- 1Japan Science and Technology Agency, PRESTO, Kawaguchi, Japan
- 2Graduate School of Agricultural and Life Sciences, University of Tokyo, Tokyo, Japan
- 3Institute of Crop Science, NARO, Tsukuba, Japan
- 4Graduate School of Bioagricultural Sciences, Nagoya University, Nagoya, Japan
- 5UWA School of Agriculture and Environment, The University of Western Australia, Crawley, WA, Australia
Lysigenous aerenchyma, which develops by death and subsequent lysis of the cortical cells in roots, is essential for internal long-distance oxygen transport from shoot base to root tips of plants in waterlogged soil. Although many studies focus on the amounts of aerenchyma in roots, significance of the size of the root cortex in which aerenchyma forms has received less research attention. In the present study, we evaluated the cross-sectional area of each root tissue in adventitious roots of upland crops, wheat (Triticum aestivum) and maize (Zea mays ssp. mays), and the wetland crop, rice (Oryza sativa) under aerated or stagnant deoxygenated conditions; the latter can mimic the changes in gas composition in waterlogged soils. Our analyses revealed that the areas of whole root and cortex of the three species increased under stagnant conditions. In rice roots, cortex to stele ratio (CSR) and aerenchyma to cortex ratio (ACR), which is associated with the areas of gas spaces, were much higher than those in wheat and maize roots, suggesting that these anatomical features are essential for a high capacity for oxygen transport along roots. To test this hypothesis, rates of radial oxygen loss (ROL), which is the diffusive flux of oxygen from within a root to the external medium, from thick and thin adventitious roots of rice were measured using a cylindrical (root-sleeving) oxygen electrode, for plants with shoots in air and roots in an oxygen-free medium. As expected, the rate of ROL from thick roots, which have larger cortex and aerenchyma areas, was higher than that of thin roots. The rate of ROL was highest at the apical part of rice roots, where aerenchyma was hardly detected, but at which cuboidal cell arrangement in the cortex provides tissue porosity. We conclude that high CSR in combination with large root diameter is a feature which promotes oxygen transport from shoot base to root tips of plants. Moreover, we propose that CSR should be a useful quantitative index for the evaluation and improvement of root traits contributing to tolerance of crops to soil waterlogging.
Introduction
Roots are mainly composed of four concentric cell layers: from the outside to inside, these cell layers are the epidermis, cortex, endodermis, and stele (Scheres et al., 2002). The stele contains xylem vessels and phloem tissue which are essential for the transport of water, inorganic ions, and organic compounds (Nissen, 1974; Petricka et al., 2012), as well as a pith in the case of monocots (Watt et al., 2008). The primary root of Arabidopsis (Arabidopsis thaliana) has a single cortex cell layer which is produced by the asymmetric cell division of cortex/endodermis initial cell (Dolan et al., 1993; Laurenzio et al., 1996). By contrast, roots of rice (Oryza sativa) have several cortex cell layers produced by the sequential cell divisions of the endodermal cells (Kamiya et al., 2003). Unlike Arabidopsis, many plant species including the family Poaceae, members of which include important crops, have several cortex cell layers (Armstrong, 1979; Justin and Armstrong, 1987).
The principal cause of damage to plants grown in waterlogged soil is inadequate supply of oxygen to submerged roots (Armstrong, 1979; Colmer and Voesenek, 2009). Therefore, internal oxygen transport from shoot base to root tips is crucial for the survival of plants under waterlogged conditions (Armstrong, 1979; Colmer, 2003b). Diffusivity of oxygen in water is approximately 10,000-fold slower than in air (Armstrong and Drew, 2002). Because plants have no active oxygen transport mechanisms, oxygen movement from shoot to submerged tissues is dominated by diffusion (Armstrong and Armstrong, 2014). Aerenchyma consists of longitudinally connected gas spaces which enhances the diffusion of oxygen within plants (Armstrong, 1979; Colmer, 2003b). Lysigenous aerenchyma in roots is formed by the creation of gas spaces as a result of death and the subsequent lysis of cortical cells (Evans, 2003). Plants in the family Poaceae, such as wheat (Triticum aestivum), maize (Zea mays ssp. mays), and rice form lysigenous aerenchyma in the roots (Yamauchi et al., 2018). Under aerobic conditions, roots of wheat and maize typically form small amounts of aerenchyma, but the amount of aerenchyma nevertheless increases under oxygen-deficient conditions (Abiko et al., 2012; Mano and Omori, 2013; Yamauchi et al., 2014b). Rice forms aerenchyma constitutively, and its formation further increases under oxygen-deficient conditions (Colmer et al., 2006; Shiono et al., 2011; Yamauchi et al., 2016, 2017). Oxygen within aerenchyma will be consumed by the cells in adjacent tissues and/or diffuse radially to the rhizosphere (Armstrong, 1979; Colmer, 2003b). In addition to the large amount of aerenchyma, rice roots form a barrier impermeable to radial oxygen loss (ROL), and this further enhances longitudinal oxygen diffusion from shoot base to the root tip (Armstrong, 1979; Colmer et al., 1998; Kotula et al., 2009; Shiono et al., 2011). In contrast to the many wetland species, none of the upland crops have ROL barrier in the roots, and thus its formation is an important mechanism for plants to adapt to waterlogging (Colmer, 2003b).
The amount of longitudinal oxygen diffusion along submerged organs is determined by anatomical, morphological, and physiological characteristics (Colmer, 2003b). Root thickness is a morphological feature which associates with the porosities (i.e., gas volumes) within roots (Colmer, 2003b). Root porosities under oxygen-deficient conditions are higher in the wetland species having thicker root diameters when compared with the dryland species with thinner root diameters (Visser et al., 2000a,b). From the anatomical aspects, thick root diameter is associated with large cortex and aerenchyma areas. Indeed, thicker roots of wheat seedlings that emerge under oxygen-deficient conditions have a larger aerenchyma area than thinner roots that emerge under aerobic conditions (Yamauchi et al., 2014a). On the other hand, mathematical modelings showed that large stele in which porosity is lower than in the cortex has a disadvantage in terms of oxygen diffusion within the root (Armstrong and Beckett, 1987; Armstrong et al., 1994, 2000). Indeed, the proportion of the stele area within roots is smaller in the wetland species than that in dryland species (McDonald et al., 2002). These studies suggest that not only aerenchyma, but also root thickness and ratio of cortex-to-stele within roots are essential to consider the plant adaptability to waterlogging.
The aim of this study was to understand the significance of higher CSR, which is associated with larger cortex area, for the adaptation of plants to waterlogging. Specifically, we addressed the following questions. (1) How is CSR related with plant adaptation to waterlogging? (2) Does CSR affect the amount of gas space in root? (3) What is the adaptive value of high CSR in terms of root aeration? To this end, we evaluated growth of wheat, maize, and rice seedlings under aerated or stagnant deoxygenated conditions, which can mimic the changes in gas composition in waterlogged soils. Moreover, we measured areas of stele, cortex, and aerenchyma in cross sections of adventitious roots and calculated the ratio of each tissue. To further evaluate the advantage of larger cortex to enhance internal oxygen movement within roots, we measured rates of ROL from thick and thin adventitious roots of rice seedlings by a cylindrical oxygen electrode, when in an oxygen-free root zone. We also measured elongation of the two types of adventitious roots under stagnant conditions. Based upon these results, here we discuss the adaptive consequence of high CSR to waterlogging and the potential application of CSR to improve tolerance of crops to waterlogging.
Materials and Methods
Plant Materials and Growth Conditions
Seeds of wheat (cv. Bobwhite), maize (inbred line B73), and rice (cv. Nipponbare) were sterilized with 0.5% (v/v) sodium hypochlorite for 30 min and then rinsed thoroughly with deionized water. Seeds of maize and wheat were germinated on filter paper in Petri dishes with deionized water. Each species was in a different growth chamber; maize was at 28°C in light [photosynthetically active radiation (PAR), 200–250 μmol m−2 s−1] for 16 h and 25°C in dark for 8 h, and wheat at 23°C under continuous light conditions (PAR, 200–250 μmol m−2 s−1), respectively. Seeds of rice were germinated in Petri dishes with deionized water in growth chamber at 28°C under dark conditions. After 2 days, maize seedlings were placed on a mesh-float with an aerated half-strength nutrient solution [28°C in light (PAR, 200–250 µmol m−2 s−1) for 16 h and 25°C in dark for 8 h]. Wheat and rice seedlings were each placed on each different mesh-floats with an aerated quarter-strength nutrient solution [23°C (wheat) or 28°C (rice) continuous light conditions; PAR, 200–250 μmol m−2 s−1]. Composition of the nutrient solution for maize was as described by Watanabe et al. (2017), and that of the nutrient solution for wheat and rice was as described by Colmer et al. (2006). After 7 days (9 days old), wheat, maize, and rice seedlings were transferred to 2-L pots (2 plants per pot, 250 mm height × 80 mm length × 110 mm width) containing aerated full-strength nutrient solution (aerated conditions) or stagnant deoxygenated solution (stagnant conditions) and grown for 7 days, and 16-day-old seedlings were used for each experiment. For maize, FeSO4 (final concentration of 1 μM) was added to aerated nutrient solution every day on days 9–15. For 14 days of treatment of rice seedlings, stagnant deoxygenated solution was renewed at 7 days after the start of treatment (16 days old), and 23-day-old seedlings were used for each experiment. Stagnant deoxygenated solution contained 0.1% (w/v) dissolved agar and was deoxygenated (dissolved oxygen, <0.5 mg L−1) prior to use by flushing with nitrogen gas (Wiengweera et al., 1997).
Measurements of Growth and Leaf Chlorophyll Content
Wheat, maize, and rice seedlings were harvested at 7 days (16 days old) after the transfer to aerated or stagnant conditions. Lengths of shoots, longest seminal roots, and longest adventitious roots were measured using a ruler, and number of emerged leaves, adventitious roots, and tillers were counted. Chlorophyll content of leaves was measured three times at the middle part of leaves using a Soil Plant Analysis Development (SPAD) meter (SPAD-502, Konica Minolta), and the average value was used as the SPAD value of each individual. Wheat, maize, and rice seedlings were divided into shoots and roots and dried at 50°C for 7 days, and shoot and root dry weights were measured.
Anatomical Analysis of Root Cross Sections
Root segments at 10, 20, 30, 40, and 50 mm (±2 mm) from the tips were prepared from 80- to 100-mm-long adventitious roots of wheat, maize, and rice seedlings grown under aerated or stagnant conditions for 7 days (16 days old). For the comparison between the thick and thin adventitious roots, root segments at 5, 10, 20, 30, 40, and 50 mm (±2 mm) from the tips were prepared from 110- to 130-mm-long adventitious roots of rice seedlings grown under stagnant conditions for 14 days (23 days old). Root cross sections were prepared by hand sectioning with a razor blade. Each section was photographed using an optical microscope (BX60, OLYMPUS) with a CCD camera (DP70, OLYMPUS). Areas of each root tissue were measured using ImageJ software (Ver. 1.43u, US National Institutes of Health), and the ratio (proportion) of each root tissue was calculated using the cross-sectional areas.
Detection of Oxygen Loss From Roots by Redox Indicator Dyes
Methylene blue is a redox indicator dye which enables qualitative assessments of spatial ROL from roots (Armstrong and Armstrong, 1988). Crystal violet was applied as an alternative redox indicator dye. The reduced forms of methylene blue and crystal violet are colorless, but when they react with oxygen this results in blue and purple colors, respectively. Methylene blue and crystal violet were added to a deoxygenated solution containing 0.1% (w/v) agar at final concentration of 30 and 10 μM, respectively. Subsequently, sodium dithionite (Na2S2O3) was added at a final concentration of 300 μM to reduce the indicator dyes. One each of thick and thin adventitious roots (110–130 mm long) were selected from rice seedlings grown under stagnant conditions for 14 days (23 days old), and all of the other roots were trimmed off immediately before the start of staining. The shoot base with the adventitious root was immersed in a transparent acrylic pot (350 mm height × 250 mm length × 15 mm width) containing the reduced methylene blue or crystal violet solution. The shoot-root junction was 20 mm below the surface of the solution. The staining patterns of methylene blue and crystal violet on the adventitious roots were evaluated every 30 min for a period of 2 h, and photographs were taken at 2 h (methylene blue) and 1 h (crystal violet) after the start of the staining experiments.
Measurement of Oxygen Loss From Roots by a Cylindrical Electrode
One each of thick and thin adventitious roots (110–130 mm long) were selected from rice seedlings grown under stagnant conditions for 14 days (23 days old). Oxygen loss from the adventitious roots of intact rice seedlings was measured by using a cylindrical oxygen electrode in accordance with the method of Armstrong and Wright (1975). The adventitious roots were immersed in a transparent acrylic pot (250 mm height × 80 mm length × 110 mm width) containing a deoxygenated solution of 5 mM KCl, 0.5 mM CaSO4, and 0.1% (w/v) dissolved agar (Colmer, 2003a), and placed in a growth chamber [28°C, light conditions; PAR, 200–250 μmol m−2 s−1]. The shoot-root junction was immersed 20 mm below the surface of the deoxygenated solution. One adventitious root was inserted through the cylindrical electrode (inner diameter: 2.25 mm; height: 5 mm), and the oxygen loss from the root surface was measured at 5, 10, 20, 30, 40, 50, and 60 mm (±2.5 mm) from the tips of adventitious roots. After the measurements, root cross sections were prepared as described above. The rates of total oxygen loss (ng min−1) within the range of cylindrical electrode was calculated by [−4.974 × I], and the rates of ROL (ng cm−2 min−1) were calculated by [−4.974 × I/A1] as described by Armstrong and Wright (1975), where I = diffusion current (μA) with the adventitious roots in the cylindrical electrode, and the A1 = surface area of adventitious roots within the electrode (m−2). After the calculations, the units of the rates of total oxygen loss and ROL were converted into nmol s−1 and nmol m−2 s−1, respectively.
Measurement of Root Elongation
One each of thick and thin adventitious roots (10–40 mm long) were selected from rice seedlings grown under stagnant conditions for 7 days (16 days old). The selected roots were marked by threads, and their lengths were measured every day on days 16–26. Stagnant deoxygenated solution was renewed at day 16, when the measurement started.
Statistical Analyses
Statistical differences between means were calculated using two-sample t-test. For multiple comparisons, data were analyzed by one-way ANOVA and post hoc Tukey’s test using SPSS Statistics Version 19 (IBM Software).
Results
Growth of Wheat, Maize, and Rice Seedlings Under Aerated and Stagnant Conditions
To evaluate the effect of stagnant conditions on growth of wheat, maize, and rice, 9-day-old aerobically grown seedlings were further grown under aerated or stagnant conditions for 7 days (Figures 1A–F). Stagnant conditions significantly decreased the shoot lengths of wheat and maize, and also reduced leaf and tiller numbers of maize and wheat, respectively (Table 1). Shoot dry weights of wheat and maize decreased by 11 and 13%, respectively (Table 1). In rice, stagnant conditions did not affect shoot length, leaf, and tiller numbers, and thus shoot dry weight was similar under aerated and stagnant conditions (Table 1). The chlorophyll content (SPAD value) of second and third leaves of wheat and maize significantly decreased under stagnant conditions (Table 1). In rice, the chlorophyll content of the second leaf was not affected, and that of the third leaf rather increased when plants were growing under stagnant conditions (Table 1).
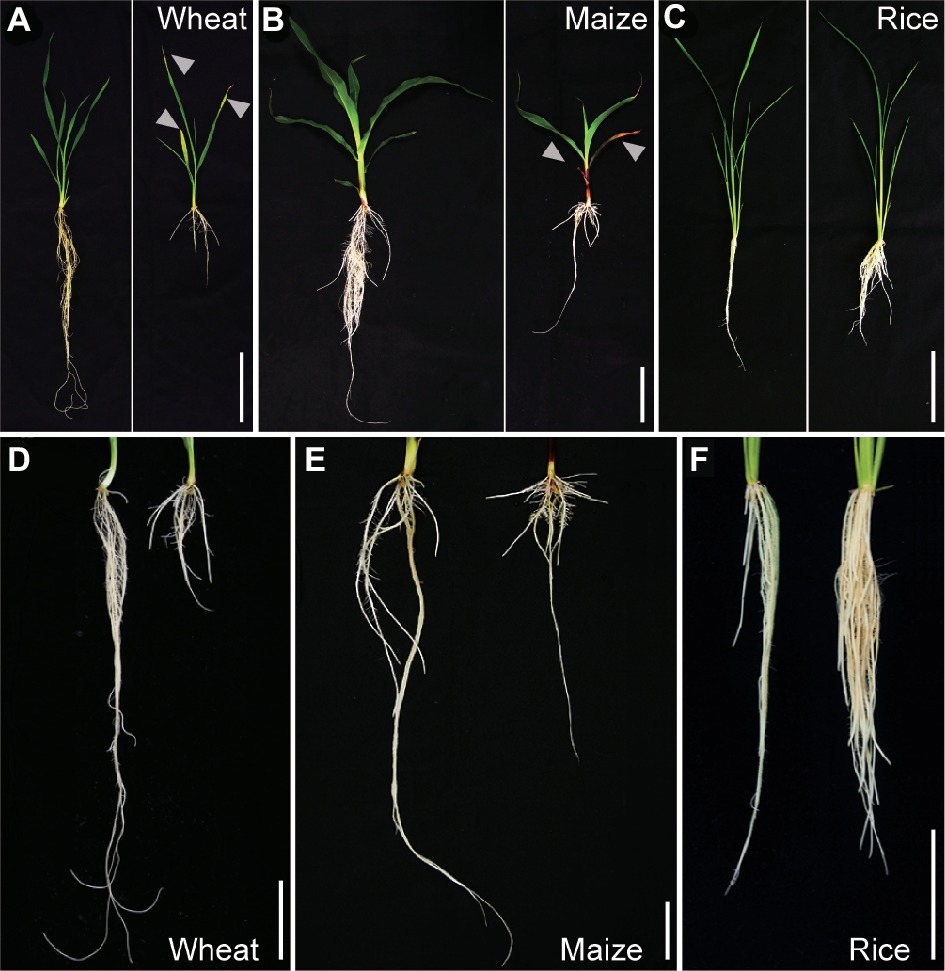
Figure 1. Growth of wheat, maize, and rice under aerated or stagnant conditions. Pictures of seedlings of wheat (A), maize (B), and rice (C) and roots of wheat (D), maize (E), and rice (F) under aerated conditions (left) or stagnant conditions (right). Nine-day-old wheat, maize, and rice seedlings were further grown under aerated or stagnant conditions for 7 days. Yellowing or brownish parts of leaves are indicated by arrowheads. Bars = 10 cm.
Root dry weights of wheat and maize respectively decreased by 70 and 27% under stagnant conditions, whereas that of rice increased by 132% (Table 1). The lengths of longest adventitious roots decreased by 43, 67, and 21% in wheat, maize, and rice under stagnant conditions, respectively (Table 1). Stagnant conditions increased the numbers of adventitious roots in wheat and rice by 14 and 29%, respectively (Table 1). By contrast, the number of adventitious roots in maize was not affected (Table 1). Seminal roots strongly contribute to the root dry weights of wheat and maize under aerated conditions, but most of those roots died during the growth under stagnant conditions (Figures 1D, E; Table 1). These results clearly show that rice is more adaptive to stagnant conditions (which mimic waterlogging) than wheat and maize.
Size of Each Root Tissue of Wheat, Maize, and Rice Under Aerated and Stagnant Conditions
Root cross sections at 10, 20, 30, 40, and 50 mm from the tips of 80- to 100-mm-long adventitious roots were prepared from wheat, maize, and rice seedlings grown under aerated or stagnant conditions, and the size of each root tissue was measured (at 50 mm; Figures 2A–C). Areas of whole root, stele, and cortex at 50 mm from the tips of wheat, maize, and rice roots, except for stele area of wheat roots, significantly increased under stagnant conditions (Figures 2D–F). Stele areas in maize and rice roots increased by 72 and 40%, respectively (Figure 2E). In wheat, maize, and rice, areas of cortex respectively increased by 49, 164, and 88% under stagnant conditions (Figure 2F). These results indicate that the increases of the cortex areas are greater than those of stele areas under stagnant conditions. Similar results were obtained at 10, 20, 30, and 40 mm from the tips of adventitious roots of wheat, maize, and rice seedlings (Supplementary Figures S1A–C, S2A–C, S3A–C).
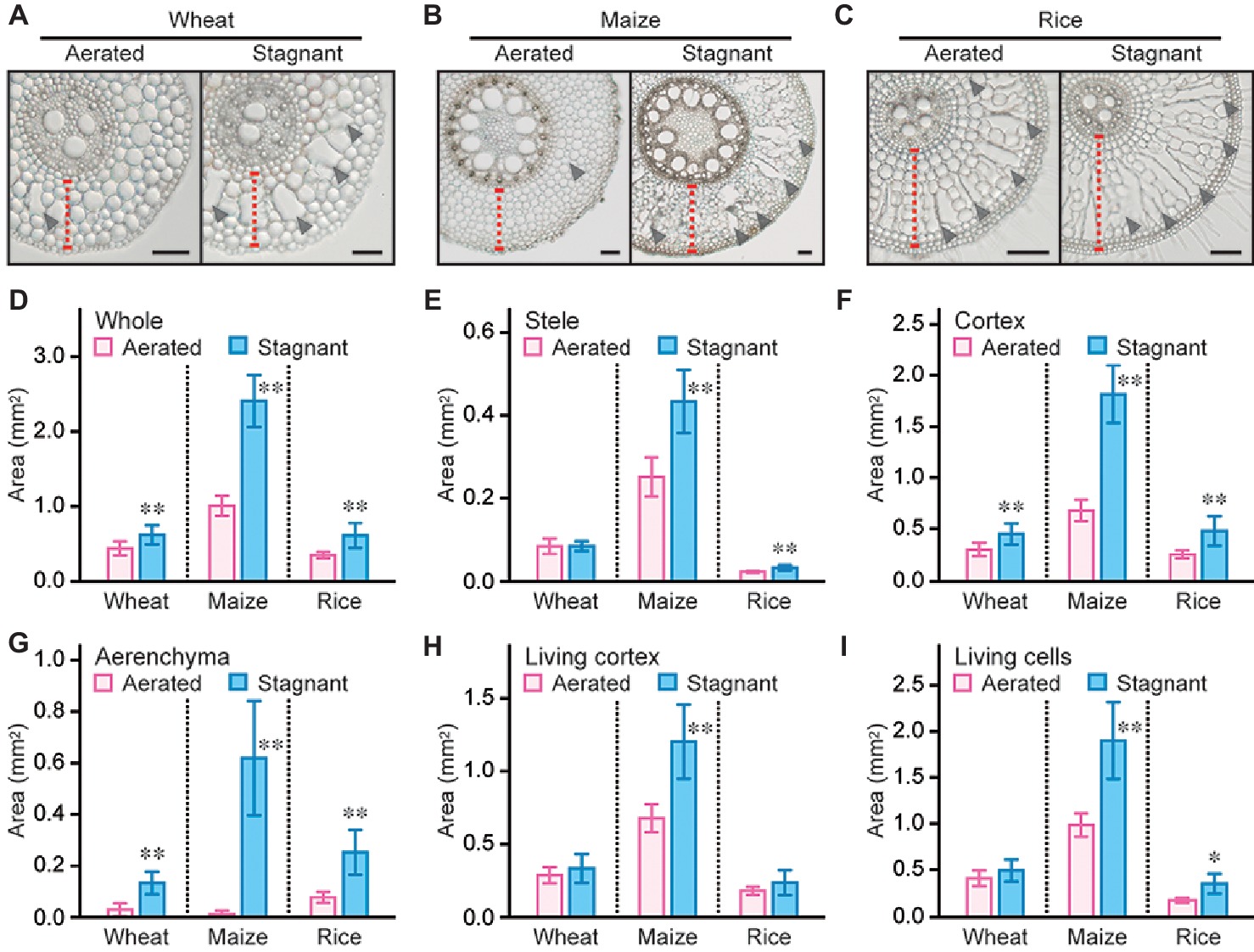
Figure 2. Area of each tissue in adventitious roots of wheat, maize, and rice. Cross sections at 50 mm from the tips of adventitious roots of wheat (A), maize (B), and rice (C) under aerated or stagnant conditions. Cortex cell layers and lysigenous aerenchyma are indicated by red dashed lines and black arrowheads, respectively. Bars = 100 μm. Areas of whole root (D), stele (E), cortex (F), aerenchyma (G), living cortex (cortex minus aerenchyma) (H), and living cells (whole root minus aerenchyma) (I) at 50 mm from the tips of adventitious roots of wheat, maize, and rice seedlings under aerated or stagnant conditions. Nine-day-old wheat, maize, and rice seedlings were further grown under aerated or stagnant conditions for 7 days. Significant differences between aerated and stagnant conditions at p < 0.01 and p < 0.05 (two-sample t-test) are denoted by ∗∗ and ∗, respectively. Values are means ± SD (n = 9).
Although aerenchyma formation was minor in wheat and maize roots under aerated conditions, aerenchyma formation gradually increased toward basal parts of the roots under stagnant conditions (Supplementary Figures S1D, S2D). In rice roots, aerenchyma formation was first detected at 20 mm from the tips both under aerated and stagnant conditions, and its formation gradually increased toward basal parts of the roots (Supplementary Figure S3D). Areas of aerenchyma at 50 mm from the root tips significantly increased in wheat, maize, and rice roots under stagnant conditions (Figure 2G).
Because the area of whole roots in maize strongly increased under stagnant conditions (Figure 2D), areas of living cortex (cortex minus aerenchyma; 77%) and living root cells (whole roots minus aerenchyma; 92%) also strongly increased (Figures 2H,I). In wheat roots, areas of living cortex and living root cells were comparable between aerated and stagnant conditions (Figures 2H, I). In rice roots, areas of living cortex were comparable between aerated and stagnant conditions, and the area of living root cells increased by 32% under stagnant conditions (Figures 2H, I). Areas of living cortex gradually decreased toward basal parts of the roots in maize and rice, but not in wheat, under stagnant conditions (Supplementary Figures S1E, S2E, S3E). Area of living root cells in rice also gradually decreased toward the basal part of the roots, whereas those of living root cells did not significantly change along wheat and maize roots (Supplementary Figures S1F, S2F, S3F).
Ratio of Each Root Tissue Size of Wheat, Maize, and Rice Under Aerated and Stagnant Conditions
To compare the root anatomical features of wheat, maize, and rice, the ratio of each root tissue was calculated (Figure 3; Supplementary Figures S4–S6). Stagnant conditions significantly increased aerenchyma to cortex ratio (ACR) and cortex to stele ratio (CSR) at 50 mm from the tips of adventitious roots in wheat, maize, and rice (Figures 3A,B). ACR and CSR in rice roots were much higher than those in wheat and maize roots both under aerated and stagnant conditions (Figures 3A, B). ACR in rice roots under stagnant conditions was respectively 1.8-fold and 1.5-fold higher than that in wheat and maize roots (Figure 3A), and CSR in rice roots under stagnant conditions was respectively 2.8-fold and 3.5-fold higher than that in wheat and maize roots (Figure 3B). These results indicate that rice roots have more aerenchyma within cortex when compared with wheat and maize roots, and suggest that higher CSR in rice roots further amplifies the ratio of aerenchyma within whole root. Indeed, aerenchyma to whole root ratio in rice roots under stagnant conditions was respectively 2.0-fold and 1.6-fold higher than that in wheat and maize roots (Figure 3C), and the proportion of difference between rice roots and wheat or maize roots was larger than ACR (Figures3A, C). Moreover, ratio of living cells to whole root in rice roots under stagnant conditions was 1.3-fold lower than that in wheat and maize roots under stagnant conditions (Figure 3D), and ratio of aerenchyma to living cells in rice roots was respectively 2.7-fold and 2.2-fold higher than that in wheat and maize roots under stagnant conditions (Figure 3E). These results indicate that rice has larger area of aerenchyma and smaller area of living cells within roots when compared with wheat and maize. In other words, rice roots suppress oxygen demands of living cells and enhance oxygen diffusion rates through aerenchyma to adapt to waterlogging.
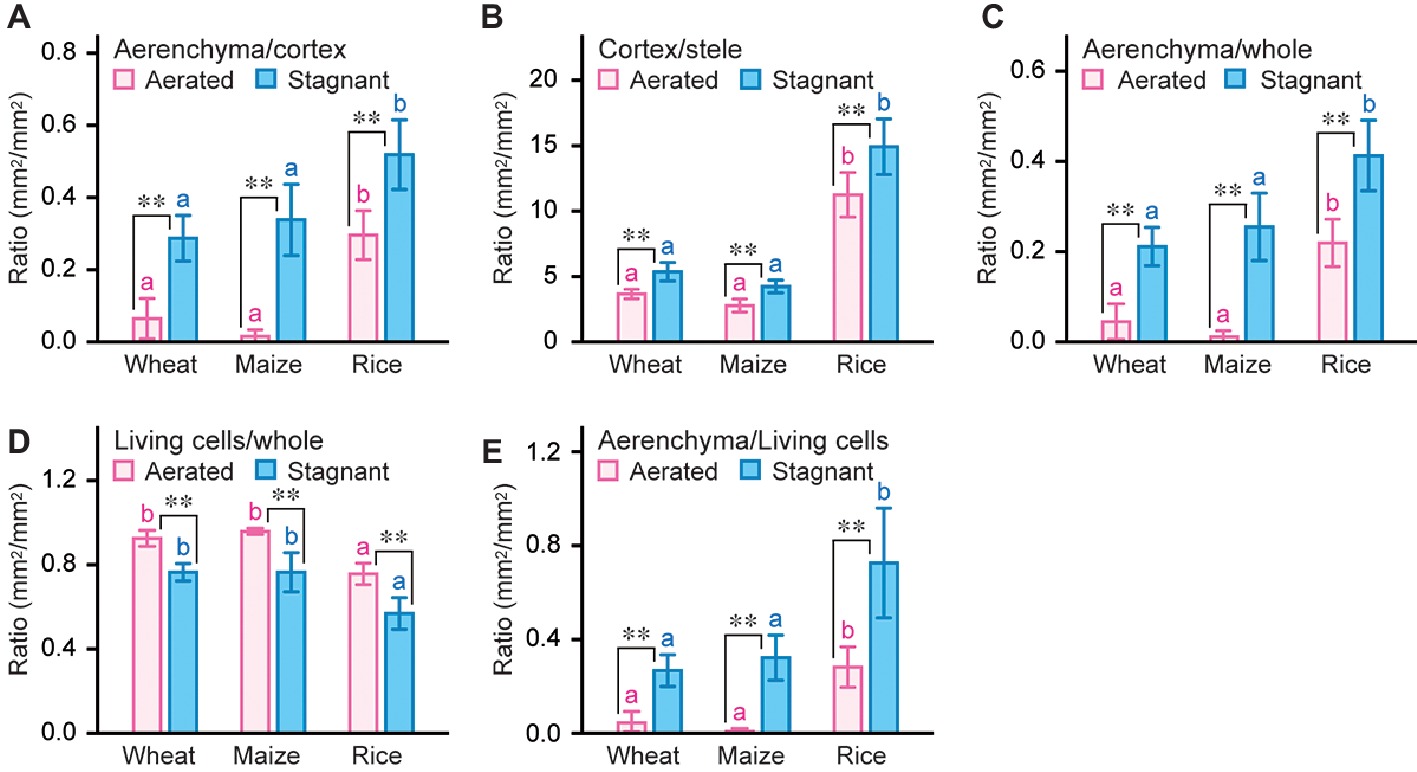
Figure 3. Ratio of each tissue size in adventitious roots of wheat, maize, and rice. Ratio of aerenchyma to cortex (A), cortex to stele (B), aerenchyma to whole root (C), living cells to whole root (D), and aerenchyma to living cells (E) at 50 mm from the tips of adventitious roots of wheat, maize, and rice under aerated or stagnant conditions. Nine-day-old wheat, maize, and rice seedlings were further grown under aerated or stagnant conditions for 7 days. Significant differences between aerated and stagnant conditions at p < 0.01 (two-sample t-test) are denoted by ∗∗. Different lowercase letters denote significant differences among different species (p < 0.05, one-way ANOVA and then Tukey’s test for multiple comparisons). Values are means ± SD (n = 9).
Anatomy of Thick and Thin Rice Roots Under Stagnant Conditions
Analysis of root anatomical features in wheat, maize, and rice revealed that the ratio of cortex area within roots in rice is higher than that in wheat and maize roots (Figure 3B). To demonstrate the advantages of high CSR in rice roots for oxygen transport from shoot to root, one each of thick and thin adventitious roots (110- to 130-mm long) were selected from each rice seedling grown under stagnant conditions for 14 days, and anatomical features of those roots were evaluated (Figures 4A, B). Whole root areas at 10, 20, 30, 40, and 50 mm from the tips of thick roots were 1.9- to 2.1-fold larger than those of thin roots (Supplementary Figure S7A). Although stele and cortex areas of thick roots were respectively 1.8- to 1.9-fold and 2.0- to 2.2-fold larger than those of thin roots (Figures 4C, D), CSR was not significantly different between the two types of roots (Supplementary Figure S7B). Areas of aerenchyma at 10 and 20 mm of thick and thin roots were comparable with each other, whereas those at 30–50 mm were 1.8- to 2.0-fold larger in thick roots (Figure 4E). Areas of living cells of thick roots were 1.9- to 2.2-fold larger than those of thin roots (Figure 4F). Interestingly, the longitudinal gradients of areas of aerenchyma and living cells were more prominent in thick roots than those in thin roots (Figures 4E, F). Aerenchyma to whole root ratio, living cells to whole root ratio, and aerenchyma to living cells ratio were not significantly different between thick and thin roots (Supplementary Figures S7C–E). These results suggest that thick roots have larger oxygen demands at the apical part due to having more living cells, and that thick roots have larger amounts of aerenchyma at the basal part when compared with thin roots. Taken together, these features could synergistically enhance oxygen diffusion from shoot base to the tips of thick roots.
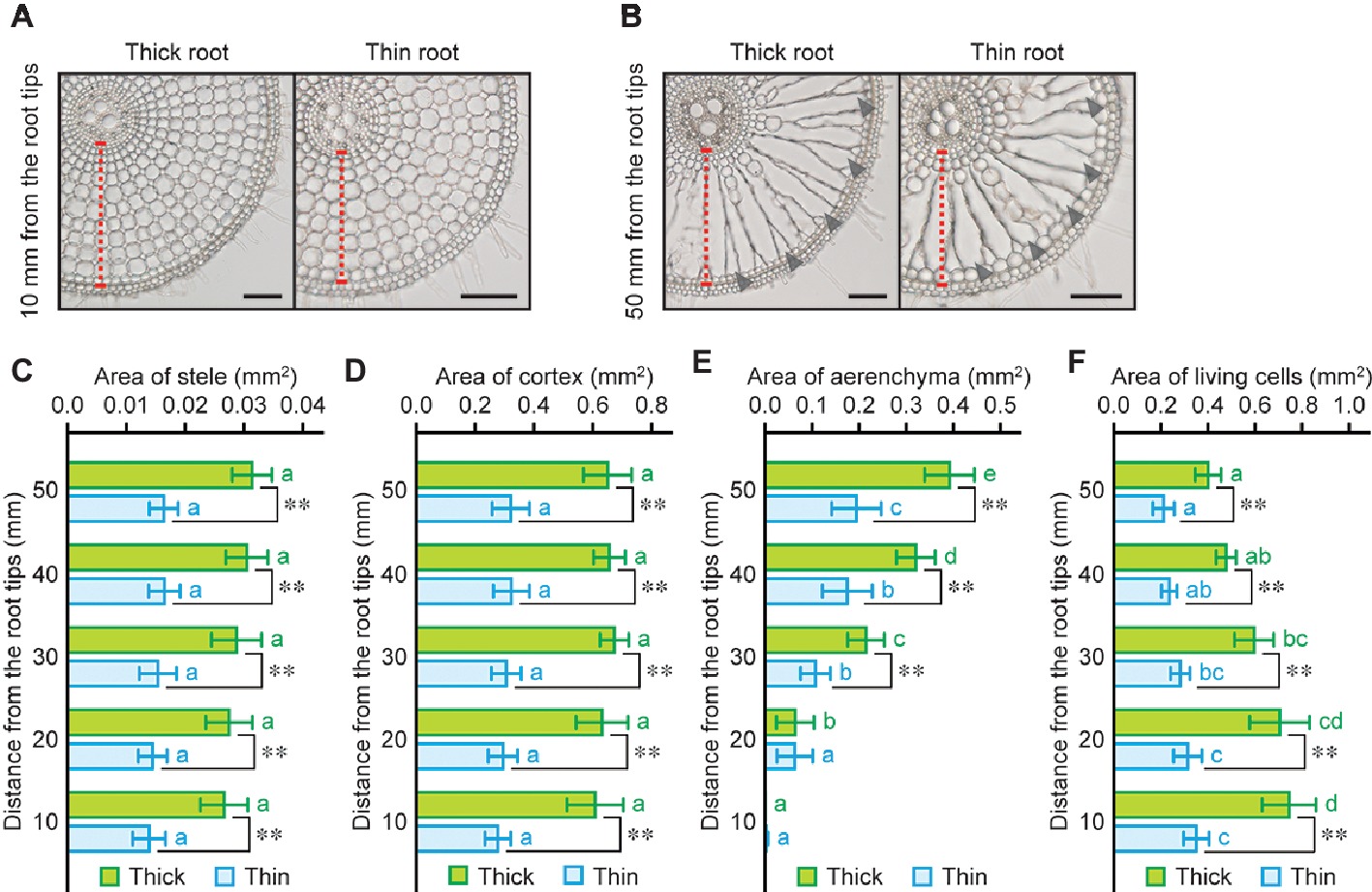
Figure 4. Size of each tissue in thick and thin adventitious roots of rice. Cross sections at 10 mm (A) and 50 mm (B) from the tips of thick and thin adventitious roots of rice under stagnant conditions. Cortex cell layers and lysigenous aerenchyma are indicated by red dashed lines and black arrowheads, respectively. Bars = 100 μm. Area of stele (C), cortex (D), aerenchyma (E), and living cells (F) at 10, 20, 30, 40, and 50 mm from the tips of thick and thin adventitious roots of rice seedlings under stagnant conditions. Nine-day-old rice seedlings were further grown under stagnant conditions for 14 days. Significant differences between thick and thin roots at p < 0.01 (two-sample t-test) are denoted by ∗∗. Different lowercase letters denote significant differences among different positions of roots (p < 0.05, one-way ANOVA and then Tukey’s test for multiple comparisons). Values are means ± SD (n = 8).
Oxygen Loss From Thick and Thin Rice Roots Under Stagnant Conditions
To evaluate the contribution of cortex to the oxygen transport from shoot base to the root tips, oxygen loss from the thick and thin adventitious roots of rice seedlings were detected by redox indicator dyes, methylene blue, and crystal violet, under the deoxygenated solution. We found that thick roots with larger cortex and aerenchyma areas showed more dense and broad staining patterns (Figures 5A, B). Subsequently, rates of oxygen loss within a cylindrical oxygen electrode (total oxygen loss) from thick and thin adventitious roots were measured at 5, 10, 20, 30, 40, 50, and 60 mm (±2.5 mm) in deoxygenated solution (Figure 5C). The total oxygen loss was hardly detectable at 30–60 mm from the tips of thick and thin roots, indicating that the both types of roots had formed a strong barrier to ROL in our experimental conditions (Figure 5C). Although the patterns of total oxygen loss along roots were similar between both types of roots, the rates of total oxygen loss at 5 and 10 mm of thick roots were 1.8- and 2.2-fold higher than those in thin roots, respectively (Figure 5C). Root diameters of thick roots at 5–60 mm from the tips were 1.3- to 1.5-fold higher than those in thin roots (Figure 5D). Nevertheless, the rates of ROL (total oxygen loss/root surface area) at 5 and 10 mm of thick roots were 1.4- and 1.6-fold higher than those of thin roots (Figure 5E). These results indicate that large cortex and aerenchyma areas strongly contribute to the efficient oxygen transport within rice roots.
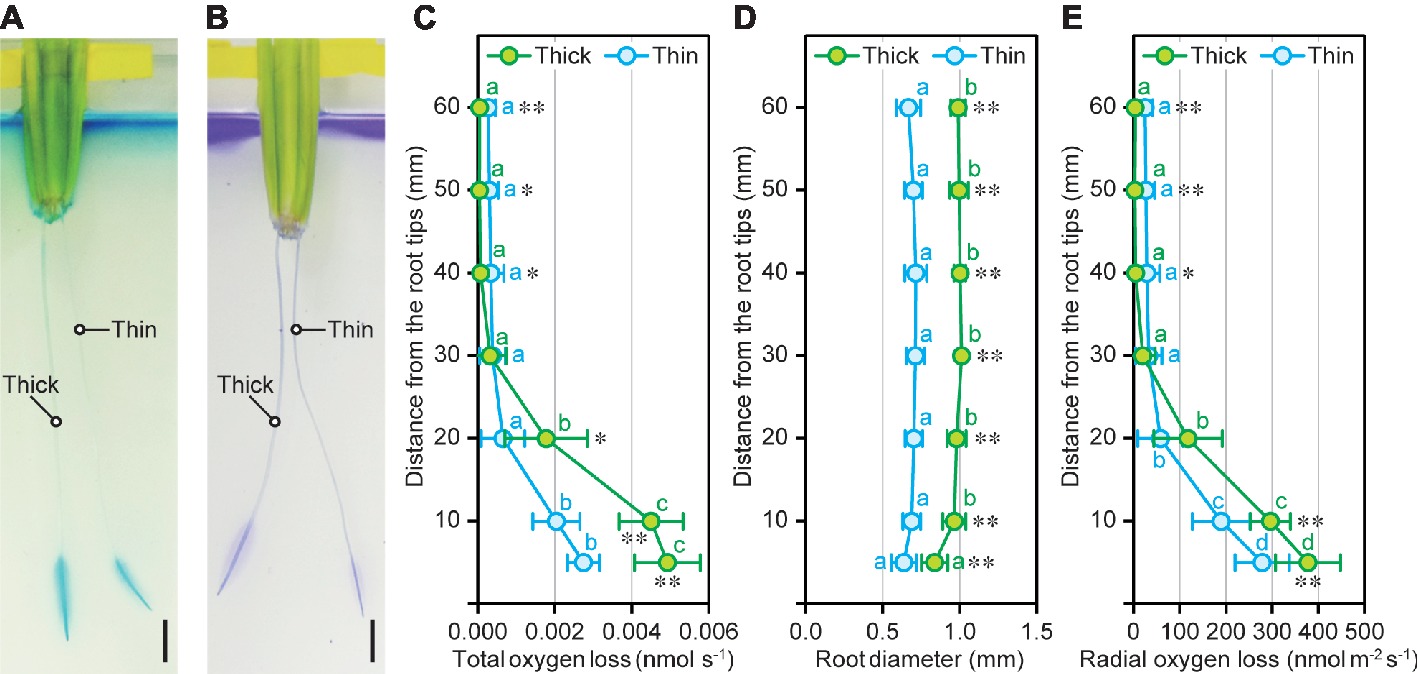
Figure 5. Oxygen movement in thick and thin adventitious roots of rice. Methylene blue staining (A) and crystal violet staining (B) of thick and thin adventitious roots of rice seedlings under stagnant conditions. Bars = 10 mm. Total oxygen loss from adventitious roots (C), adventitious root diameter (D), and radial oxygen loss (ROL) from adventitious roots (E) at 5, 10, 20, 30, 40, 50, and 60 mm from the tips of thick and thin adventitious roots of rice seedlings under stagnant conditions. Nine-day-old rice seedlings were further grown under stagnant conditions for 14 days. Significant differences between thick and thin roots at p < 0.01 and p < 0.05 (two-sample t-test) are denoted by ∗∗ and ∗, respectively. Different lowercase letters denote significant differences among different positions of roots (p < 0.05, one-way ANOVA and then Tukey’s test for multiple comparisons). Values are means ± SD (n = 8).
Intercellular Space of Thick and Thin Rice Roots Under Stagnant Conditions
High rates of oxygen loss were detected at 5 mm from the tips of thick and thin adventitious roots of rice seedlings (Figure 5E), even though aerenchyma formation was hardly detectable (at 10 mm; see Figure 4E). Although thick roots have larger cortex area (Figure 4D), areas of aerenchyma at 10 and 20 mm of thick roots were comparable with those of thin roots (Figure 4E). Moreover, ACR at 20 mm was smaller in thick roots than that in thin roots (Figures 6A, B). To further evaluate the contribution of large cortex area to the efficient oxygen transport within roots, areas of intercellular spaces at 5 mm of thick and thin roots were measured. Because number of cortex cell layers of thick roots was larger than that in thin roots (Figures 6C, D), area of intercellular spaces per two cortical cell files was significantly larger in thick roots (1.4-fold; Figure 6E). Moreover, thick roots have more cortical cell files (Figure 6F), and thus they have greater total intercellular spaces in the cortex than those in thin roots (1.7-fold; Figure 6G). Interestingly, area of intercellular spaces at 5 mm of thick roots was almost equal to half of aerenchyma area at 20 mm of thick roots (Figures 4E, 6G). These results indicate that larger cortex contributes to form larger gas spaces, which are comprised of not only aerenchyma but also intercellular spaces, and thus, it is essential for efficient internal oxygen transport from shoot base to the root tips of rice.
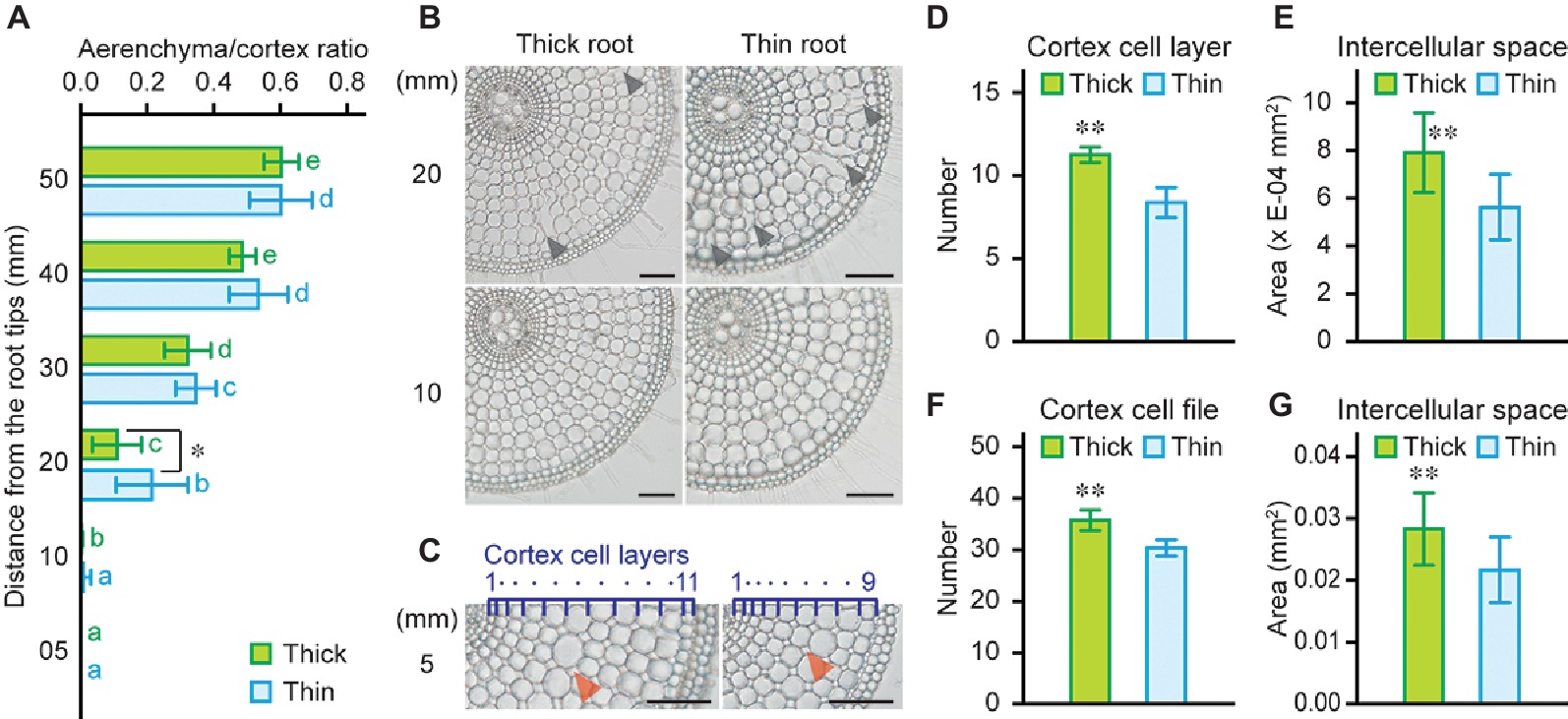
Figure 6. Intercellular spaces of thick and thin adventitious roots of rice. (A) Ratio of aerenchyma to cortex at 5, 10, 20, 30, 40, and 50 mm from the tips of thick and thin adventitious roots of rice under stagnant conditions. (B) Cross-sections at 10 and 20 mm from the tips of thick and thin adventitious roots under stagnant conditions. Lysigenous aerenchyma is indicated by black arrowheads. Bars = 100 μm. (C) Cross-sections at 5 mm from the tips of thick and thin adventitious roots under stagnant conditions. Cortex cell layers are indicated by dark blue numbers and dots. Intercellular spaces are indicated by red arrowheads. Bars = 100 μm. Number of cortex cell layers (D), area of intercellular spaces (per two cortical cell files) (E), number of cortex cell files at the middle part of cortex cell layers (F), and area of total intercellular spaces (G) at 5 mm from the tips of thick and thin adventitious roots under stagnant conditions. Nine-day-old rice seedlings were further grown under stagnant conditions for 14 days. (A,D–G) Significant differences between thick and thin adventitious roots at p < 0.01 and p < 0.05 (two-sample t-test) are denoted by ∗∗ and ∗, respectively. (A) Different lowercase letters denote significant differences among different positions of roots (p < 0.05, one-way ANOVA and then Tukey’s test for multiple comparisons). Values are means ± SD (n = 8).
Elongation of Thick and Thin Rice Roots Under Stagnant Conditions
To further evaluate the advantage of larger gas spaces in thick rice roots on the growth under stagnant conditions, lengths of thick and thin adventitious roots were measured every day up to 10 days (on days 16–26) under stagnant conditions (Figure 7A). Root lengths were comparable between thick and thin roots until 4 days after the start of treatment (Figure 7A). Elongation rate of thin roots became slower than that of thick roots at day 4, and it almost stopped on days 9–10 (Figure 7B). By contrast, the elongation rate of thick roots did not change until day 10 (Figure 7B), and thus differences in lengths of thick and thin roots gradually increased between days 5 and 10 (Figure 7A). These results further support that thick roots with larger cortex and gas spaces are better adapted to waterlogged conditions than thin roots.
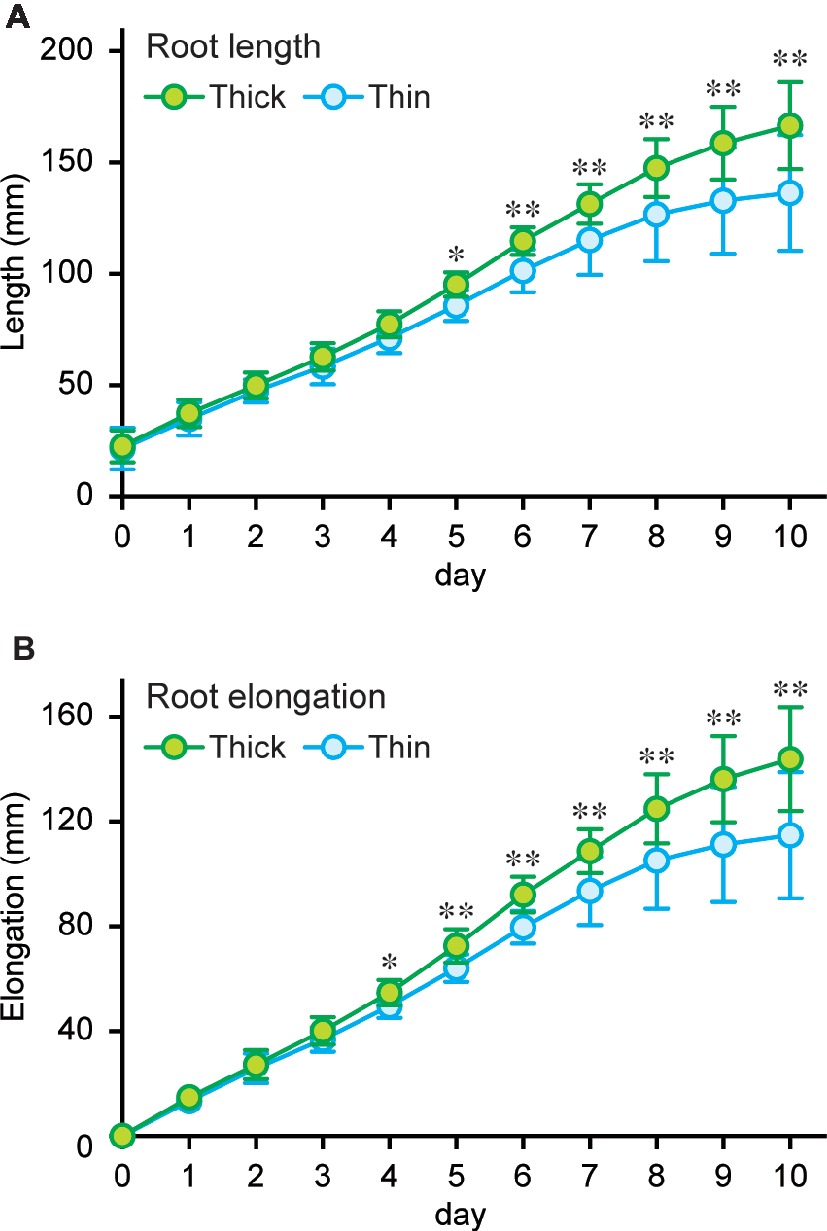
Figure 7. Elongation of thick and thin adventitious roots of rice. Lengths (A) and elongation (B) of thick and thin adventitious roots of rice under stagnant conditions. Nine-day-old rice seedlings were further grown under stagnant conditions for 7 days, and lengths of thick and thin adventitious roots were measured every day on days 16–26 under stagnant conditions. Significant differences between thick and thin adventitious roots at p < 0.01 and p < 0.05 (two-sample t-test) are denoted by ∗∗ and ∗, respectively. Values are means ± SD (n = 12).
Discussion
Growth of rice under stagnant conditions, which mimic the changes in gas composition in waterlogged soil, was greater than for upland crops, wheat and maize (Figure 1; Table 1). Roots of rice, which is generally cultivated in waterlogged paddy fields, have higher ratio of aerenchyma within roots (i.e., ACR) and higher ratio of cortex to stele (i.e., CSR) than those of wheat and maize both under aerated and stagnant conditions (Figures 3A, B). Because the cortex is the tissue in which aerenchyma formation occurs, large CSR in rice roots amplifies the aerenchyma to whole root ratio (Figure 3C), and this also results in rice roots maintaining a smaller amount of living cells (Figure 3D). On the other hand, whole root areas increased in wheat, maize, and rice under stagnant conditions (Figure 2D), and this led to the increases in cortex areas of the three species (Figure 2F). These results suggest that increase in root diameter is a common trait for wetland and upland species in response to waterlogging. While aerenchyma is essential for the efficient internal oxygen transport within roots (Colmer, 2003b; Yamauchi et al., 2018), the cortical cell death (i.e., aerenchyma formation) also contributes to reduce the respiratory costs of roots under oxygen-deficient conditions (Drew, 1997). Indeed, larger CSR in rice roots (Figure 3B), which was associated with higher aerenchyma to whole root ratio and less living cells to whole root ratio (Figures 3C,D), was associated with the better growth of rice under stagnant conditions when compared with wheat and maize having smaller CSRs in the roots (Figure 3B; Table 1). Stele contains xylem vessels and which are essential for water and nutrient transports within roots (Petricka et al., 2012). In maize and barley (Hordeum vulgare), anoxia (no oxygen) or hypoxia (low oxygen) in the stelar cells severely restricted the loading of essential ions into the xylem (Gibbs et al., 1998; Colmer and Greenway, 2011; Kotula et al., 2015). Because the proportion of the stele area within roots is smaller in the wetland species than that in dryland species (McDonald et al., 2002), smaller stele which is associated with larger cortex and aerenchyma areas, could be more adaptive to waterlogged conditions.
Shoot and root dry weights of maize seedlings were much greater than those of wheat and rice seedlings both under aerated and stagnant conditions (Table 1), and the stele area of maize roots was also much larger than those of wheat and rice roots (Figure 2E). A study of 19 grass species showed that plant height at maturity stages correlated positively with the root cross-sectional areas of stele (Pearson correlation coefficient, r = 0.52, p < 0.05) and total xylems (r = 0.63, p < 0.01; Wahl and Ryser, 2000), suggesting that smaller capacities of water and nutrient transports (i.e., smaller stele and xylem vessels) is a disadvantage for the growth of plants. One way to resolve this problem would be to increase the number of roots to compensate less effective water and nutrient transports caused by the smaller stele and xylem sizes. Indeed, adventitious root number of the wetland species Rumex palustris is much larger than that of the non-wetland species Rumex thyrsiflorus both under aerated and stagnant conditions (Visser et al., 1995, 1996). In our experimental conditions, CSR in rice roots was much higher than that in wheat and maize roots, and rice also has a much larger number of roots than wheat and maize (Figure 3B; Table 1). Interestingly, both CSR and root numbers in wheat and rice significantly increased under stagnant conditions (Figure 3B; Table 1). By contrast, root number in maize was not significantly different between aerated and stagnant conditions (Table 1). Previous study showed that root number of another maize inbred line Mi29 is comparable between under aerated and stagnant conditions, whereas the soil waterlogging significantly increases root number when compared with that under drained soil conditions (Abiko et al., 2012). Although further studies are required to demonstrate the correlation between CSR and root number of plants under waterlogging, having high cortex ratio is essential to express the efficient oxygen transport from shoot base to root tips. On the other hand, rice (and other wetland species) may require a large number of roots to compensate for the less efficient water and nutrient uptakes due to the smaller stele ratio than upland species (Figure 3B).
From the results of anatomical analyses along the adventitious roots, we found that the patterns of areas of living cells along roots were different among wheat, maize, and rice (Supplementary Figures S1F, S2F, S3F). In wheat and maize under stagnant conditions, the areas of living cells at apical parts of the roots were not much changed when compared with those at basal parts of the roots (Supplementary Figures S1F, S2F), even though areas of aerenchyma gradually increased toward basal part of the roots (Supplementary Figures S1D, S2D). By contrast, the areas of living cells at apical part of rice roots was much larger than those at basal part of the roots (Supplementary Figure S3F), and the areas of aerenchyma and living cells gradually increased and decreased toward basal part of the roots, respectively (Supplementary Figures S3D,F). Longitudinal oxygen gradient along the root axis is essential for the oxygen diffusion longitudinally from shoot base to root tip (Colmer, 2003b). Oxygen within gas spaces in roots not only diffuses but also is consumed by the root cells, and thus, the death of cells in the root cortex reduces not only the physical resistance of oxygen diffusion but also the respiratory consumption within roots. (Armstrong, 1979; Drew, 1997; Colmer, 2003b). Taking these into account, longitudinal gradient of respiratory activity along roots is particularly important for the efficient oxygen transport from shoot base to root tips. In rice, basal part of the roots has more aerenchyma and less living cells than apical part of the roots (Supplementary Figures S3D,F). If the apical part of rice roots has higher oxygen demands due to larger respiratory activity of living cells, rice roots may have more prominent longitudinal oxygen gradient than wheat and maize roots, although the steepness of the gradient is also influenced by the resistance along the path.
Many wetland species, including rice, form a barrier to ROL at basal parts of the roots, and this further enhances longitudinal oxygen diffusion from shoot base to the root tips (Armstrong, 1979; Colmer, 2003b). Therefore, a barrier to ROL is one of the most important mechanisms for the roots of wetland species to adapt to waterlogging (Colmer 2003b). Profiles of the rates of ROL along thick and thin adventitious roots of rice revealed that thick roots can transport greater amounts of oxygen from shoot base to the root tips than thin roots, even though both types of roots form a barrier to ROL under stagnant conditions (Figure 5C). The rate of ROL, which is expressed on a surface area basis of root to derive the rate, in thick roots was still higher than that in the thin roots (Figure 5E), thereby demonstrating that thick roots have an ability to sustain greater internal oxygen movement than thin roots. The longitudinal gradients of the areas of aerenchyma and living cells along the roots were more prominent in thick roots than those in thin roots (Figures 4E, F), suggesting that the respiratory activity at the apical part of the roots, which results in an oxygen concentration gradient that is the driving force of oxygen diffusion (Colmer, 2003b), is higher in the thick roots than that in the thin roots, and that respiratory consumption at the basal part of roots, which in turn reduces longitudinal oxygen diffusion (Colmer, 2003b), is relatively low in thick roots. Indeed, thick roots elongated more than thin roots under stagnant conditions (Figures 7A, B), further indicating that large cortex and aerenchyma areas within thick roots contribute to efficient oxygen transport from shoot base to the root tips. Although rates of oxygen loss from roots to the external medium depend not only on the amounts of gas spaces but also on root respiration and the tightness of barriers to ROL (Colmer, 2003b), the total flux of oxygen from just behind the root tip was substantially greater for the thicker than the thinner roots (Figure 5C), which supports that these thicker roots have more internal oxygen movement. Further analysis is needed to reveal the entire pictures of all resistances and respiratory consumption along the diffusion path for internal aeration in thick and thin adventitious roots of rice, and also for the roots of wheat and maize.
Interestingly, ROL at the apical part of rice roots, where little aerenchyma formation was detected (Figure 4E), was very high under stagnant conditions (Figure 5E). Further anatomical analysis revealed that intercellular spaces in the cortex strongly contribute to constitute gas spaces in rice roots (Figure 6G), as noted also by Armstrong (1971). Moreover, area of intercellular spaces was larger in thick roots having much cortex cell layers and the cell files than that in thin roots (Figure 6G). Arrangement of the cortical cells can be divided into two types, one is cuboidal and another is hexagonal packing (Colmer, 2003b). The root porosity was much higher at the apical parts of roots with cuboidal packing of cortex cells than those with hexagonal packing of cortical cells (Armstrong, 1971; Justin and Armstrong, 1987). Interestingly, rice roots have cortex cells with cuboidal packing and wheat roots have those with hexagonal packing, and maize roots have cortex cells with mixtures of both types (Figures 2A–C; Supplementary Figures S8A–C). These results suggest that the different arrangements of cortical cells among wheat, maize, and rice roots are also associated with their adaptabilities to waterlogging. It should be noted that the amount of intercellular spaces in cortex is amplified in roots with high CSR as is the case with the amount of aerenchyma in cortex.
From the detailed anatomical analyses and ROL measurements, we suggest that high CSR with large root diameter contributes to the efficient oxygen transport from shoot base to root tips of plants, and thus, it is essential for plant adaptation to waterlogging. Soil waterlogging negatively impacts agricultural yields of upland crops all over the world (Bailey-Serres et al., 2012; Pucciariello et al., 2014; Voesenek and Bailey-Serres, 2015; Herzog et al., 2016), so that the development of waterlogging-tolerant crops by introgression of quantitative trait loci (QTL) associated with root aeration into elite cultivars is urgent (Yamauchi et al., 2018). QTLs for root aerenchyma formation in cereal crops were identified using mapping populations of barley (Zhang et al., 2016) and hybridization of a wetland wild relative Zea nicaraguensis with a maize inbred line (Mano et al., 2016). Here, we showed that CSR is a reliable quantitative index, which likely contributes to waterlogging tolerance and is available for consideration in future QTL analysis of cereal crops. Moreover, CSR might synergistically enhance the root aeration with aerenchyma formation. It is attractive to identify major QTL for CSR using mapping populations of cereal crops and to improve waterlogging tolerance of cereal crops in the future.
Data Availability
All datasets generated for this study are included in the manuscript and/or the Supplementary Files.
Author Contributions
TY designed the research plans, performed the experiments, and wrote the article. MN provided important advice on the research plans, and read the article and gave suggestions. FA and NT provided advice on the research plans, and read the article and gave suggestions.
Funding
This work was supported by PRESTO from Japan Science and Technology Agency (JST) to TY.
Conflict of Interest Statement
The authors declare that the research was conducted in the absence of any commercial or financial relationships that could be construed as a potential conflict of interest.
Acknowledgments
We thank the International Maize and Wheat Improvement Center (CIMMYT), Mexico, for providing the seeds of wheat cv. Bobwhite. We also thank Dr. Yoshiro Mano for kindly providing the seeds of maize cv. B73, reading manuscript, and stimulating discussion, and Drs. Timothy D. Colmer and Ole Pedersen for reading manuscript and stimulating discussion. We also thank Yusaku Uga, Al Imran Marik, Hirokazu Takahashi, Kohtaro Watanabe, Masaru Fujimoto, and Hideki Takanashi for stimulating discussion.
Supplementary Material
The Supplementary Material for this article can be found online at: https://www.frontiersin.org/articles/10.3389/fpls.2019.00259/full#supplementary-material
References
Abiko, T., Kotula, L., Shiono, K., Malik, A. I., Colmer, T. D., and Nakazono, M. (2012). Enhanced formation of aerenchyma and induction of a barrier to radial oxygen loss in adventitious roots of Zea nicaraguensis contribute to its waterlogging tolerance as compared with maize (Zea mays ssp. mays). Plant Cell Environ. 35, 1618–1630. doi: 10.1111/j.1365-3040.2012.02513.x
Armstrong, W. (1971). Radial oxygen losses from intact rice roots as affected by distance from the apex, respiration and waterlogging. Physiol. Plant. 25, 192–197. doi: 10.1111/j.1399-3054.1971.tb01427.x
Armstrong, W., and Armstrong, J. (1988). Phragmites australis – a preliminary study of soil-oxidizing sites and internal gas transport pathways. New Phytol. 108, 373–382. doi: 10.1111/j.1469-8137.1988.tb04177.x
Armstrong, W., and Armstrong, J. (2014). Plant internal oxygen transport (diffusion and convection) and measuring and modeling oxygen gradients. Plant Cell Monographs 21, 267–297. doi: 10.1007/978-3-7091-1254-0_14
Armstrong, W., and Beckett, P. M. (1987). Internal aeration and the development of stelar anoxia in submerged roots: a multishelled mathematical model combining axial diffusion of oxygen in the cortex with radial losses to the stele, the wall layers and the rhizosphere. New Phytol. 105, 221–245. doi: 10.1111/j.1469-8137.1987.tb00860.x
Armstrong, W., Cousins, D., Armstrong, J., Turner, D. W., and Beckett, P. M. (2000). Oxygen distribution in wetland plant roots and permeability barriers to gas-exchange with the rhizosphere: a microelectrode and modelling study with Phragmites australis. Ann. Bot. 86, 687–703. doi: 10.1006/anbo.2000.1236
Armstrong, W., and Drew, M. C. (2002). “Root growth and metabolism under oxygen deficiency” in Plant roots: the hidden half. 3rd edn. eds. Y. Waisel, A. Eshel, and U. Kafkafi (New York and Basel: Marcel Dekker), 729–761.
Armstrong, W., Strange, M. E., Cringle, S., and Beckett, P. M. (1994). Microelectrode and modelling study of oxygen distribution in roots. Ann. Bot. 74, 287–299. doi: 10.1006/anbo.1994.1120
Armstrong, W., and Wright, E. J. (1975). Radial oxygen loss from roots: the theoretical basis for the manipulation of flux data obtained by the cylindrical platinum electrode technique. Physiol. Plant. 35, 21–26. doi: 10.1111/j.1399-3054.1975.tb03861.x
Bailey-Serres, J., Lee, S. C., and Brinton, E. (2012). Waterproofing crops: effective flooding survival strategies. Plant Physiol. 160, 1698–1709. doi: 10.1104/pp.112.208173
Colmer, T. D. (2003a). Aerenchyma and an inducible barrier to radial oxygen loss facilitate root aeration in upland, paddy and deep-water rice (Oryza sativa L.). Ann. Bot. 91, 301–309. doi: 10.1093/aob/mcf114
Colmer, T. D. (2003b). Long-distance transport of gases in plants: a perspective on internal aeration and radial oxygen loss from roots. Plant Cell Environ. 26, 17–36. doi: 10.1046/j.1365-3040.2003.00846.x
Colmer, T. D., Cox, M. C. H., and Voesenek, L. A. C. J. (2006). Root aeration in rice (Oryza sativa): evaluation of oxygen, carbon dioxide, and ethylene as possible regulators of root acclimatizations. New Phytol. 170, 767–778. doi: 10.1111/j.1469-8137.2006.01725.x
Colmer, T. D., Gibberd, M. R., Wiengweera, A., and Tinh, T. K. (1998). The barrier to radial oxygen loss from roots of rice (Oryza sativa L.) is induced by growth in stagnant solution. J. Exp. Bot. 49, 1431–1436. doi: 10.1093/jxb/49.325.1431
Colmer, T. D., and Voesenek, L. A. C. J. (2009). Flooding tolerance: suites of plant traits in variable environments. Funct. Plant Biol. 36, 665–681. doi: 10.1071/FP09144
Colmer, T. D., and Greenway, H. (2011). Ion transport in seminal and adventitious roots of cereals during O2 deficiency. J. Exp. Bot. 62, 39–57. doi: 10.1093/jxb/erq271
Dolan, L., Janmaat, K., Willemsen, V., Linstead, P., Poethig, S., Roberts, K., et al. (1993). Cellular organization of the Arabidopsis thaliana root. Development 119, 71–84.
Drew, M. C. (1997). Oxygen deficiency and root metabolism: injury and acclimation under hypoxia and anoxia. Annu. Rev. Plant Physiol. Plant Mol. Biol. 48, 223–250. doi: 10.1146/annurev.arplant.48.1.223
Evans, D. E. (2003). Aerenchyma formation. New Phytol. 161, 35–49. doi: 10.1046/j.1469-8137.2003.00907.x
Gibbs, J., Turner, D. W., Armstrong, W., Darwent, M. J., and Greenway, H. (1998). Response to oxygen deficiency in primary maize roots. I. Development of oxygen deficiency in the stele reduces radial solute transport to the xylem. Aust. J. Plant Physiol. 25, 745–758.
Herzog, M., Striker, G. G., Colmer, T. D., and Pedersen, O. (2016). Mechanisms of waterlogging tolerance in wheat - a review of root and shoot physiology. Plant Cell Environ. 39, 1068–1086. doi: 10.1111/pce.12676
Justin, S. H. F. W., and Armstrong, W. (1987). The anatomical characteristics of roots and plant response to soil flooding. New Phytol. 106, 465–495. doi: 10.1111/j.1469-8137.1987.tb00153.x
Kamiya, N., Ito, J.-I., Morikami, A., Nagato, Y., and Matsuoka, M. (2003). The SCARECROW gene’s role in asymmetric cell divisions in rice plants. Plant J. 36, 45–54. doi: 10.1046/j.1365-313X.2003.01856.x
Kotula, L., Clode, P. L., Striker, G. G., Pedersen, O., Läuchli, A., Shabala, S., et al. (2015). Oxygen deficiency and salinity affect cell-specific ion concentrations in adventitious roots of barley (Hordeum vulgare). New Phytol. 208, 1114–1125. doi: 10.1111/nph.13535
Kotula, L., Ranathunge, K., Schreiber, L., and Steudle, E. (2009). Functional and chemical comparison of apoplastic barriers to radial oxygen loss in roots of rice (Oryza sativa L.) grown in aerated or deoxygenated solution. J. Exp. Bot. 60, 2155–2167. doi: 10.1093/jxb/erp089
Laurenzio, L. D., Wysocka-Diller, J., Malamy, J. E., Pysh, L., Helariutta, Y., Freshour, G., et al. (1996). The SCARECROW gene regulates an asymmetric cell division that is essential for generating the radial organization of the Arabidopsis root. Cell 86, 423–433. doi: 10.1016/S0092-8674(00)80115-4
Mano, Y., and Omori, F. (2013). Flooding tolerance in interspecific introgression lines containing chromosome segments from teosinte (Zea nicaraguensis) in maize (Zea mays subsp. mays). Ann. Bot. 112, 1125–1139. doi: 10.1093/aob/mct160
Mano, Y., Omori, F., Tamaki, H., Mitsuhashi, S., and Takahashi, W. (2016). DNA marker-assisted selection approach for developing flooding-tolerant maize. Jpn. Agric. Res. Q. 50, 175–182. doi: 10.6090/jarq.50.175
McDonald, M. P., Galwey, N. W., and Colmer, T. D. (2002). Similarity and diversity in adventitious root anatomy as related to root aeration among a range of wetland and dryland grass species. Plant Cell Environ. 25, 441–451. doi: 10.1046/j.0016-8025.2001.00817.x
Nissen, P. (1974). Uptake mechanisms: inorganic and organic. Annu. Rev. Plant Physiol. 25, 25–53. doi: 10.1146/annurev.pp.25.060174.000413
Petricka, J. J., Winter, C. M., and Benfey, P. N. (2012). Control of Arabidopsis root development. Annu. Rev. Plant Biol. 63, 563–590. doi: 10.1146/annurev-arplant-042811-105501
Pucciariello, C., Voesenek, L. A. C. J., Perata, P., and Sasidharan, R. (2014). Plant responses to flooding. Front. Plant Sci. 5:226. doi: 10.3389/fpls.2014.00226
Scheres, B., Benfey, P., and Dolan, L. (2002). Root development. Arabidopsis Book 1:e0101. doi: 10.1199/tab.0101
Shiono, K., Ogawa, S., Yamazaki, S., Isoda, H., Fujimura, T., Nakazono, M., et al. (2011). Contrasting dynamics of radial O2-loss barrier induction and aerenchyma formation in rice roots of two lengths. Ann. Bot. 107, 89–99. doi: 10.1093/aob/mcq221
Visser, E. J. W., Bögemann, G. M., Blom, C. W. P. M., and Voesenek, L. A. C. J. (1996). Ethylene accumulation in waterlogged Rumex plants promotes formation of adventitious roots. J. Exp. Bot. 47, 403–410. doi: 10.1093/jxb/47.3.403
Visser, E. J. W., Bögemann, G. M., Van de Steeg, H. M., Pierik, R., and Blom, C. W. P. M. (2000a). Flooding tolerance of Carex species in relation to field distribution and aerenchyma formation. New Phytol. 148, 93–103. doi: 10.1046/j.1469-8137.2000.00742.x
Visser, E. J. W., Colmer, T. D., Blom, C. W. P. M., and Voesenek, L. A. C. J. (2000b). Changes in growth, porosity, and radial oxygen loss from adventitious roots of selected mono- and dicotyledonous wetland species with contrasting types of aerenchyma. Plant Cell Environ. 23, 1237–1245. doi: 10.1046/j.1365-3040.2000.00628.x
Visser, E. J. W., Heijink, C. J., van Hout, K. J. G. M., and Voesenek, L. A. C. J. (1995). Regulatory role of auxin in adventitious root formation in two species of Rumex, differing in their sensitivity to waterlogging. Physiol. Plant. 93, 116–122. doi: 10.1034/j.1399-3054.1995.930117.x
Voesenek, L. A. C. J., and Bailey-Serres, J. (2015). Flood adaptive traits and processes: an overview. New Phytol. 206, 57–73. doi: 10.1111/nph.13209
Wahl, S., and Ryser, P. (2000). Root tissue structure is linked to ecological strategies of grasses. New Phytol. 148, 459–471. doi: 10.1046/j.1469-8137.2000.00775.x
Watanabe, K., Takahashi, H., Sato, S., Nishiuchi, S., Omori, F., Malik, A. I., et al. (2017). A major locus involved in the formation of the radial oxygen loss barrier in adventitious roots of teosinte Zea nicaraguensis is located on the short-arm of chromosome 3. Plant Cell Environ. 40, 304–316. doi: 10.1111/pce.12849
Watt, M., Magee, L. J., and McCully, M. E. (2008). Types, structure and potential for axial water flow in the deepest roots of field-grown cereals. New Phytol. 178, 135–146. doi: 10.1111/j.1469-8137.2007.02358.x
Wiengweera, A., Greenway, H., and Thomson, C. J. (1997). The use of agar nutrient solution to simulate lack of convection in waterlogged soils. Ann. Bot. 80, 115–123. doi: 10.1006/anbo.1996.0405
Yamauchi, T., Abe, F., Kawaguchi, K., Oyanagi, A., and Nakazono, M. (2014a). Adventitious roots of wheat seedlings that emerge in oxygen-deficient conditions have increased root diameters with highly developed lysigenous aerenchyma. Plant Signal. Behav. 9:e28506. doi: 10.4161/psb.28506
Yamauchi, T., Colmer, T. D., Pedersen, O., and Nakazono, M. (2018). Regulation of root traits for internal aeration and tolerance to soil waterlogging-flooding stress. Plant Physiol. 176, 1118–1130. doi: 10.1104/pp.17.01157
Yamauchi, T., Tanaka, A., Mori, H., Takamure, I., Kato, K., and Nakazono, M. (2016). Ethylene-dependent aerenchyma formation in adventitious roots is regulated differently in rice and maize. Plant Cell Environ. 39, 2145–2157. doi: 10.1111/pce.12766
Yamauchi, T., Watanabe, K., Fukazawa, A., Mori, H., Abe, F., Kawaguchi, K., et al. (2014b). Ethylene and reactive oxygen species are involved in root aerenchyma formation and adaptation of wheat seedlings to oxygen-deficient conditions. J. Exp. Bot. 65, 261–273. doi: 10.1093/jxb/ert371
Yamauchi, T., Yoshioka, M., Fukazawa, A., Mori, H., Nishizawa, N. K., Tsutsumi, N., et al. (2017). An NADPH oxidase RBOH functions in rice roots during lysigenous aerenchyma formation under oxygen-deficient conditions. Plant Cell 29, 775–790. doi: 10.1105/tpc.16.00976
Keywords: aerenchyma, cereal crops, cortex to stele ratio, intercellular spaces, longitudinal oxygen transport, radial oxygen loss, waterlogging
Citation: Yamauchi T, Abe F, Tsutsumi N and Nakazono M (2019) Root Cortex Provides a Venue for Gas-Space Formation and Is Essential for Plant Adaptation to Waterlogging. Front. Plant Sci. 10:259. doi: 10.3389/fpls.2019.00259
Edited by:
Francesco Licausi, University of Pisa, ItalyReviewed by:
Gustavo Gabriel Striker, CONICET Instituto de Investigaciones Fisiológicas y Ecologicas Vinculadas a la Agricultura (IFEVA), ArgentinaMaría Laura Vidoz, Instituto de Botánica del Nordeste (IBONE-CONICET), Argentina
Copyright © 2019 Yamauchi, Abe, Tsutsumi and Nakazono. This is an open-access article distributed under the terms of the Creative Commons Attribution License (CC BY). The use, distribution or reproduction in other forums is permitted, provided the original author(s) and the copyright owner(s) are credited and that the original publication in this journal is cited, in accordance with accepted academic practice. No use, distribution or reproduction is permitted which does not comply with these terms.
*Correspondence: Takaki Yamauchi, YXRreWFtYUBtYWlsLmVjYy51LXRva3lvLmFjLmpw