- 1Centro de Biotecnologia y Genomica de Plantas, Instituto Nacional de Investigacion y Tecnologia Agraria y Alimentaria (INIA), Universidad Politécnica de Madrid (UPM), Campus Montegancedo UPM, Madrid, Spain
- 2Departamento de Biotecnologia-Biologia Vegetal, Escuela Tecnica Superior de Ingenieria Agronomica, Alimentaria y de Biosistemas, Universidad Politécnica de Madrid (UPM), Madrid, Spain
- 3Departamento de Mejora Genética Vegetal, Instituto de Agricultura Sostenible (IAS-CSIC), Córdoba, Spain
Plant proteases play a crucial role in many different biological processes along the plant life cycle. One of the most determinant stages in which proteases are key protagonists is the plant germination through the hydrolysis and mobilization of other proteins accumulated in seeds and cereal grains. The most represented proteases in charge of this are the cysteine proteases group, including the C1A family known as papain-like and the C13 family also called legumains. In cereal species such as wheat, oat or rye, gluten is a very complex mixture of grain storage proteins, which may affect the health of sensitive consumers like celiac patients. Since gluten proteins are suitable targets for plant proteases, the knowledge of the proteases involved in storage protein mobilization could be employed to manipulate the amount of gluten in the grain. Some proteases have been previously found to exhibit promising properties for their application in the degradation of known toxic peptides from gluten. To explore the variability in gluten-degrading capacities, we have now analyzed the degradation of gluten from different wheat cultivars using several cysteine proteases from barley. The wide variability showed highlights the possibility to select the protease with the highest potential to alter grain composition reducing the gluten content. Consequently, new avenues could be explored combining genetic manipulation of proteolytic processes with silencing techniques to be used as biotechnological tools against gluten-related disorders.
Introduction
Plant proteases have been described to accomplish multiple roles in different physiological processes along the plant life cycle, such as programmed cell death, senescence, abscission, fruit ripening, plant growth, and N homeostasis (Grudkowska and Zagdańska, 2004; van der Hoorn, 2008; Liu et al., 2018; Tornkvist et al., 2019). In response to abiotic and biotic stresses, proteases are also involved in nutrient remobilization associated with leaf and root proteins degradation to ensure yield (Diaz-Mendoza et al., 2014; Velasco-Arroyo et al., 2016, 2018; Gomez-Sanchez et al., 2019; James et al., 2019), or in triggering the response of the plant to pathogens and phytophagous insects and acari (van der Hoorn and Jones, 2004; Shindo and van der Hoorn, 2008; Misas-Villamil et al., 2016; Diaz-Mendoza et al., 2017). Moreover, plant proteases play a crucial role in the plant seed germination, through the mobilization of other proteins accumulated in seeds and cereal grains (Grudkowska and Zagdańska, 2004; Cambra et al., 2012; Diaz-Mendoza et al., 2016; Szewinska et al., 2016; Liu et al., 2018; Radchuk et al., 2018).
Cereals grains include proteins with many different functions. Around 80% of these proteins are storage proteins, packed in the endosperm together with starch and lipids (Shewry et al., 1995; Shewry and Halford, 2002). These proteins are synthesized during grain development and maturation, subsequently included as storage proteins, and finally degraded during germination. Several groups of proteases have been implicated in seed germination. The most represented proteases in charge of the mobilization and degradation of storage proteins are the cysteine proteases (CysProt) (Grudkowska and Zagdańska, 2004; Tan-Wilson and Wilson, 2011; Szewinska et al., 2016). Among CysProt, the C1A family known as papain-like and the C13 family also called legumains or vacuolar processing enzymes (VPEs), have been the most studied (Hara-Nishimura et al., 1995, 1998; Kinoshita et al., 1999; Grudkowska and Zagdańska, 2004; Prabucka and Bielawski, 2004; Martínez and Díaz, 2008; Shi and Xu, 2009; Szewinska et al., 2016).
Pharmaceutical, food and beverage, detergent, and biofuel industries have for long time exploited enzyme catalysis in commercial-scale applications, being the use of papain an example of success in food industry over the last four decades (Fernández-Lucas et al., 2017). In recent years, newly identified plant proteases have been found to exhibit promising properties for their application in the food industry (Feijoo-Siota and Villa, 2011). A case of particular interest is the degradation of peptides toxic for celiac patients by proteases involved in the germination of cereals (Hartmann et al., 2006; Kiyosaki et al., 2007; Stenman et al., 2009, 2010). In particular, the 33-mer peptide of gluten is the most immunogenic peptide known so far. It is resistant to human proteases and responsible for eliciting about 90% of the allergic response induced by the full complement of wheat proteins (Tye-Din et al., 2010). The immunogenic peptide can be hydrolyzed by a combination of at least six different peptidases from sourdough lactobacilli and fungal proteases provided as a food supplement (De Angelis et al., 2010). However, the finding and use of plant proteases have several advantages compared to bacterial and fungal peptidases; they are natural components of harmless food and, unlike the fungal proteases, their safety must not be proven with potential benefits for both therapeutics and food processing (Comino et al., 2013). Therefore, the co-administration of exogenous proteases with food is a very appealing therapeutic treatment for celiac patients by facilitating gluten degradation. In particular, the knowledge of the proteases involved in storage protein mobilization could be employed to discover proteases able to degrade gluten efficiently. Several pathologies have been described with gluten intake: celiac disease (CD), an autoimmune disorder with a prevalence of about 0.7–2% in the human population (Rewers, 2005) that has increased in the last fifty years (Rubio-Tapia et al., 2009); and non-celiac wheat sensitivity (NCWS), a new pathology which is estimated to occur in about 6% of the population in western countries (Sapone et al., 2011).
Plant Proteases in the Germination Process of Barley
In cereal grains, different proteases have been involved in the germination process (Müntz et al., 2002; Grudkowska and Zagdańska, 2004; Tan-Wilson and Wilson, 2011; Szewinska et al., 2016). In maize, wheat and barley the CysProts are responsible of around 90% of the proteolytic activity (De Barros and Larkins, 1994; Zhang and Jones, 1995; Bottari et al., 1996). Plant CysProts from the papain family (C1A) (Mikkonen et al., 1996; Prabucka and Bielawski, 2004; Shi and Xu, 2009; Cambra et al., 2012; Diaz-Mendoza et al., 2016) and CysProts of the legumain family (C13) (Hara-Nishimura et al., 1998; Radchuk et al., 2011, 2018), are the most representative groups in proteolysis and mobilization of storage proteins in cereal grain. Other proteases have been implicated in the germination process in cereal grains, such as the S10 serine carboxypeptidases (Ser-) (Dal Degan et al., 1994; Washio and Ishikawa, 1994; Dominguez and Cejudo, 1999; Domínguez et al., 2002; Li et al., 2016).
Barley is one of the grain cereals further studied. Proteases, amylases, dextrinases and other hydrolases are crucial for the survival of the seedling until the photosynthesis is finally established (Figure 1). In the barley germination, proteases of the papain-like C1A (Koehler and Ho, 1988, 1990a,b; Poulle and Jones, 1988; Zhang and Jones, 1995; Sreenivasulu et al., 2008) and the legumain-like C13 families (Linnestad et al., 1998; Radchuk et al., 2011, 2018) have mainly been identified. Besides, the expression of six serine carboxypeptidases (Ser-CPs) during maturation and germination of the barley grain has been documented (Dal Degan et al., 1994; Table 1).
Two cathepsin L-like, EP-A and EP-B, were the first barley CysProts described to participate in the proteolytic degradation of the storage proteins in barley grains (Koehler and Ho, 1988, 1990a,b; Poulle and Jones, 1988; Mikkonen et al., 1996). These CysProts are synthesized in the scutellar epithelium and the aleurone layer and, then, secreted to the endosperm upon germination (Mikkonen et al., 1996; Martínez et al., 2009). An aleurain belonging to the cathepsin H-like group of CysProts has been isolated from aleurone (Rogers et al., 1985; Holwerda and Rogers, 1992) and a cathepsin B-like protein, HvCathB, has been detected in aleurone and developing endosperm (Martínez et al., 2003). Similarly, a member of the cathepsin F-like group, the HvPap-1, has been detected in germinating barley grains (Sreenivasulu et al., 2008). HvPap-1 takes part in the proteolytic mobilization of stored proteins like hordeins, albumins and globulins in the barley endosperm and its activity is modulated by its own propeptide (Cambra et al., 2012). HvPap-1 contributes to barley grain filling and germination since over-expression or silencing of HvPap-1 gene in barley transgenic plants alters the metabolite composition of the grain, and modifies the germination process by delaying or accelerating it (Diaz-Mendoza et al., 2016). Likewise, the presence of HvPap-4, -6 and -10 in the germinating embryo and aleurone layers has been demonstrated, as well as the capacity of hordein degradation by HvPap-6 and 10 (Martínez et al., 2009). Regarding the barley C13 legumain-like family, their ability to process other CysProts in order to activate them to take part in the proteolytic degradation of the storage proteins it has been also described (Cambra et al., 2010). This is the case of the HvLeg-2 legumain of barley, which is highly expressed during germination and could be involved in the mobilization of storage proteins either by direct proteolytic degradation or by processing and activation of other CysProts (Cambra et al., 2010; Julián et al., 2013). Consequently, for the hydrolysis and mobilization of storage proteins in barley grains the cooperative proteolysis of papain-like CysProt and legumains is essential (Cambra et al., 2010). Overall, the diversity and proteolytic specificity of barley proteases involved in the degradation of storage compounds makes them a starting point to select a potential candidate for further applications.
Potential Application of Barley Proteases in Celiac Disease
From an applied point of view, this wide knowledge on barley proteases acting in the germination process could be employed to manipulate and improve the grain composition not only in barley but also in those cereal species such as wheat, oat, rye and related species and hybrids, in which a number of proteins, known as gluten proteins, affect the health of sensitive consumers.
Celiac disease is the best-characterized pathology associated with gluten consumption and there is a major environmental factor, the ingestion of gluten proteins not only from wheat but also from barley and rye. It has also an important genetic risk factor related to the genes encoding for the human leukocyte antigen (HLA) -DQ2 or -DQ8 (Schuppan, 2000). A lifelong gluten free diet reverses signs and symptoms of celiac disease and NCWS. Nevertheless, this is difficult to follow due to the wide gluten presence in many diet foods. Gluten is a very complex mixture of storage proteins classified into glutenins and gliadins, comprising around 80% of the total grain proteins, with about 30% gliadins and 50% glutenins. Most of the CD related epitopes have been found in the gliadin fraction (Arentz-Hansen et al., 2002). Gliadins are rich in the amino acids proline, and glutamine, which make them resistant to being fully digested in the gastrointestinal tract. This is the case of human proteases, which do not accept proline at their cleavage sites. Partial digestion of gluten generates small peptides which induces a CD4+ T-cell inflammatory response leading to villous atrophy through a two-signal model (Brandtzaeg, 2006). According to this model, a first innate immune response is triggered by certain peptides, such as the 19-mer gliadin peptide, resulting in the production of interleukin 15 (IL-15) by epithelial cells. The result is the disruption of the epithelial barrier by increasing its permeability. Then, other immune-adaptive peptides, like the 33-mer, can reach the lamina propria and they are deaminated by the tissue transglutaminase (tTG2), providing a strong negative charge to gliadin peptide enhancing their affinity to bind within the HLA-DQ2/8 bound (Bethune and Khosla, 2012).
Different therapeutic alternatives are being developed, as the inhibition of transglutaminase, the antagonism of peptide binding to HLA-DQ2 or HLA-DQ8, the enzymatic detoxification of gluten, or the introduction of natural amino acid substitution to eliminate toxicity (Schuppan et al., 2009; Ruiz-Carnicer et al., 2019). Some other strategies have been developed trying to relieve the negative effect of gluten proteins (Scherf et al., 2018). A promising approach is the down-regulation of genes encoding for gliadins by RNAi technologies, generating transgenic wheat lines with low levels of toxicity for celiacs (Gil-Humanes et al., 2010). Previous investigations have already developed wheat with a down regulation of gliadins expression by hairpin technology. As result, a very low or null T-cells stimulation was proven (Gil-Humanes et al., 2010). The reduced-gliadin breads showed baking and sensory properties, and overall acceptance, similar to those of normal flour, but with up to 97% lower gliadin content (Gil-Humanes et al., 2014). Their results showed that targeting of genes related to celiac disease is feasible and may reduce T-cell epitopes. More recent, low-gluten wheat was engineered with CRISPR/Cas9 technology (Jouanin et al., 2018; Sánchez-León et al., 2018), providing bread and durum with reduced amount of α-gliadins in the seed kernel and a low immunoreactivity for gluten intolerant consumers.
In addition, enzymes able to degrade gliadins have been suggested as hopeful therapeutic agents, as is the barley CysProt EP-B2, which efficiently hydrolyzed a recombinant wheat gluten protein, α2-gliadin, which contains sequences with known immunotoxicity in celiac sprue patients (Bethune et al., 2006). Some prolyl endoproteases from Aspergillus niger (AN-PEP) and Sphingomonas capsulate (SC-PEP), with also gliadin degradative capacity, have been characterized (Gass et al., 2007). An EP-B2 and SC-PEP combination has been studied as a promising therapeutic tool, being EP-B2 responsible for hydrolyzing gluten proteins into short oligopeptides, which are still toxic, and SC-PEP for breaking down these oligopeptides into non-toxic metabolites (Gass et al., 2007).
Wheat Gluten Hydrolysis by Barley Cysprot
To further explore the enzymatic strategy, we have now analyzed the degradation of six different gliadin fractions from wheat cultivars and breeding lines (Perico, THA1, THA7, THA53, BW2003, and BW208) (Gil-Humanes et al., 2012) by four different CysProt from barley (HvPap-1, -4, -6, and -16) (Supplementary Material). These proteases have been previously identified, characterized and purified in Escherichia coli (Martínez et al., 2009). With the use of these four CysProt as a representation of different groups of L-like cathepsins and F-like cathepsins, a wide view of their activity on gliadin proteolysis has been achieved. The final goal has been to explore their potential to reduce gliadin content in the wheat gluten or to use them in therapy treatments.
Hydrolysis of the different wheat gliadins by purified barley CysProt was observed by western blot analysis. When gliadins from the six wheat cultivars were treated with HvPap-1, -4, -6, and -16 for 1 and 12 h, different degradation patterns by each CysProt were observed (Figure 2A). Degradation in all gliadin samples was appreciated after 1 h of incubation with HvPap-1, -4, -6, and -16 proteases. In most cases, higher bands remained stable while lower molecular weight bands started to disappear after 1 h of treatment. After 12 h of incubation with barley proteases, an increased differential degradation of gliadin bands was observed. Whereas higher molecular weight bands remained stable after HvPap-16 and HvPap-1 treatments (Figures 2A,A–L), degradation was almost complete after HvPap-4 and HvPap-6 action (Figures 2A,M–X). To discard any processing or degradation effect due to instability or autohydrolysis, gliadins were incubated for 12 h without proteases (Figure 2B). Likewise, as HvPap-1, -4 and -16 were activated by adding pepsin, an analysis of gliadins stability in presence of this commercial peptidase was carried out (Figure 2B). Although some smaller bands were hydrolysed by pepsin, in all treatments the band with higher molecular weight remains stable after 12 h incubation, in contrast with the total degradation exerted by HvPap-4 and HvPap-6. These proteases seem to be the most promising gliadin-degrading enzymes. Furthermore, as HvPap-6 does not need to be activated by pepsin, this protease should be selected over HvPap-4 since degradation is only due to its own activity.
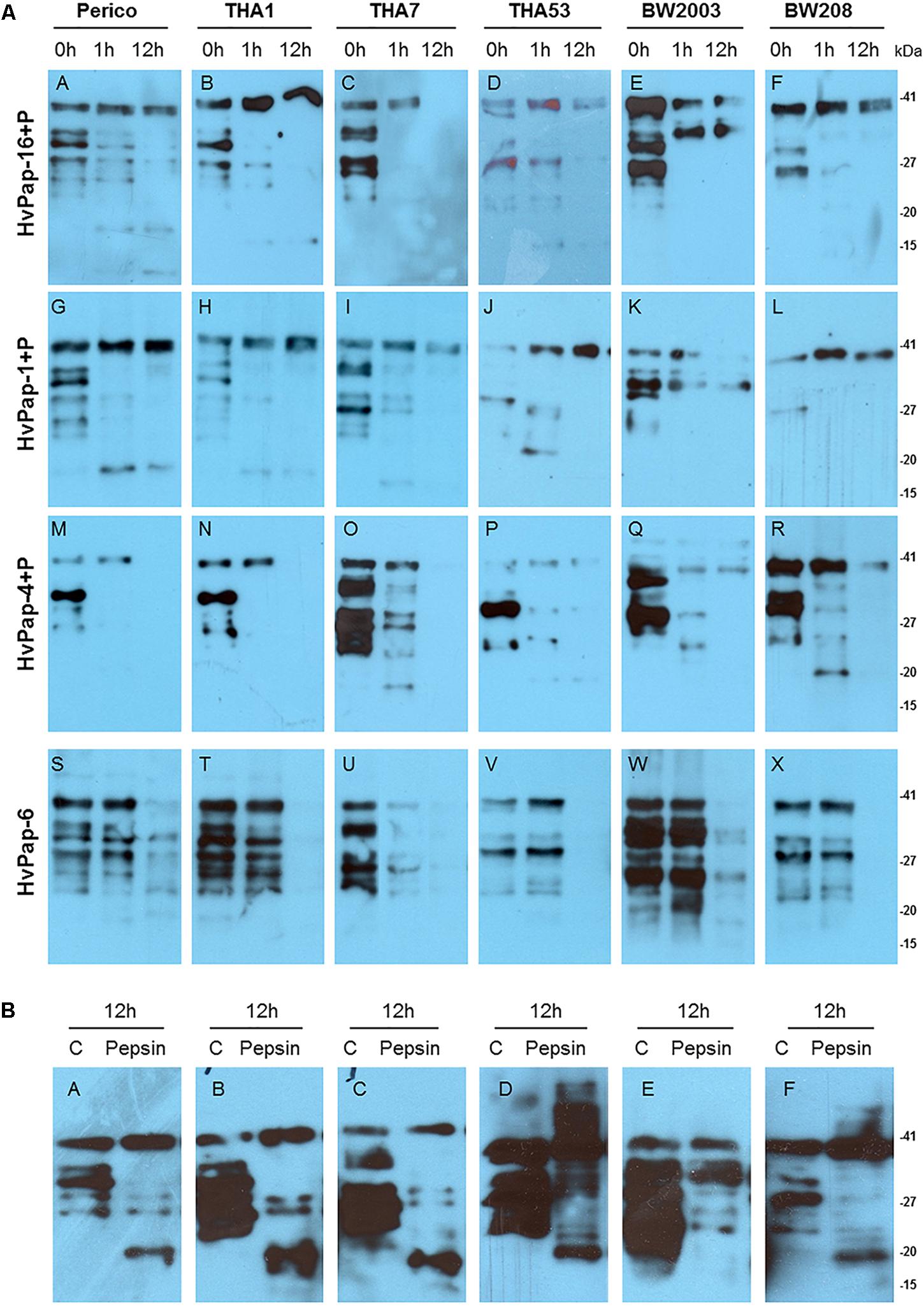
Figure 2. (A) Western blots of 8 μg of gliadins from Perico, THA1, THA7, THA53, BW2003, and BW208 wheat cultivars, incubated with 2 μg of each barley CysProt for 0, 1, and 12 h at 28 °C. Gliadin incubation with HvPap-16+P (A–F); HvPap-1+P (G–L); HvPap-4+P (M–R); HvPap-6 (S–X). (B) Gliadins stability assays after 12 h of incubation without pepsin as control and gliadin treatments with 6 μg of pepsin for 12 h (A–F). Immunoblotting was performed with Gluten Tox G12 HRP-conjugate antibody, 200× (Biomedal diagnostics) following the manufacturer instructions. Pepsin (P) and molecular weight in kilo Dalton unit (kDa).
Plant Proteases and Celiac Disease: Future Perspectives
The potential of plant proteases to alter grain content and the mobilization of stored proteins greatly supports their use as biotechnological tools. As an example, gluten proteins responsible of celiac disease and other gluten-related pathologies are suitable targets for these enzymes. For gluten intolerant patients, the only available treatment is a gluten-free diet, which is extremely difficult to maintain due to gluten ubiquity in human diets. Given the negative impact of quality of life of celiac patients, there is an urgent need to develop food suitable for them. In our research, we have provided new information on the potential of plant CysProt to reduce gliadin content. HvPap-6 CysProt ranks as the best candidate given the encouraging results of this study. The final aim would be the development of transgenic lines of wheat overexpressing this CysProt under endosperm specific promoters to reduce gluten toxicity. This cereal would be used afterward to obtain flour with which to elaborate products suitable for humans with this autoimmune enteropathy. Transgenic wheat lines overexpressing the HvPap-6 gene from barley would have advantages in comparison of oral enzyme therapy since consumers will not be exposed to harmful gluten. Unwanted reactions in celiac patients probably would decrease by consuming lower or even null levels of toxic gluten from transgenic wheat lines. Administration of hydrolytic glutenases as food supplement is an alternative to deliver the therapeutic agents directly to the small intestine, for further degradation of wheat gluten. One step beyond would be to produce transgenic wheat lines combining the overexpression of a CysProt with high gliadin-degrading efficiency and the downregulation of the expression of gliadins using genome editing techniques. The next step should be to evaluate the in vivo efficiency of selected proteases for degrading wheat gliadins in the endosperm. For that, overexpression of these proteases under the control of endosperm specific promoters, such as those from HMW, gamma or alpha-gliadins would be necessary. A recent publication by Osorio et al. (2019), expressing an endoprotease from barley reinforced our perspective about this approach. These authors overexpressed the barley protease EP-B2, combined with prolyl endopeptidases from bacterium, under the control of the endosperm specific wheat 1Dy high-molecular wheat glutenin promoter (pHMWg). A significant reduction (up to 67%) of the amount of the indigestible gluten peptides of all prolamin families was achieved. This approach will contribute, in combination with other strategies like CRISPR/Cas9, to provide wheat varieties suitable for celiac and other gluten intolerance patients. Overall, with new goals, the potential of exploring the properties of plant proteases acting along seed germination will open a great field of research, as a biotechnological alternative to provide new prevention strategies in protein-caused disorders. In conclusion, this is an alternative approach for enzymatic gluten degradation to generate gluten-free wheat to manufacture gluten free products.
Data Availability
All datasets generated for this study are included in the manuscript and/or the Supplementary Files.
Author Contributions
ID, FB, MM, and MD-M conceived the research. SG-C, MG, and MD-M performed the experimental research. All authors contributed to the final version of the manuscript, and read and approved the final manuscript.
Funding
This work was supported by projects from Ministerio de Economia y Competitividad of Spain (project BIO2014-53508-R and AGL2016-80566-P).
Conflict of Interest Statement
The authors declare that the research was conducted in the absence of any commercial or financial relationships that could be construed as a potential conflict of interest.
Supplementary Material
The Supplementary Material for this article can be found online at: https://www.frontiersin.org/articles/10.3389/fpls.2019.00721/full#supplementary-material
References
Arentz-Hansen, H., Mc Adam, S. N., Molberg, Ø, Fleckenstein, B., Lundin, K. E., Jørgensen, T. J., et al. (2002). Celiac lesion T cells recognize epitopes that cluster in regions of gliadins rich in proline residues. Gastroenterology 123, 803–809. doi: 10.1053/gast.2002.35381
Bethune, M. T., and Khosla, C. (2012). Oral enzyme therapy for celiac sprue. Methods Enzymol. 502, 241–271. doi: 10.1016/B978-0-12-416039-2.00013-6
Bethune, M. T., Strop, P., Tang, Y., Sollid, L. M., and Khosla, C. (2006). Heterologous expression, purification, refolding and structural-functional characterization of EP-B2, a self-activating barley cysteine endoprotease. Chem. Biol. 13, 637–647. doi: 10.1016/j.chembiol.2006.04.008
Bottari, A., Capocchi, A., Galleschi, A., Jopova, A., and Saviozzi, E. (1996). Asparaginyl endopeptidase during maturation and germination of durum wheat. Physiol. Plant. 97, 475–480. doi: 10.1111/j.1399-3054.1996.tb00506.x
Brandtzaeg, P. (2006). The changing immunological paradigm in coeliac disease. Immunol. Lett. 105, 127–139. doi: 10.1016/j.imlet.2006.03.004
Cambra, I., Garcia, F. J., and Martínez, M. (2010). Clan CD of cysteine peptidases as an example of evolutionary divergences in related protein families across plant clades. Gene 449, 59–69. doi: 10.1016/j.gene.2009.09.003
Cambra, I., Martínez, M., Dáder, B., González-Melendi, P., Gandullo, J., Santamaría, M. E., et al. (2012). A cathepsin F-like peptidase involved in barley grain protein mobilization, HvPap-1, is modulated by its own propeptide and by cystatins. J. Exp. Bot. 63, 4615–4629. doi: 10.1093/jxb/ers137
Comino, I., Moreno, M., de, L., Real, A., Rodriguez-Herrera, A., Barro, F., et al. (2013). The gluten-free diet: testing alternative cereals tolerated by celiac patients. Nutrients 5, 4250–4268. doi: 10.3390/nu5104250
Dal Degan, F., Rocher, A., Cameron-Mills, V., and von Wettstein, D. (1994). The expression of serine carboxypeptidases during maturation and germination of the barley grain. Proc. Natl. Acad. Sci. U.S.A. 91, 8209–8213. doi: 10.1073/pnas.91.17.8209
De Angelis, M., Cassone, A., Rizzello, C. G., Gagliardi, F., Minervini, F., Calasso, M., et al. (2010). Mechanism of degradation of immunogenic gluten epitopes from Triticum turgidum L. var. durum by sourdough lactobacilli and fungal proteases. Appl. Environ. Microbiol. 76, 508–518. doi: 10.1128/AEM.01630-09
De Barros, E. G., and Larkins, B. A. (1994). Cloning of a cDNA encoding a putative cysteine protease gliadin during wheat germination. Phytochemistry 43Z, 39–44. doi: 10.1016/0168-9452(94)90176-7
Diaz-Mendoza, M., Dominguez-Figueroa, J. D., Velasco-Arroyo, B., Cambra, I., Gonzalez-Melendi, P., Lopez-Gonzalvez, A., et al. (2016). HvPap-1 C1A protease and HvCPI-2 cystatin contribute to barley grain filling and germination. Plant Physiol. 170, 2511–2524. doi: 10.1104/pp.15.01944
Diaz-Mendoza, M., Velasco-Arroyo, B., Gonzalez-Melendi, P., Martinez, M., and Diaz, I. (2014). C1A cysprot-cystatin interactions in leaf senescence. J. Exp. Bot. 65, 3825–3833. doi: 10.1093/jxb/eru043
Diaz-Mendoza, M., Velasco-Arroyo, B., Santamaria, M. E., Diaz, I., and Martinez, M. (2017). HvPap-1 C1A protease participates differentially in the barley response to a pathogen and an herbivore. Front. Plant Sci. 12:1585. doi: 10.3389/fpls.2017.01585
Dominguez, F., and Cejudo, F. J. (1999). Patterns of starchy endosperm acidification and protease gene expression in wheat grains following germination. Plant Physiol. 119, 81–88. doi: 10.1104/pp.119.1.81
Domínguez, F., González, M. C., and Cejudo, F. J. (2002). A germination-related gene encoding a serine carboxypeptidase is expressed during the differentiation of the vascular tissue in wheat grains and seedlings. Planta 215, 727–734. doi: 10.1007/s00425-002-0809-2
Feijoo-Siota, L., and Villa, T. G. (2011). Native and biotechnologically engineered plant proteases with industrial applications. Food Biopro. Technol. 4,1066–1088. doi: 10.1007/s11947-010-0431-4
Fernández-Lucas, J., Castañeda, D., and Hormigo, D. (2017). New trends for a classical enzyme: papain, a biotechnological success story in the food industry. Trends Food Sci. Technol. 68, 91–101. doi: 10.1016/j.tifs.2017.08.017
Gass, J., Bethune, M. T., Siegel, M., Spencer, A., and Khosla, C. (2007). Combination enzyme therapy for gastric digestion of dietary gluten in patients with celiac sprue. Gastroenterology 133, 472–480. doi: 10.1053/j.gastro.2007.05.028
Gil-Humanes, J., Pistón, F., Altamirano-Fortoul, R., Real, A., Comino, I., Sousa, C., et al. (2014). Reduced-gliadin wheat bread: an alternative to the gluten-free diet for consumers suffering gluten-related pathologies. PLoS One 9:e90898. doi: 10.1371/journal.pone.0090898
Gil-Humanes, J., Pistón, F., Giménez, M. J., Martín, A., and Barro, F. (2012). The intogression of RNAi silencing of γ-gliadins into commercial lines of bread wheat changes the mixing and technological properties of the dough. PLoS One 7:e45937. doi: 10.1371/journal.pone.0045937
Gil-Humanes, J., Pistón, F., Tollefsen, S., Sollid, L. M., and Barro, F. (2010). Effective shutdown in the expression of celiac disease-related wheat gliadin T-cell epitopes by RNA interference. Proc. Natl. Acad. Sci. U.S.A. 107,17023–17028. doi: 10.1073/pnas.1007773107
Gomez-Sanchez, A., Gonzalez-Melendi, P., Santamaria, M. E., Arbona, V., Lopez-Gonzalvez, A., Garcia, A., et al. (2019). Repression of drought-induced cysteine-protease genes alter barley leaf structure and the response to abiotic and biotic stresses. J. Exp. Bot. 70, 2143–2155. doi: 10.1093/jxb/ery410
Grudkowska, M., and Zagdańska, B. (2004). Multifunctional role of plant cysteine proteinases. Acta Biochim. Pol. 51, 609–624.
Hara-Nishimura, I., Kinoshita, T., Hiraiwa, N., and Nishimura, M. (1998). Vacuolar processing enzymes in protein-storage vacuoles and lytic vacuoles. J. Plant Physiol. 152, 668–674. doi: 10.1016/S0176-1617(98)80028-X
Hara-Nishimura, I., Shimada, T., Hiraiwa, N., and Nishimura, M. (1995). Vacuolar processing enzyme responsible for maturation of seed protein. J. Plant Physiol. 145, 6412–6417. doi: 10.1016/S0176-1617(11)81275-7
Hartmann, G., Koehler, P., and Wieser, H. (2006). Rapid degradation of gliadin peptides toxic for celiac disease patients by proteases from germinating cereals. J. Cereal Sci. 44, 368–371. doi: 10.1016/j.jcs.2006.10.002
Holwerda, B. C., and Rogers, J. C. (1992). Purification and characterization of aleurain: a plant thiol protease functionally homologous to mammalian cathepsin h. Plant Physiol. 99, 848–855. doi: 10.1104/pp.99.3.848
James, M., Masclaux-Daubresse, C., Marmagne, A., Azzopardi, M., Laîné, P., Goux, D., et al. (2019). A new role for SAG12 cysteine protease in roots of Arabidopsis thaliana. Front. Plant Sci. 9:1998. doi: 10.3389/fpls.2018.01998
Jouanin, A., Boyd, L., Visser, R. G. F., and Smulders, M. J. M. (2018). Development of wheat with hypoimmunogenic gluten obstructed by the gene editing policy in Europe. Front. Plant Sci. 9:1523. doi: 10.3389/fpls.2018.01523
Julián, I., Gandullo, J., Santos-Silva, L. K., Diaz, I., and Martínez, M. (2013). Phylogenetically distant barley legumains have a role in both seed and vegetative tissues. J. Exp. Bot. 64, 2929–2941. doi: 10.1093/jxb/ert132
Kinoshita, T., Yamada, K., Hiraiwa, N., Nishimura, M., and Hara-Nishimura, I. (1999). Vacuolar processing enzyme is up-regulated in the lytic vacuoles of vegetative tissues during senescence and under various stressed conditions. Plant J. 19, 43–53. doi: 10.1046/j.1365-313X.1999.00497.x
Kiyosaki, T., Matsumoto, I., Asakura, T., Funaki, J., Kuroda, M., Misaka, T., et al. (2007). Gliadain, a gibberellin-inducible cysteine proteinase occurring in germinating seeds of wheat, Triticum aestivum L., specifically digests gliadin and is regulated by intrinsic cystatins. FEBS J. 164, 470–477. doi: 10.1111/j.1742-4658.2007.05749.x
Koehler, S. M., and Ho, T. D. (1988). Purification and characterization of gibberelic acid-induced cysteine endoproteases in barley aleurone layers. Plant Physiol. 87, 251–258. doi: 10.1104/pp.87.1.95
Koehler, S. M., and Ho, T. D. (1990a). A major gibberellic acid-induced barley aleurone cysteine proteinase which digests hordein. Plant Physiol. 94, 251–258. doi: 10.1104/pp.94.1.251
Koehler, S. M., and Ho, T. D. (1990b). Hormonal regulation, processing, and secretion of cysteine proteinases in barley aleurone layers. Plant Cell 2, 769–783. doi: 10.1105/tpc.2.8.769
Li, Z., Tang, L., Qiu, J., Zhang, W., Wang, Y., Tong, X., et al. (2016). Serine carboxypeptidase 46 regulates grain filling and seed germination in rice (Oryza sativa L.). PLoS One 11:e0159737. doi: 10.1371/journal.pone.0159737
Linnestad, C., Doan, D. N. P., Brown, R. C., Lemmon, B. E., Meyer, D. J., Jung, R., et al. (1998). Nucellain, a barley homolog of the dicot vacuolar-processing protease is localized in nucellar cell walls. Plant Physiol. 118, 1169–1180. doi: 10.1104/pp.118.4.1169
Liu, H., Hu, M., Wang, Q., Cheng, L., and Zhang, Z. (2018). Role of papain-like cysteine proteases in plant development. Front. Plant Sci. 9:1717. doi: 10.3389/fpls.2018.01717
Martínez, M., Cambra, I., Carrillo, L., Diaz-Mendoza, M., and Diaz, I. (2009). Characterization of the entire cystatin gene family in barley and their target cathepsin L-like cysteine proteases, partners in the hordein mobilization during seed germination. Plant Physiol. 151, 1531–1545. doi: 10.1104/pp.109.146019
Martínez, M., and Díaz, I. (2008). The origin and evolution of plant cystatins and their target cysteine proteinases indicate a complex functional relationship. BMC Evol. Biol. 8:198. doi: 10.1186/1471-2148-8-198
Martínez, M., Rubio-Somoza, I., Carbonero, P., and Diaz, I. (2003). A cathepsin B-like CysProt gene from Hordeum vulgare (gene catb) induced by GA in aleurone cells is under circadian control in leaves. J. Exp. Bot. 54, 951–959. doi: 10.1093/jxb/erg099
Mikkonen, A., Porali, I., Cercós, M., and Ho, T. D. (1996). Major cysteine proteinase, EPB, in germinating barley seeds: structure of two intronless genes and regulation of expression. Plant Mol. Biol. 31, 239–254. doi: 10.1007/BF00021787
Misas-Villamil, J. C., Van Der Hoorn, R. A., and Doehlemann, G. (2016). Papainlike cysteine proteases as hubs in plant immunity. New Phytol. 212, 902–907. doi: 10.1111/nph.14117
Müntz, K., Blattner, F. R., and Shutov, A. D. (2002). Legumains – a family of asparagine-specific cysteine endopeptidases involved in propolypeptide processing and protein breakdown in plants. J. Plant Physiol. 160, 1281–1293. doi: 10.1078/0176-1617-00853
Osorio, C. E., Wen, N., Mejias, J. H., Liu, B., Reinbothe, S., von Wettstein, D., et al. (2019). Development of wheat genotypes expressing a glutamine-specific endoprotease from barley and a prolyl endopeptidase from Flavobacterium meningosepticum or Pyrococcus furiosus as a potential remedy to celiac disease. Funct. Integr. Genomics 19:123. doi: 10.1007/s10142-018-0632-x
Poulle, M., and Jones, B. L. (1988). A proteinase from germinating barley. Purification and some physical properties. J. Plant Physiol. 88, 1454–1460. doi: 10.1104/pp.88.4.1454
Prabucka, B., and Bielawski, W. (2004). Purification and partial characteristic of major gliadin-degrading cysteine endopeptidase from germinating triticale seeds. Acta Physiol. Plant. 26, 383–391. doi: 10.1007/s11738-004-0027-6
Radchuk, V., Tran, V., Radchuk, R., Diaz-Mendoza, M., Weier, D., Fuchs, J., et al. (2018). Vacuolar processing enzyme 4 controls grain size in barley by executing programmed cell death in pericarp. New Phytol. 218, 1127–1142. doi: 10.1111/nph.14729
Radchuk, V., Weier, D., Radchuk, R., Weschke, W., and Weber, H. (2011). Development of maternal seed tissue in barley is mediated by regulated cell expansion and cell disintegration and coordinated with endosperm growth. J. Exp. Bot. 62, 1217–1227. doi: 10.1093/jxb/erq348
Rewers, M. (2005). Epidemiology of celiac disease: what are the prevalence, incidence, and progression of celiac disease? Gastroenterology 128, S47–S51. doi: 10.1053/j.gastro.2005.02.030
Rogers, J. C., Dean, D., and Heck, G. R. (1985). Aleurain: a barley thiol protease closely related to mammalian cathepsin H. Proc. Natl. Acad. Sci. U.S.A. 82, 6512–6516. doi: 10.1073/pnas.82.19.6512
Rubio-Tapia, A., Kyle, R. A., Kaplan, E. L., Johnson, D. R., Page, W., Erdtmann, F., et al. (2009). Increased prevalence and mortality in undiagnosed celiac disease. Gastroenterlogy 137, 88–93. doi: 10.1053/j.gastro.2009.03.059
Ruiz-Carnicer, Á, Comino, I., Segura, V., Ozuna, C. V., Moreno, M. L., López-Casado, M. Á, et al. (2019). Celiac immunogenic potential of α-gliadin epitope variants from Triticum and Aegilops species. Nutrients 22:E220. doi: 10.3390/nu11020220
Sánchez-León, S., Gil-Humanes, J., Ozuna, C. V., Giménez, M. J., Sousa, C., Voytas, D. F., et al. (2018). Low-gluten, nontransgenic wheat engineered with CRISPR/Cas9. Plant Biotechnol. J. 16, 902–910. doi: 10.1111/pbi.12837
Sapone, A., Lammers, K. M., Casolaro, V., Cammarota, M., Giuliano, M. T., De Rosa, M., et al. (2011). Divergence of gut permeability and mucosal immune gene expression in two gluten-associated conditions: celiac disease and gluten sensitivity. BMC Med. 9:23. doi: 10.1186/1741-7015-9-23
Scherf, K. A., Wieser, H., and Koehler, P. (2018). Novel approaches for enzymatic gluten degradation to create high-quality gluten-free products. Food Res. Int. 110, 62–72. doi: 10.1016/j.foodres.2016.11.021
Schuppan, D. (2000). Current concepts of celiac disease pathogenesis. Gastroenterology 119, 234–242. doi: 10.1053/gast.2000.8521
Schuppan, D., Junker, Y., and Barisani, D. (2009). Celiac disease: from pathogenesis to novel therapies. Gastroenterology 137, 1912–1933. doi: 10.1053/j.gastro.2009.09.008
Shewry, P. R., and Halford, N. G. (2002). Cereal seed storage proteins: structures, properties and role in grain utilization. J. Exp. Bot. 53, 947–958. doi: 10.1093/jexbot/53.370.947
Shewry, P. R., Napier, J. A., and Tatham, A. S. (1995). Seed storage proteins: structures and biosynthesis. Plant Cell 7, 945–956. doi: 10.1105/tpc.7.7.945
Shi, C., and Xu, L. L. (2009). Characters of cysteine endopeptidases in wheat endosperm during seed germination and subsequent seedling growth. J. Integr. Plant Biol. 51, 52–57. doi: 10.1111/j.1744-7909.2008.00778.x
Shindo, T., and van der Hoorn, R. A. L. (2008). Papain-like cysprot: key players at molecular battlefields employed by both plants and their invaders. Mol. Plant Pathol. 1, 119–125. doi: 10.1111/j.1364-3703.2007.00439.x
Sreenivasulu, N., Usadel, B., Winter, A., Radchuk, V., Scholz, U., Stein, N., et al. (2008). Barley grain maturation and germination: metabolic pathway and regulatory network commonalities and differences highlighted by new mapman/pageman profiling tools. Plant Physiol. 146, 1738–1758. doi: 10.1104/pp.107.111781
Stenman, S. M., Lindfors, K., Venäläinen, J. I., Hautala, A., Männistö, P. T., Garcia-Horsman, J. A., et al. (2010). Degradation of coeliac disease-inducing rye secalin by germinating cereal enzymes: diminishing toxic effects in intestinal epithelial cells. Clini. Exper. Immunol. 161, 242–249. doi: 10.1111/j.1365-2249.2010.04119.x
Stenman, S. M., Venäläinen, J. I., Lindfors, K., Auriola, S., Mauriala, T., Kaukovirta-Norja, A., et al. (2009). Enzymatic detoxification of gluten by germinating wheat proteases: implications for new treatment of celiac disease. Ann. Med. 41, 390–400. doi: 10.1080/07853890902878138
Szewinska, J., Siminska, J., and Bielawski, W. (2016). The roles of cysteine proteases and phytocystatins in development and germination of cereal seeds. J. Plant Physiol. 207, 10–21. doi: 10.1016/j.jplph.2016.09.008
Tan-Wilson, A. L., and Wilson, K. A. (2011). Mobilization of seed protein reserves. Physiol. Plant. 145, 140–153. doi: 10.1111/j.1399-3054.2011.01535.x
Tornkvist, A., Liu, C., and Moschou, P. N. (2019). Proteolysis and nitrogen: emerging insights. J. Exp. Bot. 70, 2009–2019. doi: 10.1093/jxb/erz024
Tye-Din, J. A., Stewart, J. A., Dromey, J. A., Beissbarth, T., van Heel, D. A., Tatham, A., et al. (2010). Comprehensive, quantitative mapping of T cell epitopes in gluten in celiac disease. Sci. Transl. Med. 2, 1–14. doi: 10.1126/scitranslmed.3001012
van der Hoorn, R. A. (2008). Plant proteases: from phenotypes to molecular mechanisms. Annu. Rev. Plant Biol. 59, 191–223. doi: 10.1146/annurev.arplant.59.032607.092835
van der Hoorn, R. A. L., and Jones, J. D. G. (2004). The plant proteolytic machinery and its role in defense. Curr. Opin. Plant Biol. 7, 400–407. doi: 10.1016/j.pbi.2004.04.003
Velasco-Arroyo, B., Diaz-Mendoza, M., Santamaria, M. E., Gonzalez-Melendi, P., Gomez-Sanchez, A., Arnaiz, A., et al. (2016). Senescence-associated genes in response to abiotic/biotic stresses. Prog. Bot. 79, 89–109. doi: 10.1007/124-2017-1
Velasco-Arroyo, B., Martínez, M., Díaz, I., and Diaz-Mendoza, M. (2018). Differential response of silencing HvIcy2 barley plants against Magnaporthe oryzae infection and light deprivation. BMC Plant Biol. 18:337. doi: 10.1186/s12870-018-1560-6
Washio, K., and Ishikawa, K. (1994). Organ-specific and hormone-dependent expression of genes for serine carboxypeptidases during development and following germination of rice grains. Plant Physiol. 105, 1275–1280. doi: 10.1104/pp.105.4.1275
Keywords: cysteine protease, germination, proteolysis, gluten, celiac disorders
Citation: Martinez M, Gómez-Cabellos S, Giménez MJ, Barro F, Diaz I and Diaz-Mendoza M (2019) Plant Proteases: From Key Enzymes in Germination to Allies for Fighting Human Gluten-Related Disorders. Front. Plant Sci. 10:721. doi: 10.3389/fpls.2019.00721
Received: 22 February 2019; Accepted: 16 May 2019;
Published: 29 May 2019.
Edited by:
Agnieszka Ludwików, Adam Mickiewicz University in Poznań, PolandReviewed by:
Barend Juan Vorster, University of Pretoria, South AfricaPhilippe Etienne, University of Caen Normandy, France
Jerica Sabotič, Jožef Stefan Institute (IJS), Slovenia
Copyright © 2019 Martinez, Gómez-Cabellos, Giménez, Barro, Diaz and Diaz-Mendoza. This is an open-access article distributed under the terms of the Creative Commons Attribution License (CC BY). The use, distribution or reproduction in other forums is permitted, provided the original author(s) and the copyright owner(s) are credited and that the original publication in this journal is cited, in accordance with accepted academic practice. No use, distribution or reproduction is permitted which does not comply with these terms.
*Correspondence: Mercedes Diaz-Mendoza, bWVyY2VkZXMuZGlhei5tZW5kb3phQHVwbS5lcw==