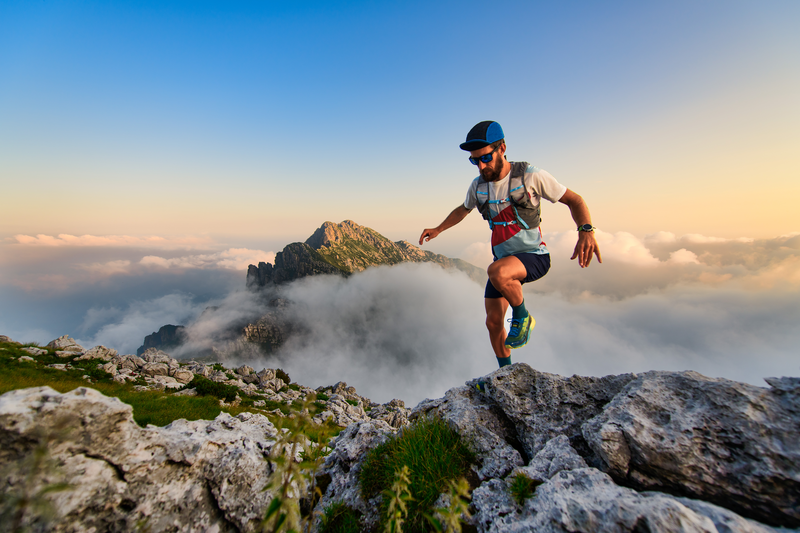
94% of researchers rate our articles as excellent or good
Learn more about the work of our research integrity team to safeguard the quality of each article we publish.
Find out more
MINI REVIEW article
Front. Plant Sci. , 15 March 2019
Sec. Plant Pathogen Interactions
Volume 10 - 2019 | https://doi.org/10.3389/fpls.2019.00269
This article is part of the Research Topic The Role of Plant Hormones in Plant-Microbe Symbioses View all 15 articles
Plant hormones play key roles in nodulation and arbuscular mycorrhizal (AM) associations. These two agriculturally and ecologically important symbioses enable plants to gain access to nutrients, in particular, nitrogen in the case of nodulation and phosphorous in the case of AM. Work over the past few decades has revealed how symbioses with nitrogen-fixing rhizobia, restricted almost exclusively to legumes, evolved in part from ancient AM symbioses formed by more than 80% of land plants. Although overlapping, these symbiotic programs also have important differences, including the de novo development of a new organ found only in nodulation. One emerging area of research is the role of two plant hormone groups, the gibberellins (GAs) and brassinosteroids (BRs), in the development and maintenance of these symbioses. In this review, we compare and contrast the roles of these hormones in the two symbioses, including potential interactions with other hormones. This not only focuses on legumes, most of which can host both symbionts, but also examines the role of these in AM development in non-legumes. GA acts by suppressing DELLA, and this regulatory module acts to negatively influence both rhizobial and mycorrhizal infection but appears to promote nodule organogenesis. While an overall positive role for BRs in nodulation and AM has been suggested by studies using mutants disrupted in BR biosynthesis or response, application studies indicate that BR may play a more complex role in nodulation. Given the nature of these symbioses, with events regulated both spatially and temporally, future studies should examine in more detail how GAs and BRs may influence precise events during these symbioses, including interactions with other hormone groups.
While the application of fertilizer to crop plants may compensate for poor nutrient availability in the soil, there may be negative impacts such as the use of non-renewable resources (e.g., phosphate), cost, enhancing the growth of weeds, or runoff into waterways which may cause eutrophication. An alternative way to enhance growth under low nutrient conditions is by increasing plant nutrient uptake via plant-microbe symbioses with arbuscular mycorrhizal fungi or rhizobial bacteria. Arbuscular mycorrhizal symbioses are ancient and widespread, occurring in over 80% of land plant taxa (Smith and Smith, 2011). In these symbioses, the fungi act as an extension of the plant’s root system, increasing the surface area for nutrient uptake (Yang et al., 2015). In return, the plants provide the fungi with energy in the form of fatty acids and sugars (e.g., Smith and Smith, 2011; Yang et al., 2015; Jiang et al., 2017). Arbuscular mycorrhizal symbioses can increase plant growth and yield in soils poor in essential nutrients, particularly phosphorus (Baum et al., 2015; Yang et al., 2015). Another important plant microbe symbiosis almost completely restricted to legumes is nodulation (Mus et al., 2016), where nitrogen-fixing rhizobial bacteria are housed in nodules in the root system. The nitrogen-fixing bacteria convert atmospheric nitrogen into ammonia, which the plant can use as a nitrogen source (Mus et al., 2016). Nodulation is thought to have evolved in part from the ancient arbuscular mycorrhizal program (Delaux et al., 2013; Martin et al., 2017).
Both nodulation and arbuscular mycorrhizal symbioses are regulated by a variety of plant signals, including many of the plant hormones (Ferguson and Mathesius, 2014; Gutjahr, 2014; Bedini et al., 2018; Liao et al., 2018). This review focuses on how two families of plant growth hormones, the gibberellins (GAs) and brassinosteroids (BR), influence the development of rhizobial and AM symbioses. It is timely to synthesize an overview across species and to examine any parallels between the two symbioses. In particular, we examine the stage of the symbiosis at which the hormones operate, as these are complex processes with distinct infection and development phases that can occur across different spatial and temporal scales. Although interactions between GA and BR has been suggested to occur at the level of signaling (for review see Ross and Quittendon, 2016), this has not yet been explored during symbioses. Although there is evidence that some rhizobial bacteria may produce GA (Tatsukami et al., 2016; Nett et al., 2017), it is not yet clear if mycorrhizal fungi produce GA, as this has yet to be tested using modern quantitative techniques (e.g., Barea and Azcón-Aguilar, 1982). As the GAs and BRs also have powerful effects on many other aspects of plant development, for example, stem elongation (Ross et al., 2011), in this synthesis, we take care to examine the evidence to determine whether the effect of the hormones on a symbiosis is direct or may result from indirect effects of these hormones on other aspects of the plant’s phenotype.
GAs are a group of diterpenoid growth hormones strongly associated with promoting growth, including stem elongation and germination (e.g., Ross and Reid, 2010). The bioactive GAs signal by binding to intracellular receptors from the GID protein family, which then complex with DELLA transcription factors and an E3 ubiquitin ligase. The E3 ubiquitin ligase polyubiquitinates the DELLA proteins, tagging them for degradation (Daviere and Achard, 2013). DELLA proteins can act as represses of transcription; thus, the loss of the DELLA transcription factors in the presence of GA derepresses gene expression inducing GA response (Daviere and Achard, 2013).
There is good evidence that arbuscular mycorrhizal colonization is inhibited by GA signaling (Figure 1A). Several studies have shown that the application of GAs inhibits the formation of arbuscular mycorrhizae, including studies in pea (El Ghachtouli et al., 1996), Lotus japonicus (Takeda et al., 2015), and tomato (Martín-Rodríguez et al., 2015). A negative role for GAs in arbuscular mycorrhizal development was confirmed by mutant and transgenic studies in pea, Medicago truncatula, rice, and wheat (Floss et al., 2013; Foo et al., 2013; Yu et al., 2014). The severely GA-deficient pea mutant na, which has a non-functional ent-kaurenoic acid oxidase enzyme (Davidson et al., 2003), has more arbuscular mycorrhizae than wild-type plants (Foo et al., 2013). This influence of low gibberellins on mycorrhizal colonization appears to be independent of ethylene (Foo et al., 2016). However, it acts through the DELLA proteins since a DELLA-deficient double mutant of pea, la cry-s, which results in permanently high GA signaling, and the na la cry-s triple mutant display reduced levels of arbuscular mycorrhizal colonization compared with wild-type plants (Foo et al., 2013). The formation of arbuscular mycorrhizal symbiosis occurs in several stages, including spore germination and hyphal branching, hyphopodium formation, and penetration into the root, followed by the development of hyphae and branched arbuscules (Figure 1; Genre et al., 2005). GA signaling through DELLA appears to be particularly involved in arbuscule initiation, rather than arbuscule branching or hyphal colonization, as loss-of-function della1 della2 double mutants in pea and Medicago truncatula and the della mutant slr1 in rice display a more dramatic reduction in arbuscules than wild-type plants compared to other fungal structures (Floss et al., 2013; Foo et al., 2013; Yu et al., 2014). Consistent with this, a rice SLR-YFP overexpression line and a wheat Rht1/Rht2 gain-of-function DELLA line displayed increased arbuscule formation (Floss et al., 2013; Yu et al., 2014). Although they are altered in number, the arbuscules that do form in plants with altered GA or DELLA status appear relatively normal (Floss et al., 2013; Foo et al., 2013).
Figure 1. Schematic of the proposed roles of gibberellin (A) and brassinosteroids (B) and their interaction with ethylene in arbuscular mycorrhizal colonization (LHS) and nodulation (RHS). Key players in hormone perception and potential downstream elements are indicated. For arbuscular mycorrhizal colonization, a spore, hyphae, and arbuscules are represented. For nodulation, numerals indicate the stages of development: (i) Infection thread formation, (ii) initial cortical cell divisions, (iii) nodule organogenesis, and (iv) development of the nodule into a nitrogen-fixing organ. *Proteins that have been proposed to influence this transcription factor complex include CCaMK, IPD3, NSP2, and NF-YA1 (See the text for references).
The establishment of a symbiosis requires the recognition, uptake, and accommodation of the microbe. For both nodulation and arbuscular mycorrhizal associations, this is initiated by the plant’s perception of unique microbial-derived lipochitooligosaccharides or chitin oligomers, known as Nod and Myc factors, respectively (Martin et al., 2017). Genetic studies in legumes have begun to define the parts of this pathway that the two symbioses share, known as the common symbiotic signaling cascade, and the outputs unique to each symbiosis. One key output of the perception of the Nod and Myc factors is the induction of calcium spiking that is perceived by the CCaMK protein. In the formation of mycorrhizal symbioses, CCaMK phosphorylates the transcription factor CYCLOPS/IPD3, which activates a variety of symbiosis genes that facilitate fungal infection and arbuscule formation (Zipfel and Oldroyd, 2017). An important downstream target of this pathway is RAM1. RAM1 is a GRAS transcription factor required for arbuscule initiation and branching (Pimprikar et al., 2016). Several studies have examined how DELLA may influence elements of this mycorrhizal signaling cascade. Transactivation studies, yeast two hybrid studies, bimolecular fluorescence complementation and co-immunoprecipitation studies have revealed that DELLA proteins may participate in a complex with CYCLOPS (Jin et al., 2016) and CCaMK to control RAM1 expression (Pimprikar et al., 2016). Genetic evidence for such interactions during AM development are complex and are complicated by the fact that aspects may rely on AM activation of the cortical program by CCaMK (Floss et al., 2013). Overall, studies with mutants, transgenic lines, and application of GA or GA biosynthesis inhibitors are consistent with DELLA acting with CCaMK and CYCLOPS to promote arbuscule formation via RAM1 (Floss et al., 2013; Pimprikar et al., 2016). However, it is important to note that gain-of-function DELLA overexpression or GA biosynthesis inhibitors can restore arbuscule formation in cyclops mutants; this indicates the potential for other DELLA interacting partners (Pimprikar et al., 2016).
Another approach to examine the dynamics of GA during arbuscular mycorrhizal colonization was undertaken in a study in Lotus japonicus, in which transgenic plants expressing the GUS reporter gene under the control of promoters of the GA biosynthesis genes GA20ox1 and GA20ox2 were generated (Takeda et al., 2015). Co-staining for GUS and arbuscular mycorrhizae revealed cells expressing these GA biosynthesis genes corresponded to cells hosting the AM fungus (Takeda et al., 2015). Bioactive GA1 levels in whole infected roots were also somewhat elevated compared to uninoculated roots. Plants forming an arbuscular mycorrhizal symbiosis accumulate GAs in their roots, potentially due to increased activity in the 13-hydroxylation GA biosynthesis pathway (Martín-Rodríguez et al., 2015). In addition, the study by Takeda et al. (2015) proposed that exogenous GA influences fungal entry into the plant and hyphal branching of the mycorrhizal fungi, although as these studies were necessarily conducted in planta, it is difficult to distinguish direct effects on the fungi from indirect effects due to altered plant growth.
GA can have both positive and negative effects of the number of nodules formed. Application of bioactive GAs and/or GA biosynthesis inhibitors can both promote and inhibit nodulation, depending on the species, dose, and application method (e.g., Thurber et al., 1958; Mes, 1959; Ferguson et al., 2005; Lievens et al., 2005; Fonouni-Farde et al., 2016). This is consistent with genetic studies, where low nodule numbers are observed in pea, Medicago, and Lotus mutants with high GA signaling, due to della mutations, and also in pea mutants with low GA signaling, due to disruption of GA biosynthesis (Ferguson et al., 2005; Fonouni-Farde et al., 2016; Jin et al., 2016; McAdam et al., 2018).
While this apparent paradox may be explained by stating that there is an optimal level of GA for nodulation overall, this may disguise the fact that GA exerts different effects on specific stages of nodulation (Figure 1B; Ferguson et al., 2005; Ferguson et al., 2011; McAdam et al., 2018). As outlined above, nodulation is induced by Nod factor signaling via the common symbiotic pathway. This signaling not only facilitates infection at the epidermis but also induces concomitant induction of nodule organogenesis in the root cortex. These initially spatially separated events can occur independently, as demonstrated by the development of spontaneous nodules in the absence of rhizobia in gain-of-function CCaMK or cytokinin receptor mutants (e.g., Marsh et al., 2007; Tirichine et al., 2007). GA appears to have a strong negative effect on the infection stage of nodulation since loss-of-function della mutants or transgenic lines in Medicago and pea display a reduced number of infection threads compared with wild-type plants (Fonouni-Farde et al., 2016; Jin et al., 2016; McAdam et al., 2018). Consistent with this, infection thread formation is also suppressed by exogenous GA in a range of species (Maekawa et al., 2009; Fonouni-Farde et al., 2016; Jin et al., 2016; McAdam et al., 2018). Similarly, GA-deficient mutants of pea display elevated infection thread formation (McAdam et al., 2018). Yeast three hybrid and co-immunoprecipitation studies in Medicago suggest that this negative influence of GA signaling on infection may be mediated through physical interaction of the DELLA proteins with key components of the Nod factor signaling pathway including NF-YA1, NSP1, NSP2, and IPD3/CYCLOPS (Fonouni-Farde et al., 2016; Jin et al., 2016). DELLAs have also been reported to increase the phosphorylation state of IPD3/CYCLOPS in vitro (Fonouni-Farde et al., 2016). Consistent with this, Nod factor-activated gene expression was suppressed by GA treatment in wild-type Lotus and Medicago and, in the absence of GA treatment, in Medicago della mutant lines (Maekawa et al., 2009; Fonouni-Farde et al., 2016; Jin et al., 2016).
Several different approaches have been used to examine the role of GA during nodule initiation, organogenesis, and ultimate function. In addition to displaying reduced infection, lines with loss of DELLA function also display a reduced number of nodules (Ferguson et al., 2011; Fonouni-Farde et al., 2016; Jin et al., 2016; McAdam et al., 2018). However, the nodules that do form on della mutants appear similar to wild type and in pea appear to fix nitrogen at a similar rate to wild-type nodules (McAdam et al., 2018). This suppression of nodule initiation in della mutants, but not nodule development or function, may be due to a reduction in infection events or a more direct role for DELLAs in nodule initiation. The latter hypothesis is supported by the fact that della mutants of Medicago do not form nodule-like structures in transgenic roots overexpressing mutant gain of function versions of CCaMK or the cytokinin receptor cre1 (Jin et al., 2016; Fonouni-Farde et al., 2017). Nodule-like structures were also observed in wild-type lines expressing della1-Δ18 dominant-active protein (Fonouni-Farde et al., 2017). However, several lines of evidence suggest a positive role for GA in nodule initiation and development. Firstly, an increased number of infection threads but not nodule numbers were observed in wild-type lines expressing a dominant version of MtDELLA1 resistant to degradation by GA (Fonouni-Farde et al., 2016), suggesting a negative role for GA during infection but not necessarily nodule organogenesis. Secondly, GA-deficient na mutants of pea display strongly elevated numbers of infection threads, but a reduced number of nodules, and often no nodules at all (McAdam et al., 2018). Indeed, the few nodules that do form in severely GA-deficient lines are small, contain undifferentiated bacteria, and appear to fix less nitrogen than wild-type nodules (McAdam et al., 2018). This suggests an important role for GA in promoting the initiation and development of nodules into nitrogen fixing organs. This is consistent with the expression of GA biosynthesis genes within the nodule during formation and maturation in several species (Kouchi et al., 2004; Lievens et al., 2005; Hayashi et al., 2012).
Several studies have examined if this influence of GA on nodulation may be via interaction with other hormones with prominent roles in nodulation. In particular, studies have examined a link with ethylene, a gaseous hormone that has an overall negative influence on infection and nodule initiation and also influences the spatial arrangement of nodules (for review see Guinel, 2015). In pea, severely GA-deficient lines evolve more ethylene (Ferguson et al., 2011). To explore the interaction between GA and ethylene, double mutant lines were generated that combine the severely GA-deficient na mutant with ein2, a mutant lacking an essential element of the ethylene perception pathway (Weller et al., 2015; Foo et al., 2016). Infection, nodule initiation, and nodule function were assessed in these double mutants and compared to the respective single mutants and wild-type plants. Ethylene and GA appear to influence infection relatively independently, but GA suppression of ethylene biosynthesis may be an important mechanism to promote nodule initiation, as na ein2 mutants develop many nodules. However, the nodules that do develop on na ein2 plants have the characteristic arrested development of na (GA-deficient) single mutant plants and appear to fix little nitrogen, suggesting that GA is important for nodule development (McAdam et al., 2018). It is important to note that these na ein2 plants still display severely reduced shoot size, suggesting that it is not shoot size per se that restricts nodule initiation in GA-deficient lines (Foo et al., 2016). More recently, a potential crossover between the GA and cytokinin pathways has been suggested in Medicago roots (Fonouni-Farde et al., 2017), although future studies are required to determine if this occurs during nodulation.
Future studies could explore the spatial and temporal regulation of GA signaling during infection and nodule development to resolve the role of GA during various stages of nodulation. This will complement ongoing studies that also indicate a role for bacterial-derived GA in some, but not all, legume-rhizobial partnerships (Tatsukami et al., 2016; Nett et al., 2017).
Brassinosteroids are a family of growth promoting steroid hormones. They have been implicated in a range of developmental processes, such as shoot elongation and vascular development by promoting cell elongation and division (Singh and Sigal, 2015). Only a handful of studies have investigated the role of brassinosteroids in arbuscular mycorrhizae. Overall, they appear to have a positive influence. Mutations in brassinosteroid biosynthesis genes resulting in severe BR-deficiency result in reduced arbuscular mycorrhizal colonization in tomato, rice, and pea mutants compared to wild-type plants (Bitterlich et al., 2014a,b; Foo et al., 2016) and foliar application of synthetic brassinosteroids to wheat resulted in increased in AM colonization (Tofighi et al., 2017). How BRs influences AM development is not known. As observed in the severely GA-deficient lines outlined above, severely BR-deficient lines also evolve more ethylene (Ross and Reid, 1986). Double mutant studies with ethylene insensitive plants deficient in BRs (lk ein2) suggest that the influence of BRs on AM is independent of ethylene (Foo et al., 2016). Other studies suggest that BRs may influence sucrose transport, potentially increasing the amount of sugar available to the fungus (Bitterlich et al., 2014a,b). Future studies are required to delineate the precise role of BRs during fungal infection, accommodation, and nutrient exchange, including any interaction with other plant hormones.
Most of the early studies into the role of brassinosteroids in nodulation were based on applying synthetic brassinosteroids and brassinosteroid biosynthesis inhibitors and produced mixed results, including both positive and negative effects of BR on nodulation across a range of legumes (Vardhini and Rao, 1999; Hunter, 2001; Terakado et al., 2005; Yusuf et al., 2012). This is similar to studies with GAs outlined above, where both positive and negative effects of exogenous GAs were observed on nodule number. Genetic studies using BR biosynthesis and receptor mutants in pea and a BR receptor mutant in Medicago truncatula suggest that BRs act as promoters of nodule number, as all the mutants form less nodules than wild type (Ferguson et al., 2005; Foo et al., 2014, 2016; Cheng et al., 2017). BRs appear to influence nodulation in part through ethylene. The elevated ethylene of severely BR-deficient pea lk mutant appears to explain at least in part the low nodulation phenotype of lk mutants, as lk ein2 double mutants display an elevated nodulation phenotype, similar to ein2 single mutants (Foo et al., 2016). Cheng et al. (2017) noted that the nodules that formed on the Medicago BR receptor mutant, Mtbri1, were white, suggesting that they were non-functional. However, this phenotype has not been observed in pea BR mutants (Ferguson et al., 2005). A more direct measurement of nitrogen fixation is required to determine if BR influences nodule function in addition to nodule number. It will also be interesting to examine the various stages of nodulation in BR mutants to determine if the inconsistent application results outlined above may be due to BR influencing different stages of nodule development.
It is also important to note that grafting studies in pea suggest that BR may act through a shoot-derived signal(s) to influence nodulation. Plants with reduced BR biosynthesis in the shoot system produce less nodules than those with a wild-type shoot system, irrespective of BR production in the root system (Ferguson et al., 2005). As previous grafting studies have shown that BRs are not mobile in the plant (Symons and Reid, 2004; Symons et al., 2008), BRs must be acting through a mobile signal(s). Analysis of auxin and GA levels in these grafts suggest that BR does not act via auxin or GA (Ferguson et al., 2005). As double mutant studies ruled out a role for BR acting upstream of the systemic autoregulation of nodulation (AON) pathway (Foo et al., 2014), future studies are required to clarify this systemic effect.
Two of the major plant growth hormone families, the GAs and BRs, influence both nodulation and arbuscular mycorrhizal symbioses. The GAs, acting through DELLAs, appear to inhibit arbuscular mycorrhizal symbioses and rhizobial infection-thread formation at least in part via elements of the common symbiotic pathway. GAs also appear to have a second role in nodulation, promoting nodule initiation and organogenesis. Genetic studies have to date indicated overall that BRs promote both nodulation and arbuscular mycorrhizal symbioses. However, application studies have also indicated a potentially complex role for BRs in nodulation, with both positive and negative effects. Future studies could examine how GAs and BRs influence the spatial and temporal stages of hosting symbiotic microbes, including any intersection with the common symbiotic pathway and other hormones, including those with well-defined roles in nodulation such as auxin and cytokinin.
EF conceived the project. PM, JR, and EF wrote the manuscript.
We thank the Australian Research Council for financial support to EF and JR (FF140100770 and DP140101709).
The authors declare that the research was conducted in the absence of any commercial or financial relationships that could be construed as a potential conflict of interest.
Barea, J. M., and Azcón-Aguilar, C. (1982). Production of plant growth-regulating substances by the vesicular-arbuscular mycorrhizal fungus Glomus mosseae. Appl. Environ. Microbiol. 43, 810–813.
Baum, C., El-Tohamy, W., and Gruda, N. (2015). Increasing the productivity and product quality of vegetable crops using arbuscular mycorrhizal fungi: a review. Sci. Hortic. 187, 131–141. doi: 10.1016/j.scienta.2015.03.002
Bedini, A., Mercy, L., Schneider, C., Franken, P., and Lucic-Mercy, E. (2018). Unraveling the initial plant hormone signaling, metabolic mechanisms and plant defense triggering the endomycorrhizal symbiosis behavior. Front. Plant Sci. 9:1800. doi: 10.3389/fpls.2018.01800
Bitterlich, M., Krügel, U., Boldt-Burisch, K., Franken, P., and Kühn, C. (2014a). Interaction of brassinosteroid functions and sucrose transporter SlSUT2 regulate the formation of arbuscular mycorrhiza. Plant Signal. Behav. 9:e970426. doi: 10.4161/15592316.2014.970426
Bitterlich, M., Krügel, U., Boldt-Burisch, K., Franken, P., and Kühn, C. (2014b). The sucrose transporter SlSUT2 from tomato interacts with brassinosteroid functioning and affects arbuscular mycorrhiza formation. Plant J. 78, 887–899. doi: 10.1111/tpj.12515
Cheng, X., Gou, X., Yin, H., Mysore, K. S., Li, J., and Wen, J. (2017). Functional characterisation of brassinosteroid receptor MtBRI1 in Medicago truncatula. Sci. Rep. 7:9327. doi: 10.1038/s41598-017-09297-9
Davidson, S. E., Elliott, R. C., Helliwell, C. A., Poole, A. T., and Reid, J. B. (2003). The pea gene NA encodes ent-kaurenoic acid oxidase 1. Plant Physiol. 131, 335–344. doi: 10.1104/pp.012963
Daviere, J. M., and Achard, P. (2013). Gibberellin signaling in plants. Development 140, 1147–1151. doi: 10.1242/dev.087650
Delaux, P. M., Séjalon-Delmas, N., Bécard, G., and Ané, J. M. (2013). Evolution of the plant-microbe symbiotic “toolkit”. Trends Plant Sci. 18, 298–304. doi: 10.1016/j.tplants.2013.01.008
El Ghachtouli, N., Martin-Tanguy, J., Paynot, M., and Gianinazzi, S. (1996). First-report of the inhibition of arbuscular mycorrhizal infection of Pisum sativum by specific and irreversible inhibition of polyamine biosynthesis or by gibberellic acid treatment. FEBS Lett. 385, 189–192. doi: 10.1016/0014-5793(96)00379-1
Ferguson, B. J., Foo, E., Ross, J. J., and Reid, J. B. (2011). Relationship between gibberellin, ethylene and nodulation in Pisum sativum. New Phytol. 189, 829–842. doi: 10.1111/j.1469-8137.2010.03542.x
Ferguson, B. J., and Mathesius, U. (2014). Phytohormone regulation of legume-rhizobia interactions. J. Chem. Ecol. 40, 770–790. doi: 10.1007/s10886-014-0472-7
Ferguson, B. J., Ross, J. J., and Reid, J. B. (2005). Nodulation phenotypes of gibberellin and brassinosteroid mutants of pea. Plant Physiol. 138, 2396–2405. doi: 10.1104/pp.105.062414
Floss, D. S., Levy, J. G., Levesque-Tremblay, V., Pumplin, N., and Harrison, M. J. (2013). DELLA proteins regulate arbuscule formation in arbuscular mycorrhizal symbiosis. Proc. Natl. Acad. Sci. USA 110, E5025–E5034. doi: 10.1073/pnas.1308973110
Fonouni-Farde, C., Kisiala, A., Brault, M., Emery, R. J. N., Diet, A., and Frugier, F. (2017). DELLA1-mediated gibberellin signaling regulates cytokinin-dependent symbiotic nodulation. Plant Physiol. 175, 1795–1806. doi: 10.1104/pp.17.00919
Fonouni-Farde, C., Tan, S., Baudin, M., Brault, M., Wen, J., Mysore, K. S., et al. (2016). DELLA-mediated gibberellin signalling regulates Nod factor signalling and rhizobial infection. Nat. Commun. 7:12636. doi: 10.1038/ncomms12636
Foo, E., Ferguson, B. J., and Reid, J. B. (2014). The potential roles of strigolactones and brassinosteroids in the autoregulation of nodulation pathway. Ann. Bot. 113, 1037–1045. doi: 10.1093/aob/mcu030
Foo, E., McAdam, E. L., Weller, J. L., and Reid, J. B. (2016). Interactions between ethylene, gibberellins, and brassinosteroids in the development of rhizobial and mycorrhizal symbioses of pea. J. Exp. Bot. 67, 2413–2424. doi: 10.1093/jxb/erw047
Foo, E., Ross, J. J., Jones, W. T., and Reid, J. B. (2013). Plant hormones in arbuscular mycorrhizal symbioses: an emerging role for gibberellins. Ann. Bot. 111, 769–779. doi: 10.1093/aob/mct041
Genre, A., Chabaud, M., Timmers, T., Bonfante, P., and Barker, D. G. (2005). Arbuscular mycorrhizal fungi elicit a novel intracellular apparatus in Medicago truncatula root epidermal cells before infection. Plant Cell 17, 3489–3499. doi: 10.1105/tpc.105.035410
Guinel, F. C. (2015). Ethylene, a hormone at the center-stage of nodulation. Front. Plant Sci. 6:1121. doi: 10.3389/fpls.2015.01121
Gutjahr, C. (2014). Phytohormone signaling in arbuscular mycorhiza development. Curr. Opin. Plant Biol. 20, 26–34. doi: 10.1016/j.pbi.2014.04.003
Hayashi, S., Reid, D. E., Lorenc, M. T., Stiller, J., Edwards, D., Gresshoff, P. M., et al. (2012). Transient Nod factor-dependent gene expression in the nodulation-competent zone of soybean (Glycine max [L.] Merr.) roots. Plant Biotechnol. J. 10, 995–1010. doi: 10.1111/j.1467-7652.2012.00729.x
Hunter, W. J. (2001). Influence of root-applied epibrassinolide and carbenoxolone on thenodulation and growth of soybean (Glycine max L.) seedlings. J. Agron. Crop Sci. 186, 217–221. doi: 10.1046/j.1439-037x.2001.00466.x
Jiang, Y., Wang, W., Xie, Q., Liu, N., Liu, L., Wang, D., et al. (2017). Plants transfer lipids to sustain colonization by mutualistic mycorrhizal and parasitic fungi. Science 356, 1172–1173. doi: 10.1126/science.aam9970
Jin, Y., Liu, H., Luo, D., Yu, N., Dong, W., Wang, C., et al. (2016). DELLA proteins are common components of symbiotic rhizobial and mycorrhizal signalling pathways. Nat. Commun. 7:12433. doi: 10.1038/ncomms12433
Kouchi, H., Shimomura, K., Hata, S., Hirota, A., Wu, G. J., Kumagai, H., et al. (2004). Large-scale analysis of gene expression profiles during early stages of root nodule formation in a model legume, Lotus japonicus. DNA Res. 11, 263–274. doi: 10.1093/dnares/11.4.263
Liao, D., Wang, S., Cui, M., Liu, J., Chen, A., and Xu, G. (2018). Phytohormones regulate the development of arbuscular mycorrhizal symbiosis. Int. J. Mol. Sci. 19:3146. doi: 10.3390/ijms19103146
Lievens, S., Goormachtig, S., Den Herder, J., Capoen, W., Mathis, R., Hedden, P., et al. (2005). Gibberellins are involved in nodulation of Sesbania rostrata. Plant Physiol. 139, 1366–1379. doi: 10.1104/pp.105.066944
Maekawa, T., Maekawa-Yoshikawa, M., Takeda, N., Imaizumi-Anraku, H., Murooka, Y., and Hayashi, M. (2009). Gibberellin controls the nodulation signaling pathway in Lotus japonicus. Plant J. 58, 183–194. doi: 10.1111/j.1365-313X.2008.03774.x
Marsh, J. F., Rakocevic, A., Mitra, R. M., Brocard, L., Sun, J., Eschstruth, A., et al. (2007). Medicago truncatula NIN is essential for rhizobial-independent nodule organogenesis induced by autoactive calcium/calmodulin-dependent protein kinase 1. Plant Physiol. 144, 324–335. doi: 10.1104/pp.106.093021
Martin, F. M., Uroz, S., and Barker, D. G. (2017). Ancestral alliances: plant mutualistic symbioses with fungi and bacteria. Science 356:eaad4501. doi: 10.1126/science.aad4501
Martín-Rodríguez, J. Á., Ocampo, J. A., Molinero-Rosales, N., Tarkowská, D., Ruíz-Rivero, O., and García-Garrido, J. M. (2015). Role of gibberellins during arbuscular mycorrhizal formation in tomato: new insights revealed by endogenous quantification and genetic analysis of their metabolism in mycorrhizal roots. Physiol. Plant. 154, 66–81. doi: 10.1111/ppl.12274
McAdam, E. L., Reid, J. B., and Foo, E. (2018). Gibberellins promote nodule organogenesis but inhibit the infection stages of nodulation. J. Exp. Bot. 69, 2117–2130. doi: 10.1093/jxb/ery046
Mes, M. G. (1959). Influence of gibberellic acid and photoperiod on the growth, flowering, nodulation and nitrogen assimilation of Vicia villosa. Nature 184, 2035–2036. doi: 10.1038/1842035a0
Mus, F., Crook, B. M., Garcia, K., Costas, A. G., Geddes, B. A., Kouri, E. G., et al. (2016). Symbiotic nitrogen fixation and the challenges to its extension to nonlegumes. Appl. Environ. Microbiol. 82, 3698–3710. doi: 10.1128/AEM.01055-16
Nett, R. S., Contreras, T., and Peters, R. J. (2017). Characterization of CYP115 as a gibberellin 3-oxidase indicates that certain rhizobia can produce bioactive gibberellin A4. ACS Chem. Biol. 12, 912–917. doi: 10.1021/acschembio.6b01038
Pimprikar, P., Carbonnel, S., Paries, M., Katzer, K., Klingl, V., Bohmer, M. J., et al. (2016). A CCaMK-CYCLOPS-DELLA complex activates transcription of RAM1 to regulate arbuscule branching. Curr. Biol. 26, 987–998. doi: 10.1016/j.cub.2016.01.069
Ross, J. J., and Quittendon, L. J. (2016). Interactions between brassinosteroids and gibberellins: Synthesis or Signaling?. The Plant Cell 28, 829–832. doi: 10.1105/tpc.15.00917
Ross, J. J., and Reid, J. B. (1986). Internode length in Pisum. The involvement of ethylene with the gibberellin-insensitive erectoides phenotype. Physiol. Plant. 67, 673–679. doi: 10.1111/j.1399-3054.1986.tb05076.x
Ross, J. J., and Reid, J. B. (2010). Evolution of growth-promoting plant hormones. Funct. Plant Biol. 37, 795–805. doi: 10.1071/FP10063
Ross, J. J., Weston, D. E., Davidson, S. E., and Reid, J. B. (2011). Plant hormone interactions: how complex are they? Physiol. Plant. 141, 299–309. doi: 10.1111/j.1399-3054.2011.01444.x
Singh, A. P., and Sigal, S. (2015). Growth control: brassinosteroid activity gets context. J. Exp. Bot. 66, 1123–1132. doi: 10.1093/jxb/erv026
Smith, S. E., and Smith, F. A. (2011). Roles of arbuscular mycorrhizas in plant nutrition and growth: new paradigms from cellular to ecosystem scales. Annu. Rev. Plant Biol. 62, 227–250. doi: 10.1146/annurev-arplant-042110-103846
Symons, G. M., and Reid, J. B. (2004). Brassinosteroids do not undergo long-distance transport in pea. Implications for the regulation of endogenous brassinosteroid levels. Plant Physiol. 135, 2196–2206. doi: 10.1104/pp.104.043034
Symons, G. M., Ross, J. J., Jager, C. E., and Reid, J. B. (2008). Brassinosteroid transport. J. Exp. Bot. 59, 17–24. doi: 10.1093/jxb/erm098
Takeda, N., Handa, Y., Tsuzuki, S., Kojima, M., Sakakibara, H., and Kawaguchi, M. (2015). Gibberellins interfere with symbiosis signaling and gene expression and alter colonization by arbuscular mycorrhizal fungi in Lotus japonicus. Plant Physiol. 167, 545–557. doi: 10.1104/pp.114.247700
Tatsukami, Y., Ueda, M., and Ueda, M. (2016). Rhizobial gibberellin negatively regulates host nodule number. Sci. Rep. 6:27998. doi: 10.1038/srep27998
Terakado, J., Fujihara, S., Terakado, J., Goto, S., Kuratani, R., Suzuki, Y., et al. (2005). Systemic effect of a brassinosteroid on root nodule formation in soybean as revealed by the application of brassinolide and brassinazole. Soil Sci. Plant Nutr. 51, 389–395. doi: 10.1111/j.1747-0765.2005.tb00044.x
Thurber, G. A., Douglas, J. R., and Galston, A. W. (1958). Inhibitory effect of gibberellins on nodulization in dwarf beans, Phaseolus vulgaris. Nature 181, 1082–1083. doi: 10.1038/1811082a0
Tirichine, L., Sandal, N., Madsen, L. H., Radutoiu, S., Albrektsen, A. S., Sato, S., et al. (2007). A gain-of-function mutation in a cytokinin receptor triggers root nodule organogenesis. Science 315, 104–107. doi: 10.1126/science.1132397
Tofighi, C., Khavari-Nejad, R. A., Najafi, F., Razavi, K., and Rejali, F. (2017). Brassinosteroid (BR) and arbuscular mycorrhizal (AM) fungi alleviate salinity in wheat. J. Plant Nutr. 40, 1091–1098. doi: 10.1080/01904167.2016.1263332
Vardhini, B. V., and Rao, S. S. R. (1999). Effect of brassinosteroids on nodulation and nitrogenase activity in groundnut (Arachis hypogaea L.). Plant Growth Regul. 28, 165–167.
Weller, J. L., Foo, E. M., Hecht, V., Ridge, S., Vander Schoor, J. K., and Reid, J. B. (2015). Ethylene signaling influences light-regulated development in pea. Plant Physiol. 169, 115–124. doi: 10.1104/pp.15.00164
Yang, H., Zhang, Q., Dai, Y., Liu, Q., Tang, J., Bian, X., et al. (2015). Effects of arbuscular mycorrhizal fungi on plant growth depend on root system: a meta-analysis. Plant Soil 389, 361–374. doi: 10.1007/s11104-014-2370-8
Yu, N., Luo, D., Zhang, X., Liu, J. J., Wang, W., Jin, Y., et al. (2014). A della protein complex controls the arbuscular mycorrhizal symbiosis in plants. Cell Res. 24, 130–133. doi: 10.1038/cr.2013.167
Yusuf, M., Fariduddin, Q., and Ahmad, A. (2012). 24-Epibrassinolide modulates growth, nodulation, antioxidant system, and osmolyte in tolerant and sensitive varieties of Vigna radiata under different levels of nickel: a shotgun approach. Plant Physiol. Biochem. 57, 143–153. doi: 10.1016/j.plaphy.2012.05.004
Keywords: nodulation, arbuscular mycorrhizae, plant hormone, gibberellin, brassinosteroid, DELLA, symbioses
Citation: McGuiness PN, Reid JB and Foo E (2019) The Role of Gibberellins and Brassinosteroids in Nodulation and Arbuscular Mycorrhizal Associations. Front. Plant Sci. 10:269. doi: 10.3389/fpls.2019.00269
Received: 18 December 2018; Accepted: 19 February 2019;
Published: 15 March 2019.
Edited by:
Sergio Rasmann, University of Neuchâtel, SwitzerlandReviewed by:
Marta Bjornson, University of Zurich, SwitzerlandCopyright © 2019 McGuiness, Reid and Foo. This is an open-access article distributed under the terms of the Creative Commons Attribution License (CC BY). The use, distribution or reproduction in other forums is permitted, provided the original author(s) and the copyright owner(s) are credited and that the original publication in this journal is cited, in accordance with accepted academic practice. No use, distribution or reproduction is permitted which does not comply with these terms.
*Correspondence: Eloise Foo, ZWxvaXNlLmZvb0B1dGFzLmVkdS5hdQ==
Disclaimer: All claims expressed in this article are solely those of the authors and do not necessarily represent those of their affiliated organizations, or those of the publisher, the editors and the reviewers. Any product that may be evaluated in this article or claim that may be made by its manufacturer is not guaranteed or endorsed by the publisher.
Research integrity at Frontiers
Learn more about the work of our research integrity team to safeguard the quality of each article we publish.