- 1Triticeae Research Institute, Sichuan Agricultural University, Chengdu, China
- 2Joint International Research Laboratory of Crop Resources and Genetic Improvement, Sichuan Agricultural University, Chengdu, China
- 3College of Resources, Sichuan Agricultural University, Chengdu, China
Essential transition metals and non-essential metals often co-exist in arable soils. In plants, some transition metal transporters, such as the natural resistance-associated macrophage proteins (NRAMPs), poorly selectively transport metals with similar chemical properties whether they are essential or non-essential. In this study, a member of the NRAMP transporter family, TpNRAMP3, was identified from dwarf Polish wheat (Triticum polonicum L.). TpNRAMP3 encodes a plasma membrane-localized protein and was highly expressed in leaf blades and roots at the jointing and booting stages, and in the first nodes at the grain filling stage. Expression of TpNRAMP3 increased sensitivity to Cd and Co, but not Zn, and increased the Cd and Co concentrations in yeast. TpNRAMP3 expression in Arabidopsis increased concentrations of Cd, Co, and Mn, but not Fe or Zn, in roots, shoots, and whole plant. However, TpNRAMP3 did not affect translocation of Cd, Co, or Mn from roots to shoots. These results suggest that TpNRAMP3 is a transporter for Cd, Co, and Mn accumulation, but not for Fe or Zn. However, Cd and Co are non-essential toxic metals; selective genetic manipulation of TpNRAMP3 will help breed low Cd- and Co-accumulating cultivars.
Introduction
Essential transition metals, such as Fe, Zn, Mn, and Cu, are cofactors of numerous plant proteins and enzymes; therefore, they are required for plant growth and development (Thomine et al., 2000; Lin and Aarts, 2012; Yamaji et al., 2013). Conversely, non-essential metals, such as Cd, Co, and Pb, are highly toxic metals that damage the photosynthetic apparatus, affect respiratory and nitrogen metabolism, and alter the balance of water and nutrient uptake, ultimately inhibiting plant growth and development (Herbette et al., 2006; Balen et al., 2011; Lange et al., 2017). Essential transition metals and non-essential metals often co-exist in arable soils. Therefore, plants need to absorb essential metals to ensure the proper homeostasis of nutrient elements, while excluding non-essential metals to prevent damage (Lahner et al., 2003; Xiao et al., 2008; Lin and Aarts, 2012). However, some transition metal transporters, such as natural resistance-associated macrophage proteins (NRAMPs) and iron-regulated transporters (IRTs), poorly selectively transport metals with similar chemical properties whether they are essential or non-essential (Nevo and Nelson, 2006; Sasaki et al., 2012; Barberon et al., 2014; Pottier et al., 2015).
To date, numerous plant NRAMPs have been characterized from different plant species (Thomine et al., 2000; Nevo and Nelson, 2006; Sasaki et al., 2012; Tejada-Jiménez et al., 2015; Qin et al., 2017; Gao et al., 2018). For Arabidopsis, five of six NRAMP genes have been functionally identified. Among the identified AtNRAMPs, AtNRAMP1, AtNRAMP3, and AtNRAMP4 all transport Fe, Mn, and Cd (Curie et al., 2000; Thomine et al., 2000, 2003; Lanquar et al., 2005, 2010; Cailliatte et al., 2010; Pottier et al., 2015). AtNRAMP6 also transports Cd, but not Fe or Mn (Cailliatte et al., 2009). Additionally, AtNRAMP3, AtNRAMP4, and AtNRAMP6 are intracellular metal transporters for Cd, Fe, and Mn sequestration and distribution, but not for their uptake (Lanquar et al., 2005, 2010; Cailliatte et al., 2009; Pottier et al., 2015). Only AtNRAMP1 functions in metal uptake of Cd, Fe, and Mn (Curie et al., 2000; Thomine et al., 2000, 2003; Lanquar et al., 2005, 2010; Cailliatte et al., 2009).
In rice, seven NRAMP genes have been annotated; five of which have also been functionally characterized. Although the characterized OsNRAMPs are localized at the plasma membrane, they exhibit different metal transport properties (Curie et al., 2000; Xia et al., 2010; Takahashi et al., 2011; Ishimaru et al., 2012; Sasaki et al., 2012; Yamaji et al., 2013; Yang et al., 2013; Li et al., 2014; Tiwari et al., 2014; Peris-Peris et al., 2017). Among them, three OsNRAMPs including OsNRAMP1, OsNRAMP4, and OsNRAMP5 function to take up metals. OsNRAMP1 transports Cd, Fe, As, and Mn (Curie et al., 2000; Takahashi et al., 2011; Tiwari et al., 2014), OsNRAMP4 only absorbs Al, not Fe, Mn or Cd (Xia et al., 2010; Li et al., 2014), and OsNRAMP5 transports Mn, Cd, and Fe (Ishimaru et al., 2012; Sasaki et al., 2012; Takahashi et al., 2014). Although OsNRAMP6 transports Fe and Mn but not Cd or As in yeast (Peris-Peris et al., 2017), it has not been confirmed whether it functions in metal uptake or intracellular transport. Additionally, OsNRAMP3 transports Mn for Mn distribution and remobilization, but not Fe, Cd, or Zn (Yamaji et al., 2013; Yang et al., 2013).
Some NRAMP genes have also been identified from other plant species, such as GmNRAMPs from soybean (Kaiser et al., 2003; Qin et al., 2017), MbNRAMP1 from Malus baccata (Xiao et al., 2008), NtNRAMP5 from tobacco (Tang et al., 2017), TjNRAMP4 from Thlaspi japonicum (Mizuno et al., 2005), and MtNRAMP1 from Medicago truncatula (Tejada-Jiménez et al., 2015). Most of their metal transport properties were functionally identified in yeast; only MtNRAMP1 was characterized as a Fe uptake protein in M. truncatula (Tejada-Jiménez et al., 2015). However, there is no information on NRAMP genes from wheat.
In the present study, a NRAMP gene, named TpNRAMP3, was isolated from dwarf Polish wheat (DPW, 2n = 4x = 28, AABB, Triticum polonicum L.) that its seedling exhibit high tolerance to Cd and Zn (Wang et al., 2017). Its homologous gene, rice OsNRAMP3, encoded a plasma membrane-localized protein, functions as a switch in response to environmental Mn changes, but does not transport Cd, Fe, and Zn (Yamaji et al., 2013; Yang et al., 2013). These results indicate that OsNRAMP3, as a Mn-specific transporter, only mediates Mn distribution in rice. Meanwhile, NRAMPs in different species and different NRAMPs in the same species exhibit different functions for transport of different substrates. Thus, we hypothesized that the function of TpNRAMP3 would differ from those of reported NRAMPs from other species. To test this hypothesis, expression pattern, subcellular localization, and transport activities toward metals (including Cd, Mn, Co, Fe, and Zn) of TpNRAMP3 were investigated.
Materials and Methods
RNA Sample Collection, Isolation, and cDNA Synthesis
For expression pattern of TpNRAMP3 grown in normal wheat growth season, 5 (root, basal stem, leaf sheath, leaf blade, and young leaf), 11 (root, basal stem, low leaf sheath, low leaf blade, node III, inter node II, node II, node I, flag leaf sheath, flag leaf blade, and peduncle), and 7 tissues (root, node I, flag leaf sheath, flag leaf blade, inter node I, lemma, and immature grain) were individually collected at jointing, booting, and grain filling stages with three biological replicates.
For response to metal stresses grown in Hoagland nutrient solution in a growth chamber at 25°C with 16/8 h light/dark, seedlings of DPW at the three-leaf stage were treated with 8 mM MgCl2 (Mg), 8 mM ZnSO4 (Zn), 8 mM FeCl3 (Fe), 8 mM CuCl2 (Cu), 40 μM CdSO4 (Cd), 40 μM PbCl2 (Pb), or 40 μM NiCl2 (Ni) for 24 h. Roots and leaves were separately collected (three biological replicates).
All collected samples were snap-frozen in liquid nitrogen and stored at -80°C. The Total RNA Kit II (Omega, United States) was used to isolate total RNA of each sample. Meanwhile, cDNA was synthesized from 2 μg total RNA using the M-MLV First Strand cDNA Synthesis Kit (Omega, United States).
TpNRAMP3 Cloning
PCR primers (F: 5′-TCATTGGGAGAGAGTGAGCAT-3′; R: 5′-ACATATCTAGTTT CCTCGCTGC-3′) for the full-length cDNA of TpNRAMP3 were designed according to DPW transcript (Wang et al., 2016). PCR volume included 2.5 μl 10× buffer, 2.0 μl MgCl2 (25 mM), 2.0 μl dNTP mixture (2.5 Mm each), 2.0 μl forward primer (4 pM), 2.0 μl reverse primer (4 pM), 3.0 μl cDNA, 0.5 μl ExTaq, and added sterilized distilled water up to 25 μl. PCR conditions were 95°C for 3 min, 30 cycles (95°C for 30 s, 60°C for 30 s, 72°C for 3 min), 72°C for 10 min, and 4°C hold. The amplified fragment was cloned into the pMD19-T vector for sequencing, and the results were analyzed with Invitrogen Vector NTI 11.5.1 (Invitrogen, United States).
Bioinformatics and Phylogenetic Analysis
Amino acid sequence, gene structure and chromosome localization, putative subcellular localization, transmembrane (TM) domains and phylogenetic analysis of TpNRAMP3 were performed as described by Peng et al. (2018). Protein structures of TpNRAMP3 and OsNRAMP3 were predicted using I-TASSER1, which used the crystal structure of EcoNRAMP (from Eremococcus coleocola) as a model (Ehrnstorfer et al., 2017). The CIS regulatory elements of the promoters of TpNRAMP3 and OsNRAMP3 were predicted using PlantCARE2.
Expression Analysis of TpNRAMP3
Quantitative real-time PCR with TpNRAMP3-specific primers (forward: 5′-ACTCTGATGCTCCTGTTCCT-3′; reverse: 5′-GCCTCGCACAACTTCTGAA-3′) was performed as described by Wang et al. (2015) with nine technological replicates of each sample. The actin gene (Wang et al., 2015) was used as a reference gene to normalize relative expression level of TpNRAMP3 which was calculated by CFX Manager 3.1 (Bio-Rad, United States) using the 2ΔΔCt method.
Expression in Yeast
The open reading frame of TpNRAMP3 was inserted into the pYES2 vector at the BamHI and EcoRI sites. The recombinant plasmid and the empty vector were transformed into different yeast strains including wild type (WT) BY4743, Cd-sensitive mutant Δycf1, Co-sensitive mutant YK40 and Zn-sensitive mutant Δzrc1 as described by Peng et al. (2018). The positively transformed yeasts were selected on SD solid medium (6.7 g/L yeast nitrogen base without amino acids, 1.92 g/L yeast synthetic drop-out medium supplements, 20 g/L bacteriological agar and 20 g/L glucose) with ampicillin, and were confirmed by PCR using TpNRAMP3-specific primers.
Positively transformed yeast cells were grown in liquid SD medium at 30°C for 16 h. Each cell suspension was diluted to OD600 = 0.8 and then subjected to four sequential dilutions (1:10, 1:100, 1:1000, and 1:10000). Five microliters of each diluted cell suspension was spotted on plates of SD medium containing CdCl2 (0 or 80 μM), CoCl2 (0 or 200 μM), or ZnSO4 (0 or 4 mM), with 2% galactose. All plates were incubated at 30°C for 3 days to compare their sensitivity to Cd, Co, and Zn with three replicates of each metal stress.
To confirm metal tolerance, yeast growth (the OD600 value) was quantified at different times. 50 μl of positive yeast cells with OD600 = 0.8 were cultured in 10 mL SD liquid medium with 2% galactose and CdCl2 (0, 20, or 40 μM) or CoCl2 (0, 200, or 400 μM). For Cd sensitivity, OD600 values were observed at 0, 6, and 24 h; for Co sensitivity, OD600 values were observed at 0, 12, and 36 h. All values were measured using a microplate spectrophotometer (Fisher Scientific, United States) with three replicates.
Meanwhile, Cd and Co concentration of yeast was measured to understand whether TpNRAMP3 transports Cd or Co in yeast. Positively transformed cells were grown in SD liquid medium with CdCl2 (20 μM) for 48 h or CoCl2 (50 μM) for 60 h. Metal concentration of each sample was determined by inductively coupled plasma-mass spectrometry (ICP-MS, Fisher Scientific, United States) as described by Peng et al. (2018) with three biological replicates.
Subcellular Localization of TpNRAMP3 in Arabidopsis Protoplasts
The open reading frame of TpNRAMP3 was sub-cloned into the Arabidopsis protoplast expression vector HBT95-green fluorescence protein (GFP) at the BamHI and SpeI sites under the 35S promoter. As described by Yoo et al. (2007), Arabidopsis mesophyll protoplast was prepared and transformed. Meanwhile, the plasma membrane marker, RFP-SCAMP1 (Cai et al., 2011), was used to confirm the subcellular localization. A confocal laser scanning microscope (Olympus, Japan) was used to detect GFP and RFP signals.
Overexpression of TpNRAMP3 in Arabidopsis thaliana
The open reading frame of TpNRAMP3 was sub-cloned into the pCAMBIA1305.1 vector at the BamHI and SalI sites under the CaMV35s promoter. The recombined vector and the empty vector were individually introduced into Agrobacterium, and then transformed into WT Arabidopsis thaliana plants using floral infiltration (three time-independent transformations). The positive and homozygous lines were selected using hygromycin selection and PCR with TpNRAMP3-specific primers. The overexpression level was analyzed as described as the section of “Expression analysis of TpNRAMP3.”
To test metal tolerance of TpNRAMP3 in transformed seedlings, the WT, an empty vector line and two independently homozygous lines (each line contained three plant lines selected from an independent transformation; totally six plant lines) were seeded on 1/2 MS solid plates containing 25 μM CdCl2, 80 μM CoCl2, or 500 μM MnCl2. After 4°C treatment for 24 h, the plates were placed in a light incubator with 120 μE m-2 s-1 illumination intensity, a 16/8 h light/dark period, 22°C temperature, and 50% humidity. On the 10th day, the root lengths of the treated plants were determined with three biological replicates.
To investigate metal transport properties of TpNRAMP3 in plant, the WT, an empty vector line and two independent homozygous lines were germinated on 1/2 MS medium. Seedlings were transplanted into soil-filling plot at the four-leaf stage (12 plots of each WT and empty line; 36 plots of each independent homozygous line). After 3 weeks, 100 mg CdCl2 or CoCl2 was added to 2.5 kg soil (four plots of each metal stress of each line). At the mature stage, roots and aerial parts were individually collected and dried at 60°C for 48 h with three biological replicates. Dry weight of each plant was calculated using the formula [(root dry weight + aerial part dry weight)/collected plant number]. All dried samples were digested in 80% nitric acid at 220–280°C, and diluted in deionized water. Metal concentration of each sample was determined using ICP-MS (Fisher Scientific, United States). Translocation factor (TF), representing metal translocation from roots to shoots, was calculated by the shoot-to-root concentration ratio.
Data Analysis
Differences of samples or treatments were analyzed using SPSS 20.0 with Tukey’s test at P ≤ 0.05. All figures were drawn using Sigmaplot 12.0.
Results
Cloning and Phylogenetic Analysis of TpNRAMP3
The full-length cDNA of TpNRAMP3 (KX165384) including a 19-bp 5′-UTR, 1644-bp open reading frame, and 36-bp 3′-UTR was successfully amplified. It encoded 548 amino acids (ANT73691), which had 90.0% identity to OsNRAMP3, 72.1% identity to AtNRAMP1 and 71.2% identity to AtNRAMP6 (Supplementary Figure S1). Blasting the cDNA of TpNRAMP3 against the wheat genome (The International Wheat Genome Sequencing Consortium, 2014) revealed that TpNRAMP3 was localized on the chromosome 7BL (gene: TRIAE_CS42_7BL_TGACv1_576780_AA1854780). It comprised 12 introns and 13 exons. Compared with structure of OsNRAMP3, TpNRAMP3 had a longer α-helix in its C-terminus (Supplementary Figure S2), which implied that its function might differ from that of OsNRAMP3.
TpNRAMP3 Expression
Before investigation of the expression pattern of TpNRAMP3, we compared the promoters of TpNRAMP3 and OsNRAMP3 to search for distinct CIS regulatory elements. Ten TpNRAMP3-specific and five OsNRAMP3-specific CIS regulatory elements were individually predicted from the promoters of TpNRAMP3 and OsNRAMP3 (Supplementary Table 1). These distinct CIS regulatory elements between the promoters of TpNRAMP3 and OsNRAMP3 implied that the expression pattern of TpNRAMP3 might differ from OsNRAMP3.
The expression pattern of TpNRAMP3 was investigated in different tissues at three growth stages of wheat grown in a field. At the jointing stage, TpNRAMP3 showed the highest expression in leaf blades, followed by roots and new leaves, and the lowest expression in basal stems and leaf sheaths (Figure 1A). At the booting stage, the expression of TpNRAMP3 was highest in old leaf blades, followed by old leaf sheaths and flag leaf blades, then in roots and flag leaf sheaths, and lowest in immature spikes (Figure 1A). At the grain filling stage, TpNRAMP3 expression was highest in the first nodes (node I) and lowest in roots and grains (Figure 1A).
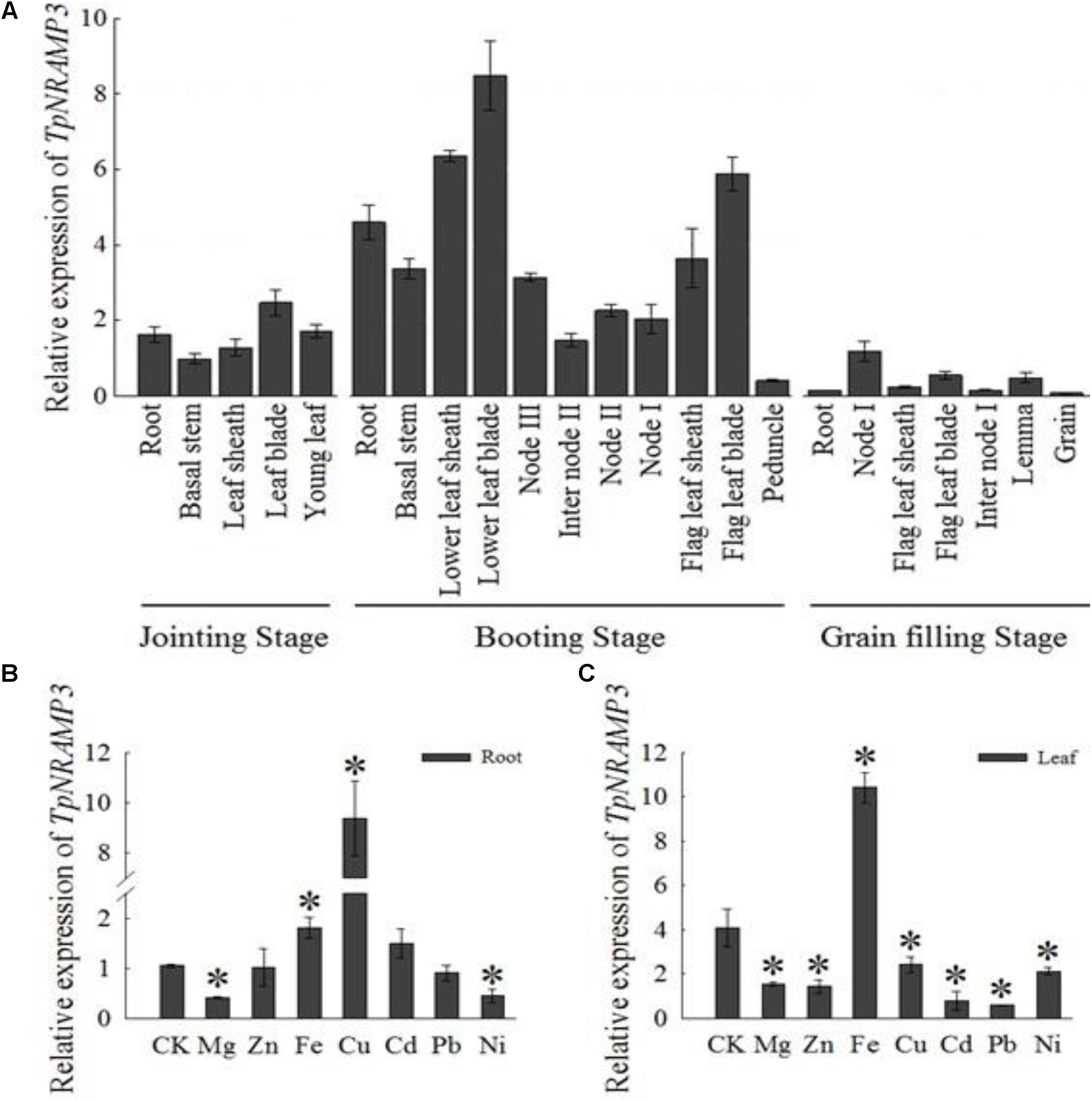
FIGURE 1. Expression pattern of TpNRAMP3. (A) At different growth stages, the relative expression of TpNRAMP3 in various tissues that were collected from DPW grown in a field at natural wheat growth season (B,C): the relative expression of TpNRAMP3 in roots (B) and shoots (C) that treated with Mg, Zn, Fe, Cu, Cd, Pb, or Ni for 24 h. Asterisk indicated significant difference from control at P < 0.05 by Tukey’s test with three independently biological replicates. Jointing stage: the basal stem emerges above the soil line; booting stage: the developing head with the sheath of the flag leaf becomes visibly enlarged; internode I: the first internode below the spike; internode II: the second internode below the spike; node I: the node with the flag leaf; node II: the first node below the node I; node III: the second node below the node I. CK was treated without supplementation with metals.
We also investigated the responses of TpNRAMP3 to supplementation with metals including Mg, Zn, Fe, Cu, Cd, Pb, and Ni. In the roots, TpNRAMP3 expression was significantly down-regulated by Mg and Ni, and up-regulated by Fe and Cu, but not affected by Zn, Cd, or Pb (Figure 1B). However, TpNRAMP3 expression was affected by all metal stresses in the shoots (Figure 1C).
Subcellular Localization of TpNRAMP3
TpNRAMP3 was predicted to be a plasma membrane protein with 11 TM domains (Supplementary Figure S3). To confirm the subcellular localization of TpNRAMP3, a TpNRAMP3-GFP fusion protein and a plasma membrane marker were transiently co-transformed into Arabidopsis leaf protoplasts. Green fluorescence from the empty vector (HBT95) was localized in the cytosol, nucleus, and plasma membrane (Wang et al., 2018). However, green fluorescence from the fusion protein (HBT95-TpNRAMP3-GFP) was mostly merged with the red fluorescence of the membrane marker, which indicated that TpNRAMP3 was localized at the plasma membrane (Figure 2).
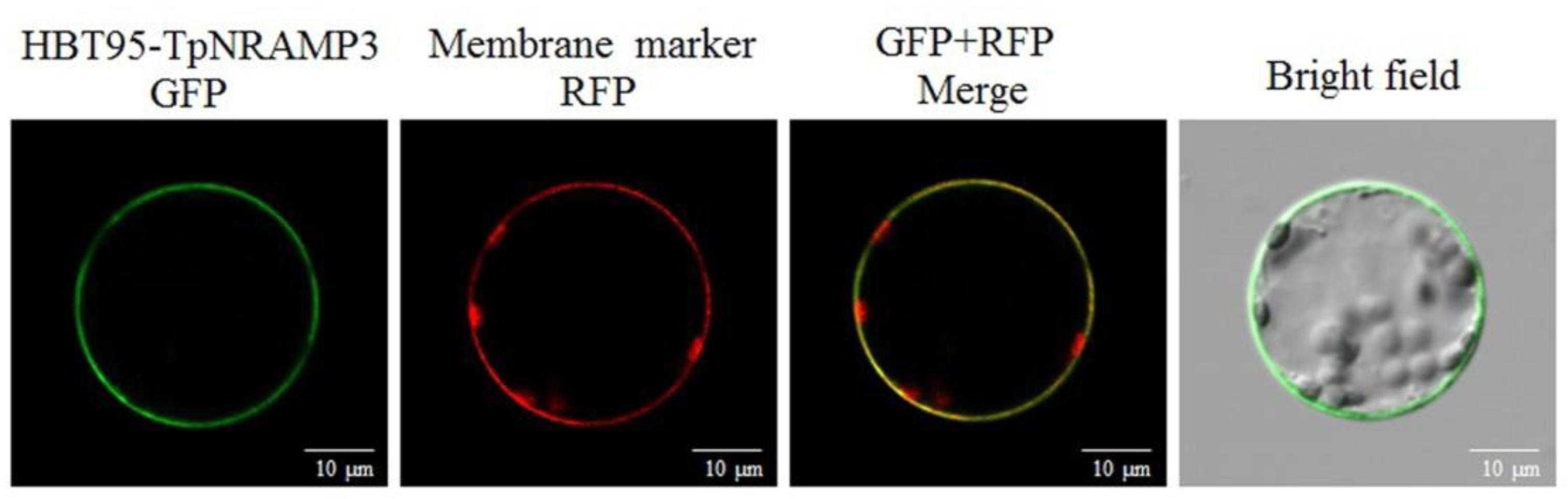
FIGURE 2. Subcellular localization of TpNRAMP3. A plasma membrane marker (RFP-SCAMP1) was used in this study. TpNRAMP3 was localized at the plasma membrane.
Metal Transport in Yeast
To understand TpNRAMP3’s function, we firstly expressed TpNRAMP3 in Cd-, Co-, and Zn-sensitive mutant yeasts for metal transport assays. In the presence of galactose, no growth difference was observed among the transformed yeasts under 0 μM Cd stress (Figures 3A,B). The expression of TpNRAMP3 significantly inhibited the growth of Δycf1 strains compared with Δycf1 strains transformed with pYES2 under Cd stress (Figure 3A). The growth inhibition induced by TpNRAMP3 was confirmed by the growth curves under 20 and 40 μM CdCl2 stresses (at 6 and 24 h) (Figure 3B). Furthermore, the Cd concentrations in the yeast cells were investigated. Δycf1 cells expressing TpNRAMP3 had significantly higher Cd concentration than cells transformed with pYES2 (Figure 3C). Similar results were observed under Co stress (Figure 4). The expression of TpNRAMP3 significantly inhibited the growth of YK40 strains whether they were grown on SD plates with 200 μM Co (Figure 4A) or SD liquid medium with 200 or 400 μM Co (Figure 4B). TpNRAMP3 expression also significantly increased Co concentration under 50 μM Co stress compared with yeast cells transformed with pYES2 (Figure 4C). These results indicated that TpNRAMP3 is a Cd and Co transporter. However, Zn did not affect yeast growth regardless of whether the cells were expressing TpNRAMP3 or transformed with pYES2 (Supplementary Figure S4).
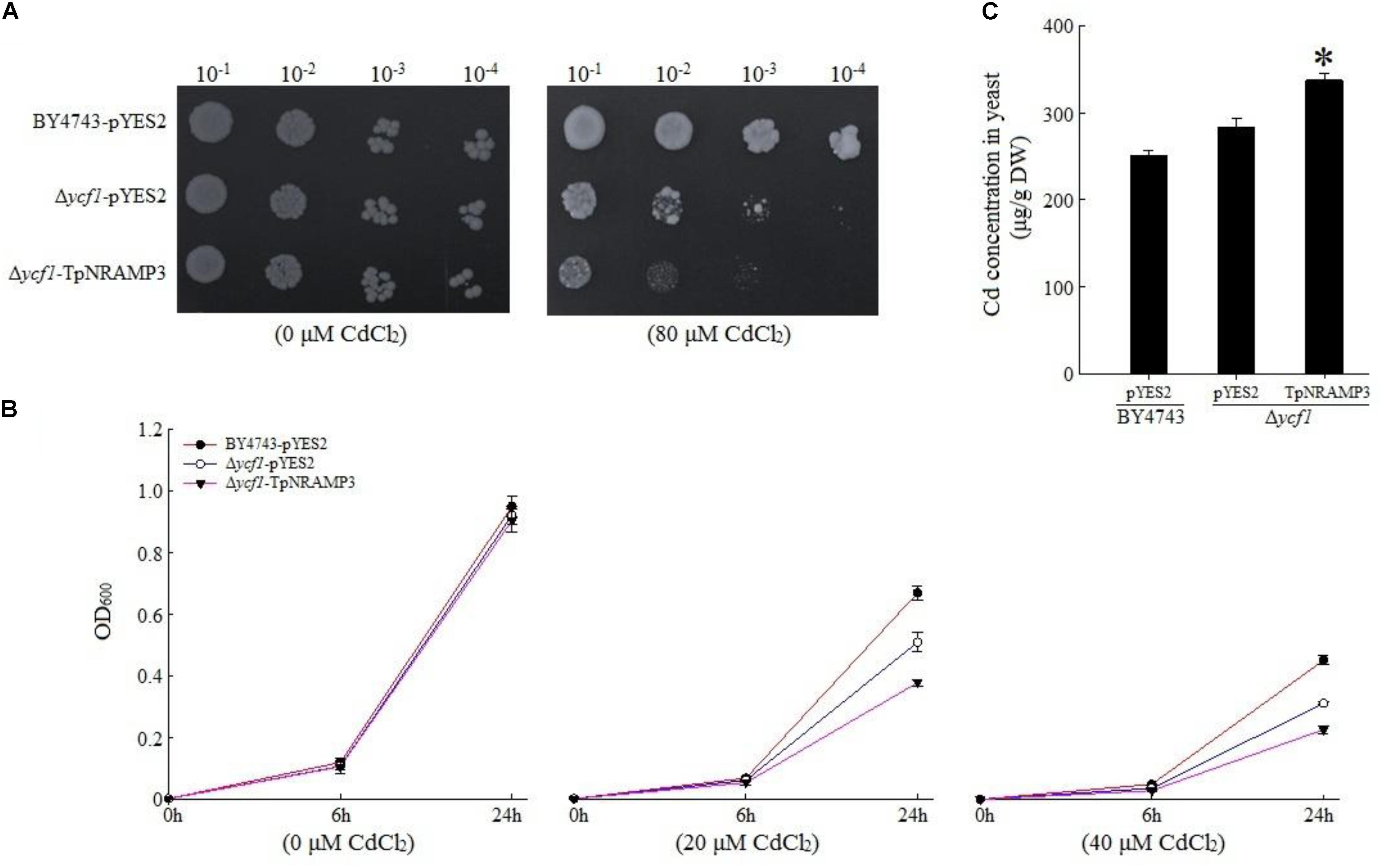
FIGURE 3. Sensitivity to Cd and Cd concentration in yeast. (A) Sensitivity to Cd when grown on plate SD medium with 80 μM CdCl2 (B) sensitivity to Cd when grown in liquid SD medium with 20 and 40 μM CdCl2; (C) Cd concentration in yeast when grown in SD medium with 20 μM CdCl2 for 48 h. Asterisk indicated significant difference when compared with WT at P < 0.05; value was mean ± standard deviation (three biological replicates).
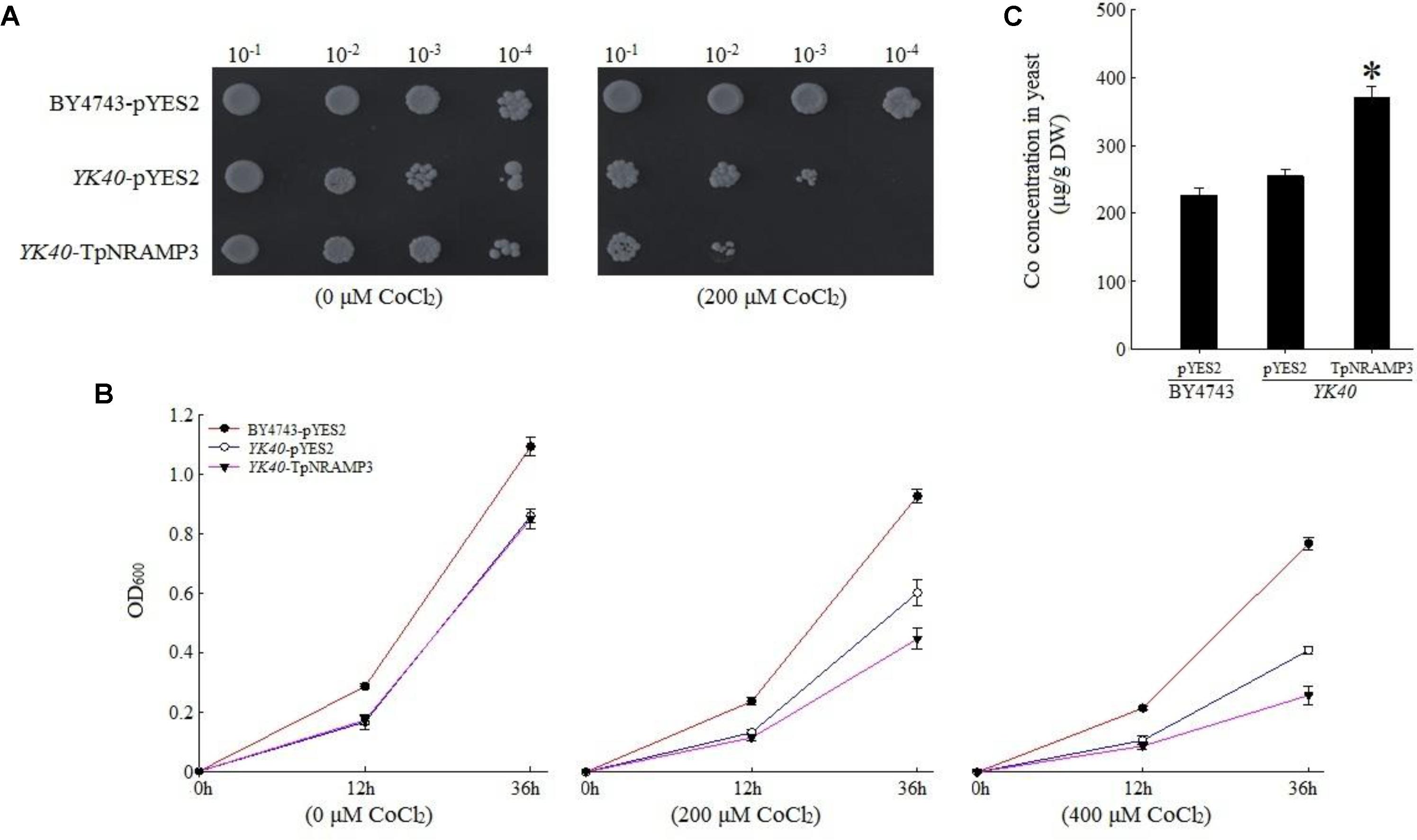
FIGURE 4. Sensitivity to Co and the Co concentration in yeast. (A) Sensitivity to Co when grown on plate SD medium with 200 μM CoCl2; (B) sensitivity to Co when grown in liquid SD medium with 200 and 400 μM CdCl2; (C) Co concentration in yeast when grown in SD medium with 50 μM CoCl2 for 60 h. Asterisk indicated significant difference when compared with WT at P < 0.05; value was mean ± standard deviation (three biological replicates).
Functional Analysis of TpNRAMP3 in Arabidopsis
To determine whether TpNRAMP3 transports Cd and Co, or other metals such as Fe and Mn in plants, we expressed TpNRAMP3 in Arabidopsis under the 35S promoter. Two independent homozygous overexpression lines were developed (Supplementary Figure S5A). Under normal growth conditions, expression of TpNRAMP3 increased plant growth compared with the WT and empty vector line (Figure 5A). Under 500 μM MnCl2 stress, expression of TpNRAMP3 dramatically increased seedling root lengths (Figure 5B). Under 25 μM CdCl2 and 80 μM CoCl2 stresses, expression of TpNRAMP3 did not affect root lengths (Supplementary Figures S5B,C). However, whether plants were under 40 mg/Kg CoCl2 stress or not, expression of TpNRAMP3 slightly increased dry weight per plant (Figure 5C). Although 40 mg/kg CdCl2 did not cause Cd-toxicity in the WT and empty vector line, it did cause Cd-toxicity (red speckles on the leaves, Figure 5D) and reduce the dry weight per plant in TpNRAMP3-expressing lines compared with their individual controls (CK) (Figure 5E).
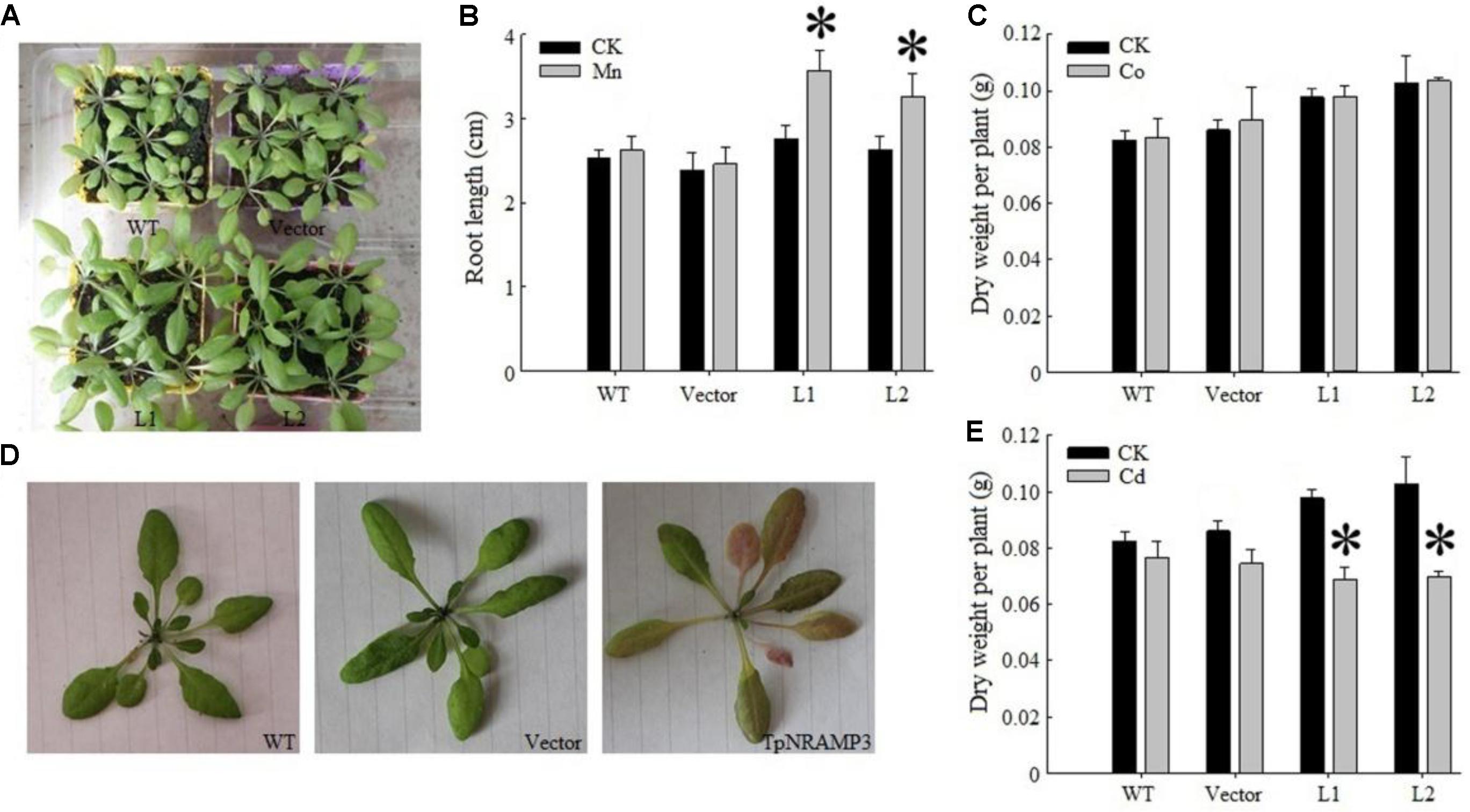
FIGURE 5. Phenotypes of TpNRAMP3-expressing Arabidopsis treated with different metals. (A) Expression of TpNRAMP3 slightly improved the plant growth grown in soil for 4 weeks; (B) expression of TpNRAMP3 significantly increased the length of seedling root on 1/2 MS medium with 500 μM MnCl2 for 10 days; (C) expression of TpNRAMP3 did not affect the dry weight of adult plant when grown in soil with 40 mg/kg Co stress; (D,E) 40 mg/kg Cd caused the Cd toxicity in the leaves (D) and reduced the dry weight of adult plant (E) of TpNRAMP3-expressing lines. Asterisk indicated significant difference when compared with their individual control (CK) at P < 0.05; value was mean ± standard deviation (three biological replicates).
Under 40 mg/kg CdCl2 stress, expression of TpNRAMP3 significantly increased Cd concentration in roots (Figure 6A), shoots (Figure 6B) and whole plant (Figure 6C) compared with the WT and empty vector line. However, it did not affect Cd translocation from roots to shoots (Figure 6D). Under 40 mg/kg CoCl2 stress, expression of TpNRAMP3 significantly increased Co concentration in roots (Figure 7A), shoots (Figure 7B), and whole plant (Figure 7C), but did not affect Co translocation from roots to shoots (Figure 7D). Under normal growth conditions, expression of TpNRAMP3 also dramatically increased Mn concentration in roots (Figure 8A), shoots (Figure 8B), and whole plant (Figure 8C), but did not affect Mn translocation from roots to shoots (Figure 8D). However, expression of TpNRAMP3 did not affect Fe or Zn concentration in roots (Supplementary Figures S6A,C) or shoots (Supplementary Figures S6B,D). These results indicated that TpNRAMP3 is a metal transporter for Cd, Co, and Mn accumulation, but not for Fe or Zn accumulation.
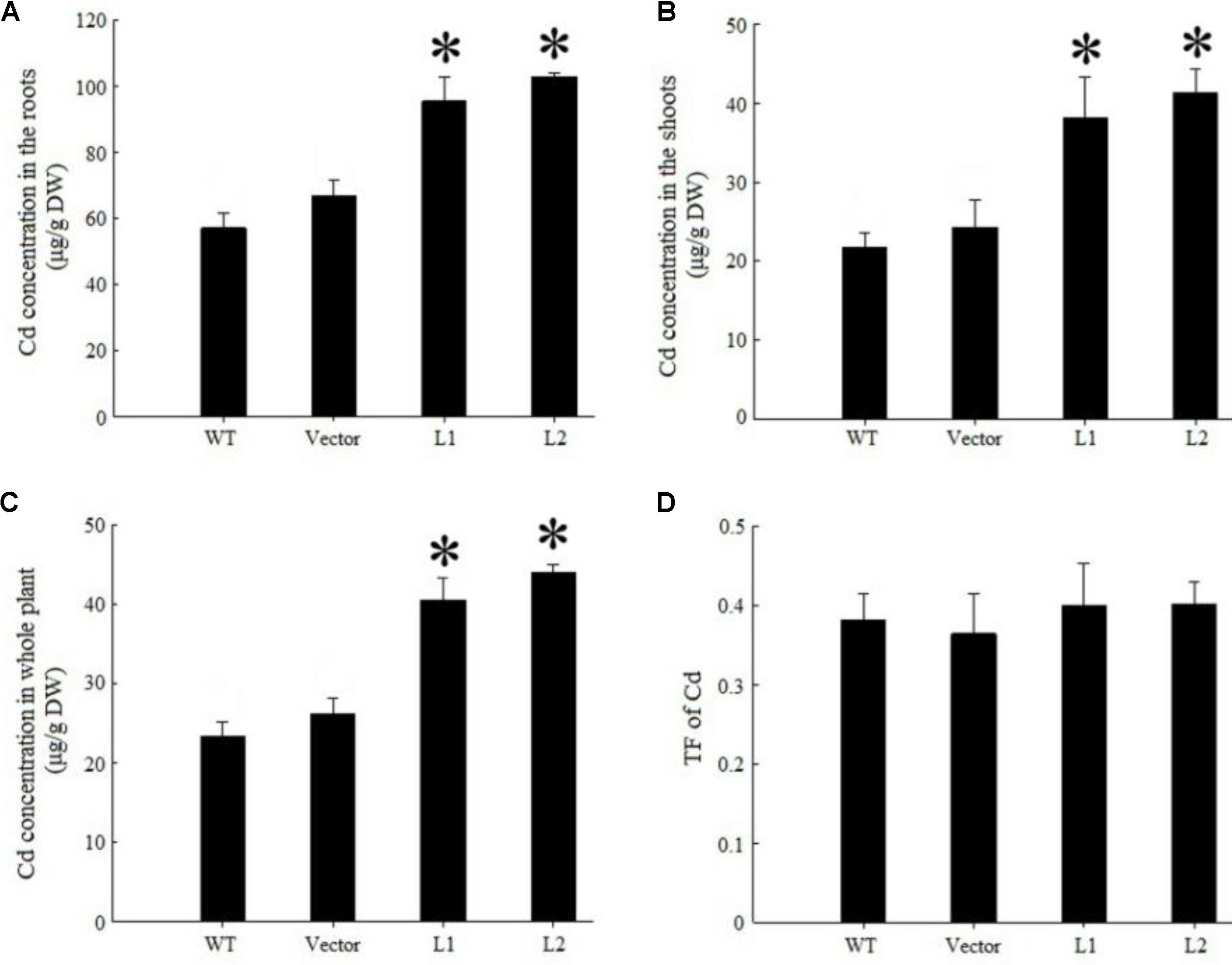
FIGURE 6. Cd concentration and translocation factor of TpNRAMP3-expressing Arabidopsis. (A–C) Cd concentration in roots, shoots, and whole plant, respectively; (D) Cd translocation factor (TF) represented Cd translocation from roots to shoots. Asterisk indicated significant difference when compared with WT at P < 0.05; value was mean ± standard deviation (three biological replicates).
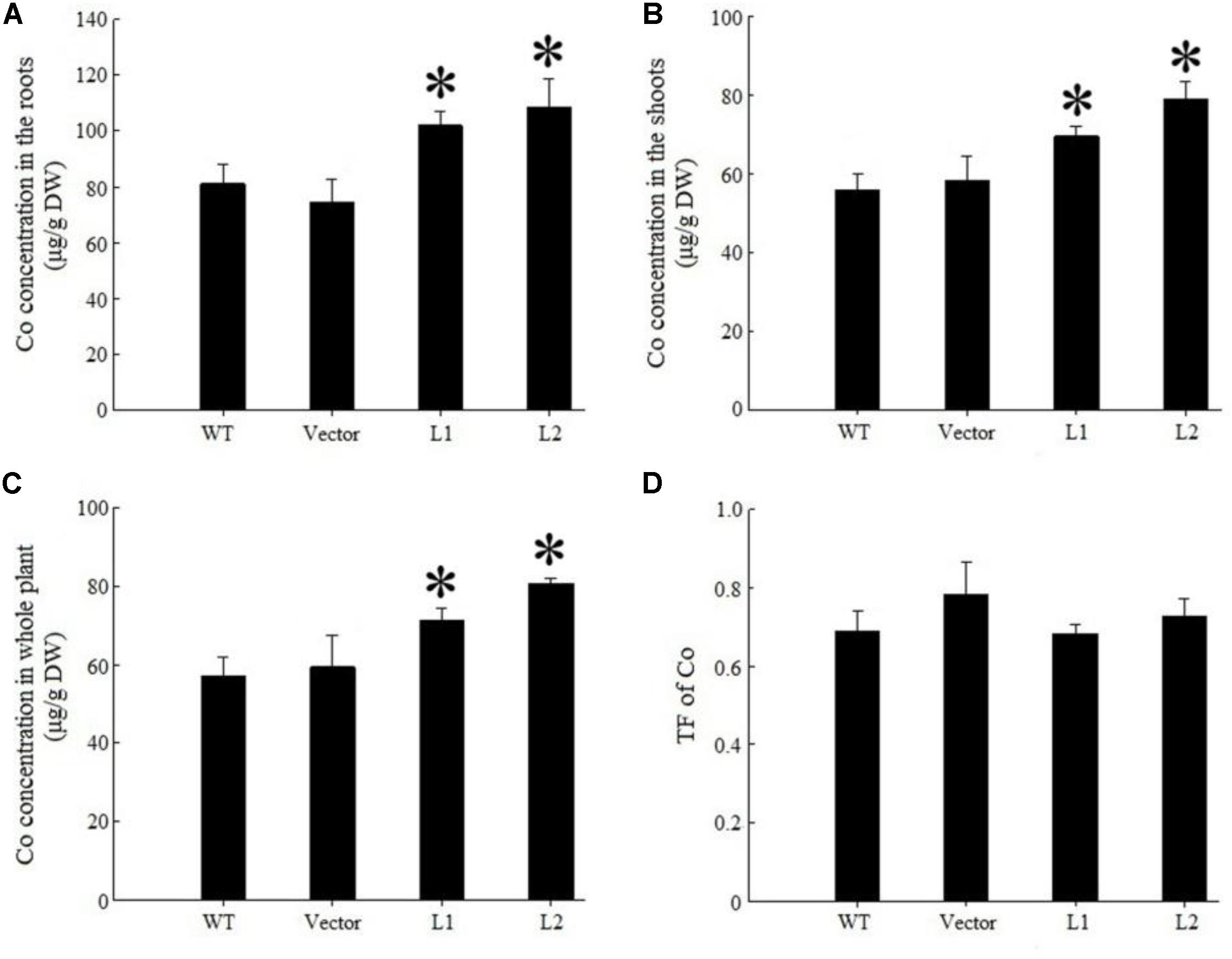
FIGURE 7. Co concentration and TF of TpNRAMP3-expressing Arabidopsis. (A–C) Co concentration in roots, shoots, and whole plant, respectively; (D) Co TF. Asterisk indicated significant difference when compared with WT at P < 0.05; value was mean ± standard deviation (three biological replicates).
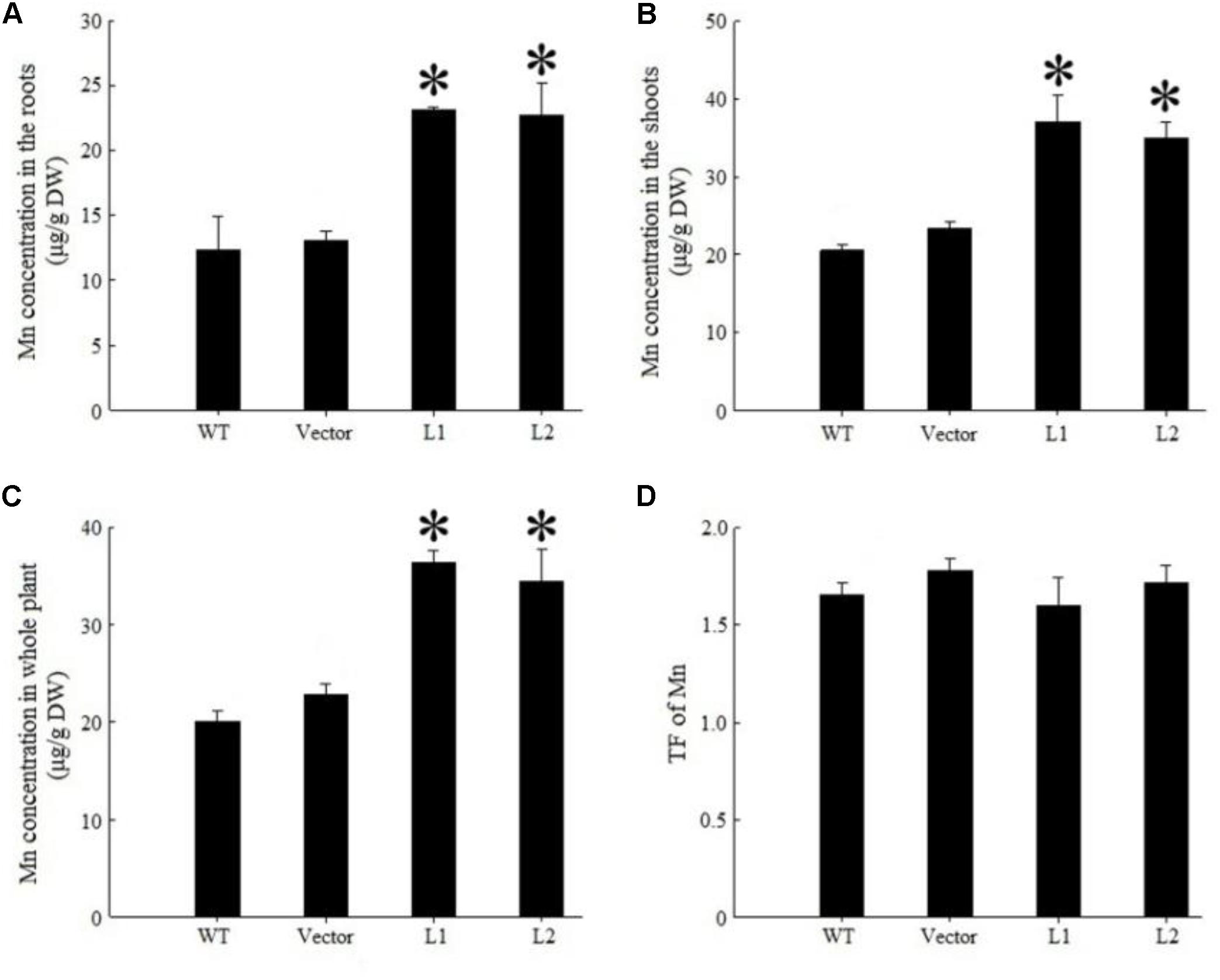
FIGURE 8. Mn concentration and TF of TpNRAMP3-expressing Arabidopsis. (A–C) Mn concentration in roots, shoots, and whole plant, respectively; (D) Mn TF. Asterisk indicated significant difference when compared with WT at P < 0.05; value was mean ± standard deviation (three biological replicates).
Discussion
TpNRAMP3 Is a Metal Transporter for Co, Mn, and Cd Accumulation, but Does Not Transport Fe or Zn
In the present study, we found that TpNRAMP3 is a Co, Mn, and Cd transporter, and is potentially responsible for Co, Mn, and Cd accumulation in wheat. This conclusion is based on the following evidence: (1) although TpNRAMP3 was mainly expressed in leaf blade at the jointing stage, and older leaf blade and sheath, and flag leaf blade at the booting stage, it was also highly expressed in roots at these stages (Figure 1A); (2) TpNRAMP3 was localized at the plasma membrane (Figure 2); (3) expression of TpNRAMP3 increased sensitivity to Co and Cd, and increased Co and Cd accumulation in yeast (Figures 3, 4); (4) expression of TpNRAMP3 in Arabidopsis increased Cd (Figure 6), Co (Figure 7) and Mn (Figure 8) concentrations in roots, shoots and whole plant, and caused Cd-toxicity in leaves (Figure 5D) and reduced dry weight (Figure 5E). However, expression of TpNRAMP3 did not alter Fe or Zn concentration in Arabidopsis (Supplementary Figure S6) or Zn sensitivity in yeast (Supplementary Figure S4).
Mn is an essential micronutrient element for plant growth and development (Socha and Guerinot, 2014). It is involved in many processes, such as photosynthesis, respiration, protein synthesis, and hormone activation (Shao et al., 2017). However, the uptake, translocation and sequestration of Mn may share transporters with Fe. In rice, yellow stripe 1-like 2 (OsYSL2) is implicated in Fe and Mn transport (Koike et al., 2004). Expression of OsNRAMP6 showed Fe and Mn transport activity in yeast (Gao et al., 2018). Additionally, knockout of OsNRAMP5, which localizes to the root plasma membrane, reduced Fe and Mn uptake (Ishimaru et al., 2012; Sasaki et al., 2012). In Arabidopsis, AtNRAMP1 localizes at the plasma membrane and is responsible for Mn uptake (Cailliatte et al., 2010) and Fe homoeostasis (Curie et al., 2000). In this study, expression of TpNRAMP3 in Arabidopsis dramatically increased Mn accumulation in roots (Figure 8A), shoots (Figure 8B), and whole plant (Figure 8C), reasonably explaining why expression of TpNRAMP3 promoted the growth of plants in normal soils (Figure 5A) and on MS medium with 500 μM MnCl2 (Figure 5B). However, expression of TpNRAMP3 in Arabidopsis did not alter Fe concentration in roots or shoots (Supplementary Figures S6A,B). Mn accumulation therefore has its own transporter in wheat, and does not share this transporter with Fe.
Co is a non-essential nutrient for plants, which is taken up from soil by IRT1 in Arabidopsis (Korshunova et al., 1999; Barberon et al., 2014). The absorbed Co is sequestrated in root vacuoles by ferroportins IRON REGULATED2 (IREG2/FPN2) (Morrissey et al., 2009) and AtHMA3 (Morel et al., 2009). Additionally, it is loaded into the xylem by IREG1/FPN1 and transported to the shoot (Morrissey et al., 2009; Lange et al., 2017). IRT1, IREG1/FPN1, and IREG2/FPN2 also function as Fe transporters; therefore, the uptake and translocation of Co is also Fe-regulated (Morrissey et al., 2009). In the present study, although TpNRAMP3 increased Co accumulation in yeast (Figure 4C) and Arabidopsis (Figures 7A–C), it did not alter Co translocation (Figure 7D), and did not change Fe or Zn concentration in Arabidopsis (Supplementary Figure S6). Thus, its function is different from those of IREG2/FPN2 (Morrissey et al., 2009), AtHMA3 (Morel et al., 2009), IRT1 (Korshunova et al., 1999; Barberon et al., 2014), and IREG1/FPN1 (Morrissey et al., 2009; Lange et al., 2017).
Cd is also a toxic heavy metal that affects plant growth and development. Theoretically, no specific transporter is responsible for Cd uptake and translocation (Sasaki et al., 2012). Because of the similar physical and chemical characteristics between Cd and essential metals including Zn and Fe (Chesworth, 1991), they may share transporters (Sasaki et al., 2012). For example, OsNRAMP1 and OsNRAMP5 transport Fe and Cd in plants and/or yeast (Takahashi et al., 2011; Ishimaru et al., 2012; Sasaki et al., 2012; Takahashi et al., 2014). However, some transporters that transport Cd but not Fe have been discovered, such as AtNRAMP6 (Peris-Peris et al., 2017) and HvNRAMP5 (Wu et al., 2016). In this study, we found that TpNRAMP3 was a Cd transporter responsible for Cd accumulation (Figures 3C, 6), but did not accumulate Fe or Zn (Supplementary Figure S6). Its function is different from that of HvNRAMP5, because HvNRAMP5 is mainly expressed in the roots (Wu et al., 2016).
Wheat flour is the main contributor to the average daily dietary intake of Cd (Greger and Löfstedt, 2004). Therefore, limiting Cd accumulation in wheat grains is necessary to protect human health. Cd accumulation in wheat grains is controlled by the root uptake of Cd, the translocation of Cd from the roots to shoots, and the distribution of Cd in the leaves and grain coat (Greger and Löfstedt, 2004). Thus, reducing Cd uptake is the first and most important step to reduce Cd accumulation in wheat grains. Since TpNRAMP3 potentially increases Cd and Mn accumulation in wheat, knockout or knockdown of TpNRAMP3 will reduce Cd transport from the soil to wheat grains in the future.
TpNRAMP3 and OsNRAMP3 Have Different Expression Patterns and Metal Transport Functions
There was 90% identity of amino acid sequence between TpNRAMP3 and OsNRAMP3, which implied that functional divergence between TpNRAMP3 and OsNRAMP3. Previous studies indicated that OsNRAMP3 was mainly expressed in nodes, culms, and basal stems, with very low expression in roots and leaf blades and sheaths (Yamaji et al., 2013; Yang et al., 2013). Additionally, the expression of OsNRAMP3 was unaffected by Fe, Zn, Cu, or Mn deficiency (Yamaji et al., 2013; Yang et al., 2013). In this study, TpNRAMP3 was mainly expressed in leaf blades, leaf sheaths, and roots, but had very low expression in nodes and internodes at the jointing and booting stages (Figure 1A). Interestingly, TpNRAMP3 was mainly expressed in the first node at the grain filling stage (Figure 1A). Additionally, the expression of TpNRAMP3 was regulated by many metals including Fe, Zn, Cu, Pb, Ni, Cd, and Mg (Figure 1B). Thus, the expression patterns of OsNRAMP3 and TpNRAMP3 differ, which might be results of distinct sequences (Supplementary Figure S3), CIS regulatory elements of the promoters (Supplementary Table 1), and/or species.
In rice, OsNRAMP3 functions as a switch in response to environmental Mn changes (Yamaji et al., 2013). At low Mn concentrations, OsNRAMP3 transports Mn to young leaves and panicles; however, at high Mn concentrations, Mn transport to old leaves is caused by OsNRAMP3 degradation (Yamaji et al., 2013). Thus, OsNRAMP3 is a Mn transporter responsible for Mn distribution and contributes to the remobilization of Mn from old to young leaves (Yamaji et al., 2013; Yang et al., 2013). Although the amino acid sequence of TpNRAMP3 has high identity (90%) to that of OsNRAMP3 (Supplementary Figure S1), our results suggest their metal transport functions are different. This conclusion is supported by the following: (1) TpNRAMP3 functions as a Cd and Co transporter in yeast (Figures 3, 4) and Arabidopsis (Figures 6, 7), but OsNRAMP3 is not a Cd transporter (Yamaji et al., 2013); and (2) TpNRAMP3 expression increased Mn concentrations in roots (Figure 8A), shoots (Figure 8B), and whole plant (Figure 8C), while knockout of OsNRAMP3 did not affect Mn concentration in roots or shoots (Yamaji et al., 2013; Yang et al., 2013). These differences are probably caused by a few residues in the primary sequences of TpNRAMP3 and OsNRAMP3 (Supplementary Figure S3), because residue differences change the putative protein structures (Supplementary Figure S2) and can alter metal transport substrates, such as in AtNRAMP4 (Pottier et al., 2015) and NtNRAMP5 (Tang et al., 2017).
Conclusion
TpNRAMP3 is localized on the chromosome 7BL. It was highly expressed in leaf blades and roots at the jointing and the booting stages, and in the first nodes at the grain filling stage. TpNRAMP3 was localized at the plasma membrane. The expression of TpNRAMP3 was significantly down-regulated by Mg and Ni, and up-regulated by Fe and Cu in seedling roots, but not affected by Zn, Cd, or Pb. In yeast, TpNRAMP3 functions as a metal transporter for Cd and Co accumulation, but not Zn. Expression of TpNRAMP3 in Arabidopsis showed that TpNRAMP3 is a metal transporter for Cd, Co, and Mn accumulation. Thus, the function of TpNRAMP3 is different from that of OsNRAMP3, which functions as a Mn switch (Yamaji et al., 2013; Yang et al., 2013), OsNRAMP1, which is involved in Cd (but not Mn) uptake and translocation from roots to shoots (Takahashi et al., 2011; Tiwari et al., 2014), and OsNRAMP5, which functions as a Cd, Mn, and Fe transporter (Ishimaru et al., 2012; Sasaki et al., 2012; Takahashi et al., 2014).
Author Contributions
FP, CW, and YC conducted all the experiments. YW, FP, YZ, and JZ designed the manuscript. FP, CW, YW, HK, XF, and HZ analyzed the data. FP, CW, YW, YZ, YC, and LS drafted and revised the manuscript.
Funding
The authors thank the National Natural Science Foundation of China (Nos. 31671688 and 31470305), Bureau of Science and Technology of Sichuan Province, China, for all financial support.
Conflict of Interest Statement
The authors declare that the research was conducted in the absence of any commercial or financial relationships that could be construed as a potential conflict of interest.
Acknowledgments
The authors thank Prof. Yi Cai and associated Prof. Yan Huang (Sichuan Agricultural University) for supporting the plasmid of RFP-SCAMP1 and the technological direction of subcellular localization analysis.
Supplementary Material
The Supplementary Material for this article can be found online at: https://www.frontiersin.org/articles/10.3389/fpls.2018.01354/full#supplementary-material
Footnotes
- ^ https://zhanglab.ccmb.med.umich.edu/I-TASSER/
- ^ http://bioinformatics.psb.ugent.be/webtools/plantcare/html/
References
Balen, B., Tkalec, M., Šikić, S., Tolić, S., Cvjetko, P., Pavlica, M., et al. (2011). Biochemical responses of Lemna minor experimentally exposed to cadmium and zinc. Ecotoxicology 20, 815–826. doi: 10.1007/s10646-011-0633-1
Barberon, M., Dubeaux, G., Kolb, C., Isono, E., Zelazny, E., and Vert, G. (2014). Polarization of Iron-Regulatied Transoprter 1 (IRT1) to the plant-soil interface plays crucial role in metal homeostasis. Proc. Natl. Acad. Sci. U.S.A. 111, 8293–8298. doi: 10.1073/pnas.1402262111
Cai, Y., Jia, T., Lam, S. K., Ding, Y., Gao, C., San, M. W. Y., et al. (2011). Multiple cytosolic and transmembrane determinants are required for the trafficking of SCAMP1 via an ER-Golgi-TGN-PM pathway. Plant J. 65, 882–896. doi: 10.1111/j.1365-313X.2010.04469.x
Cailliatte, R., Lapeyre, B., Briat, J. F., Mari, S., and Curie, C. (2009). The NRAMP6 metal transporter contributes to cadmium toxicity. Biochem. J. 422, 217–228. doi: 10.1042/BJ20090655
Cailliatte, R., Schikora, A., Briat, J. F., Mari, S., and Curie, C. (2010). High-affinity manganese uptake by the metal transporter NRMAP1 is essential for Arabidopsis growth in low manganese conditions. Plant Cell 22, 904–917. doi: 10.1105/tpc.109.073023
Chesworth, W. (1991). “Geochemistry of micronutrients,” in Micronutrientsin Agriculture, ed. R. M. Welch (Madison: Soil Science Society of America), 1–30.
Curie, C., Alonso, J. M., Le Jean, M., Ecker, J. R., and Briat, J. F. (2000). Involvement of NRAMP1 from Arabidopsis thaliana in iron transport. Biochem. J. 347, 749–755. doi: 10.1042/bj3470749
Ehrnstorfer, I. A., Manatschal, C., Arnold, F. M., Laederach, J., and Dutzler, R. (2017). Structural and mechanistic basis of proton-coupled metal ion transport in the SLC11/NRAMP family. Nat. Commun. 8:14033. doi: 10.1038/ncomms14033
Gao, H., Xie, W., Yang, C., Xu, J., Li, J., Wang, H., et al. (2018). Nramp2, a trans-Golgi network-localized manganese transporter, is required for Arabidopsis root growth under manganese deficiency. New Phytol. 217, 179–193. doi: 10.1111/nph.14783
Greger, M., and Löfstedt, M. (2004). Comparison of uptake and distribution of cadmium in different cultivars of bread and durum wheat. Crop Sci. 44, 501–507. doi: 10.2135/cropsci2004.5010
Herbette, S., Taconnat, L., Hugouvieux, V., Piette, L., Magniette, M., Cuine, S., et al. (2006). Genome-wide transcriptome profiling of the early cadmium response of Arabidopsis roots and shoots. Biochimie 88, 1751–1765. doi: 10.1016/j.biochi.2006.04.018
Ishimaru, Y., Takahashi, R., Bashir, K., Shimo, H., Senoura, T., Sugimoto, K., et al. (2012). Characterizing the role of rice NRAMP5 in manganese, iron and cadmium transport. Sci. Rep. 2:286. doi: 10.1038/srep00286
Kaiser, B. N., Moreau, S., Castelli, J., Thomson, R., Lambert, A., Bogliolo, S., et al. (2003). The soybean NRAMP homologue, GmDMT1, is a symbiotic divalent metal transporter capable of ferrous iron transport. Plant J. 35, 295–304. doi: 10.1046/j.1365-313X.2003.01802.x
Koike, S., Inoue, H., Mizuno, D., Takahashi, M., Nakanishi, H., Mori, S., et al. (2004). OsYSL2 is a rice metal-nicotianamine transporter that is regulated by iron and expressed in the phloem. Plant J. 39, 415–424. doi: 10.1111/j.1365-313X.2004.02146.x
Korshunova, Y. O., Eide, D., Clark, W. G., Guerinot, M. L., and Pakrasi, H. B. (1999). The IRT1 protein from Arabidopsis thaliana is a metal transporter with a broad substrate range. Plant Mol. Biol. 40, 37–44. doi: 10.1023/A:1026438615520
Lahner, B., Gong, J., Mahmoudian, M., Smith, E. L., Abid, K. B., Rogers, E. E., et al. (2003). Genomic scale profiling of nutrient and trace elements in Arabidopsis thaliana. Nat. Biotechnol. 21, 1215–1221. doi: 10.1038/nbt865
Lange, B., van der Ent, A., Baker, A. J. M., Echevarria, G., Mahy, G., Malaisse, F., et al. (2017). Copper and cobalt accumulation in plants: a critical assessment of the current state of knowledge. New Phytol. 213, 537–551. doi: 10.1111/nph.14175
Lanquar, V., Lelièvre, F., Bolte, S., Hamès, C., Alcon, C., Neumann, D., et al. (2005). Mobilization of vacuolar iron by AtNRAMP3 and AtNRAMP4 is essential for seed germination on low iron. EMBO J. 24, 4041–4051. doi: 10.1038/sj.emboj.7600864
Lanquar, V., Ramos, M. S., Lelièvre, F., Barbier-Brygoo, H., Krieger-Liszkay, A., Krämer, U., et al. (2010). Export of vacuolar manganese by AtNRAMP3 and AtNRAMP4 is required for optimal photosynthesis and growth under manganese deficiency. Plant Physiol. 152, 1986–1999. doi: 10.1104/pp.109.150946
Li, J., Liu, J., Dong, D., Jia, X., McCouch, S. R., and Kochian, L. V. (2014). Natural variation underlies alterations in Nramp aluminum transporter (NRAT1) expression and function that play a key role in rice aluminum tolerance. Proc. Natl. Acad. Sci. U.S.A. 111, 6503–6508. doi: 10.1073/pnas.1318975111
Lin, Y. F., and Aarts, M. G. M. (2012). The molecular mechanism of zinc and cadmium stress response in plants. Cell. Mol. Life Sci. 69, 3187–3206. doi: 10.1007/s00018-012-1089-z
Mizuno, T., Usui, K., Horie, K., Nosaka, S., Mizuno, N., and Obata, H. (2005). Cloning of three ZIP/Nramp transporter genes from a Ni hyperaccumulator plant Thlaspi japonicum and their Ni2+-transport abilities. Plant Physiol. Biochem. 43, 793–801. doi: 10.1016/j.plaphy.2005.07.006
Morel, M., Grouzet, J., Gravot, A., Auroy, P., Leonhardt, N., Vavasseur, A., et al. (2009). AtHMA3, a P1b-ATPase allowing Cd/Zn/Co/Pb vacuolar storage in Arabidopsis. Plant Physiol. 149, 894–904. doi: 10.1104/pp.108.130294
Morrissey, J., Baxter, I., Lee, J., Li, L., Lahner, B., Grotz, N., et al. (2009). The ferroportin metal efflux proteins functions in iron and cobalt homeostasis in Arabidopsis. Plant Cell 21, 3326–3338. doi: 10.1105/tpc.109.069401
Nevo, Y., and Nelson, N. (2006). The NRAMP family of metal-ion transporters. Biochim. Biophys. Acta Mol. Cell Res. 1763, 609–620. doi: 10.1016/j.bbamcr.2006.05.007
Peng, F., Wang, C., Zhu, J., Zeng, J., Kang, H., Fan, X., et al. (2018). Expression of TpNRAMP5, a metal transport from polish wheat (Triticum polonicum L.), enhances the accumulation of Cd, Co and Mn in transgenic Arabidopsis plants. Planta 247, 1395–1406. doi: 10.1007/s00425-018-2872-3
Peris-Peris, C., Serra-Cardona, A., Sánchez-Sanuy, F., Campo, S., Ariño, J., and San Segundo, B. (2017). Two NRAMP6 isoforms function as iron and manganese transporters and contribute to disease resistance in rice. Mol. Plant Microbe Interact. 30, 385–398. doi: 10.1094/MPMI-01-17-0005-R
Pottier, M., Oomen, R., Picco, C., Giraudat, J., Scholz-Starke, J., Richaud, P., et al. (2015). Identification of mutations allowing natural resistance associated macrophage proteins (NRAMP) to discriminate against cadmium. Plant J. 83, 625–637. doi: 10.1111/tpj.12914
Qin, L., Han, P., Chen, L., Walk, T. C., Li, Y., Hu, X., et al. (2017). Genome-wide identification and expression analysis of NRAMP family genes in soybean (Glycine Max L.). Front. Plant Sci. 8:1436. doi: 10.3389/fpls.2017.01436
Sasaki, A., Yamaji, N., Yokosho, K., and Ma, J. F. (2012). Nramp5 is a major transporter responsible for manganese and cadmium uptake in rice. Plant Cell 24, 2155–2167. doi: 10.1105/tpc.112.096925
Shao, J. F., Yamaji, N., Shen, R. F., and Ma, J. F. (2017). The key to Mn homeostasis in plants: regulation of Mn transporters. Trends Plant Sci. 22, 215–224. doi: 10.1016/j.tplants.2016.12.005
Socha, A. L., and Guerinot, M. L. (2014). Mn-euvering manganese: the role of transporter gene family members in manganese uptake and mobilization in plants. Front. Plant Sci. 5:106. doi: 10.3389/fpls.2014.00106
Takahashi, R., Ishimaru, Y., Senoura, T., Shimo, H., Ishikawa, S., Arao, T., et al. (2011). The OsNRAMP1 iron transporter is involved in Cd accumulation in rice. J. Exp. Bot. 62, 4843–4850. doi: 10.1093/jxb/err136
Takahashi, R., Ishimaru, Y., Shimo, H., Bashir, K., Senoura, T., Sugimoto, K., et al. (2014). From laboratory to field: OsNRAMP5-knockdown rice is a promising candidate for Cd phytoremediation in paddy fields. PLoS One 9:e98816. doi: 10.1371/journal.pone.0098816
Tang, Z., Cai, H., Li, J., Lv, Y., Zhang, W., and Zhao, F. (2017). Allelic variation of NtNramp5 associated with cultivar variation in cadmium accumulation in tobacco. Plant Cell Physiol. 58, 1583–1593. doi: 10.1093/pcp/pcx087
Tejada-Jiménez, M., Castro-Rodríguez, R., Kryvoruchko, I., Lucas, M. M., Udvardi, M., Imperial, J., et al. (2015). Medicago truncatula natural resistance-associate macrophage protein1 is required for iron uptake by rhizobia-infected nodule cells. Plant Physiol. 168, 258–272. doi: 10.1104/pp.114.254672
The International Wheat Genome and Sequencing Consortium (2014). A chromosome-based draft sequence of the hexaploid bread wheat (Triticum aestivum) genome. Science 345:1251788. doi: 10.1126/science.1251788
Thomine, S., Lelièvre, F., Debarbieus, E., Schroeder, J. I., and Barbier-Brygoo, H. (2003). AtNRAMP3, a multispecific vacuolar metal transporter involved in plant responses to iron deficiency. Plant J. 34, 685–695. doi: 10.1046/j.1365-313X.2003.01760.x
Thomine, S., Wang, R., Ward, J. M., Crawford, N. M., and Schroeder, J. I. (2000). Cadmium and iron transport by members of a plant metal transporter family in Arabidopsis with homology to Nramp genes. Proc. Natl. Acad. Sci. U.S.A. 97, 4991–4996. doi: 10.1073/pnas.97.9.4991
Tiwari, M., Sharma, D., Dwivedi, S., Singh, M., Tripathi, R. D., and Trivedi, P. K. (2014). Expression in Arabidopsis and cellular localization reveal involvement of rice NRAMP, OsNRAMP1, in arsenic transport and tolerance. Plant Cell Environ. 37, 140–152. doi: 10.1111/pce.12138
Wang, X., Wang, C., Sheng, H., Wang, Y., Zeng, J., Kang, H., et al. (2017). Transcriptome-wide identification and expression analysis of ABC transporters in dwarf polish wheat under metal stresses. Biol. Plant. 61, 293–304. doi: 10.1007/s10535-016-0697-0
Wang, Y., Wang, C., Liu, Y., Yu, K., and Zhou, Y. (2018). GmHMA3 sequesters Cd to the root endoplasmic reticulum to limit translocation to the stems in soybean. Plant Sci. 270, 23–29. doi: 10.1016/j.plantsci.2018.02.007
Wang, Y., Wang, C., Wang, X., Peng, F., Wang, R., Jiang, Y., et al. (2016). De Novo sequencing and characterization of the transcriptome of dwarf polish wheat (Triticum polonicum L.). Int. J. Genomics 2016:5781412. doi: 10.1155/2016/5781412
Wang, Y., Wang, X., Gu, M., Kang, H., Zeng, J., Fan, X., et al. (2015). Cloning and characterization of four SnRK2 genes form Triticum polonicum. Biol. Plant. 59, 211–219. doi: 10.1007/s10535-015-0501-6
Wu, D., Yamaji, N., Yamane, M., Kashino-Fujii, M., Sato, K., and Ma, J. (2016). The HvNRAMP5 transporter mediates uptake of cadmium and manganese, but not iron. Plant Physiol. 172, 1899–1910. doi: 10.1104/pp.16.01189
Xia, J., Yamaji, N., Kasai, T., and Ma, J. (2010). Plasma membrane-localized transporter for aluminum in rice. Proc. Natl. Acad. Sci. U.S.A. 107, 18381–18385. doi: 10.1073/pnas.1004949107
Xiao, H., Yin, L., Xu, X., Li, T., and Han, Z. (2008). The iron-regulated transporter. Ann. Bot. 102, 881–889. doi: 10.1093/aob/mcn178
Yamaji, N., Sasaki, A., Xia, J., Yokosho, K., and Ma, J. (2013). A node-based switch for preferential distribution of manganese in rice. Nat. Commun. 4:2442. doi: 10.1038/ncomms3442
Yang, M., Zhang, W., Dong, H., Zhang, Y., Lv, K., Wang, D., et al. (2013). OsNRAMP3 is a vascular bundles-specific manganese transporter that is responsible for manganese distribution in rice. PLoS One 8:e83990. doi: 10.1371/journal.pone.0083990
Keywords: wheat, NRAMP, metal, plasma membrane, transport, accumulation
Citation: Peng F, Wang C, Cheng Y, Kang H, Fan X, Sha L, Zhang H, Zeng J, Zhou Y and Wang Y (2018) Cloning and Characterization of TpNRAMP3, a Metal Transporter From Polish Wheat (Triticum polonicum L.). Front. Plant Sci. 9:1354. doi: 10.3389/fpls.2018.01354
Received: 10 May 2018; Accepted: 28 August 2018;
Published: 20 September 2018.
Edited by:
Sebastien Thomine, Centre National de la Recherche Scientifique (CNRS), FranceCopyright © 2018 Peng, Wang, Cheng, Kang, Fan, Sha, Zhang, Zeng, Zhou and Wang. This is an open-access article distributed under the terms of the Creative Commons Attribution License (CC BY). The use, distribution or reproduction in other forums is permitted, provided the original author(s) and the copyright owner(s) are credited and that the original publication in this journal is cited, in accordance with accepted academic practice. No use, distribution or reproduction is permitted which does not comply with these terms.
*Correspondence: Yi Wang, wangyi@sicau.edu.cn
†These authors have contributed equally to this work