- 1Dipartimento di Scienze ed Innovazione Tecnologica, Università del Piemonte Orientale, Vercelli, Italy
- 2Agroécologie, AgroSup Dijon, CNRS, INRA, Univ. Bourgogne Franche-Comté, Dijon, France
- 3Dipartimento di Scienze ed Innovazione Tecnologica, Università del Piemonte Orientale, Alessandria, Italy
Arbuscular mycorrhizal fungi (AMF) colonize the roots of most terrestrial plant species, improving plant growth, nutrient uptake and biotic/abiotic stress resistance and tolerance. Similarly, plant growth promoting bacteria (PGPB) enhance plant fitness and production. In this study, three different AMF (Funneliformis mosseae, Septoglomus viscosum, and Rhizophagus irregularis) were used in combination with three different strains of Pseudomonas sp. (19Fv1t, 5Vm1K and Pf4) to inoculate plantlets of Fragaria × ananassa var. Eliana F1. The effects of the different fungus/bacterium combinations were assessed on plant growth parameters, fruit production and quality, including health-promoting compounds. Inoculated and uninoculated plants were maintained in a greenhouse for 4 months and irrigated with a nutrient solution at two different phosphate levels. The number of flowers and fruits were recorded weekly. At harvest, fresh and dry weights of roots and shoots, mycorrhizal colonization and concentration of leaf photosynthetic pigments were measured in each plant. The following fruit parameters were recorded: pH, titratable acids, concentration of organic acids, soluble sugars, ascorbic acids, and anthocyanidins; volatile and elemental composition were also evaluated. Data were statistically analyzed by ANOVA and PCA/PCA-DA. Mycorrhizal colonization was higher in plants inoculated with R. irregularis, followed by F. mosseae and S. viscosum. In general, AMF mostly affected the parameters associated with the vegetative portion of the plant, while PGPB were especially relevant for fruit yield and quality. The plant physiological status was differentially affected by inoculations, resulting in enhanced root and shoot biomass. Inoculation with Pf4 bacterial strain increased flower and fruit production per plant and malic acid content in fruits, while decreased the pH value, regardless of the used fungus. Inoculations affected fruit nutritional quality, increasing sugar and anthocyanin concentrations, and modulated pH, malic acid, volatile compounds and elements. In the present study, we show for the first time that strawberry fruit concentration of some elements and/or volatiles can be affected by the presence of specific beneficial soil microorganisms. In addition, our results indicated that it is possible to select the best plant-microorganism combination for field applications, and improving fruit production and quality, also in terms of health promoting properties.
Introduction
Plants interact with a huge variety of beneficial microorganisms such as arbuscular mycorrhizal fungi (AMF) and plant growth-promoting bacteria (PGPB), which can improve both plant fitness and production.
AMF belong to the Glomeromycotina subphylum (Spatafora et al., 2016) and are symbiotically associated to the roots of the majority of land plants, including the main crop species. They play an ecologically important role and provide various ecosystem services such as the improvement of nutrient uptake, soil aggregation, and protection against biotic and abiotic stress (Lingua et al., 2008; Gianinazzi et al., 2010; Smith et al., 2010; Antunes et al., 2012; Boyer et al., 2015).
PGPB comprise different functional and taxonomic groups (Ghosh et al., 2003), among which Pseudomonas fluorescens is one of the most extensively studied species (Duijff et al., 1997; Vazquez et al., 2000). They directly enhance plant growth by a variety of mechanisms, such as mobilization of soil nutrients, atmospheric nitrogen fixation, phosphorus solubilization, and phytohormone synthesis, especially IAA (Indole-3-Acetic Acid) (Glick, 1995). PGPB can also act indirectly, suppressing phytopathogens (Benizri et al., 2001). Some fluorescent pseudomonads were shown to improve mycorrhizal root colonization (Gamalero et al., 2008, 2010), extraradical hyphal growth (Mugnier and Mosse, 1987) and AMF spore germination (Frey-Klett et al., 2007), functioning as mycorrhiza helper bacteria (MHB). Conversely, AMF can influence the chemical composition of root exudates, which are a major nutrient source for the bacteria in the rhizosphere (Hegde et al., 1999; Artursson et al., 2006).
The strawberry, Fragaria × ananassa Duch., belonging to the family Rosaceae, is one of the most cultivated berry crops in Europe and all around the world (Akhatou and Fernandez-Recamales, 2014a; Prat et al., 2014). The large size and the deep red color of its false fruit, besides its characteristic aroma (Ulrich et al., 2007), are the results of the crossing between the North American strawberry Fragaria virginiana Mill. with the Chilean strawberry Fragaria chiloensis (L.) Mill. (Darrow and Wallace, 1966). The consumption of this fruit, fresh, frozen, or processed (juices, jams, yogurts, etc.), in the daily diet is an important source of healthy compounds (Tulipani et al., 2009) such as fibers, vitamins (in particular C and B9), minerals (mainly potassium and magnesium), and antioxidants (flavonoids, phenolic acids, and ellagitannins) that can prevent or reduce certain types of cancer, cardiovascular diseases, obesity, type II diabetes and cellular damage induced by reactive oxygen species (ROS) (Olsson et al., 2004; Wang and Lewers, 2007; He and Giusti, 2010; Giampieri et al., 2012). Therefore, the strawberry nutritional values and beneficial properties of its bioactive components (a heterogeneous group of biologically active non-nutrients, mainly represented by polyphenols), together with a complex blend of volatile organic compounds, make it a highly appreciated fruit (Diamanti et al., 2012). Among polyphenols, flavonoids, especially anthocyanins in the form of pelargonidin and cyanidin derivatives, are responsible for the strawberry bright red color. These two characteristics are markers of ripening, a process in which anthocyanin accumulation in the fruit flesh and/or skin is highest (da Silva Pinto et al., 2008; Giampieri et al., 2012; Jaakola, 2013). Moreover, the key role of these molecules, both in fruit tolerance to the environmental stresses and in ameliorating post-harvest quality and shelf life, is well-known (Xu et al., 2014). The biosynthesis and accumulation of anthocyanins in fruits are regulated by different factors: genetic (e.g., MYB genes), environmental (light exposure, temperature, nutrient availability such as nitrogen and calcium) and developmental (sugars, in particular sucrose, and hormones including abscisic acid, jasmonate, and ethylene) (Carbone et al., 2009; Jaakola, 2013).
Texture (firmness, juiciness, and crispness), color, sweetness and aroma are the most important quality indicators for strawberry consumers (Christensen, 1983; Colquhon et al., 2012; Negri et al., 2015). Sugars and organic acids are the principal soluble component in ripe fruit (Perez et al., 1992; Moing et al., 2001). The ratio between sugars and acids strongly affects fruit aroma and flavor (Montero et al., 1996; Kallio et al., 2000). For this reason it is considered an index of fruit quality and its variations depend on plant genotype, ripening stage, climatic factors, and soil type (Kallio et al., 2000; Akhatou and Fernandez-Recamales, 2014b; Valentinuzzi et al., 2014). Fructose, glucose, and sucrose, the most abundant soluble solids in strawberries, are responsible for fruit sweetness (Perez et al., 1997). Sugars are involved in many biological processes: in particular, sucrose is involved in fruit ripeness (Jia et al., 2011) and anthocyanin synthesis (Gollop et al., 2002), while fructose is a precursor of the volatile compounds furanones (Sanz et al., 1997; Forney et al., 2000), that contribute to the caramel aroma of the fruit (Prat et al., 2014). Organic acids are important for the maintenance of nutritional values and fruit quality (Mikulic-Petkovsek et al., 2012), besides their involvement in the gelling process of pectin (Cordenunsi et al., 2002). The most abundant organic acid in strawberry is citric acid, whose concentration is responsible for about 92% of total acidity (Cordenunsi et al., 2002; Mahmood et al., 2012), while malic, tartaric, shikimic, quinic, and fumaric acids are present in very small amounts.
Strawberry aroma is mainly due to the complex blend of volatile organic compounds (known as volatilome—Bicchi and Maffei, 2012; Ulrich et al., 2018), which also contribute to the fruit flavor (Forney et al., 2000; Forney, 2001; Prat et al., 2014; Schwieterman et al., 2014). Although these molecules have a big impact on fruit sensorial qualities, they are found in very low concentrations (ranging between about 0.001 and 0.01% of fruit fresh weight) (Buttery, 1981). Approximately 360 volatile molecules seem to be involved in odor and aroma perception of F. × ananassa fruits by humans, with esters, aldehydes, ketones, alcohols, furanones, and terpenoids being the most representative (Larsen et al., 1992; Prat et al., 2014). The volatile profile changes according to cultivars, stage of ripeness and environmental conditions (Forney et al., 2000). Also soil microorganisms, such as AMF and PGPB (that are considered either as “biofertilizers”—Vessey, 2003; Rai, 2006—or “bioprotectors”—Borowicz, 2001; Antunes et al., 2012) can modulate the volatile profile in various plant species (Bona et al., 2016).
While the effects of bacterial and fungal inocula on the physiology and biochemistry of the whole plant have been investigated in several studies (reviewed in Vessey, 2003; Saharan and Nehra, 2011; Zeng et al., 2013; Sbrana et al., 2014 and references therein), only in recent years the attention has been focused both on sensorial qualities and nutritional/nutraceutical values (mainly healthy compounds) of the edible portions of various crop plants (Nautiyal et al., 2008; Giovannetti et al., 2013; Berta et al., 2014; Bona et al., 2015; Hart et al., 2015). Although several studies (about 70–80) describing strawberry aroma, flavor, sweetness and production of health-promoting compounds in uninoculated plants have been published before (Olsson et al., 2004; Tulipani et al., 2008; Akhatou and Fernandez-Recamales, 2014a,b; Prat et al., 2014; Valentinuzzi et al., 2014; Negri et al., 2015), those related to inoculated ones are fewer (Castellanos-Morales et al., 2010; Lingua et al., 2013; Palencia et al., 2013; Pešaković et al., 2013; Bona et al., 2015; Boyer et al., 2015) and no one concerned the volatile profile. In strawberry, it has been shown that AM colonization stimulates plant growth (Hršelová et al., 1990), enhances photosynthesis (Borkowska, 2002), induces early flowering and fruit production (Lu and Koide, 1994; Gaur et al., 2000; Poulton et al., 2002; Sohn et al., 2003), increases the concentration of sugar and anthocyanins (Castellanos-Morales et al., 2010), thereby resulting in fruits of higher quality. On the contrary, only few studies have been performed to investigate the effect of PGPB on strawberry (Lingua et al., 2013; Bona et al., 2015). A synergistic effect on strawberry growth following co-inoculation with AMF and Pseudomonas putida and a stimulation of AMF root colonization by Agrobacterium radiobacter have previously been reported (Vosatka et al., 1992, 2000). Therefore, in this work we wanted to test the effects of three AMF and three PGPB strains on F. × ananassa (var. Eliana F1) plants grown in a greenhouse at low P level. Both plant (mycorrhizal colonization, root and shoot dry weight, number of flowers, chlorophyll concentration, fruit number, weight, and size) and fruit (pH, total acidity; organic acid, sugar, and anthocyanidin concentration; volatile profile and element composition) parameters were considered in order to establish which fungus/bacterium combination gave the best results especially in terms of fruit quality and production. Moreover, by using a multivariate statistical approach (Principal Component Analysis-Discriminant Analysis, PCA-DA) it was possible to determine which bacterium or fungus induced changes on each parameter. To our knowledge the present study shows, for the first time, how different soil beneficial microorganisms (AMF and PGPB) can affect the volatile profile and chemical element composition in strawberry fruits.
Materials and Methods
Plant Material and Growth Conditions
Seedlings of Fragaria × ananassa Duch var. Elyana F1 with continuous flowering habit, were transplanted in plastic pots (400 mL) in a soil (Brill Ortopack, Agrochimica, Bolzano, Italy; pH 5.5–6.5) previously sterilized by flowing steam (101°C for 1 h), and kept in a greenhouse for rooting. After 1 month, they were transplanted in new plastic pots (900 mL) in a 2/1 v/v mixture of sterile soil (the same used in the first transplant) and sand (sterilized in oven at 180°C for 3 h), and inoculated or not with one of three different AMF in combination with one of three different bacterial strains (Table 1). Plants were initially irrigated 3 times per week. When the plants began to produce fruits, they were transplanted again in plastic pots of 3 L capacity. Starting from 1 week after the last transplant, they were irrigated daily: once per week with a Long Ashton (LA) nutrient solution (Trotta et al., 1996), and with tap water on the other days. In particular, half of the control plants (C) were watered with LA 32 μM phosphate, while the remaining controls (C-P) and all the inoculated plants were fed with LA 16 μM phosphate (Table 1) until harvest. Strawberry plants were maintained in greenhouse for 16 weeks.
Fungal Inocula and Mycorrhizal Colonization
The arbuscular mycorrhizal fungus Rhizophagus irregularis (Ri) (DAOM197-198) was provided by INRA (Recorbet and Bernaud, Dijon). Funneliformis mosseae (Fm) (BEG12) was provided by the European Bank of Glomales (Dijon). Septoglomus viscosum (Sv), collected from an Italian soil (Tuscany, Italy), was produced and provided by Mybasol S.r.l. (Alessandria, Italy). The three inocula, prepared as a mixture of soil, mycorrhizal roots, hyphae, and spores, were mixed (11% v/v) with the plant growth medium.
At harvest, mycorrhizal colonization was assessed on 60 root pieces (1 cm long) randomly chosen from each plant. Samples were cleared in 10% KOH for 30 min at 60°C, stained with 1% methyl blue in lactic acid and mounted on a slide. Frequency of mycorrhization (F%), percentage of colonized root tissue (M%), and abundance of arbuscules (A%) and vesicles (V%) were calculated according to Trouvelot et al. (1986).
Bacterial Inocula
Pseudomonas fluorescens strain Pf4 (Pf4) was isolated from a forest soil located in Sassello (Savona, Italy) and characterized by Berta et al. (2014). Pseudomonas sp. 5Vm1K (5Vm) was isolated from the rhizosphere of blueberry plants grown in a larch woodland (Bellino, Cuneo, Italy) and characterized as described by Bona et al. (2015). P. fluorescens strain 19Fv1t (19Fv) was provided by Mybasol s.r.l (Alessandria, Italy) and characterized by Bona et al. (2017). Bacterial 16S rDNA sequences were deposited in the NCBI database GenBank with the accession numbers KF234076, KF233995, KF752592 for Pf4, 5Vm, and 19Fv, respectively.
Bacterial cells were grown on tryptic soy agar (TSA) at 28°C for 48 h and suspended in 0.1M MgSO4. Bacterial density (600 nm) was adjusted to 109 CFU mL−1. Each plant was inoculated with 8 mL of bacterial suspension, except uninoculated ones that were irrigated with the same quantity of MgSO4. After 1 week the plants were inoculated again.
Plant Parameters
The number of flowers and fruits was recorded weekly during all the experiment. Mature fruits were harvested, weighed, measured (large and small diameters), frozen in liquid nitrogen, and stored at −80°C until biochemical/chemical analyses.
For each plant, fresh weights (FW) of root and shoot (without flowers and fruits) were recorded at harvest. Then, each shoot and root system was dried in oven at 60°C for three days and dry weight was (DW) recorded.
Leaf chlorophyll and total carotenoid concentrations were determined spectrophotometrically. Fresh leaf samples (0.03 g from each plant) were kept in the dark at 4°C for 3 days in N, N-dimethyl-formamide (1.5 mL) for pigment extraction. Samples were analyzed according to Porra et al. (1989) using a 0.1–0.5 nm resolution range spectrophotometer.
Fruit Biochemical Analyses
In order to investigate the effect of PGPB and AMF combinations on strawberry quality, we randomly chose five plants per treatment (55 plants in total) for the assessment of pH, titratable acids, content of organic acids (malic, quinic, citric, and fumaric), concentration of sugars (sucrose, glucose, and fructose), ascorbic acid (vitamin C), and anthocyanidins (pelargonidin and cyanidin).
pH and total acidity of strawberries were determined diluting the fruit homogenate (5 g from each plant) in deionized, degassed water (30 mL). Samples were centrifuged at 10,000 rpm at room temperature (RT) for 20 min. The supernatant was used for the determination of pH. The same samples were well mixed and 0.1 M NaOH was added dropwise until reaching the titration end-point (pH 8.1) according to official method published by the Italian Government in DM 03.02.1989. Results were expressed as percentage of citric acid per fresh weight unit.
Organic acid concentration was determined by HPLC (Dionex, Sunnyvale, CA, USA; injection volume 20 μL, column Aphera C18 polymer 25 cm × 4.6 mm, 5 μm, column temperature 30°C, flow rate 0.4 mL min−1) at 210 nm, according to Keutgen and Pawelzik (2007), with some modifications as reported in Bona et al. (2015).
Ascorbic acid concentration was determined by diluting fruit homogenate (5 g) with 50 mL of deionized water. The solution was shaken at 200 rpm for 10 min at RT, filtered with filter paper and decolorized twice with PolyVinylPyrrolidone (PVP-0.1 g per 10 mL). The pH of the samples ranged between 3.5 and 4.0 in agreement with the instructions of the enzymatic analytical kit (Cat. Nr. 10409677035, R-Biopharm, Roche, Germany). The vitamin C content was measured at 578 nm.
Sucrose, D-glucose and D-fructose concentrations were determined spectrophotometrically at 340 nm using a standard enzymatic analytical kit (Cat. Nr. 10716260035, R-Biopharm, Roche, Germany). Fruit homogenates (5 g) were diluted to 50 mL with deionized water, shaken at 200 rpm for 1 h, filtered through filter paper and decolored twice with PVP. The colorless serum (1 mL) was diluted in deionized water (1/2 v/v) and used for the analyses.
Anthocyanins were extracted from strawberry homogenate (10 g) and separated isocratically by HPLC according to Comandini et al. (2008) with some modifications as reported in Bona et al. (2015). Commercial standards of pelargonidin chloride and cyanidin chloride (Sigma-Aldrich, St. Louis, MO, USA) were used to quantify the content of each anthocyanin according to the equation reported in Chandra et al. (2001); moreover, their identities were also confirmed by MALDI-TOF analysis Voyager DE-PRO (AB Sciex, Framingham, United States).
Elements Extraction and Determination
An inductively coupled plasma with mass spectrometry (ICP-MS) X Series II system (Thermo Electron Corporation, Waltham, USA) and an inductively coupled plasma with optical emission (ICP-OES, Spectro Genesis, Kleve, Germany) were used.
Fruit homogenate (1.0 g) was put in an Erlenmeyer flask with nitric acid (4.0 mL, ≥69.0% Trace Select, Fluka) and hydrogen peroxide (2.4 mL, ≥30% Trace Select, Fluka) and subjected to wet digestion for 3 h at 320°C. After cooling, the sample was diluted to 50.0 mL with water and nitric acid before ICP-MS or ICP-OES analysis.
Ca, K, Mg, Na, P, and S were determined by ICP-OES, while Ag, Al, As, Ba, Be, Bi, Cd, Ce, Co, Cr, Cs, Cu, Dy, Er, Eu, Fe, Gd, Hf, Hg, Ho, Ir, La, Li, Lu, Mn, Nd, Ni, Pb, Pd, Pm, Pt, Rb, Ru, Sb, Sc, Se, Sm, Sn, Sr, Ta, Tb, Th, Tl, U, V, Y, Yb, and Zn were determined by ICP-MS.
Volatile Profile
Volatiles were analyzed by head space solid phase microextraction method coupled to gas chromatography-mass spectrometry (HS SPME GC-MS). The GC-MS system (Varian, Walnut Creek, USA) was equipped with HP-INNOWAX capillary column (30 m × 0.25 mm, 0.25 μm, Varian). The extraction was performed using an SPME fiber Divinylbenzene/Carboxen/Polydimethylsiloxane 50/30 μm coating (Supelco, Pennsylvania, USA) after conditioning.
One gram of homogenized sample was added with 1.0 mL sodium chloride 0.10 M, vortexed for 2 min and centrifuged for 10 min at 10,000 rpm at 4°C. The supernatant (1.0 mL) was put in a 20 mL vial sealed by a PTFE/silicone septum; benzaldehyde at 100 mg L−1 (Sigma Aldrich, MO, USA) was added as internal standard. Equilibration was performed for 5 min at 33°C, while extraction for 30 min. The fiber was left in the injection part of the GC-MS at 250°C for 20 min. Before sampling, the fiber was reconditioned and blank runs were done periodically to reveal possible carryover effects. Helium was used as carrier gas at 1.0 mL min−1; the oven temperature was held isothermal at 35°C for 3 min, raised to 60°C (2°C min−1), then from 60 to 130°C (10°C min−1), maintained at 130°C for 8 min, raised from 130 to 250°C (20°C min−1) and held isothermal for 5 min. MS detection was performed in full scan acquisition from 35 to 350 m/z with electron ionization at 70 eV.
Statistical Analysis, PCA and PCA-DA Analysis
Data were analyzed by two-way ANOVA (R software package) using “Fungus” and “Bacterium” as factors. A one-way ANOVA followed by Fisher test (StatView 4.5, Abacus) with significance cut-off at P < 0.05 was used to assess differences among the treatments.
Data were also treated by multivariate statistical tools as Principal Component Analysis (PCA) (Massart et al., 1998; Negri et al., 2011) and PCA-Discriminant Analysis (PCA-DA).
In PCA-DA (Hoogerbrugge et al., 1983) the space defined by the Principal Components (PCs) is used to identify linear discriminant functions (LDFs) able to separate the samples in the classes present. In two class problems, only one LDF is provided, able to discriminate the samples in the two classes present.
Results
Mycorrhizal Colonization
Only rare traces of mycorrhizal colonization (M% always < 0.1) were detected in C-P uninoculated plants (Figure 1B). The three AMF species differently colonized the strawberry root system (Figures 1A–D). S. viscosum poorly colonized strawberry roots, producing extremely rare arbuscules and no vesicles. F. mosseae colonization was slightly higher (4 < M% < 9) and arbuscules, but not vesicles, were observed in all treatments. R. irregularis was the best colonizer, showing a significant increase (three-to four-fold) of M% (between 15 and 25%) in respect to Fm-plants; arbuscules and vesicles were always detected. The two-way ANOVA (Figures 1A–D, insets) indicated that co-inoculation with PGPB did not affect AMF colonization, with the exception of vesicle percentage, that was influenced by the fungus-bacterium interaction.
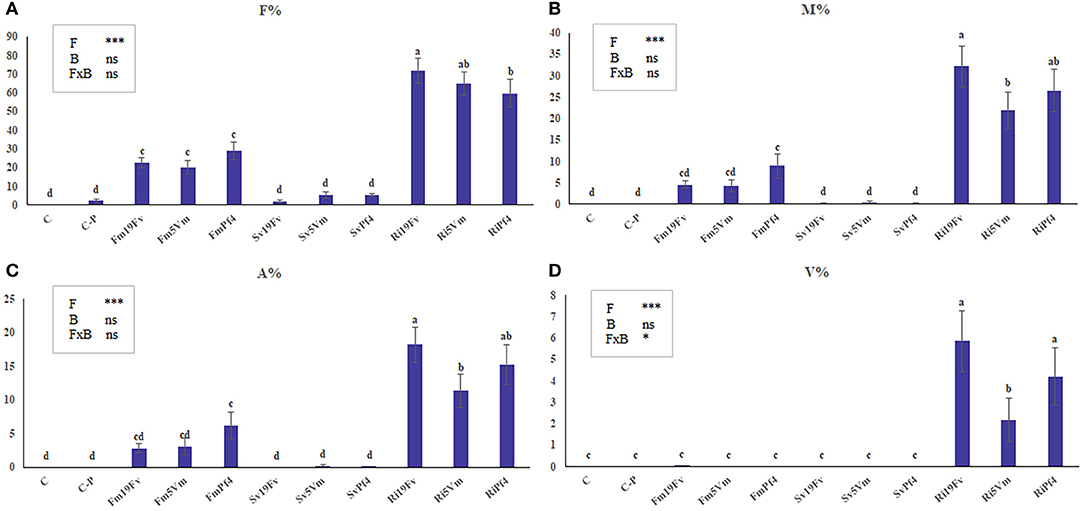
Figure 1. Mycorrhizal colonization in strawberry root. Mycorrhizal parameters of F. × ananassa roots inoculated with three AMF [F. mosseae (Fm), S. viscosum (Sv) or R. irregularis (Ri)] in combination with three strains of Pseudomonas sp. (19Fv1t, 5Vm1k, and Pf4). F%, frequency of colonization (A); M%: intensity of the mycorrhizal colonization (B); arbuscular (A%; C) and vesicles (V%; D) abundance in the mycorrhizal root. Data (n = 10) were analyzed by one-way ANOVA with Fisher test, p < 0.05. Bars represent the standard error and different letters indicate significant differences between the various treatments. Moreover a two-way ANOVA is presented in the inset of each graph, considering the two factors fungus (F), bacterium (B) and their interaction (FxB): ns not significant; *p < 0.05; **p < 0.01; ***p < 0.0001.
Effects of AMF and PGPB Inoculation on Plant Growth and Production
Plant growth parameters were affected by the combination of low phosphate input and co-inoculation (Table 2).
The cumulative number of flowers and fruits (Figures 2A,B) was recorded weekly. In SvPf4 plants, inoculation led to a significant induction of flowering (approximately +35%), if compared to the other treatments, especially C-P and Ri5Vm ones, that showed the lowest flower production (Figure 2A). These results were also confirmed by the average number of flowers produced per plant (Table 2). The two-way ANOVA revealed that flowering was affected by all the considered factors (F, B, and F × B). The low input fertilization used in this work (C-P) did not affect flowering and fruit yield compared to control condition (C), while positive effects induced by the inoculated microorganisms were observed (Figures 2A,B). In particular, the strain Pf4 was the most efficient in cooperating with all the used AMF; indeed, an important impact on fruit number (at least +22%) and fruit weight per plant (between +14 and +20%) was observed in all Pf4-plants compared to C-P plants. On the contrary, a significant reduction of total fruit fresh weight was recorded in Ri5Vm and Fm19Fv plants (approximately −17%). Different results were observed for the average fruit fresh weight per plant and for fruit size (large and small diameters) (Table 2): plants inoculated with P. fluorescens strain 19Fv (Ri19Fv and Sv19Fv) produced on average larger and heavier fruits, but fewer in number (except Ri19Fv) if compared to the Pf4-plants. In agreement with these observations, the two-way ANOVA pointed out the influence of the factor “Bacterium” on fruit size and weight.
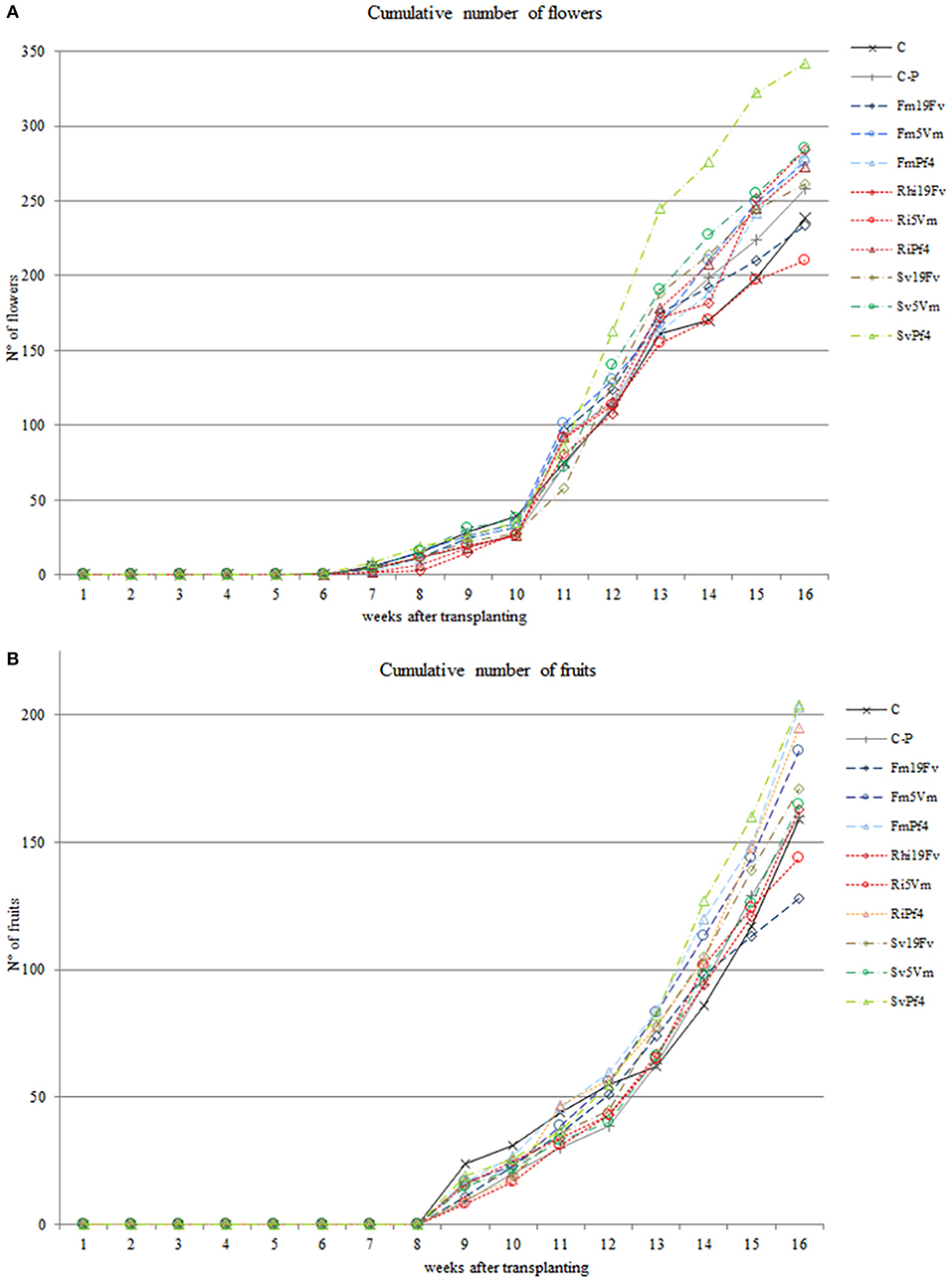
Figure 2. Cumulative number of flowers and fruits. Number of flowers (A) and fruits (B) produced by strawberry plants inoculated or not with three AMF [F. mosseae (Fm), S. viscosum (Sv) or R. irregularis (Ri)] in combination with three strains of Pseudomonas sp. (19Fv1t, 5Vm1k, and Pf4). Flowers were produced for 11 weeks (starting 6 weeks after inoculations), fruits for 8 weeks (starting 9 weeks after inoculations). Data (n = 10) were analyzed by one-way ANOVA with Fisher's post-hoc test, p < 0.05.
In general, inoculation with S. viscosum increased root DW and root/shoot ratio with the highest values in Sv19Fv plants, that showed significant differences either with plants inoculated with the two other fungi (Fm and Ri) or with uninoculated ones (C and C-P). Similar results were observed for shoot DW, but in this case, SvPf4 plants showed the highest biomass with no significant differences to the controls. The lowest values of root and shoot DW and root/shoot ratio were found in Fm19Fv and Fm5Vm plants, followed by Ri5Vm ones. The two-way ANOVA (Table 2) confirmed that biomass production was affected by both mycorrhizal (F factor) and bacterial (B factor) inoculation. Moreover, concerning root DW and root/shoot ratio, significant differences between the various treatments were attributable to co-inoculation (F × B).
Chlorophyll a and b (Table 2), as well as carotenoid (Table S1) concentrations in leaves were determined. Only the factor fungus (F) strongly improved chlorophyll production. Fm-plants showed the highest values regardless of the used bacterium, with significant differences to Sv- and control (C and C-P) plants, especially for chlorophyll b content. The lowest values of photosynthetic pigment content were observed in C plants. No significant differences between the various treatments were recorded for carotenoid concentration (Table S1).
Effects of AMF and PGPB Inoculation on Fruit Quality
The pH measured in strawberry fruits ranged between 3.34 (Sv5Vm) and 3.54 (Ri19Fv) (Table 3). Inoculation with S. viscosum induced a decrease of pH values if compared to the other treatments. The same trend was observed for titratable acidity (expressed as the percentage of citric acid per fresh weight unit—Table 3). The two-way ANOVA suggested that these two parameters were significantly affected both by mycorrhizal (F) and even more by bacterial (B) inoculation (Table 3), but not by the F × B interaction.
Since organic acids are major components involved in fruit taste, their concentrations were monitored. No differences were detected for quinic, citric, fumaric and ascorbic (vitamin C) acid (Table S1), while the concentration of malic acid significantly changed in the various treatments (Table 3), with the highest values in Sv19Fv-plants. Ri5Vm plants presented the lowest malic acid concentration. The two factors F and B, but not F × B, were responsible for these variations (Table 3).
The concentration of glucose, fructose, and sucrose, the main sugars involved in strawberry taste and flavor, was investigated. Plants fed with a reduced nutrient supply (C-P) produced fruits containing less sucrose, glucose, and fructose than controls (C) (Table 3). No significant differences between the treatments were observed both in the glucose and total sugar concentrations (Table 3 and Table S1), while those of sucrose and fructose changed depending on the various fungus-bacterium combinations.
In general, inoculation with 19Fv bacterial strain, irrespective of fungal inoculation, induced a decrease in sucrose content. Fruits of FmPf4 plants contained the highest level of sucrose. Regarding fructose concentration, C plants showed the highest values followed by plants inoculated with F. mosseae and S. viscosum in the presence of 19Fv or Pf4 bacterial strains. Two-way ANOVA indicated that the only relevant factor was bacterial inoculation (B).
The chromatogram obtained by the HPLC analysis (Figure S1A) of strawberry anthocyanidins showed six peaks, identified, also by MS analysis (Figure S1B), as cyanidin 3-glucoside (peak 1), pelargonidin 3-glucoside (peak 2), pelargonidin 3-rutinoside (peak 3), cyanidin malonyl glucoside (peak 4), pelargonidin malonyl glucoside (peak 5), and pelargonidin acetyl glucoside (peak 6), respectively. The concentration of pelargonidin 3-glucoside significantly varied between the different treatments (Figure 3), displaying the highest values in fruits of Sv5Vm plants that showed marked differences in particular with FmPf4, SvPf4, Ri5Vm, and C-P treatments.
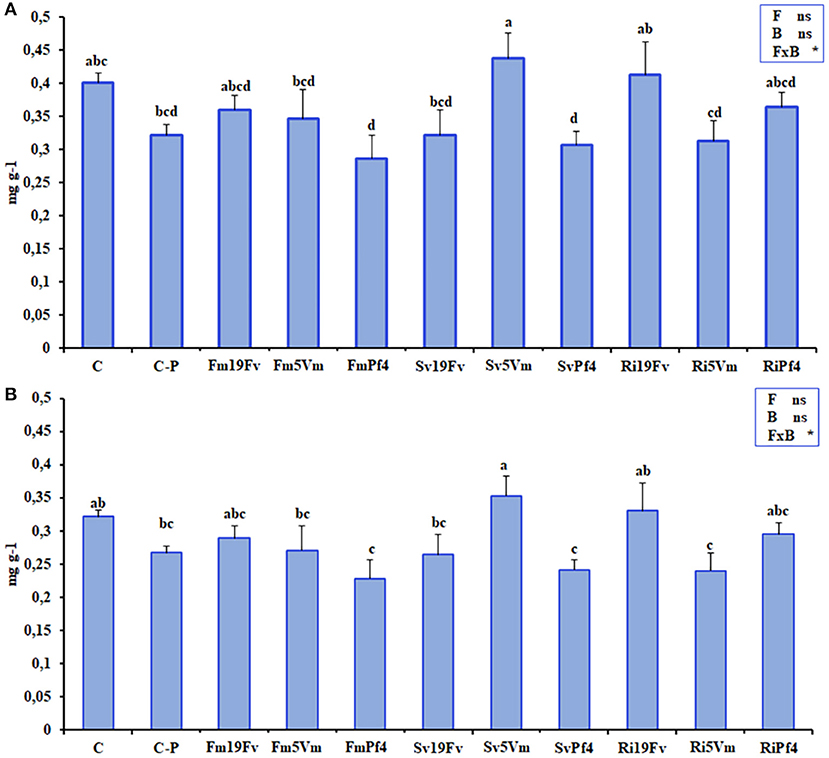
Figure 3. Anthocyanidins. Total anthocyanidin (A) and pelargonidin 3-glucoside (B) concentrations in fruits of strawberry plants inoculated with three AMF [F. mosseae (Fm), S. viscosum (Sv) or R. irregularis (Ri)] in combination with three strains of Pseudomonas sp. (19Fv1t, 5Vm1k, and Pf4). Data (n = 5) were analyzed by one-way ANOVA with Fisher test, p < 0.05. Bars represent the standard error and different letters represent significant differences between the various treatments. Table with two-way ANOVA results were reported in the right part of the graphs. The analysis was performed using Fungus (F), Bacteria (B), or the F×B combination as factors: *represent significant results while “ns” not statistically significant differences.
Since pelargonidin 3-glucoside was the most abundant anthocyanidin in strawberry samples, it was also responsible for the total anthocyanidin variation (Figures 3A,B), so the data of these two parameters showed the same pattern and were both affected by the co-inoculation as indicated by two-way ANOVA analysis. The concentrations related to all the other analyzed anthocyanidins did not show any significant differences between the various treatments (Table S1).
Soil Microorganisms Influenced Element Concentration in Fruits
A total of 51 elements (mainly belonging to transitional elements, rare earth, alkali and alkali earth metals—Table 4 and Figure 4A) were detected in fruit homogenates. No significant differences due to the inoculation were detected for the concentration of the main nutrients, such as P (Table 3) Na, K, Fe, Mg, Mn, Cu, and Zn (data not shown). On the contrary, P levels significantly changed either in shoots or roots, according also to the mycorrhizal colonization (and therefore according to the fungal species—Table 3 and Table S2): Ri-plants presented an increase of phosphate in both organs, while a decrease was observed in SvPf4 plants that did not show any significant difference with C-P ones.
Concerning the other elements, only Co, Rb, Sr, Cs, and Ba varied significantly in the different treatments (Table 5) and these changes were mainly due to the fungus presence. Co and Ba concentrations were highest in fruits of strawberry plants inoculated with F. mosseae independently of the combination with the different bacteria. S. viscosum affected the concentration of Sr, Rb, and Cs (Table 5). The concentrations of the last two were also affected by the inoculation with Pf4 (Figure 5, Table 4). In particular, fruits of all Sv-plants showed significantly higher values of Rb, if compared to the other treatments. On the contrary, the lowest Rb concentration was observed in Fm5Vm plants (Table 5). Similar results were obtained from Sr and Cs analyses, although for Cs concentration no significant differences were recorded between Sv and control plants (Table 5).
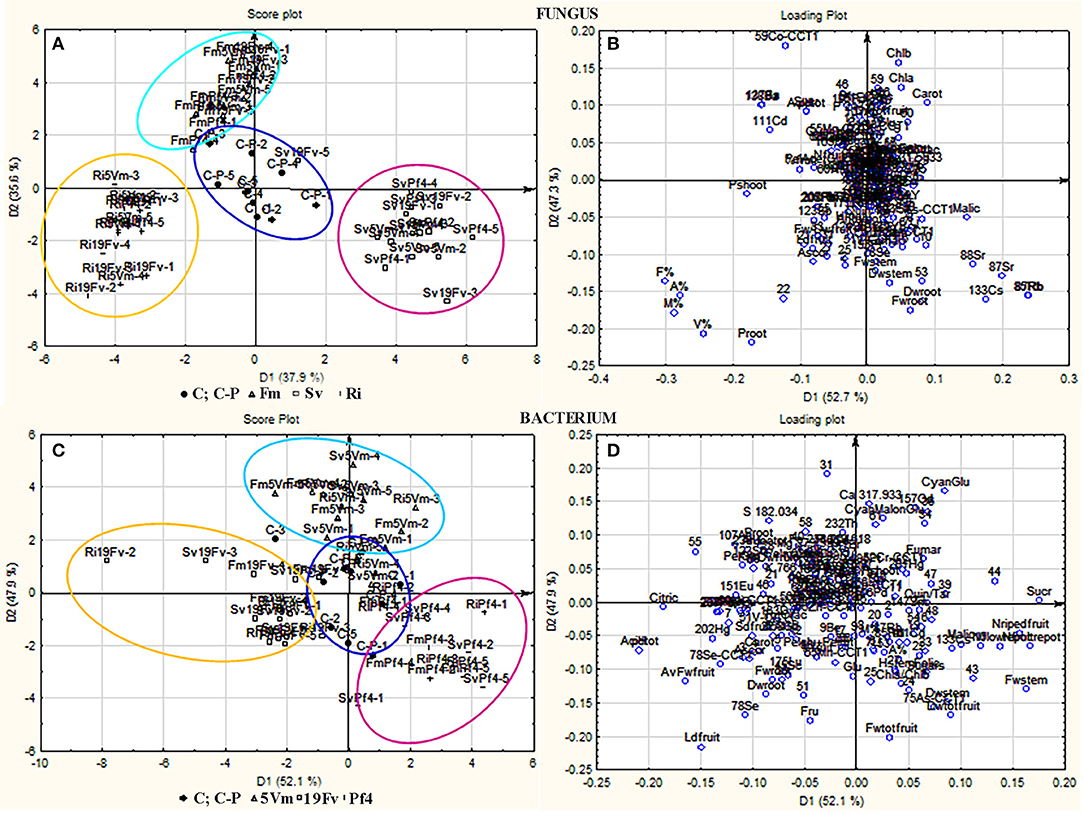
Figure 5. PCA-DA analysis. Score plots (A,C) and loading plots (B,D) from PCA-DA of volatile compounds, elements and all other parameters recorded in strawberry plants grown or not in presence of different AMF/PGPB mixed inocula. (A,B) Samples were separated on the base of the different fungal treatments: F. mosseae (F1, marked with Δ and grouped in a light blue circle), S. viscosum (F2, marked with □ and grouped in a fuxia circle), R. irregularis (F3, marked with + and grouped in a yellow circle); controls (C and C-P) were marked with • and grouped in a blue circle. (C,D) Samples were separated on the base of the different bacterial treatments: Pseudomonas sp. 5Vm (B1, marked with Δ and grouped in a light blue circle), P. fluorescens 19Fv (B2, marked with □ and grouped in a fuxia circle), P. fluorescens Pf4 (B3, marked with + and grouped in a yellow circle); controls (C and C-P) were marked with • and grouped in a blue circle.
Changes of Volatiles in Response to the Different Microbial Treatments
Strawberry volatile profile revealed the presence of 58 molecules; of these 55 were identified by comparing their experimental mass spectra with the theoretical ones of compounds present in the library NIST 2.0. They belong to different chemical classes: esters, alcohols, aldehydes, ketones, acids and furanones (Figure 4B): esters (39%), alcohols (24.6%), and aldehydes (13.1%) were the most represented.
When compared individually, only two of these compounds showed a significant variation in relation to microorganism inoculation: 3-methyl-1-butyl acetate (ester) and 1,6-heptadien-4-ol (alcohol) (Table 5). The first one significantly increased in SvPf4 plants compared to all the other treatments (Tables 5, 6). Concerning 1,6-heptadien-4-ol, Fm19Fv plants exhibited the highest concentration with significant differences either to controls (C and C-P) or to all Pf4-plants, regardless of the used fungus.
PCA-DA of All Analyzed Parameters
All the considered variables were analyzed by PCA-DA: the samples were separated on the basis of either “fungal” or “bacterial” treatments (score plot analysis Figures 5A,C, respectively). Each fungal inoculation resulted in well separated clusters from the other fungal treatments, and also from the controls (C, C-P), that occupied the central part of the graph. Strawberries treated with F. mosseae, S. viscosum, and R. irregularis displayed variable values of different compounds. As far as the fungal inoculants are concerned, the first canonical variable (D1, 37.9% of explained variance) separated plants inoculated with S. viscosum from those inoculated with R. irregularis or F. mosseae, while the second variable (D2, 35.6% of explained variance) separated plants inoculated with F. mosseae from those inoculated with S. viscosum or R. irregularis (Figure 5A).
The pattern of bacterial treatments resulted as well in a generally good separation (score plot Figure 5C), but with a slight overlapping. However, the cluster of plants inoculated with Pf4 was separated from that of plants inoculated with 5Vm by the first canonical variable (D1, 52.1% of explained variance), while the second variable (D2, 47.9% of explained variance) clearly separated plants inoculated with Pf4 from those inoculated with 19Fv.
In addition, loading plots (Figures 5B,D) showed the distribution of all the analyzed parameters in relation to the axes, allowing to correlate the variables with the specific fungal or bacterial treatments. In particular, the colonization parameters (A%, F% M%, and V%) and P concentration in shoot and root were strongly associated to the presence of R. irregularis (Figure 5B and Table 7), in accordance with the data presented above. Moreover, R. irregularis also modulated some volatiles (ethyl acetate—ester; 1,1-dimethyloxobenzene—eter; 2-butyl octanol, 2,6-dimethyl-heptanol, 3,7-dimethyl-3-octanol, linalool and octanol—alcohols) and chemical elements (Sb—semi metal and Pb—transitional element) (Figure 5B and Tables 4, 6).
S. viscosum mainly influenced growth parameters, in particular root and shoot biomass and chla/chlb ratio, besides the content of malic and fumaric acid in fruits. Many volatile molecules changed in response to S. viscosum inoculation (Table 6): two aldehydes (benzene-acetaldehyde and 4-methyl benzaldehyde), four esters (methyl 3-methylbutanoate, butyl acetate, 3-methyl-1-butyl acetate and 3-methylbutyl butanoate) and one alcohol (tridecanol). Concerning the elements, Rb, Sr, Cs, Li (alkali metals), Y, Yb (rare earth metals), As, Se (semi metal), and V (transitional element) were the most affected by the inoculation with S. viscosum.
Inoculation with F. mosseae was strictly related to the increase in photosynthetic pigment concentration (Figure 5B) in accordance with the data presented in Table 2. The fruit acidity (pH, total acidity and citric acid) as well as sweetness (sucrose and total sugar concentration) and some antioxidant compounds (cyanidin glucoside, cyanidin malonyl glucoside) also varied in presence of F. mosseae. Six esters (butane dien 1, 3 acetate, trans-2-hexenyl acetate, ethyl butanoate, methyl 2-hydroxy-benzoate and 4-ethylbenzoic acid), two aldehydes (2-hexanal and 2, 4-hexadienal) and two alcohols (2-hexyl-1-decanol, hepta-1,6-dien-4-ol) were associated to F. mosseae inoculation, as well as different elements (Table 4) (Co, Cd, Cu, Pd, Ag—transitional elements; Ba—alkali metal; Eu—rare earth metal; P—non metal).
The PCA-DA loading plots also showed that fruit production was mostly influenced by PGPB inoculation (Figure 5D). In particular Pf4 enhanced flower and fruit number and weight, besides increasing shoot biomass. The concentrations of total sugars and malic acid were also positively correlated with Pf4 inoculation, as well as the 3-methyl-1-butyl acetate (ester), phenyl methyl acetate (ester) and tetradecyloxirane (eter) concentration (Tables 6, 7). Concerning the element composition, Pf4 modulated both Cs (alkali metal) and As (semi metal) concentrations in fruits (Table 4).
Inoculation with P. fluorescens 19Fv affected fruit size, acidity and sweetness (especially fructose concentration). The aroma and flavor of 19Fv-fruits were mainly due to the following volatiles compounds: orthoacetic acid trimethylester (ester), methyl butanoate (ester), decyl acetate (ester); dichloromethane (alkane); benzeneacetaldehyde (aldehyde), 2-propylheptanol (alcohol), acetic acid (organic acid). The Se (semi metal), Ni (transitional element), Sc, and Eu (rare earth metals) concentrations increased following 19Fv inoculation.
Inoculation with 5Vm influenced chlorophyll b concentration in leaves, besides the content of fumaric acid and the two types of cyanidin (3-glucoside and malonyl glucoside) in fruits (Table 7). The volatile profile was also affected by the inoculation with this bacterial strain that increased the amounts of 2,4-dimethyl hexane (alkane), 4-methyl benzaldehyde (aldehyde), dioctyl ether (ether); ethyl butanoate (ester), isopropyl dodecanoate (ester); 2,4-bis (1,1-dimethyl) (alcohol), linalool (alcohol), nerolidol (alcohol) and 2,6-di-tert-butylhydroquinone (alcohol). In addition to this, element composition of 5Vm fruits was different from that found in 19Fv- and Pf4-inoculated strawberries. In fact, in this case, inoculation affected the concentration of Gd, Fe, Ag (transitional elements), Ca, Mg (alkali earth metals), Th, Ce, Pr (rare earth metals), Sb (semi metal), S, and P (non-metals).
Discussion
Plant Growth and Production
Inoculation with AMF and PGPB differently affected strawberry plant physiology and biochemistry, also increasing yield and improving fruit quality compared to uninoculated plants grown at the same, low, P level. As a consequence, they were similar (and in some cases even better, depending on the inoculated microorganisms and the considered parameter) to control plants grown at the higher P concentration.
In this study, we used three of the most common fungal species that had been found to be associated with roots of strawberry cultivars in many agricultural soils (Santos-Gonzáles et al., 2011), in combination with three bacterial strains previously tested on strawberry individually or in combination with a mycorrhizal consortium (Lingua et al., 2013; Bona et al., 2015). It is well-known that the outcome of the symbiosis is strictly related to the different fungal-plant combinations: the results obtained inoculating different cultivars with the same fungus (and conversely) can be very different (Gosling et al., 2006 and references therein). This is also true in the case of bacteria (Lingua et al., 2013; Guerrero-Molina et al., 2014; Bona et al., 2015).
In our work, mycorrhizal colonization (M%) in AM plants ranged between 0.3 and 32%, depending on the fungal strain, and was in line with the values presented in other studies (Taylor and Harrier, 2001; Stewart et al., 2005; Bona et al., 2015; Tomè et al., 2015). The best root colonizer was R. irregularis, that generally increased P concentration in both roots and shoots compared to the C-P plants and even to C ones, which were grown at a higher phosphate level. In agreement with various studies (Bucher, 2007; Smith and Smith, 2011; Mensah et al., 2015), higher mycorrhizal colonization was positively correlated with improved P nutrition.
Concerning bacterial inoculation, we did not observe any enhancement of mycorrhization in relation to the three different Pseudomonads (as confirmed by the two-way ANOVA), underlining that, in the present case, they had not a role as MHB; previous reports show that bacterial inoculation can induce decrease (Gamalero et al., 2010) or increase (Pivato et al., 2009) of mycorrhizal colonization, suggesting that effects on root colonization may depend on the different microbial combinations.
Generally, two-way ANOVA suggested that in this experiment the production of shoot and root biomass was significantly affected either by co-inoculation (F × B) or by the factor “Fungus” alone. Although S. viscosum poorly colonized the strawberry root system, Sv-plants gave the best results in terms of root and shoot biomass and root/shoot ratio, possibly depending on the combination with the three different bacterial strains.
F. mosseae, presenting intermediate values of mycorrhization (if compared to the other two fungi), negatively affected plant biomass, albeit showing a higher concentration of photosynthetic pigments (mainly chlorophyll b). Only the factor “Fungus” influenced this last parameter. As previously shown in different studies, AM symbiosis can affect the production of photosynthetic pigments (Mena-Violante et al., 2006; Baslam et al., 2013; Mo et al., 2016).
In contrast with Bona et al. (2015), that previously reported earlier flowering and fruiting in plants of F. ananassa var. Selva inoculated with a “consortium” of AMF (R. intraradices, G. aggregatum, G. viscosum, Claroideoglomus etunicatum, and C. claroideum) and PGPB (the same of our experiment), we did not observe any variation of these two parameters. While such differences could be due to the use of a different strawberry cultivar (Selva vs. Elyana F1), this trend greatly changed from the 5th week after inoculation, showing an increased productivity in inoculated plants, especially in terms of number of flowers and fruits, both cumulative and per plant, if compared to the controls. In particular, Pf4 enhanced fruit production, regardless of the used fungus, either in comparison to the other bacterial treatments or to the controls. A positive effect of AMF-Pf4 inoculation on the number of fruits and yield had also been observed in Bona et al. (2015). As shown by the two-way ANOVA, the factor “Bacterium” significantly affected fruit number, size, and weight, besides the strawberry quality. The increased yield can be explained by a higher number of flowers and larger fruit size (Bona et al., 2015). Moreover, it is well-known that the phytohormones auxin and gibberellins are involved in strawberry fruit development and growth, especially in the enlargement of receptacle, the main factor determining fruit size and shape (Roussos et al., 2009; Csukasi et al., 2011). Since our PGPB strains are able to produce auxin (Berta et al., 2014; Bona et al., 2015, 2017) we can expect that they were also able to affect the fruit size and development.
Fruit Nutritional Quality
Strawberry fruit size, color, and scent are the main factors that influence the consumer to purchase. All these parameters are strictly related to fruit ripeness, a complex process in which the content of sugars, organic acids, vitamins, and volatile compounds greatly changes, affecting the chemical and sensory characteristics of fruits (e.g., pH, total acidity, sweetness, aroma), besides its health-promoting properties (Crespo et al., 2010; Mahmood et al., 2012).
The values of pH recorded in our strawberries were comparable with those found in other varieties (Mahmood et al., 2012; Bona et al., 2015; Concha-Meyer et al., 2016) and reflected the trend of total acidity. The factor “Fungus,” but even more the factor “Bacterium,” significantly modulated these two parameters. Organic acids, partly responsible for the sourness, are also involved in the pH regulation and in the stabilization of anthocyanins, therefore affecting strawberry color (Kallio et al., 2000). The balance between acidity and sweetness plays a key role in the flavor determination (Kallio et al., 2000). Moreover, the concentration of citric and malic acid, the most abundant organic acids in strawberry, can influence the sugar perception. In fact, sweetness is not due to the sugar concentration alone, but also to the low level of organic acids, especially malic (Mikulic-Petkovsek et al., 2007, 2012). According to this, fruits of Ri5Vm plants showed the lowest concentration of malic acid and a high value of sweetness/acidity ratio (Table 3 and Table S1). In general, we detected a significant variation in total acidity and sugar concentration between the various treatments: with few exceptions, the values of acidity were lower in inoculated plants, if compared to C-P ones, leading to an imbalance of sweetness/acidity ratio toward sweetness. The amount of fructose and glucose in fruits was higher than that of sucrose in agreement with different studies reported in the literature (Castellanos-Morales et al., 2010; Akhatou and Fernandez-Recamales, 2014a; Bona et al., 2015). Although the differences between the treatments were not always significant, we observed a general reduction of fructose concentration in fruit of inoculated plants, respect to those of C plants (un-inoculated and grown at higher P level), and this could be explained by the fact that AMF can act as carbon sink (Douds et al., 2000).
FmPf4 fruits had a higher sucrose concentration (Table 3 and Table S1), compared to C-P ones, suggesting that this combination could modulate the production of sugars during photosynthesis. Several studies showed that sugars (mainly sucrose) can influence anthocyanin biosynthesis in fruits (Carbone et al., 2009; Jaakola, 2013; Li et al., 2015). In our work, anthocyanidin concentration was affected by co-inoculation (F × B, as shown by two-way ANOVA), while no effect was attributable to the factors “Fungus” or “Bacterium” alone. Lingua et al. (2013) found a positive effect of microorganism inoculation on cyanidin-3-glucoside and pelargonidin-3-glucoside concentrations. In particular, the 5Vm Pseudomonas strain (the same used in this work) gave the best results if used alone. In agreement with these data, we observed an increased amount of pelargonidin-3-glucoside (and also of total anthocyanins, Figure 3) not in all 5Vm-plants but only in those inoculated with S. viscosum, the fungus that showed the lowest mycorrhizal colonization. Several studies highlighted a significant variation in strawberry anthocyanin concentration in relation to species, cultivars, genotype, growth conditions (temperature, light, fertilization, irrigation), and degree of ripeness (Carbone et al., 2009; Jaakola, 2013; Li et al., 2015), besides to the fact that microorganism inoculation in soil strongly affects the production of secondary metabolites, depending on the used inoculum (Castellanos-Morales et al., 2010; Lingua et al., 2013; Bona et al., 2015).
Fruit Volatilome
A modulation of volatile/aromatic compound production in response to bacterial/fungal inoculation was observed in many plants of economic interest (Copetta et al., 2006; Bailly and Weisskopf, 2012; Hart et al., 2015; Bona et al., 2016 and references therein). About these molecules, no data on fruits from inoculated strawberry plants were found in the literature. Certain volatile compounds in fleshy fruits are markers of the physiological status of the fruit (un-ripe, ripe, decay) and together with sugar, organic acid and phenolic content, is responsible for their characteristic flavor (Forney, 2001; Prat et al., 2014; Feng et al., 2015; Klee and Tieman, 2018). The volatile profile found in our strawberry was comparable with those recorded in other cultivars (irrespective of the method used for the analysis) with esters as the dominant class, followed by alcohols, aldehydes, ketones, ethers, and other compounds present in small amounts (Forney, 2001; Kim et al., 2013; Samykanno et al., 2013; Prat et al., 2014). About 360 different volatile molecules have been identified in strawberries, and only some of them significantly contribute to the unique strawberry flavor and aroma (Larsen et al., 1992; Prat et al., 2014). The characteristic odor and flavor have been described by five basic sensory notes: caramel, fruity, green, lactone-like, and buttery (Cotton, 2012), that are due to compounds belonging to the classes of molecules mentioned above; variations in the chemical composition and proportion of the different compounds drive the perception changes between cultivars and fruit ripening stages. In our case, the analyzed fruits contained molecules responsible for the abovementioned notes, with the exception of buttery (due in general to the presence of 2,3-butanedione—Schieberle and Hofmann, 1997). Esters and aldehydes contribute to the fresh, green and fruity notes of the strawberry flavor (Latrasse, 1991). In particular, esters are qualitatively the most important compounds in strawberry, providing sweet-fruity and floral characteristics to the taste and odor (Forney et al., 2000; Forney, 2001). In our fruits, methyl butanoate, ethyl butanoate, butyl acetate, methyl hexanoate, and ethyl hexanoate were the most represented esters, in agreement with the data reported in the literature (Ozcan and Barringer, 2011). Esters derive from alcohols and acids and are synthesized by enzymatic or chemical processes. Enzymatic formation of esters in plants is catalyzed by alcohol acyltransferase (AAT): a high activity of AAT produces esters in a large amount, resulting in fruits with strong aroma (Perez et al., 1996). Ester enzymatic biosynthesis occurs during ripening and is not affected by homogenization or by other fruit treatments (Perez et al., 1992; Ozcan and Barringer, 2011). Such reports are in accordance with our results since the fruits were harvested at maturity and no differences were recorded between fresh and frozen samples (data not shown). Among the esters, only the amount of 3-methyl-1-butyl acetate was affected by inoculation with significant differences between SvPf4-plants and all the other treatments.
On the basis of PCA-DA, it was observed that ester concentrations in fruits were mostly affected by FmPf4 and SvPf4 inoculations, suggesting a role for microbial treatments in the determination of the sweet-fruity note in strawberry taste and odor. However, the two clusters of points did not overlap, indicating that the molecules affected in the two treatments were different.
Aldehydes contribute to the green note of flavor, and 2-hexenal is mostly responsible of the intense green-fresh odor (Ulrich et al., 1997) of strawberries. In general, the presence of aldehydes in fruits seems to decrease gradually as ripeness progresses, but studies on different cultivars reported contradictory results (Azodanlou et al., 2004). Unsaturated aldehydes (such as hexanal and its derivatives) are considered as the products of fatty acid oxidation. In our strawberries, 3-hexenal was not detected, while hexanal and 2-hexenal were present in all treatments and their concentration followed the trend [hexanal] < [2-hexenal], in agreement with analyses carried out on various berries (Azodanlou et al., 2004; Du et al., 2011; Hempfling et al., 2013). Moreover, as shown by PCA-DA, inoculation with Fm modulated the expression of unsaturated aldehydes, responsible for the fresh-green note of strawberries, while Sv in combination with 19Fv or 5Vm bacterial strains affected the content of aromatic aldehydes (such as benzeneacetaldehyde and 4-methylbenzaldehyde), mainly responsible for fruity cherry odor and honey, sweet and floral taste, with spicy nuances (http://www.thegoodscentscompany.com/).
Alcohols are one of the most representative classes of compounds in strawberries and this was true also in the present study. Terpenoid alcohols, especially the monoterpene linalool and sesquiterpene nerolidol, positively influence fruit flavor and aroma, providing sweet, floral, citrus-like notes and rose, apple, green notes, respectively (Aharoni et al., 2004). Nerolidol and linalool are present in cultivated strawberries, while olefinic monoterpenes (α-pinene, α-terpineol, ß-myrcene, etc.) are characteristic of the woody, turpentine-like, and resinous odor of wild strawberries (Aharoni et al., 2004; Bianchi et al., 2014); this was in agreement with our results, since no olefinic monoterpenes were found in our fruits. Although no significant differences were recorded for the concentrations of individual alcohols between the various treatments, except for Fm19Fv plants that showed the highest value of 1,6-heptadien-4-ol, PCA-DA indicated that the inoculation with Ri and 5Vm was closely associated to the presence of alcohols in fruits.
Fruit Mineral Composition
Strawberries contain many minerals (Perez et al., 1997) that are involved in plant metabolism, besides having an important role in human diet (mainly K, P, Ca, and Mg). We analyzed the elemental composition of strawberry and we detected a total of 51 elements, all at non-toxic concentrations, among which only Ba, Rb, Sr, Cs (alkali metals), and Co (transitional element) significantly varied between some of the treatments. These variations were due to the fungal inoculation (as shown by two-way ANOVA), suggesting that AMF can influence the uptake of these elements and therefore their availability for the accumulation in fruits (Taylor and Harrier, 2001; Hart et al., 2015; Mensah et al., 2015). To our knowledge, no data were recorded in the literature on the effects of microorganism inoculation on alkali metal accumulation in fleshy fruits. PCA-DA showed that the presence of different microorganisms in soil also modulated the fruit elemental composition in different ways. It is noteworthy that each fungus was associated with variations in the concentrations of different elements, and the same was observed also for the three bacterial strains. This trend is in accordance with the data concerning the volatile molecules.
Conclusions
Present results indicate that co-inoculation with appropriate PGPB and AMF can enhance many physiological parameters and improve strawberry yield and quality, as shown by the increased sugar and anthocyanidin concentrations, the variations of pH and of the concentrations of malic acid, volatile compounds and elements.
The factor “Fungus” mostly affected the parameters associated with the vegetative portion of the plant, while the factor “Bacterium” was especially relevant for fruit yield and quality. Moreover, as shown by PCA-DA, the volatile profile and elemental composition were unique to each treatment, and this diversity was due to the influence of each microorganism combination on the production of secondary metabolites, in particular those related to volatile components. Therefore, fruits from each microbial treatment were different and this can result in unique organoleptic and qualitative characteristics. In the present study, it was shown for the first time that the fruit concentration of some elements and/or volatiles can be affected by the presence of specific microorganisms in the soil.
In addition, our results allow to prospect the selection of optimal plant-microorganism combinations for in-field inoculation, reducing chemical input, leading to economical and ecological soil sustainability and improving fruit production and quality, also in terms of health promoting properties.
Author Contributions
Experimental planning was carried out by GB, DW, and GL. Experimental procedures were mainly carried out by VT and NA, with the help of EB (sample preparation), FG (GC-MS and ICP-OES analyses), EMZ (GC-MS and ICP-OES analyses), FM (HPLC analysis), NM (plant harvest, sample preparation). Statistical data analysis was performed by GL, VT, and ER (multivariate statistical analysis and model interpretation). Data interpretation and overview was carried out by VT, NA, GL, GB, DW, LB, ER, and EMR (the last two for multivariate statistical analysis and model interpretation). The manuscript was written by VT and NL and revised by all the authors.
Conflict of Interest Statement
The authors declare that the research was conducted in the absence of any commercial or financial relationships that could be construed as a potential conflict of interest.
Acknowledgments
This work was supported by the Vinci program (French-Italian University) for the mobility of NA.
We wish to thank Ms. Donata Vigani and Mr. Marco Sobrero for technical support.
Supplementary Material
The Supplementary Material for this article can be found online at: https://www.frontiersin.org/articles/10.3389/fpls.2018.01611/full#supplementary-material
References
Aharoni, A., Giri, A. P., Verstappen, F. W. A., Bertea, C. M., Sevenier, R., Sun, Z., et al. (2004). Gain and loss of fruit flavor compounds produced by wild and cultivated strawberry species. Plant Cell 16, 3110–3131. doi: 10.1105/tpc.104.023895
Akhatou, I., and Fernandez-Recamales, A. (2014a). Influence of cultivar and culture system on nutritional and organoleptic quality of strawberry. J. Sci. Food Agric. 94, 866–875. doi: 10.1002/jsfa.6313
Akhatou, I., and Fernandez-Recamales, A. (2014b). Nutritional and nutraceutical quality of strawberries in relation to harvest time and crop conditions. J. Sci. Food Agric. 62, 5749–5760. doi: 10.1021/jf500769x
Antunes, P. M., Franken, P., Schwarz, D., Rillig, M. C., Cosme, M., Scott, M., et al. (2012). “Linking soil biodiversity and human health: do arbuscular mycorrhizal fungi contribute to food nutrition?” in Soil Ecology and Ecosystem Services, eds D. H. Wall, R. D. Bardgett, V. Behan-Pelletier, J. E. Herrick, T. Hefin Jones, K. Ritz, J. Six, D. R. Strong and W. H. van der Putten. (Oxford, UK: Oxford University Press), 153–172. doi: 10.1093/acprof:oso/9780199575923.003.0015
Artursson, V., Finlay, R. D., and Jansson, J. K. (2006). Interactions between arbuscular mycorrhizal fungi and bacteria and their potential for stimulating plant growth. Environ. Microbiol. 8, 1–10. doi: 10.1111/j.1462-2920.2005.00942.x
Azodanlou, R., Darbellay, C., Luisier, J. L., Villettaz, J. C., and Amado, R. (2004). Changes in flavor and texture during the ripening of strawberries. Eur. Food Res. Technol. 218, 167–172. doi: 10.1007/s00217-003-0822-0
Bailly, A., and Weisskopf, L. (2012). The modulating effect of bacterial volatiles on plant growth: current knowledge and future challenges. Plant Signal. Behav. 7, 79–85. doi: 10.4161/psb.7.1.18418
Baslam, M., Esteban, R., García-Plazaola, J. I., and Goicoechea, N. (2013). Effectiveness of arbuscular mycorrhizal fungi (AMF) for inducing the accumulation of major carotenoids, chlorophylls and tocopherol in green and red leaf lettuces. Appl. Microbiol. Biotechnol. 97, 3119–3128. doi: 10.1007/s00253-012-4526-x
Benizri, E., Baudoin, E., and Guckert, A. (2001). Root colonization by inoculated plant growth promoting rhizobacteria. Biocontrol Sci. Technol. 11, 557–574. doi: 10.1080/09583150120076120
Berta, G., Copetta, A., Gamalero, E., Bona, E., Cesaro, P., Scarafoni, A., et al. (2014). Maize development and grain quality are differentially affected by mycorrhizal fungi and a growth-promoting pseudomonad in the field. Mycorrhiza 24,161–170. doi: 10.1007/s00572-013-0523-x
Bianchi, G., Lovazzano, A., Lanubile, A., and Marocco, A. (2014). Aroma quality of fruits of wild and cultivated strawberry (Fragaria spp.) in relation to the flavour-related gene expression. J. Hortic. Res. 22, 77–84. doi: 10.2478/johr-2014-0009
Bicchi, C., and Maffei, M. E. (2012). “The plant volatilome: methods of analysis,” in High-Throughput Phenotyping in Plants. Methods in Molecular Biology (Methods and Protocols), ed J. Normanly (Totowa, NJ: Humana Press), 289–310. doi: 10.1007/978-1-61779-995-2_15
Bona, E., Cantamessa, S., Massa, N., Manassero, P., Marsano, F., Copetta, A., et al. (2017). Arbuscular mycorrhizal fungi and plantgrowth-promoting pseudomonads improve yield, quality and nutritional value of tomato: a field study. Mycorrhiza 27, 1–11. doi: 10.1007/s00572016-0727-y
Bona, E., Lingua, G., Manassero, P., Cantamessa, S., Marsano, F., Todeschini, V., et al. (2015). AM fungi and PGP pseudomonads increase flowering, fruit production, and vitamin content in strawberry grown at low nitrogen and phosphorus levels. Mycorrhiza 25, 181–193. doi: 10.1007/s00572-014-0599-y
Bona, E., Lingua, G., and Todeschini, V. (2016). “Effect of bioinoculants on the quality of crops” in Bioformulations: For Sustainable Agriculture, eds N. K. Arora, S. Mehnaz, and R. Balestrini (New Delhi: Springer), 93–124. doi: 10.1007/978-81-322-2779-3_5
Borkowska, B. (2002). Growth and photosynthetic activity of micropropagated strawberry plants inoculated with endomycorrhizal fungi (AMF) and growing under drought stress. Acta Physiol. Plant. 24, 365–370. doi: 10.1007/s11738-002-0031-7
Borowicz, V. A. (2001). Do arbuscular mycorrhizal fungi alter plant pathogen relations? Ecology 82, 3057–3068. doi: 10.1890/0012-9658(2001)082[3057:DAMFAP]2.0.CO;2
Boyer, L. R., Brain, P., Xu, X. M., and Jeffries, P. (2015). Inoculation of drought-stressed strawberry with a mixed inoculum of two arbuscular mycorrhizal fungi: effects on population dynamics of fungal species in roots and consequential plant tolerance to water deficiency. Mycorrhiza 25, 215–227. doi: 10.1007/s00572-014-0603-6
Bucher, M. (2007). Functional biology of plant phosphate uptake at root and mycorrhiza interfaces. New Phytol. 173, 11–26. doi: 10.1111/j.1469-8137.2006.01935.x
Buttery, R. G. (1981). “Vegetable and fruit flavors” in Flavor Research: Recent Advances, eds R. Teranishi, R. A. Flath, and H. Sugisawa (New York, NY: Marcel Dekker), 175–216.
Carbone, F., Preuss, A., De Vos, R. C., D'Amico, E., Perrotta, G., Bovy, A. G., et al. (2009). Developmental, genetic and environmental factors affect the expression of flavonoid genes, enzymes, and metabolites in strawberry fruits. Plant Cell Environ. 32, 1117–1131. doi: 10.1111/j.1365-3040.2009.01994.x
Castellanos-Morales, V., Villegas, J., Wendelin, S., Vierheilig, H., Ederc, R., and Cardenas-Navarroa, R. (2010). Root colonisation by the arbuscular mycorrhizal fungus Glomus intraradices alters the quality of strawberry fruits (Fragaria × ananassa Duch.) at different nitrogen levels. J. Sci. Food Agric. 90, 1774–1782. doi: 10.1002/jsfa.3998
Chandra, A., Rana, J., and Li, Y. (2001). Separation, identification, quantification, and method validation of anthocyanins in botanical supplement raw materials by HPLC and HPLC-MS. J. Agric. Food Chem. 49, 3515–3521. doi: 10.1021/jf010389p
Christensen, C. (1983). Effect of color on aroma, flavor and texture judgment of food. J. Food Sci. 48, 787–790. doi: 10.1111/j.1365-2621.1983.tb14899.x
Colquhon, T., Levin, L., Mosckowitz, H., Whiteker, V., Clark, D., and Folta, K. (2012). Framing the perfect strawberry: an exercise in consumer-assisted selection of fruit crops. J. Berry Res. 2, 45–61. doi: 10.3233/JBR-2011-027
Comandini, P., Blanda, G., Cardinali, A., Cerretani, L., Bendini, A., and Caboni, M. F. (2008). CZE separation of strawberry anthocyanins with acidic buffer and comparison with HPLC. J. Sep. Sci. 31, 3257–3264. doi: 10.1002/jssc.200800199
Concha-Meyer, A. A., D'Ignoti, V., Saez, B., Diaz, R. I., and Torres, C. A. (2016). Effect of storage on the physico-chemical and antioxidant properties of strawberry and kiwi leathers. J. Food Sci. 81, C569–C577. doi: 10.1111/1750-3841.13214
Copetta, A., Lingua, G., and Berta, G. (2006). Effects of three AM fungi on growth, distribution of glandular hairs, and essential oil production in Ocimum basilicum L. var. Genovese. Mycorrhiza 16, 485–494. doi: 10.1007/s00572-006-0065-6
Cordenunsi, B. R., Oliveira do Nascimento, J. R., Genovese, M. I., and Lajolo, F. M. (2002). Influence of cultivar on quality parameters and chemical composition of strawberry fruits grown in Brazil. J. Agric. Food Chem. 50, 2581–2586. doi: 10.1021/jf011421i
Crespo, P., Bordonaba, J. G., Terry, L. A., and Carlen, C. (2010). Characterization of major taste and health-related compounds of four strawberry genotypes grown at different Swiss production sites. Food Chem. 122, 16–24. doi: 10.1016/j.foodchem.2010.02.010
Csukasi, F., Osorio, S., Gutierrez, J. R., Kitamura, J., Giavalisco, P., Nakajima, M., et al. (2011). Gibberellin biosynthesis and signalling during development of the strawberry receptacle. New Phytol. 191, 376–390. doi: 10.1111/j.1469-8137.2011.03700.x
da Silva Pinto, M., Lajolo, F. M., and Genovese, M. I. (2008). Bioactive compounds and quantification of total ellagic acid in strawberries (Fragaria x ananassa Duch.). Food Chem. 107, 1629–1635. doi: 10.1016/j.foodchem.2007.10.038
Darrow, G. M., and Wallace, H. A. (1966). The Strawberry: History, Breeding, and Physiology. San Francisco, CA: Holt, Rinehart and Winston.
Diamanti, J., Capocasa, F., Balducci, F., Battino, M., Hancock, J., and Mezzetti, B. (2012). Increasing strawberry fruit sensorial and nutritional quality using wild and cultivated germplasm. PLoS ONE 7:e46470. doi: 10.1371/journal.pone.0046470
Douds, D. D., Pffefer, P. E., and Shachar-Hill, Y. (2000). “Carbon partitioning, cost and metabolism of arbuscular mycorrhizas” in Arbuscular Mycorrhizas: Physiology and Function, eds Y. Kapulnick and D. D. Douds (Dordrecht: Springer), 107–129. doi: 10.1007/978-94-017-0776-3_6
Du, X., Plotto, A., Song, M., Olmstead, J., and Rouseff, R. (2011). Volatile composition of four Southern highbush blueberry cultivars and effect of growing location and harvest date. J. Agric. Food Chem. 59:8347–8357. doi: 10.1021/jf201184m
Duijff, B. J., Gianinazzi-Pearson, V., and Lemanceau, P. (1997). Involvement of the outer membrane lipopolysaccharides in the endophytic colonization of tomato roots by biocontrol Pseudomonas fluorescens strain WCS417r. New Phytol. 135, 325–334. doi: 10.1046/j.1469-8137.1997.00646.x
Feng, Y., Liu, M., Ouyang, Y., Zhao, X., Ju, Y., and Fang, Y. (2015). Comparative study of aromatic compounds in fruit wines from raspberry, strawberry, and mulberry in central Shaanxi area. Food Nutr. Res. 59:29290. doi: 10.3402/fnr.v59.29290
Forney, C. F. (2001). Horticultural and other factors affecting aroma volatile composition of small fruit. HortTechnol. 11, 529–538.
Forney, C. F., Kalt, W., and Jordan, M. A. (2000). The composition of strawberry aroma is influenced by cultivar, maturity, and storage. HortSci. 35, 1022–1026.
Frey-Klett, P., Garbaye, J., and Tarkka, M. (2007). The mycorrhiza helper bacteria revisited. New Phytol. 176, 22–36. doi: 10.1111/j.1469-8137.2007.02191.x
Gamalero, E., Berta, G., Massa, N., Glick, B. R., and Lingua, G. (2008). Synergistic interactions between the ACC deaminase-producing bacterium Pseudomonas putida UW4 and the AM fungus Gigaspora rosea positively affect cucumber plant growth. FEMS Microbiol. Ecol. 64, 459–467. doi: 10.1111/j.1574-6941.2008.00485.x
Gamalero, E., Berta, G., Massa, N., Glick, B. R., and Lingua, G. (2010). Interactions between Pseudomonas putida UW4 and Gigaspora rosea BEG9 and their consequences on the growth of cucumber under salt stress conditions. J. Appl. Microbiol. 108, 236–245. doi: 10.1111/j.1365-2672.2009.04414.x
Gaur, A., Gaur, A., and Adholeya, A. (2000). Growth and flowering in Petunia hybrida, Callistephus chinensis and Impatiens balsamina inoculated with mixed AM inocula or chemical fertilizers in a soil of low P fertility. Sci. Hortic. 84, 151–162. doi: 10.1016/S0304-4238(99)00105-3
Ghosh, S., Penterman, J. N., Little, R. D., Chavez, R., and Glick, B. R. (2003). Three newly isolated plant growth-promoting bacilli facilitate the seedling growth of canola, Brassica campestris. Plant Physiol. Biochem. 41, 277–281. doi: 10.1016/S0981-9428(03)00019-6
Giampieri, F., Tulipani, S., Alvarez-Suarez, J. M., Quiles, J. L., Mezzetti, B., and Battino, M. (2012). The strawberry: composition, nutritional quality, and impact on human health. Nutrition 28, 9–19. doi: 10.1016/j.nut.2011.08.009
Gianinazzi, S., Gollotte, A., Binet, M. N., van Tuinen, D., Redecker, D., and Wipf, D. (2010). Agroecology: the key role of arbuscular mycorrhizas in ecosystem services. Mycorrhiza 20 , 519–530. doi: 10.1007/s00572-010-0333-3
Giovannetti, M., Avio, L., and Sbrana, C. (2013). “Improvement of nutraceutical value of food by plant symbionts” in Natural Products, eds K. G. Ramawat and J. M. Merillon (Berlin; Heidelberg: Springer), 2641–2662. doi: 10.1007/978-3-642-22144-6_187
Glick, B. R. (1995). The enhancement of plant growth by free-living bacteria. Can. J. Microbiol. 41, 109–117. doi: 10.1139/m95-015
Gollop, R., Even, S., Colova-Tsolova, V., and Perl, A. (2002). Expression of the grape dihydroflavonol reductase gene and analysis of its promoter region. J. Exp. Bot. 53, 1397–1409. doi: 10.1093/jxb/53.373.1397
Gosling, P., Hodge, A., Goodlass, G., and Bending, G. D. (2006). Arbuscular mycorrhizal fungi and organic farming. Agric. Ecosyst. Environ. 113, 17–35. doi: 10.1016/j.agee.2005.09.009
Guerrero-Molina, M. F., Lovaisa, N. C., Salazar, S. M., Diaz-Ricci, J. C., and Pedraza, R. O. (2014). Elemental composition of strawberry plants inoculated with the plant growth-promoting bacterium Azospirillum brasilense REC3, assessed with scanning electron microscopy and energy dispersive X-ray analysis. Plant Biol. 16, 726–731. doi: 10.1111/plb.12114
Hart, M., Ehret, D. L., Krumbein, A., Leung, C., Murch, S., Turi, C., et al. (2015). Inoculation with arbuscular mycorrhizal fungi improves the nutritional value of tomatoes. Mycorrhiza 25, 359–376. doi: 10.1007/s00572-014-0617-0
He, J., and Giusti, M. M. (2010). Anthocyanins: natural colorants with health-promoting properties. Annu. Rev. Food Sci. Technol. 1, 163–187. doi: 10.1146/annurev.food.080708.100754
Hegde, D. M., Dwived, B. S., and Sudhakara, S. N. (1999). Biofertilizers for cereal production in India-a review. Indian, J. Agric. Sci. 69, 73–83.
Hempfling, K., Fastowski, O., Celik, J., and Engel, K. H. (2013). Analysis and sensory evaluation of jostaberry (Ribes x nidigrolaria Bauer) volatiles. J. Agric. Food Chem. 61, 9067–9075. doi: 10.1021/jf403065e
Hoogerbrugge, R., Willig, S. J., and Kistemaker, P. G. (1983). Discriminant analysis by double stage principal component analysis. Anal. Chem. 55, 1710–1712. doi: 10.1021/ac00261a016
Hršelová, H., Gryndler, M., and Vančura, V. (1990). Influence of inoculation with VA mycorrhizal fungus Glomus sp. on growth of strawberries and runner formation. Agric. Ecosyst. Environ. 29, 193–197. doi: 10.1016/0167-8809(90)90276-J
Jaakola, L. (2013). New insights into the regulation of anthocyanin biosynthesis in fruits. Trends Plant Sci. 18, 477–483. doi: 10.1016/j.tplants.2013.06.003
Jia, H. F., Chai, Y. M., Li, C. L., Lu, D., Luo, J. J., Qin, L., et al. (2011). Abscisic acid plays an important role in the regulation of strawberry fruit ripening. Plant Physiol. 157, 188–199. doi: 10.1104/pp.111.177311
Kallio, H., Hakala, M., Pelkkikangas, A. M., and Lapveteläinen, A. (2000). Sugars and acids of strawberry varieties. Eur. Food Res. Technol. 212, 81–85. doi: 10.1007/s002170000244
Keutgen, A., and Pawelzik, E. (2007). Food chemistry modifications of taste-relevant compounds in strawberry fruit under NaCl salinity. Food Chem. 105, 1487–1494. doi: 10.1016/j.foodchem.2007.05.033
Kim, Y. H., Kim, K. H., Szulejko, J. E., and Parker, D. (2013). Quantitative analysis of fragrance and odorants released from fresh and decaying strawberries. Sensors 13, 7939–7978. doi: 10.3390/s130607939
Klee, H. J., and Tieman, D. M. (2018). The genetics of fruit flavour preferences. Nature Rev. Genet. 19, 347–356. doi: 10.1038/s41576-018-0002-5
Larsen, M., Poll, L., and Olsen, C. (1992). Evaluation of the aroma composition of some strawberry (Fragaria ananassa Duch) cultivars by use of odor threshold values. Z. Lebensm. Unters Forsch. 195, 536–539.
Latrasse, A. (1991). “Fruits III” in Volatile Compounds in Foods and Beverages, ed. H. Maarse (New York, NY: Marcel Dekker), 329–387.
Li, D., Li, L., Luo, Z., Mou, W., Mao, L., and Ying, T. (2015). Comparative transcriptome analysis reveals the influence of abscisic acid on the metabolism of pigments, ascorbic acid and folic acid during strawberry fruit ripening. PLoS ONE 10:e0130037. doi: 10.1371/journal.pone.0130037
Lingua, G., Bona, E., Manassero, P., Marsano, F., Todeschini, V., Cantamessa, S., et al. (2013). Arbuscular mycorrhizal fungi and plant growth-promoting pseudomonads increases anthocyanin concentration in strawberry fruits (Fragaria x ananassa var. Selva) in conditions of reduced fertilization. Int. J Mol. Sci. 14, 16207–16225. doi: 10.3390/ijms140816207
Lingua, G., Franchin, C., Todeschini, V., Castiglione, S., Biondi, S., Burlando, B., et al. (2008). Arbuscular mycorrhizal fungi differentially affect the response to high zinc concentrations of two registered poplar clones. Environ. Pollut. 153. 147–157. doi: 10.1016/j.envpol.2007.07.012
Lu, X., and Koide, R. T. (1994). The effects of mycorrhizal infection on components of plant growth and reproduction. New Phytol. 128, 211–218. doi: 10.1111/j.1469-8137.1994.tb04004.x
Mahmood, T., Anwar, F., Abbas, M., Boyce, M. C., and Saari, N. (2012). Compositional variation in sugars and organic acids at different maturity stages in selected small fruits from Pakistan. Int. J. Mol. Sci. 13, 1380–1392. doi: 10.3390/ijms13021380
Massart, D. L., Vanderghinste, B. G. M., Buydens, L. M. C., De Jong, S., Lewi, P. J., and Smeyers-Verbeke, J. (1998). Handbook of Chemometrics and Qualimetrics: Part A. Amsterdam: Elsevier.
Mena-Violante, H. G., Ocampo-Jiménez, O., Dendooven, L., Martínez-Soto, G., González-Castañeda, J., Davies, F. T., et al. (2006). Arbuscular mycorrhizal fungi enhance fruit growth and quality of chile ancho (Capsicum annuum L. cv San Luis) plants exposed to drought. Mycorrhiza 16, 261–267. doi: 10.1007/s00572-006-0043-z
Mensah, J. A., Koch, A. M., Antunes, P. M., Kiers, E. T., Hart, M., and Bücking, H. (2015). High functional diversity within species of arbuscular mycorrhizal fungi is associated with differences in phosphate and nitrogen uptake and fungal phosphate metabolism. Mycorrhiza 25, 533–546. doi: 10.1007/s00572-015-0631-x
Mikulic-Petkovsek, M., Schmitzer, V., Slatnar, A., Stampar, F., and Veberic, R. (2012). Composition of sugars, organic acids, and total phenolics in 25 wild or cultivated berry species. J. Food Sci. 77, C1064–C1070. doi: 10.1111/j.1750-3841.2012.02896.x
Mikulic-Petkovsek, M., Stampar, F., and Veberic, R. (2007). Parameters of inner quality of the apple scab resistant and susceptible apple cultivars (Malus domestica Borkh.). Sci. Hortic. 114, 37–44. doi: 10.1016/j.scienta.2007.05.004
Mo, Y., Wang, Y., Yang, R., Zheng, J., Liu, C., Li, H., et al. (2016). Regulation of plant growth, photosynthesis, antioxidation and osmosis by an arbuscular mycorrhizal fungus in watermelon seedlings under well-watered and drought conditions. Front. Plant Sci. 7:644. doi: 10.3389/fpls.2016.00644
Moing, A., Renaud, C., Gaudillère, M., Raymond, P., Roudeillac, P., and Denoyes-Rothan, B. (2001). Biochemical changes during fruit development of four strawberry cultivars. J. Amer. Soc. Hort. Sci. 126, 394–403.
Montero, T. M., Mollà, E. M., Esteban, R. M., and Lòpez-Andréu, F. J. (1996). Quality attributes of strawberry during ripening. Sci. Hort. 65, 239–250. doi: 10.1016/0304-4238(96)00892-8
Mugnier, J., and Mosse, B. (1987). Vesicular-arbuscular mycorrhizal infections in transformed Ri T-DNA roots grown axenically. Phytopathology 77, 1045–1050.
Nautiyal, C. S., Govindarajan, R., Lavania, M., and Pushpangadan, P. (2008). Novel mechanism of modulating natural antioxidants in functional foods: involvement of plant growth promoting rhizobacteria NRRL B-30488. J. Agric. Food Chem. 56, 4474–4481. doi: 10.1021/jf073258i
Negri, A. S., Allegra, D., Simoni, L., Rusconi, F., Tonelli, C., Espen, L., et al. (2015). Comparative analysis of fruit aroma patterns in the domesticated wild strawberries “Profumata di Tortona” (F. moschata) and “Regina delle Valli” (F. vesca). Front. Plant Sci. 6:56. doi: 10.3389/fpls.2015.00056
Negri, A. S., Robotti, E., Prinsi, B., Espen, L., and Marengo, E. (2011). Proteins involved in biotic and abiotic stress responses as the most significant biomarkers in the ripening of Pinot Noir skins. Funct. Integr. Genomics 11 , 341–355. doi: 10.1007/s10142-010-0205-0
Olsson, M. E., Ekvall, J., Gustavsson, K. E., Nilsson, J., Pillai, D., Sjöholm, I., et al. (2004). Antioxidants, low molecular weight carbohydrates, and total antioxidant capacity in strawberries (Fragaria x ananassa): effects of cultivar, ripening, and storage. J. Agric. Food Chem. 52, 2490–2498. doi: 10.1021/jf030461e
Ozcan, G., and Barringer, S. (2011). Effect of enzymes on strawberry volatiles during storage, at different ripeness level, in different cultivars, and during eating. J. Food Sci.76, C324–C332. doi: 10.1111/j.1750-3841.2010.01999.x
Palencia, P., Martinez, F., and Weiland, C. (2013). Effect of arbuscular mycorrhizal fungi on quality of strawberry fruit in soilless growing system. Acta Hortic. 1013, 493–498. doi: 10.17660/ActaHortic.2013.1013.61
Perez, A. G., Olias, J. M., Espada, J., Olias, J. M., and Sanz, C. (1997). Rapid determination of sugars, nonvolatile acids, and ascorbic acid in strawberry and other fruits. J. Agric. Food Chem. 45, 3545–3549. doi: 10.1021/jf9701704
Perez, A. G., Rios, J. J., Sanz, C., and Olias, J. M. (1992). Aroma components and free amino acids in strawberry variety Chandler during ripening. J. Agric. Food Chem. 40, 2232–2235. doi: 10.1021/jf00023a036
Perez, A. G., Sanz, C., Olas, R., Ros, J. J., and Olias, J. M. (1996). Evolution of strawberry alcohol acyltransferase activity during fruit development and storage. J. Agric. Food Chem. 44, 3286–3290. doi: 10.1021/jf960040f
Pešaković, M., Karaklajić-Stajić, Ž., Milenković, S., and Mitrović, O. (2013). Biofertilizer affecting yield related characteristics of strawberry (Fragaria×ananassa Duch.) and soil micro-organisms. Sci. Hortic. 150, 238–243. doi: 10.1016/j.scienta.2012.11.016
Pivato, B., Offre, P., Marchelli, S., Barbonaglia, B., Mougel, C., Lemanceau, P., et al. (2009). Bacterial effects on arbuscular mycorrhizal fungi and mycorrhiza development as influenced by the bacteria, fungi, and host plant. Mycorrhiza 19, 81–90. doi: 10.1007/s00572-008-0205-2
Porra, R. J., Thompson, W. A., and Kriedemann, P. E. (1989). Determination of accurate extinction coefficients and simultaneous equations for assaying chlorophylls a and b extracted with four different solvents: verification of the concentration of chlorophyll standards by atomic absorption spectrometry. Biochim. Biophys. Acta 975, 384–394. doi: 10.1016/S0005-2728(89)80347-0
Poulton, J. L., Bryla, D., Koide, R. T., and Stephenson, A. G. (2002). Mycorrhizal infection and high soil phosphorus improve vegetative growth and the female and male functions in tomato. New Phytol. 154, 255–264. doi: 10.1046/j.1469-8137.2002.00366.x
Prat, L., Espinoza, M. I., Agosin, E., and Silva, H. (2014). Identification of volatile compounds associated with the aroma of white strawberries (Fragaraia chiloensis). J. Sci. Food Agric. 94, 752–759. doi: 10.1002/jsfa.6412
Roussos, P. A., Denaxa, N. K., and Damvakaris, T. (2009). Strawberry fruit quality attributes after application of plant growth stimulating compounds. Sci Hortic. 119, 138–146. doi: 10.1016/j.scienta.2008.07.021
Saharan, B. S., and Nehra, V. (2011). Plant growth promoting rhizobacteria: a critical review. Rec. Adv. Biol. Med. 21, 1–30.
Samykanno, K., Pang, E., and Marriott, P. J. (2013). Chemical characterisation of two Australian-grown strawberry varieties by using comprehensive two-dimensional gas chromatography-mass spectrometry. Food Chem. 141, 1997–2005. doi: 10.1016/j.foodchem.2013.05.083
Santos-Gonzáles, J. C., Nallanchakravarthula, S., Alström, S., and Finlay, R. D. (2011). Soil, but not cultivar, shapes the structure of arbuscular mycorrhizal fungal assemblages associated with strawberry. Microb. Ecol. 62, 25–35. doi: 10.1007/s00248-011-9834-7
Sanz, C., Olias, J. M., and Perez, A. G. (1997). “Aroma biochemistry of fruits and vegetables” in Phytochemistry of Fruit and Vegetables, eds F. A. Tomás-Barberán and R. J. Robins (Oxford: Clarendon Press), 125–155.
Sbrana, C., Avio, L., and Giovannetti, M. (2014). Beneficial mycorrhizal symbionts affecting the production of health-promoting phytochemicals. Electrophoresis 35, 1535–1546. doi: 10.1002/elps.201300568
Schieberle, P., and Hofmann, T. (1997). Evaluation of the character impact odorants in fresh strawberry juice by quantitative measurements and sensory studies on model mixtures. J Agric. Food Chem. 45, 227–232. doi: 10.1021/jf960366o
Schwieterman, M. L., Colquhoun, T. A., Jaworski, E. A., Bartoshuk, L. M., Gilbert, J. L., Tieman, D. M., et al. (2014). Strawberry Flavor: diverse chemical compositions, a seasonal influence, and effects on sensory perception. PLoS ONE 9:e88446. doi: 10.1371/journal.pone.0088446
Smith, S., Facelli, E., Pope, S., and Smith, A. F. (2010). Plant performance in stressful environments: interpreting new and established knowledge of the roles of arbuscular mycorrhizas. Plant Soil 326, 3–20.
Smith, S. E., and Smith, F. A. (2011). Roles of arbuscular mycorrhizas in plant nutrition and growth: new paradigms from cellular to ecosystem scales. Annu. Rev. Plant Biol. 62, 227–250. doi: 10.1146/annurev-arplant-042110-103846
Sohn, B. K., Kim, K. Y., Chung, S. J., Kim, W. S., Park, S. M., Kang, J. G., et al. (2003). Effect of the different timing of AMF inoculation on plant growth and flower quality of chrysanthemum. Sci. Hortic. 98, 173–183. doi: 10.1016/S0304-4238(02)00210-8
Spatafora, J. W., Chang, Y., Benny, G. L., Lazarus, K., Smith, M. E., Berbee, M. L., et al. (2016). A phylum-level phylogenetic classification of zygomycete fungi based on genome-scale data. Mycologia 108, 1028–1046. doi: 10.3852/16-042
Stewart, L. I., Hamel, C., Hogue, R., and Moutoglis, P. (2005). Response of strawberry to inoculation with arbuscular mycorrhizal fungi under very high soil phosphorus conditions. Mycorrhiza 15, 612–619. doi: 10.1007/s00572-005-0003-z
Taylor, J., and Harrier, L. A. (2001). A comparison of development and mineral nutrition of micropropagated Fragaria × ananassa cv. Elvira (strawberry) when colonised by nine species of arbuscular mycorrhizal fungi. Appl. Soil Ecol. 18, 205–215. doi: 10.1016/S0929-1393(01)00164-0
Tomè, E., Tagliavini, M., and Scandellari, F. (2015). Recently fixed carbon allocation in strawberry plants and concurrent inorganic nitrogen uptake through arbuscular mycorrhizal fungi. J. Plant Physiol. 179, 83–89. doi: 10.1016/j.jplph.2015.02.008
Trotta, A., Varese, G. C., Gnavi, E., Fusconi, A., Sampo, S., and Berta, G. (1996). Interactions between the soilborne root pathogen Phytophthora nicotianae var parasitica and the arbuscular mycorrhizal fungus Glomus mosseae in tomato plants. Plant Soil 185, 199–209. doi: 10.1007/BF02257525
Trouvelot, A., Kough, J., and Gianinazzi-Pearson, V. (1986). “Mesure du taux de mycorrhization VA d'un système Radiculaire in Recherche de Méthodes D'estimation Ayant une Signification Functionnelle” in Physiological and Genetical Aspects of Mycorrhizae, eds V. Gianninazzi-Pearson and S. Gianinazzi (Paris: INRA), 217–221.
Tulipani, S., Mezzetti, B., and Battino, M. (2009). Impact of strawberries on human health: insight into marginally discussed bioactive compounds for the Mediterranean diet. Public Health Nutr. 12, 1656–1662. doi: 10.1017/S1368980009990516
Tulipani, S., Mezzetti, B., Capocasa, F., Bompadre, S., Beekwilder, J., de Vos, C. H. R., et al. (2008). Antioxidants, phenolic compounds and nutritional quality in different strawberry genotypes. J. Agric. Food. Chem. 56, 696–704. doi: 10.1021/jf0719959
Ulrich, D., Hoberg, E., Rapp, A., and Kecke, S. (1997). Analysis of strawberry flavor-discriminant of aroma types by quantification of volatile compounds. Z. Lebensm. Unters. Forsch. A 205, 218–223. doi: 10.1007/s002170050154
Ulrich, D., Kecke, S., and Olbrich, K. (2018). What do we know about the chemistry of strawberry aroma? J. Agric. Food Chem. 66, 3291–3301. doi: 10.1021/acs.jafc.8b01115
Ulrich, D., Komes, D., Olbricht, K., and Hoberg, E. (2007). Diversity of aroma patterns in wild and cultivated Fragaria accessions. Genet. Resour. Crop Evol. 54, 1185–1196. doi: 10.3233/JBR-140067
Valentinuzzi, F., Mason, M., Scampicchio, M., Andreotti, C., Cesco, S., and Mimmo, T. (2014). Enhancement of the bioactive compound content in strawberry fruits grown under iron and phosphorus deficiency. J. Sci. Food Agric. 85, 2088–2094. doi: 10.1002/jsfa.6924
Vazquez, P., Holguin, G., Puente, M. E., Lopez-Cortes, A., and Bashan, Y. (2000). Phosphate-solubilizing microorganisms associated with the rhizosphere of mangroves in a semiarid coastal lagoon. Biol. Fertil. Soils 30, 460–468. doi: 10.1007/s003740050024
Vessey, J. K. (2003). Plant growth promoting rhizobacteria as biofertilizers. Plant Soil 255, 571–586. doi: 10.1023/A:1026037216893
Vosatka, M., Gryndler, M., Jansa, J., and Vohnik, M. (2000). Post vitro mycorrhization and bacterization of micropropagated strawberry, potato and azalea. Acta Hortic. 530, 313–324. doi: 10.17660/ActaHortic.2000.530.37
Vosatka, M., Gryndler, M., and Prikryl, Z. (1992). Effect of the rhizosphere bacterium Pseudomonas putida, arbuscular mycorrhizal fungi and substrate composition on the growth of strawberry. Agronomie 12, 859–863. doi: 10.1051/agro:19921021
Wang, S. Y., and Lewers, K. S. (2007). Antioxidant capacity and flavonoid content in wild strawberries. J. Am. Soc. Hortic. Sci. 132, 629–637.
Xu, W., Peng, H., Yang, T., Whitaker, B., Huang, L., Sun, J., et al. (2014). Effect of calcium on strawberry fruit flavonoid pathway gene expression and anthocyanin accumulation. Plant Physiol. Biochem. 82, 289–298. doi: 10.1016/j.plaphy.2014.06.015
Keywords: PGPB, AMF, strawberry, nutritional quality, volatile compounds, sustainable agriculture, biofertilizers, chemometrics
Citation: Todeschini V, AitLahmidi N, Mazzucco E, Marsano F, Gosetti F, Robotti E, Bona E, Massa N, Bonneau L, Marengo E, Wipf D, Berta G and Lingua G (2018) Impact of Beneficial Microorganisms on Strawberry Growth, Fruit Production, Nutritional Quality, and Volatilome. Front. Plant Sci. 9:1611. doi: 10.3389/fpls.2018.01611
Received: 28 June 2018; Accepted: 17 October 2018;
Published: 16 November 2018.
Edited by:
Andrea Genre, Università degli Studi di Torino, ItalyReviewed by:
Erica Lumini, Istituto per la Protezione Sostenibile delle Piante (IPSP), ItalyPhilipp Franken, Leibniz-Institut für Gemüse- und Zierpflanzenbau (IGZ), Germany
Alessandra Durazzo, Consiglio per la ricerca in Agricoltura e l'analisi Dell'economia Agraria (CREA), Italy
Copyright © 2018 Todeschini, AitLahmidi, Mazzucco, Marsano, Gosetti, Robotti, Bona, Massa, Bonneau, Marengo, Wipf, Berta and Lingua. This is an open-access article distributed under the terms of the Creative Commons Attribution License (CC BY). The use, distribution or reproduction in other forums is permitted, provided the original author(s) and the copyright owner(s) are credited and that the original publication in this journal is cited, in accordance with accepted academic practice. No use, distribution or reproduction is permitted which does not comply with these terms.
*Correspondence: Valeria Todeschini, valeria.todeschini@uniupo.it