- 1Jiangxi Province Key Laboratory of Watershed Ecosystem Change and Biodiversity, Center for Watershed Ecology, Institute of Life Science and School of Life Sciences, Nanchang University, Nanchang, China
- 2National Ecosystem Research Station of Jiangxi Poyang Lake Wetland, Nanchang, China
- 3Ministry of Education Key Laboratory for Biodiversity Science and Ecological Engineering, Institute of Biodiversity Science, Fudan University, Shanghai, China
Environmental changes, e.g., eutrophication, in aquatic ecosystems can greatly alter light available to submerged macrophytes. In dioecious plants, given potential for sex-specific differences in resource requirements (i.e., high-carbon for seeds vs. high-nitrogen for pollen), females and males are expected to divergently adjust allocations toward resource acquisition structures when resources are limited during growth. Here, Vallisneria spinulosa was used as a representative dioecious submerged macrophyte to detect sex-specific responses to light limitation and assess whether sexual dimorphism varied with resource availability. Plants were grown under varying levels of light availability in nine outdoor mesocosms for 14 weeks. Late in the reproductive season, allocations to vegetative and reproductive traits for both sexes were determined and relative allocation to reproduction vs. vegetative growth was analyzed. Female and male reproductive plants differed in adjustments of resource allocation in response to light availability. Under low light, females showed a smaller reduction in allocation of resources to vegetative tissues and greater leaf area than males, suggesting female plasticity to increase carbon capture. Under low light, males showed a smaller reduction in reproductive allocation than females (flowers and inflorescences in males vs. fruits in females), suggesting that carbon limitation has greater impacts on sexual reproduction by females than males. Our study provides evidence of differences in reproductive costs and currencies for female vs. male reproduction in aquatic macrophytes, as V. spinulosa responded plastically to reduced light, with sexually dimorphic allocation strategies. Sex-related resource currencies are potentially important drivers for sex-specific variations in allocation patterns, with females safeguarding their vegetative carbon-rich biomass to satisfy future fruit and seed production.
Introduction
Most flowering plants are hermaphroditic, with dioecy in only 5 to 6% of angiosperm species (Renner, 2014). Separate sexes have evolved repeatedly from hermaphroditic ancestors, in different lineages, probably in response to selection either for inbreeding avoidance (Charlesworth and Charlesworth, 1978) or sexual specialization (Charnov et al., 1976; Charlesworth, 1999). Transitions from hermaphroditism to dioecy are often related to evolution of sexual dimorphism. Once plants have evolved separated sexes, males and females have distinct roles; therefore, selection may favor divergence in life-history traits to optimize seed and pollen production (Barrett and Hough, 2013), presumably enabling sex-specific strategies of resource acquisition and reallocation.
Sexual dimorphism occurs in many dioecious terrestrial plants, with sexual morphs differing in: number, size and morphology of flowers and inflorescences; rates of growth, time to reproduction and timing of senescence; physiological traits, e.g., rates of photosynthesis and water uptake; and allocation toward life-history traits and anti-herbivore defenses (reviewed by Ågren et al., 1999; Dawson and Geber, 1999; Delph, 1999; Eckhart, 1999). Degree of sexual dimorphism varies among plant and animal species (Harris and Pannell, 2010; Laiolo et al., 2013; Tonnabel et al., 2014) and among populations within a species (Teder and Tammaru, 2005; Tonnabel et al., 2017).
Whereas sexual selection resulting from variation among individuals in mating success is the most likely cause of sexual dimorphism in animals (Ågren et al., 1999), evolution of sexually dimorphic characters in plants probably occurs due to differential resource requirements for reproduction. Female function (i.e., seed and fruit production) generally requires much carbon (Antos and Allen, 1990; McDowell et al., 2000), whereas male function probably has larger nitrogen requirements for pollen production (Harris and Pannell, 2008; Van Drunen and Dorken, 2012). Given female and male reproduction are differently limited by contrasting resources or “currencies,” selection on resource-harvesting traits likely differs between sexes, potentially eliciting sex-specific differences in “somatic cost of reproduction” for such different types of currencies (Obeso, 2002).
The differential plasticity hypothesis proposed that males and females differ in plastic responses to environmental factors, causing variation in degree of sexual dimorphism across environmental gradients (Delph and Bell, 2008). Divergent resource currency requirements for female vs. male reproduction may result in evolution of sex-specific plasticity in resource acquisition traits in response to environmental changes in resource availability (i.e., carbon and nitrogen). Previous studies examining the differential plasticity hypothesis focused on effects of nitrogen limitation from the perspective of relative resource investment in functionally different organs. For example, under low-nitrogen conditions, males likely benefit more than females from increasing allocation to belowground structures, as they harvest nitrogen needed for pollen production (Harris and Pannell, 2008; Teitel et al., 2016). However, some studies have also found that under dune soils, characterized by low-nitrogen contents, female plants showed greater belowground biomass than males (Sanchez-Vilas et al., 2012). If reproductive effort in females is limited more by carbon than nitrogen, females may exhibit plasticity in growth when exposed to carbon limitation to secure more carbon than males to ensure future carbon-rich reproduction. Females may also deploy sufficient resources to aboveground vegetative organs before involving carbon allocation toward flowering, as a way to maximize carbon acquisition. However, sex-specific variations in reproductive effort in response to a carbon limitation, which potentially limits female function, is not well understood (Tonnabel et al., 2017).
Submerged macrophytes play an important role in maintaining healthy aquatic ecosystems. Eutrophication and degraded underwater light climate is one of the fundamental reasons causing worldwide disappearance of submerged macrophytes (Schelske et al., 2010; Zhang et al., 2017). Variations in light reception influence the potential of a plant to capture carbon, which may limit plant growth and photosynthesis. Substantial phenotypic plasticity for resource capture is important for submerged macrophytes (Barrett et al., 1993; Going et al., 2008; Hyldgaard and Brix, 2012), enabling them to be more competitive (Chambers and Kalff, 1987). For instance, submerged species are highly responsive to light shortage and can have morphological and structural adaptations over the period of growth to optimize light utilization by increasing shoot length, decreasing branches, producing longer, wider and thinner leaves and changing resource allocation to various plant structures (Riis et al., 2012; Schneider et al., 2015; Chen et al., 2016). Numerous studies have been conducted to address the responses of submerged macrophytes to decreased light, albeit mostly from the perspective of growth conditions. To our knowledge, whether male and female plants are optimized by different resource allocation strategies in response to altered light availability is not well known. Thus, we herein address the responses of submerged macrophytes to limited light conditions from the perspective of sexual dimorphism.
In shallow freshwaters, substantial changes in light availability in populations of submerged macrophytes could be induced by eutrophication (Cao et al., 2011; Zhang et al., 2016). Plants generally respond plastically to changed resource availability during growth, even after reproduction commences. However, whether such a response is sexually dimorphic and reflects expectations based on potential existence of sex-specific resource currency requirements, remains to be investigated. Here, we used Vallisneria spinulosa as a representative dioecious submerged macrophyte to investigate potential responses in vegetative and reproductive traits to light availability and to evaluate patterns of sexual dimorphism, based on the differential plasticity hypothesis. In an aquatic mesocosm experiment that varied light availability of plants during their growing season, we tested three hypotheses: (1) females respond to reduced light by decreasing allocation toward aboveground growth less than males to satisfy carbon demands for future reproduction; (2) with increasing light limitation, females decrease allocation toward sexual reproduction more than males to ensure future capacity for carbon acquisition; and (3) sexual dimorphism in resource allocation is enhanced under stressful environmental conditions (e.g., limited light). Our results demonstrated that variations in light availability differentially affected males and females in their ability to perform photosynthesis, with implications for further understanding the effects of eutrophication on submerged macrophytes and also evolution of aquatic plants to varied environments.
Materials and Methods
Study Species
Vallisneria spinulosa S. Z. Yan (Hydrocharitaceae) is a dioecious, rosette-forming clonal submerged macrophyte with a hydrophilous pollination system. Light interception and utilization in V. spinulosa are determined by its long, strap-shaped leaves rather than by its short basal meristem stem; therefore, leaf growth impacts survival and reproduction. This plant commonly occurs in ponds, streams and lakes in mid-lower reaches of the Yangtze River in China (Wang et al., 2010) and grows well in waters with medium turbidity (Chambers and Kalff, 1987). It provides food for waterfowl, nursery habitats for fish and a substrate for invertebrates, and may modulate water quality (Wang et al., 2010).
In common with other Vallisneria species (Sculthorpe, 1967; Cox, 1988), female flowers develop underwater and are brought to the surface singly on elongated slender peduncles and at anthesis float on the surface. Male inflorescences (spathes) are located among bases of leaves with hundreds of packed male flowers. At maturity, male flowers are released to the water surface and aggregate around female flowers. Pollinated flowers are retracted underwater via a helix of peduncles of female flowers, where fleshy fruit develop. Clonal growth results from production of rosette-like ramet along elongating stolons and in the autumn, ramets produce several tubers that overwinter in sediment and germinate the following spring.
Tuber Collection
Numerous V. spinulosa tubers were randomly collected (February 2017) from a large dioecious population at Bang Lake (115°55′–116°06′E, 29°11′–29°18′N; average water depth, 3.0 m) in Poyang Lake National Nature Reserve (Jiangxi Province, China, 115°55′–116°03′E, 29°05′–29°15′N). The tubers could not be sexed when they were collected. Tubers were cleaned, transported to the laboratory and kept in a water-filled plastic container maintained in darkness at 4°C for approximately 4 months.
Experimental Design
To investigate sex-specific patterns of phenotypic plasticity in response to light availability and how sexual dimorphism in resource allocation varied across light gradients, an outdoor experiment was conducted in nine concrete mesocosms (2 × 2m, with water 1.6 m deep) at the Poyang Lake Laboratory for Wetland Ecosystem Research, Chinese Academy of Sciences (116°03′E, 29°26′N) located in Lushan City, China. Initially, 234 mature V. spinulosa tubers of uniform size (mean fresh mass ± SE = 0.906 ± 0.146 g; range of tuber mass = 0.722–1.335 g) were individually planted into plastic pots (23 cm diameter and 17 cm deep) containing a 4:1 mixture of Poyang Lake sediment and fine sand. Sediment was dried, ground and filtered through a sieve with a 0.5-cm mesh. Water (TN: 1.50 mg l−1; TP: 0.05 mg l−1; Chla: 6.6 μg l−1) was pumped from Poyang Lake and filtered (64-μm diameter net). There were three levels of light availability in a randomized block design, with each of the three blocks containing one replicate of each treatment. Six mesocosms were covered with black neutral shade nets to achieve the following light levels as percent of incoming light: 20% and 60% ambient light, representing low and medium light intensity, respectively. The other three mesocosms were unshaded and thus received 100% of ambient light, representing high light intensity. There were 234 pots randomly assigned to nine concrete mesocosms. For each light treatment, three replicate mesocosms were used and each mesocosm contained 26 pots (n = 78 pots/treatment). Each pot was tied with a nylon rope to a galvanized metal tube, suspended at 60 cm water depth. To renew water and maintain a consistent level, two or three times weekly, water from a common header tank was added to each mesocosm. Epiphyton on the leaves of V. spinulosa was removed with a soft brush every month to exclude shading of epiphyton on macrophyte. Plants grew in mesocosms for 14 weeks during summer and autumn (4 June to 10 September 2017). Sexual dimorphism is inconspicuous in pre-reproductive juveniles and plants can only be accurately sexed in V. spinulosa a few days after onset of flowering, based on spathe morphology. Therefore, because we used randomly chosen plants once tubers geminated, sex ratios of flowering plants in each treatment showed a strong male bias (average sex ratio = 2.87).
Photosynthetically active radiation (PAR) was measured with a Li-COR UWQ-192SA sensor coupled with a Li-1400 data logger (Li-Cor, Lincoln, NE, United States) at 10:00–12:00 under clear skies on 16 July 2017. PAR at water surface in low to high light regimes was 436.82 ± 6.45, 889.17 ± 16.45 and 1787.89 ± 18.75 μmol m−2 s−1 (mean ± SE, n = 9), respectively. For sediment in pots, contents of total N, total P and organic matter were 2.45 mg g−1, 0.73 mg g−1 and 6.61% (n = 3 pots).
Allocation Measurements
At fruit maturation (98 days after planting), above- and below-ground portions of well-developed, intact plants of both sexes in each mesocosm were harvested. Final numbers of harvested male plants were 59, 44, and 35 for low, medium, and high light treatments, respectively, and those of harvested female plants were 19, 22, and 10. Because some stunted plants grew very poorly and did not flower and others were grazed by aquatic insects, only plants that grew vigorously and flowered were harvested. After removal from sediments, plants were carefully washed with tap water.
To assess male and female resource allocations, traits for both growth and reproduction were measured. Plant height, ramet number, shoot biomass (i.e., leaf biomass), leaf number and leaf area are key traits related to plant size and competitive interactions for light. Plant height was measured as length of the longest leaf. Total number of leaves for 10–15 plants per treatment were recorded and entire leaf surface area for each plant determined with an AM-350 Leaf Area Meter (ADC Bioscientific, Hoddesdon, United Kingdom). To determine percentage of flowering ramets, total and flowering ramet numbers for each plant were counted. Number of tubers and for males, number of spathes (including intact spathes and pedicles of spathes that had fallen off) and for females, number of fruits, were counted. Every plant was separated into three components: vegetative biomass (including leaves, roots, and stolons); sexual reproductive biomass (spathes or peduncles and fruits); and clonal reproductive biomass (tubers). To determine dry mass for each component, samples were dried at 75°C for 3 days and then weighed on a SI-114 four-decimal place gram balance (Denver Instrument, Denver, CO, United States). Because dispersal of pollen from some male spathes occurred when plants were harvested, we randomly sampled 10 non-dehiscent, mature male spathes from five male plants within each light regime and weighed each spathe individually after drying. Data from these 10 male spathes obtained in each light regime were averaged and multiplied by the total number of spathes produced per male to estimate biomass of sexual reproductive tissues of male plants for each light regime. In this study, dry mass of leaves, roots, and stolons (i.e., non-reproductive parts) was combined as vegetative investment (i.e., vegetative growth). Sexual reproductive effort was calculated by dividing total mass of male spathes or female peduncles and fruits by resources allocated to the entire plant. Allocation to clonal reproduction was determined by dividing tuber biomass by total biomass.
Statistical Analyses
To investigate if sexual dimorphism in resource allocation varied with light availability, a Restricted Maximum Likelihood (REML) linear mixed model was used. For this model, sex and light treatment were fixed factors and block was random factor, with each life-history trait analyzed independently. For all traits, the interaction between sex and light pointed to significant effect of light on sexual dimorphism. Post hoc comparisons among groups used a Tukey honestly significant difference (HSD) test procedure (α < 0.05). To investigate our differential plasticity hypothesis, for each sex, relative allocation to vegetative and sexual reproductive biomass was calculated as percentage change in biomass under low light compared to mean allocation under high light. Tests of equality of means between sexes were done with a Student’s t-test (α = 0.05). All analyses used SPSS software, version 19.0 (SPSS Inc., Chicago, IL, United States).
Results
Sexual Dimorphism in Aboveground Growth and Reproductive Traits
Of traits representing allocation to growth, all but one, “plant height,” had significant sexual dimorphism (Table 1). Under high or medium light, all vegetative traits showed a non-significant tendency to be greater in females compared to males (Figure 1). Under low light, females exhibited significantly greater investment in leaf and vegetative biomass, ramet number, leaf number, and leaf area than males (Figures 1B–F).
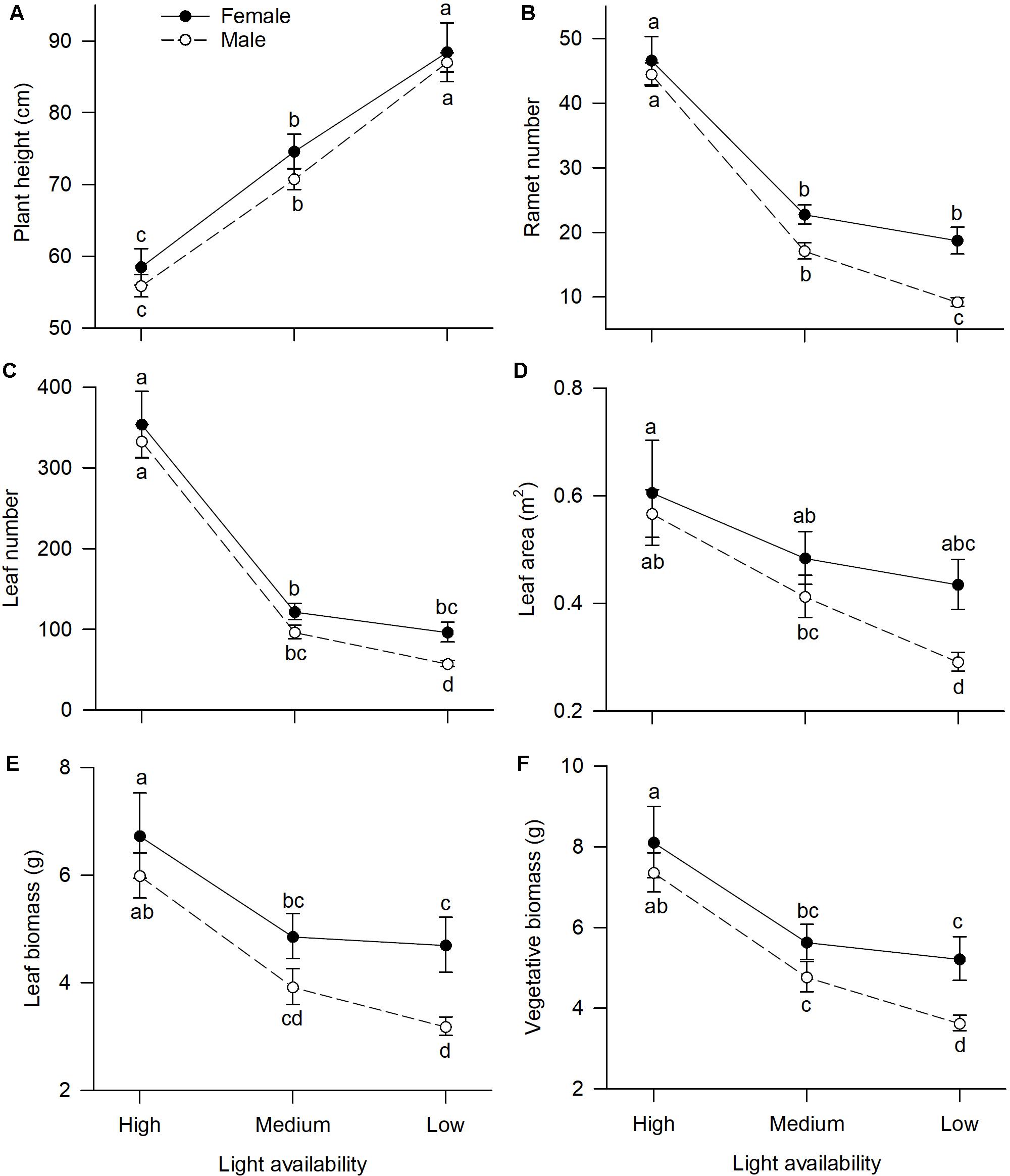
Figure 1. Effects of light availability on allocation to vegetative tissues for both males and females of V. spinulosa at reproductive maturity: (A) plant height, (B) ramet number, (C) leaf number, (D) leaf area, (E) leaf biomass, and (F) vegetative biomass. Letters denote significant difference (P < 0.05), using Tukey HSD comparisons. Error bars show ± SE. Curves are usually used to display differences between sexes under different environmental conditions (Harris and Pannell, 2008; Hesse and Pannell, 2011).
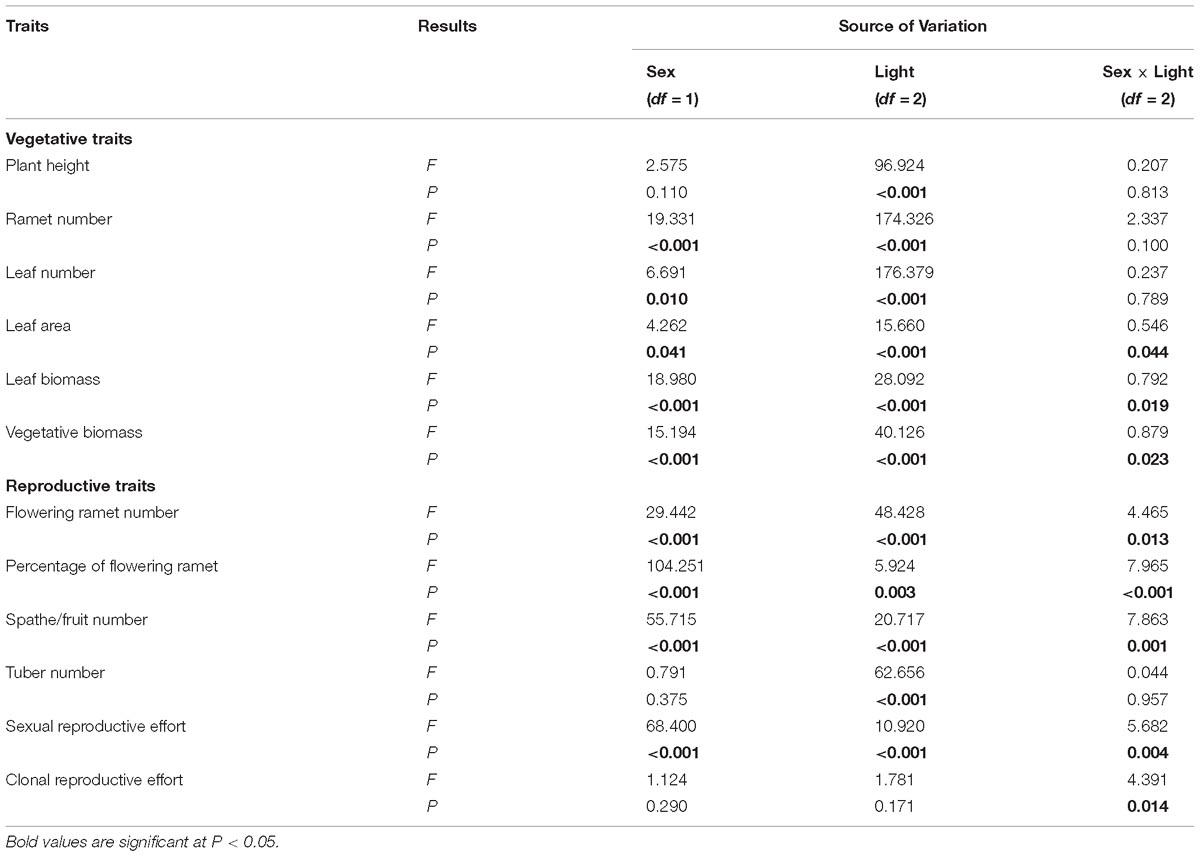
Table 1. Results of the Restricted Maximum Likelihood (REML) linear mixed model analyses examining effects of sex and light treatment on traits of V. spinulosa.
All measured reproductive traits, except those reflecting allocation toward clonal propagation (tuber number, clonal reproductive effort) displayed significant sexual dimorphism (Table 1). Males had a higher percentage of flowering ramets than females, but only in medium or low light treatments (Figure 2B). Males produced more flowering ramets and inflorescences than females under high or medium light (Figures 2A,C), but there was no significant difference between sexes under low light. In contrast, females invested proportionally more resources in sexual reproductive structures than males across all light treatments (Figure 2E).
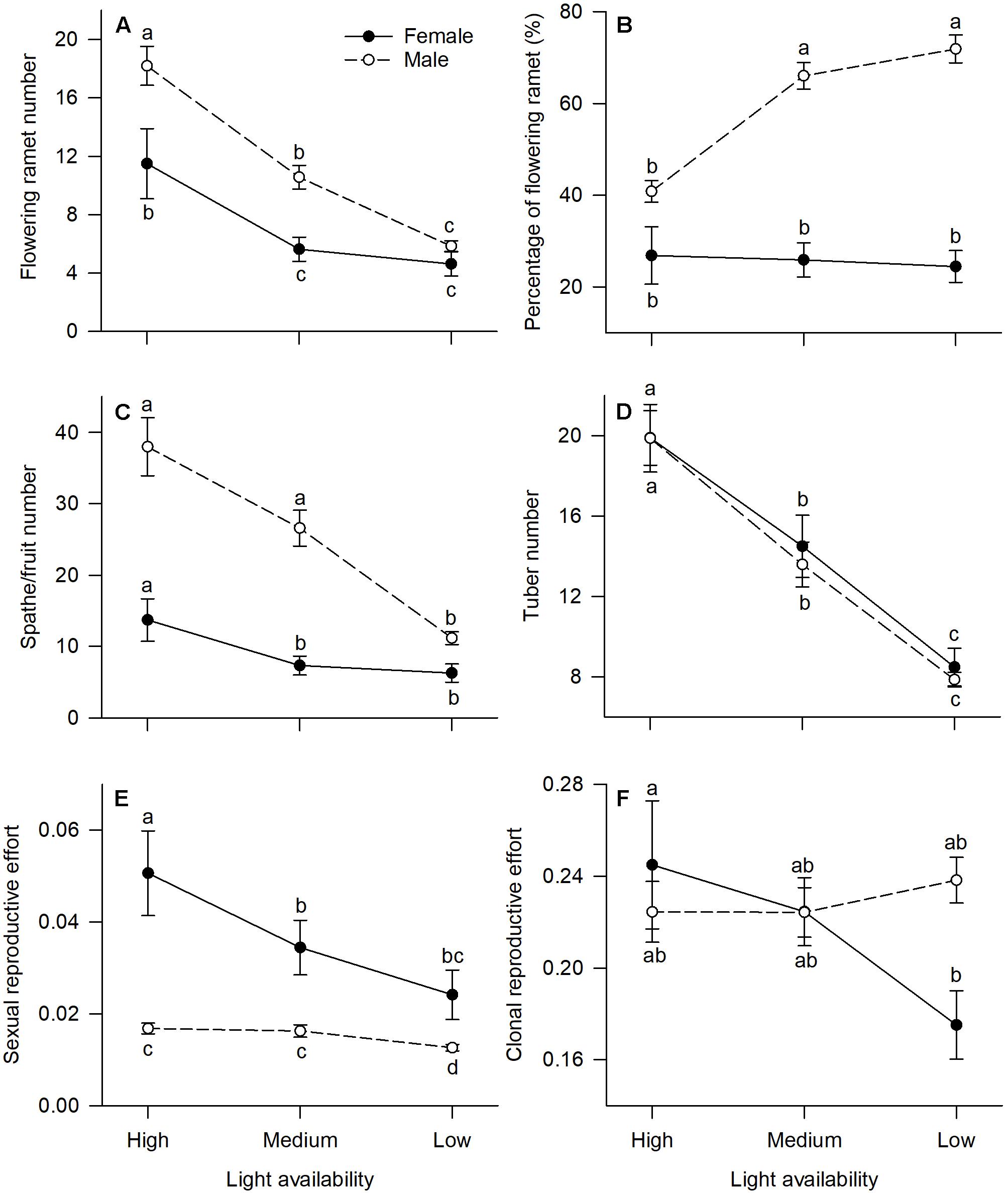
Figure 2. Effects of light availability on allocation to reproductive tissues for males and females of V. spinulosa at reproductive maturity: (A) flowering ramet number, (B) percentage of flowering ramet, (C) spathe/fruit number, (D) tuber number, (E) sexual reproductive effort, and (F) clonal reproductive effort. For each individual sexual and clonal reproductive efforts were calculated as the ratio between dry mass of the respective tissues (male: spathes; female: fruits) and total biomass. Letters denote significant difference (P < 0.05), using Tukey HSD comparisons. Error bars show ± SE. Curves are usually used to display differences between sexes under different environmental conditions (Harris and Pannell, 2008; Hesse and Pannell, 2011).
Sex-Specific Plasticity in Response to Variable Light
Light availability differentially affected males and females both in leaf biomass (sex × light interaction: P = 0.019; Table 1 and Figure 1E) and vegetative biomass (sex × light interaction: P = 0.023; Table 1 and Figure 1F). Males and females reduced leaf and vegetative biomass with decreasing light intensity; however, males did so more than females (Figures 1E,F). Leaf area also exhibited sex-differential plasticity in response to light availability (sex × light interaction: P = 0.044; Table 1 and Figure 1D). Whereas female leaf area was independent of light availability, males decreased their leaf area under low light (Figure 1D). Males and females both increased plant height with decreasing light (Figure 1A), whereas ramet number and leaf number had an opposite pattern (Figures 1B,C). Light availability did not affect sexual dimorphism in plant height (sex × light interaction: P = 0.813; Table 1 and Figure 1A), ramet number (sex × light interaction: P = 0.100; Table 1 and Figure 1B), and leaf number (sex × light interaction: P = 0.789; Table 1 and Figure 1C).
In both males and females, flowering ramet and inflorescence number were significantly reduced in low light (Figures 2A,C), but males had steeper decreases in flowering ramets (sex × light interaction: P = 0.013; Table 1 and Figure 2A) and inflorescences (sex × light interaction: P = 0.001; Table 1 and Figure 2C) than females, as light intensity decreased. Number of tubers in both sexes was reduced similarly with decreasing light (sex × light interaction: P = 0.957; Table 1 and Figure 2D).
Growth vs. Reproductive Allocation
The degree of sexual dimorphism in sexual reproductive effort (proportion of biomass allocated to sexual reproductive structures) varied with light treatment (sex × light interaction: P = 0.004; Table 1 and Figure 2E). Both males and females displayed plastic responses in their relative allocation to sexual reproductive vs. vegetative tissues; this ratio was significantly lower in low light for both sexes (Male: post hoc comparison: P = 0.007; Female: post hoc comparison: P = 0.047; Figure 2E). However, decreased light caused females to reduce relative allocation to sexual reproduction more than males (Figure 2E). Vegetative biomass tended to decrease less in females than in males under lower light levels (Figure 3A), whereas allocation toward sexual reproductive biomass under lower light levels tended to decrease more in females than in males (Figure 3B). Whereas females reduced clonal reproductive effort under limited light (post hoc comparison: P = 0.028; Figure 2F), males maintained the same ratio among all light conditions (post hoc comparison: P = 0.564; Figure 2F).
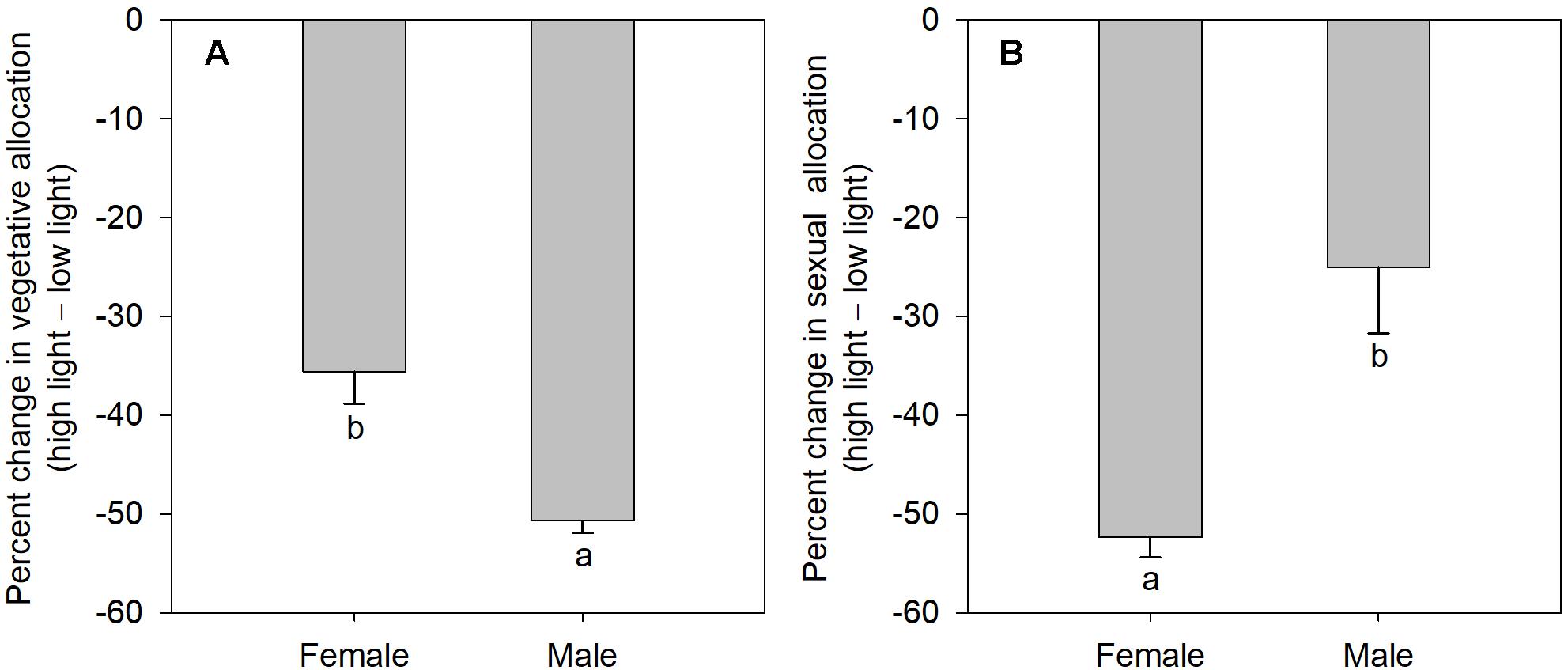
Figure 3. The mean percentage change in vegetative and sexual reproductive tissues under low light regime relative to their average allocation under high light regime; values are means ± SE, for males and females of V. spinulosa. (A) Vegetative and (B) sexual reproductive allocations were calculated as percentage change in biomass of the respective tissues compared to the mean allocation to these tissues under high light regime for each sex. Values with different letters denote statistically significant differences between sexes (P < 0.05), calculating using likelihood ratio test.
Discussion
Effects of altered light availability on performance of submerged macrophytes are well known (e.g., Xie et al., 2007; Riis et al., 2012; Yuan et al., 2012; Eller et al., 2015), but sex-specific plasticity in sexual dimorphism in response to varied light levels over an entire growing season has not been well characterized. The present study provides evidence supporting the differential plasticity hypothesis in aquatic environment, particularly in the context of sex-specific resource requirements, with insights into evolution of sexual dimorphism in dioecious macrophytes. Reproductive males and females differentially adjusted resource allocation to reproduction and aboveground vegetative growth in response to decreased underwater light availability. Whereas females showed a greater allocation of resources to vegetative tissues and leaf area than males under limited light, males showed a smaller reduction of resource allocation to sexual reproduction than females. Because carbon acquisition is more critical for female reproduction, under conditions of light limitation females are expected to decrease their reproductive allocation more than males, as a way to conserve resources for vegetative organs to ensure their carbon needs for future reproduction. Relevant results can help further understanding of the effects of eutrophication on submerged macrophytes and evolution of aquatic plants to varied environments.
Plastic Resource Allocation With Varied Light
In our experiment, resource allocation in V. spinulosa was highly responsive to a change in light availability. Although various macrophyte species may respond differently to light deficiency (Chambers, 1987), decreased light in water often reduces coverage and decreases growth of macrophytes in the littoral zone and vice versa (Vallisneria americana, French and Moore, 2003; Vallisneria natans, Chen et al., 2016). In our experiment, both vegetative and reproductive traits tended to decrease as light decreased from 100% to 20% incident light. Unlike canopy formers (e.g., Potamogeton maackianus), V. spinulosa, being rosette-forming, cannot rapidly elongate to reach the water surface where light availability is high. Thus, aboveground vegetative growth (leaf biomass) of V. spinulosa declined in limited light condition, as also reported in V. americana (French and Moore, 2003) and V. natans (Fox et al., 2013) in adaptation experiments to shading.
A plastic response to decreased light in V. spinulosa involved linear increases in plant height through leaf elongation, decreased ramet number and leaf number and thereby decreased leaf area. Thus, reproductively mature plants showed less lateral growth and more elongated growth with decreasing light gradients, resulting in a shift of leaf area into better illuminated areas (Valladares and Niinemets, 2008). Such a plastic response has the same effect as foraging concept in most herbaceous terrestrial plants with erect stems, which hypothesizes that plants develop longer stems and less branches in low-light conditions to improve resource acquisition and reduce respiratory cost (de Kroon and Hutchings, 1995). Greater resource allocation to vertical growth also involved a trade-off, causing decreased resource allocation to reproduction, as reported (Harper, 1977), but decreasing total plant biomass. In the present study, plants also responded plastically to reduced light by altering resource allocation to reproductive traits. A negative effect on sexual reproduction is likely to influence seed dispersal, re-establishment after disappearance and may reduce resistance to other environmental stressors, due to lower genetic diversity (Boedeltje et al., 2008).
Evidence of Differential Plasticity Hypothesis in Macrophytes
Our study highlighted flexibility of plants in resource allocation and how reproductive male and female plants responded plastically to light stress with resource allocation that maximized fitness. The differential plasticity hypothesis has been tested in several terrestrial plants (e.g., Silene latifolia, Lovett Doust et al., 1987; Mercurialis annua, Harris and Pannell, 2008; Rumex hastatulus, Teitel et al., 2016), with mixed results. Regarding the differential plasticity hypothesis, we emphasized plant flexibility in responding to local environments, particularly in varying allocation to growth vs. reproduction. Greater sexual dimorphism in vegetative tissues and smaller sexual dimorphism in resource allocation to sexual reproduction for dioecious V. spinulosa under low light was consistent with the differential plasticity hypothesis.
Flexible reproductive strategies are likely an adaptive advantage in aquatic environments, which often have strong spatio-temporal heterogeneity (Eckert et al., 2016). In aquatic ecosystems, environmental heterogeneity, e.g., eutrophication, can strongly affect access to light for submerged macrophytes, similar to that we manipulated in our study. We inferred plasticity in sexual dimorphism is advantageous to aquatic plants in the face of adverse environmental conditions caused by eutrophication, such as high turbidity and low irradiance penetration. In accordance with the differential plasticity hypothesis, males and females of V. spinulosa differentially adjusted their relative allocation of resources among vegetative and reproductive tissues with decreasing underwater light availability, reflecting sex-specific adaptation. Divergent plastic responses of male and female plants in vegetative and reproductive traits implied a potential reason for intraspecific variation in sexual dimorphism in resource allocation under light limitation (i.e., more stressful environment).
More specifically, decreased light for a submerged plant during its life cycle, as in our study, could be induced by re-suspension of sediment, variations in periphyton and phytoplankton abundance, or by concentrations of dissolved materials due to nutrient enrichment. If resource limitation elicits sex-specific plastic responses, spatial variation in this resource will cause spatial variation in degree of sexual dimorphism (Barrett and Hough, 2013). There is also ample evidence that higher reproductive expenditure and/or greater sensitivity to stress in females should result in more male-biased sex ratios among populations of a species along gradients of resource availability (e.g., Pickering and Hill, 2002; Li et al., 2007). Our results reflect environmental variation between sexes in their allocation to vegetative tissues and reproduction and provide support for differential plasticity contributing to variations in sexual dimorphism in aquatic macrophytes. A growing evidence from studies of sexual dimorphism under varying resource levels supported the differential plasticity hypothesis (Harris and Pannell, 2008; Hesse and Pannell, 2011; Teitel et al., 2016; Li et al., 2019). However, details of how resource currencies are differently reallocated from sources to sinks and how patterns of variation in sexual dimorphism are driven by different resource currencies, remain largely unknown.
Sex-Specific Strategies of Resource Allocation
Our results illustrated that high levels of plasticity in response to the environment affected traits that can be sexually dimorphic in dioecious plants; therefore, indices of dimorphism themselves become plastic. In V. spinulosa, how resource currencies were reallocated from sources to sinks was altered in response to light limitation, consistent with responses in dioecious M. annua (Tonnabel et al., 2017). Sex-specific allocation patterns in M. annua vary temporally and also respond to environmental heterogeneity (Harris and Pannell, 2008). Males and females used disparate resource allocation strategies, exhibiting sex-specific resource trade-offs, which can involve disparate resource currencies in females and males. In many dioecious plants, female reproductive costs may exceed those of males, due to high investments in carbon-rich fruits and seeds (Barrett and Hough, 2013). In particular, flowering in many submerged macrophytes requires considerable investment in reproductive accessory structures, e.g., production of peduncles in female V. spinulosa, to bear flowers at or above the water surface. Reproductive costs result in physiological trade-offs in resource distributions, which can influence future vegetative growth and reproduction. Therefore, females of V. spinulosa are expected to have stronger trade-offs with other life-history traits, due to their typically higher investment in sexual reproduction.
Our results conformed to expectations arising from the differential plasticity hypothesis; namely, that females maximize allocation to aboveground growth under conditions of light limitation more than males, as carbon acquisition is more critical for female reproduction. This pattern was due to a stronger reduction of allocation to reproductive tissues in females than in males and a smaller reduction of allocation to vegetative tissues in females than in males when light availability decreased. This points to existence of a greater trade-off in females than males between current and future reproduction in terms of carbon; with limited light, females decreased sexual reproductive effort more than males. This response may be due to a difference in resource currencies that limit female vs. male functions, e.g., carbon may limit reproduction by females more than that by males (Antos and Allen, 1990; McDowell et al., 2000). Perhaps carbon limitation causes a smaller decrease in allocation to carbon-harvesting organs (i.e., shoot and leaves) in females than in males, as females probably safeguard capacity to acquire carbon for future seed and fruit production.
Consistent with our results, similar evidence on sex-specific resource (carbon) budgets and differential allocation strategies in response to low light have been reported. For example, females reduced their allocation to vegetative growth in response to competition for light less than males (Lovett Doust et al., 1987; Tonnabel et al., 2017), perhaps due to a higher total carbon cost for female reproduction. In contrast, in some studies, females experienced a steeper or similar decrease in resource allocation to aboveground biomass in response to reduced access to light (Hesse and Pannell, 2011; Labouche and Pannell, 2016). These observations were contrary to the expectation, on the basis of a differential somatic cost of carbon between sexes, that females reduce aboveground biomass less than males when carbon becomes limiting. Therefore, our study on submerged macrophyte added to growing evidence providing support for a stronger somatic cost of carbon in females, potentially causing a sex-specific plastic response to limited carbon.
Conclusion
In conclusion, our results highlighted that males and females have different strategies of resource allocation in response to carbon limitation. A decrease in light availability led to a smaller decrease in allocation to carbon harvesting organs in females than in males for the sake of maintaining a capacity for carbon acquisition for later seed and fruit production. Limited light condition caused a smaller reduction of reproductive allocation in males than in females, suggesting that the production of reproductive organs in females is more carbon-limited than in males (fruits in females vs. flowers and inflorescences in males). Eutrophication and degraded underwater light climate is a fundamental cause of worldwide declines in submerged macrophytes (Zhang et al., 2017). Therefore, investigating sex-specific strategies of resource allocation in response to varying light availability is essential for better understanding the adaptation and evolution of submerged macrophytes in freshwater habitats.
Author Contributions
LL designed the study, executed the experiments and wrote the first draft of the manuscript. LL and MD conducted the statistical analyses. JC, ZL, and YZ provided the scientific advice.
Funding
This work was co-supported by the National Key R&D Program of China (2018YFD0900904), the National Natural Science Foundation of China (31400403), and the Natural Science Foundation of Jiangxi Province (20161BAB214157).
Conflict of Interest Statement
The authors declare that the research was conducted in the absence of any commercial or financial relationships that could be construed as a potential conflict of interest.
Acknowledgments
The authors thank Siyuan Xu and Fangyan Liu for help during data collection.
References
Ågren, J., Danell, K., Elmqvist, T., Ericson, L., and Hjalten, J. (1999). “Sexual dimorphism and biotic interactions,” in Gender and Sexual Dimorphism in Flowering Plants, eds M. A. Geber, T. E. Dawson, and L. F. Delph (Heidelberg: Springer), 217–246. doi: 10.1007/978-3-662-03908-3_8
Antos, J. A., and Allen, G. A. (1990). A comparison of reproductive effort in the dioecious shrub Oemleria cerasiformis using nitrogen, energy and biomass as currencies. Am. Midl. Nat. 124, 254–262. doi: 10.2307/2426174
Barrett, S. C. H., Eckert, C. G., and Husband, B. C. (1993). Evolutionary processes in aquatic plant populations. Aquat. Bot. 46, 813–826. doi: 10.1016/0304-3770(93)90068-8
Barrett, S. C. H., and Hough, J. (2013). Sexual dimorphism in flowering plants. J. Exp. Bot. 64, 67–82. doi: 10.1093/jxb/ers308
Boedeltje, G., Qzinga, W. A., and Prinzing, A. (2008). The trade-off between vegetative and generative reproduction among angiosperms influences regional hydrochorous propagule pressure. Global Ecol. Biogeogr. 17, 50–58. doi: 10.1111/j.1466-8238.2007.00365.x
Cao, T., Ni, L. Y., Xie, P., Xu, J., and Zhang, M. (2011). Effects of moderate ammonium enrichment on three submersed macrophytes under contrasting light availability. Freshw. Biol. 56, 1620–1629. doi: 10.1111/j.1365-2427.2011.02601.x
Chambers, P. A. (1987). Light and nutrients in the control of aquatic plant community structure. II. In situ observations. J. Ecol. 75, 621–628. doi: 10.2307/2260194
Chambers, P. A., and Kalff, J. (1987). Light and nutrients in the control of aquatic plant community structure. i. in situ experiments. J. Ecol. 75, 611–619. doi: 10.2307/2260193
Charlesworth, B., and Charlesworth, D. (1978). A model for the evolution of dioecy and gynodioecy. Am. Nat. 112, 975–997. doi: 10.1086/283342
Charlesworth, D. (1999). “Theories of the evolution of dioecy,” in Gender and Sexual Dimorphism in Flowering Plants, eds M. A. Geber, T. E. Dawson, and L. F. Delph (Heidelberg: Springer), 33–60. doi: 10.1007/978-3-662-03908-3_2
Charnov, E. L., Bull, J. J., and Maynard Smith, J. (1976). Why be an hermaphrodite? Nature 263, 125–126. doi: 10.1038/263125a0
Chen, J. F., Cao, T., Zhang, X. L., Xi, Y. L., Ni, L. Y., and Jeppesen, E. (2016). Differential photosynthetic and morphological adaptations to low light affect depth distribution of two submersed macrophytes in lakes. Sci. Rep. 6:34028. doi: 10.1038/srep34028
Cox, P. A. (1988). Hydrophilous pollination. Annu. Rev. Ecol. Evol. S. 19, 261–279. doi: 10.1146/annurev.es.19.110188.001401
Dawson, T. E., and Geber, M. A. (1999). “Sexual dimorphism in physiology and morphology,” in Gender and Sexual Dimorphism in Flowering Plants, eds M. A. Geber, T. E. Dawson, and L. F. Delph (Heidelberg: Springer), 175–215. doi: 10.1007/978-3-662-03908-3_7
de Kroon, H., and Hutchings, M. J. (1995). Morphological plasticity in clonal plants: the foraging concept reconsidered. J. Ecol. 83, 142–143. doi: 10.2307/2261158
Delph, L. F. (1999). “Sexual dimorphism in life history,” in Gender and Sexual Dimorphism in Flowering Plants, eds M. A. Geber, T. E. Dawson, and L. F. Delph (Heidelberg: Springer), 149–173. doi: 10.1007/978-3-662-03908-3_6
Delph, L. F., and Bell, D. L. (2008). A test of the differential-plasticity hypothesis for variation in the degree of sexual dimorphism in Silene latifolia. Evol. Ecol. Res. 10, 61–75. doi: 10.1159/000084019
Eckert, C. G., Dorken, M. E., and Barrett, S. C. H. (2016). Ecological and evolutionary consequences of sexual and clonal reproduction in aquatic plants. Aquat. Bot. 135, 46–61. doi: 10.1016/j.aquabot.2016.03.006
Eckhart, V. M. (1999). “Sexual dimorphism in flowers and inflorescences,” in Gender and Sexual Dimorphism in Flowering Plants, eds M. A. Geber, T. E. Dawson, and L. F. Delph (Heidelberg: Springer), 123–148. doi: 10.1007/978-3-662-03908-3_5
Eller, F., Alnoee, A. B., Boderskov, T., Guo, W. Y., Kamp, A. T., Sorrell, B. K., et al. (2015). Invasive submerged freshwater macrophytes are more plastic in their response to light intensity than to the availability of free CO2 in air-equilibrated water. Freshw. Biol. 60, 929–943. doi: 10.1111/fwb.12547
Fox, A. D., Meng, F. J., Shen, X., Yang, X., Yang, W., and Cao, L. (2013). Effects of shading on Vallisneria natans (Lour.) H. Hara Growth. Knowl. Manag. Aquat. Ecol. 410:07. doi: 10.1051/kmae/2013062
French, G. T., and Moore, K. A. (2003). Interactive effects of light and salinity stress on the growth, reproduction, and photosynthetic capabilities of Vallisneria americana (wild celery). Estuar. Coast. 26, 1255–1268. doi: 10.1007/BF02803628
Going, B., Simpson, J., and Even, T. (2008). The influence of light on the growth of watercress (Nasturtium officinale R. Br.). Hydrobiologia 607, 75–85. doi: 10.1007/s10750-008-9368-2
Harper, J. L. (1977). Population Biology of Plants. London: Academic Press. doi: 10.1007/s10750-008-9368-2
Harris, M. S., and Pannell, J. R. (2008). Roots, shoots and reproduction: sexual dimorphism in size and costs of reproductive allocation in an annual herb. P. R. Soc. B Biol. Sci. 275, 2595–2602. doi: 10.1098/rspb.2008.0585
Harris, M. S., and Pannell, J. R. (2010). Canopy seed storage is associated with sexual dimorphism in the woody dioecious genus Leucadendron. J. Ecol. 98, 509–515. doi: 10.1111/j.1365-2745.2009.01623.x
Hesse, E., and Pannell, J. R. (2011). Sexual dimorphism in a dioecious population of the wind-pollinated herb Mercurialis annua: the interactive effects of resource availability and competition. Ann. Bot. 107, 1039–1045. doi: 10.1093/aob/mcr046
Hyldgaard, B., and Brix, H. (2012). Intraspecies differences in phenotypic plasticity: invasive vs. non-invasive populations of Ceratophyllum demersum. Aquat. Bot. 97, 49–56. doi: 10.1016/j.aquabot.2011.11.004
Labouche, A. M., and Pannell, J. R. (2016). A test of the size-constraint hypothesis for a limit to sexual dimorphism in plants. Oecologia 181, 873–884. doi: 10.1007/s00442-016-3616-3
Laiolo, P., Illera, J. C., and Obeso, J. R. (2013). Local climate determines intra- and interspecific variation in sexual size dimorphism in mountain grasshopper communities. J. Evol. Biol. 26, 2171–2183. doi: 10.1111/jeb.12213
Li, C., Xu, G., Zang, R., Korpelainen, H., and Berninger, F. (2007). Sex-related differences in leaf morphological and physiological response in Hippophae rhamnoides along an altitudinal gradient. Tree Physiol. 27, 399–406. doi: 10.1093/treephys/27.3.399
Li, L., Barrett, S. C. H., Song, Z. P., and Chen, J. K. (2019). Sex-specific plasticity of reproductive allocation in response to water depth in a clonal, dioecious macrophyte. Am. J. Bot. 106, 42–50. doi: 10.1002/ajb2.1218
Lovett Doust, J., O’Brien, G., and Lovett Doust, L. (1987). Effect of density on secondary sex characteristics and sex ratio in Silene alba (Caryophyllaceae). Am. J. Bot. 74, 40–46. doi: 10.2307/2444329
McDowell, S. C. L., McDowell, N. G., Marshall, J. D., and Hultine, K. (2000). Carbon and nitrogen allocation to male and female reproduction in Rocky Mountain Douglas-fir (Pseudotsuga menziesii var. galuca, Pinaceae). Am. J. Bot. 87, 539–546. doi: 10.2307/2656598
Obeso, J. R. (2002). The cost of reproduction in plants. New Phytol. 155, 321–348. doi: 10.1046/j.1469-8137.2002.00477.x
Pickering, C. M., and Hill, W. (2002). Reproductive ecology and the effect of altitude on sex ratios in the dioecious herb Aciphylla simplicifolia (Apiaceae). Aust. J. Bot. 50, 289–300. doi: 10.1071/bt01043
Renner, S. S. (2014). The relative and absolute frequencies of angio-sperm sexual systems: dioecy, monoecy, gynodioecy, and an updated online database. Am. J. Bot. 101, 1588–1596. doi: 10.3732/ajb.1400196
Riis, T., Olesen, B., Clayton, J. S., Lambertini, C., Brix, H., and Sorrell, B. K. (2012). Growth and morphology in relation to temperature and light availability during the establishment of three invasive aquatic plant species. Aquat. Bot. 102, 56–64. doi: 10.1016/j.aquabot.2012.05.002
Sanchez-Vilas, J., Bermúdez, R., and Retuerto, R. (2012). Soil water content and patterns of allocation to below- and above-ground biomass in the sexes of the subdioecious plant Honckenya peploides. Ann. Bot. 110, 839–848. doi: 10.1093/aob/mcs157
Schelske, C. L., Lowe, E. F., and Kenney, W. F. (2010). How anthropogenic darkening of Lake Apopka induced benthic light limitation and forced the shift from macrophyte to phytoplankton dominance. Limnol. Oceanogr. 55, 1201–1212. doi: 10.4319/lo.2010.55.3.1201
Schneider, S. C., Pichler, D. E., Andersen, T., and Melzer, A. (2015). Light acclimation in submerged macrophytes: the roles of plant elongation, pigmentation and branch orientation differ among Chara species. Aquat. Bot. 120, 121–128. doi: 10.1016/j.aquabot.2014.05.002
Sculthorpe, C. D. (1967). The Biology of Aquatic Vascular Plants. London: Edward Arnold. doi: 10.1016/j.aquabot.2014.05.002
Teder, T., and Tammaru, T. (2005). Sexual size dimorphism within species increases with body size in insects. Oikos 108, 321–334. doi: 10.1111/j.0030-1299.2005.13609.x
Teitel, Z., Pickup, M., Field, D., and Barrett, S. C. H. (2016). The dynamics ofresource allocation, and costs of reproduction in a sexually dimorphic, wind-pollinated, dioecious plant. Plant Biol. 18, 98–103. doi: 10.1111/plb.12336
Tonnabel, J., David, P., and Pannell, J. R. (2017). Sex-specific strategies of resource allocation in response to competition for light in a dioecious plant. Oecologia 185, 675–686. doi: 10.1007/s00442-017-3966-5
Tonnabel, J., Mignot, A., Douzery, E. J. P., Rebelo, T., Schurr, F., Midgley, J., et al. (2014). Convergent and correlated evolution of major life-history traits in the angiosperm genus Leucadendron (Proteaceae). Evolution 68, 2775–2792. doi: 10.1111/evo.12480
Valladares, F., and Niinemets, Ü. (2008). Shade tolerance, a key plant feature of complex nature and consequences. Annu. Rev. Ecol. Evol. Syst. 39, 237–257. 10.1146/annurev.ecolsys.39.110707.173506
Van Drunen, W. E., and Dorken, M. E. (2012). Trade-offs between clonal and sexual reproduction in Sagittaria latifolia (Alismataceae) scale up to affect the fitness of entire clones. New Phytol. 196, 606–616. doi: 10.1111/j.1469-8137.2012.04260.x
Wang, B., Song, Z. P., Liu, G. H., Lu, F., and Li, W. (2010). Comparison of the extent of genetic variation of Vallisneria natans and its sympatric congener V. spinulosa in lakes of the middle–lower reaches of the Yangtze River. Aquat. Bot. 92, 233–238. doi: 10.1016/j.aquabot.2009.12.006
Xie, Y. H., Luo, W. B., Ren, B., and Li, F. (2007). Morphological and physiological responses to sediment type and light availability in roots of the submerged plant Myriophyllum spicatum. Ann. Bot. 100, 1517–1523. doi: 10.1093/aob/mcm236
Yuan, L. Y., Li, W., Liu, G. H., and Deng, G. (2012). Effects of different shaded conditions and water depths on the growth and reproductive strategy of Vallisneria spinulosa. Pak. J. Bot. 44, 911–918. doi: 10.1179/0373668712Z.00000000039
Zhang, Y. L., Jeppesen, E., Liu, X. H., Qin, B. Q., Shi, K., Zhou, Y. Q., et al. (2017). Global loss of aquatic vegetation in lakes. Earth Sci. Rev. 173, 259–265. doi: 10.1016/j.earscirev.2017.08.013
Keywords: carbon limitation, dioecious plant, light intensity, plasticity, resource allocation, sexual dimorphism, submersed macrophyte
Citation: Li L, Ding M, Lan Z, Zhao Y and Chen J (2019) Light Availability and Patterns of Allocation to Reproductive and Vegetative Biomass in the Sexes of the Dioecious Macrophyte Vallisneria spinulosa. Front. Plant Sci. 10:572. doi: 10.3389/fpls.2019.00572
Received: 20 January 2019; Accepted: 16 April 2019;
Published: 03 May 2019.
Edited by:
Sidinei Magela Thomaz, Universidade Estadual de Maringá, BrazilReviewed by:
Haijun Wang, Chinese Academy of Sciences, ChinaRubén Retuerto, University of Santiago de Compostela, Spain
Copyright © 2019 Li, Ding, Lan, Zhao and Chen. This is an open-access article distributed under the terms of the Creative Commons Attribution License (CC BY). The use, distribution or reproduction in other forums is permitted, provided the original author(s) and the copyright owner(s) are credited and that the original publication in this journal is cited, in accordance with accepted academic practice. No use, distribution or reproduction is permitted which does not comply with these terms.
*Correspondence: Lei Li, lilei77@ncu.edu.cn