- 1Laboratory of Plant Breeding and Biometry, Department of Crop Science, Agricultural University of Athens, Athens, Greece
- 2Laboratory of Mycology, Department of Phytopathology, Benaki Phytopathological Institute, Athens, Greece
- 3Laboratory of Pomology, Department of Crop Science, Agricultural University of Athens, Athens, Greece
- 4Laboratory of Vegetable Production, Department of Crop Science, Agricultural University of Athens, Athens, Greece
Several studies regarding the imposition of stresses simultaneously in plants have shown that plant responses are different under individual and combined stress. Pathogen infection in combination with drought can act both additively and antagonistically, suggesting a tailored-made plant response to these stresses. The aforementioned combination of stresses can be considered as one of the most important factors affecting global crop production. In the present research we studied eggplant responses to simultaneous Verticillium dahliae infection and drought with respect to the application of the individual stresses alone and investigated the extent to which these responses were cultivar dependent. Two eggplant cultivars (Skoutari and EMI) with intermediate resistance to V. dahliae were subjected to combined stress for a 3-week period. Significant differences in plant growth, several physiological and biochemical parameters (photosynthesis rate, leaf gas exchanges, Malondialdehyde, Proline) and gene expression, were found between plants subjected to combined and individual stresses. Furthermore, plant growth and molecular (lipid peroxidation, hydrogen peroxide, gene expression levels) changes highlight a clear discrimination between the two cultivars in response to simultaneous V. dahliae infection and drought. Our results showed that combined stress affects significantly plants responses compared to the application of individual stresses alone and that these responses are cultivar dependent.
Introduction
Plants in their natural habitats are constantly and most of the time simultaneously exposed to a diverse range of biotic (fungi, bacteria, nematodes, insects, and non cellular pathogens such as viruses and viroids) and abiotic (drought, heat, cold, salinity, heavy metals) stresses. Being sessile, plants have developed specific mechanisms to overcome these challenges and survive. These mechanisms involve overlapping regulatory networks functioning principally at the cellular level and include molecular components that allow plants to perceive environmental changes and pathogen recognition; biochemical changes (such as inorganic ion fluxes, alteration of the redox status and metabolite homeostasis); plant hormones and activated/deactivated protein cascades. These networks participate in stress-signaling mediation as well as in the regulation of local defense responses such as, changes in cell wall and membrane composition and properties, callose deposition, production of reactive oxygen species (ROS), secondary metabolites and osmoprotectants and increase of soluble solutes (Fujita et al., 2006; Atkinson and Urwin, 2012; Kissoudis et al., 2014; Ramegowda and Senthil-Kumar, 2015). These mechanisms also involve systemic signals that prime plant defense to counter subsequent infection attempts and abiotic stresses (Prime-A-Plant Group et al., 2006; Conrath et al., 2015; Nejat and Mantri, 2017) and initiate adaptive responses to abiotic stresses through plant morphological and physiological changes such as changes in root system architecture, cuticular wax composition and leaf water potential (Xiong and Yang, 2003; Enright and Cipollini, 2011; Matus et al., 2014).
Previous evidence from laboratory studies regarding molecular and -omics data analyses (Ma et al., 2006; Seo et al., 2011; Rasmussen et al., 2013; Shaik and Ramakrishna, 2014) as well as field studies (Rizhsky et al., 2004; Mittler, 2006; Mittler and Blumwald, 2010), has shown that there is a synergistic or antagonistic crosstalk between plants responses to combined stress, and that plants respond to a specific combination of stresses in a tailored-made fashion rather than in an additive effect manner. A sequential occurrence of a certain combination of biotic and abiotic stresses may have different impacts, either positive or negative on the plant’s responses regarding pathogen resistance, abiotic stress tolerance, plant performance and fitness costs (Atkinson and Urwin, 2012; Kissoudis et al., 2014; Ramegowda and Senthil-Kumar, 2015; Nejat and Mantri, 2017; Pandey et al., 2017; Zhang and Sonnewald, 2017). These responses do not only depend on the nature of the stresses per se (type of stress, combination of stress types), but should be studied in relation to stress intensity, the order of occurrence (i.e., pathogen infection prior to an abiotic challenge or acclimation response followed by biotic stress), and the species genetic background (Kissoudis et al., 2014; Nejat and Mantri, 2017). The challenge to study the combinatory effect of multiple environmental stresses on plants performance becomes more evident, taking into consideration the effects of a climate change scenario on future agriculture needs. Under such environmental conditions plant–pathogen–abiotic stress interactions are drastically affected.
Drought stress is undoubtedly one of the most important of several, environmental factors that can greatly influence the productivity of crop plants around the world (Reddy et al., 2004). Plants respond to drought through multiple mechanisms such as altering shoot/root ratio (Bootraa et al., 2010), reducing plant growth rates (Reddy et al., 2004) and increasing water use efficiency (WUE) (Sivamani et al., 2000; Bootraa et al., 2010). On the other hand, plant pathogenic fungi are responsible for serious yield losses on an annual basis (Savary et al., 2012). Verticillium dahliae Kleb., is a widely distributed soilborne fungus, causing destructive vascular wilt to a wide range of herbaceous and woody plant hosts and severe yield losses (Pegg and Brady, 2002; Daayf, 2015). Since there are no effective chemical treatments to control the disease, management strategies encounter preventive measures (Blok et al., 2000), biological control regimes (Mercado-Blanco, 2012; Angelopoulou et al., 2014; Markakis et al., 2016) and use of cultivars that tolerate the disease to a certain extent (López-Escudero et al., 2004; Zhang et al., 2015). Many biochemical responses induced by drought are similar to those induced by pathogen attack, including increases in proline content (Farooq et al., 2009; Verslues and Sharma, 2010; Liang et al., 2013), ROS formation (Borden and Higgins, 2002; Degara et al., 2003), and upregulation of gene expression (Ramirez et al., 2009; Asano et al., 2012).
Previous publications show that in the cases of combined stresses, there is either a positive or negative effect of the preceding stress (i.e., drought or a specific pathogen attack) on plant responses toward the succeeding stress. In general drought influences negatively the plants tolerance to pathogen infection, causing detrimental effects to plants pathogen resistance (Mayek-Perez et al., 2002; Wang et al., 2009). On the other hand, increased resistance of barley plants to barley powdery mildew under drought conditions was observed through shared defense responses (Wiese et al., 2004). Additionally, tomato plants growth under drought conditions showed enhanced resistance to Botrytis cinerea and Oidium neolycopersici (Achuo et al., 2006). Pathogen infection often results in lower photosynthetic rates and reduction of WUE (Grimmer et al., 2012) and increases the susceptibility to drought stress (Audebert et al., 2000). Nevertheless, Arabidopsis plants infected with V. dahliae showed increased drought tolerance due to new xylem formation (Reusche et al., 2012). Furthermore, beneficial microbes such as arbuscular mycorrhizae and certain types of bacteria were shown to affect positively drought tolerance (Aroca et al., 2008; Kohler et al., 2008).
Eggplant (Solanum melongena L.) is one of the most important vegetable crops, especially for the Mediterranean basin, after potato (Solanum tuberosum) and tomato (Lycopersicon esculentum) (Rotino et al., 2014). Eggplants are very susceptible to many abiotic and biotic limiting factors throughout their entire biological cycle. They are very sensitive to water deficit, as they need substantial amount of water for their growth and development (Fu et al., 2013) and are susceptible to important fungal pathogens, such as the Fusarium oxysporum and V. dahliae (Collonnier et al., 2001), which cause significant crop damage. Therefore, one of the main objectives of eggplant breeding programs is the improvement of resistance to biotic and abiotic stresses. As eggplant is one of the most important vegetables for the Mediterranean region, and climate change will enforce the frequency as well as the intensity of combined stresses, it is of great importance to address the issues of climate-resilient vegetables.
In the present work we studied eggplant responses to the combined application of two main stress factors that affect its cultivation (V. dahliae and drought), compared to the application of the individual stresses alone. In addition, we investigated the extent to which these responses to combined stress are cultivar dependent. We showed that combined stress affects significantly the plants physiological and biochemical responses compared to application of individual stresses alone and that cultivar dependent plant growth significant differences are also affected by the type and combination of stresses applied. Furthermore, we showed that the expression of specific eggplant abiotic and biotic stress marker genes is induced by both drought and V. dahliae infection and that combined stress strongly enhances the expression of biotic stress marker genes in the leaves and roots of both eggplant cultivars.
Materials and Methods
Plant Material and Treatments
Two of the most common Greek eggplant cultivars, “Skoutari” and “EMI” with intermediate resistance to V. dahliae (Bletsos, unpublished results) were selected for this study. The cultivars were provided by the Agricultural Research Center of Northern Greece, ELGO-Demetra. Seeds were surface sterilized in 1.25% sodium hypochlorite for 20 min, rinsed three times in sterile distilled water, sown in sterile Klasmann-TS2 soil (Klasmann-Deilmann, Geeste, Germany) distributed in 50-ml QP Standard plastic pot trays (Agrohoum, Athens, Greece) and allowed to germinate in darkness. Plantlets were grown in a glasshouse under controlled conditions of 24°C, 70–80% RH, 16 h light – 8 h dark photoperiod, maximum light intensity 1110 μmol photons m-2 s-1. The eggplant seedlings were transplanted to 10 cm diameter pots when they were 7 weeks old at the stage of four true leaves. The selected seedlings were artificially inoculated either with a 106 mL-1 V. dahliae conidial suspension or with water for mock controls, just before transplantation, according to the dip-root method reported by Cappelli et al. (1995). The virulent BPIC 2681 V. dahliae isolate from eggplant, maintained in the BPI official collection of fungi, was used for inoculation. The seedlings were then watered and fertilized with Nutri-Leaf 20:20:20 (Miller Chemical & Fertilizer, LLC, Hanover, PA, United States) every week. Pots were arranged in a complete randomized design. Drought stress regimes were initiated the day the first wilting symptoms appeared, which was 17 days post inoculation (DPI) with the pathogen. For a time-interval of 3 weeks, four groups of plants were formed, each subjected to a specific treatment: (a) plants watered up to 100% of soil field capacity (FC) that served as a control, (b) plants infected with V. dahliae and watered up to 100% of FC, (c) plants watered up to 25% of FC, and (d) plants infected with V. dahliae and watered up to 25% of FC.
Leaf and Root Material Sampling
For all measurements and analyses performed, each plant constituted a single biological replicate. Leaf and root material sampling was performed during the last day of the treatments applied. For the determination of the H2O2, Malondialdehyde (MDA), and Proline concentrations and for RNA extraction, leaf and root material were collected from individual plants for each of the different treatments. For the leaf material the first, third, and forth fully developed leaves from the shoot apex were collected. The whole root system was collected after washing out the soil from the rhizosphere for a time interval of up to 3 min and using tap water previously acclimated at glasshouse controlled temperature conditions. Samples were briefly dried, immediately frozen in liquid nitrogen and stored at -80°C before subsequent use.
Plant Growth Parameters
The length of the stems from the base to the tip and the number of leaves were measured in each plant at an interval of 3 days through the duration of the experiment. Relative Growth Rate (RGR) respect to stem elongation was estimated as follows: RGR = [(T2-T1)/t]⋅1/T2, where T1 and T2 represent the stem length at the beginning and at the end of a time (t), respectively. Root length was measured at the end of the experiment. Fresh leaves, stems and roots were harvested, and oven dried at 85°C for 48 h to determine the dry weights.
Leaf Gas Exchange Parameters
Net photosynthetic rate (PN), stomatal conductance (gs), transpiration rate (E), and intercellular CO2 concentration (Ci), were measured twice per plant per treatment, using both the third and forth fully developed leaves from the shoot apex. The measurements were carried out with the Li-6400XT (Li-COR, Lincoln, NE, United States) portable photosynthesis measuring system under steady light intensity (800 μmol m-2 s-1) and CO2 concentration (400 mg l-1), while leaf temperature ranged between 27.5 and 29.8°C. WUE was computed as the ratio between PN and E of each one measurement. Similarly, the carbon dioxide use efficiency and the ratio between net photosynthetic rate and stomatal conductance were calculated by dividing the PN values with Ci or gs values of the recorded at each one measurement, respectively.
Lipid Peroxidation and Hydrogen Peroxide
Leaf tissue (250 mg) was homogenized in 10 ml 0.1% trichloracetic acid (TCA) at 4°C. After centrifugation at 4000 rpm for 15 min, the supernatant was used for the determination of both lipid peroxidation level and H2O2 concentration. Lipid peroxidation was measured as MDA content determined by reaction with 0.5% 2-thiobarbituric acid (TBA) in 20% TCA (w/v). The concentration of MDA was calculated from the difference of the absorbance at 532 and 600 nm using the extinction coefficient of 155 mmol-1 cm-1 (Heath and Packer, 1968). Hydrogen peroxide was also measured spectrophotometrically, after reaction with potassium iodide (KI). The reaction mixture consisted of 0.5 mL 0.1% trichloroacetic acid (TCA), leaf extract supernatant, 0.5 mL of 0.1 M potassium-phosphate buffer (pH 7) and 1 mL 1 M KI (w/v) reagent. The reaction color was developed for 45 min in darkness and absorbance was measured at 390 nm. The amount of hydrogen peroxide was calculated using a standard curve prepared with eight known concentrations of H2O2.
Proline Concentration
Proline content in root and leaf tissues was measured via reaction with ninhydrin (Bates et al., 1973). For colorimetric determinations, a solution of proline, ninhydrin acid and glacial acetic acid (1:1:1) was incubated at 90°C for 1 h. The reaction was then cooled in an ice bath. The chromophore was extracted using 2 ml of toluene and its absorbance at 520 nm was determined by a BioMate spectrophotometer (Thermo Fisher Scientific, Waltham, MA, United States).
Primer Sequences
Solanum melongena EST sequences were retrieved after homology search from GenBank and Sol Genomics Network. Primers (Table 1) for quantitative real time PCR experiments were designed using the OligoPerfectTM Designer (Thermo Fisher Scientific, Waltham, MA, United States), following standard primer design criteria. Primers were tested in end-point and real time PCR reactions using 10-fold dilutions of S. melongena genomic DNA ranging from 50 ng to 50 fg.
cDNA Synthesis and Relative Gene Expression Study
Total RNA was extracted using the TRI Reagent (Sigma-Aldrich, St. Louis, MI, United States) reagent following the instructions of the manufacturers. cDNA was synthesized using the PrimeScriptTM RT reagent Kit with gDNA Eraser (Takara Bio, Shiga, Japan) and 1 μg of total RNA according to the manufacturer’s instructions. The Applied Biosystems 7500 Fast Real-Time PCR system (Applied Biosystems, Foster City, CA, United States) was used for real time PCR assays. Samples were prepared using the KAPA SYBR FAST qPCR kit 2X master mix (KAPA Biosystems, Inc., Boston, MA, United States), 200 nM of each primer and either 1 μl of cDNA template (1/20th of cDNA reaction volume) or RNase free-H2O for non-template controls, in a final reaction volume of 15 μl. Four individual biological replicates for each of the different treatments were assayed per RT-PCR run, in two individual runs considered as technical replicates. Non-reverse transcribed samples were also assayed in real time PCR runs prior to sample analyses. For all target genes assayed the PCR amplification thermal profile used consisted of one initial denaturation cycle of 3 min at 95°C followed by 40 cycles of 3 s at 95°C and 20 s at 60°C. Melting curves were programmed as 15 s at 95°C, 15 s at 60°C, 20 min slow ramp, and 15 s at 95°C. The data for the dissociation curve were captured during this slow ramp, and the melt curve was visualized using the ABI PRISM 7900 software. The relative gene expression was determined with the comparative Ct method (Livak and Schmittgen, 2001), calculating the mean threshold cycle (Ct) values of the target and endogenous control genes for four individual biological replicates of both technical replicate runs.
Statistical Analysis
Analysis of Variance (three-way ANOVA) was carried out with V. dahliae infection, water stress level and cultivars as fixed factors (sample size: 24 or 32 plants). Differences between means were assessed using Fisher’s Protected least significant difference (LSD) (P < 0.05). The statistical package used for analyzing the data was GENSTAT 10th edition. Normality was checked by examining residual plots produced which provide a way of checking the normality and the equal variance assumptions of the ANOVA and the Shapiro–Wilk test. The data was found to be normally distributed. In addition, Analysis of Variance (one-way ANOVA) was carried out for the parameters presented in Figures 2, 3 to test differences between treatments within each cultivar. Differences between means were assessed using Student Newman Keuls test (P < 0.05).
Results
Verticillium dahliae, Drought Stress and Eggplant Cultivars Double and Triple Interactions Significantly Affect the Plant Relative Growth Rate and the Shoot to Root Fresh Weight Ratio
The significance of the pathogen, water stress and cultivars main effects and their double and triple interactions on RGR, root to stem length ratio and shoot to root fresh and dry weight ratios, are presented in Tables 2 and 3. Triple interactions are further presented in Figure 1 by creating separate plots which illustrate the interaction of cultivar and V. dahliae stress (Vd-/+) at normally irrigated (No DS) and water stressed (DS) plants. In the graphs, the mean value of each parameter assesses/measured and the LSD values are presented.
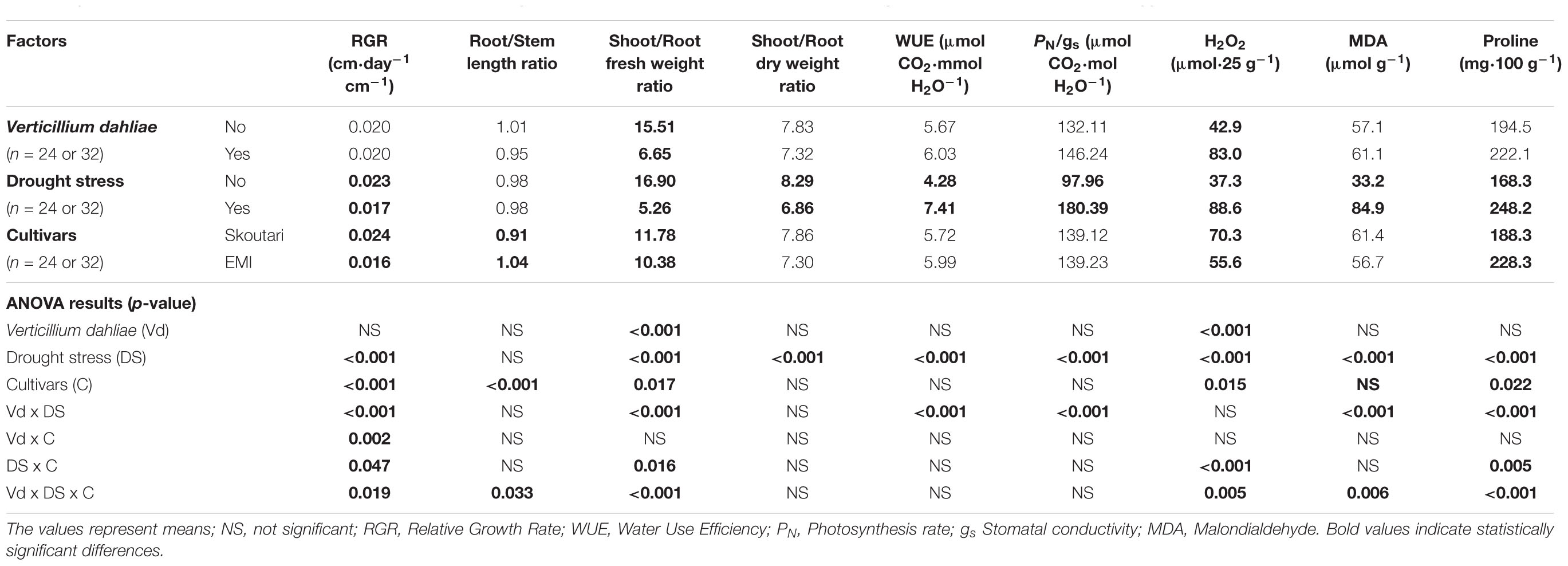
TABLE 2. Effect of, and interactions between, Verticillium dahliae, drought stress and cultivars on developmental, physiological and biochemical parameters eggplants (3-factor ANOVA).
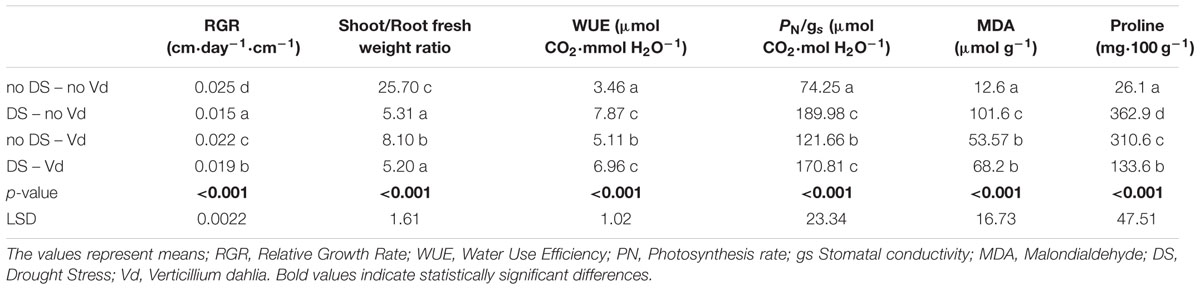
TABLE 3. Effect of Verticillium dahliae infection and drought stress on eggplant developmental, physiological and biochemical parameters.
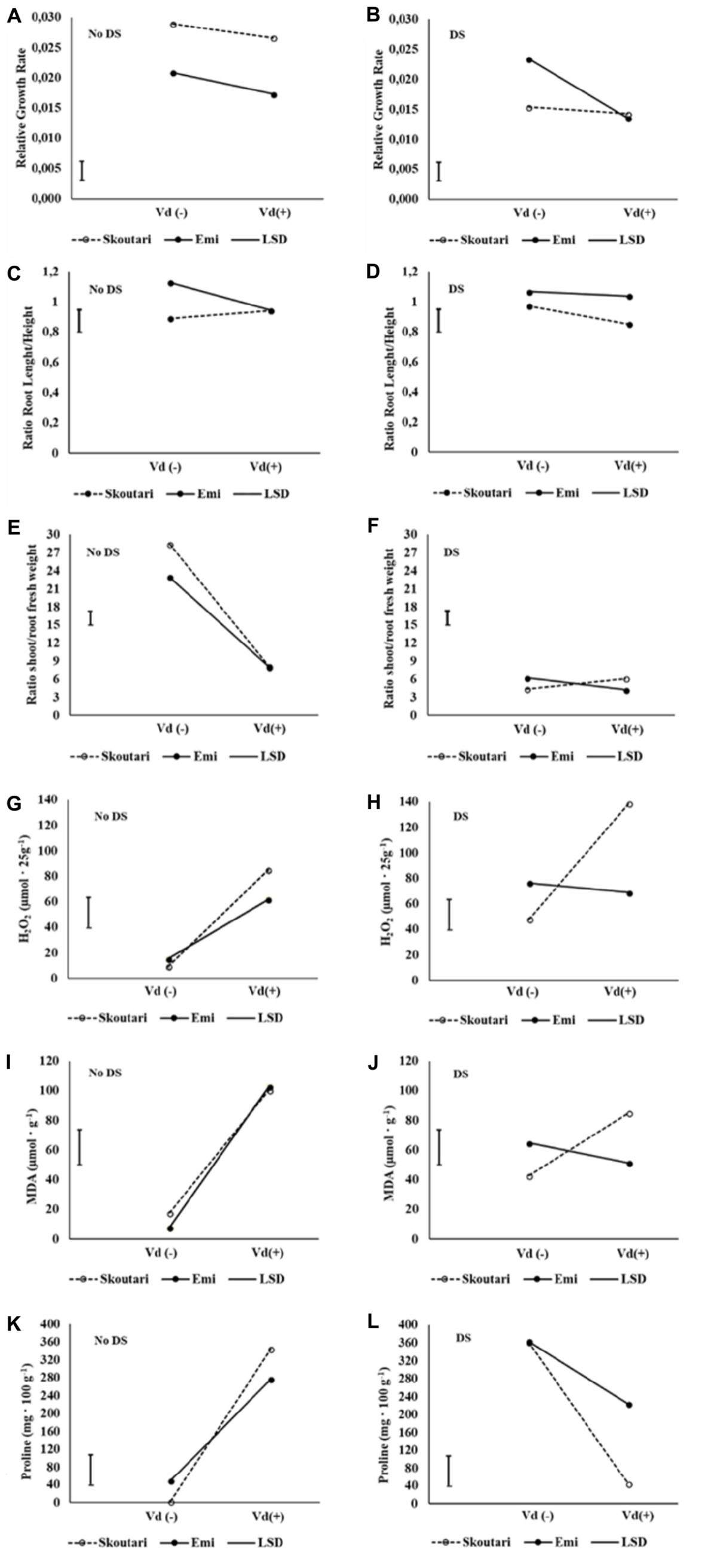
FIGURE 1. Three-way interaction plots of RGR (A,B), root to stem length ratio (C,D), shoot to root fresh weight ratio (E,F), H2O2 (G,H), MDA (I,J) and Proline (K,L) concentrations, on two eggplant cultivars (Skoutari and Emi), at V. dahliae infection and drought stress. Vd (±): Verticillium dahliae, DS: drought stress.
Drought stress and cultivars as single factors had significant effect on RGR (Table 2). V. dahliae, water stress and cultivars imposed together had also significant effect on RGR as indicated in all double and triple interactions, presented in Table 2 and Figures 1A,B. As presented in Table 3, for the V. dahliae and drought stress combination (irrespective of the cultivar) RGR values for all plant treatments were significantly reduced compared to the control (0.025 cm day-1 cm-1). Plants under combined stress had an intermediate RGR value (0.019 cm day-1 cm-1) with respect to V. dahliae infected (0.022 cm day-1 cm-1) and drought-stressed (0.015 cm day-1 cm-1) alone.
Cultivars was the only significant main factor to affect the plants root to stem length ratio (mean values of 0.91 and 1.04 for Skoutari and EMI cultivars, respectively). Triple interaction of cultivar with the two stress types were also found to be statistically significant (Table 2). All three factors affected significantly the shoot to root fresh weight ratio, as main factors and in their double and triple interactions except the interaction between V. dahliae and cultivars (mean values and significance levels are presented in Table 2 and Figures 1C,D). As presented in Table 3 for the V. dahliae and water stress interaction, the ratio was much lower in infected plants (8.10) respect to the control (25.70) and was reduced further in drought stressed plants (5.31) and plants under combined stress (5.20). The cultivars significant effect with the V. dahliae and drought stress interaction on the shoot to root fresh weight ratio is presented in Figures 1E,F. The shoot to root dry weight ratio was affected significantly only by drought stress (mean values of 8.29 and 6.86 for control and stressed plants, respectively, Table 2). The values of the dry weight ratio for the V. dahliae infected and drought stressed plants were higher respect to their corresponding fresh weight ratio values.
Similar Reduction in Photosynthesis Rate and Leaf Gas Exchange Parameters in Plants Under Drought and Combined Stress
Photosynthesis rate (PN), and leaf gas exchange parameters (gs, E, and Ci), were reduced in both cultivars in all stresses applied respect to the untreated control (Figures 2A–D). Furthermore, the same pattern regarding the reduction levels was observed in between stress treatments. In both cultivars, all parameters measured were affected more (with respect to the mean values) by drought stress and less by V. dahliae infection, whereas the combined stress resulted to intermediate mean values respect to those of drought stressed and pathogen infected plants alone (Figures 2A–D). For the Skoutari cultivar, statistically significant differences for the three out of four parameters tested were observed between treated plants (irrespective the type of stress) and untreated controls. For the EMI cultivar, statistically significant differences were observed for all parameters tested between plants treated either with drought or combined stress in comparison to control or V. dahliae infected plants (Figures 2A–D).
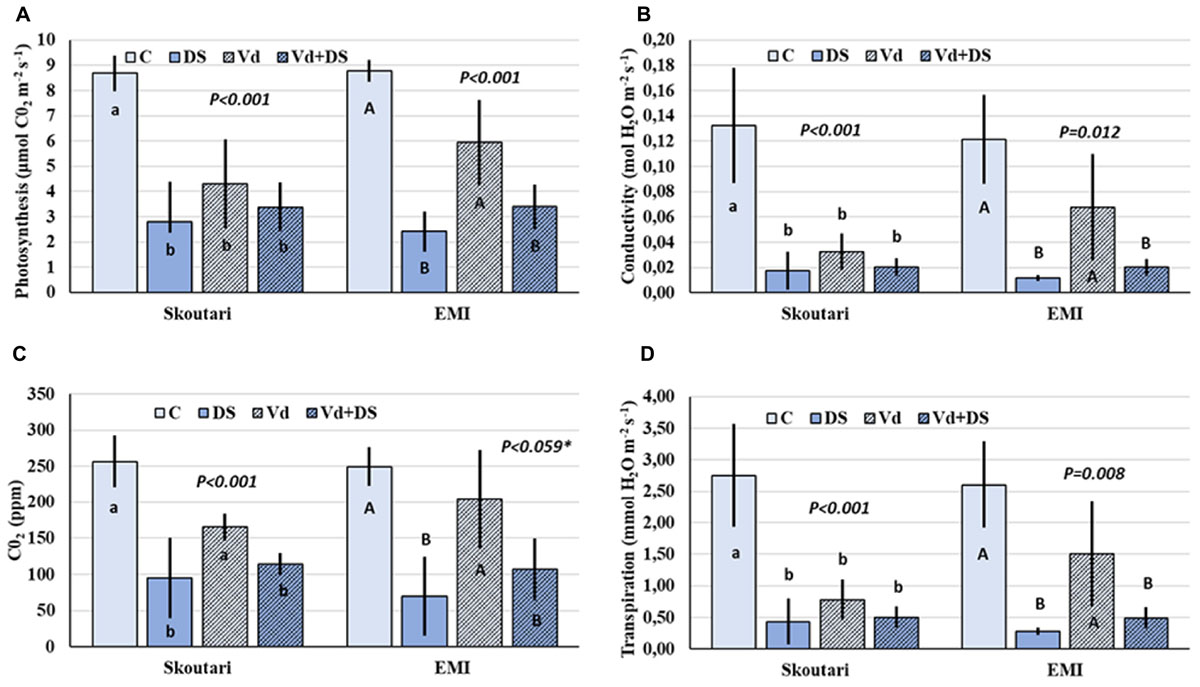
FIGURE 2. Photosynthesis rate and leaf gas exchange parameters in eggplant cultivars subjected to abiotic, biotic and combined stresses. The net photosynthetic rate (PN), stomatal conductance (gs), intercellular CO2 concentration (Ci) and transpiration rate (E) (A–D, respectively), were measured in eggplant cultivars under individual and combined stress. C, control; DS, drought stress; Vd, Verticillium dahliae; Vd+DS, Combined stress. Bars represent standard deviation. Means within the same cultivar with different lower (Skoutari) or upper (EMI)-case letters are significantly different according to Student Newman Keuls test (P < 0.05 or P < 0.1∗).
A statistical analysis of the pathogen, drought stress and cultivar main effects as individual factors and the significance of their double and triple interactions, on WUE and Photosynthesis rate/Conductivity ratio (PN/gs) are presented in Tables 2,3. Drought stress had a significant effect on both ratios, while V. dahliae and cultivars had no significant effect on either ratio (Table 2). With respect to V. dahliae, drought stress and cultivar double interactions, combined stress (irrespective the cultivar) was the only one to affect significantly both ratios (Tables 2,3). Specifically, WUE increased in V. dahliae infected plants (5.11 μmol CO2⋅mmol H2O-1) compared to the control (3.46 μmol CO2⋅mmol H2O-1) and doubled in plants under drought and combined stresses (7.87 and 6.96 μmol CO2⋅mmol H2O-1, respectively) (Table 3). The PN/gs ratio followed the same pattern as WUE and increased in V. dahliae infected plants (121.66 μmol CO2 mol H2O-1) with respect to the control (74.25 μmol CO2⋅mol H2O-1) and was more than double in plants under drought and combined stresses (189.98 and 170.81 μmol CO2⋅mol H2O-1, respectively) (Table 3). The combination of cultivar, V. dahliae and drought stress had no significant effect on WUE and PN/gs ratio as presented in Table 2.
Determination of H2O2, MDA, and Proline Concentrations in Leaves of Plants Treated With Individual and Combined Stresses
The concentration of H2O2 in leaves increased after application of either individual or combined stresses with respect to the untreated control plants, however, with a different mode for the two cultivars (Figure 3A). The highest H2O2 concentration (138.81 μmol⋅25 g-1 of tissue fresh weight) was observed in Skoutari plants under combined stress. In the same cultivar, H2O2 mean levels in individually drought stressed or V. dahliae infected plants were at 85.00 and 47.91 μmol⋅25 g-1, respectively, higher than the untreated control (9.36 μmol⋅25 g-1). For Skoutari plants statistical differences were observed between all treatments and the control. On the other hand, H2O2 in leaves of EMI treated plants was similar with mean values of 68.81, 61.90, and 76.45 μmol⋅25 g-1, for plants under combined stress, drought stress or V. dahliae infection, respectively. They all are statistically different from the untreated control (15.36 μmol⋅25 g-1).
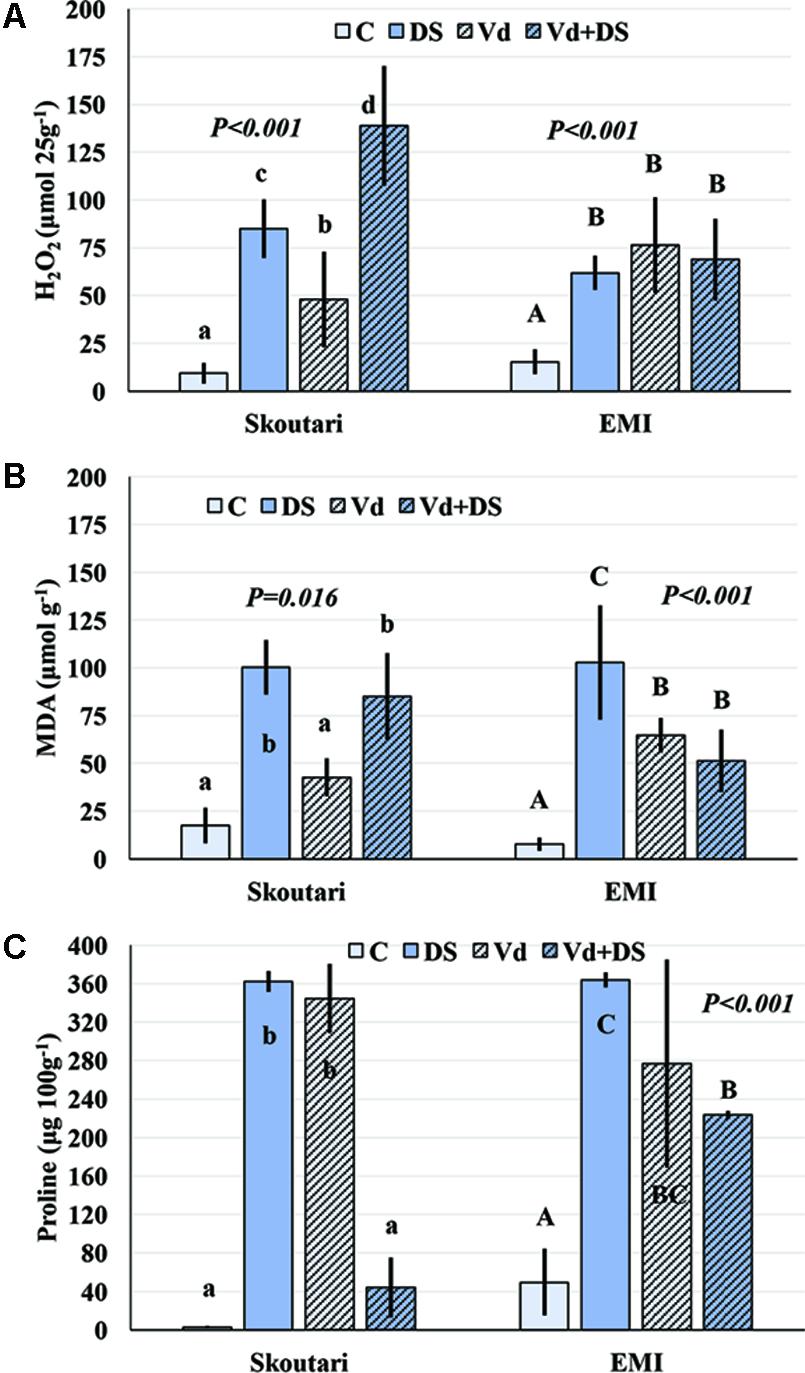
FIGURE 3. H2O2, MDA, and Proline concentration alterations in eggplant cultivars subjected to abiotic, biotic and combined stresses. The H2O2, MDA, and Proline concentration (A–C, respectively) were measured in eggplant cultivars under individual and combined stress. C, control, DS, drought stress, Vd, Verticillium dahliae, Vd+DS, Combined stress. Bars represent standard deviation. Means within the same cultivar with different lower (Skoutari) or upper (EMI)-case letters are significantly different according to Student Newman Keuls test (P < 0.05).
Malondialdehyde concentrations varied between treatments in both cultivars (different statistical significance in each cultivar), as seen in Figure 3B. The highest mean values were observed in drought stressed plants of both cultivars (100.38 and 102.83 μmol g-1 for Skoutari and EMI, respectively), whereas, V. dahliae infected plants showed lower MDA mean values (42.71 and 64.77 μmol g-1 for Skoutari and EMI, respectively) compared to drought stressed plants. In plants subjected to combined stress, MDA ranged at the same levels as drought stressed plants (mean value of 85.03 μmol g-1) for Skoutari, however, was lower (mean value of 51.35 μmol g-1) for EMI. In untreated controls MDA mean values were 17.54 and 7.74 μmol g-1 for Skoutari and EMI, respectively.
As presented in Figure 3C, proline concentration was increased in all treated samples. A high increase was observed respect to the controls (mean values of 2.7 mg⋅100 g-1 and 49.5 mg⋅100 g-1 for Skoutari and EMI, respectively) in drought stressed (362.1 mg⋅100 g-1 and 363.7 mg⋅100 g-1 for Skoutari and EMI, respectively) and V. dahliae infected plants (344.4 mg⋅100 g-1 and 276.7 mg⋅100 g-1 for Skoutari and EMI, respectively). It was, however, reduced in plants subjected to combined stress (43.9 mg⋅100 g-1 for Skoutari and 223.3 mg⋅100 g-1 for EMI). Although, a similar pattern of the plant’s response to the stresses applied is observed for both cultivars, statistically significant differences vary in between stress types for each cultivar.
The statistical analysis of the pathogen, drought stress and cultivar main effects as individual factors and the significance of their double and triple interactions, on H2O2, MDA, and Proline concentrations are presented in Tables 2, 3. V. dahliae had a significant effect on H2O2 only, while drought stress influenced all three parameters tested. Cultivar as single factor had a significant effect on both H2O2 and Proline (Table 2). The combined V. dahliae and drought stress (irrespective the cultivar) had significant effect on MDA and Proline concentrations as indicated in Tables 2, 3. Specifically, MDA concentrations were significantly increased in all treated samples respect to the control, with the highest mean value observed (Table 3) in drought stressed plants (101.6 μmol g-1). MDA concentration was reduced to nearly half in V. dahliae infected plants (53.57 μmol g-1) and had an intermediate value (68.2 μmol g-1) for plants under combined stress, though not statistically different (LSD, 16.73) from the former. Proline concentrations (Table 3) were significantly increased in plants subjected to individual stress (mean values of 362.9 and 310.0 mg⋅100 g-1 for drought stressed and V. dahliae infected plants, respectively), respect to the control (26.1 mg⋅100 g-1). Plants under combined stress had an intermediate mean value (133.6 mg⋅100 g-1) though statistically different from the control (LSD, 47.51). No significant interaction of V. dahliae and variety was observed for all three parameters tested, while the drought stress and variety interaction were only significant for H2O2 and Proline (Table 2). The cultivar, V. dahliae and drought stress triple interaction had a combined effect on H2O2, MDA, and Proline (Table 2) with significant differences between plants of the two cultivars observed under combined stress (Figures 1G–L).
Combined V. dahliae and Drought Stress Enhances the Expression of Biotic Stress Marker Genes
Figure 4 shows the gene expression fold differences of selected marker genes for the two cultivars subjected under individual and combined stresses. For the PR1 and PR5 biotic stress marker genes a basal level of expression was detected, as for the abiotic stress marker genes, in untreated controls in all cases. Expression of the two genes was induced in V. dahliae infected plants as expected and in plants under drought stress, in leaves and roots of both cultivars. A strong upregulation of gene expression was observed in plants under combined stress compared to plants either under biotic or drought stress alone, with the highest values (fold changes respect to untreated controls) observed in leaves with respect to roots of both cultivars for the PR1 gene (76-fold and 94-fold for the Skoutari and EMI cultivars, respectively) and in roots with respect to leaves for the PR5 gene (102-fold and 87-fold for the Skoutari and EMI cultivars, respectively). The expression of the LOX gene did not vary significantly between the different treatments and the control for both cultivars and organ types.
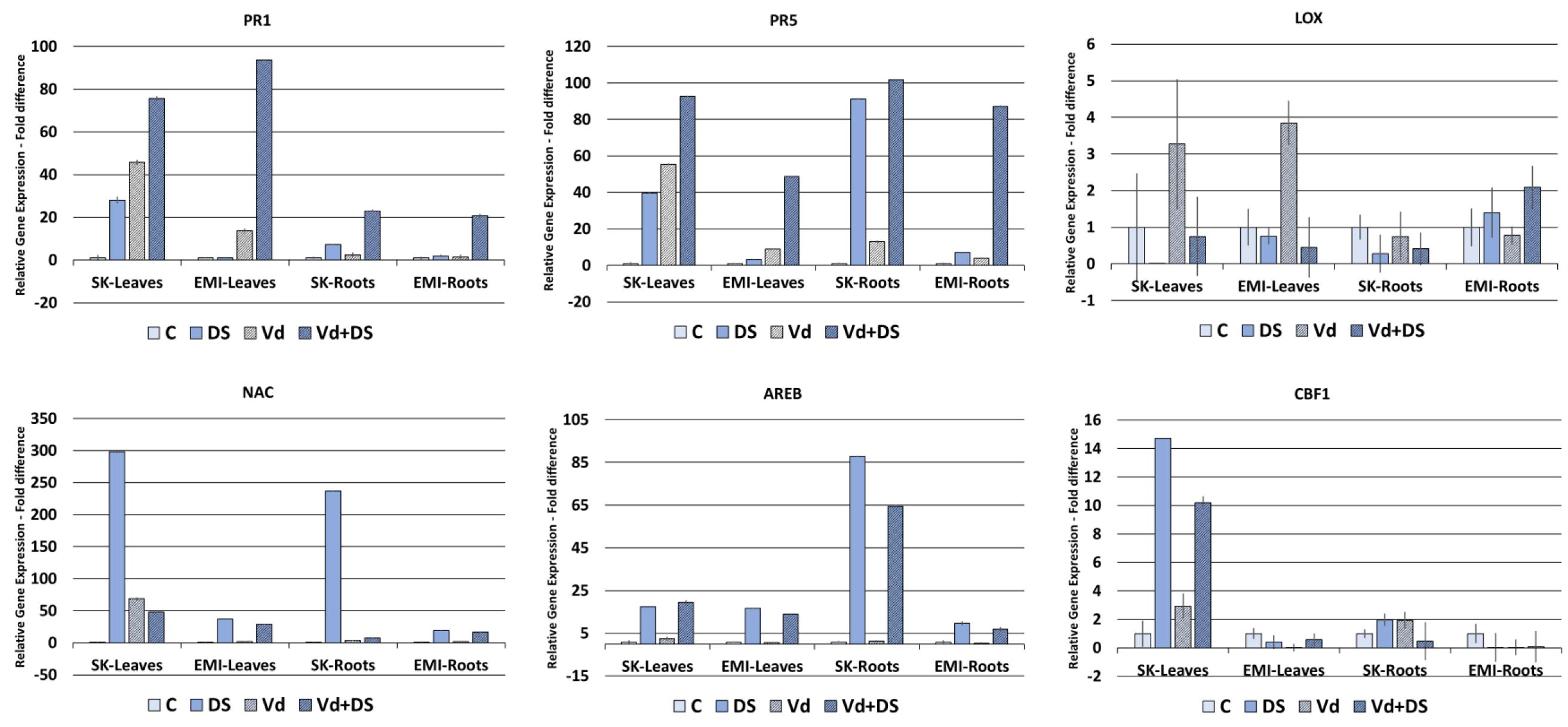
FIGURE 4. Relative gene expression of the PR1, PR5, LOX, NAC, AREB, and CBF1 marker genes in leaves and roots of eggplant plants subjected to abiotic, biotic, and combined stresses. The relative expression of selected marker genes was measured in eggplant cultivars under individual and combined stress. C, control; DS, drought stress; Vd, Verticillium dahliae; Vd+DS, Combined stress. Bars represent standard error.
The NAC marker gene was significantly induced in drought treated plants showing the highest values (fold changes respect to untreated controls) in both tissues of the Skoutari cultivar (298-fold and 237-fold, respectively). Significant induction was also observed in plants subjected to combined stress, however, fold changes ranged at the same levels as of drought induced plants for the EMI cultivar in both leaves and roots (29-fold and 37-fold for leaves and 17-fold and 19-fold for roots, respectively), but where significantly downregulated compared to drought induced plants for the Skoutari cultivar (48-fold and 298-fold for leaves and 8-fold and 237-fold for roots, respectively). For the AREB marker gene significant induction of expression was observed in plants treated with drought and plants under combined stress. In both cases the levels of fold differences did not vary between the two types of treatment irrespectively the cultivar or type of organ with the highest values observed in Skoutari roots (88-fold and 65-fold for drought and combined stress, respectively). Significant gene expression induced by biotic stress alone was observed only for NAC in Skoutari leaves (69-fold respect to untreated control). Significant differences in the expression of CBF1 were observed only in Skoutari leaves. Expression was induced by all types of treatments with the highest fold differences observed in drought and combined stresses (15-fold and 10-fold compared to untreated control, respectively).
Discussion
A limited number of studies regarding the imposition of stresses simultaneously in plants revealed that plants respond in a different manner under individual and combined stress (Xu et al., 2008; Atkinson et al., 2013; Prasch and Sonnewald, 2015; Gupta et al., 2016). The combination of two stresses (abiotic–abiotic or abiotic–biotic) does not always have a negative effect on plants. Reports have shown that drought in combination with pathogen infection can act both additively and antagonistically. This combination can be considered as one of the most important stress combinations affecting global crop production, given the number of reports of plant diseases caused by drought stress and the frequency of water stress incidences. Pathogens that induce wilt, such as V. dahliae, impose a physiological drought stress effect in plants by blocking the xylem (Genin, 2010). When wilt disease coincides with drought, a new net effect of combined stress could be expected that is different from the individual stresses (Abd El-Rahim et al., 1998; Choi et al., 2013; Sinha et al., 2016). To study this assumption, the impact of the combined occurrence of V. dahliae and drought was compared with the impact of the two stressors imposed separately, by measuring several morphological, physiological and biochemical parameters. The simultaneous imposition of drought and a vascular pathogen is often found to reduce several morphological and physiological parameters such as the plant height, total leaf area and transpiration (Pennypacker et al., 1991; Abd El-Rahim et al., 1998; Choi et al., 2013; Sinha et al., 2017).
Our results indicate that the concurrent effect of pathogen and drought stresses is different in each cultivar compared to the net effect of drought stress and V. dahliae applied individually. Similarly, the effect of drought and Verticillium wilt on eggplant cultivars had been studied under field conditions (Bletsos et al., 1999) and showed that different irrigation regimes in combination with Verticillium wilt significantly affected fruit quantitative characteristics of different eggplant cultivars.
Specifically, when drought and pathogen stress occurred simultaneously, they caused a significant decrease in RGR. Plant growth reduction can be the outcome of a pathogen infection (Johnstone et al., 2005). Results by Buhtz et al. (2017) demonstrated that V. dahliae infection greatly compromised plant growth of tomato plants. Nevertheless, this reduction can limit the water requirements of the plant thus improving plant survival during the simultaneous imposition of drought as shown in other studies (Xu et al., 2008). Interestingly our results indicate further that significant interactions of cultivar, drought and V. dahliae stresses were also obtained. One of the most common suite of traits that plants utilize to circumvent the stressful environments is the reduced stem/root ratio, thus optimizing the use of their available resources (Chapin et al., 1993; Gargallo-Garriga et al., 2014; Koevoets et al., 2016; Buhtz et al., 2017; Pandey et al., 2017). Even though, this was not shown in the double interaction, there was a significant difference in the cultivars effect (as single factor) and in the triple interaction. Our results demonstrated a significantly higher root/shoot length ratio of EMI plants compared to Skoutari plants during the stress, indicating that EMI alters its morphological traits in order to circumvent more efficiently the combination of stressors.
Stressful environments, including drought, pathogen infection and their combination, greatly influence the process of photosynthesis in most plants by altering several photosynthetic parameters such as WUE and stomatal regulation (Ashraf and Harris, 2013; Pandey et al., 2017). One of the key mechanisms of plants in order to adapt to multiple stresses is stomatal regulation by allowing plants to make the optimum CO2 assimilation toward evapotranspiration. In several cases of combined stresses (either abiotic-abiotic or abiotic-biotic) photosynthesis rate (PN), and leaf gas exchange parameters (gs, E, and Ci) dramatically decreased under such conditions compared to single stress (Choi et al., 2013; Suzuki et al., 2014). In our study, the highest reduction of the photosynthetic rate and of leaf gas exchange parameters (Figure 2) was shown when plants were subjected either to severe drought or to combined stress, suggesting that the decline of photosynthesis rate is due to stomatal closure. Additionally, WUE and PN/gs ratios were mainly dependent on water stress alone and to combined stress. Xu et al. (2008) demonstrated that when virus infection preceded water stress, plants responded better to the two stressors due to partial stomatal closure and lower transpiration rates. Our results highlighted that both varieties maintained their photosynthetic activity during the combined stress. Moreover, WUE and Pn/g were higher under combined stress than biotic stress alone (Table 3) The maintenance of plants photosynthetic activity is very important for their acclimation to a combination of pathogen and drought stress (Pandey et al., 2015).
During abiotic and/or biotic stress interactions, plants produce rapidly and transiently ROS, functioning as signaling molecules (i.e., induce production of ROS scavengers) and being one of their first defense responses against stresses. Likewise, fungi infection would result in oxidative stress in plants, and any concomitant stress could create inevitably additional production of ROS (Dikilitas et al., 2016). Continuous stress can cause an accumulation of ROS at the plasma membrane, producing an oxidative stress that leads to increased membrane damage and MDA content (Hasanuzzaman et al., 2013; Schieber and Chandel, 2014; Choudhury et al., 2017). Oxidative stress is often associated with excessive concentrations of H2O2 that is considered the central ROS signaling molecule (Sies, 2017). Interestingly, significant increase in H2O2 levels was monitored in all treatments compared to controls (Tables 2 and 3) and significant differences were observed between the two cultivars for plants under combined stress and controls (Figures 1G,H). The increased H2O2 levels at the end of the experiment indicated an additive effect of combined stress on H2O2 formation and a prolonged oxidative stress for the plants of Skoutari cultivar (Figure 3). Similarly, the triple interaction of the cultivar, V. dahliae and water stress had a significant combined effect on MDA one of the most well studied markers of lipid peroxidation and oxidative stress (Figures 1I,J). It is noteworthy that researchers have associated powerful oxidative stress with significant decrease of root growth as well (Miller et al., 2009). It should be noted that EMI cultivar exhibited better membrane homoeostasis and lower H2O2 accumulation under stress combination suggesting that may have a stronger capacity for membrane lipid replacement than Skoutari (Figure 1). In the review by Suzuki et al. (2014) it is clearly highlighted that the inhibition of oxidative stress can at least partially ensure plants protection against stress combination.
On the other hand, proline has been reported to be the most abundant osmolyte accumulated in response to multiple environmental stresses including water stress and pathogen infection, which acts as an ROS scavenger as well (De Ronde et al., 2000; Hanci and Cebeci, 2014; Rodziewicz et al., 2014). Recent findings have demonstrated that water stressed eggplants accumulated osmoprotectants like proline, as well as glucose and fructose and this phenomenon has been correlated with increased tolerance toward water deficit and prevention from wilting (Mibei et al., 2018). Our results showed significant difference between control plants and water stressed or V. dahliae infected plants in proline concentration, however, no significant difference was observed between control plants and plants under combined stress for the Skoutari cultivar. On the contrary, significant difference was observed between control plants and plants under combined stress for the EMI cultivar. This indicates that other osmoprotectants could be up-regulated during concomitant imposition of V. dahliae and water stress in certain eggplant cultivars.
Though the availability of genome sequences for other members of the Solanaceae plant family such as tomato and potato enabled gene expression and transcriptome studies for these species, no such genome sequence facilities were available for eggplant until the publication of the draft eggplant genome sequence (Hirakawa et al., 2014) and a pre-publication version from the Eggplant Genome Project1. Since then, various publications on comparative transcriptome analyses regarding the phylogenetic relations of eggplant and closely related wild species (Yang et al., 2014, 2015), the determination of molecular markers (Gramazio et al., 2016), characterization of genes and gene families (Barbierato et al., 2016; Li et al., 2016), gene function (Na et al., 2016), or miRNAs (Yang et al., 2013), have been made. To study the combined effect of water stress and V. dahliae infection at gene transcriptional level, we have selected specific marker genes, after homology search in GenBank and Sol Genomics Network and designed suitable primers for quantitative real-time polymerase chain reaction (RT-qPCR). Pathogenesis related (PR1 and PR5) and lipoxygenase (LOX) genes had been shown to be induced by Salicylic acid (SA) and Jasmonic acid (JA), respectively, and are commonly used as biotic stress markers in gene expression studies (Aime et al., 2008; Kissoudis et al., 2017; Mahesh et al., 2017), AREB, and CBF1 are water and cold stress related markers (Hsieh et al., 2002; Wang et al., 2016), while NAC genes are reported to have roles in both abiotic and biotic stresses (Tweneboah and Oh, 2017). Previous studies on the validation of eggplant reference genes for RT-qPCR assays, have indicated appropriate candidates for use in quantitative gene expression studies (Gantasala et al., 2013; Zhou et al., 2014). For our study we have selected ACTIN as reference gene (Zhou et al., 2014).
Our gene expression study results (Figure 4) showed a strong upregulation of PR1 and PR5 genes by V. dahliae infection as well as by water stress. Interestingly, their expression was further enhanced when combined stress was applied. These results taken together with the fact that V. dahliae infection preceded the water stress applied, suggest a crosstalk between biotic and abiotic stress biochemical regulatory pathways and a strong influence of water stress on biotic stress plant responses at the transcriptional level. Furthermore, the relative gene expression fold changes observed in plants under combined stress compared to plants under single stress, and particularly in the EMI cultivar, suggest that the regulation of gene expression does not necessarily fall in a mode of an additive pattern, but supports a cultivar dependent tailored made regulation. The enhanced expression of PR1 and PR5 under combined stress appears tissue dependent as well. PR1 is strongly induced in leaves respect to roots of both cultivars, while PR5 has a higher induction in roots, indicating possibly a different involvement in tissue-specific gene expression in the overall plants responses to the stresses. The low relative expression levels of LOX in all treatments compared to the untreated control in both cultivars, in conjunction with the strong upregulation of PR1 and PR5, indicate that the plants responses to all types of stresses applied are regulated principally via SA. Our results also show that the high expression of NAC in leaves of Skoutari water stressed plants is reduced in plants under combined stress, however, not in the EMI cultivar. This supports further a crosstalk between the biochemical pathways regulating the responses to biotic and abiotic stress and indicates a cultivar dependent mechanism that masks or suppresses the NAC expression. The expression patterns for AREB and CBF1 suggest that these genes are principally induced/regulated by water stress, since no differences are observed in plants under combined stress.
Our study showed that Skoutari and EMI responses to combined stress are cultivar dependent. Both cultivars were also subjected to milder drought stress (50% of FW) and we found clear difference in their responses, with EMI being the more tolerant to drought stress (data not shown). It can be speculated that the morphological adaptations, the stronger capacity for membrane lipid replacement and the higher osmoprotectant accumulation of EMI enhanced its tolerance to the combined stress. On the other hand, the increased plant growth and plant tolerance to stressful environments act in an antagonistic manner. This is the case with our cultivars as well. Skoutari cultivar exhibited the higher RGR in all conditions. However, it showed less osmoprotectant accumulation and more lipid peroxidation of membranes. Consequently, improving traits such as stability of leaf gas exchange parameters, membrane homeostasis and ROS regulation in cultivars that produce higher biomass should be further explored to gain better adaptation to higher stress environments.
It is of our interest to define further, cultivar dependent molecular responses of eggplant to either V. dahliae, drought stress and their possible combinations by profound transcriptomic and proteomic studies. These would allow us to dissect specific molecular pathways and define eggplant key regulatory genes for theses stresses. Recently, a characterization of an indigenous eggplant cultivars and landraces collection has been performed (Ganopoulos et al., 2015). It would be interesting to define further their responses to combined V. dahliae and drought stress and utilize them in future breeding projects.
Author Contributions
ET and DK were involved in the experimental design of the work, performed experiments, analyzed, and interpreted the data and wrote the paper. EM performed and interpreted statistical analysis. IP, DT, GL, DM, and IK performed experimental work. All authors approved the final version of the manuscript to be submitted for publication and agreed to be accountable for all aspects of the work in ensuring that questions related to the accuracy and integrity of any part of the work are appropriately investigated and resolved.
Funding
This project has received funding from the Agricultural University of Athens and the Benaki Phytopathological Institute.
Conflict of Interest Statement
The authors declare that the research was conducted in the absence of any commercial or financial relationships that could be construed as a potential conflict of interest.
Acknowledgments
We are grateful to Assistant Researcher PV Mylona from ELGO-“Demetra,” Agricultural Research Center of Northern Greece, for providing the Skoutari and EMI eggplant cultivar seeds. We are also grateful to Joseph Pentheroudakis for carefully editing our manuscript. Finally, we would also like to thank Sylvia Pentheroudaki for graphical editing.
Footnotes
- ^ www.eggplantgenome.org, 2017
References
Abd El-Rahim, M. F., Fahmy, G. M., and Fahmy, Z. M. (1998). Alterations in transpiration and stem vascular tissues of two maize cultivars under conditions of water stress and late wilt disease. Plant Pathol. 47, 216–223.
Achuo, E. A., Prinsen, E., and Hofte, M. (2006). Influence of drought, salt stress and abscisic acid on the resistance of tomato to Botrytis cinerea and Oidium neolycopersici. Plant Pathol. 55, 178–186.
Aime, S., Cordier, C., Alabouvette, C., and Olivain, C. (2008). Comparative analysis of PR gene expression in tomato inoculated with virulent Fusarium oxysporum f. sp. Lycopersici and the biocontrol strain F. oxysporum Fo47. Physiol. Mol. Plant Pathol. 73, 9–15.
Angelopoulou, D. J., Naska, E. J., Paplomatas, E. J., and Tjamos, S. E. (2014). Biological control agents (BCAs) of verticillium wilt: influence of application rates and delivery method on plant protection, triggering of host defence mechanisms and rhizosphere populations of BCAs. Plant Pathol. 63, 1062–1069 doi: 10.1111/ppa.12198
Aroca, R., Vernieri, P., and Ruiz-Lozano, J. M. (2008). Mycorrhizal and nonmycorrhizal Lactuca sativa plants exhibit contrasting responses to exogenous ABA during drought stress and recovery. J. Exp. Bot. 59, 2029–2041.
Asano, T., Hayashi, N., Kobayashi, M., Aoki, N., Miyao, A., Mitsuhara, I., et al. (2012). A rice calcium-dependent protein kinase OsCPK12 oppositely modulates salt-stress tolerance and blast disease resistance. Plant J. 69, 26–36.
Ashraf, M., and Harris, P. J. C. (2013). Photosynthesis under stressful environments: an overview. Photosynthetica 51, 163–190.
Atkinson, N. J., Lilley, C. J., and Urwin, P. E. (2013). Identification of genes involved in the response of Arabidopsis to simultaneous biotic and abiotic stresses. Plant Physiol. 162, 2028–2041. doi: 10.1104/pp.113.222372
Atkinson, N. J., and Urwin, P. E. (2012). The interaction of plant biotic and abiotic stresses: from genes to the field. J. Exp. Bot. 63, 3523–3543. doi: 10.1093/jxb/ers100
Audebert, A., Coyne, D. L., Dingkuhn, M., and Plowright, R. A. (2000). The influence of cyst nematodes (Heterodera sacchari) and drought on water relations and growth of upland rice in Cote d’Ivoire. Plant Soil 220, 235–242.
Barbierato, V., Toppino, L., Rinaldi, P., Sala, T., Bassolino, L., Vale, G., et al. (2016). Phenotype and gene expression analyses of the Rfo-sa1 resistant aubergine interaction with Fusarium oxysporum f. sp. melongenae and Verticillium dahliae. Plant Pathol. 65, 1297–1309.
Bates, L. S., Waldren, R. P., and Teare, I. D. (1973). Rapid determination of free proline for water-stress studies. Plant Soil 39, 205–207. doi: 10.1007/BF00018060
Bletsos, F., Thanassoulopoulos, C., and Roupakias, D. (1999). Water stress and Verticillium wilt severity on eggplant (Solanum melongena L.). J. Phytopathol. 147, 243–248.
Blok, W. J., Lamers, J. G., Termorshuizen, A. J., and Bollen, G. J. (2000). Control of soilborne plant pathogens by incorporating fresh organic amendments followed by tarping. Phytopathology 90, 253–259. doi: 10.1094/PHYTO.2000.90.3.253
Bootraa, T., Akhkha, A., Al-Shoaibi, A. A., and Alhejeli, A. M. (2010). Effect of water stress on growth and water use efficiency (WUE) of some wheat cultivars (Triticum durum) grown in Saudi Arabia. JTUSCI 3, 39–48.
Borden, S., and Higgins, V. J. (2002). Hydrogen peroxide plays a critical role in the defense response of tomato to Cladosporium fulvum. Physiol. Mol. Plant Pathol. 61, 227–236.
Buhtz, A., Hohe, A., Schwarz, D., and Grosch, R. (2017). Effects of Verticillium dahliae on tomato root morphology considering plant growth response and defense. Plant Pathol. 66, 667–676.
Cappelli, C., Stravato, V. M., Rotino, G. L., and Buonaurio, R. (1995). “Sources of resistance among Solanum spp. to an Italian isolate of Fusarium oxysporum f. sp. melongenae,” in Proceedings of the 9th Meeting of EUCARPI, Genetics and Breeding of Capsicum and Eggplant, eds A. Andrasfalvi, A. Moor, and L. Zatyko (Hungary: SINCOP), 221–224.
Chapin, F. S. III, Autumn, K., and Pugnaire F. (1993). Evolution of suites of traits in response to environmental stress. Am. Nat. 142(S1), S78–S92.
Choi, H. -K., Iandolino, A., Goes da Silva, F., and Cook, D. R. (2013). Water deficit modulates the response of Vitis vinifera to the Pierce’s disease pathogen Xylella fastidiosa. Mol. Plant Microbe Interact. 26, 643–657. doi: 10.1094/MPMI-09-12-0217-R
Choudhury, F. K., Rivero, R. M., Blumwald, E., and Mittler, R. (2017). Reactive oxygen species, abiotic stress and stress combination. Plant J. 90: 856–867. doi: 10.1111/tpj.13299
Collonnier, C., Fock, I., Kashyap, V., Rotino, G., Daunay, M., Lian, Y., et al. (2001). Applications of biotechnology in eggplant. Plant Cell Tiss Org. Cult. 65, 91–107.
Conrath, U., Beckers, G. J., Langenbach, C. J., and Jaskiewicz, M. R. (2015). Priming for enhanced defense. Annu. Rev. Phytopathol. 53, 97–119. doi: 10.1146/annurev-phyto-080614-120132
Daayf, F. (2015). Verticillium wilts in crop plants: pathogen invasion and host defense responses. Can. J. Plant Pathol. 37, 8–20, doi: 10.1080/07060661.2014.989908
De Ronde, J. A., Van Der Mescht, A., and Steyn, H. S. F. (2000). Proline accumulation in response to water and heat stress in cotton. Afr. Crop. Sci. J. 8, 85–92
Degara, L., De Pinto, M. C., and Tommasi, F. (2003). The antioxidant system vis-a-vis reactive oxygen species during plant-pathogen interaction. Plant Physiol. Biochem. 41, 863–870.
Dikilitas, M., Karakas, S., Hashem, A., Abd Allah, E. F., and Ahmad, P. (2016). “Oxidative stress and plant responses to pathogens under water conditions,” in Water Stress and Crop Plants: A Sustainable Approach, ed. P. Ahmad (Chichester: John Wiley & Sons, Ltd.,). doi: 10.1002/9781119054450.ch8
Enright, S., and Cipollini, D. (2011). Overlapping defense responses to water limitation and pathogen attack and their consequences for resistance to powdery mildew disease in garlic mustard, Alliaria petiolata. Chemoecology 21:89. doi: 10.1007/s00049-011-0072-8
Farooq, M., Wahid, A., Kobayashi, N., Fujita, D., and Basra, S. M. A. (2009). Plant drought stress: effects, mechanisms and management. Agron. Sustain. Dev. 29, 185–212.
Fu, Q. S., Yang, R. C., Wang, H. S., Zhao, B., Zhou, C. L., Ren, S. X., et al. (2013). Leaf morphological and ultrastructural performance of eggplant (Solanum melongena L.) in response to water stress. Photosynthetica 51:109. doi: 10.1007/s11099-013-0005-6
Fujita, M., Fujita, Y., Noutoshi, Y., Takahashi, F., Narusaka, Y., Yamaguchi-Shinozaki, K., et al. (2006). Crosstalk between abiotic and biotic stress responses: a current view from the points of convergence in the stress signaling networks. Curr. Opin. Plant Biol. 4, 436–442. doi: 10.1016/j.pbi.2006.05.014
Ganopoulos, I., Xanthopoulou, A., Mastrogianni, A., Drouzas, A., Kalivas, A., Bletsos, F., et al. (2015). High Resolution Melting (HRM) analysis in eggplant (Solanum melongena L.): A tool for microsatellite genotyping and molecular characterization of a Greek Genebank collection. Biochem. Syst. Ecol. 50, 64–71.
Gantasala, N. P., Papolu, P. K., Thakur, P. K., Kamaraju, D., Sreevathsa, R., and Rao, U. (2013). Selection and validation of reference genes for quantitative gene expression studies by real-time PCR in eggplant (Solanum melongena L). BMC Res Notes 6:312. doi: 10.1186/1756-0500-6-312
Gargallo-Garriga, A., Sardans, J., Perez-Trujillo, M., Rivas-Ubach, A., Oravec, M., and Vecerova, K., et al. (2014). Opposite metabolic responses of shoots and roots to drought. Sci. Rep. 4:6829.
Genin, S. (2010). Molecular traits controlling host range and adaptation to plants in Ralstonia solanacearum. New Phytol. 187, 920–928. doi: 10.1111/j.1469-8137.2010.03397.x
Gramazio, P., Blanca, J., Ziarsolo, P., Herraiz, F. J., Plazas, M., and Prohens, J. et al. (2016). Transcriptome analysis and molecular marker discovery in Solanum incanum and S. aethiopicum, two close relatives of the common eggplant (Solanum melongena) with interest for breeding. BMC Genomics 17:300. doi: 10.1186/s12864-016-2631-4
Grimmer, M. K., John Foulkes, M., and Paveley, N. D. (2012). Foliar pathogenesis and plant water relations: a review. J. Exp. Bot. 63, 4321–4331. doi: 10.1093/jxb/ers143
Gupta, A., Dixit, S. K., and Senthil-Kumar, M. (2016). Drought stress predominantly endures Arabidopsis thaliana to Pseudomonas syringae infection. Front. Plant Sci. 7:808 doi: 10.3389/fpls.2016.00808
Hanci, F., and Cebeci, E. (2014). Investigation of proline, chlorophyll andcarotenoids changes under water stress in some onion (Allium cepa L.) cultivars. Turk. J. Agric. Nat. Sci. 7, 1499–1504
Hasanuzzaman, M., Nahar, K., Alam, M. M., Roychowdhury, R., and Fujita, M. (2013). Physiological, biochemical, and molecular mechanisms of heat stress tolerance in plants. Int. J. Mol. Sci. 14, 9643–9684. doi: 10.3390/ijms14059643
Heath, R. L., and Packer, L. (1968). Photoperoxidation in isolated chloroplasts: I. Kinetics and stoichiometry of fatty acid peroxidation. Arch. Biochem. Biophys. 125, 189–198. doi: 10.1016/0003-9861(68)90654-1
Hirakawa, H., Shirasawa, K., Miyatake, K., Nunome, T., Negoro, S., and Ohyama, A., et al. (2014). Draft genome sequence of eggplant (Solanum melongena L.): the representative solanum species indigenous to the old world. DNA Res. 21, 649–660. doi: 10.1093/dnares/dsu027
Hsieh, T.-H., Lee, J.-T., Charng, Y.-Y., and Chan, M.-T. (2002). Tomato plants ectopically expressing arabidopsis cbf1 show enhanced resistance to water deficit stress. Plant Physiol. 130, 618–626, doi: 10.1104/pp.00678
Johnstone, M., Chatterton, S., Sutton, J. C., and Grodzinski, B. (2005). Net Carbon gain and growth of bell peppers, capsicum annuum ‘cubico’, following root infection by Pythium aphanidermatum. Phytopathology 95, 354–361.
Kissoudis, C., Seifi, A., Yan, Z., Islam, A. T., van der Schoot, H., and van de Wiel, C. C., et al. (2017). Ethylene and abscisic acid signaling pathways differentially influence tomato resistance to combined powdery mildew and salt stress. Front. Plant Sci. 7:2009. doi: 10.3389/fpls.2016.02009
Kissoudis, C., van de Wiel, C., Visser, R. G. F., and Van Der Linden, G. (2014). Enhancing crop resilience to combined abiotic and biotic stress through the dissection of physiological and molecular crosstalk. Front. Plant Sci. 5:207. doi: 10.3389/fpls.2014.00207
Koevoets, I. T., Venema, J. H., Elzenga, J. T. M., and Testerink, C. (2016). Roots withstanding their environment: exploiting root system architecture responses to abiotic stress to improve crop tolerance. Front. Plant Sci. 7:1335. doi: 10.3389/fpls.2016.01335
Kohler, J., Hernandez, J. A., Caravaca, F., and Roldan, A. (2008). Plantgrowth-promoting rhizobacteria and arbuscular mycorrhizal fungi modify alleviation biochemical mechanisms in water-stressed plants. Funct. Plant Biol. 35, 141–151.
Li, J., Jiang, M. M., Ren, L., Liu, Y., and Chen, H. Y. (2016). Identification and characterization of CBL and CIPK gene families in eggplant (Solanum melongena L.). Mol Genet Gen. 291, 1769–1781. doi: 10.1007/s00438-016-1218-8
Liang, X., Zhang, L., Natarajan, S. K., and Becker, D. F. (2013). Proline mechanisms of stress survival. Antioxid. Redox Signal. 19, 998–1011. doi: 10.1089/ars.2012.5074
Livak, K. J., and Schmittgen, T. D. (2001). Analysis of relative gene expression data using real-time quantitative PCR and the 2(-Delta Delta C(T)) Method. Methods 25, 402–408.
López-Escudero, F. J., Del Río, C., Caballero, J. M., and Blanco-López, M. A. (2004). Evaluation of olive cultivars for resistance to Verticillium dahliae. Eur. J. Plant Pathol. 110, 79–85.
Ma, S., Gong, Q., and Bohnert, H. J. (2006). Dissecting salt stress pathways. J. Exp. Bot. 57, 1097–1107. doi: 10.1093/jxb/erj098
Mahesh, H. M., Murali, M., Anup Chandra Pal, M., Melvin, P., and Sharada, M. S. (2017). Salicylic acid seed priming instigates defense mechanism by inducing PR-Proteins in Solanum melongena L. upon infection with Verticillium dahliae Kleb. Plant Physiol. Biochem. 117, 12–23. doi: 10.1016/j.plaphy.2017.05.012
Markakis, E. A., Tjamos, S. E., Antoniou, P. P., Paplomatas, E. J., and Tjamos, E. C. (2016). Biological control of Verticillium wilt of olive by Paenibacillus alvei, strain K165. BioControl 61, 293–303 doi: 10.1007/s10526-015-9669-0
Matus, J. T., Aquea, F., Espinoza, C., Vega, A., Cavallini, E., Dal Santo, S., et al. (2014). Inspection of the grapevine BURP superfamily highlights an expansion of RD22 genes with distinctive expression features in berry development and ABA-mediated stress responses. PLoS One 9:e110372. doi: 10.1371/journal.pone.0110372
Mayek-Perez, N., Garcia-Espinosa, R., Lopez-Castaneda, C., Acosta-Gallegos, J. A., and Simpson J. (2002). Water relations, histopathology and growth of common bean (Phaseolus vulgaris L.) during pathogenesis of Macrophomina phaseolina under drought stress. Physiol. Mol. Plant Pathol. 60, 185–195.
Mercado-Blanco, J. (2012). Biological control of Verticillium wilt of olive within an integrated disease management framework. IOBC/WPRC Bull. 79, 149–154.
Mibei, E. K., Owino, W. O., Ambuko, J., Giovannoni, J. J., and Onyango, A. N. (2018). Metabolomic analyses to evaluate the effect of water stress on selected African Eggplant accessions. J. Sci. Food Agric. 98, 205–216. doi: 10.1002/jsfa.8458
Miller, G., Schlauch, K., Tam, R., Cortes, D., Torres, M. A., Shulaev V., et al. (2009). The plant NADPH oxidase RbohD mediates rapid, systemic signaling in response to diverse stimuli. Sci. Signal. 2:ra45. doi: 10.1126/scisignal.2000448
Mittler, R. (2006). Abiotic stress, the field environment and stress combination. Trends Plant Sci. 11, 15–19.
Mittler, R., and Blumwald, E. (2010). Genetic engineering for modern agriculture: challenges and perspectives. Annu. Rev. Plant Biol. 61, 443–462.
Na, C., Shuanghua, W., Jinglong, F., Bihao, C., Jianjun, L., and Changming, C., et al. (2016). Overexpression of the Eggplant (Solanum melongena) NAC family transcription factor SmNAC suppresses resistance to bacterial wilt. Sci. Rep. 6:31568. doi: 10.1038/srep31568
Nejat, N., and Mantri, N. (2017). Plant immune system: crosstalk between responses to biotic and abiotic stresses the missing link in understanding plant defense. Curr Issues Mol Biol. 23, 1–16. doi: 10.21775/cimb.023.001
Pandey, P., Irulappan, V., Bagavathiannan, M. V., and Senthil-Kumar, M. (2017). Impact of combined abiotic and biotic stresses on plant growth and avenues for crop improvement by exploiting physio-morphological traits. Front. Plant Sci. 8:537. doi: 10.3389/fpls.2017.00537
Pandey, P., Ramegowda, V., and Senthil-Kumar, M. (2015). Shared and unique responses of plants to multiple individual stresses and stress combinations: physiological and molecular mechanisms. Front. Plant Sci. 6:723. doi: 10.3389/fpls.2015.00723
Pennypacker, B. W., Leath, K. T., Hill, R. R., and Jr. (1991). Impact of drought stress on the expression of resistance to Verticillium albo-atrum in Alfalfa. Phytopathology 81, 1014–1024. doi: 10.1094/Phyto-81-1014
Prasch, C. M., and Sonnewald, U. (2015), Signaling events in plants: stress factors in combination change the Picture. Environ. Exp. Bot. 114, 4–14.
Prime-A-Plant Group, Conrath, U., Beckers, G. J., Flors, V., García-Agustín, P., Jakab, G., et al. (2006). Priming: getting ready for battle. Mol. Plant Microbe Interact. 19, 1062–1071.
Ramegowda, V., and Senthil-Kumar, M. (2015). The interactive effects of simultaneous biotic and abiotic stresses on plants: mechanistic understanding from drought and pathogen combination. J. Plant Physiol. 176, 47–54. doi: 10.1016/j.jplph.2014.11.008
Ramirez, V., Coego, A., Lopez, A., Agorio, A., Flors, V., and Vera, P. (2009). Drought tolerance in Arabidopsis is controlled by the OCP3 disease resistance regulator. Plant J. 58, 578–591.
Rasmussen, S., Barah, P., Suarez-Rodriguez, M. C., Bressendorff, S., Friis, P., Costantino, P., et al. (2013). Transcriptome responses to combinations of stresses in Arabidopsis. Plant Physiol. 161, 1783–1794. doi: 10.1104/pp.112.210773
Reddy, A. R., Chaitanya, K. V., and Vivekenandan, M. (2004). Drought-induced responses of photosynthesis and antioxidant metabolism in higher plants. J. Plant Physiol. 161, 1189–1202.
Reusche, M., Thole, K., Janz, D., Truskina, J., Rindfleisch, S., Drubert, C., et al. (2012). Verticillium infection triggers VASCULAR-RELATED NAC DOMAIN7-dependent de novo xylem formation and enhances drought tolerance in Arabidopsis. Plant Cell 24, 3823–3837. doi: 10.1105/tpc.112.103374
Rizhsky, L., Liang, H., Shuman, J., Shulaev, V., Davletova, S., and Mittler, R. (2004). When defense pathways collide. The response of Arabidopsis to a combination of drought and heat stress. Plant Physiol. 134, 1683–1696. doi: 10.1104/pp.103.033431
Rodziewicz, P., Swarcewicz, B., Chmielewska, K., Wojakowska, A., and Stobiecki, M. (2014). Influence of abiotic stresses on plant proteomeand metabolome changes. Acta Physiol Plant 36, 1–19
Rotino, G. L., Sala, T., and Toppino, L. (2014). “Eggplant” in Alien Gene Transfer in Crop Plants, Vol. 2, eds A. Pratap and J. Kumar (Dordrecht: Springer Science Business Media), 381–409.
Savary, S., Ficke, A., Aubertot, J. N., and Hollier, C. (2012). Crop losses due to diseases and their implications for global food production losses and food security. Food Sec. 4, 519–537.
Schieber, M., and Chandel, N. S. (2014). ROS function in redox signaling and oxidative stress. Curr. Biol. CB 24, R453–R462.
Seo, Y. S., Chern, M., Bartley, L. E., Han, M., Jung, K. H., Lee, I., et al. (2011). Towards establishment of a rice stress response interactome. PLoS Genet. 7:e1002020. doi: 10.1371/journal.pgen.1002020
Shaik, R., and Ramakrishna, W. (2014). Machine learning approaches distinguish multiple stress conditions using stress-responsive genes and identify candidate genes for broad resistance in rice. Plant Physiol. 164, 481–495. doi: 10.1104/pp.113.225862
Sies, H. (2017). Hydrogen peroxide as a central redox signaling molecule in physiological oxidative stress: oxidative eustress. Redox Biol. 11, 613–619.
Sinha, R., Gupta, A., and Senthil-Kumar, M. (2017). Concurrent drought stress and vascular pathogen infection induce common and distinct transcriptomic responses in chickpea. Front. Plant Sci. 8:333. doi: 10.3389/fpls.2017.00333
Sinha, R., Gupta, A., and Senthil-Kumar, M. (2016). Understanding the impact of drought on foliar and xylem invading bacterial pathogen stress in chickpea. Front. Plant Sci. 7:902. doi: 10.3389/fpls.2016.00902
Sivamani, E., Bahieldin, A., Wraith, J. M., Al-Niemi, T., Dyer, W. E., Ho, T-H. D., et al. (2000). Improved biomass productivity and water use efficiency under water deficit conditions in transgenic wheat constitutively expressing the barley HVA1 gene. Plant Sci. 155, 1–9.
Suzuki, N., Rivero, R. M., Shulaev, V., Blumwald, E., and Mittler, R. (2014). Abiotic and biotic stress combinations. New Phytol. 203, 32–43. doi: 10.1111/nph.12797
Tweneboah, S., and Oh, S. K. (2017). Biological roles of NAC transcription factors in the regulation of biotic and abiotic stress responses in solanaceous crops. J. Plant Biotechnol. 44, 1–11. doi: 10.5010/JPB.2017.44.1.001
Verslues, P. E., and Sharma, S. (2010). Proline metabolism and its implications for plant-environment interaction. Arabidopsis Book 8:e0140
Wang, G., Zhang, S., Ma, X., Wang, Y., Kong, F., and Meng, Q. (2016). A stress-associated NAC transcription factor (SlNAC35) from tomato plays a positive role in biotic and abiotic stresses. Physiol. Plant. 158, 45–64. doi: 10.1111/ppl.12444
Wang,Y., Bao, Z., Zhu,Y., and Hua, J. (2009). Analysis of temperature modulation of plant defense against biotrophic microbes. Mol. Plant Microbe Interact. 22, 498–506. doi: 10.1094/MPMI-22-5-0498
Wiese, J., Kranz, T., and Schubert, S. (2004). Induction of pathogen resistance in barley by abiotic stress. Plant Biol. 6, 529–536.
Xiong, L., and Yang, Y. (2003). Disease resistance and abiotic stress tolerance in rice are inversely modulated by an abscisic acid–inducible mitogen-activated protein kinase. Plant Cell 15, 745–759. doi: 10.1105/tpc.008714
Xu, P., Chen, F., Mannas, J. P., Feldman, T., Sumner, L. W., and Roossinck, M. J. (2008). Virus infection improves drought tolerance. New Phytol. 180, 911–921. doi: 10.1111/j.1469-8137.2008.02627.x
Yang, L., Jue, D., Li, W., Zhang, R., Chen, M., and Yang, Q. (2013). Identification of miRNA from eggplant (Solanum melongena L.) by small RNA deep sequencing and their response to Verticillium dahliae infection. PLoS One 8:e72840. doi: 10.1371/journal.pone.0072840
Yang, X., Cheng, Y. F., Deng, C., Ma, Y, Wang, Z. W., Chen, X. H., et al. (2014). Comparative transcriptome analysis of eggplant (Solanum melongena L.) and turkey berry (Solanum torvum Sw.): phylogenomics and disease resistance analysis. BMC Genomics 15:412. doi: 10.1186/1471-2164-15-412
Yang, X., Deng, C., Zhang, Y., Cheng, Y., Huo, Q., and Xue, L. (2015). The WRKY transcription factor genes in eggplant (Solanum melongena L.) and Turkey Berry (Solanum torvum Sw.). Int. J. Mol. Sci. 16, 7608–626. doi: 10.3390/ijms16047608
Zhang, H., and Sonnewald, U. (2017). Differences and commonalities of plant responses to single and combined stresses. Plant J. 90, 839–855. doi: 10.1111/tpj.13557
Zhang, J., Yu, J., Pei, W., Li, X., Said, J., Song, M., et al. (2015). Genetic analysis of Verticillium wilt resistance in a backcross inbred line population and a meta-analysis of quantitative trait loci for disease resistance in cotton. BMC Genomics 16:577. doi: 10.1186/s12864-015-1682-2
Keywords: eggplant, Verticillium dahliae, water stress, combined stress, cultivar, plant growth, plant physiological, molecular responses
Citation: Tani E, Kizis D, Markellou E, Papadakis I, Tsamadia D, Leventis G, Makrogianni D and Karapanos I (2018) Cultivar-Dependent Responses of Eggplant (Solanum melongena L.) to Simultaneous Verticillium dahliae Infection and Drought. Front. Plant Sci. 9:1181. doi: 10.3389/fpls.2018.01181
Received: 15 January 2018; Accepted: 24 July 2018;
Published: 13 August 2018.
Edited by:
Elsa Ballini, Montpellier SupAgro, FranceReviewed by:
Cristina Nali, Università degli Studi di Pisa, ItalyYong Zhuang, Jiangsu Academy of Agricultural Sciences (JAAS), China
Robert D. Hancock, James Hutton Institute, United Kingdom
Copyright © 2018 Tani, Kizis, Markellou, Papadakis, Tsamadia, Leventis, Makrogianni and Karapanos. This is an open-access article distributed under the terms of the Creative Commons Attribution License (CC BY). The use, distribution or reproduction in other forums is permitted, provided the original author(s) and the copyright owner(s) are credited and that the original publication in this journal is cited, in accordance with accepted academic practice. No use, distribution or reproduction is permitted which does not comply with these terms.
*Correspondence: Dimosthenis Kizis, ZC5raXppc0BicGkuZ3I=
†These authors have contributed equally to this work
‡These authors have contributed equally to this work