- 1CAS Key Laboratory of Forest Ecology and Management, Institute of Applied Ecology, Chinese Academy of Sciences, Shenyang, China
- 2Qingyuan Forest CERN, Shenyang, China
- 3School of Resources and Civil Engineering, Northeastern University, Shenyang, China
- 4Earth Systems Research Center, Morse Hall, University of New Hampshire, Durham, NH, United States
- 5Center for Ecological Research, Kyoto University, Shiga, Japan
- 6Institute of Surface-Earth System Science, Tianjin University, Tianjin, China
- 7College of Resources and Environment, University of Chinese Academy of Sciences, Beijing, China
- 8School of Life Sciences, Henan University, Kaifeng, China
Fundamental questions of how plant species within secondary forests and plantations in northeast China partition limited nitrogen (N) resource remain unclear. Here we conducted a 15N tracer greenhouse study to determine glycine, ammonium, and nitrate uptake by the seedlings of two coniferous species, Pinus koraiensis (Pinus) and Larix keampferi (Larix), and two broadleaf species, Quercus mongolica (Quercus) and Juglans mandshurica (Juglans), that are common in natural secondary forests in northeast China. Glycine contributed 43% to total N uptake of Pinus, but only 20, 11, and 21% to N uptake by Larix, Quercus, and Juglans, respectively (whole plant), whereas nitrate uptake was 24, 74, 88, and 68% of total uptake for these four species, respectively. Retention of glycine carbon versus nitrogen in Pinus roots indicated that 36% of glycine uptake was retained intact. Nitrate was preferentially used by Larix, Quercus, and Juglans, with nitrate uptake constituting 68∼88% of total N use by these three species. These results demonstrated that these dominant tree species in secondary forests in northeast China partitioned limited N resource by varying uptake of glycine, ammonium and nitrate, with all species, except Pinus, using nitrate that are most abundant within these soils. Such N use pattern may also provide potential underlying mechanisms for the higher retention of deposited nitrate than ammonium into aboveground biomass in these secondary forests.
Introduction
In Northeast China, natural secondary forests (NSFs) account for as much as 70% of the regional forests (Hao et al., 2000). They are formed through natural regeneration of primary forests following afforestation (Zhu and Liu, 2007). Since the 1950s, large areas of the NSFs have been turned into larch plantations for fast timber production (Wang et al., 2006), resulting in mosaic plantation/secondary forest landscapes. These two forest types are common in China and are regionally important in providing ecosystem services of regulating regional climate. However, several problems have emerged, including soil acidification and nutrient leaching from these disturbed forest sites (Liu et al., 1998; Yang et al., 2010). Relatively lower soil nutrient concentrations, especially available nitrogen, in these secondary forests and larch plantations compared to those well-developed and less-disturbed forests may constrain tree growth (Yang et al., 2012). However, fundamental questions of how plant species within these forests partition and compete for limited soil N resource remain unclear.
A complete understanding of forest N cycling requires that we understand how much N plants take up, and which form of inorganic or organic N they assimilate. Soil N occurs in a variety of organic and inorganic forms that are differentially available to plant roots. Complex organic polymers in litter are depolymerized into bioavailable, N-containing monomers (such as amino acids, Schimel and Bennett, 2004) that are then mineralized by microorganisms, producing ammonium () and nitrate (). Plant species differ in their capacity to take up ammonium, nitrate or organic N as their primary N source partly because of physiological trade-offs in taking up different N forms (Stewart et al., 1992). While nitrate is readily available due to its high solubility in soil water, plants must use energy to reduce nitrate before incorporation into amino acids. Thus, it may be more efficient for plants to take up ammonium because it can be immediately incorporated into amino acids. However, ammonium is not always available for uptake due to its strong adsorption to soil exchange sites and poor mobility in soil solution. Free amino acids, which are found in large quantities in some ecosystems, could also be a major N source for plant due to their ready incorporation into plant protein (Persson and Näsholm, 2001; Näsholm et al., 2009). On the other hand, nitrate is often faster transported out of roots than amino acids or ammonium, bypassing the reducing and assimilation steps in the roots (Persson et al., 2006; Scott and Rothstein, 2011).
In addition, plants differ in their N form preference. For example, early and late successional tree species had different uptake rates for nitrate versus ammonium, respectively, which were related to the availability of these N forms along successional gradients (Kronzucker et al., 1997). Conifer trees may not grow well in disturbed sites because of their preference for ammonium and their poor ability to use nitrate that often dominates disturbed sites (Ingestad, 1979; Kronzucker et al., 1997). In N-limited arctic and alpine tundra plant communities, species composition correlated with the partitioning of differentially available forms of N, with dominance being linked to the ability of a species to exploit the most abundant N form (McKane et al., 2002; Ashton et al., 2010). On the other hand, plants are often flexible in their capacity to take up the chemical forms of N that are most readily available (Scott and Rothstein, 2011). For example, based on 15N natural abundance in vegetation and soils, tree species in Hawaiian tropical forests along a rainfall gradient did not specialize on different soil N pools, but rather responded to changes in soil N availability to exploit the most abundant N form (Houlton et al., 2007). In the tropical montane forest in Panama, 15N tracer studies indicated flexibility in uptake of N forms in tropical plants across root trait groups, with only a few species (5 out of 11) weakly preferring specific N forms (Andersen et al., 2017). Whether plants within forests prefer specific N forms or are plastic in their response is important to understand because variability in N uptake implies variability in N retention within forested watersheds.
Most previous studies on uptake of different forms of N by temperate tree species used hydroponic experiments, in which either intact or excised roots were submerged in 15N tracer solutions and the 15N enrichment within roots determined after several hours (Warren and Adams, 2007; Liu et al., 2017, references cited therein). These studies demonstrated the inherent uptake capacity of tree species for different N forms, reflecting the functional niche of specific species within an ecosystem (McKane et al., 2002). Using this method, Liu et al. (2017) demonstrated that tree species in temperate forest in Donglingshan, China, prefer ammonium, with ammonium uptake accounting for 76% of the total N uptake of these tree species, including Juglans mandshurica Maxim. Other studies directly labeled the soil in pots (Templer and Dawson, 2004) or in the field (McKane et al., 2002; Persson et al., 2003; Gallet-Budynek et al., 2009; Andersen et al., 2017) and studied 15N incorporation into whole plants. In these studies, plants either preferred different N forms, with dominant plants using the most available forms of N within local soil (McKane et al., 2002; Templer and Dawson, 2004; Gallet-Budynek et al., 2009), or plants took up all N provided, regardless of N form (Persson et al., 2003; Andersen et al., 2017).
In this study, we selected two coniferous species, Pinus koraiensis and Larix keampferi and two broadleaf species, Quercus mongolica and Juglans mandshurica, that are common in NSFs and larch plantations in northeast China to study their uptake of different N forms. We used both 15N natural abundance (δ15N) methods and a 15N tracer experiment with ammonium, nitrate and glycine to determine if these tree species show preference or flexibility in N use. Glycine is the most frequently used amino acid in field studies of plant uptake of amino acids (Jones et al., 2005) because of its high abundance in forest soils (Finzi and Berthrong, 2005; Berthrong and Finzi, 2006; Liu et al., 2017), mobility in the soil (Owen and Jones, 2001) and poor substrate quality for microbial growth (Lipson et al., 1999). We, on one hand, used isotope mixing models to calculate the contributions of soil dissolved organic N (DON), ammonium and nitrate to plant N use by these four tree species based on the δ15N values of different soil N pools and δ15N signature of plant leaves (Houlton et al., 2007; Takebayashi et al., 2010; Liu et al., 2018). In addition, we labeled tree seedlings of these four species growing in pots in greenhouses with 15N tracer to determine short-term uptake and assimilation pattern of glycine, ammonium, and nitrate. We hypothesized that (1) the two coniferous species Pinus koraiensis and Larix keampferi will prefer ammonium or glycine to nitrate (Kronzucker et al., 1997); (2) the two broadleaf tree species Quercus mongolica and Juglans mandshurica will prefer nitrate since nitrate is often the dominant inorganic N form in disturbed forest sites (Hope et al., 2003); and (3) the N uptake patterns of these tree species should be related to the availability of these N forms in their natural habitat.
Materials and Methods
Species Selection and Seedling Preparation
We selected four common tree species in the NSF near Qingyuan Experimental Station of Forest Ecology of the Institute of Applied Ecology, Chinese Academy of Sciences, which is located in a mountainous region in the eastern Liaoning Province, northeast China (41°51′ N, 124°54′ E, elevation 500–1100 m above sea level). These species include two coniferous species, Pinus koraiensis and Larix keampferi, and two broadleaf species, Quercus mongolica and Juglans mandshurica, which make up 5, 30, 8, and 27% of the mature tree composition in this forest, respectively (forest survey, Li et al., unpublished data). These four species are known to be associated with different mycorrhizal types, with Juglans mandshurica associated with arbuscular mycorrhizal fungi, with the three other species, Pinus koraiensis, Larix keampferi, and Quercus mongolica all being ectomycorrhizal type (Guo et al., 2008; Liu et al., 2017). The continental monsoon climate of the region has a humid and rainy summer and a cold and snowy winter. Mean annual air temperature varies between 3.9 and 5.4°C and ranges from -37.6 to 36.5°C. The mean annual precipitation ranges between 700 and 850 mm. 80% of the rain falls in June, July, and August. The current wet N deposition rate in this forest is 19 kg N ha-1 yr-1 (mean value during 2014 to 2017), and the ratio of ammonium to nitrate is 2:1 (Huang et al., 2019). The soil type is a typical brown forest soil with a thickness of 60–80 cm.
One-year old seedlings of these four species were purchased from a local nursery and transplanted to the greenhouse in Yunong Campus, Institute of Applied Ecology, Chinese Academy of Sciences (41°90′ N, 123°58′ E). Soils for transplanting these seedlings were topsoils from low hills adjacent to the greenhouse, which are dominated by Pinus koraiensis, Ulmus laciniata, and were classified as brown forest soils, with pH of 6. The seedlings were transplanted individually to each pot (15.3 L). All pots received natural light levels and were watered every 3 days with tap water to keep the soil surface moist. The average high temperature in the greenhouse during the experiment was 26.5°C and the average low temperature was 18.3°C. Pots of all seedlings were arranged randomly and were moved within the greenhouse regularly to reduce the effects of environmental variation. These seedlings were acclimated to greenhouse conditions for 1 year prior to the 15N labeling experiment. No nutrients were added to the pots during this 1 year acclimation.
15N Labeling Experiment
Most leaves reached full expansion by middle May. We labeled each individual seedling with 15N tracers in July 2018. For 15N labeling, we randomly allocated four individuals of each species to one of the three N species treatments (U-13C2, 15N-glycine, 15NH4Cl, or Na15NO3). Seedlings received 10.17 mg 15N per individual (100 mg 15N m-2, equal to 0.34 μg 15N g-1) dissolved in 20 mL deionized water containing equimolar concentrations of nitrate, ammonium, and glycine, with only one N form labeled. The total amount of N (including 15N) added to each plant individual was 1.02 μg N g-1soil, with 1/3 15N, representing a roughly 16∼37% increase in DIN (ammonium+nitrate) and a 8∼14% increase in total N. The organic N source was provided as U-13C2, 15N-glycine (both 13C and 15N at 99 atom%), while ammonium and nitrate were supplied as 15NH4Cl (99.08 atom%) and Na15NO3 (99.26 atom%). We could then test for uptake preferences of each of the labeled N forms by individual plant species and also test the proportion of glycine taken up directly by plants, as evidenced by the relative enrichment of plant tissue with 13C and 15N, or as mineral N after microbial mineralization (Bardgett et al., 2003). The nitrification inhibitor dicyandiamide (DCD) was added at 50 μg g-1 to all three 15N labeling solutions to prevent potential nitrification of ammonium. In a preliminary experiment, 50 μg g-1 of the nitrification inhibitor DCD effectively inhibited nitrification over 48 h under Pinus soil (data not shown). A control group with no added N provided background 15N values for 15N uptake rates calculations.
We removed any leaf litter within the surface of the pots and injected nitrogen tracer solutions into the soil at four locations (5 ml for each) equally spaced around, and approximately 3 cm from, the stem of each seedling to a depth of 10 cm using a syringe and needle with four lateral holes. After 24 and 48 h, seedlings and intact root systems were harvested and bulk soil surrounding each seedling (∼3 cm radius or within the 15N-label zone) was collected for analysis. Seedlings were immediately rinsed, separated into coarse roots (>2 mm), fine roots (<2 mm), stems, and leaves, dried at 60°C for 48 h, weighed, and ground for 15N and 13C isotope analyses by elemental analyzer-isotope ratio mass spectrometry (Elementar AnalysenSysteme GmbH, Germany; IsoPrime100, IsoPrime Limited, United Kingdom). Four compounds were used as references: L-histidine, D-glutamic acid, glycine, and acetanilide. The analytical precision for δ15N and δ13C were both 0.2‰. Whole plant δ15N values were calculated from mass-weighted δ15N values of each plant organ.
15N atom% excess (APE) was calculated as the difference in atom% 15N between 15N-labeled plant organs and unlabeled (control) organs. 15N uptake rates (μg 15N g-1 dry mass h-1) were calculated by multiplying plant organ N content (μg g-1 plant organ biomass) by the corresponding (APE/100), divided by labeling time in hours and (atom% 15N/100) of the applied 15N-labeled N form (99 atom% for U-13C2, 15N-glycine, 99.08 atom% for 15NH4Cl, 99.26 atom% for Na15NO3) as follows: (N content (μg g-1 plant organ biomass) × APE/100)/(time (h) × (at% 15N tracer/100)) (Liu et al., 2017). Uptake rates of each N form were normalized to the sum of uptake rates of the three N forms to calculate its contribution to total N uptake or assimilation. Here we only report results of 48 h after N tracer addition, since preliminary results showed 48 h to be necessary to detect obvious 15N enrichment in plant leaves (data not shown).
Soil Chemical and Isotopic Analyses
Total dissolved N (TDN), ammonium, and nitrate were immediately extracted within 4 h of sampling in 50 mL of 2 mol/L KCl on a shaker table for 1 h, filtered through Whatman GF/A filters (Whatman PLC, Maidstone, United Kingdom), and frozen at -36°C until colorimetric determination of nitrate+nitrite and ammonium using a continuous chemical analyzer (SmartChem 200, Rome, Italy). Gravimetric soil water content was 66 ± 9.7% on average at the time of harvest. Total dissolved N in KCl extracts was determined by alkaline persulfate digestion (Cabrera and Beare, 1993; Doyle et al., 2004) and nitrate detection by continuous chemical analyzer (SmartChem 200, Rome, Italy).
The δ15N measurements of actual and derived nitrate samples were then made using the modified azide method (Tu et al., 2016). Methodological details, including standard preparation, were detailed previously (Tu et al., 2016). The δ15N values of KCl-extracted ammonium were analyzed using the acidified disk diffusion method to isolate ammonium from the remainder of the KCl-extracted TDN (Stephan and Kavanagh, 2009). The δ15N value of dissolved organic N (δ15NDON) was calculated as the mass-weighted difference between the concentrations of KCl-extracted TDN, ammonium, and nitrate, and the δ15N values obtained from the modified azide method (oxidized TDN and actual nitrate) or diffusion (ammonium) methods using the following equation: δ15NDON = (δ15NTDN × [TDN] - (δ15Nammonium × [ammonium] + δ15Nnitrate × [nitrate]))/[DON].
15N Natural Abundance Method
We used δ15N values of soil N (DON, ammonium, and nitrate) in control pots and δ15N values of the leaves in a Bayesian isotope-mixing model [Stable Isotope Analysis in R (SIAR)] to estimate the proportional contributions (f, %) of the three N forms (DON, ammonium, and nitrate) to total N of each species. The SIAR model uses a Bayesian framework to establish a logical prior distribution (60) for estimating f values, and then determines the probability distribution for the f values of each soil N source (fDON, fammonium and fnitrate, presented here as the average probability) to the mixture (δ15Nleaves, in this study). The mixing model considers isotope effects during plant N uptake (15𝜀 values hereafter). We used the normalized 15𝜀 values for worldwide plants associated with ectomycorrhizae (ECM) and arbuscular mycorrhizae (AM) (-3.2 and -2.0‰, respectively; Craine et al., 2009) to correct the δ15Nleaves value:
1 = fDON + fammonium+ fnitrate;
∗δ15Nleaves = δ15Nleaves + 3.2‰ (for ECM species Pinus, Larix, and Quercus);
∗δ15Nleaves = δ15Nleaves + 2‰ (for AM species Juglans);
∗δ15Nleaves = δ15NDON × fDON + δ15Nammonium × fammonium + δ15Nnitrate ×fnitrate.
The differences in 15N uptake rates among the three labeled N forms for each species were tested through one-way analysis of variance. P-values < 0.05 were considered to indicate a significant difference among means. Analyses were followed by Tukey’s b post hoc test. All statistical analyses were conducted using SPSS 19.0 (SPSS, Inc., Chicago, IL, United States).
Results
15N Natural Abundance and N Source Partitioning
Before 15N tracer addition, plant organs of the four species had δ15N values ranging from -3.1 to -0.5‰, with N concentrations ranging from 0.4 to 3.6% (Table 1). The calculated whole plant δ15N values ranged from -3.0‰ for Larix to -1.1‰ for Quercus (Table 1), with Quercus having the highest δ15N values of leaf, stem, coarse, and fine roots (Table 1). δ15N of soil N pools, on the other hand, ranged from 5.0 to 7.2‰ for DON, 3.1 to 15.7‰ for ammonium, and -5.2 to -2.1‰ for nitrate (Figure 1A). DON was the dominant N form within these soils (Table 2), with concentration ranging from 4.4 ± 1.4 to 7.0 ± 0.7 μg g-1, followed by nitrate (1.5 ± 0.2 to 3.5 ± 1.3 μg g-1) and ammonium (1.2 ± 0.1 to 2.5 ± 0.4 μg g-1). The nitrate : ammonium ratios varied between 1.3 ± 0.2 and 2.1 ± 0.4 (Table 2). Soils planted with Quercus had the lowest N availability (DON + ammonium + nitrate) after 1 year growth (Table 2), but had highest δ15N values of leaf, stem, coarse roots, and fine roots (Table 1).
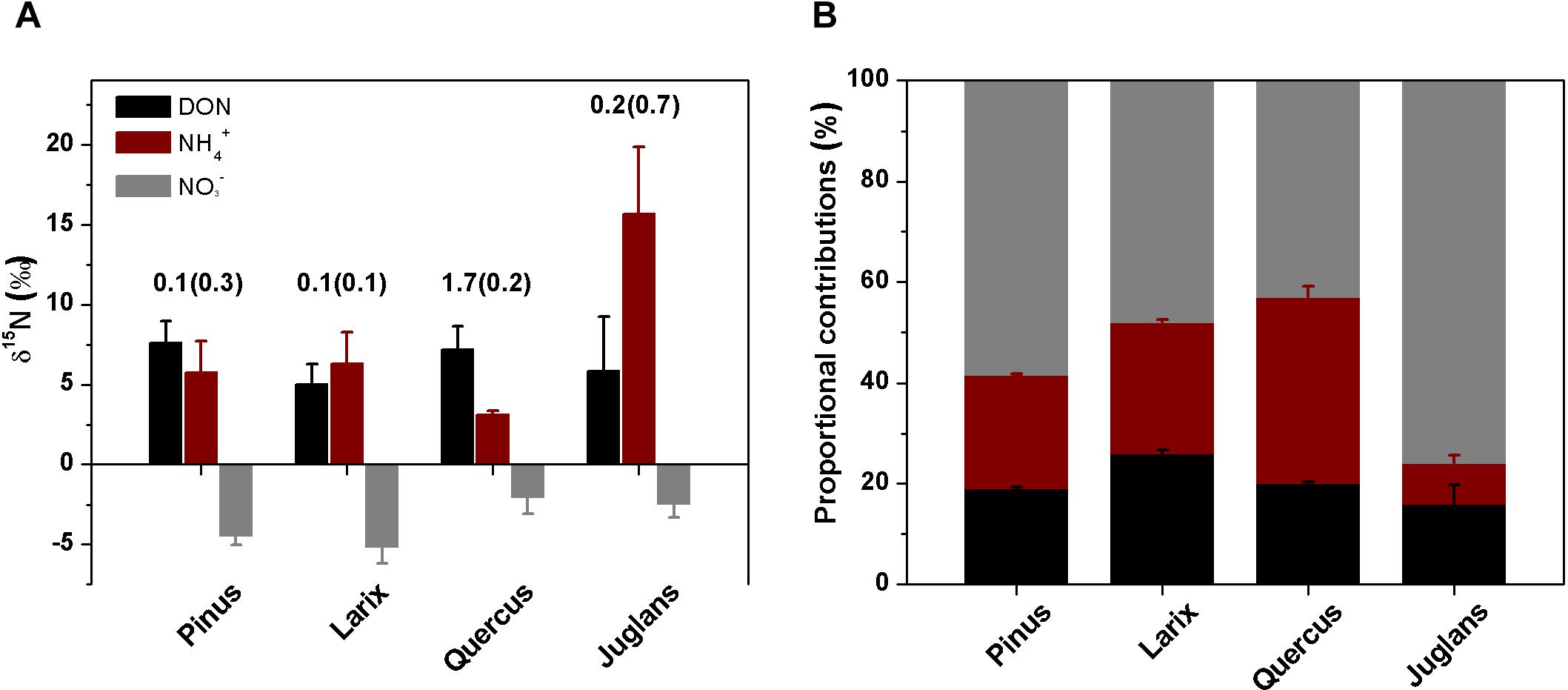
Figure 1. δ15N values (A, ‰) of soil DON, ammonium, nitrate (bars, means ± SE) and of leaves after corrected for the 15𝜀 (numbers above the bars) and the estimated proportional contributions (B, %, means ± SD) of the three N forms to plant N nutrition of the four taxa Pinus, Larix, Quercus, and Juglans using an isotope mixing model (see section “Materials and Methods”).

Table 2. Soil pH, dissolved organic nitrogen (DON), ammonium, nitrate, the nitrate to ammonium ratio, plant dry weights and root : shoot ratios of seedlings of the four common tree species in northeast China after 1 year of pot growth.
Bayesian isotope-mixing model estimates using natural δ15N values of soil N (DON, ammonium, and nitrate) in control soils and δ15N values of the leaves after correction of the fractionation factor (15𝜀) showed that soil nitrate was the major N source for all species, with fnitrate values ranging from 43 to 76% (Figure 1B), followed by DON (fDON, 16% ∼26%) and ammonium (fammonium, 7 ∼ 37%, Figure 1B).
15N, 13C Uptake and Assimilation
All four species could access N from the three chemical forms supplied. At 24 h after 15N addition, Pinus and Larix varied widely in 15N uptake by coarse and fine roots, and only assimilated small amounts of 15N in leaves, which limited our ability to detect N uptake preference in these two species (Supplementary Figure S1). After 48 h of 15N tracer addition, plant organs of Larix, Quercus, and Juglans receiving 15 treatment had the highest 15N uptake rates, followed by those receiving U-13C2, 15N-glycine. Across all plant species and plant organs, 15N uptake rates for all three N forms ranged from 0.001∼0.8 μg 15N g-1h-1, with the highest being the uptake of nitrate by Quercus fine roots (0.8 ± 0.2 μg 15N g-1h-1), and the lowest being the assimilation of ammonium to Quercus stems (0.001 ± 0.001 μg 15N g-1h-1; Figure 2C). Nitrate uptake rates, at 0.006 ∼ 0.8 μg 15N g-1h-1, were 2 ∼ 12 times that of glycine (0.002 ∼ 0.12 μg 15N g-1h-1) and 8 ∼ 85 times that of ammonium (0.001∼0.04 μg 15N g-1h-1). Quercus had significantly higher uptake and assimilation rates for nitrate than for glycine or ammonium (P < 0.001 for all plant organs and whole plants; Figure 2C).
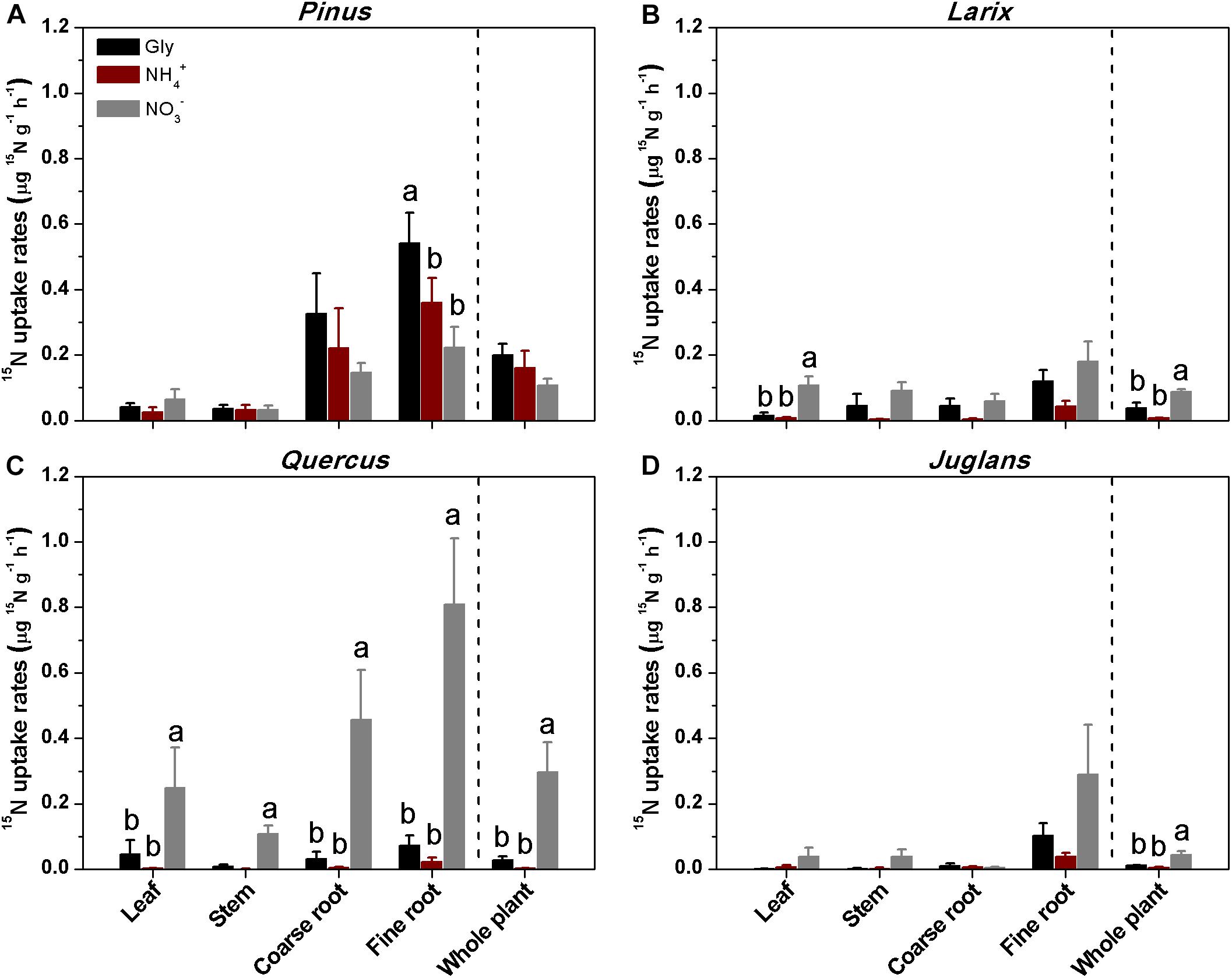
Figure 2. Mean ( ± 1 SE, n = 4) uptake rates of 15N by different plant organs and whole plants of the four taxa (A–D) at 48 h after soil injection of 15N as glycine, ammonium, or nitrate. Different letters above the bars indicate significant differences in uptake rates between N forms for each species (one-way ANOVA, P < 0.05).
In contrast to the other three species, Pinus fine roots incorporated most 15N from glycine (0.54 ± 0.1 μg 15N g-1h-1), followed by ammonium and nitrate (0.36 ± 0.07 and 0.23 ± 0.06 μg 15N g-1h-1, respectively; P < 0.01; Figure 2A). Corresponding to its higher uptake of dual-labeled glycine (U-13C2, 15N-glycine), the coarse and fine roots of Pinus were associated with significantly higher δ13C values (P < 0.05 for both; Figure 3A). The correlation between the 15N excess (y) and the 13C excess (x) under U-13C2, 15N-glycine treatment had a slope of 0.71 (y = 0.71x + 0.45, r2 = 0.96, P < 0.001) at 48 h after 15N tracer addition, indicating that 36% of glycine was derived from intact uptake by Pinus roots (Figure 4). In addition, the coarse roots, fine roots, and whole plant of Juglans were significantly enriched in 13C following U-13C2, 15N-glycine treatment (Figures 3B–D), but the 15N excess (y) and the13C excess of coarse and fine roots of this species were not significantly correlated (data not shown).
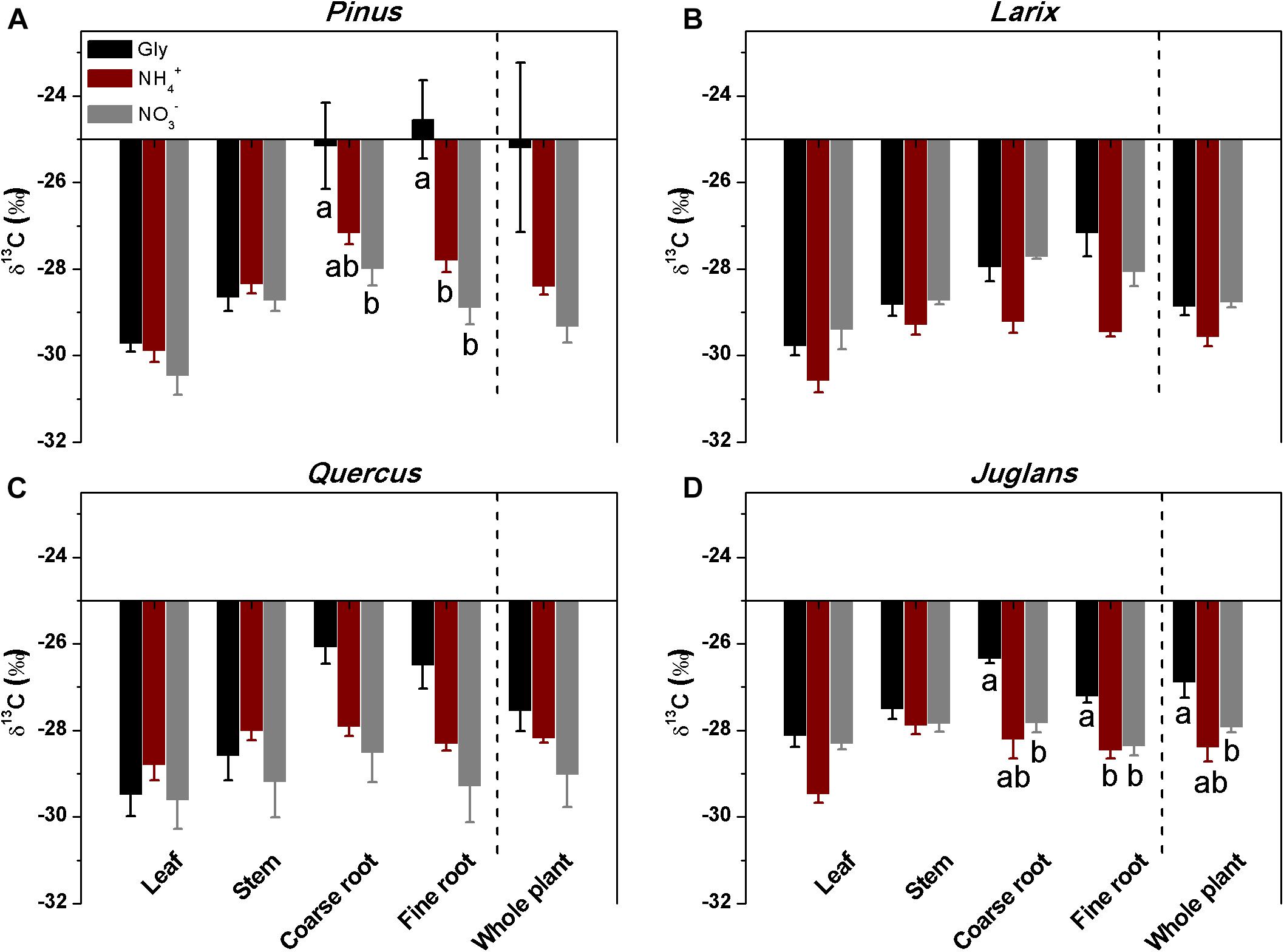
Figure 3. Stable carbon isotope composition (δ13C) of different plant organs of the four studied species (A–D) that had received mixtures of glycine, ammonium and nitrate, with a single form isotopically labeled. The δ13C of the whole plant was calculated from mass-weighted δ13C of plant organs (Means ± SE, n = 4). Different letters below the bars indicate significant differences in δ13C between N forms for each species (one-way ANOVA, P < 0.05).
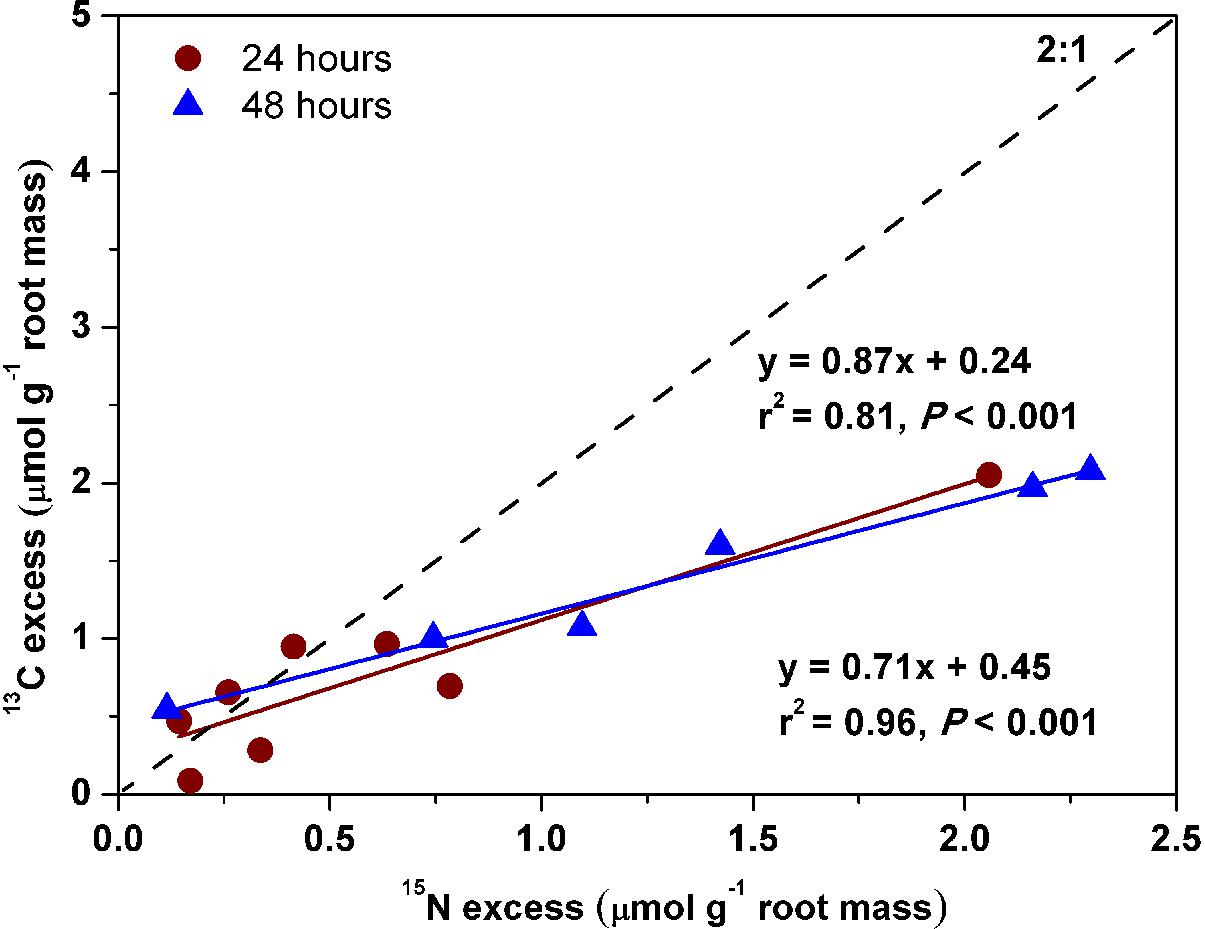
Figure 4. Relationships of 15N excess with 13C excess in coarse and fine roots of Pinus koraiensis that had received U-13C2, 15N-glycine treatment after 24 and 48 h, respectively. The relationships fitted were: 13C excess = 0.87 × 15N excess + 0.24, r2 = 0.81, P < 0.001 at 24 h and 13C excess = 0.71 ×15N excess + 0.45, r2 = 0.96, P < 0.001 at 48 h. The expected 2:1 relationship was shown.
Overall, for the whole plant, the relative uptake proportion for the three N forms was glycine > ammonium > nitrate (P < 0.05) for Pinus, and nitrate > glycine > ammonium (P < 0.001) for Larix, Quercus, and Juglans (Figure 5). For Pinus, uptake of glycine, ammonium, and nitrate accounted for 43, 33, and 24% of total N uptake, respectively (sum of uptake of the three N forms, calculated with whole plant value) (Figure 5A). In contrast, nitrate uptake contributed 74, 88, and 68% and glycine contributed 20, 11, and 21% to total N uptake by Larix, Quercus, and Juglans, respectively (whole plant values, Figures 5B–D).
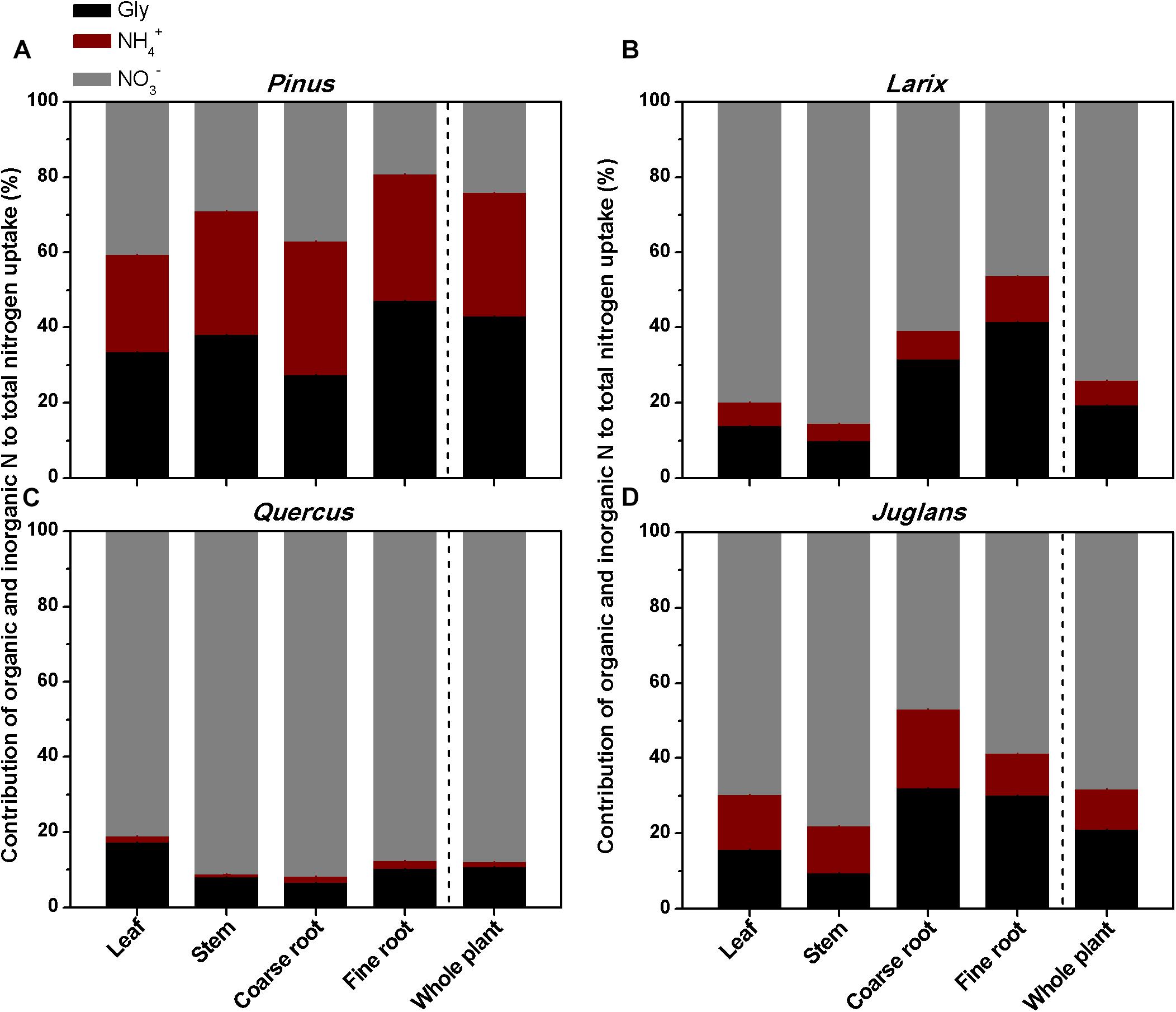
Figure 5. The contribution of glycine, ammonium, and nitrate to total N uptake by different plant organs (leaf, stem, coarse root, fine roots) and whole plant of the four tree species (A–D) estimated from the 15N labeling experiment.
Discussion
Nutrient acquisition strategies incorporate nutrient uptake rates, mycorrhizal associations, nutrient requirements, and preferences for chemical forms of N, which are analogous to above-ground economic strategies (Wright et al., 2004). In this study, we tracked plant N uptake rates for three N forms from the short-term incorporation of 15N-labeled glycine, ammonium, or nitrate by four tree species common in secondary forests and larch plantations in northeast China. These species exhibited different preferences for different N forms, with Pinus predominantly accumulating glycine into fine roots and coarse roots, followed by ammonium, while the other three species primarily used nitrate, followed by glycine. These results partly supported our hypothesis, suggesting that tree species within these secondary forests and larch plantations partition N through preferential uptake of different N forms. Nitrate was the most available N form in these sites and was preferred by all species except Pinus.
Nevertheless, given that this experiment was done in pots and trees were kept in a greenhouse for a year prior to the study, these results may not reflect what would occur in the field. Being a pot study in greenhouses, the soil within pots might be warmer than field soils, which may enhance both N mineralization and nitrification processes, with the net effects on soil ammonium and nitrate availability not straightforward. Despite this, all species were exposed to the same conditions, so the differences among species are noteworthy.
Moreover, across all four species and all plant organs, the 15N uptake rates ranged from 0.001∼0.8 μg 15N g-1h-1. These uptake rates, however, were low comparing with those by plant species from temperate forests in China (root uptake rates, 0.8∼12.8 μg 15N g-1h-1 in Liu et al., 2017), in a wet temperate forest in Tasmania (root uptake rates, 13.5∼173 μg g-1h-1; Warren and Adams, 2007), and from temperate deciduous forest in eastern North America (22.5 ∼ 255 μg 15N g-1 fine root h-1; Scott and Rothstein, 2011), all of which used hydroponic techniques. Thus these differences might be related to the different labeling methods used by this and other studies. In this study soils within the pots growing tree seedlings were labeled and the whole plants were harvested. Under such conditions, fine roots get access to the N after interceptions by soil microbes and soil abiotic reactions. In contrast, in the hydroponic experiments, where excised or intact roots were subjected to 15N labeling solutions to study 15N uptake, adsorption by soil particles or soil microbes was minimized.
Pinus Roots Uptake of Glycine
All four species studied could take up organic N, in the form of glycine, as has been shown for other tree species (Schmidt and Stewart, 1999; Öhlund and Näsholm, 2001; Finzi and Berthrong, 2005). Pinus koraiensis seedlings assimilated more glycine than the other species, with glycine contributing 43% to total plant uptake by this species (whole plant value). The overall uptake pattern in Pinus was glycine > ammonium > nitrate. Our results agree with studies on Pinus sylvestris L. and Picea abies L. seedlings growing in pots (peat as medium) showing similar uptake rates of glycine, arginine and ammonium, being 7 to 8 times higher than uptake rates for nitrate (Öhlund and Näsholm, 2001). The relatively high Pinus koraiensis fine roots and coarse roots uptake of glycine and ammonium reflected the possibly higher numbers of the transporter proteins for ammonium than for nitrate in the plasma membrane of conifer roots (Kronzucker et al., 1997).
The linear relationship of 13C excess with 15N excess in Pinus (Figure 4; Näsholm and Persson, 2001; Warren and Adams, 2007) indicated that roughly 36% of glycine-N was taken up intact. In this study, the slope of the relationship was 0.71 after 48 h of 15N tracer addition, which is less than the expected slope of 2.0, indicating that intact uptake could have been underestimated because post-uptake metabolism of glycine can lead to loss of 13C (Warren and Adams, 2007). For example, Hobbie and Hobbie (2012) reported that 25∼38% of added glycine was respired in soils and planktonic systems. Thus, we conclude that the Pinus koraiensis seedlings preferentially use glycine and ammonium over nitrate under greenhouse conditions.
We note that we cannot determine the actual chemical form of N that the plant roots took up, nor whether N was directly taken up by plant roots or via mycorrhizal hyphae. Nitrogen transformations can be rapid in temperate forests (Finzi and Berthrong, 2005) and microbially mediated N uptake may have resulted in plants taking up a chemical form of N different from the original 15N source. Half-lives of amino acids in temperate soils vary from < 2 to 29 h (Lipson and Näsholm, 2001). Finzi and Berthrong (2005), who examined the cycling of 15N-enriched glycine in temperate forest soils in Connecticut, United States, observed rapid immobilization and mineralization of glycine to ammonium by soil microbes, with substantial subsequent nitrification. They considered that sampling at 2 and 5 h following isotope addition might be suitable to assess intact uptake (Finzi and Berthrong, 2005; Gallet-Budynek et al., 2009). Thus, given the rapid turnover time of amino acids in soil (Jones and Hodge, 1999; Finzi and Berthrong, 2005), our sampling time of 48 h may have allowed some glycine to be transformed to ammonium prior to Pinus uptake. However, the 36% labeling level of 13C derived from glycine indicated considerable intact uptake. The fast turnover of amino acids in soils implies that amino acids may serve as a significant N source even at relatively low concentrations in the soil solution (Kielland et al., 2006).
Pinus are obligate symbionts with ectomycorrhizal fungi. At seedling harvest, ectomycorrhizal colonization (fungal sheaths on fine root tips) was observed for each Pinus individual (Zhu and Dai, personal observation). Plants with mycorrhizal associations are generally favored over non-mycorrhizal plants in N-limited ecosystems, where most soil N cycling will largely occur in organic forms. Due to the wider enzymatic capabilities of mycorrhizal fungi compared to plant roots (Chalot and Brun, 1998) and because of the small size of hyphae, fungi are better able to penetrate to the sites of organic matter decomposition and compete directly with other microorganisms for the decomposition products (Kong et al., 2017). Thus, it was possible that the detected intact uptake of amino acids may be largely contributed by the mycorrhizal symbiont (Jones, 1999; Näsholm and Persson, 2001).
Preference of Nitrate by the Other Three Species
In contrast to the Pinus species, the other three species, Larix, Quercus, and Juglans primarily used nitrate, followed by glycine. Such uptake patterns differ from those in temperate forests from China (Donglingshan, Liu et al., 2017), in a wet temperate forest in Tasmania (Warren and Adams, 2007), and two temperate forests in northeastern United States on acidic soils (pH 4; Finzi and Berthrong, 2005), in all of which found ammonium to be preferred by plants over glycine. However, our results resembled that from a sugar maple-white ash site in northeastern United States on dolomitic soil (pH 5.8, Gallet-Budynek et al., 2009), where nitrate and ammonium were preferred over glycine. In addition, previous long-term 15N tracer studies on the different fates of deposited ammonium and nitrate in a natural Korean pine and broad-leaved mixed forest at Changbai Mountain, northeastern China, revealed that broad-leaved trees assimilated more nitrate than ammonium over 410 days, with recovery of 16% of added 15 in aboveground biomass, in contrast to 6% recovery of 15 (Liu et al., 2016). In the NSF in Qingyuan, plants also retain more nitrate than ammonium after 3 months of tracer addition (Li et al., 2019).
The following potential mechanisms may cause the preferential uptake and assimilation of nitrate by Larix, Quercus, and Juglans in this study:
The primary reliance on nitrate over glycine and ammonium might be related to the ambient soil availability of these N forms. After 1 year growth of all four species, soils were characterized by higher nitrate than ammonium concentrations, with a nitrate to ammonium ratio of 1.3 ∼ 2.1 (Table 2). Thus, the observed higher uptake of nitrate than glycine or ammonium corresponded well with this predominance of nitrate over ammonium in these soils. In studies of N uptake in tree species along N availability gradients, N uptake rates changed markedly depending on atmospheric N deposition and soil N availability (Högberg, 1997). In this study, we supplied four species of tree seedlings with identical concentrations of 15N tracers in the three forms (e.g., glycine: ammonium : nitrate being 1:1:1), and three of the species took up more nitrate than other forms. Considering the dominance of nitrate over ammonium in the ambient soils (nitrate : ammonium greater than 1 : 1; Table 2, Xi et al., 2016), these trees may also take up more nitrate than ammonium in their native environments.
Secondly, the higher uptake capacity for nitrate may reflect the mobility of this ion in the soil. In a temperate grassland soil, nitrate diffused through soil water 36 times faster than glycine and 123 times faster than ammonium (Owen and Jones, 2001), due to the easy sorption of ammonium by negatively charged clay particles and soil organic matter (Barber, 1995). In contrast, glycine is amphoteric and nitrate is negatively charged; they adsorb less readily than ammonium onto exchange sites in soil and both forms are mobile in soil water, often moving to existing root surfaces through transpirational water (Raven et al., 1992; Chapin et al., 2002). Overall, these possible underlying mechanisms to some extent explained the greater reliance of the three species on nitrate over glycine and ammonium, which was also reflected by variations in the pool sizes within the soils.
Thirdly, higher assimilation of 15 into leaves and stems of the three species Larix, Quercus, and Juglans (Figure 2) also reflected the characteristic of faster transport of nitrate within plants than amino acids or ammonium (Andrews, 1986). Nitrate can be transported directly from roots to other plant organs before being assimilated into organic compounds via nitrate reductase and the glutamine synthetase/glutamate synthase (GS/GOGAT) system (Andrews, 1986). In contrast, ammonium must be assimilated in roots via the GS/GOGAT system into glutamine before it can be transported throughout a plant (Andrews, 1986). Scott and Rothstein (2011) observed the most 15N partition into aboveground organs in the 15 treatment, the least in the 15 treatment, and intermediary levels in the 15N-amino acid treatments. Greater distribution of nitrate in leaves compared to ammonium and amino acid N cited a faster transport of nitrate out of roots compared to amino acids or ammonium (Persson et al., 2006).
Implications
Distinguishing the different fates of ammonium and nitrate is a prerequisite to better understand the consequences of increasing N deposition. Deposited ammonium and nitrate are processed differently in a mixed forest in Changbai Moutain (Liu et al., 2016) and in NSF in Qingyuan (Li et al., 2019). In both forests, aboveground plant biomass was a bigger sink for nitrate than for ammonium over 90 days (Li et al., 2019) and 410 days (Liu et al., 2016). Our results from a 48-h labeling experiment provided evidence of plant preferential uptake of nitrate by three species (Larix, Quercus, and Juglans), corresponding well to the observed different sink strength for ammonium and nitrate by these plants over relative longer terms.
Recently increased proportions of nitrate in N deposition has decreased the mean ammonium : nitrate ratio from 6 in 1980 to 2 in 2010 (Liu et al., 2013). This may increase soil nitrate in these disturbed forest stands, resulting in larger nitrate : ammonium ratios. Our results that Pinus assimilated predominantly glycine into fine roots and coarse roots, in contrast to primarily nitrate assimilation by the other three species, Larix, Quercus, and Juglans, implied that some coniferous trees may compete less well than other species, particularly if they are unable to shift root preference toward increased nitrate availability. Therefore, increasing N deposition may influence the coexistence of plant species and lead to community composition change in these forest stands.
Data Availability
The raw data supporting the conclusions of this manuscript will be made available by the authors, without undue reservation, to any qualified researcher.
Author Contributions
YF and FZ conceived and designed the experiments. FZ and LD performed the data acquisition. FZ analyzed the data and wrote the manuscript. YF, EH, KK, XL, GG, SHu, SL, YL, and SHa commented and edited the article.
Funding
This work was financially supported by the National Key Research and Development Program of China (Grant No. 2016YFA0600800), the Strategic Priority Research Program of Chinese Academy of Sciences (Grant No. XDB15020200), the National Natural Science Foundation of China (Grant Nos. 31400386, 31770498, and 41773094), the Key Research Program of Frontier Sciences of Chinese Academy of Sciences (Grant No. QYZDB-SSW-DQC002), and the K. C. Wong Education Foundation.
Conflict of Interest Statement
The authors declare that the research was conducted in the absence of any commercial or financial relationships that could be construed as a potential conflict of interest.
Acknowledgments
We are grateful to Prof. Weixing Zhu of the University of Binghamton and Prof. Per Gundersen of the University of Copenhagen for suggestions on the organization of the manuscript, the presentation of results and the novelty of the study.
Supplementary Material
The Supplementary Material for this article can be found online at: https://www.frontiersin.org/articles/10.3389/fpls.2019.00799/full#supplementary-material
FIGURE S1 | Mean (± 1 SE, n = 4) uptake rates of 15N by different plant organs and whole plants of the four taxa (A–D) at 24 h after soil injection of 15N as glycine, ammonium, or nitrate. Different letters above the bars indicate significant differences in uptake rates between N forms for each species (one-way ANOVA, P < 0.05).
References
Andersen, K. M., Mayor, J. R., and Turner, B. L. (2017). Plasticity in nitrogen uptake among plant species with contrasting nutrient acquisition strategies in a tropical forest. Ecology 98, 1388–1398. doi: 10.1002/ecy.1793
Andrews, M. (1986). The partitioning of nitrate assimilation between root and shoot of higher plants. Plant Cell Environ. 9, 511–519. doi: 10.1111/j.1365-3040.1986.tb01582.x
Ashton, I. W., Miller, A. E., Bowman, W. D., and Suding, K. N. (2010). Niche complementarity due to plasticity in resource use: plant partitioning of chemical N forms. Ecology 91, 3252–3260. doi: 10.1890/09-1849.1
Barber, S. A. (1995). Soil Nutrient Bioavailability: a Mechanistic Approach, 2nd Edn. New York, NY: Wiley.
Bardgett, R. D., Streeter, T. C., and Bol, R. (2003). Soil microbes compete effectively with plants for organic nitrogen inputs to temperate grasslands. Ecology 84, 1277–1287. doi: 10.1890/0012-9658(2003)084[1277:SMCEWP]2.0.CO;2
Berthrong, S. T., and Finzi, A. C. (2006). Amino acid cycling in three cold temperate forests of the northeastern USA. Soil Biol. Biochem. 38, 861–869. doi: 10.1016/j.soilbio.2005.08.002
Cabrera, M., and Beare, M. (1993). Alkaline persulfate oxidation for determining total nitrogen in microbial biomass extracts. Soil Sci. Soc. Am. J. 57, 1007–1012.
Chalot, M., and Brun, A. (1998). Physiology of organic nitrogen acquistion by ectomycorrhizal fungi and ectomycorrhizas. FEMS Microbiol. Rev. 22, 21–44. doi: 10.1016/s0168-6445(98)00004-7
Chapin, F. S., Matson, P., and Mooney, H. (2002). Principles of Terrestrial Ecosystem Ecology. New York, NY: Springer.
Craine, J. M., Elmore, A. J., Aidar, M. P. M., Bustamante, M., Dawson, T. E., Hobbie, E. A., et al. (2009). Global patterns of foliar nitrogen isotopes and their relationships with climate, mycorrhizal fungi, foliar nutrient concentrations, and nitrogen availability. New Phytol. 183, 980–992. doi: 10.1111/j.1469-8137.2009.02917.x
Doyle, A., Weintraub, M., and Schimel, J. (2004). Persulfate digestion and simultaneous colorimetric analysis of carbon and nitrogen in soil extracts. Soil Sci. Soc. Am. J. 68, 669–676.
Finzi, A., and Berthrong, S. (2005). The uptake of amino acids by microbes and trees in three cold-temperate forests. Ecology 86, 3345–3353. doi: 10.1890/04-1460
Gallet-Budynek, A., Brzostek, E., Rodgers, V. L., Talbot, J. M., Hyzy, S., and Finzi, A. C. (2009). Intact amino acid uptake by northern hardwood and conifer trees. Oecologia 160, 129–138. doi: 10.1007/s00442-009-1284-2
Guo, D., Xia, M., Wei, X., Chang, W., Liu, Y., Wang, Z., et al. (2008). Anatomical traits associated with absorption and mycorrhizal colonization are linked to root branch order in twenty-three Chinese temperate tree species. New Phytol. 180, 673–683. doi: 10.1111/j.1469-8137.2008.02573.x
Hao, Z. Q., Wang, Q. L., and Dai, L. M. (2000). “The importance of the national program for natural forests conservation on biodiversity conservation in northeast state owned forest areas of China,” in Go Forward the 21st Century’s Chinese Biodiversity Conservation. ed. Z. H. Xu (Beijing: China Forestry Publishing House), 21–26.
Hobbie, J. E., and Hobbie, E. A. (2012). Amino acid cycling in plankton and soil microbes studied with radioisotopes: measured amino acids in soil do not reflect bioavailability. Biogeochemistry 107, 339–360. doi: 10.1007/s10533-010-9556-9
Högberg, P. (1997). 15N natural abundance in soil-plant systems. New Phytol. 137, 179–203. doi: 10.1046/j.1469-8137.1997.00808.x
Hope, G. D., Prescott, C. E., and Blevins, L. L. (2003). Responses of available soil nitrogen and litter decomposition to openings of different sizes in dry interior Douglas-fir forests in British Columbia. For. Ecol. Manag. 186, 33–46. doi: 10.1016/s0378-1127(03)00241-x
Houlton, B. Z., Sigman, D. M., Schuur, E. A. G., and Hedin, L. O. (2007). A climate driven switch in plant nitrogen acquisition within tropical forest communities. Proc. Natl. Acad. Sci. U.S.A. 104, 8902–8906. doi: 10.1073/pnas.0609935104
Huang, S., Elliott, E. M., Felix, J. D., Pan, Y., Liu, D., Li, S., et al. (2019). Seasonal pattern of ammonium 15N natural abundance in precipitation at a rural forested site and implications for NH3 source partitioning. Environ. Pollut. 247, 541–549. doi: 10.1016/j.envpol.2019.01.023
Ingestad, T. (1979). Mineral nutrient requirements of Pinus sylvestris and Picea abies seedlings. Physiol. Plant. 45, 373–380. doi: 10.1111/j.1399-3054.1979.tb02599.x
Jones, D. L. (1999). Amino acid biodegradation and its potential effects on organic nitrogen capture by plants. Soil Biol. Biochem. 31, 613–622. doi: 10.1016/s0038-0717(98)00167-9
Jones, D. L., Healey, J., Willett, V., Farrar, J. F., and Hodge, A. (2005). Dissolved organic nitrogen uptake by plants – An important N uptake pathway? Soil Biol. Biochem. 37, 413–423. doi: 10.1016/j.soilbio.2004.08.008
Jones, D. L., and Hodge, A. (1999). Biodegradation kinetics and sorption reactions of three differently charged amino acids in soil and their effects on plant organic nitrogen availability. Soil Biol. Biochem. 31, 1331–1342. doi: 10.1016/s0038-0717(99)00056-5
Kielland, K., Mcfarland, J. W., and Olson, K. (2006). Amino acid uptake in deciduous and coniferous taiga ecosystems. Plant Soil 288, 297–307. doi: 10.1007/s11104-006-9117-0
Kong, D., Wang, J., Zeng, H., Liu, M., Miao, Y., Wu, H., et al. (2017). The nutrient absorption–transportation hypothesis: optimizing structural traits in absorptive roots. New Phytol. 213, 1569–1572. doi: 10.1111/nph.14344
Kronzucker, H., Siddiqi, M., and Glass, A. (1997). Conifer root discrimination against soil nitrate and the ecology of forest succession. Nature 385, 59–61. doi: 10.1038/385059a0
Li, S., Gurmesa, G. A., Zhu, W., Gundersen, P., Zhang, S., Xi, D., et al. (2019). Fate of atmospherically deposited and in two temperate forests in China: temporal pattern and redistribution. Ecol. Appl. 0:e01920.
Lipson, D., and Näsholm, T. (2001). The unexpected versatility of plants: organic nitrogen use and availability in terrestrial ecosystems. Oecologia 128, 305–316. doi: 10.1007/s004420100693
Lipson, D. A., Raab, T. K., Schmidt, S. K., and Monson, R. K. (1999). Variation in competitive abilities of plants and microbes for specific amino acids. Biol. Fertil. Soils 29, 257–261. doi: 10.1007/s003740050550
Liu, J., Peng, B., Xia, Z., Sun, J., and Bai, E. (2016). Different fates of deposited and in a temperate forest in northeast China: a 15N tracer study. Global Change Biol. 23, 2441–2449. doi: 10.1111/gcb.13533
Liu, M., Li, C. C., Xu, X. L., Wanek, W., Jiang, N., Wang, H., et al. (2017). Organic and inorganic nitrogen uptake by 21 dominate tree species in temperate and tropical forests. Tree Physiol. 37, 1515–1526. doi: 10.1093/treephys/tpx046
Liu, S. R., Li, X. M., and Niu, L. M. (1998). The degradation of soil fertility in pure larch plantations in the northeastern of China. Ecol. Eng. 10, 75–86. doi: 10.1016/s0925-8574(97)10024-6
Liu, X. J., Zhang, Y., Han, W. X., Tang, A., Shen, J., Cui, Z., et al. (2013). Enhanced nitrogen deposition over China. Nature 494, 459–462. doi: 10.1038/nature11917
Liu, X. Y., Keisuke, K., Koyama, L. A., Hobbie, S. E., Weiss, M. S., Inagaki, Y., et al. (2018). Nitrate is an important nitrogen source for arctic tundra plants. Proc. Natl. Acad. Sci. U.S.A. 115, 3398–3403. doi: 10.1073/pnas.1715382115
McKane, R. B., Johnson, L. C., Shaver, G. R., Nadelhoffer, K. J., Rastetter, E. B., Fry, B., et al. (2002). Resource-based niches provide a basis for plant species diversity and dominance in arctic tundra. Nature 415, 68–71. doi: 10.1038/415068a
Näsholm, T., Kielland, K., and Ganeteg, U. (2009). Uptake of organic nitrogen by plants. New Phytol. 182, 31–48. doi: 10.1111/j.1469-8137.2008.02751.x
Näsholm, T., and Persson, J. (2001). Plant acquisition of organic nitrogen in boreal forests. Physiol. Plant. 111, 419–426. doi: 10.1034/j.1399-3054.2001.1110401.x
Öhlund, J., and Näsholm, T. (2001). Growth of conifer seedlings on organic and inorganic nitrogen sources. Tree Physiol. 21, 1319–1326. doi: 10.1093/treephys/21.18.1319
Owen, A. G., and Jones, D. L. (2001). Competition for amino acids between wheat roots and rhizosphere microorganisms and the role of amino acids in plant N acquisition. Soil Biol. Biochem. 33, 651–657. doi: 10.1016/s0038-0717(00)00209-1
Persson, J., Gardeström, P., and Näsholm, T. (2006). Uptake, metabolism and distribution of organic and inorganic nitrogen sources by Pinus sylvestris. J. Exp. Bot. 57, 2651–2659. doi: 10.1093/jxb/erl028
Persson, J., Hogberg, P., Ekblad, A., Hogberg, M. N., Nordgren, A., and Nasholm, T. (2003). Nitrogen acquisition from inorganic and organic sources by boreal forest plants in the field. Oecologia 137, 252–257. doi: 10.1007/s00442-003-1334-0
Persson, J., and Näsholm, T. (2001). Amino acid uptake: a widespread ability among boreal forest plants. Ecol. Lett. 4, 434–438. doi: 10.1046/j.1461-0248.2001.00260.x
Raven, J. A., Wollenweber, B., and Handley, L. L. (1992). A comparison of ammonium and nitrate as nitrogen sources for photolithotrophs. New Phytol. 121, 19–32. doi: 10.1111/j.1469-8137.1992.tb01088.x
Schimel, J. P., and Bennett, J. (2004). Nitrogen mineralization: challenges of a changing paradigm. Ecology 85, 591–602. doi: 10.1890/03-8002
Schmidt, S., and Stewart, G. R. (1999). Glycine metabolism by plant roots and its occurrence in Australian plant communities. Funct. Plant Biol. 26, 253–264.
Scott, E., and Rothstein, D. (2011). Amino acid uptake by temperate tree species characteristic of low- and high-fertility habitats. Oecologia 167, 547–557. doi: 10.1007/s00442-011-2009-x
Stephan, K., and Kavanagh, K. L. (2009). Suitability of the diffusion method for natural abundance nitrogen-15 analysis. Soil Sci. Soc. Am. J. 73, 293–302.
Stewart, G. R., Joly, C. A., and Smirnoff, N. (1992). Partitioning of inorganic nitrogen assimilation between the roots and shoots of cerrado and forest trees of contrasting plant communities of South East Brasil. Oecologia 91, 511–517. doi: 10.1007/BF00650324
Takebayashi, Y., Koba, K., Sasaki, Y., Fang, Y. T., and Yoh, M. (2010). The natural abundance of 15N in plant and soil-available N indicates a shift of main plant N resources to from along the N leaching gradient. Rapid Commun. Mass Spectrom. 24, 1001–1008. doi: 10.1002/rcm.4469
Templer, P. H., and Dawson, T. E. (2004). Nitrogen uptake by four tree species of the Catskill Mountains, New York: implications for forest N dynamics. Plant Soil 262, 251–261. doi: 10.1023/B:PLSO.0000037047.16616.98
Tu, Y., Fang, Y. T., Liu, D. W., and Pan, Y. P. (2016). Modifications to the azide method for nitrate isotope analysis. Rapid Commun. Mass Spectrom. 30, 1213–1222. doi: 10.1002/rcm.7551
Wang, C. M., Oy, H., Shao, B., Tian, Y. Q., Zhao, J. G., and Xu, H. Y. (2006). Soil carbon changes following afforestation with Olga Bay Larch (Larix olgensis Henry) in North-eastern China. J. Integr. Plant Biol. 48, 503–512. doi: 10.1111/j.1744-7909.2006.00264.x
Warren, C. R., and Adams, P. R. (2007). Uptake of nitrate, ammonium and glycine by plants of Tasmanian wet eucalypt forests. Tree Physiol. 17, 413–419. doi: 10.1093/treephys/27.3.413
Wright, I. J., Reich, P. B., Westoby, M., Ackerly, D. D., Baruch, Z., Bongers, F., et al. (2004). The worldwide leaf economics spectrum. Nature 428, 821–827.
Xi, D., Bai, R., Zhang, L., and Fang, Y. (2016). Contribution of anammox to nitrogen removal in two temperate forest soils. Appl. Environ. Microbiol. 82, 4602–4612. doi: 10.1128/AEM.00888-16
Yang, K., Zhu, J. J., Yan, Q. L., and Zhang, J. X. (2012). Soil enzyme activities as potential indicators of soluble organic nitrogen pools in forest ecosystems of Northeast China. Ann. For. Sci. 69, 795–803. doi: 10.1007/s13595-012-0198-z
Yang, K., Zhu, J. J., Zhang, M., Yan, Q. L., and Sun, O. J. (2010). Soil microbial biomass carbon and nitrogen in forest ecosystems of Northern China: a comparison between natural secondary forest and larch plantation. J. Plant Ecol. 3, 175–182. doi: 10.1093/jpe/rtq022
Keywords: glycine, ammonium, nitrate, nitrogen uptake, nitrogen availability, stable isotopes, secondary forest in northeast China
Citation: Zhu F, Dai L, Hobbie EA, Koba K, Liu X, Gurmesa GA, Huang S, Li S, Li Y, Han S and Fang Y (2019) Uptake Patterns of Glycine, Ammonium, and Nitrate Differ Among Four Common Tree Species of Northeast China. Front. Plant Sci. 10:799. doi: 10.3389/fpls.2019.00799
Received: 15 January 2019; Accepted: 03 June 2019;
Published: 02 July 2019.
Edited by:
Tarja Lehto, University of Eastern Finland, FinlandReviewed by:
Barbara Hawkins, University of Victoria, CanadaConcepcion Avila, University of Málaga, Spain
Copyright © 2019 Zhu, Dai, Hobbie, Koba, Liu, Gurmesa, Huang, Li, Li, Han and Fang. This is an open-access article distributed under the terms of the Creative Commons Attribution License (CC BY). The use, distribution or reproduction in other forums is permitted, provided the original author(s) and the copyright owner(s) are credited and that the original publication in this journal is cited, in accordance with accepted academic practice. No use, distribution or reproduction is permitted which does not comply with these terms.
*Correspondence: Yunting Fang, fangyt@iae.ac.cn
†These authors have contributed equally to this work