- 1Biosciences Division, Oak Ridge National Laboratory, Oak Ridge, TN, United States
- 2Center for Bioenergy Innovation, Oak Ridge National Laboratory, Oak Ridge, TN, United States
- 3Department of Plant Sciences, University of Tennessee, Knoxville, TN, United States
Plant cell walls provide structural support for growth and serve as a barrier for pathogen attack. Plant cell walls are also a source of renewable biomass for conversion to biofuels and bioproducts. Understanding plant cell wall biosynthesis and its regulation is of critical importance for the genetic modification of plant feedstocks for cost-effective biofuels and bioproducts conversion and production. Great progress has been made in identifying enzymes involved in plant cell wall biosynthesis, and in Arabidopsis it is generally recognized that the regulation of genes encoding these enzymes is under a transcriptional regulatory network with coherent feedforward and feedback loops. However, less is known about the transcriptional regulation of plant secondary cell wall (SCW) biosynthesis in woody species despite of its high relevance to biofuels and bioproducts conversion and production. In this article, we synthesize recent progress on the transcriptional regulation of SCW biosynthesis in Arabidopsis and contrast to what is known in woody species. Furthermore, we evaluate progress in related emerging regulatory machineries targeting transcription factors in this complex regulatory network of SCW biosynthesis.
Introduction
Trees are important natural sources of sustainable energy and have important ecological and economical values (Tuskan, 1998; Richmond, 2000; Ragauskas et al., 2014). The majority of biomass of trees resides in the wood of stems, branches and roots. Wood is the major product of secondary growth derived from a lateral meristem, i.e., the vascular cambium, which forms xylem inwards and phloem outwards (Zhang et al., 2015). Prior to forming specialized cell types, cells in xylem and phloem undergo cell expansion and primary cell wall biosynthesis. However, wood is primarily composed of secondary cell walls (SCW) (Sundell et al., 2017). As the most abundant plant biomass worldwide, wood and fibers are widely used for various industrial applications, such as energy, pulping and textiles. In xylem, all the cell type firstly undergo SCW thickening and lignification, after which vessel elements and fibers undergo programmed cell death (PCD) (Courtois-Moreau et al., 2009).
Secondary cell walls, composed of lignin, cellulose and hemicelluloses, play an important role in plant development and stress responses (Houston et al., 2016). The maturation of SCWs reinforces specialized cells such as fibers and vessels, allowing them form mechanical tissues to provide structural support and protection while enabling negative pressure gradients generated during transpiration (Zhong et al., 2010a). The formation of SCW is a complex process requiring coordination of several metabolic pathways. Understanding the regulatory mechanism controlling SCW formation is critical for providing molecular and genetic basis for industrial applications (Zhong et al., 2013).
To date, a regulatory network consisting of several different types of transcription factors (TFs) and controlling SCW formation in the model plant Arabidopsis has been constructed (Zhong et al., 2010a; Taylor-Teeples et al., 2015). Recently, Rao and Dixon (2018) compared the transcriptional regulation models of SCW biosynthesis in grasses and Arabidopsis, and showed that the regulatory network of SCW development in grasses is relatively conserved with divergences. Compared to the annual herbaceous Arabidopsis and grasses, perennial woody species display extreme secondary growth that undergo seasonal changes that are impacted by various environmental stresses. Wood formation in perennial woody species is a dynamic and continuous process, which includes cambial cell proliferation, xylem cell differentiation, SCW thickening and PCD (Zhang et al., 2014). A comprehensive transcriptional regulatory network controlling secondary cell wall formation in woody species is still lacking. This review synthesizes the current advances of SCW regulatory network in plants in general and aims to highlight the recent progresses in this area in woody species. We also discuss the direction for future research in woody species.
The First Layer of Transcription Factors in the Regulatory Network in SCW Formation
NAC (NAM, ATAF, and CUC) TFs are plant-specific transcriptional regulators and are widely involved in various biological processes, including growth/development and stress responses (Olsen et al., 2005). During SCW formation, a group of closely-related NAC TFs function as master switches, which were named SECONDARY WALL NACs (SWNs). In the first layer of the SCW regulatory network, SWNs are comprised of two types of NACs: VASCULAR-RELATED NAC DOMAINS (VNDs; VND1-7) and NAC SECONDARY WALL THICKENING PROMOTING FACTOR (NST)/SECONDARY WALL-ASSOCIATED NAC DOMAIN PROTEIN (SND) (NST1-3) (Figure 1). SWNs can bind to a 19 bp secondary wall NAC binding element (SNBE) sequences, (T/A)NN(C/T)(T/C/G)TNNNNNNNA(A/C)GN(A/C/T)(A/T), and directly activate the expression of downstream TFs in the second layer, as well as structural genes involved in SCW biosynthesis, cell wall modification, and PCD (Zhong et al., 2010c). In addition, a 11 bp tracheary element-regulating cis-element (TERE) [CT(T/C)NAA(A/C)GCN(A/T)] was identified through an in vitro tracheary element (TE) transdifferentiation study and was shown to be essential for TE-specific expression mediated by VNDs (Pyo et al., 2007; Ohashi-Ito et al., 2010; Yamaguchi et al., 2011).
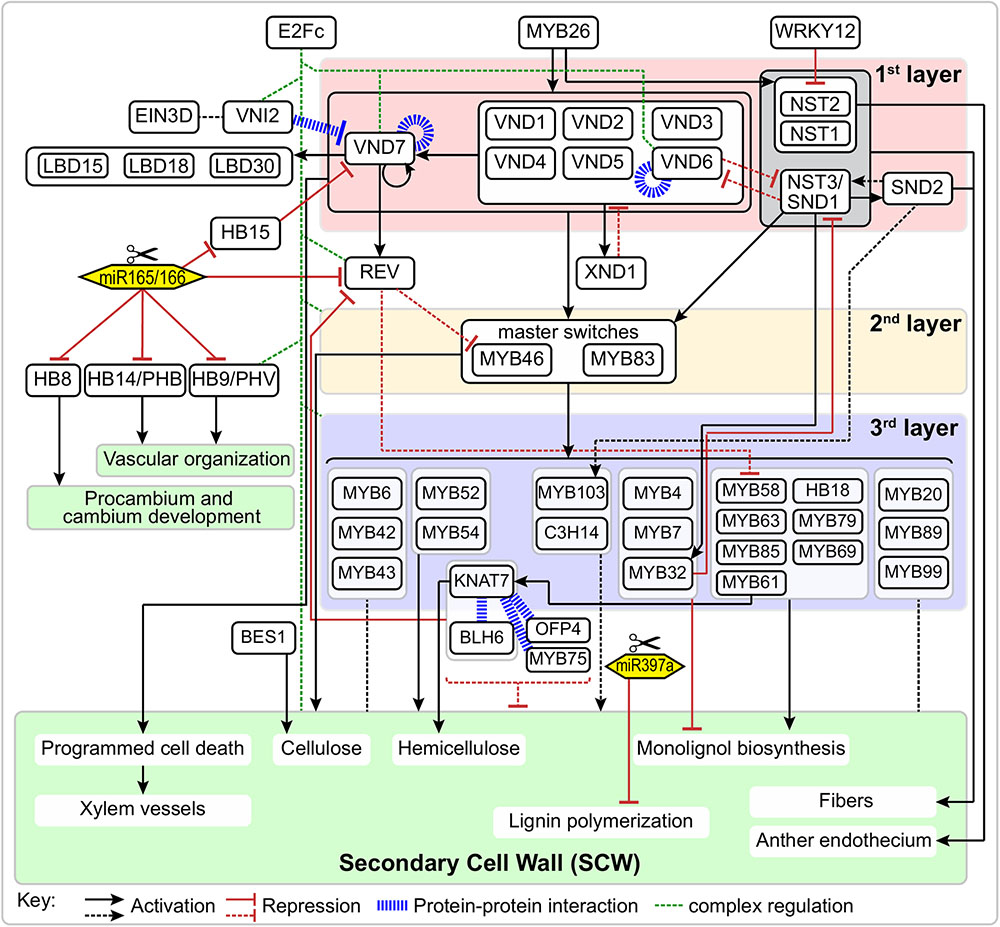
FIGURE 1. Schematic representation of the regulatory network of secondary cell wall formation. Arrows and “T” at the ends of lines represent activation and repression, respectively. Blue bold dash lines represent protein-protein interactions. Solid and dotted lines represent direct or indirect regulation, respectively.
The function of NACs in SCW formation was first reported in Zinnia elegans, in which a NAC TF Z567 was found to be up-regulated during the transdifferentiation from mesophyll cell into TEs in an in vitro culture system (Demura et al., 2002). Subsequently, in Arabidopsis suspension cells, seven homologs of Z567 were shown to be up-regulated during xylem vessel cell differentiation, which were named VND1 through VND7 (Kubo et al., 2005). VNDs individually display specific expression patterns and functions. For example, VND1-5 are expressed in the vessels of stem, but not expressed in interfascicular fibers. Moreover, VND4 and VND5 are expressed in vessels of the root hypocotyl. Overexpressing VND1-5 can activate the expression of TFs and structural genes involved in SCW biosynthesis and PCD (Zhou et al., 2014). VND6 and VND7 are specifically expressed in vessels, directing metaxylem and protoxylem vessel differentiation, respectively (Kubo et al., 2005). Different with the function of VNDs in vessels, NST1 and NST3/SND1 are master regulators of SCW biosynthesis in fibers (Mitsuda et al., 2007). In Arabidopsis nst1-1 nst3-1 double mutant, the SCW thickening was completely suppressed in interfascicular fibers and secondary xylem without affecting the cell formation (Mitsuda and Ohme-Takagi, 2008). Similar to the functional redundancy of NST1 and NST3 in stem, NST1 and NST2 function redundantly in SCW formation in anthers (Mitsuda et al., 2005).
These master regulators have relatively conserved functions across plant species, though copy numbers vary by species. In Medicago truncatula, only one member, MtNST1, was identified corresponding to the three sequence homologs NST1-3 in Arabidopsis. A loss-of-function mutant, mtnst1, results in reduced lignin and cell wall polysaccharide contents through regulating the expression of most lignin biosynthetic and cellulose and hemicellulose biosynthetic genes (Zhao et al., 2010). Oryza sativa secondary wall NAC domain protein 1 (OsSWN1), an ortholog of Arabidopsis NST3/SND1, also regulates SCW formation in rice (Zhong et al., 2011; Chai et al., 2015). In Arabidopsis, ectopic expression of OsSWN1 induced massive ectopic deposition of lignified SCW in leaf mesophyll cells and in the epidermis and cortical cells of the inflorescence stems (Zhong et al., 2011). When OsSWN1 was heterologously expressed driven by the Arabidopsis NST3 promoter in the nst1 nst3 double mutant, the pendent stem phenotype and the SCW lignification of inflorescent fibers were effectively rescued (Zhong et al., 2011), suggesting that OsSWN1 is functionally equivalent to Arabidopsis NST3/SND1. Subsequently, Sakamoto et al. (2016) overexpressed OsSWN1 in poplar using Arabidopsis NST3 promoter. The transgenic poplars displayed thickened SCW in xylem cells and phloem fiber cells but not in xylem vessels. A follow-up study indicated that overexpression of OsSWN1 in Populus altered lignin structure, but not lignin content, due to an unbalance induction of lignin biosynthetic genes (Nuoendagula et al., 2017). This further confirms that the function of the master switches is conserved across different species, whether they are annual or perennial, herbaceous or woody. Consistent with this notion, the similar regulatory pathway is also observed in Zea mays, where ZmNST3 and ZmNST4 were specifically expressed in SCW-forming cells and functioned as master switches for SCW deposition through regulating the expression of ZmMYB109/128/149 (Xiao et al., 2018).
In woody species, similar master switches have also been identified in Populus and Eucalyptus. In Populus, a group of wood-associated NAC domain TFs, PtrWNDs, were identified as master transcriptional switches in SCW biosynthesis. Ohtani et al. (2011) isolated 16 Populus NAC TFs and designated them as PtVNS (VND-, NST/SND- and SMB-related)/PtrWND. Among them, 12 members in NST and VND groups are expressed in developing xylem and phloem fibers, whereas only the VND group members are expressed in primary xylem vessels. A homolog of SND2 from P. trichocarpa, PtSND2, plays a similar role in the SCW biosynthesis. Chimeric repressor of PtSND2 reduced the SCW thickness of xylem fibers and decreased lignin and cellulose contents in Populus (Wang et al., 2013). PtrSND1-A2/PtrWND1B (Potri.001G448400) was shown to be specifically expressed in secondary xylem fiber cells and suppression of PtrWND1B significantly inhibited fiber SCW thickening (Li Q. Z. et al., 2012; Zhao et al., 2014). Moreover, these master regulators function in gymnosperm trees. Pinus pinaster PpNAC1 is a NST group TF, and it is a key regulator of phenylalanine biosynthesis through activating the expression of itself and PpMYB4 (Table 1 and Figure 2) (Pascual et al., 2018). These results suggest that SWNs are ancestral master switches for the SCW formation, and that these master switches are functionally conserved across different plant species, including woody species.
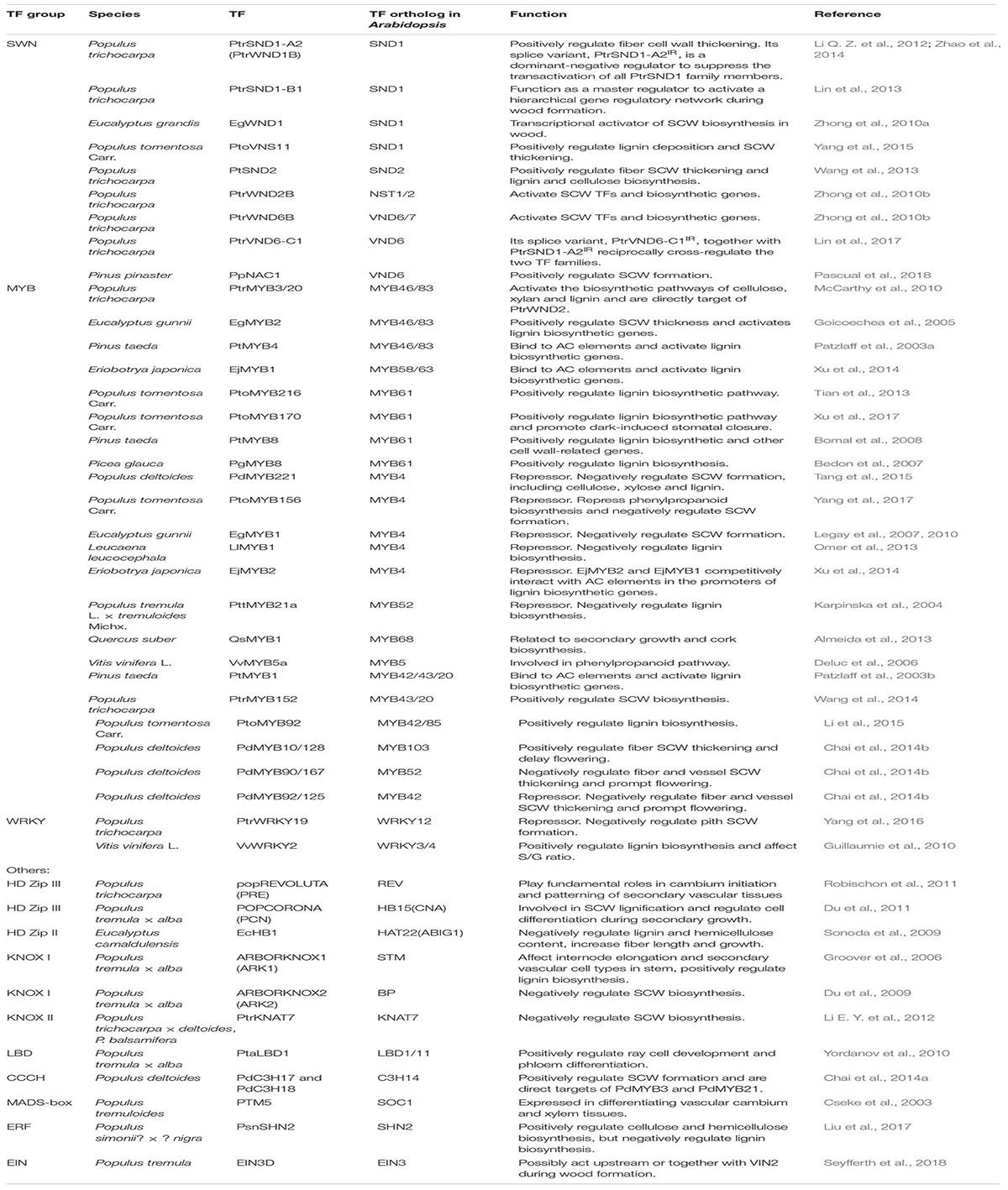
TABLE 1. Summary of the transcription factors involved in secondary cell wall formation in woody species.
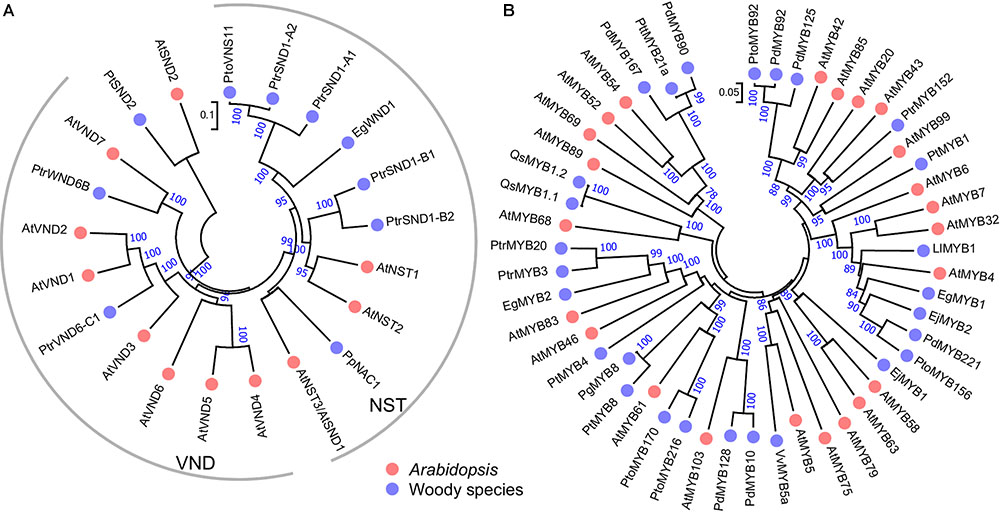
FIGURE 2. Phylogenetic trees of SWNs (A) and MYBs (B) in woody species and Arabidopsis. The unrooted phylogenetic trees were constructed with amino acid sequences of the known SWNs or MYBs in woody species from Table 1 and their homologous in Arabidopsis by the neighbor-joining method with 1000 bootstraps.
Regulators Associated With the First Layer of Transcription Factors
In the first layer of SCW regulatory network, several TFs are involved in regulation or interaction with the master switches (Figure 1). For instance, VND-INTERACTING2 (VNI2) interacts with VND7 and VND1-5. Here, VNI2 functions as a transcriptional repressor to limit the expression of VND7-regulated vessel-specific genes (Yamaguchi et al., 2010). XYLEM NAC DOMAIN1 (XND1) is up-regulated in xylem, and it can negatively regulate xylem vessel differentiation (Zhao et al., 2008). A recent study indicates the function of XND1 in xylem differentiation depends on its C-terminal region containing linear motifs (KII-acidic, LXCXE, E2FTD-like and LXCXE-mimic) which can interact with the cell cycle and differentiation regulator RETINOBLASTOMA-RELATED (RBR) (Zhao et al., 2017). By using enhanced yeast one hybrid assays, Taylor-Teeples et al. (2015) identified E2Fc as a key upstream regulator of VND6, VND7 and other SCW biosynthetic genes. E2Fc is a known negative regulator of endoreduplication (del Pozo et al., 2002, 2006), but it can also act as a transcriptional activator (Kosugi and Ohashi, 2002; Heckmann et al., 2011). Prior to terminal differentiation, the elongating xylem cells likely undergo endoreduplication before SCW deposition via E2Fc-mediated activation or repression of VND7 in a concentration-dependent manner (Taylor-Teeples et al., 2015).
Other regulators associated with master switches in the first layer of SCW regulatory network include Homeobox HD-Zip class III (HD-Zip III), a small TF family that consists of five members in the Arabidopsis genome, i.e., REVOLUTA/INTERFASCICULAR FIBERLESS1 (REV/IFL1), PHABULOSA (PHB), PHAVOLUTA (PHV), HB8, and HB15 (CORONA). The HD-Zip III genes are negatively regulated by highly conserved miRNAs (Floyd et al., 2006) and all five HD-Zip III TFs are necessary for xylem cell specification and SCW synthesis. In Populus, popREVOLUTA (ortholog of REV) plays fundamental roles in the initiation of the cambium and in the regulation of the patterning of secondary vascular tissues (Robischon et al., 2011). The promoters of REV and PHB can be bound and regulated by VND7 (Taylor-Teeples et al., 2015). HB15 is necessary for repressing SCW biosynthesis in pith and disruption of the expression of HB15 causes ectopic lignification in pith cells. An ortholog in Populus, POPCORONA, is involved in SCW lignification and regulates cell differentiation during secondary vascular growth (Du et al., 2011). Noticeably, the expression of WRKY12 is up-regulated in athb15 mutant (Du et al., 2015). As a negative regulator, WRKY12 can directly bind to the NST2 promoter to repress its expression, thus repressing the SCW thickening in pith cells (Wang et al., 2010). Finally, three homologous LOB domain TFs (LBD15, LBD18, and LBD30) are expressed in differentiating TEs and enhance the transcription of VND7 in a positive feedback loop (Soyano et al., 2008; Ohashi-Ito et al., 2018).
In addition to the transcriptional regulation, the post-translational modifications play important roles in regulating the master switches in the first layer of SCW regulatory network. A study using tobacco BY-2 cells expressing VND7-YFP together with the treatment of proteasome inhibitor MG-132 showed that VND7 is also regulated by proteolysis (Yamaguchi et al., 2008). Recently, Kawabe et al. (2018) identified a recessive mutant with inhibited ectopic xylem cell differentiation in 35S::VND7-VP16-GR lines and found this mutant is caused by a single amino acid substitution (E36K) in S-nitrosoglutathione reductase (GSNOR1). GSNOR was first reported as a glutathione-dependent formaldehyde dehydrogenase and regulates the turnover of S-nitrosoglutathione, a natural nitric oxide donor. VND7 can be S-nitrosylated at Cys264 and Cys320, which are located near the transactivation domain. The in vivo S-nitrosylation of VND7 mediated by GSNOR1 affects VND7-downstream signaling events and thereby leading to deficient xylem vessel differentiation (Kawabe et al., 2018). Collectively, these regulators work with the first layer master switches to regulate their transcription or protein activity by providing post-translational modifications. These provide an additional layer of regulation at the top level to regulate SCW biosynthesis, which may possibly involve the integration of developmental or environmental signals since many of these regulators play roles in these processes (Jin et al., 2000; Preston et al., 2004; Romano et al., 2012).
The Second Layer of Transcription Factors in the Regulatory Network in SCW Formation
A series of additional TFs make up the second layer of regulation of the expression of SCW biosynthetic genes and other downstream genes. The master switches in the second layer are MYB46 and MYB83 (Figure 1), which are directly regulated by SND1 and its close homologs (NST1, NST2, VND6 and VND7) (Zhong et al., 2007; McCarthy et al., 2009). MYB46 and MYB83 are functional redundant and are specifically expressed in fibers and vessels where SCW thickening occurs. Overexpression of MYB46 or MYB83 enhanced the biosynthetic pathways of lignin, cellulose and xylan, and resulted in ectopic deposition of SCW; whereas RNAi or dominant repression of MYB46 and MYB83 reduced SCW thickening of fibers and vessels (Zhong et al., 2007; McCarthy et al., 2009).
MYB46 and MYB83 can regulate other SCW-related TFs or directly regulate the SCW structural genes. Based on results from the estrogen-inducible direct activation system, several downstream TFs, including MYB43, MYB52, MYB54, MYB58, MYB63 and KANT7, have been identified as direct targets of MYB46/83. A 7-bp sequence ACC(A/T)A(A/C)(T/C) has been designated as the secondary wall MYB-responsive element (SMRE) (Zhong and Ye, 2012), similar to binding sequences of AC element [ACC(T/A)ACC] (Fornale et al., 2010) and P1 [CC(T/A)ACC] (Grotewold et al., 1994). Another 8-bp sequence [(T/C)ACC(A/T)A(A/C)(T/C)] has also been identified as MYB46 specific binding sequence, namely MYB46-responsive cis-regulatory element (M46RE) (Kim et al., 2012; Ko et al., 2014). In addition, MYB46/83 can directly regulate SCW structural genes. For example, MYB46 directly regulates all three SCW-associated cellulose synthase genes (CesA4, CesA7 and CesA8) (Kim et al., 2013) and a mannan synthase CSLA9 (Kim et al., 2014b). Noticeably, the promoters of these genes contain multiple M46REs. A genome-wide screen of promoter sequences indicates the xylan biosynthetic genes (IRX8, IRX9, IRX10, IRX14, IRX15 and IRX15-L) (Jensen et al., 2011; Kim et al., 2014a), lignin biosynthesis-related laccase (LAC4/IRX12, LAC10 and LAC11) (Zhao et al., 2013), cytoskeleton-related genes (Myosin5, microtubule-associated protein), and homologous of IRX15/15-L (DUF579s) also contain multiple M46REs in their promoter regions (Jensen et al., 2011).
Similar to the master switches in the first layer of SCW regulatory network, the function of MYB46 and MYB83 is also highly conserved in woody species. For instance, PtrMYB3 and PtrMYB20 from Populus, EgMYB2 from Eucalyptus, and PtMYB4 from Pinus taeda, are orthologs of MYB46/83 and perform the same function as MYB46/83 from Arabidopsis in SCW biosynthesis (Figure 2). In Populus developing wood, PtrMYB3 and PtrMYB20 are highly expressed in vessels and fibers and can regulate the biosynthesis of lignin, cellulose and xylan (McCarthy et al., 2010). Eucalyptus EgMYB2 is identified based on a quantitative trait locus (QTL) for lignin content. EgMYB2 can specifically bind to the promoters of lignin biosynthetic genes, such as CINNAMOYL-COENZYME A REDUCTASE (CCR) and CINNAMYL ALCOHOL DEHYDROGENASE (CAD). Overexpression of EgMYB2 enhanced SCW thickness in transgenic tobacco (Goicoechea et al., 2005). In loblolly pine, Pinus taeda MYB4 (PtMYB4), the homolog of Arabidopsis MYB46/83, is expressed in lignificating xylem cells. PtMYB4 can bind to AC elements and activate the expression of target genes (Patzlaff et al., 2003a). Collectively, these results suggest that the orthologs of MYB46/83 function conservatively as the second layer master regulators in SCW biosynthesis in woody plants.
The Third Layer of Regulatory Network in SCW Formation
In addition to the master switches in the second layer of SCW regulatory network, there are TFs that regulate SCW biosynthesis, whose expression are regulated by the master switches MYB46/83, and act as downstream TFs in the third layer of SCW regulatory network (Figure 1). Most of these TFs belong to the MYB gene family. The first identified lignin-specific TFs were MYB58, MYB63, and MYB85 (Zhong et al., 2008; Zhou et al., 2009). Most monolignol biosynthetic genes contain AC elements in their promoter region and are direct target of MYB58 (Zhou et al., 2009). Moreover, MYB6, MYB20, MYB42, MYB43, MYB52, MYB54, MYB61, MYB103, etc. are also developmentally associated with cells undergoing SCW thickening (Zhong et al., 2008; Romano et al., 2012). MYB52, MYB54, MYB85 and MYB103 are able to induce SCW biosynthetic genes. Overexpression of MYB85 led to ectopic lignin deposition in epidermal and cortical cells; overexpression of MYB103 increased SCW thickening in fibers; whereas dominant repression of MYB52, MYB54, MYB85, or MYB103 reduced SCW thickening in fiber cells (Zhong et al., 2008). In contrast, MYB61 plays multiple regulatory roles in plant development, including lignification, dark-photomorphogenesis (Newman et al., 2004), stomatal aperture (Liang et al., 2005) and seed coat mucilage deposition (Penfield et al., 2001). Analysis of loss-of-function mutant of MYB61, atmyb61, showed that MYB61 can activate the expression of CAFFEOYL-COA 3-O-METHYLTRANSFERASE (CCoAOMT) and PECTIN METHYLESTERASE (PME) and affect xylem formation and xylem cell structure (Romano et al., 2012).
While most of these TFs activate the expression of their targets and positively regulate SCW biosynthesis, several members in MYB family play negative roles in SCW biosynthesis. Arabidopsis MYB4 is induced by UV-B. Overexpression of MYB4 can repress the transcription of 4CL, C4H and CAD in tobacco (Jin et al., 2000). MYB7 and MYB32 share high sequence similarity with MYB4, act as repressors, and are strongly activated by MYB46 (Ko et al., 2009). MYB32 negatively regulates lignin pathway through repressing other targets, such as COMT (Preston et al., 2004). In addition, there is a feedback regulation between MYB32 and SWNs. The transcription of MYB32 is repressed in the nst1 nst3 double mutant (Mitsuda et al., 2007). A later study based on in vitro trans-activation assays and electrophoretic mobility shift assay (EMSA) further confirmed that MYB32 is directly regulated by SND1 (Wang et al., 2011). Furthermore, SND1 is negatively regulated by MYB32 (Wang et al., 2011), implying that both positive and negative feedforward loop exist in SCW regulatory network.
Populus PttMYB21a, a homolog of MYB52 (Figure 2), can negatively regulate the expression of CCoAOMT and the acid soluble lignin content (Karpinska et al., 2004). In grapevine, VvMYB5a can regulate both anthocyanin/proanthocyanidin biosynthesis and lignin biosynthesis (Deluc et al., 2006). In Eucalyptus gunnii, EgMYB1 binds to the promoter of CCR and CAD to repress the monolignol biosynthesis (Legay et al., 2007). In Pinus taeda, PtMYB1, closely related to Arabidopsis MYB42, MYB43 and MYB20, is most abundantly expressed in differentiating xylem and functions as a transcriptional activator through binding the AC elements (Patzlaff et al., 2003b). Loquat (Eriobotrya japonica) EjMYB1 (ortholog of MYB58 and MYB63) functions as transcriptional activator and can activate both Arabidopsis and loquat lignin biosynthetic genes. EjMYB2 (ortholog of MYB4) functions as a repressor and can counter the induction by EjMYB1 (Xu et al., 2014). The large abundance of TFs in the third layer provide multiple opportunities to connect to the master switches in the first and second layers and the structural genes in SCW biosynthesis, and to fine tuning the pathways.
Regulators Associated With the Third Layer of Transcription Factors
Several genes in other TF families cooperate with MYBs or act independently to regulate the SCW biosynthesis (Figure 1). KNOTTED ARABIDOPSIS THALIANA7 (KNAT7) is a Knotted-like homeobox (KNOX) protein, is a target of MYB46 (Ko et al., 2009) and SND1 (Zhong et al., 2008), and can also be regulated by MYB61 (Romano et al., 2012). Dominant repression of KNAT7 reduced SCW thickening in vessels and fibers (Zhong et al., 2008). In Nicotiana, virus-induced silence and RNAi of NbKNAT7 inhibited the thickening of fiber cell walls and repressed the expression of lignin, cellulose and xylan biosynthetic genes (Pandey et al., 2016). KNAT7 was known as a transcriptional repressor that negatively regulates SCW biosynthesis, and it can physically interact with MYB75, OFP4 and BLH6 (Li et al., 2011; Li E. Y. et al., 2012; Bhargava et al., 2013; Liu et al., 2014). Arabidopsis MYB75 positively regulates anthocyanin biosynthesis, but it functions as a repressor in SCW biosynthesis. A loss-of-function mutant myb75-1 enhanced the expression of lignin, cellulose and xylan biosynthetic genes and increased SCW thickness in xylary and interfascicular fibers (Bhargava et al., 2010). In Arabidopsis, the KNAT7-MYB75 complex is involved in modulating SCW formation in both inflorescence stem and seed coat (Bhargava et al., 2013). OFP4 is an Ovate Family Protein transcriptional co-regulator and can interact with KNAT7 and enhance the repression activity of KNAT7 in SCW biosynthesis (Li et al., 2011). BLH6 is a BELL1-LIKE HOMEODOMAIN protein and functions as a transcriptional repressor. It specifically interacts with KNAT7 to enhance its repression activity. BLH6 and KNAT7 can repress the expression of REV through directly binding to its promoter (Liu et al., 2014).
In Populus, KNAT7 functions as a repressor in a negative feedback loop in SCW formation (Li E. Y. et al., 2012). However, a recent study indicates that KNAT7 positively regulates xylan biosynthesis through directly activating the expression of IRX9 (He et al., 2018). Another member in KNOX family, BERVIPEDICELLUS (BP)/KNAT1, also plays a role in the lignin pathway. BP binds to the promoters of genes in the lignin pathway (COMT, CCoAOMT, etc.) and overexpressing BP significantly decreases the SCW lignification (Mele et al., 2003). In addition, the tandem CCCH zinc finger (TZF) TF, C3H14, is able to activate SCW biosynthetic genes and is directly regulated by MYB46 and SND1 (Ko et al., 2009). Its orthologs in Populus deltoides, PdC3H17 and PdC3H18, also positively regulate SCW formation in both Populus and Arabidopsis, and are direct targets of PdMYB3 and PdMYB21 (Chai et al., 2014a). These regulators associated with the third layer of transcription factors provide opportunities for fine tuning SCW biosynthesis at the very downstream level.
Ethylene Related Tfs in SCW Biosynthesis
Recently, a class of ethylene signaling-related TFs have attracted the attention of researchers due to their function in wood development (Figure 1). Ethylene is the smallest phytohormone with the simple structure C2H4, and is involved in various plant developmental processes including leaf development, senescence, fruit ripening, germination, stress responses, etc. (Dubois et al., 2018). Notably, ethylene is also involved in multiple process during wood formation, including cambial growth, xylem cell morphogenesis, and vessels/fibers/rays ontogenesis (Little and Savidge, 1987). In angiosperm trees, ethylene, as an important signaling molecule, is involved in the remodeling of wood formation upon tension wood induction. Exogenous application of ethylene or its precursor 1-aminocyclopropanel-1-carboxylic acid (ACC) enhances xylem growth in hybrid aspen (Populus tremula × P. tremuloides) (Love et al., 2009). In addition, gene expression and enzyme activity of ACC oxidase are up-regulated on the tension wood surface (Andersson-Gunneras et al., 2003).
The ethylene perception and signal transduction cascades depend on ethylene-induced Ethylene Response Factors (ERFs). In Arabidopsis, ERF1, ERF018 and ERF109 are involved in the vascular cell division (Etchells et al., 2012), suggesting that ERFs-mediated ethylene signaling is important for vascular development. Vahala et al. (2013) performed a genome-wide screen for ERFs in hybrid aspen stem. Among 170 ERFs in Populus, 50 ERFs were induced greater than five-fold by ethylene. During tension wood formation, 17 and 8 ERFs were induced greater than two-fold and ten-fold, respectively. Subsequently, the function of these ERFs was further confirmed in transgenic Populus (Vahala et al., 2013). Overexpression of ERF18, ERF21, ERF30, ERF85 and ERF139 in wood-forming tissues modified the wood chemotype in hybrid aspen. Overexpression of ERF139 repressed longitudinal and lateral growth with altered wood development, overexpression of ERF18, ERF34, and ERF105 enhanced diameter growth, whereas overexpression of ERF71 and ERF85 suppressed diameter growth.
Despite this work the role of ERFs-mediated ethylene signaling in the SCW regulatory network remains elusive (Figure 1). Liu et al. (2017) reported that Populus ERF type TF, PsnSHN2, is predominantly expressed in xylem tissues, and that it positively regulates cellulose and hemicellulose biosynthesis but negatively regulates lignin biosynthesis. Recently, Seyfferth et al. (2018) constructed an ethylene-related gene expression network during SCW formation, ETHYLENE INSENSITIVE 3D (EIN3D) and 11 ERFs were identified as hub genes. Interestingly, a VNI2 homolog is highly associated with EIN3D, suggesting EIN3D may act upstream or together with VIN2 during SCW formation. How to precisely position these unresolved TFs into the SCW transcriptional regulatory network deserves further investigation.
Post-Transcriptional Regulation of Tfs Involved in SCW Formation
The activity of transcriptional regulators and the gene expression are also affected by post-transcriptional regulation. Alternative splicing is an important model of post-transcriptional regulation. It plays important roles for enhancing proteomic diversity in diverse cellular processes (Chen and Manley, 2009). In plants, more than 60% of intron-containing genes undergo alternative splicing (Syed et al., 2012). However, the knowledge of alternative splicing in wood formation is limited. By analyzing the xylem transcriptome in 20 P. trichocarpa genotypes, Bao et al. (2013) found that about 36% of the genes expressed in xylem undergo alternative splicing, especially those cell wall biosynthetic genes including glycosyl transferases and C2H2 TFs.
Interestingly, most key TFs in the first layer of SCW regulatory network undergo alternative splicing. In Populus, a “stem-differentiating xylem”-specific variant of SND1, PtrSND1-A2IR, was identified as a dominant-negative regulator of SND1-mediated pathway (Li Q. Z. et al., 2012). The retained intron 2 in PtrSND1-A2IR cDNA introduces a premature stop codon resulting in a truncated protein lacking the activation domain. Hence PtrSND1-A2IR loses DNA binding and transactivation abilities, and it represses the transcription of PtrSND1 members and PtrMYB021 via its retained dimerization capability. This is the first report on the auto-repression of a TF family by its own splice variant in plants. Subsequently, Zhao et al. (2014) compared the function of the two isoforms, PtrSND1-A2 (also named PtrWND1B-s) and PtrSND1-A2IR (also named PtrWND1B-l), during wood formation. Overexpression of PtrWND1B-s or PtrWND1B-l oppositely regulate fiber SCW thickening in Populus. This alternative splicing type was also detected in SND1 ortholog in Eucalyptus grandis (Eucgr.E01053), but not in Arabidopsis, implying that the alternative splicing regulation of SND1 may be different between woody species and herbaceous plants (Li Q. Z. et al., 2012; Zhao et al., 2014). Recently, Lin et al. (2017) reported that another key TF in the first layer of SCW regulatory network, VND6, also undergoes alternative splicing during wood formation. Its splice variant retained intron 2, PtrVND6-C1IR, which suppresses the protein function of all PtrVND6 and PtrSND1 family members, including PtrSND1-A2. In addition, PtrVND6-C1 can also be suppressed by PtrSND1-A2IR. PtrVND6-C1IR and PtrSND1-A2IR function together for reciprocal cross-regulation of VND and SND members to maintain homeostasis for xylem differentiation and plant development. Whether other key TFs in SCW regulatory network also undergo alternative splicing is still an open question. This intron-retained splice variant-introduced reciprocal cross-regulation provides an additional insight for studying the regulation mechanism of SCW formation and appears to be woody species-specific.
In addition to alternative splicing, the TFs and structural genes in SCW regulatory network are regulated by non-coding RNAs (ncRNAs). In the past few decades, ncRNAs have been shown to play key regulatory roles in various biological processes of development and stress response (Mallory and Vaucheret, 2006; Wierzbicki, 2012). Plant ncRNAs can be classified into various types according to their molecular structures, including microRNA (miRNA), small interfering RNA (siRNA), long ncRNA (lncRNA), circular RNA (circRNA), etc. (Sunkar et al., 2007; Kim and Sung, 2012). Here, we focus on the role of miRNA and lncRNA in SCW formation, in particular on their regulation of SCW-related TFs. Lu et al. (2005) identified 21 miRNA families from the developing xylem of P. trichocarpa stems. Among them, 11 miRNA families have conserved sequences in Arabidopsis but exhibit species-specific developmental expression patterns, while 10 Populus-specific miRNA families might be involved in tree-specific processes. Several members in miRNA families have been reported to play important roles in SCW formation. miRNA165/166 are known to target HD-Zip III TFs, and control xylem differentiation through modulating the PHB gradients in the stele to maintain PHB at a low dosage in protoxylem and a high dosage in metaxylem differentiation (Carlsbecker et al., 2010; Miyashima et al., 2011). In hybrid aspen (Populus tremula × P. alba), Pta-miRNA166 targets PtaHB1, a homolog of REV, to regulate secondary growth (Ko et al., 2006). In a gain-of-function Arabidopsis MIR166a mutant, the transcript level of HB15 was decreased and xylem and interfascicular region were expanded in vascular tissue (Kim et al., 2005). In Populus, synthetic miRNA knock-down of POPCORONA (PCN), an ortholog of HB15, disturbed the lignification of pith cells, whereas overexpression of a miRNA-resistant PCN delayed the lignification of xylem and phloem fibers (Du et al., 2011). Laccases (LAC) belong to the blue copper oxidase family and polymerize monolignols into lignin. Among the 49 LAC genes in the Populus genome, 29 were predicted as the targets of ptr-miRNA397a. Overexpression of Ptr-MIRNA397a reduced lignin content without changing monolignol biosynthesis in Populus (Lu et al., 2013). Recently, another miRNA, miRNA319, was also shown to be able to target TCP4 and decrease the SCW formation in Arabidopsis stem. TCP4 TF can directly activate the expression of VND7 via binding to its promoter (Sun et al., 2017). lncRNAs are also involved in wood formation. Chen et al. (2015) performed a genome-wide analysis and compared the expression profiles of lncRNA in the xylem of normal wood, opposite wood and tension wood in Populus tomentosa. A total of 16 genes in cellulose or lignin biosynthetic pathways were targeted by lncRNAs. Combining whole-genome resequencing with growth and wood-property traits of 435 P. tomentosa individuals, Zhou et al. (2017) further identified 8 lncRNAs and 15 potential target genes in the phenylpropanoid pathway. These diversified post-transcriptional regulatory mechanisms offer new perspectives to the SCW regulatory network through modifying gene expression or protein diversity of the key TFs.
Conclusion
In this review, we provide a summary of current knowledge of the transcriptional regulation of SCW biosynthesis in woody species and contrast to what is known in other plant species, particularly in the model plant Arabidopsis. Woody species and the herbaceous model plant Arabidopsis share conserved master switches in the SCW transcriptional regulatory network, especially in the first and second layers of the network. However, the large abundance of TFs in the third layer and diversified post-transcriptional regulatory mechanisms make the SCW regulatory network more complex in woody plants. For example, the alternative splicing events of SND and VND genes appeared to be woody species-specific. This poses more challenges for fully revealing the SCW regulatory mechanism in woody species. Recent advances in high-throughput sequencing provide great potentials for improving the genome annotation and identifying alternative splicing events and lncRNAs during SCW formation. In addition, expression quantitative trait loci (eQTL) analysis provides an effective and efficient way to identify novel regulators, especially in tree species with long life cycle. Recently, Zhang et al. (2018) identified a Populus hydroxycinnamoyl-CoA:shikimate hydroxycinnamoyl transferase PtHCT2 controlling caffeoylquinic acid biosynthesis and its upstream regulators through eQTL analysis, which provides a new strategy to identify novel transcriptional regulators in woody plants. Considering the ecological and economic values of woody species, it is important to understand the woody species-specific transcriptional regulation of SCW formation, and this is a fruitful area for further research.
Author Contributions
JZ collected and synthesized data from literature and wrote the manuscript. MX, GT, WM and J-GC revised the manuscript.
Funding
This research was supported by the Center for Bioenergy Innovation (CBI). CBI is supported by the Office of Biological and Environmental Research (BER) in the U.S. Department of Energy Office of Science. Oak Ridge National Laboratory is managed by UT-Battelle, LLC for the U.S. Department of Energy under Contract Number DE-AC05-00OR22725.
Conflict of Interest Statement
The authors declare that the research was conducted in the absence of any commercial or financial relationships that could be construed as a potential conflict of interest.
References
Almeida, T., Menendez, E., Capote, T., Ribeiro, T., Santos, C., and Goncalves, S. (2013). Molecular characterization of Quercus suber MYB1, a transcription factor up-regulated in cork tissues. J. Plant Physiol. 170, 172–178. doi: 10.1016/j.jplph.2012.08.023
Andersson-Gunneras, S., Hellgren, J. M., Bjorklund, S., Regan, S., Moritz, T., and Sundberg, B. (2003). Asymmetric expression of a poplar ACC oxidase controls ethylene production during gravitational induction of tension wood. Plant J. 34, 339–349. doi: 10.1046/j.1365-313X.2003.01727.x
Bao, H., Li, E., Mansfield, S. D., Cronk, Q. C., El-Kassaby, Y. A., and Douglas, C. J. (2013). The developing xylem transcriptome and genome-wide analysis of alternative splicing in Populus trichocarpa (black cottonwood) populations. BMC Genomics 14:359. doi: 10.1186/1471-2164-14-359
Bedon, F., Grima-Pettenati, J., and Mackay, J. (2007). Conifer R2R3-MYB transcription factors: sequence analyses and gene expression in wood-forming tissues of white spruce (Picea glauca). BMC Plant Biol. 7:17. doi: 10.1186/1471-2229-7-17
Bhargava, A., Ahad, A., Wang, S. C., Mansfield, S. D., Haughn, G. W., Douglas, C. J., et al. (2013). The interacting MYB75 and KNAT7 transcription factors modulate secondary cell wall deposition both in stems and seed coat in Arabidopsis. Planta 237, 1199–1211. doi: 10.1007/s00425-012-1821-9
Bhargava, A., Mansfield, S. D., Hall, H. C., Douglas, C. J., and Ellis, B. E. (2010). MYB75 Functions in regulation of secondary cell wall formation in the Arabidopsis inflorescence stem. Plant Physiol. 154, 1428–1438. doi: 10.1104/pp.110.162735
Bomal, C., Bedon, F., Caron, S., Mansfield, S. D., Levasseur, C., Cooke, J. E. K., et al. (2008). Involvement of Pinus taeda MYB1 and MYB8 in phenylpropanoid metabolism and secondary cell wall biogenesis: a comparative in planta analysis. J. Exp. Bot. 59, 3925–3939. doi: 10.1093/jxb/ern234
Carlsbecker, A., Lee, J. -Y., Roberts, C. J., Dettmer, J., Lehesranta, S., Zhou, J., et al. (2010). Cell signalling by microRNA165/6 directs gene dose-dependent root cell fate. Nature 465, 316–321. doi: 10.1038/nature08977
Chai, G., Qi, G., Cao, Y., Wang, Z., Yu, L., Tang, X., et al. (2014a). Poplar PdC3H17 and PdC3H18 are direct targets of PdMYB3 and PdMYB21, and positively regulate secondary wall formation in Arabidopsis and poplar. New Phytol. 203, 520–534. doi: 10.1111/nph.12825
Chai, G., Wang, Z., Tang, X., Yu, L., Qi, G., Wang, D., et al. (2014b). R2R3-MYB gene pairs in Populus: evolution and contribution to secondary wall formation and flowering time. J. Exp. Bot. 65, 4255–4269. doi: 10.1093/jxb/eru196
Chai, M. F., Bellizzi, M., Wan, C. X., Cui, Z. F., Li, Y. B., and Wang, G. L. (2015). The NAC transcription factor OsSWN1 regulates secondary cell wall development in Oryza sativa. J. Plant Biol. 58, 44–51. doi: 10.1007/s12374-014-0400-y
Chen, J., Quan, M., and Zhang, D. (2015). Genome-wide identification of novel long non-coding RNAs in Populus tomentosa tension wood, opposite wood and normal wood xylem by RNA-seq. Planta 241, 125–143. doi: 10.1007/s00425-014-2168-1
Chen, M., and Manley, J. L. (2009). Mechanisms of alternative splicing regulation: insights from molecular and genomics approaches. Nat. Rev. Mol. Cell Biol. 10, 741–754. doi: 10.1038/nrm2777
Courtois-Moreau, C. L., Pesquet, E., Sjödin, A., Muñz, L., Bollhöner, B., Kaneda, M., et al. (2009). A unique program for cell death in xylem fibers of Populus stem. Plant J. 58, 260–274. doi: 10.1111/j.1365-313X.2008.03777.x
Cseke, L. J., Zheng, J., and Podila, G. K. (2003). Characterization of PTM5 in aspen trees: a MADS-box gene expressed during woody vascular development. Gene 318, 55–67. doi: 10.1016/S0378-1119(03)00765-0
del Pozo, J. C., Boniotti, M. B., and Gutierrez, C. (2002). Arabidopsis E2Fc functions in cell division and is degraded by the ubiquitin-SCFAtSKP2 pathway in response to light. Plant Cell 14, 3057–3071. doi: 10.1105/tpc.006791
del Pozo, J. C., Diaz-Trivino, S., Cisneros, N., and Gutierrez, C. (2006). The balance between cell division and endoreplication depends on E2FC-DPB, transcription factors regulated by the ubiquitin-SCFSKP2A pathway in Arabidopsis. Plant Cell 18, 2224–2235. doi: 10.1105/tpc.105.039651
Deluc, L., Barrieu, F., Marchive, C., Lauvergeat, V., Decendit, A., Richard, T., et al. (2006). Characterization of a grapevine R2R3-MYB transcription factor that regulates the phenylpropanoid pathway. Plant Physiol. 140, 499–511. doi: 10.1104/pp.105.067231
Demura, T., Tashiro, G., Horiguchi, G., Kishimoto, N., Kubo, M., Matsuoka, N., et al. (2002). Visualization by comprehensive microarray analysis of gene expression programs during transdifferentiation of mesophyll cells into xylem cells. Proc. Natl. Acad. Sci. U.S.A. 99, 15794–15799. doi: 10.1073/pnas.232590499
Du, J., Mansfield, S. D., and Groover, A. T. (2009). The Populus homeobox gene ARBORKNOX2 regulates cell differentiation during secondary growth. Plant J. 60, 1000–1014. doi: 10.1111/j.1365-313X.2009.04017.x
Du, J., Miura, E., Robischon, M., Martinez, C., and Groover, A. (2011). The Populus class III HD ZIP transcription factor POPCORONA affects cell differentiation during secondary growth of woody stems. PLoS One 6:e17458. doi: 10.1371/journal.pone.0017458
Du, Q., Avci, U., Li, S., Gallego-Giraldo, L., Pattathil, S., Qi, L., et al. (2015). Activation of miR165b represses AtHB15 expression and induces pith secondary wall development in Arabidopsis. Plant J. 83, 388–400. doi: 10.1111/tpj.12897
Dubois, M., Van Den Broeck, L., and Inze, D. (2018). The pivotal role of ethylene in plant growth. Trends Plant Sci. 23, 311–323. doi: 10.1016/j.tplants.2018.01.003
Etchells, J. P., Provost, C. M., and Turner, S. R. (2012). Plant vascular cell division is maintained by an interaction between PXY and ethylene signalling. PLoS Genet. 8:e1002997. doi: 10.1371/journal.pgen.1002997
Floyd, S. K., Zalewski, C. S., and Bowman, J. L. (2006). Evolution of class III homeodomain–leucine zipper genes in streptophytes. Genetics 173, 373–388. doi: 10.1534/genetics.105.054239
Fornale, S., Shi, X. H., Chai, C. L., Encina, A., Irar, S., Capellades, M., et al. (2010). ZmMYB31 directly represses maize lignin genes and redirects the phenylpropanoid metabolic flux. Plant J. 64, 633–644. doi: 10.1111/j.1365-313X.2010.04363.x
Goicoechea, M., Lacombe, E., Legay, S., Mihaljevic, S., Rech, P., Jauneau, A., et al. (2005). EgMYB2, a new transcriptional activator from Eucalyptus xylem, regulates secondary cell wall formation and lignin biosynthesis. Plant J. 43, 553–567. doi: 10.1111/j.1365-313X.2005.02480.x
Groover, A. T., Mansfield, S. D., Difazio, S. P., Dupper, G., Fontana, J. R., Millar, R., et al. (2006). The Populus homeobox gene ARBORKNOX1 reveals overlapping mechanisms regulating the shoot apical meristem and the vascular cambium. Plant Mol. Biol. 61, 917–932. doi: 10.1007/s11103-006-0059-y
Grotewold, E., Drummond, B. J., Bowen, B., and Peterson, T. (1994). The MYB-homologous P-gene controls phlobaphene pigmentation in maize floral organs by directly activating a flavonoid biosynthetic gene subset. Cell 76, 543–553. doi: 10.1016/0092-8674(94)90117-1
Guillaumie, S., Mzid, R., Mechin, V., Leon, C., Hichri, I., Destrac-Irvine, A., et al. (2010). The grapevine transcription factor WRKY2 influences the lignin pathway and xylem development in tobacco. Plant Mol. Biol. 72, 215–234. doi: 10.1007/s11103-009-9563-1
He, J. B., Zhao, X. H., Du, P. Z., Zeng, W., Beahan, C. T., Wang, Y. Q., et al. (2018). KNAT7 positively regulates xylan biosynthesis by directly activating IRX9 expression in Arabidopsis. J. Integr. Plant Biol. 60, 514–528. doi: 10.1111/jipb.12638
Heckmann, S., Lermontova, I., Berckmans, B., De Veylder, L., Baumlein, H., and Schubert, I. (2011). The E2F transcription factor family regulates CENH3 expression in Arabidopsis thaliana. Plant J. 68, 646–656. doi: 10.1111/j.1365-313X.2011.04715.x
Houston, K., Tucker, M. R., Chowdhury, J., Shirley, N., and Little, A. (2016). The plant cell wall: a complex and dynamic structure as revealed by the responses of genes under stress conditions. Front. Plant Sci. 7:984. doi: 10.3389/fpls.2016.00984
Jensen, J. K., Kim, H., Cocuron, J. C., Orler, R., Ralph, J., and Wilkerson, C. G. (2011). The DUF579 domain containing proteins IRX15 and IRX15-L affect xylan synthesis in Arabidopsis. Plant J. 66, 387–400. doi: 10.1111/j.1365-313X.2010.04475.x
Jin, H., Cominelli, E., Bailey, P., Parr, A., Mehrtens, F., Jones, J., et al. (2000). Transcriptional repression by AtMYB4 controls production of UV-protecting sunscreens in Arabidopsis. EMBO J. 19, 6150–6161. doi: 10.1093/emboj/19.22.6150
Karpinska, B., Karlsson, M., Srivastava, M., Stenberg, A., Schrader, J., Sterky, F., et al. (2004). MYB transcription factors are differentially expressed and regulated during secondary vascular tissue development in hybrid aspen. Plant Mol. Biol. 56, 255–270. doi: 10.1007/s11103-004-3354-5
Kawabe, H., Ohtani, M., Kurata, T., Sakamoto, T., and Demura, T. (2018). Protein S-nitrosylation regulates xylem vessel cell differentiation in Arabidopsis. Plant Cell Physiol. 59, 17–29. doi: 10.1093/pcp/pcx151
Kim, E. -D., and Sung, S. (2012). Long noncoding RNA: unveiling hidden layer of gene regulatory networks. Trends Plant Sci. 17, 16–21. doi: 10.1016/j.tplants.2011.10.008
Kim, J., Jung, J. H., Reyes, J. L., Kim, Y. S., Kim, S. Y., Chung, K. S., et al. (2005). microRNA-directed cleavage of ATHB15 mRNA regulates vascular development in Arabidopsis inflorescence stems. Plant J. 42, 84–94. doi: 10.1111/j.1365-313X.2005.02354.x
Kim, W. C., Kim, J. Y., Ko, J. H., Kang, H., and Han, K. H. (2014a). Identification of direct targets of transcription factor MYB46 provides insights into the transcriptional regulation of secondary wall biosynthesis. Plant Mol. Biol. 85, 589–599. doi: 10.1007/s11103-014-0205-x
Kim, W. C., Ko, J. H., and Han, K. H. (2012). Identification of a cis-acting regulatory motif recognized by MYB46, a master transcriptional regulator of secondary wall biosynthesis. Plant Mol. Biol. 78, 489–501. doi: 10.1007/s11103-012-9880-7
Kim, W. C., Ko, J. H., Kim, J. Y., Kim, J., Bae, H. J., and Han, K. H. (2013). MYB46 directly regulates the gene expression of secondary wall-associated cellulose synthases in Arabidopsis. Plant J. 73, 26–36. doi: 10.1111/j.1365-313x.2012.05124.x
Kim, W. C., Reca, I. B., Kim, Y., Park, S., Thomashow, M., Keegstra, K., et al. (2014b). Transcription factors that directly regulate the expression of CSLA9 encoding mannan synthase in Arabidopsis thaliana. Plant Mol. Biol. 84, 577–587. doi: 10.1007/s11103-013-0154-9
Ko, J. H., Jeon, H. W., Kim, W. C., Kim, J. Y., and Han, K. H. (2014). The MYB46/MYB83-mediated transcriptional regulatory programme is a gatekeeper of secondary wall biosynthesis. Ann. Bot. 114, 1099–1107. doi: 10.1093/aob/mcu126
Ko, J. H., Kim, W. C., and Han, K. H. (2009). Ectopic expression of MYB46 identifies transcriptional regulatory genes involved in secondary wall biosynthesis in Arabidopsis. Plant J. 60, 649–665. doi: 10.1111/j.1365-313X.2009.03989.x
Ko, J. H., Prassinos, C., and Han, K. H. (2006). Developmental and seasonal expression of PtaHB1, a Populus gene encoding a class III HD-Zip protein, is closely associated with secondary growth and inversely correlated with the level of microRNA (miR166). New Phytol. 169, 469–478. doi: 10.1111/j.1469-8137.2005.01623.x
Kosugi, S., and Ohashi, Y. (2002). Interaction of the Arabidopsis E2F and DP proteins confers their concomitant nuclear translocation and transactivation. Plant Physiol. 128, 833–843. doi: 10.1104/pp.010642
Kubo, M., Udagawa, M., Nishikubo, N., Horiguchi, G., Yamaguchi, M., Ito, J., et al. (2005). Transcription switches for protoxylem and metaxylem vessel formation. Genes Dev. 19, 1855–1860. doi: 10.1101/gad.1331305
Legay, S., Lacombe, E., Goicoechea, M., Briere, C., Seguin, A., Mackay, J., et al. (2007). Molecular characterization of EgMYB1, a putative transcriptional repressor of the lignin biosynthetic pathway. Plant Sci. 173, 542–549. doi: 10.1016/j.plantsci.2007.08.007
Legay, S., Sivadon, P., Blervacq, A. S., Pavy, N., Baghdady, A., Tremblay, L., et al. (2010). EgMYB1, an R2R3 MYB transcription factor from eucalyptus negatively regulates secondary cell wall formation in Arabidopsis and poplar. New Phytol. 188, 774–786. doi: 10.1111/j.1469-8137.2010.03432.x
Li, C. F., Wang, X. Q., Ran, L. Y., Tian, Q. Y., Fan, D., and Luo, K. M. (2015). PtoMYB92 is a transcriptional activator of the lignin biosynthetic pathway during secondary cell wall formation in Populus tomentosa. Plant Cell Physiol. 56, 2436–2446. doi: 10.1093/pcp/pcv157
Li, E. Y., Bhargava, A., Qiang, W. Y., Friedmann, M. C., Forneris, N., Savidge, R. A., et al. (2012). The class II KNOX gene KNAT7 negatively regulates secondary wall formation in Arabidopsis and is functionally conserved in Populus. New Phytol. 194, 102-115. doi: 10.1111/j.1469-8137.2011.04016.x
Li, E. Y., Wang, S. C., Liu, Y. Y., Chen, J. G., and Douglas, C. J. (2011). OVATE FAMILY PROTEIN4 (OFP4) interaction with KNAT7 regulates secondary cell wall formation in Arabidopsis thaliana. Plant J. 67, 328–341. doi: 10.1111/j.1365-313X.2011.04595.x
Li, Q. Z., Lin, Y. C., Sun, Y. H., Song, J., Chen, H., Zhang, X. H., et al. (2012). Splice variant of the SND1 transcription factor is a dominant negative of SND1 members and their regulation in Populus trichocarpa. Proc. Natl. Acad. Sci. U.S.A. 109, 14699–14704. doi: 10.1073/pnas.1212977109
Liang, Y. K., Dubos, C., Dodd, I. C., Holroyd, G. H., Hetherington, A. M., and Campbell, M. M. (2005). AtMYB61, an R2R3-MYB transcription factor controlling stomatal aperture in Arabidopsis thaliana. Curr. Biol. 15, 1201–1206. doi: 10.1016/j.cub.2005.06.041
Lin, Y. -C., Li, W., Sun, Y. -H., Kumari, S., Wei, H., Li, Q., et al. (2013). SND1 transcription factor–directed quantitative functional hierarchical genetic regulatory network in wood formation in Populus trichocarpa. Plant Cell 25, 4324–4341. doi: 10.1105/tpc.113.117697
Lin, Y. J., Chen, H., Li, Q., Li, W., Wang, J. P., Shi, R., et al. (2017). Reciprocal cross-regulation of VND and SND multigene TF families for wood formation in Populus trichocarpa. Proc. Natl. Acad. Sci. U.S.A. 114, E9722–E9729. doi: 10.1073/pnas.1714422114
Little, C., and Savidge, R. (1987). The Role Of Plant Growth Regulators In Forest Tree Cambial Growth. Berlin: Springer.
Liu, Y., Wei, M., Hou, C., Lu, T., Liu, L., Wei, H., et al. (2017). Functional characterization of Populus PsnSHN2 in coordinated regulation of secondary wall components in tobacco. Sci. Rep. 7:42. doi: 10.1038/s41598-017-00093-z
Liu, Y. Y., You, S. J., Taylor-Teeples, M., Li, W. L., Schuetz, M., Brady, S. M., et al. (2014). Bel1-like homeodomain6 and knotted Arabidopsis thaliana7 interact and regulate secondary cell wall formation via repression of revoluta. Plant Cell 26, 4843–4861. doi: 10.1105/tpc.114.128322
Love, J., Bjorklund, S., Vahala, J., Hertzberg, M., Kangasjarvi, J., and Sundberg, B. (2009). Ethylene is an endogenous stimulator of cell division in the cambial meristem of Populus. Proc. Natl. Acad. Sci. U.S.A. 106, 5984–5989. doi: 10.1073/pnas.0811660106
Lu, S., Sun, Y.-H., Shi, R., Clark, C., Li, L., and Chiang, V. L. (2005). Novel and mechanical stress–responsive microRNAs in Populus trichocarpa that are absent from Arabidopsis. Plant Cell 17, 2186–2203. doi: 10.1105/tpc.105.033456
Lu, S. F., Li, Q. Z., Wei, H. R., Chang, M. J., Tunlaya-Anukit, S., Kim, H., et al. (2013). Ptr-miR397a is a negative regulator of laccase genes affecting lignin content in Populus trichocarpa. Proc. Natl. Acad. Sci. U.S.A. 110, 10848–10853. doi: 10.1073/pnas.1308936110
Mallory, A. C., and Vaucheret, H. (2006). Functions of microRNAs and related small RNAs in plants. Nat. Genet. 38, S31–S36. doi: 10.1038/ng1791
McCarthy, R. L., Zhong, R. Q., Fowler, S., Lyskowski, D., Piyasena, H., Carleton, K., et al. (2010). The poplar MYB transcription factors, PtrMYB3 and PtrMYB20, are involved in the regulation of secondary wall biosynthesis. Plant Cell Physiol. 51, 1084–1090. doi: 10.1093/pcp/pcq064
McCarthy, R. L., Zhong, R. Q., and Ye, Z. H. (2009). MYB83 is a direct target of SND1 and acts redundantly with MYB46 in the regulation of secondary cell wall biosynthesis in Arabidopsis. Plant Cell Physiol. 50, 1950–1964. doi: 10.1093/pcp/pcp139
Mele, G., Ori, N., Sato, Y., and Hake, S. (2003). The knotted1-like homeobox gene BREVIPEDICELLUS regulates cell differentiation by modulating metabolic pathways. Genes Dev. 17, 2088–2093. doi: 10.1101/gad.1120003
Mitsuda, N., Iwase, A., Yamamoto, H., Yoshida, M., Seki, M., Shinozaki, K., et al. (2007). NAC transcription factors, NST1 and NST3, are key regulators of the formation of secondary walls in woody tissues of Arabidopsis. Plant Cell 19, 270–280. doi: 10.1105/tpc.106.047043
Mitsuda, N., and Ohme-Takagi, M. (2008). NAC transcription factors NST1 and NST3 regulate pod shattering in a partially redundant manner by promoting secondary wall formation after the establishment of tissue identity. Plant J. 56, 768–778. doi: 10.1111/j.1365-313X.2008.03633.x
Mitsuda, N., Seki, M., Shinozaki, K., and Ohme-Takagi, M. (2005). The NAC transcription factors NST1 and NST2 of Arabidopsis regulate secondary wall thickenings and are required for anther dehiscence. Plant Cell 17, 2993–3006. doi: 10.1105/tpc.105.036004
Miyashima, S., Koi, S., Hashimoto, T., and Nakajima, K. (2011). Non-cell-autonomous microRNA165 acts in a dose-dependent manner to regulate multiple differentiation status in the Arabidopsis root. Development 138, 2303–2313. doi: 10.1242/dev.060491
Newman, L. J., Perazza, D. E., Juda, L., and Campbell, M. M. (2004). Involvement of the R2R3-MYB, AtMYB61, in the ectopic lignification and dark-photomorphogenic components of the det3 mutant phenotype. Plant J. 37, 239–250. doi: 10.1046/j.1365-313X.2003.01953.x
Nuoendagula, Tsuji, Y., Takata, N., Sakamoto, S., Nakagawa-Izumi, A., Taniguchi, T., et al. (2017). Change in lignin structure, but not in lignin content, in transgenic poplar overexpressing the rice master regulator of secondary cell wall biosynthesis. Physiol. Plant. 163, 170–182. doi: 10.1111/ppl.12684
Ohashi-Ito, K., Iwamoto, K., and Fukuda, H. (2018). LOB DOMAIN-CONTAINING PROTEIN 15 positively regulates expression of VND7, a master regulator of tracheary elements. Plant Cell Physiol. 59, 989–996. doi: 10.1093/pcp/pcy036
Ohashi-Ito, K., Oda, Y., and Fukuda, H. (2010). Arabidopsis vascular-related nac-domain6 directly regulates the genes that govern programmed cell death and secondary wall formation during xylem differentiation. Plant Cell 22, 3461–3473. doi: 10.1105/tpc.110.075036
Ohtani, M., Nishikubo, N., Xu, B., Yamaguchi, M., Mitsuda, N., Goue, N., et al. (2011). A NAC domain protein family contributing to the regulation of wood formation in poplar. Plant J. 67, 499–512. doi: 10.1111/j.1365-313X.2011.04614.x
Olsen, A. N., Ernst, H. A., Leggio, L. L., and Skriver, K. (2005). NAC transcription factors: structurally distinct, functionally diverse. Trends Plant Sci. 10, 79–87. doi: 10.1016/j.tplants.2004.12.010
Omer, S., Kumar, S., and Khan, B. M. (2013). Over-expression of a subgroup 4 R2R3 type MYB transcription factor gene from Leucaena leucocephala reduces lignin content in transgenic tobacco. Plant Cell Rep. 32, 161–171. doi: 10.1007/s00299-012-1350-9
Pandey, S. K., Nookaraju, A., Fujino, T., Pattathil, S., and Joshi, C. P. (2016). Virus-induced gene silencing (VIGS)-mediated functional characterization of two genes involved in lignocellulosic secondary cell wall formation. Plant Cell Rep. 35, 2353–2367. doi: 10.1007/s00299-016-2039-2
Pascual, M. B., Llebres, M. T., Craven-Bartle, B., Canas, R. A., Canovas, F. M., and Avila, C. (2018). PpNAC1, a main regulator of phenylalanine biosynthesis and utilization in maritime pine. Plant Biotechnol. J. 16, 1094–1104. doi: 10.1111/pbi.12854
Patzlaff, A., Mcinnis, S., Courtenay, A., Surman, C., Newman, L. J., Smith, C., et al. (2003a). Characterisation of a pine MYB that regulates lignification. Plant J. 36, 743–754. doi: 10.1046/j.1365-313X.2003.01916.x
Patzlaff, A., Newman, L. J., Dubos, C., Whetten, R., Smith, C., Mcinnis, S., et al. (2003b). Characterisation of PtMYB1, an R2R3-MYB from pine xylem. Plant Mol. Biol. 53, 597–608. doi: 10.1023/B:PLAN.0000019066.07933.d6
Penfield, S., Meissner, R. C., Shoue, D. A., Carpita, N. C., and Bevan, M. W. (2001). MYB61 is required for mucilage deposition and extrusion in the Arabidopsis seed coat. Plant Cell 13, 2777–2791. doi: 10.1105/tpc.010265
Preston, J., Wheeler, J., Heazlewood, J., Li, S. F., and Parish, R. W. (2004). AtMYB32 is required for normal pollen development in Arabidopsis thaliana. Plant J. 40, 979–995. doi: 10.1111/j.1365-313X.2004.02280.x
Pyo, H., Demura, T., and Fukuda, H. (2007). TERE; a novel cis-element responsible for a coordinated expression of genes related to programmed cell death and secondary wall formation during differentiation of tracheary elements. Plant J. 51, 955–965. doi: 10.1111/j.1365-313X.2007.03180.x
Ragauskas, A. J., Beckham, G. T., Biddy, M. J., Chandra, R., Chen, F., Davis, M. F., et al. (2014). Lignin valorization: improving lignin processing in the biorefinery. Science 344:1246843. doi: 10.1126/science.1246843
Rao, X., and Dixon, R. A. (2018). Current models for transcriptional regulation of secondary cell wall biosynthesis in grasses. Front. Plant Sci. 9:399. doi: 10.3389/fpls.2018.00399
Richmond, T. (2000). Higher plant cellulose synthases. Genome Biol. 1, reviews3001.1–reviews3001.6. doi: 10.1186/gb-2000-1-4-reviews3001
Robischon, M., Du, J., Miura, E., and Groover, A. (2011). The Populus class III HD ZIP, popREVOLUTA, influences cambium initiation and patterning of woody stems. Plant Physiol. 155, 1214–1225. doi: 10.1104/pp.110.167007
Romano, J. M., Dubos, C., Prouse, M. B., Wilkins, O., Hong, H., Poole, M., et al. (2012). AtMYB61, an R2R3-MYB transcription factor, functions as a pleiotropic regulator via a small gene network. New Phytol. 195, 774–786. doi: 10.1111/j.1469-8137.2012.04201.x
Sakamoto, S., Takata, N., Oshima, Y., Yoshida, K., Taniguchi, T., and Mitsuda, N. (2016). Wood reinforcement of poplar by rice NAC transcription factor. Sci. Rep. 6:19925. doi: 10.1038/srep19925
Seyfferth, C., Wessels, B., Jokipii-Lukkari, S., Sundberg, B., Delhomme, N., Felten, J., et al. (2018). Ethylene-related gene expression networks in wood formation. Front. Plant Sci. 9:272. doi: 10.3389/fpls.2018.00272
Sonoda, T., Koita, H., Nakamoto-Ohta, S., Kondo, K., Suezaki, T., Kato, T., et al. (2009). Increasing fiber length and growth in transgenic tobacco plants overexpressing a gene encoding the Eucalyptus camaldulensis HD-Zip class II transcription factor. Plant Biotechnol. 26, 115–120. doi: 10.5511/plantbiotechnology.26.115
Soyano, T., Thitamadee, S., Machida, Y., and Chua, N. H. (2008). ASYMMETRIC LEAVES2-LIKE19/LATERAL ORGAN BOUNDARIES DOMAIN30 and ASL20/LBD18 regulate tracheary element differentiation in Arabidopsis. Plant Cell 20, 3359–3373. doi: 10.1105/tpc.108.061796
Sun, X., Wang, C., Xiang, N., Li, X., Yang, S., Du, J., et al. (2017). Activation of secondary cell wall biosynthesis by miR319-targeted TCP4 transcription factor. Plant Biotechnol. J. 15, 1284–1294. doi: 10.1111/pbi.12715
Sundell, D., Street, N. R., Kumar, M., Mellerowicz, E. J., Kucukoglu, M., Johnsson, C., et al. (2017). AspWood: high-spatial-resolution transcriptome profiles reveal uncharacterized modularity of wood formation in Populus tremula. Plant Cell 29, 1585–1604. doi: 10.1105/tpc.17.00153
Sunkar, R., Chinnusamy, V., Zhu, J., and Zhu, J. -K. (2007). Small RNAs as big players in plant abiotic stress responses and nutrient deprivation. Trends Plant Sci. 12, 301–309. doi: 10.1016/j.tplants.2007.05.001
Syed, N. H., Kalyna, M., Marquez, Y., Barta, A., and Brown, J. W. (2012). Alternative splicing in plants–coming of age. Trends Plant Sci. 17, 616–623. doi: 10.1016/j.tplants.2012.06.001
Tang, X., Zhuang, Y., Qi, G., Wang, D., Liu, H., Wang, K., et al. (2015). Poplar PdMYB221 is involved in the direct and indirect regulation of secondary wall biosynthesis during wood formation. Sci. Rep. 5:12240. doi: 10.1038/srep12240
Taylor-Teeples, M., Lin, L., De Lucas, M., Turco, G., Toal, T. W., Gaudinier, A., et al. (2015). An Arabidopsis gene regulatory network for secondary cell wall synthesis. Nature 517, 571–575. doi: 10.1038/nature14099
Tian, Q., Wang, X., Li, C., Lu, W., Yang, L., Jiang, Y., et al. (2013). Functional characterization of the poplar R2R3-MYB transcription factor PtoMYB216 involved in the regulation of lignin biosynthesis during wood formation. PLoS One 8:e76369. doi: 10.1371/journal.pone.0076369
Tuskan, G. (1998). Short-rotation woody crop supply systems in the United States: what do we know and what do we need to know? Biomass Bioenergy 14, 307–315. doi: 10.1016/S0961-9534(97)10065-4
Vahala, J., Felten, J., Love, J., Gorzsás, A., Gerber, L., Lamminmäki, A., et al. (2013). A genome-wide screen for ethylene-induced Ethylene Response Factors (ERFs) in hybrid aspen stem identifies ERF genes that modify stem growth and wood properties. New Phytol. 200, 511–522. doi: 10.1111/nph.12386
Wang, H. H., Tang, R. J., Liu, H., Chen, H. Y., Liu, J. Y., Jiang, X. N., et al. (2013). Chimeric repressor of PtSND2 severely affects wood formation in transgenic Populus. Tree Physiol. 33, 878–886. doi: 10.1093/treephys/tpt058
Wang, H. Z., Avci, U., Nakashima, J., Hahn, M. G., Chen, F., and Dixon, R. A. (2010). Mutation of WRKY transcription factors initiates pith secondary wall formation and increases stem biomass in dicotyledonous plants. Proc. Natl. Acad. Sci. U.S.A. 107, 22338–22343. doi: 10.1073/pnas.1016436107
Wang, H. Z., Zhao, Q., Chen, F., Wang, M. Y., and Dixon, R. A. (2011). NAC domain function and transcriptional control of a secondary cell wall master switch. Plant J. 68, 1104–1114. doi: 10.1111/j.1365-313X.2011.04764.x
Wang, S. C., Li, E. Y., Porth, I., Chen, J. G., Mansfield, S. D., and Douglas, C. J. (2014). Regulation of secondary cell wall biosynthesis by poplar R2R3 MYB transcription factor PtrMYB152 in Arabidopsis. Sci. Rep. 4:5054. doi: 10.1038/srep05054
Wierzbicki, A. T. (2012). The role of long non-coding RNA in transcriptional gene silencing. Curr. Opin. Plant Biol. 15, 517–522. doi: 10.1016/j.pbi.2012.08.008
Xiao, W. H., Yang, Y., and Yu, J. J. (2018). ZmNST3 and ZmNST4 are master switches for secondary wall deposition in maize (Zea mays L.). Plant Sci. 266, 83–94. doi: 10.1016/j.plantsci.2017.03.012
Xu, C., Fu, X., Liu, R., Guo, L., Ran, L., Li, C., et al. (2017). PtoMYB170 positively regulates lignin deposition during wood formation in poplar and confers drought tolerance in transgenic Arabidopsis. Tree Physiol. 37, 1713–1726. doi: 10.1093/treephys/tpx093
Xu, Q., Yin, X. R., Zeng, J. K., Ge, H., Song, M., Xu, C. J., et al. (2014). Activator- and repressor-type MYB transcription factors are involved in chilling injury induced flesh lignification in loquat via their interactions with the phenylpropanoid pathway. J. Exp. Bot. 65, 4349–4359. doi: 10.1093/jxb/eru208
Yamaguchi, M., Kubo, M., Fukuda, H., and Demura, T. (2008). Vascular-related NAC-DOMAIN7 is involved in the differentiation of all types of xylem vessels in Arabidopsis roots and shoots. Plant J. 55, 652–664. doi: 10.1111/j.1365-313X.2008.03533.x
Yamaguchi, M., Mitsuda, N., Ohtani, M., Ohme-Takagi, M., Kato, K., and Demura, T. (2011). VASCULAR-RELATED NAC-DOMAIN 7 directly regulates the expression of a broad range of genes for xylem vessel formation. Plant J. 66, 579–590. doi: 10.1111/j.1365-313X.2011.04514.x
Yamaguchi, M., Ohtani, M., Mitsuda, N., Kubo, M., Ohme-Takagi, M., Fukuda, H., et al. (2010). VND-INTERACTING2, a NAC domain transcription factor, negatively regulates xylem vessel formation in Arabidopsis. Plant Cell 22, 1249–1263. doi: 10.1105/tpc.108.064048
Yang, L., Hou, Y., Zhao, X., Lu, W., Li, Y., Yang, F., et al. (2015). Identification and characterization of a wood-associated NAC domain transcription factor PtoVNS11 from Populus tomentosa Carr. Trees 29, 1091–1101. doi: 10.1007/s00468-015-1188-1
Yang, L., Zhao, X., Ran, L. Y., Li, C. F., Fan, D., and Luo, K. M. (2017). PtoMYB156 is involved in negative regulation of phenylpropanoid metabolism and secondary cell wall biosynthesis during wood formation in poplar. Sci. Rep. 7:41209. doi: 10.1038/srep41209
Yang, L., Zhao, X., Yang, F., Fan, D., Jiang, Y., and Luo, K. (2016). PtrWRKY19, a novel WRKY transcription factor, contributes to the regulation of pith secondary wall formation in Populus trichocarpa. Sci. Rep. 6:18643. doi: 10.1038/srep18643
Yordanov, Y. S., Regan, S., and Busov, V. (2010). Members of the LATERAL ORGAN BOUNDARIES DOMAIN transcription factor family are involved in the regulation of secondary growth in Populus. Plant Cell 22, 3662–3677. doi: 10.1105/tpc.110.078634
Zhang, J., Nieminen, K., Serra, J. A., and Helariutta, Y. (2014). The formation of wood and its control. Curr. Opin. Plant Biol. 17, 56–63. doi: 10.1016/j.pbi.2013.11.003
Zhang, J., Serra, J. A. A., and Helariutta, Y. (2015). Wood development: Growth through knowledge. Nat. Plants 1:15060. doi: 10.1038/nplants.2015.60
Zhang, J., Yang, Y., Zheng, K., Xie, M., Feng, K., Jawdy, S. S., et al. (2018). Genome-wide association studies and expression-based quantitative trait loci analyses reveal roles of HCT2 in caffeoylquinic acid biosynthesis and its regulation by defense-responsive transcription factors in Populus. New Phytol. 220, 502–516. doi: 10.1111/nph.15297
Zhao, C. S., Avci, U., Grant, E. H., Haigler, C. H., and Beers, E. P. (2008). XND1, a member of the NAC domain family in Arabidopsis thaliana, negatively regulates lignocellulose synthesis and programmed cell death in xylem. Plant J. 53, 425–436. doi: 10.1111/j.1365-313X.2007.03350.x
Zhao, C. S., Lasses, T., Bako, L., Kong, D. Y., Zhao, B. Y., Chanda, B., et al. (2017). XYLEM NAC DOMAIN1, an angiosperm NAC transcription factor, inhibits xylem differentiation through conserved motifs that interact with RETINOBLASTOMA-RELATED. New Phytol. 216, 76–89. doi: 10.1111/nph.14704
Zhao, Q., Nakashima, J., Chen, F., Yin, Y. B., Fu, C. X., Yun, J. F., et al. (2013). LACCASE is necessary and nonredundant with PEROXIDASE for lignin polymerization during vascular development in Arabidopsis. Plant Cell 25, 3976–3987. doi: 10.1105/tpc.113.117770
Zhao, Q. A., Gallego-Giraldo, L., Wang, H. Z., Zeng, Y. N., Ding, S. Y., Chen, F., et al. (2010). An NAC transcription factor orchestrates multiple features of cell wall development in Medicago truncatula. Plant J. 63, 100–114. doi: 10.1111/j.1365-313X.2010.04223.x
Zhao, Y. J., Sun, J. Y., Xu, P., Zhang, R., and Li, L. G. (2014). Intron-mediated alternative splicing of WOOD-ASSOCIATED NAC TRANSCRIPTION FACTOR1B regulates cell wall thickening during fiber development in Populus species. Plant Physiol. 164, 765–776. doi: 10.1104/pp.113.231134
Zhong, R., Mccarthy, R. L., Haghighat, M., and Ye, Z. H. (2013). The poplar MYB master switches bind to the SMRE site and activate the secondary wall biosynthetic program during wood formation. PLoS One 8:e69219. doi: 10.1371/journal.pone.0069219
Zhong, R., Richardson, E. A., and Ye, Z. H. (2007). The MYB46 transcription factor is a direct target of SND1 and regulates secondary wall biosynthesis in Arabidopsis. Plant Cell 19, 2776–2792. doi: 10.1105/tpc.107.053678
Zhong, R. Q., Lee, C., Mccarthy, R. L., Reeves, C. K., Jones, E. G., and Ye, Z. H. (2011). Transcriptional activation of secondary wall biosynthesis by rice and maize NAC and MYB transcription factors. Plant Cell Physiol. 52, 1856–1871. doi: 10.1093/pcp/pcr123
Zhong, R. Q., Lee, C. H., and Ye, Z. H. (2010a). Evolutionary conservation of the transcriptional network regulating secondary cell wall biosynthesis. Trends Plant Sci. 15, 625–632. doi: 10.1016/j.tplants.2010.08.007
Zhong, R. Q., Lee, C. H., and Ye, Z. H. (2010b). Functional characterization of poplar wood-associated NAC domain transcription factors. Plant Physiol. 152, 1044–1055. doi: 10.1104/pp.109.148270
Zhong, R. Q., Lee, C. H., and Ye, Z. H. (2010c). Global analysis of direct targets of secondary wall NAC master switches in Arabidopsis. Mol. Plant 3, 1087–1103. doi: 10.1093/mp/ssq062
Zhong, R. Q., Lee, C. H., Zhou, J. L., Mccarthy, R. L., and Ye, Z. H. (2008). A battery of transcription factors involved in the regulation of secondary cell wall biosynthesis in Arabidopsis. Plant Cell 20, 2763–2782. doi: 10.1105/tpc.108.061325
Zhong, R. Q., and Ye, Z. H. (2012). MYB46 and MYB83 bind to the SMRE sites and directly activate a suite of transcription factors and secondary wall biosynthetic genes. Plant Cell Physiol. 53, 368–380. doi: 10.1093/pcp/pcr185
Zhou, D. L., Du, Q. Z., Chen, J. H., Wang, Q. S., and Zhang, D. Q. (2017). Identification and allelic dissection uncover roles of lncRNAs in secondary growth of Populus tomentosa. DNA Res. 24, 473–486. doi: 10.1093/dnares/dsx018
Zhou, J. L., Lee, C. H., Zhong, R. Q., and Ye, Z. H. (2009). MYB58 and MYB63 are transcriptional activators of the lignin biosynthetic pathway during secondary cell wall formation in Arabidopsis. Plant Cell 21, 248–266. doi: 10.1105/tpc.108.063321
Keywords: woody plants, Populus, secondary cell wall, transcription factor, transcriptional regulation
Citation: Zhang J, Xie M, Tuskan GA, Muchero W and Chen J-G (2018) Recent Advances in the Transcriptional Regulation of Secondary Cell Wall Biosynthesis in the Woody Plants. Front. Plant Sci. 9:1535. doi: 10.3389/fpls.2018.01535
Received: 18 June 2018; Accepted: 28 September 2018;
Published: 23 October 2018.
Edited by:
Laura Elizabeth Bartley, University of Oklahoma, United StatesReviewed by:
Berit Ebert, The University of Melbourne, AustraliaSamuel P. Hazen, University of Massachusetts Amherst, United States
Copyright © 2018 Zhang, Xie, Tuskan, Muchero and Chen. This is an open-access article distributed under the terms of the Creative Commons Attribution License (CC BY). The use, distribution or reproduction in other forums is permitted, provided the original author(s) and the copyright owner(s) are credited and that the original publication in this journal is cited, in accordance with accepted academic practice. No use, distribution or reproduction is permitted which does not comply with these terms.
*Correspondence: Wellington Muchero, bXVjaGVyb3dAb3JubC5nb3Y= Jin-Gui Chen, Y2hlbmpAb3JubC5nb3Y=