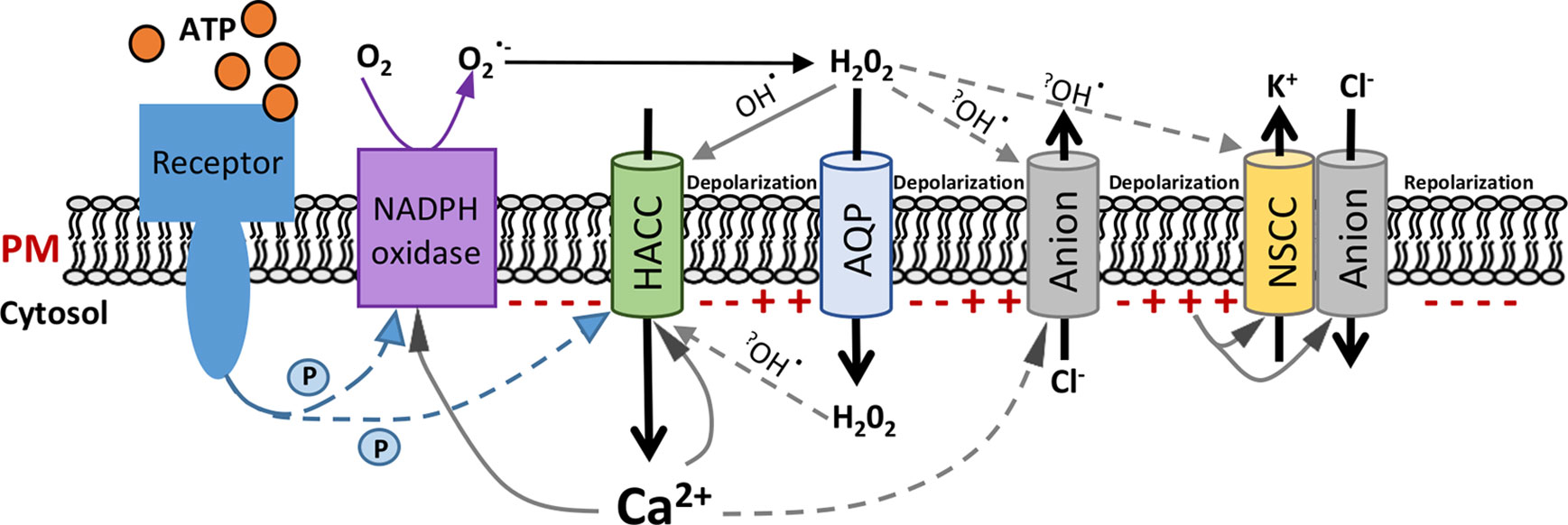
Figure 2 Schematic of a hypothetical pathway of extracellular adenosine 5′-triphosphate (eATP)-activated conductances in root epidermal plasma membrane. Hypothetical model integrating the eATP-induced plasma membrane (PM) conductances from this study and previous findings referenced in the main text. The signal cascade is presented from left to right, starting with eATP perception by the receptor. Polarity of the PM potential at the cytosolic face is represented by “−” or “+”. Phosphorylation is indicated by “P”. An early event would be Ca2+ influx through hyperpolarization-activated Ca2+ channels (HACCs). Extracellular H2O2 could enter the cytosol through aquaporins (AQPs). H2O2 could directly act on ion channels or be converted to hydroxyl radicals (OH•) through Fenton reactions (indicated by question marks). Anion channels would sequentially permit Cl− efflux; then influx and nonselective cation-permeable channels (NSCCs) would facilitate K+ efflux. The overall sequence would promote repolarization of the PM potential. Arrows indicate possible activation pathways but do not necessarily imply direct interactions. The dashed arrows are predicted pathways, which are highly recommended to be investigated in future.
As Ca2+ is transported into the cytosol, it could lead to a depolarization of the root epidermal PM and possibly have a positive feedback effect on the RBOH (through EF hands) and the HACC (Wilkins et al., 2016). It has been shown previously that increased [Ca2+]cyt shifts the HACC activation threshold to depolarized voltage and increases current magnitude (Véry and Davies, 2000; Demidchik et al., 2002). Then, a subsequent Cl− release at a more depolarized voltage through the eATP-activated anion conductance (Figure 1C) could deepen the PM depolarization (Figure 2). It may also be that Cl− efflux through the anion conductance is stimulated by the increased [Ca2+]cyt. The precedent for this comes from the Arabidopsis pollen tube apical PM, where hyperpolarization-induced [Ca2+]cyt increase causes increased Cl− efflux (Tavares et al., 2011), possibly through Ca2+-dependent protein kinases (Gutermuth et al., 2018). Another stimulator could be eATP-induced ROS (Kim et al., 2006; Demidchik et al., 2009). Indeed, it has been reported that extracellular hydroxyl radicals could induce efflux of cytosolic anions from barley elongation zone epidermal protoplasts, which could contribute to root PM depolarization (Pottosin et al., 2018). If the eATP-activated anion conductance found here were capable of releasing ascorbate to the extracellular PM face (Makavitskaya et al., 2018), it could even promote ascorbate-fueled extracellular hydroxyl radical production (Richards et al., 2015; Makavitskaya et al., 2018).
After sufficient depolarization, the activation of Cl−influx through the anion conductance (Figure 1C) and K+ efflux through the NSCC-like conductance (Wang et al., 2018) would increase. The latter was found only to be significant after 8 min of exposure to eATP (Wang et al., 2018) and may well be a late event. Qualitatively, this NSCC-like conductance resembles an elongation zone PM NSCC conductance found to be activated by extracellular hydroxyl radicals (Demidchik et al., 2003b). It may be that hydroxyl radicals are involved in eATP signaling. Alternatively, as high extracellular H2O2 inhibits K+ efflux by the PM NSCC (Demidchik et al., 2003b), late activation of the NSCC-like conductance could reflect the lowering of H2O2 concentration at the extracellular PM face. The induction of cation efflux and anion influx upon longer ATP treatment (>3 min) could finally repolarize the PM of the root epidermis. Although the NSCC-like conductance found by Wang et al. (2018) is proposed to participate in the PM repolarization in the present model (Figure 2), a potential role for the Shaker-like outward conductance (shown in Figure 1B) cannot be excluded. When PM repolarizes to a certain voltage, passing the activation potential of the NSCC-like conductance, the Shaker-like outward conductance might contribute (probably after 8 min) to continuing the PM repolarization, thus eventually hyperpolarizing the plasma membrane.
Future Directions
While eATP has been shown to depolarize the PM (Lew and Dearnaley, 2000), showing the dependence on P2K1 would be critical to start verifying this model. P2K1 has been shown to be required for the eATP-activated root epidermis PM HACC-like and NSCC-like conductances (Wang et al., 2018). Whether P2K1 (or an as yet unknown receptor; Clark and Roux, 2018) governs the eATP-induced HACC and anion currents remains, however, to be elucidated. The relationship between P2K1 and RBOHs in the root epidermis also needs to be tested, as does the possible role of ROS in activating the conductances found in the present study. It has been reported that H2O2 induces reactive carbonyl species (RCS) and that these significantly inhibit K+ inward channels in guard cell PM (Islam et al., 2016). It would be interesting to test root epidermis overexpressing 2-alkenal reductase (an RCS scavenger; Islam et al., 2016) to see whether eATP signaling would normally result in inhibition of K+ inward channels through RCS production.
Searching for the molecular identities of these root epidermal conductances in eATP signaling is imperative. Patch-clamp analyses of cyclic nucleotide-gated channel (CNGC) mutants suggested that CNGC2, CNGC4, CNGC5, and CNGC6 from Arabidopsis could contribute to HACC conductances (Ali et al., 2007; Gao et al., 2012; Wang et al., 2013; Tian et al., 2019). The CNGC family has also been proposed to encode NSCC (Köhler et al., 1999; Jammes et al., 2011; Demidchik, 2014). So far, Arabidopsis CNGC14 has been discounted as a contributor to eATP-induced [Ca2+]cyt elevation in roots (Shih et al., 2015). Arabidopsis CNGC20 may be a candidate if eATP were to promote production of intracellular ROS, as this channel subunit has been found at the PM (Fischer et al., 2013) and may have an intracellular copper-binding site to permit Fenton generation of hydroxyl radicals for its own activation (Demidchik et al., 2014). In mature epidermis and root hairs, the hydroxyl radical-activated HACC is entirely reliant on Annexin1 (Laohavisit et al., 2012), raising the possibility of this protein’s involvement in younger cells. In addition to the CNGC family and annexins, the glutamate receptor-like (GLR) family provides other candidates for HACCs and NSCC (Roy et al., 2008; Tapken and Hollmann, 2008; Swarbreck et al., 2013; Toyota et al., 2018). Arabidopsis GLR3.3 and GLR3.6 operate in wound-induced leaf [Ca2+]cyt increase (Vincent et al., 2017) and so would be prime candidates. For the Shaker-like K+ efflux conductance that appeared insensitive to eATP, it could be shaped by the guard cell outward rectifier K (GORK) channel, since this is expressed in the root epidermis and has been characterized as a root K+ outward channel in Arabidopsis (Ivashikina et al., 2001; Demidchik, 2014). Moreover, it can contribute to root cell PM hyperpolarization (Planes et al., 2014), consistent with a role in restoring the PM voltage at the end of eATP signaling. However, root PM GORK releases K+ in response to extracellular hydroxyl radicals (Demidchik et al., 2010), which is at odds with the production of this ROS in the current model. The expectation would be for GORK to be activated, but with maximal activation by radicals occurring after 15–20 min (Demidchik et al., 2010); recordings here may not have been long enough. Moreover, GORK is a tightly regulated channel, controlled by its positional clustering (Eisenach et al., 2014), 14-3-3 binding, and [Ca2+]cyt-dependent phosphorylation status (Van Kleeff et al., 2018), and so other regulatory factors could be at play.
The novel finding here of an eATP-activated anion conductance adds another component to eATP signaling. Plant PM anion fluxes can involve slow-activating and rapid-activating anion channels, provided by members of the slow anion channel-associated (SLAC) and aluminum-activated malate transporter (ALMT) families, respectively (Hedrich et al., 2012). At this point, an ALMT channel appears the most likely candidate for the eATP-activated anion channel, but members of the ATP-binding cassette superfamily should be considered given that mammalian ABC transporters can function as Cl− channels (Anderson et al., 1991). In addition to this perspective on the molecular identities of channels in eATP signaling, it is important to note two other transporters that are omitted from our simplistic model; the PM H+-ATPase and Ca2+-ATPase. The PM H+-ATPase plays a major part in generating the membrane potential, setting the electrochemical driving force for eATP-induced Ca2+ influx. AHA2 is the predominant PM H+-ATPase in Arabidopsis root cells (Falhof et al., 2016). Accordingly, Arabidopsis roots lacking the AHA2 isoform have a lower eATP-induced [Ca2+]cyt increase than wild type (Haruta and Sussman, 2012). Whether the eATP-induced [Ca2+]cyt increase regulates H+-ATPase activity remains to be determined. The PM Ca2+-ATPases (ACA8 and ACA10) that pump Ca2+ out of the cytosol to help end the eATP-induced [Ca2+]cyt signal in root cells (Behera et al., 2018) are unlikely to contribute to membrane potential repolarization as such transporters are electroneutral Ca2+:2H+ exchangers (Luoni et al., 2000). They could, however, contribute to the cytosolic acidification that lags behind the eATP-induced [Ca2+]cyt increase in root cells (Behera et al., 2018). This acidification is unlikely to affect the channels mediating Ca2+ influx (Behera et al., 2018) but could induce activation of slow anion channels (Colcombet et al., 2005) and the PM H+-ATPase (Behera et al., 2018). Whether the activation of PM H+-ATPase by the cytosolic acidification (Behera et al., 2018) could help in PM repolarization needs to be addressed.
Overall, further investigation of the functional properties of the root epidermal PM conductances activated by eATP (and other extracellular nucleotides) will be required to make progress in understanding their molecular identities and the downstream signaling pathways.
Data Availability
The datasets generated for this study are available on request to the corresponding author.
Author Contributions
LW designed and performed the experiments and then analyzed data. All authors conceived the project. LW and JD jointly conceived and wrote the manuscript with contributions from NL-F, VL, BM, and GS.
Funding
Results have been achieved within the framework of the third call of the ERA-NET for Coordinating Action in Plant Sciences, with funding from the BBSRC (BB/S004637/1) and the University of Cambridge Newton Trust.
Conflict of Interest Statement
The authors declare that the research was conducted in the absence of any commercial or financial relationships that could be construed as a potential conflict of interest.
Acknowledgments
We acknowledge the financial support of the BBSRC and University of Cambridge Newton Trust. We thank Prof. J. Hasselof (Cambridge) for the N9093 line. We apologize to authors whose work we have not cited due to manuscript length restriction.
Supplementary Material
The Supplementary Material for this article can be found online at: https://www.frontiersin.org/articles/10.3389/fpls.2019.01064/full#supplementary-material
References
Ache, P., Becker, D., Ivashikina, N., Dietrich, P., Roelfsema, M. R. G., Hedrich, R. (2000). GORK, a delayed outward rectifier expressed in guard cells of Arabidopsis thaliana, is a K+-selective, K+-sensing ion channel. FEBS Lett. 486 (2), 93–98. doi: 10.1016/S0014-5793(00)02248-1
Ali, R., Ma, W., Lemtiri-Chlieh, F., Tsaltas, D., Leng, Q., von Bodman, S., et al. (2007). Death don’t have no mercy and neither does calcium: Arabidopsis cyclic nucleotide gated channel 2 and innate immunity. Plant Cell 19 (3), 1081–1095. doi: 10.1105/tpc.106.045096
Anderson, M. P., Gregory, R. J., Thompson, S., Souza, D. W., Paul, S., Mulligan, R. C., et al. (1991). Demonstration that CFTR is a chloride channel by alteration of its anion selectivity. Science 253 (5016), 202–205. doi: 10.1126/science.1712984
Behera, S., Xu, Z., Luoni, L., Bonza, C., Doccula, F. G., DeMichelis, M. I., et al. (2018). Cellular Ca2+ signals generate defined pH signatures in plants. Plant Cell 30 (11), 2704–2719. doi: 10.1105/tpc.18.00655
Chen, D., Cao, Y., Li, H., Kim, D., Ahsan, N., Thelen, J., et al. (2017). Extracellular ATP elicits DORN1-mediated RBOHD phosphorylation to regulate stomatal aperture. Nat. Commun. 8 (1), 2265. doi: 10.1038/s41467-017-02340-3
Choi, J., Tanaka, K., Cao, Y., Qi, Y., Qiu, J., Liang, Y., et al. (2014). Identification of a plant receptor for extracellular ATP. Science 343 (6168), 290–294. doi: 10.1126/science.343.6168.290
Clark, G., Roux, S. (2018). Role of Ca2+ in mediating plant responses to extracellular ATP and ADP. Int. J. Mol. Sci. 19 (11), 3590. doi: 10.3390/ijms19113590
Clark, G., Fraley, D., Steinebrunner, I., Cervantes, A., Onyirimba, J., Liu, A., et al. (2011). Extracellular nucleotides and apyrases regulate stomatal aperture in Arabidopsis. Plant Physiol. 156 (4), 1740–1753. doi: 10.1104/pp.111.174466
Clark, G., Wu, M., Wat, N., Onyirimba, J., Pham, T., Herz, N., et al. (2010). Both the stimulation and inhibition of root hair growth induced by extracellular nucleotides in Arabidopsis are mediated by nitric oxide and reactive oxygen species. Plant Mol. Biol. 74 (4-5), 423–435. doi: 10.1007/s11103-010-9683-7
Colcombet, J., Lelièvre, F., Thomine, S., Barbier-Brygoo, H., Frachisse, J. M. (2005). Distinct pH regulation of slow and rapid anion channels at the plasma membrane of Arabidopsis thaliana hypocotyl cells. J. Exp. Bot. 56 (417), 1897–1903. doi: 10.1093/jxb/eri184
Dark, A., Demidchik, V., Richards, S. L., Shabala, S., Davies, J. M. (2011). Release of extracellular purines from plant roots and effect on ion fluxes. Plant Signal. Behav. 6 (11), 1855–1857. doi: 10.4161/psb.6.11.17014
Demidchik, V. (2014). Mechanisms and physiological roles of K+ efflux from root cells. J. Plant Physiol. 171 (9), 696–707. doi: 10.1016/j.jplph.2014.01.015
Demidchik, V., Bowen, H. C., Maathuis, F. J., Shabala, S. N., Tester, M. A., White, P. J., et al. (2002). Arabidopsis thaliana root non-selective cation channels mediate calcium uptake and are involved in growth. Plant J. 32 (5), 799–808. doi: 10.1046/j.1365-313X.2002.01467.x
Demidchik, V., Cuin, T. A., Svistunenko, D., Smith, S. J., Miller, A. J., Shabala, S., et al. (2010). Arabidopsis root K+-efflux conductance activated by hydroxyl radicals: single-channel properties, genetic basis and involvement in stress-induced cell death. J. Cell Sci. 123 (9), 1468–1479. doi: 10.1242/jcs.064352
Demidchik, V., Nichols, C., Oliynyk, M., Dark, A., Glover, B. J., Davies, J. M. (2003a). Is ATP a signaling agent in plants? Plant Physiol. 133 (2), 456–461. doi: 10.1104/pp.103.024091
Demidchik, V., Shabala, S. N., Coutts, K. B., Tester, M. A., Davies, J. M. (2003b). Free oxygen radicals regulate plasma membrane Ca2+ and K+-permeable channels in plant root cells. J. Cell Sci. 116 (1), 81–88. doi: 10.1242/jcs.00201
Demidchik, V., Shabala, S. N., Davies, J. M. (2007). Spatial variation in H2O2 response of Arabidopsis thaliana root epidermal Ca2+ flux and plasma membrane Ca2+ channels. Plant J. 49 (3), 377–386. doi: 10.1111/j.1365-313X.2006.02971.x
Demidchik, V., Shang, Z., Shin, R., Thompson, E., Rubio, L., Laohavisit, A., et al. (2009). Plant extracellular ATP signalling by plasma membrane NADPH oxidase and Ca2+ channels. Plant J. 58 (6), 903–913. doi: 10.1111/j.1365-313X.2009.03830.x
Demidchik, V., Shang, Z., Shin, R., Colaço, R., Laohavisit, A., Shabala, S., et al. (2011). Receptor-like activity evoked by extracellular ADP in Arabidopsis thaliana root epidermal plasma membrane. Plant Physiol. 156 (3), 1375–1385. doi: 10.1104/pp.111.174722
Demidchik, V., Straltsova, D., Medvedev, S. S., Pozhvanov, G. A., Sokolik, A., Yurin, V. (2014). Stress-induced electrolyte leakage: the role of K+-permeable channels and involvement in programmed cell death and metabolic adjustment. J. Exp. Bot. 65 (5), 1259–1270. doi: 10.1093/jxb/eru004
Diatloff, E., Roberts, M., Sanders, D., Roberts, S. K. (2004). Characterization of anion channels in the plasma membrane of Arabidopsis epidermal root cells and the identification of a citrate-permeable channel induced by phosphate starvation. Plant Physiol. 136 (4), 4136–4149. doi: 10.1104/pp.104.046995
Eisenach, C., Papanatsiou, M., Hillert, E. K., Blatt, M. R. (2014). Clustering of the K+ channel GORK of Arabidopsis parallels its gating by extracellular K+. Plant J. 78 (2), 203–214. doi: 10.1111/tpj.12471
Falhof, J., Pedersen, J. T., Fuglsang, A. T., Palmgren, M. (2016). Plasma membrane H+-ATPase regulation in the center of plant physiology. Mol. Plant 9 (3), 323–337. doi: 10.1016/j.molp.2015.11.002
Fischer, C., Kugler, A., Hoth, S., Dietrich, P. (2013). An IQ domain mediates the interaction with calmodulin in a plant cyclic nucleotide-gated channel. Plant Cell Physiol. 54 (4), 573–584. doi: 10.1093/pcp/pct021
Foreman, J., Demidchik, V., Bothwell, J. H., Mylona, P., Miedema, H., Torres, M. A., et al. (2003). Reactive oxygen species produced by NADPH oxidase regulate plant cell growth. Nature 422 (6930), 442–446. doi: 10.1038/nature01485
Foresi, N. P., Laxalt, A. M., Tonón, C. V., Casalongué, C. A., Lamattina, L. (2007). Extracellular ATP induces nitric oxide production in tomato cell suspensions. Plant Physiol. 145 (3), 589–592. doi: 10.1104/pp.107.106518
Gao, F., Han, X. W., Wu, J. H., Zheng, S. Z., Shang, Z. L., Sun, D. Y., Zhou, R. G., Li, B. (2012). A heat activated calcium permeable channel - Arabidopsis cyclic nucleotide-gated ion channel 6 - is involved in heat shock responses. Plant J. 70 (6), 1056–1069. doi: 10.1111/j.1365-313X.2012.04969.x
Gaymard, F., Pilot, G., Lacombe, B., Bouchez, D., Bruneau, D., Boucherez, J., et al. (1998). Identification and disruption of a plant shaker-like outward channel involved in K+ release into the xylem sap. Cell 94 (5), 647–655. doi: 10.1016/S0092-8674(00)81606-2
Gutermuth, T., Herbell, S., Lassig, R., Brosché, M., Romeis, T., Feijó, J. A., et al. (2018). Tip-localized Ca2+-permeable channels control pollen tube growth via kinase-dependent R-and S-type anion channel regulation. New Phytol. 218 (3), 1089–1105. doi: 10.1111/nph.15067
Hao, L. H., Wang, W. X., Chen, C., Wang, Y. F., Liu, T., Li, X., et al. (2012). Extracellular ATP promotes stomatal opening of Arabidopsis thaliana through heterotrimeric G protein α subunit and reactive oxygen species. Mol. Plant 5 (4), 852–864. doi: 10.1093/mp/ssr095
Haruta, M., Sussman, M. R. (2012). The effect of a genetically reduced plasma membrane protonmotive force on vegetative growth of Arabidopsis thaliana. Plant Physiol. 158, 1158–1171. doi: 10.1104/pp.111.189167
Hedrich, R., Becker, D., Geiger, D., Marten, I., Roelfsema, M. R. G. (2012). “Role of ion channels in plants,” in Patch Clamp Techniques (Tokyo: Springer), 295–322. doi: 10.1007/978-4-431-53993-3_19
Hosy, E., Vavasseur, A., Mouline, K., Dreyer, I., Gaymard, F., Porée, F., et al. (2003). The Arabidopsis outward K+ channel GORK is involved in regulation of stomatal movements and plant transpiration. Proc. Natl. Acad. Sci. U.S.A. 100 (9), 5549–5554. doi: 10.1073/pnas.0733970100
Islam, M. M., Ye, W. X., Matsushima, S., Munemasa, S., Okuma, E., Nakamura, Y., Biswas, M. S., Mano, J., Murata, Y. (2016) Reactive carbonyl species mediate ABA signaling in guard cells. Plant Cell Physiol. 57 (12), 2552–2563. doi: 10.1093/pcp/pcw166
Ivashikina, N., Becker, D., Ache, P., Meyerhoff, O., Felle, H. H., Hedrich, R. (2001). K+ channel profile and electrical properties of Arabidopsis root hairs. FEBS Lett. 508 (3), 463–469. doi: 10.1016/S0014-5793(01)03114-3
Jammes, F., Hu, H. C., Villiers, F., Bouten, R., Kwak, J. M. (2011). Calcium-permeable channels in plant cells. FEBS J. 278 (22), 4262–4276. doi: 10.1111/j.1742-4658.2011.08369.x
Kim, S. Y., Sivaguru, M., Stacey, G. (2006). Extracellular ATP in plants. Visualization, localization, and analysis of physiological significance in growth and signaling. Plant Physiol. 142 (3), 984–992. doi: 10.1104/pp.106.085670
Köhler, C., Merkle, T., Neuhaus, G. (1999). Characterisation of a novel gene family of putative cyclic nucleotide- and calmodulin-regulated ion channels in Arabidopsis thaliana. Plant J. 18 (1), 97–104. doi: 10.1046/j.1365-313X.1999.00422.x
Lang, T., Sun, H., Li, N., Lu, Y., Shen, Z., Jing, X., et al. (2014). Multiple signaling networks of extracellular ATP, hydrogen peroxide, calcium, and nitric oxide in the mediation of root ion fluxes in secretor and non-secretor mangroves under salt stress. Aquat. Bot. 119, 33–43. doi: 10.1016/j.aquabot.2014.06.009
Laohavisit, A., Shang, Z., Rubio, L., Cuin, T. A., Véry, A. A., Wang, A., et al. (2012). Arabidopsis annexin1 mediates the radical-activated plasma membrane Ca2+- and K+-permeable conductance in root cells. Plant Cell 24 (4), 1522–1533. doi: 10.1105/tpc.112.097881
Lew, R. R., Dearnaley, J. D. (2000). Extracellular nucleotide effects on the electrical properties of growing Arabidopsis thaliana root hairs. Plant Sci. 153 (1), 1–6. doi: 10.1016/S0168-9452(99)00242-3
Li, J., Zhang, H., Lei, H., Jin, M., Yue, G., Su, Y. (2016). Functional identification of a GORK potassium channel from the ancient desert shrub Ammopiptanthus mongolicus (Maxim.) Cheng f. Plant Cell Rep. 35 (4), 803–815. doi: 10.1007/s00299-015-1922-6
Luoni, L., Bonza, M. C., De Michelis, M. I. (2000). H+/Ca2+ exchange driven by the plasma membrane Ca2+-ATPase of Arabidopsis thaliana reconstituted in proteoliposomes after calmodulin-affinity purification. FEBS Lett. 482 (3), 225–230. doi: 10.1016/S0014-5793(00)02065-2
Loro, G., Drago, I., Pozzan, T., Schiavo, F., Zottini, M., Costa, A. (2012). Targeting of Cameleons to various subcellular compartments reveals a strict cytoplasmic/mitochondrial Ca2+ handling relationship in plant cells. Plant J. 71 (1), 1–13. doi: 10.1111/j.1365-313X.2012.04968.x
Maierhofer, T., Lind, C., Huttl, S., Scherzer, S., Papenfuss, M., Simon, J., et al. (2014). A single-pore residue renders the Arabidopsis root anion channel SLAH2 highly nitrate selective. Plant Cell 26 (6), 2554–2567. doi: 10.1105/tpc.114.125849
Makavitskaya, M., Svistunenko, D., Navaselsky, I., Hryvusevich, P., Mackievich, V., Rabadanova, C., et al. (2018). Novel roles of ascorbate in plants: induction of cytosolic Ca2+ signals and efflux from cells via anion channels. J. Exp. Bot. 69 (14), 3477–3489. doi: 10.1093/jxb/ery056
Pilot, G., Gaymard, F., Mouline, K., Chérel, I., Sentenac, H. (2003). Regulated expression of Arabidopsis Shaker K+ channel genes involved in K+ uptake and distribution in the plant. Plant Mol. Biol. 51 (5), 773–787. doi: 10.1023/A:1022597102282
Planes, M. D., Niñoles, R., Rubio, L., Bissoli, G., Bueso, E., García-Sánchez, M. J., et al. (2014). A mechanism of growth inhibition by abscisic acid in germinating seeds of Arabidopsis thaliana based on inhibition of plasma membrane H+-ATPase and decreased cytosolic pH, K+, and anions. J. Exp. Bot. 66 (3), 813–825. doi: 10.1093/jxb/eru442
Pottosin, I., Zepeda-Jazo, I., Bose, J., Shabala, S. (2018). An anion conductance, the essential component of the hydroxyl-radical-induced ion current in plant roots. Int. J. Mol. Sci. 19 (3), 897. doi: 10.3390/ijms19030897
Richards, S. L., Wilkins, K. A., Swarbreck, S. M., Anderson, A., Habib, N., McAinsh, M., et al. (2015). The hydroxyl radical in plants: from seed to seed. J. Exp. Bot. 66, 37–46. doi: 10.1093/jxb/eru398
Rodrigo-Moreno, A., Andrés-Colás, N., Poschenrieder, C., Gunsé, B., Peñarrubia, L., Shabala, S. (2013). Calcium- and potassium-permeable plasma membrane transporters are activated by copper in Arabidopsis root tips: linking copper transport with cytosolic hydroxyl radical production. Plant Cell Env. 36, 844–855. doi: 10.1111/pce.12020
Rodrigues, O., Reshetnyak, G., Grondin, A., Saijo, Y., Leonhardt, N., Maurel, C., et al. (2017). Aquaporins facilitate hydrogen peroxide entry into guard cells to mediate ABA- and pathogen-triggered stomatal closure. Proc. Natl. Acad. Sci. U.S.A. 114, 9200–9205. doi: 10.1073/pnas.1704754114
Roux, S. J., Steinebrunner, I. (2007). Extracellular ATP: an unexpected role as a signaler in plants. Trends Plant Sci. 12 (11), 522–527. doi: 10.1016/j.tplants.2007.09.003
Roy, S. J., Gilliham, M., Berger, B., Essah, P. A., Cheffings, C., Miller, A. J., et al. (2008). Investigating glutamate receptor-like gene co-expression in Arabidopsis thaliana. Plant Cell Env. 31 (6), 861–871. doi: 10.1111/j.1365-3040.2008.01801.x
Shabala, S., Shabala, L., Bose, J., Cuin, T., Newman, I. (2013). “Ion Flux Measurements Using the MIFE Technique,” in Plant Mineral Nutrients (Totowa, NJ: Humana Press), 171–183. ISBN 978-1-62703-151-64. doi: 10.1007/978-1-62703-152-3_11
Shang, Z., Laohavisit, A., Davies, J. M. (2009). Extracellular ATP activates an Arabidopsis plasma membrane Ca2+-permeable conductance. Plant Signal. Behav. 4 (10), 989–991. doi: 10.4161/psb.4.10.9680
Shih, H. W., DePew, C. L., Miller, N. D., Monshausen, G. B. (2015). The cyclic nucleotide-gated channel CNGC14 regulates root gravitropism in Arabidopsis thaliana. Curr. Biol. 25 (23), 3119–3125. doi: 10.1016/j.cub.2015.10.025
Song, C. J., Steinebrunner, I., Wang, X., Stout, S. C., Roux, S. J. (2006). Extracellular ATP induces the accumulation of superoxide via NADPH oxidases in Arabidopsis. Plant Physiol. 140 (4), 1222–1232. doi: 10.1104/pp.105.073072
Swarbreck, S., Colaço, R., Davies, J. (2013). Plant calcium-permeable channels. Plant Physiol. 163 (2), 514–522. doi: 10.1104/pp.113.220855
Tanaka, K., Swanson, S. J., Gilroy, S., Stacey, G. (2010). Extracellular nucleotides elicit cytosolic free calcium oscillations in Arabidopsis. Plant Physiol. 154 (2), 705–719. doi: 10.1104/pp.110.162503
Tang, W., Brady, S. R., Sun, Y., Muday, G. K., Roux, S. J. (2003). Extracellular ATP inhibits root gravitropism at concentrations that inhibit polar auxin transport. Plant Physiol. 131 (1), 147–154. doi: 10.1104/pp.013672
Tapken, D., Hollmann, M. (2008). Arabidopsis thaliana glutamate receptor ion channel function demonstrated by ion pore transplantation. J. Mol. Biol. 383 (1), 36–48. doi: 10.1016/j.jmb.2008.06.076
Tavares, B., Dias, P. N., Domingos, P., Moura, T. F., Feijó, J. A., Bicho, A. (2011). Calcium-regulated anion channels in the plasma membrane of Lilium longiflorum pollen protoplasts. New Phytol. 192 (1), 45–60. doi: 10.1111/j.1469-8137.2011.03780.x
Tian, W., Hou, C., Wang, C., Zhao, F., Dahlbeck, D., Hu, S., et al. (2019). A calmodulin-gated calcium channel links pathogen patterns to plant immunity. Nature 572 (7767), 131–135. doi: 10.1038/s41586-019-1413-y
Torres, J., Rivera, A., Clark, G., Roux, S. J. (2008). Participation of extracellular nucleotides in the wound response of Dasycladus vermicularis and Acetabularia acetabulum (Dasycladales, Chlorophyta) 1. J. Phycol. 44 (6), 1504–1511. doi: 10.1111/j.1529-8817.2008.00602.x
Toyota, M., Spencer, D., Sawai-Toyota, S., Jiaqi, W., Zhang, T., Koo, A. J., et al. (2018). Glutamate triggers long-distance, calcium-based plant defense signaling. Science 361 (6407), 1112–1115. doi: 10.1126/science.aat7744
Tyerman, S. D., Beilby, M., Whittington, J., Juswono, U., Newman, I., Shabala, S. (2001). Oscillations in proton transport revealed from simultaneous measurements of net current and net proton fluxes from isolated root protoplasts: MIFE meets patch clamp. Aust. J. Plant Physiol. 28, 591–604. doi: 10.1071/PP01030
Van Kleeff, P. J. M., Gao, J., Mol, S., Zwart, N., Zhang, H., Li, K. W., et al. (2018). The Arabidopsis GORK K+-channel is phosphorylated by calcium-dependent protein kinase 21 (CPK21), which in turn is activated by 14-3-3 proteins. Plant Physiol. Biochem. 125, 219–231. doi: 10.1016/j.plaphy.2018.02.013
Véry, A. A., Davies, J. M. (2000). Hyperpolarization-activated calcium channels at the tip of Arabidopsis root hairs. Proc. Natl. Acad. Sci. U.S.A. 97 (17), 9801–9806. doi: 10.1073/pnas.160250397
Vincent, T. R., Avramova, M., Canham, J., Higgins, P., Bilkey, N., Mugford, S. T., et al. (2017). Interplay of plasma membrane and vacuolar ion channels, together with BAK1, elicits rapid cytosolic calcium elevations in Arabidopsis during aphid feeding. Plant Cell 29 (6), 1460–1479. doi: 10.1105/tpc.17.00136
Wang, L., Guo, M. Y., Thibaud, J. B., Véry, A. A., Sentenac, H. (2019). A repertoire of cationic and anionic conductances at the plasma membrane of Medicago truncatula root hairs. Plant J. 98 (3), 418–433. doi: 10.1111/tpj.14238
Wang, F., Jia, J., Wang, Y., Wang, W., Chen, Y., Liu, T., et al. (2014). Hyperpolarization-activated Ca2+ channels in guard cell plasma membrane are involved in extracellular ATP-promoted stomatal opening in Vicia faba. J. Plant Physiol. 171 (14), 1241–1247. doi: 10.1016/j.jplph.2014.05.007
Wang, Y. F., Munemasa, S., Nishimura, N., Ren, H. M., Robert, N., Han, M., et al. (2013). Identification of cyclic GMP-activated non-selective Ca2+-permeable cation channels and associated CNGC5 and CNGC6 genes in Arabidopsis guard cells. Plant Physiol. 163, 578–590. doi: 10.1104/pp.113.225045
Wang, L., Wikins, K., Davies, J. M. (2018). Arabidopsis DORN1 extracellular ATP receptor; activation of plasma membrane K+-and Ca2+-permeable conductances. New Phytol. 218, 1301–1304. doi: 10.1111/nph.15111
Wilkins, K. A., Matthus, E., Swarbreck, S. M., Davies, J. M. (2016). Calcium-mediated abiotic stress signaling in roots. Front. Plant Sci. 7, 1296. doi: 10.3389/fpls.2016.01296
Wu, S. J., Liu, Y. S., Wu, J. Y. (2008). The signaling role of extracellular ATP and its dependence on Ca2+ flux in elicitation of Salvia miltiorrhiza hairy root cultures. Plant Cell Physiol. 49 (4), 617–624. doi: 10.1093/pcp/pcn033
Wu, Y., Qin, B., Feng, K., Yan, R., Kang, E., Liu, T., et al. (2018). Extracellular ATP promoted pollen germination and tube growth of Nicotiana tabacum through promoting K+ and Ca2+ absorption. Plant Reprod. 31 (4), 399–410. doi: 10.1007/s00497-018-0341-6
Yang, X., Wang, B., Farris, B., Clark, G., Roux, S. J. (2015). Modulation of root skewing in Arabidopsis by apyrases and extracellular ATP. Plant Cell Physiol. 56 (11), 2197–2206. doi: 10.1093/pcp/pcv134
Zhang, W. H., Ryan, P. R., Sasaki, T., Yamamoto, Y., Sullivan, W., Tyerman, S. D. (2008). Characterization of the TaALMT1 protein as an Al3+-activated anion channel in transformed tobacco (Nicotiana tabacum L.) cells. Plant Cell Physiol. 49 (9), 1316–1330. doi: 10.1093/pcp/pcn107
Zhao, N., Wang, S., Ma, X., Zhu, H., Sa, G., Sun, J., et al. (2016). Extracellular ATP mediates cellular K+/Na+ homeostasis in two contrasting poplar species under NaCl stress. Trees 30 (3), 825–837. doi: 10.1007/s00468-015-1324-y
Keywords: ATP, anion, channel, DORN1, P2K1, root epidermis, ROS
Citation: Wang L, Stacey G, Leblanc-Fournier N, Legué V, Moulia B and Davies JM (2019) Early Extracellular ATP Signaling in Arabidopsis Root Epidermis: A Multi-Conductance Process. Front. Plant Sci. 10:1064. doi: 10.3389/fpls.2019.01064
Received: 05 March 2019; Accepted: 06 August 2019;
Published: 04 September 2019.
Edited by:
Sebastien Thomine, Centre National de la Recherche Scientifique (CNRS), FranceReviewed by:
Yoshiyuki Murata, Okayama University, JapanFouad Lemtiri-Chlieh, University of Connecticut, United States
Copyright © 2019 Wang, Stacey, Leblanc-Fournier, Legué, Moulia and Davies. This is an open-access article distributed under the terms of the Creative Commons Attribution License (CC BY). The use, distribution or reproduction in other forums is permitted, provided the original author(s) and the copyright owner(s) are credited and that the original publication in this journal is cited, in accordance with accepted academic practice. No use, distribution or reproduction is permitted which does not comply with these terms.
*Correspondence: Julia M. Davies, jmd32@cam.ac.uk