- 1Department of Horticulture and Landscape Architecture, National Taiwan University, Taipei, Taiwan
- 2Department of Plant Industry, National Pingtung University of Science and Technology, Pingtung, Taiwan
- 3Department of Horticulture, National Chung Hsing University, Taichung, Taiwan
- 4Department of Biology, National Museum of Natural Science, Taichung, Taiwan
- 5Department of Life Sciences, National Chung Hsing University, Taichung, Taiwan
Phalaenopsis is one of the most important potted plants in the ornamental market of the world. Previous reports implied that crassulacean acid metabolism (CAM) orchids at their young seedling stages might perform C3 or weak CAM photosynthetic pathways, but the detailed molecular evidence is still lacking. In this study, we used a key species in white Phalaenopsis breeding line, Phalaenopsis aphrodite subsp. formosana, to study the ontogenetical changes of CAM performance in Phalaenopsis. Based on the investigations of rhythms of day/night CO2 exchange, malate contents and phosphoenolpyruvate carboxylase (PEPC) activities, it is suggested that a progressive shift from C3 to CAM occurred as the protocorms differentiated the first leaf. To understand the role of phosphoenolpyruvate carboxylase kinase (PEPC kinase) in relation to its target PEPC in CAM performance in Phalaenopsis, the expression profiles of the genes encoding PEPC (PPC) and PEPC kinase (PPCK) were measured in different developmental stages. In Phalaenopsis, two PPC isogenes were constitutively expressed over a 24-h cycle similar to the housekeeping genes in all stages, whereas the significant day/night difference in PaPPCK expression corresponds to the day/night fluctuations in PEPC activity and malate level. These results suggest that the PaPPCK gene product is most likely involved in regulation of CAM performance in different developmental stages of Phalaenopsis seedlings.
Introduction
Phalaenopsis, an epiphytic orchid, is regarded as an obligate crassulacean acid metabolism (CAM) plant because of its succulent leaf with large and highly vacuolated mesophyll cells. Previous studies have indicated that mature plants of Phalaenopsis fixed CO2 and stored as malate inside the vacuoles at night (Endo and Ikusima, 1989; Ota et al., 1991; Cui et al., 2004; Guo and Lee, 2006). However, it has been reported that CAM orchids at their protocorm or young seedling stages might perform C3 or weak CAM pathways (Goh et al., 1984). For instance, no significant day/night changes in the titratable acidity were observed during the protocorm development of Dendrobium taurinum (Hew and Khoo, 1980). Chen and Lee (2002) reported that in vitro young seedlings of Phalaenopsis absorbed more CO2 during the light than in the dark. In addition, the in vitro young seedlings of Phalaenopsis exhibited the carbon isotopic values (δ13C) of −21.4‰ to −19.5‰, suggesting a weak CAM photosynthetic pathway (Lo, 2008). These findings imply that an alteration of the photosynthetic pathway from C3 to CAM occurred at the early developmental stage of CAM orchids; however, definite proof has not yet been illustrated.
CAM is a water-preserving photosynthetic pathway adaptive in arid environments, in which stomata are closed during the daytime and opened at night In CAM plants, phosphoenolpyruvate carboxylase (PEPC, EC 4.1.1.31) serves as the key enzyme that fixes CO2 to phosphoenolpyruvate (PEP) in the cytosol during the nighttime (Osmond and Holtum, 1981; Chollet et al., 1996; Vidal and Chollet, 1997; Nimmo, 2000; Borland et al., 2014), and produces oxaloacetic acid (OAA) stored in the vacuole through the formation of malic or citric acids. In the daytime, decarboxylation of these organic acids increases internal CO2 concentration enabling CAM plants to maintain high rates of photosynthesis as the stomata are closed (Winter and Smith, 1996). This feature is an important strategy for the survival of plants in the dry habitat (Silvera et al., 2009; Rodrigues et al., 2013).
Most plants contain several PEPC isoforms encoded by a small gene family that are related to specific physiological functions (Gehrig et al., 1995; Gehrig et al., 2001). In facultative CAM plants, such as the common ice plant (Mesembryanthemum crystallinum) and Kalanchoe species, the CAM-specific PEPC isoform is induced to fulfill the primary carboxylation and carbon flux while switches to CAM pathway (Cushman et al., 1989; Gehrig et al., 1998). The PEPC isoforms, in addition to a CAM-specific isoform, is important for anapleurotic “housekeeping” or tissue-specific functional roles (Gehrig et al., 1998). Changes in PEPC activity are regulated by phosphorylation of a serine residue of PEPC at night that increases the catalytic activity of PEPC, and reduces the inhibitory effect on PEPC of its allosteric inhibitor, malate (Nimmo G. A. et al., 2001; Taybi et al., 2004). The phosphorylation state of PEPC is catalyzed by PEPC kinase, encoded by PPCKs (Chollet et al., 1996; Vidal and Chollet, 1997; Nimmo H. G. et al., 2001). In the common ice plant, the expression of CAM-specific PPC and PPCK are both induced during the induction of CAM (Cushman et al., 1989; Li and Chollet, 1994; Taybi et al., 2000). In Clusia minor, a C3-CAM intermediate species, the increased expression of PEPC kinase plays an interesting role in regulating CAM performance (Borland and Griffiths, 1997). Altogether, examining the relationship between the expression of CAM-specific PPC and PPCK could be a great strategy to illustrate the developmental changes in CAM orchids.
In orchids, protocorms are tuber-shaped structures derived from germinating embryos (Arditti, 1992). The protocorm is a transitional structure, which subsequently produces the shoot and roots, resulting in the formation of a young seedling. If the protocorms or young seedlings of Phalaenopsis perform C3 type metabolism, the developmental stage from which the plants onset the shift from C3 to CAM is of interested. To elucidate the progression from C3 to CAM photosynthetic pathway in Phalaenopsis, we investigated the expression of PPC and PPCK genes, the profiles of day/night CO2 exchange, malate contents and the PEPC activity of different developmental stages. During the past decade, commercial production of Phalaenopsis as potted flowering plants has increased massively in the world (Griesbach, 2000; U.S. Department of Agriculture [USDA], 2017). A better understanding the regulation in CAM performance will provide insights into the micropropagation and cultivation of Phalaenopsis.
Materials and Methods
Plant Materials
The mature plants of Phalaenopsis aphrodite subsp. formosana were maintained in a greenhouse with the pad-and-fan cooling system at National Museum of Natural Science, Taichung, Taiwan. To ensure good pod set and seed quantity, flowers at anthesis were hand-pollinated immediately. Mature seeds were harvested at 150 days after pollination for in vitro cultures. For in vitro seed germination, we used the 1/4-strength macro-elements (i.e., KNO3, NH4NO3, KH2PO4, MgSO4⋅7H2O and CaCl2⋅2H2O) and full-strength micro-elements (i.e., FeSO4⋅7H2O, Na2⋅EDTA, MnSO4⋅4H2O, ZnSO4⋅7H2O, CuSO4⋅5H2O, KI, CoCl2⋅6H2O, H3BO3, NaMoO4⋅2H2O) of Murashige and Skoog (MS) medium (Murashige and Skoog, 1962), supplemented with 10 g⋅L−1 sucrose (Sigma–Aldrich Co., St. Louis, MO, United States), 1 g⋅L−1 peptone (Merck KGaA, Darmstadt, Germany) and 2.2 g⋅L−1 Gelrite (Sigma–Aldrich Co.). The pH value of the media was adjusted to 5.7 with 1 N KOH prior to autoclaving at 121°C and 1.2 kg⋅cm−2 for 15 min. When the first true leaf and root emerged, the developing protocorms were subcultured onto the culture medium containing 1/2-strength macro-elements and full-strength micro-elements of MS medium, supplemented with 10 g⋅L−1 sucrose, 1 g⋅L−1 peptone and 2.2 g⋅L−1 Gelrite. The cultures were placed at the growth chamber at 30 μmol⋅m−2⋅s−1 (daylight fluorescent tube FL-20D/18, 20W, China Electric Co., Taipei) and 26 ± 2°C with a 12-h daylength (0600 to 1800 h). Different developmental stages were shown and described in Figure 1.
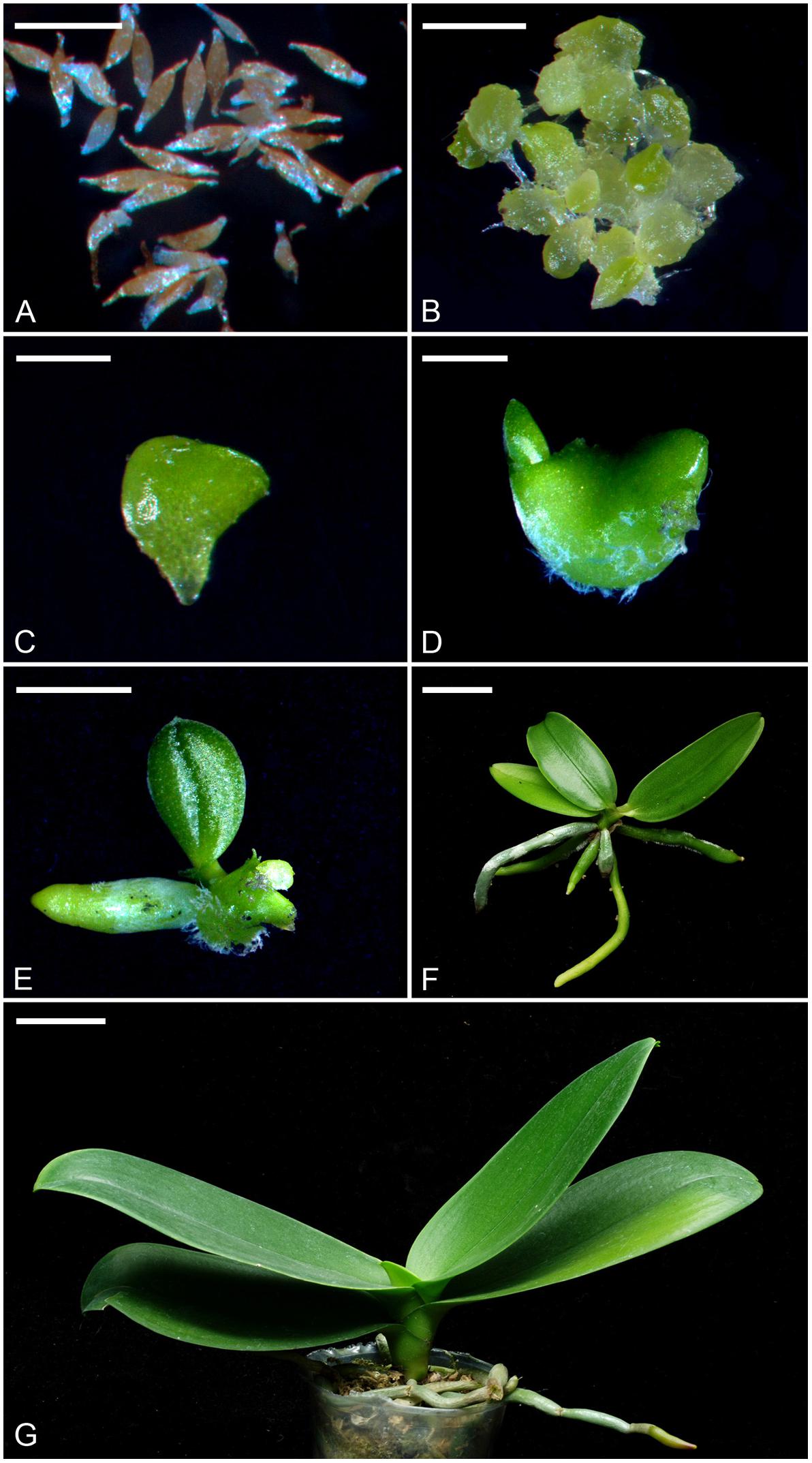
FIGURE 1. The seeds, protocorms and seedlings of P. aphrodite subsp. formosana at different developmental stages (stage 0 to stage 6). (A) Stage 0: mature seeds. Bar = 0.25 mm. (B) Stage 1: the embryo swelled and turned green after 2 weeks of culture. Bar = 1 mm. (C) Stage 2: the protocorm of diameter 1–3 mm could be observed after 4 weeks of culture. Bar = 2 mm. (D) Stage 3: the protocorm with a shoot apical meristem could be observed after 6 weeks of culture. Bar = 4 mm. (E) Stage 4: the protocorm had differentiated the first true leaf and root after 10 weeks of culture. Bar = 1 cm. (F) Stage 5: the seedling at the time for taking out of flasks after 32 weeks of culture. Bar = 2 cm. (G) Stage 6: a 3-year-old mature seedling represented the mature stage that was planted in a 9 cm pot with sphagnum moss. Bar = 4 cm.
Measurement of Diurnal CO2 Exchange
Since developing protocorms (the stages 1–3) were too small to measure the photosynthetic rate using LI-COR’s portable photosynthesis systems, we measured the changes of CO2 concentrations within the culture tubes for representing their photosynthetic profiles. For obtaining the diurnal CO2 change curves in vitro, one gram of protocorms were inoculated onto the culture medium in a culture tube (120 mm tall × 13 mm with 11 mm inside diameter) sealed with the aluminum foil. The cultures were placed in the growth room at 30 μmol⋅m−2⋅s−1 (daylight fluorescent tube FL-20D/18, 20W, China Electric Co., Taipei) and 26 ± 2°C with a 12-h daylength (0600 to 1800 h). The air within the sealed culture tube was collected by a 1-mL syringe every 2 h during a 24-h period, and the CO2 concentration was determined by IR-analyzer (UNOR 610, Maihak AG, Hamburg, Germany). Each data point was measured by the accumulation of CO2 concentration for 2 h within the culture tube. Before the first measurement, the aluminum foil was removed from the culture tube to balance the CO2 concentration from ambient air for 5 min, then the culture tube was sealed with a rubber stopper for 2 h. After each measurement, the rubber stopper was opened to balance the CO2 concentration from ambient air. Three replications were performed in each stage, and each replicate contained the measurement of three tubes. The empty tubes without protocorms were used for CO2 measurements (340 ppm) for calibration.
After the leaf differentiation (stages 4, 5, and 6), gas exchange parameters were measured on the middle part of the top second leaf of a plant by a portable Infra Red Gas Analyzer (LI-COR 6400, LI-COR, Lincoln, NE, United States) with a 2 cm2 leaf chamber every 2 h during a 24-h period. Photosynthetic responses were measured at leaf temperature of 30 ± 2.5°C with 350 μmol⋅mol−1 CO2 supplied at a flow rate of 200 μmol⋅s−1. In the stages of 4 and 5, young seedlings grown in flasks (six seedlings per flask) were take out and the roots were wrapped in the moistened sphagnum moss for measurements. The leaf in the stage 4 was too small to fit a 2 cm2 leaf chamber, and the lead area was estimated by LI-3100C Area Meter for correction of leaf area measurement according to the LI-6400 manual. In the stage 6, 3-year old seedlings grown in the greenhouse were measured. In these experiments, the cultures and seedlings were placed at the growth chamber at 30 μmol⋅m−2⋅s−1 (daylight fluorescent tube FL-20D/18, 20W, China Electric Co., Taipei) with a 12-h daylength (0600 to 1800 h). The sampled leaf was placed in the leaf chamber for 20 min before the data readings were recorded. The environmental conditions in the leaf chambers were controlled as close to those in the growth chamber as possible to reduce the variations between the sampled leaves. In this study, the completely randomized design was used, and the measurements were performed with three replications, and each replication represented the average of six seedlings of each stage.
Measurement of Malate Levels
About 0.2 g tissue from developing protocorms or leaves at each stage was sampled every 2 h during a 24-h period. In stage 5 and 6, the middle part of the top second leaf of a plant was collected for measurement. The samples were ground in a mortar with 5 mL of distilled water. The crude extracts were heated at 90°C water bath for 30 min, then cooled at room temperature. The malate content of the cooled extract was measured according to the method of Möllering and Malt (1974). The concentration of malate was determined using standard curves. For the analysis of titratable acidity, 4 mL of the supernatant was titrated with 0.01 N NaOH solution to the end point of pH value at 8.3. The amount of NaOH was used to calculate the concentration of the titratable acid, expressed as micromoles H+ per gram fresh weight. In this study, the completely randomized design was used, and the measurements were performed with three replications, and each replication represented the average of six seedlings of each stage. The significantly different by t-test between day and night in the same stage were indicated by asterisk (P < 0.05).
Assay of PEPC Activity
PEPC was extracted from developing protocorms and leaves of 0.1 g of each stage were sampled every 2 h during a 24-h period. In stage 5 and 6, the middle part of the top second leaf of a plant was collected for measurement. The sample was homogenized with 1.5 ml extraction buffer (50 mM Tris-HCl, pH 7.0, 10 mM MgCl2, 1 mM EDTA, 5 mM DTT, 2.5% PVPP and 10% glycerol) according to the method of Ku et al. (1999). The homogenate was centrifuged at 17709 × g for 15 min at 4°C. PEPC activity of the supernatant was measured according to Krömer et al. (1996). Protein concentration was determined by using Bio-Rad protein assay reagent (Bradford, 1976). In this study, the completely randomized design was used, and the measurements were performed with three replications, and each replication represented the average of six seedlings of each stage. Significantly differences between day and night in the same stage, as identified by a t-test, were indicated by asterisks (P < 0.05).
Cloning of cDNAs of PaPPCK of P. aphrodite subsp. formosana
Total RNA was extracted from the leaves of seedlings at stage 5 using RNeasy Plant Mini Kit (Qiagen, Hilden, Germany) and modified as Gehrig et al. (2000). For the double-stranded cDNA synthesis, 1 μg RNA was reverse-transcribed using the SuperScriptTM III kit (Invitrogen, Carlsbad, CA, United States) according to the manufacturer’s instruction. The synthesized cDNA fragments and the PaPPCK degenerate primers designed from the conserved amino acid sequences in the expressed sequence tag (EST) library of Phalaenopsis (FC Chen, unpublished) were used in PCR experiments. The amplified fragment containing the partial sequence of PaPPCK that showed high sequence similarity to PPCK was identified by using a blastx algorithm in a BLAST search against the NCBI database (National Center for Biotechnology Information, GenBank). The internal gene-specific primers were designed from the partial sequence of PaPPCK for 5′ and 3′ rapid amplified cDNA ends (RACE) using SMARTTM RACE cDNA amplification kit (Clontech, Palo Alto, CA, United States). The full-length cDNA for PaPPCK was obtained by PCR amplification using the PaPPCK-F and PaPPCK-R primers. All the primers used were listed in Supplementary Table S1.
Real-Time PCR Analysis
Real-time PCR was performed using ABI PRISM 7300 Sequence Detection System (Applied Biosystems, Waltham, MA, United States) with SYBR Green PCR Master Mix (Applied Biosystems) for time-course transcript measurements. Seeds (stage 0), protocorms (stages 1–3) or the top second leaves of seedlings at different stages (stages 4–6) were collected every 2 h for total RNA isolation and cDNA synthesis. The condition of real-time PCR was 95°C for 10 min, then 40 cycles of amplification (95°C for 15 s, 60°C for 1 min, 72°C for 30 s). The real-time PCR experiments were repeated at least three times. The gene-specific primers for real-time PCR for two PPC genes and one PaPPCK gene were designed using the Primer Express 2.0 (Applied Biosystems). The Actin gene of P. aphrodite subsp. formosana was used for normalization. All the primers used were listed in Supplementary Table S1.
Reverse-Transcription PCR Assay
For total RNA isolation and cDNA synthesis, the top second leaf of mature plants of P. aphrodite subsp. formosana were collected every 2 h at the growth chamber at 30 μmol⋅m−2⋅s−1 (daylight fluorescent tube FL-20D/18) with a 12-h daylength (0600 to 1800 h). Primers specific for two PEPC isogenes, i.e., PPC1 (PEQU07008) and PPC2 (PEQU14315) in RT-PCR amplification were designed using Primer3 Input (v. 0.4.0)1. The condition for amplification was one cycle of 94°C for 5 min; 35 cycles of 95°C for 30 s, 55°C for 30 s, and 72°C for 30 s; and a final elongation at 72°C for 5 min. The Actin gene of P. aphrodite subsp. formosana was used as the positive control.
Phylogenetic Analysis
The PaPPCK sequence was analyzed by using a BLASTX algorithm in a BLAST search against the NCBI database to find the closest sequence matches in the database. Multiple alignments of amino acid sequences was performed using the ClustalW program (Thompson et al., 1994) in BioEdit Ver. 7.0.0 (Hall, 1999). Phylogenetic relationships were estimated using MEGA Ver. 4 with Neighbor-joining (NJ) analysis. The scale bar indicates a genetic distance for 0.1 amino acid substitutions per site. Every branch was supported by bootstrap analysis for 1000 replications (values of more than 70% are shown below the branches). Calcium-dependent protein kinase (CDPKs) sequences were used as the outgroup. Accession numbers of genes used for alignment and phylogenetic analyses in this study were listed in Supplementary Table S2.
Results
Photosynthetic Characteristics at Different Developmental Stages
In developing protocorms, higher CO2 concentrations within culture tubes were detected during the dark period than the light period, indicating the lack of CAM expression (Figure 2A). By stage 4, only a small amount of net CO2 assimilation were detected during the light period, but no net CO2 assimilation was detected during the dark period (Figure 2B). The typical net CO2 assimilation rhythm of CAM was first observed by stage 5, which showed a major net CO2 assimilation during the dark period.
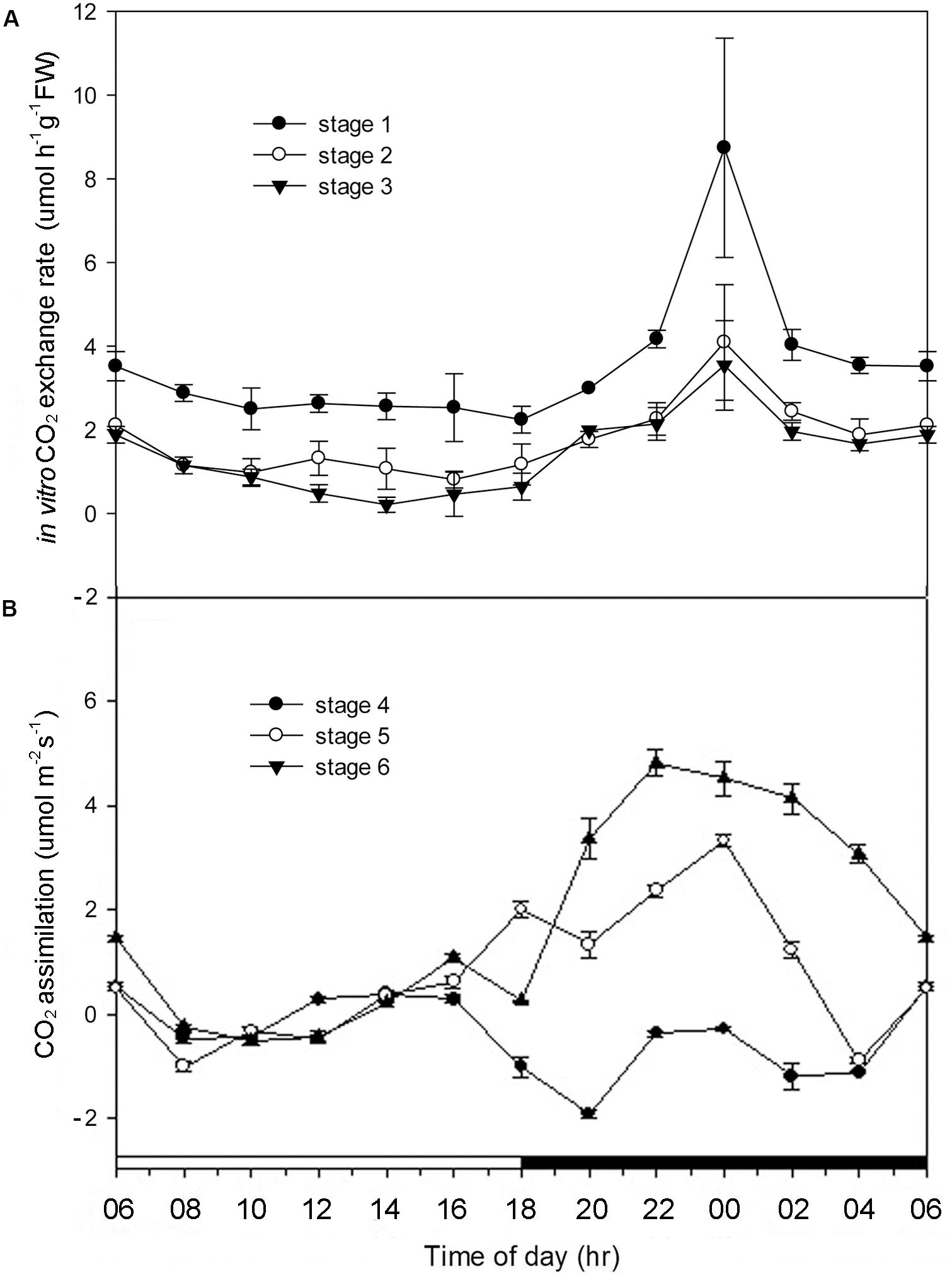
FIGURE 2. Alterations of CO2 exchange rate in Phalaenopsis at different developmental stages. (A) CO2 exchange rate of Phalaenopsis in culture tubes measured by IR-analyzer from stages 1 to 3 during a 24-h period. (B) CO2 assimilation of Phalaenopsis at stages 4–6 measured by LI-COR’s portable photosynthesis system during a 24-h period. Error bars represent standard error (SE) for three independent replications with the 3 mean of three culture tubes. The black bar on the x-axis represent the dark period.
Malate Contents and PEPC Activities at Different Developmental Stages
From stages 0 to 2, no diurnal differences in the malate contents were observed. By stage 3, the noteworthy diurnal fluctuations in the malate contents first appeared, and the diurnal fluctuations were becoming noticeable as the seedlings becoming mature (stages 4–6) (Figure 3A). In developing protocorms and seedlings, the diurnal fluctuations in PEPC activity were similar to those in malate contents (Figure 3B).
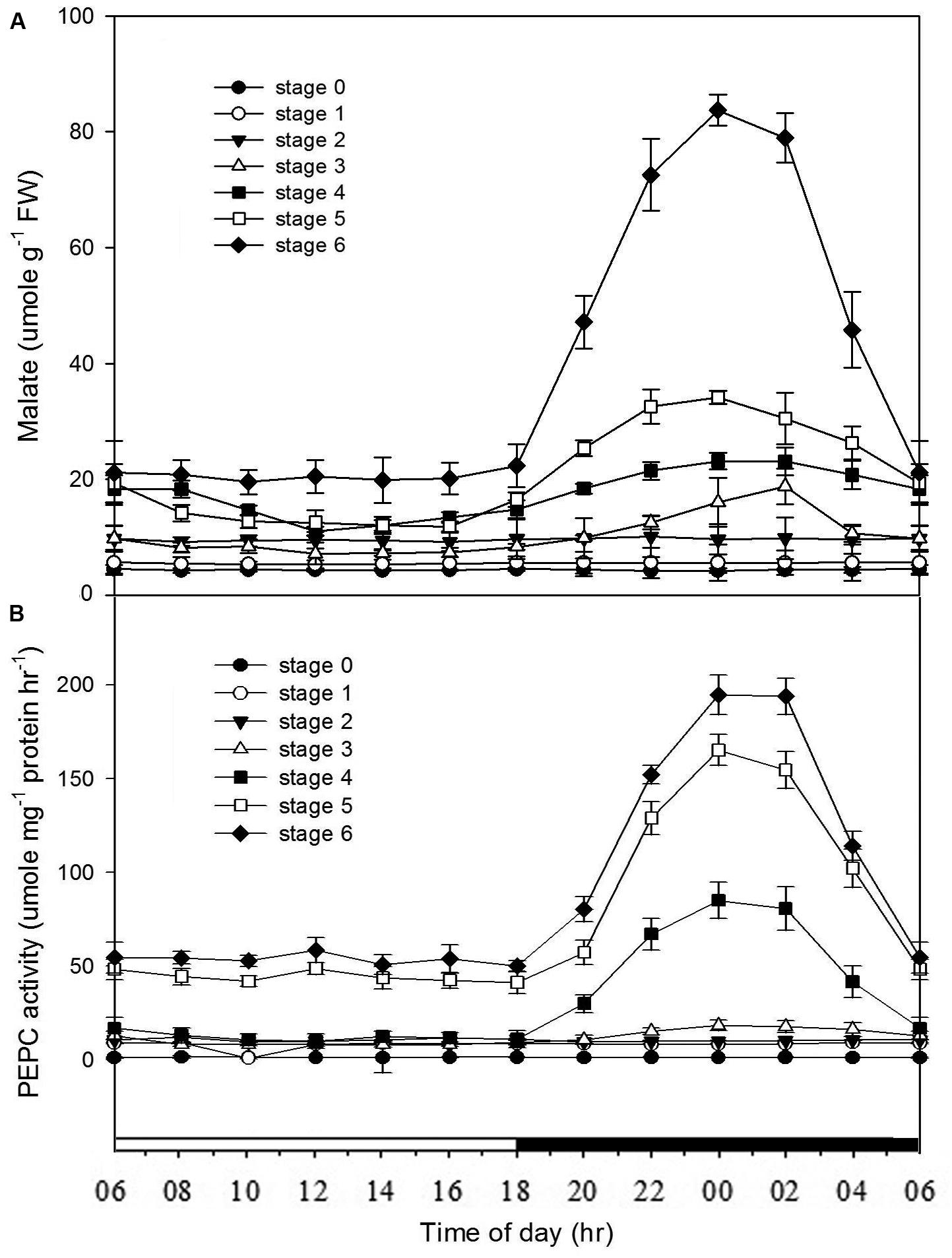
FIGURE 3. Changes of malate contents and PEPC activities in Phalaenopsis at different developmental stages. Day/night changes in (A) malate content and (B) PEPC activity in different developmental stages (stages 0–6). Malate and PEPC activity were detected in the mature seed (stage 0), the enlarged embryo (stage 1), the protocorm (stages 2 and 3) and the different developmental ages of leaf (stages 4–6). Error bars represent standard error (SE) for three independent replications with the mean of six samples.
Characterization of PEPC Isoforms in Phalaenopsis
The whole genome sequence dataset of Phalaenopsis equestris revealed two isoforms of PPC (i.e., PEQU07008 and PEQU14315) occurred in its genome (Cai et al., 2015), while only one copy of PPC (AJ300742) was found in the EST library of P. aphrodite subsp. formosana (previously known as P. amabilis) (Tsai et al., 2013). In this study, molecular phylogenetic analysis indicated that PEQU07008 and AJ300742 were nested in PPC1 clade (the CAM-related), while PEQU14315 was nested in PPC2 clade (the anapleurotic) (Supplementary Figure S1).
Isolation and Characterization of PaPPCK cDNA
Full-length of PaPPCK cDNA was successfully cloned from P. aphrodite subsp. formosana using a combination of RT-PCR and RACE strategies. The GenBank accession numbers of PaPPCK sequence was 2100748. The cDNA contains an open reading frame of 843 bp, flanked by 5′- and 3′-untranslated sequences of 35 and 233 bp, respectively. The cDNA of PaPPCK encodes a protein of 281 amino acids that demonstrates 61∼64%, 61%, and 57% sequence identity to three rice PPCKs, ice plant PPCK, and Kalanchoe fedtschenkoi PPCK, respectively (Supplementary Figure S2). The molecular phylogenetic analysis among PaPPCK and several other PPCK orthologs revealed that PaPPCK clustered with the same group of monocots (Figure 4).
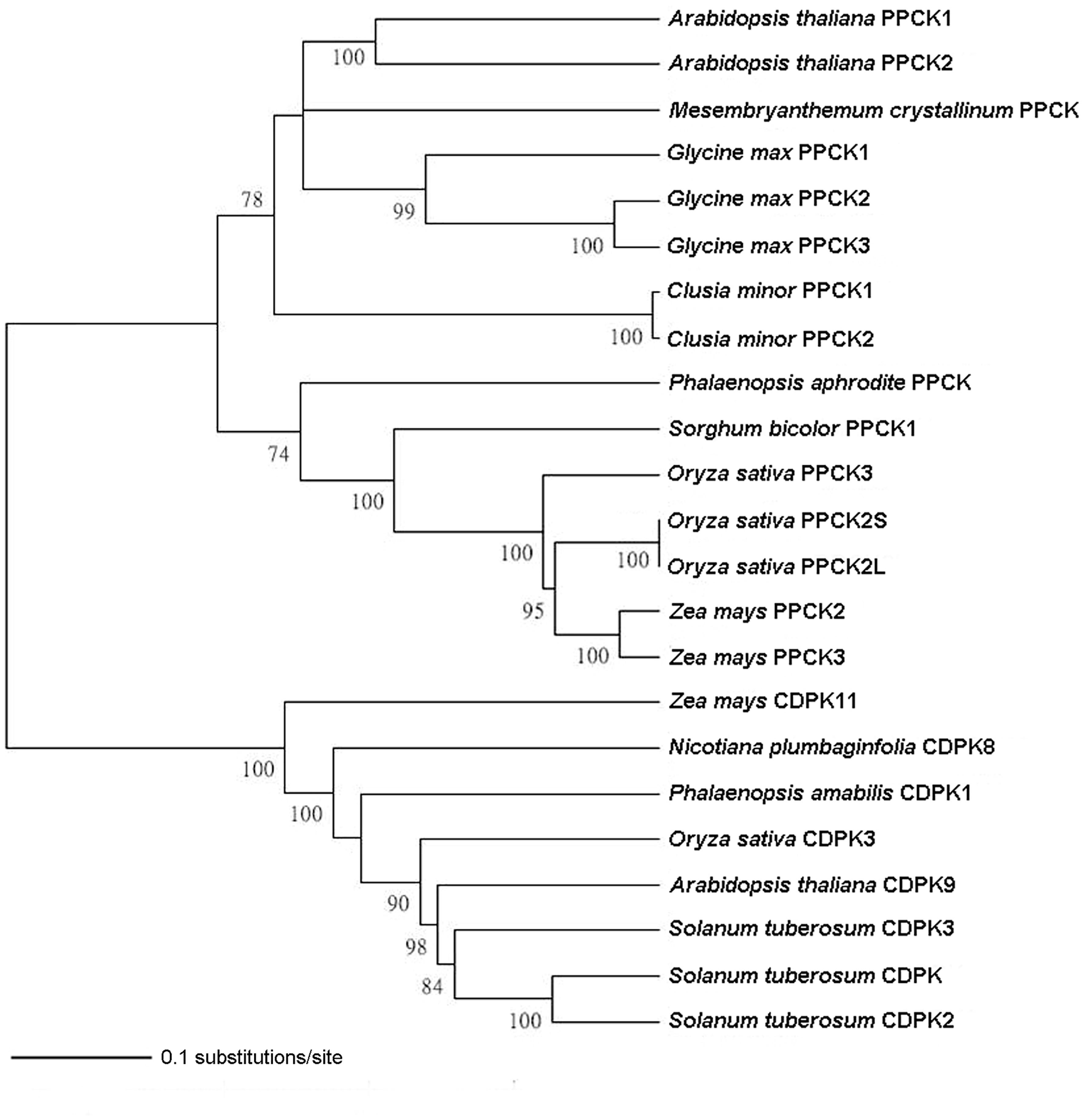
FIGURE 4. Phylogenetic analysis of the PaPPCK protein. The phylogenetic relationship is conducted using Neighbor-Joining with 1000 bootstrap replicates (bootstrap values < 70% are not shown). The scale bar indicates a genetic distance for 0.1 amino acid substitutions per site. Calcium-dependent protein kinase (CDPKs) sequences are used as the outgroup. The accession numbers of genes are given in Supplementary Table S2.
Expression and Abundance of PPC Isogenes and PaPPCK
To gain the further insight into the relationships among the CO2 assimilation, malate content, PEPC activity and gene expression patterns at different developing stages, the mRNA levels of PPC and PaPPCK were analyzed every 2 h over a 24 h period by quantitative real-time PCR analysis (Figure 5). As expressions of PPC isogenes in different developmental stages were measured, no obvious day/night fluctuations was observed, and their expressions were constitutive and rather constant. From stages 4 to 6, the expression pattern of PaPPCK showed a typical diurnal/nocturnal rhythm.
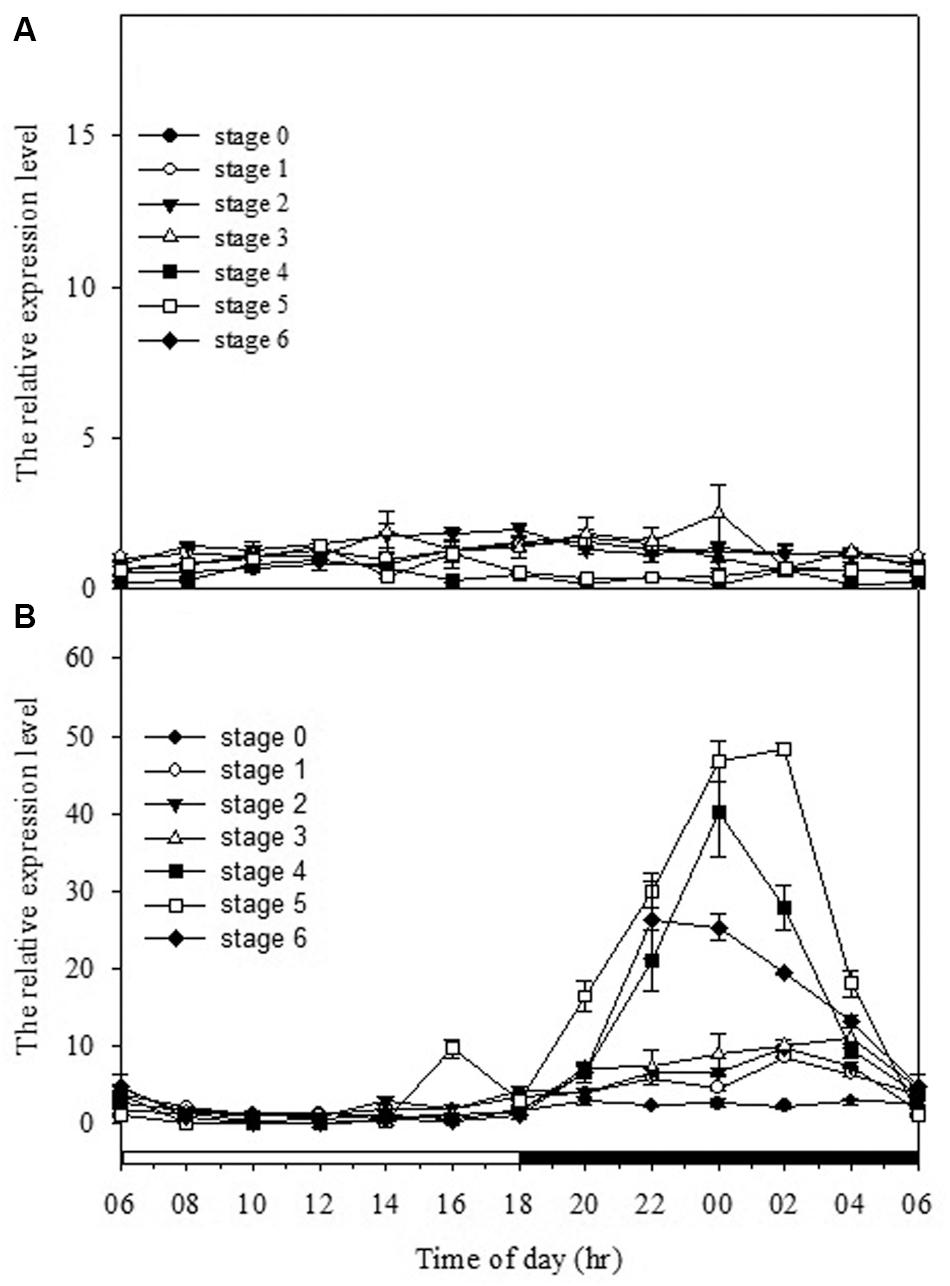
FIGURE 5. Day/night changes in levels of (A) PPC1 (AJ300742) and (B) PaPPCK (2100748) in different developmental stages (stages 0–6). Relative transcript levels are measured by real-time PCR with Actin gene of P. aphrodite subsp. formosana as loading control. Error bars represent standard error (SE) for three independent replications. The black bar on the x-axis represents the dark period.
Discussion
In Phalaenopsis, the nocturnal CO2 uptake was not observed until stage 5 (Figure 2). During stages 1–3, no obvious depletion of CO2 in vitro was detected, and the increase of CO2 concentration within the culture tubes from light to dark periods may reflect the elevated respiration rate of high-energy requirements in developing protocorms (Figure 2A). Differences between day/night malate and PEPC activity was first observed at stage 3 and the breadth of day/night fluctuation enlarged as the seedlings developed (Figure 3). These results implied a progressive shift from C3 to CAM as the protocorms differentiated the first leaf in Phalaenopsis (from stages 3–5). The age-dependent expression of CAM has been reported in a number of plants. In M. crystallinum, CAM performance is modulated ontogenetically by a genetic developmental program, although high salinity could rapidly induce CAM expression in young plants (Cushman et al., 1990; Cushman and Bohnert, 1996). Several CAM species, such as Ananas comosus, Kalanchoe fedtschenkoi, Peperomia, and Clusia species, have demonstrated that a progression from C3 to CAM occurred as the leaves becoming matured (Jones, 1975; Holthe et al., 1987; Zotz and Winter, 1996; Vaasen et al., 2006; Winter et al., 2008). During the transitional phase from C3 to CAM, the photosynthetic features (e.g., no significant nocturnal CO2 uptake but having malate accumulation in the stage 4 seedlings) were similar to CAM-cycling in which plants kept their stomata closed and accumulation organic acids by recapturing the respiratory CO2 during the night (Holthe et al., 1987; Ting et al., 1996). CAM-cycling strategy allows the maintenance of photosynthetic capacity as well as water saving during drought or in the dry season (Harris and Martin, 1991). In Phalaenopsis, upon germination, the embryo first develops into a protocorm before forming a plantlet. Since the differentiation of first root takes about 8 weeks after germination to uptake water efficiently, CAM-cycling may help protocorms to tolerate water deficit.
Previous reports have shown that the performance of CAM could be transcriptionally regulated at the mRNA amounts of PEPC as plants became mature or in response to stress conditions (Cushman et al., 1990; Haasg-Kerwer et al., 1992; Borland et al., 1996). To determine if the ontogenetic difference in CAM performance in Phalaenopsis was reflected by differences in the expression of PPC transcripts, we measured the expression and abundance of two PPC isogenes in different developmental stages. In orchids, molecular phylogenetic analysis of PPC genes indicated that PPCs can be separated into two clades that reflected their different functions, i.e., the CAM-related PPC1 and the anapleurotic function PPC2, respectively (Silvera et al., 2014; Deng et al., 2016). Our previous study (Ping et al., 2010) as well as the results in this study demonstrated that both PPC1 (the CAM-related) and PPC2 (the anapleurotic) expressed in all developmental stage regardless of the time point (Figure 5 and Supplementary Figure S3), suggesting that the day/night rhythm of PEPC activity is not likely controlled at the abundance of both PPC transcripts but another factor.
In addition to the transcriptional control of PEPC activity, the posttranslational control by PEPC kinase also plays an important role in CAM performance (Hartwell et al., 1999; Taybi et al., 2004). The activation of PEPCs in CAM is regulated through a reversible phosphorylation by PEPC kinase (Vidal and Chollet, 1997; Nimmo, 2000, 2003). PEPC kinase is encoded by a small gene family involved in a variety of functions for the precise regulation of PEPC activity (Sullivan et al., 2004; Fukayama et al., 2006; Shenton et al., 2006). In Phalaenopsis, so far only one gene encoding PEPC kinase has been found. In general, PaPPCK increases its expression levels as the seedlings grew up. High levels of PaPPCK transcripts were detected during the dark period and extremely low amounts during the light period, particularly in the plants of stage 3 (Figure 5B). In stage 5, the increased magnitudes of malate accumulation and PEPC activity were not as high as the level of PaPPCK expression (Figures 3, 5). The existence of a protein inhibitor of PEPC kinase may provide an additional layer of control over malate accumulation by PEPC activity (Nimmo G. A. et al., 2001). Indeed, the apparent day/night difference in PaPPCK expression corresponds to day/night fluctuations in PEPC activity and malate levels. In C. minor, a C3-CAM intermediate species, CAM performance is also achieved through the shift in PPCK expression (Borland and Griffiths, 1997). In facultative CAM species, e.g., K. fedtschenkoi and M. crystallinum, the phosphorylation of PEPC is primarily controlled by the abundance of the PPCK transcript, and hence facilitates the nocturnal CO2 uptake (Hartwell et al., 1999; Taybi et al., 2000). It is known that PPCK contains a protein kinase domain without regulatory regions indicated that its activity appears to be controlled primarily at the level of expression (Nimmo H. G. et al., 2001; Nimmo, 2003). In Phalaenopsis, the distinctive expression pattern of PaPPCK leads us propose that PaPPCK likely plays a critical role in regulating the phosphorylation state of PEPC during the night to CAM performance.
Conclusion
Our results demonstrated a progressive shift from C3 to CAM as the protocorms differentiated the first leaf in Phalaenopsis seedlings by measuring the profiles of day/night CO2 exchange, malate contents, PEPC activity and the expression of PPC isogenes and PaPPCK. Notably, in Phalaenopsis seedlings, the day/night fluctuations in PEPC activity and the expression of PaPPCK as well as CO2 uptake was coincident well along the developing stages. Since PPC isogenes were constitutively expressed over a 24-h cycle, PaPPCK may be involved in regulating CAM performance in Phalaenopsis.
Author Contributions
Y-IL conceived the study. Y-IL, F-CC, T-SL, and W-JY designed the study. C-YP, F-CC, and T-CC performed molecular analyses. C-YP performed qPCR and biochemical experiments. C-YP and H-LL performed photosynthesis analyses. C-YP, Y-IL, W-JY, and F-CC wrote the paper. All the authors read and approved the final manuscript.
Conflict of Interest Statement
The authors declare that the research was conducted in the absence of any commercial or financial relationships that could be construed as a potential conflict of interest.
Acknowledgments
This work was supported by the grant [98SB-05.01-ST-01(5)] from the Council of Agriculture, Executive Yuan, ROC to W-JY; from National Museum of Natural Science, Taiwan, to Y-IL.
Supplementary Material
The Supplementary Material for this article can be found online at: https://www.frontiersin.org/articles/10.3389/fpls.2018.01587/full#supplementary-material
Figure S1 | Phylogenetic analysis of PEPC amino acid sequences using Neighbor-joining method described above in the phylogenetic analysis. The other sequences of PEPC obtained from GenBank were added to the analysis by referring to Deng et al. (2016). The PEPC genes from Phalaenopsis are denoted by arrows. Branch numbers indicated bootstrap support (1000 replicates). Bootstrap values < 70% were not shown.
Figure S2 | Alignment of deduced PaPPCK amino acid sequence with PPCKs. The accession numbers of genes were given in Supplementary Table S2. Black and shadow represented identities and similarities respectively. At, Arabidopsis thaliana; Ft, Flaveria trinervia; Kf, Kalanchoë fedtschenkoi; Mc, common ice plant; Os, rice; Pa, Phalaenopsis aphrodite; Sb, Sorghum bicolor; Zm, maize.
Figure S3 | Day/night changes in levels of transcripts for two PEPC genes, i.e., PPC1 (PEQU07008 and AJ300742), PPC2 (PEQU14315), and Actin as control for equal RNA input and RT-PCR conditions in mature leaves of P. aphrodite subsp. formosana.
Table S1 | Primer pairs used in this study.
Table S2 | PPCK and CDPK sequences used for conducting the amino acid alignment and phylogenetic analysis.
Footnotes
References
Borland, A. M., and Griffiths, H. (1997). A comparative study on the regulation of C3 and C4 carboxylation processes in the constitutive crassulacean acid metabolism (CAM) plant Kalanchoë daigremontiana and the C3-CAM intermediate Clusia minor. Planta 201, 368–378. doi: 10.1007/s004250050079
Borland, A. M., Griffiths, H., Maxwell, C., Fordham, M. C., and Broadmeadow, M. S. J. (1996). CAM induction in Clusia minor L. during the transition from wet to dry season in Trinidad: the role of organic acid speciation and decarboxylation. Plant Cell Environ. 19, 655–664. doi: 10.1111/j.1365-3040.1996.tb00400.x
Borland, A. M., Hartwell, J., Weston, D. J., Schlauch, K. A., Tschaplinski, T. J., Tuskan, G. A., et al. (2014). Engineering crassulacean acid metabolism to improve water-use efficiency. Trends Plant Sci. 19, 327–338. doi: 10.1016/j.tplants.2014.01.006
Bradford, M. M. (1976). A rapid and sensitive method for the quantitation of microgram quantities utilizing the principle of protein-dye binding. Anal. Biochem. 72, 248–254. doi: 10.1006/abio.1976.9999
Cai, J., Liu, X., Vannest, K., Proost, S., Tsai, W. C., Liu, K. W., et al. (2015). The genome sequence of the orchid Phalaenopsis equestris. Nat. Genet. 47, 65–72. doi: 10.1038/ng.3149
Chen, Y. I., and Lee, N. (2002). Diurnal rhythm of carbon dioxide, ethylene and organic acid concentration in Phalaenopsis amabilis var. formosana plantlets cultured in vitro. J. Chin. Soc. Hortic. Sci. 48, 157–166.
Chollet, R., Vidal, J., and O’Leary, M. H. (1996). Phosphoenolpyruvate carboxylase: a ubiquitous, highly regulated enzyme in plants. Annu. Rev. Plant Physiol. Mol. Biol. 47, 273–298. doi: 10.1146/annurev.arplant.47.1.273
Cui, Y. Y., Pandey, D. M., Hahn, E. J., and Paek, K. Y. (2004). Effect of drought on physiological aspects of crassulacean acid metabolism in Doritaenopsis. Plant Sci. 167, 1219–1226. doi: 10.1016/j.plantsci.2004.06.011
Cushman, J. C., and Bohnert, H. J. (1996). “Transcription activation of CAM genes during development and environmental stress,” in Crassulacean acid Metabolism: Biochemistry, Ecophysiology and Evolution, eds K. Winter and J. A. C. Smith (New York, NY: Springer-Verlag), 135–158.
Cushman, J. C., Meyer, G., Michalowski, C. B., Schmitt, J. M., and Bohnert, H. J. (1989). Salt stress leads to differential expression of two isogenes of phosphoenolpyruvate carboxylase during crassulacean acid metabolism induction in the common ice plant. Plant Cell 1, 715–725. doi: 10.2307/3868962
Cushman, J. C., Michalowski, C. B., and Bohnert, H. J. (1990). Developmental control of crassulacean acid metabolism inducibility by salt stress in the common ice plant. Plant Physiol. 94, 1137–1142. doi: 10.1104/pp.94.3.1137
Deng, H., Zhang, L. S., Zhang, G. Q., Zheng, B. Q., Liu, Z. J., and Wang, Y. (2016). Evolutionary history of PEPC genes in green plants: implications for the evolution of CAM in orchids. Mol. Phylogenet. Evol. 94, 559–564. doi: 10.1016/j.ympev.2015.10.007
Endo, M., and Ikusima, I. (1989). Diurnal rhythm and characteristics of photosynthesis and respiration in the leaf and root of a Phalaenopsis plant. Plant Cell Physiol. 30, 43–47. doi: 10.1093/oxfordjournals.pcp.a077715
Fukayama, H., Tamai, T., Taniguchi, Y., Sullivan, S., Miyao, M., and Nimmo, H. G. (2006). Characterization and functional analysis of phosphoenolpyruvate carboxylase kinase genes in rice. Plant J. 47, 258–268. doi: 10.1111/j.1365-313x.2006.02779.x
Gehrig, H., Faist, K. V., and Kluge, M. (1998). Identification of phosphoenolpyruvate carboxylase isoforms in leaf, stem and roots of the obligate CAM plant Vanilla planifolia Salib (Orchidaceae): a physiological and molecular approach. Plant Mol. Biol. 38, 1215–1223. doi: 10.1023/a:1006006331011
Gehrig, H., Heute, V., and Kluge, M. (2001). New partial sequences of phosphoenolpyruvate carboxylase as molecular phylogenetic markers. Mol. Phylogenet. Evol. 20, 262–274. doi: 10.1006/mpev.2001.0973
Gehrig, H., Taybi, T., Kluge, M., and Brulfert, J. (1995). Identification of multiple PEPC isogenes in leaves of the facultative crassulacean acid metabolism (CAM) plant Kalanchoë blossfeldiana Poelln. cv. Tom Thumb. FEBS Lett. 377, 399–402. doi: 10.1016/0014-5793(95)01397-0
Gehrig, H., Winter, K., Cushman, J., Borland, A., and Taybi, T. (2000). An improved RNA isolation method for succulent plant species rich in polyphenols and polysaccharides. Plant Mol. Biol. Rep. 18, 369–376. doi: 10.1007/bf02825065
Goh, C. J., Wara-Aswapati, O., and Avadhami, P. N. (1984). Crassulacean acid metabolism in young orchid leaves. New Phytol. 96, 519–526. doi: 10.1111/j.1469-8137.1984.tb03585.x
Griesbach, R. J. (2000). Potted phalaenopsis orchid production: history, present status, and challenges for the future. Horttechnology 10:429.
Guo, W. J., and Lee, N. (2006). Effect of leaf and plant age, and day/night temperature on net CO2 uptake in Phalaenopsis amabilis var. formosa. J. Am. Soc. Hortic. Sci. 131, 320–326.
Haasg-Kerwer, A., Haag-Kerwer, A., Franco, A. C., and Luttge, U. (1992). The effects of temperature and light on gas exchange and acid accumulation in the C3-CAM plant Clusia minor L. J. Exp. Bot. 43, 345–352. doi: 10.1093/jxb/43.3.345
Hall, T. A. (1999). BioEdit: a user-friendly biological sequence alignment editor and analysis program for Windows 95/98/NT. Nucleic Acids Symp. Ser. 41, 95–98.
Harris, F. S., and Martin, C. E. (1991). Correlation between CAM-cycling and photosynthetic gas exchange in five species of Talinum (Portulacaceae). Plant Physiol. 96, 1118–1124. doi: 10.1104/pp.96.4.1118
Hartwell, J., Gill, A., Nimmo, G. A., Wilkins, M. B., Jenkins, G. I., and Nimmo, H. G. (1999). Phosphoenolpyruvate carboxylase kinase is a novel protein kinase regulated at the level of expression. Plant J. 20, 1–10. doi: 10.1046/j.1365-313x.1999.00609.x
Hew, C. S., and Khoo, S. I. (1980). Photosynthesis of young orchid seedlings. New Phytol. 86, 349–357. doi: 10.1111/j.1469-8137.1980.tb01675.x
Holthe, P. A., Sternberg, L. D. S. L., and Ting, I. P. (1987). Developmental control of CAM in Peperomia scandens. Plant Physiol. 84, 743–747. doi: 10.1104/pp.84.3.743
Jones, M. B. (1975). The effect of leaf age on leaf resistance and CO2 exchange of the CAM plant Bryophyllum fedtschenkoi. Planta 123, 91–96. doi: 10.1007/bf00388063
Krömer, S., Gardeström, P., and Samuelsson, G. (1996). Regulation of the supply of cytosolic oxaloacetate for mitochondrial metabolism via phosphoenolpyruvate carboxylase in barley leaf protoplasts. I. The effect of covalent modification on PEPC activity, pH response, and kinetic properties. BBA 1289, 343–350. doi: 10.1016/0304-4165(95)00164-6
Ku, M. S. B., Agarie, S., Nomura, M., Fukayama, H., Tsuchida, H., Ono, K., et al. (1999). High-level expression of maize phosphoenolpyruvate carboxylase in transgenic rice plants. Nat. Biotechnol. 17, 76–80. doi: 10.1038/5256
Li, B., and Chollet, R. (1994). Salt induction and the partial purification/characterization of phosphoenolpyruvate carboxylase protein-serine kinase from an inducible crassulacean-acid-metabolism (CAM) plant, Mesembryanthemum crystallinum L. Arch. Biochem. Biophys. 314, 247–254. doi: 10.1006/abbi.1994.1437
Lo, S. C. (2008). Identification of native Phalaenopsis photosynthetic characteristics. Res. Bull. Taitung District Agric. Improve. Station 18, 15–44.
Möllering, H., and Malt, L. (1974). Methoden der Enzymatischen Analyse, ed. H. U. Bergmeyer (Weinheim: Verlag Chemie Press), 1636–1639.
Murashige, T., and Skoog, F. (1962). A revised medium for rapid growth and bioassays with tobacco tissue cultures. Physiol. Plant. 15, 473–497. doi: 10.1111/j.1399-3054.1962.tb08052.x
Nimmo, G. A., Wilkins, M. B., and Nimmo, H. G. (2001). Partial purification and characterization of a protein inhibitor of phosphoenolpyruvate carboxylase kinase. Planta 213, 250–257. doi: 10.1007/s004250000501
Nimmo, H. G., Fontaine, V., Hartwell, J., Jenkins, G. I., Nimmo, G. A., and Wilkins, M. B. (2001). PEP carboxylase kinase is a novel protein kinase controlled at the level of expression. New Phytol. 151, 91–97. doi: 10.1046/j.1469-8137.2001.00155.x
Nimmo, H. G. (2000). The regulation of phosphoenolpyruvate carboxylase in CAM plants. Trends Plant Sci. 5, 75–80. doi: 10.1016/s1360-1385(99)01543-5
Nimmo, H. G. (2003). Control of the phosphorylation of phosphoenolpyruvate carboxylase in higher plants. Arch. Biochem. Biophys. 414, 189–196. doi: 10.1016/s0003-9861(03)00115-2
Osmond, C. B., and Holtum, J. A. M. (1981). “Crassulacean acid metabolism,” in The Biochemistry of Plants: A Comprehensive Treatise, Vol. 8, eds M. D. Hatch and N. K. Boardman (London: Academic), 283–328.
Ota, K., Morioka, K., and Yamamoto, Y. (1991). Effects of leaf age, inflorescence, temperature, light intensity and moisture conditions on CAM photosynthesis in Phalaenopsis. Engei Gakkai Zasshi 60, 125–132. doi: 10.2503/jjshs.60.125
Ping, C. Y., Lee, Y. I., Lin, T. S., Yang, W. J., and Lee, G. C. (2010). Crassulacean acid metabolism in Phalaenopsis aphrodite var. formosa during different developmental stages. Acta Hortic. 878, 71–77. doi: 10.17660/actahortic.2010.878.7
Rodrigues, M. A., Matiz, A., Cruz, A. B., Matsumura, A. T., Takahashi, C. A., Hamachi, L., et al. (2013). Spatial patterns of photosynthesis in thin- and thick-leaved epiphytic orchids: unravelling C3-CAM plasticity in an organ-compartmented way. Ann. Bot. 112, 17–29. doi: 10.1093/aob/mct090
Shenton, M., Fontaine, V., Hartwell, J., Marsh, J. T., Jenkins, G. I., and Nimmo, H. G. (2006). Distinct patterns of control and expression amongst members of the PEP carboxylase kinase gene family in C4 plants. Plant J. 48, 45–53. doi: 10.1111/j.1365-313x.2006.02850.x
Silvera, K., Santiago, L. S., Cushman, J. C., and Winter, K. (2009). Crassulacean acid metabolism and epiphytism linked to adaptive radiations in the Orchidaceae. Plant Physiol. 149, 1838–1847. doi: 10.1104/pp.108.132555
Silvera, K., Winter, K., Rodriguez, B. L., Albion, R. L., and Cushman, J. C. (2014). Multiple isoforms of phosphoenolpyruvate carboxylase in the Orchidaceae (subtribe Oncidiinae): implications for the evolution of crassulacean acid metabolism. J. Exp. Bot. 65, 3623–3636. doi: 10.1093/jxb/eru234
Sullivan, S., Jenkins, G. I., and Nimmo, H. G. (2004). Roots, cycles and leaves. Expression of the phosphoenolpyruvate carboxylase kinase gene family in soybean. Plant Physiol. 135, 2078–2087. doi: 10.1104/pp.104.042762
Taybi, T., Nimmo, H. G., and Borland, A. M. (2004). Expression of phosphoenolpyruvate carboxylase and phosphoenolpyruvate carboxylase kinase genes. Implications for genotypic capacity and phenotypic plasticity in the expression of crassulacean acid metabolism. Plant Physiol. 135, 587–598. doi: 10.1104/pp.103.036962
Taybi, T., Patil, S., Chollet, R., and Cushman, J. C. (2000). A minimal serine/threonine protein kinase circadianly regulates phosphoenolpyruvate carboxylase activity in CAM induces leaves of the common ice plant. Plant Physiol. 123, 1471–1482. doi: 10.1104/pp.123.4.1471
Thompson, J. D., Higgins, D. G., and Gibson, T. J. (1994). CLUSTAL W: improving the sensitivity of progressive multiple sequence alignments through sequence weighting, position specific gap penalties and weight matrix choice. Nucleic Acids Res. 22, 4673–4680. doi: 10.1093/nar/22.22.4673
Ting, I. P., Patel, A., Kaur, S., Hann, J., and Walling, L. (1996). “Ontogenetic development of crassulacean acid metabolism as modified by water stress in Peperomia,” in Crassulacean acid Metabolism: Biochemistry, Ecophysiology, and Evolution, eds K. Winter and J. A. C. Smith (New York, NY: Springer-Verlag), 204–215.
Tsai, W. C., Fu, C. H., Hsiao, Y. Y., Huang, Y. M., Chen, L. J., Wang, M., et al. (2013). OrchidBase 2.0: comprehensive collection of Orchidaceae floral transcriptomes. Plant Cell Physiol. 54:e7. doi: 10.1093/pcp/pcs187
U.S. Department of Agriculture [USDA] (2017). Floriculture Crops 2016 Summary. Washington, DC: U.S. Dept. Agr.
Vaasen, A., Begerow, D., and Hampp, R. (2006). Phosphoenolpyruvate carboxylase genes in C3, crassulacean acid metabolism (CAM) and C3/CAM intermediate species of the genus Clusia: rapid reversible C3/CAM switches are based on the C3 housekeeping gene. Plant Cell Environ. 29, 2113–2123. doi: 10.1111/j.1365-3040.2006.01583.x
Vidal, J., and Chollet, R. (1997). Regulatory phosphorylation of C4 PEP carboxylase. Trends Plant Sci. 2, 230–237. doi: 10.1016/S1360-1385(97)89548-9
Winter, K., Garcia, M., and Holtum, J. A. M. (2008). On the nature of facultative and constitutive CAM: environmental and developmental control of CAM expression during early growth of Clusia, Kalanchoe, and Opuntia. J. Exp. Bot. 59, 1829–1840. doi: 10.1093/jxb/ern080
Winter, K., and Smith, A. P. C. (1996). Crassulacean Acid Metabolism. Biochemistry, Ecophysiology and Evolution. Heidelberg: Springer-Verlag. doi: 10.1007/978-3-642-79060-7
Keywords: CAM rhythm, orchid, photosynthesis transition, phosphoenolpyruvate carboxylase, phosphoenolpyruvate carboxylase kinase
Citation: Ping C-Y, Chen F-C, Cheng T-C, Lin H-L, Lin T-S, Yang W-J and Lee Y-I (2018) Expression Profiles of Phosphoenolpyruvate Carboxylase and Phosphoenolpyruvate Carboxylase Kinase Genes in Phalaenopsis, Implications for Regulating the Performance of Crassulacean Acid Metabolism. Front. Plant Sci. 9:1587. doi: 10.3389/fpls.2018.01587
Received: 31 January 2018; Accepted: 12 October 2018;
Published: 30 October 2018.
Edited by:
Swee-Suak Ko, Academia Sinica, TaiwanReviewed by:
Daniel Wood, The University of Sheffield, United KingdomWim van Ieperen, Wageningen University & Research, Netherlands
Copyright © 2018 Ping, Chen, Cheng, Lin, Lin, Yang and Lee. This is an open-access article distributed under the terms of the Creative Commons Attribution License (CC BY). The use, distribution or reproduction in other forums is permitted, provided the original author(s) and the copyright owner(s) are credited and that the original publication in this journal is cited, in accordance with accepted academic practice. No use, distribution or reproduction is permitted which does not comply with these terms.
*Correspondence: Wen-Ju Yang, wendy@ntu.edu.tw Yung-I Lee, leeyungi@hotmail.com