- College of Horticulture, Gansu Agricultural University, Lanzhou, China
Hydrogen sulfide (H2S), as a signaling molecule, is involved in the regulation of growth and development in plants. Recent studies have indicated that H2S also plays important roles in regulating postharvest senescence of horticultural products. The focus of this review is to summarize the synthesis of H2S in plants and its potential roles in alleviating the senescence of cut flowers, fruits, and vegetables during postharvest storage. During postharvest of horticultural products, H2S could scavenge reactive oxygen species via promoting the activity of antioxidant enzymes, thereby, sustaining the integrity of the membrane. In fruits, H2S effectively enhanced the tolerance of chilling by increasing the content of proline and polyphenol compounds. During postharvest storage of perishable fruits and vegetables, H2S significantly alleviated decay, which was caused by fungi by inhibiting the growth of fungi spores. Moreover, H2S interacted with other molecules synergistically (NO) or antagonistically (ethylene) to alleviate senescence of horticultural products. At the transcriptional level, H2S regulated the expression of senescence-related genes, which were related to degradation of proteins and chlorophyll, to delay the senescence of horticultural products. Thus, H2S does not only possess positive antioxidant and antifungal properties, but also significantly regulates the senescence-related gene during postharvest of horticultural products. Future studies of H2S in postharvest storage should focus on its molecular mechanism in the posttranslational modifications of proteins as well as its safety attributes in treated fruits and vegetables.
Introduction
Hydrogen sulfide (H2S), a colorless gas with an odor of rotten eggs, is a new gaseous signaling molecule that regulates physiological processes in plants (Li et al., 2016b). Recently, many investigations have suggested that H2S is involved in various growth and development processes in plants including germination (Liu and Lal, 2015), lateral and adventitious root formation (Lin et al., 2012; Jia et al., 2015), stomatal movement (Scuffi et al., 2014; Papanatsiou et al., 2015; Jin and Pei, 2016), and photosynthesis (Duan et al., 2015). In addition, the roles of H2S in regulating plant responses to abiotic stress including drought stress (Chen et al., 2016; Jin et al., 2017), osmotic stress (Khan et al., 2017), salt stress (Lai et al., 2014), chilling stress (Fu et al., 2013), and heavy metal stress (Ali et al., 2015; Fang et al., 2017) were reported. Under abiotic stress, some researchers indicated that H2S could ameliorate the quality and nutrients of horticultural products such as Capsicum annuum L. (Kaya et al., 2018), Brassica napus L. (Qian et al., 2014), and Brassica pekinensis (Lour.) Rupr. cv. Kasumi F1 (Reich et al., 2016). In addition, a number of studies showed that H2S played an important role in delaying senescence of horticultural products during postharvest storage with examples in kiwifruits (Zhu et al., 2014), daylily (Liu et al., 2017), and cut flowers (Zhang et al., 2011). Recently, there were reviews that discussed H2S as a signal molecule or a versatile regulator in plant response to abiotic stress (Guo et al., 2016; Li et al., 2016b). However, its role in the postharvest life of horticultural products has not yet been summarized in a published review. Therefore, the main aim of this review is to summarize the importance roles of H2S in the postharvest life of horticultural products. This review concentrates on the mechanisms by which H2S is involved in maintaining the postharvest freshness such as by regulating antioxidant system, enhancing chilling tolerance, and inhibiting fungi growth. The interaction of H2S with other molecules and the regulation of senescence-related genes during postharvest storage process have also been discussed.
Generation of H2S in Plants
In higher plants, H2S emission was first observed by DeCormis in 1968 (Rennenberg, 1989). Subsequently, Wilson et al. (1978) found that many higher plants including pumpkin, cucumber, cantaloupe, corn, and soybean could release H2S dependent on light. The emission of H2S in leaf tissue of cucumber was reported by Sekiya et al. (1982). Until now, there have been six pathways through which H2S is generated in higher plants, and these could be summarized as follows: plants reduce sulfate to sulfide and incorporate it into organic metabolites. Before reduction, sulfate is activated by adenylation to form adenosine 50-phosphosulfate (APS), which is catalyzed by ATP sulfurylase (ATPS). In the plastids, APS is first reduced to sulfite by APS reductase (APR). Then, sulfite is further reduced to sulfide (including H2S) by ferredoxin-dependent sulfite reductase (SiR) (Takahashi et al., 2011; Figure 1A). Finally, sulfide is incorporated into the amino acid skeleton of O-acetylserine (OAS) by O-acetylserine thiol lyase (OAS-TL) to form cysteine, and its reverse reaction could release H2S (Li, 2015). Among these, OAS is a product from serine, which is catalyzed by serine acetyltransferase (SAT). Harrington and Smith (1980) first reported that the degradation of L-cysteine yielded H2S, pyruvate, and NH4+ in tobacco cells. Subsequently, some researchers found that the L-cysteine was catalyzed by L-cysteine desulfhydrase (L-CDes) to form H2S in other higher plants (Sekiya et al., 1982; Rennenberg, 1983; Xie et al., 2013). In addition, L-CDes also catalyzed the formation of L-alanine and elemental sulfur from L-cysteine. Then, the elemental sulfur could be reduced to H2S if a reductant was present (Papenbrock et al., 2007; Figure 1A). Two genes, AtNFS1 and AtNFS2, which encoded NifS-like cysteine desulfurase have been identified in Arabidopsis, catalyzing cysteine to form H2S (Léon et al., 2002). Whereas, Nagasawa et al. (1985, 1988) indicated that D-cysteine was decomposed into H2S by D-cysteine desulfhydrase (D-CDes) in Escherichia coli and Pseudomonas putida. The D-CDes was isolated from Arabidopsis thaliana and it could catalyze D-cysteine to release H2S (Riemenschneider et al., 2005). However, L-CDes and D-CDes were different in substrates, enzymatic inhibitors, and subcellular localization (Guo et al., 2016). Besides, the β-cyanoalanine synthase (CAS) catalyzed L-cysteine and cyanide to form H2S and β-cyanoalanine (Hatzfeld et al., 2000). Jost et al. (2000) found the three genes (CYS-C1, CYS-D1, and CYS-D2), which encoded CAS in Arabidopsis (Figure 1A). Therefore, H2S was released from L-cysteine in the presence of hydrogen cyanide, which was catalyzed by CAS in plants. The H2S, especially endogenous H2S, which acts as a signaling molecule, can move freely from one plant cell to another. Thus, endogenous H2S may play important roles in plants.
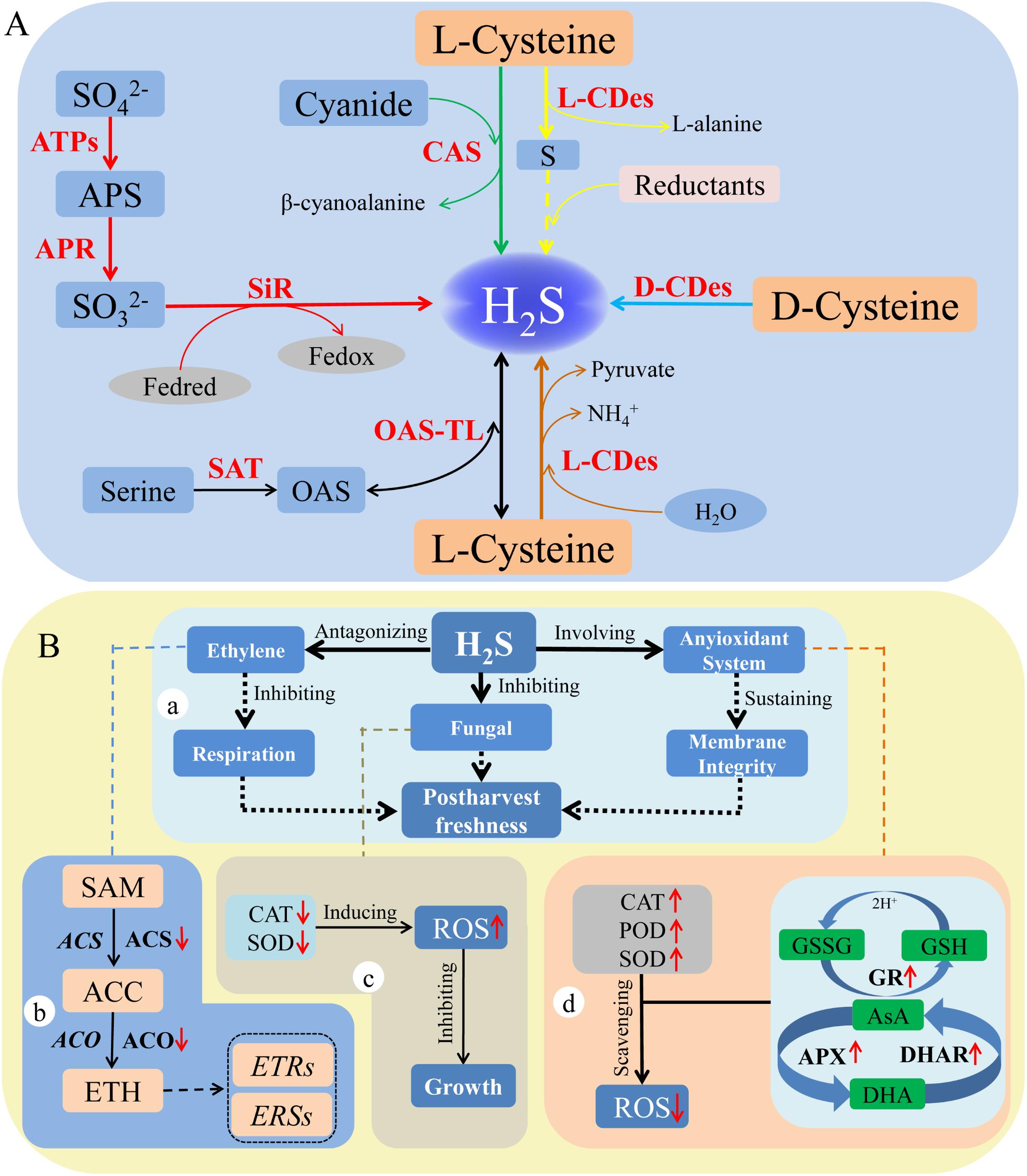
FIGURE 1. Production and function of hydrogen sulfide in plants. Production (A): The red arrows indicate sulfite is reduced to H2S (Takahashi et al., 2011); the green arrows indicate H2S is produced from L-CDes catalytic pathway (Harrington and Smith, 1980); the yellow arrows show H2S generation in the presence of reductants (Papenbrock et al., 2007); the light blue arrows represent H2S generated from the pathway catalyzed by D-CDes (Riemenschneider et al., 2005); the brown arrows show the H2S was generated from the reaction of L-cysteine and cyanide (Hatzfeld et al., 2000); the black arrows show H2S is produced from the reverse reaction of synthesis of cysteine (Li, 2015). The red words represent the key enzymes involving in various pathways. Function (B): a, the roles of H2S in delaying senescence and decay of horticultural products. b, the involvement of H2S in the generation and signaling of transduction of ethylene. c, the effect of H2S on antioxidant enzymes in vivo the fungi. d, the roles of H2S in regulating the antioxidant system in horticultural plants.
Regulatory Roles of H2S in Antioxidant System During Harvested Storage
Reactive oxygen species (ROS) caused by oxidative damage could accelerate senescence and ripening of horticultural products during postharvest storage. Recent investigations suggested that H2S could delay the senescence and ripening of horticultural products by alleviating the oxidative damage caused during postharvest storage. During postharvest of cut flowers, exogenous H2S could delay senescence of cut flowers by enhancing the activity of antioxidant enzymes (Figure 1B). For example, Zhang et al. (2011) found that a lower level of hydrogen peroxide (H2O2) and superoxide anion (O2-) in cut Erigeron annuus (L.) and Salix matsudana Koidz were sustained by exogenous H2S (Table 1). That is because the activities of catalase (CAT), superoxide dismutase (SOD), ascorbate peroxidase (APX), and peroxidase (POD) were increased by H2S. Thus, H2S could delay senescence of cut flowers by decreasing ROS-induced oxidant damage. During postharvest of fruits, Hu et al. (2012) indicated that H2S did not only improve the activities of CAT and POD, but also increased activities of APX and glutathione reductase (GR) during strawberry postharvest storage. Furthermore, H2S could also decrease the activity of lipoxygenase (LOX), which is responsible for catalyzing oxygenation of polyunsaturated fatty acids (Hu et al., 2012). Therefore, H2S played an antioxidant role in protecting tissue against the damaging effects of ROS. Studies on fresh-cut kiwifruit (Gao et al., 2013) and grape (Ni et al., 2016) were consistent with the previously mentioned results. Hu et al. (2014a) suggested that H2S could delay senescence of mulberry fruit by enhancing the activity of antioxidant enzymes, which could scavenge the ROS. Thus, H2S reduced the damage of lipid peroxidation and membrane by scavenging ROS, thereby, alleviating the senescence and ripening of fruits (Figure 1B). During postharvest of vegetables, Hu et al. (2015) reported that the postharvest senescence of water spinach was alleviated by H2S. In this process, H2S improved antioxidant capacity and sustained the energy status, which decreased the degree of leaf yellowing of water spinach. The redox balance in the senescence of daylily was promoted by H2S, which mainly reduced the overaccumulation of ROS via increasing the activity of CAT, APX, and SOD (Liu et al., 2017; Table 1). In addition, Sun et al. (2015) suggested that H2S could regulate phenolic metabolism to alleviate browning of fresh-cut lotus root slices, and the role of H2S in alleviating enzymatic browning was through inhibiting the activity of polyphenol oxidase (PPO) during postharvest storage. Thus, H2S acted as a gaseous regulator to increase the activities of antioxidant enzymes to affect the accumulation of ROS. As mentioned earlier, H2S alleviated the damage of lipid peroxidation and delayed the postharvest senescence of cut flowers, fruits, and vegetables. However, the detailed mechanism of H2S regulating the activity of antioxidant enzymes still needs further research in order to be well understood.
Involvement of H2S in Postharvest Chilling Stress
Cold storage is widely used to delay senescence and ripening of fruits during postharvest handling. However, storage at low non-freezing temperatures causes chilling stresses for fruits like banana (Luo et al., 2015) and peach (Cao et al., 2018), which are extremely sensitive to chilling injury. Chilling injury not only retards shelf life, but also reduces quality of fruits in the postharvest storage process. In recent years, some researchers reported that H2S could alleviate chilling injury, which is caused by the low temperature, during postharvest storage. Luo et al. (2015) found that H2S fumigation could alleviate chilling injury of harvested banana by promoting the antioxidant capacity and increasing the content of proline. During that process, the activities of antioxidant enzymes like SOD, CAT, and APX were increased by H2S, for these to scavenge ROS (Table 1). Aghdam et al. (2018) also found that H2S could regulate the activities of antioxidant enzymes to sustain the lower malondialdehyde (MDA), ameliorating chilling injury of hawthorn fruit. It showed that H2S could increase the activity of antioxidant enzymes to decrease the membrane lipid peroxidation, so as to sustain the high tolerance for chilling. In parallel, the content of polyphenol increased in H2S treatment, and this was because the activity of phenylalanine ammonia lyase was increased, while the activity of PPO was decreased by H2S (Luo et al., 2015). Furthermore, proline content was also elevated by H2S, which resulted from the increase of the activity of 1-pyrroline-5-carboxylate synthetase and the decrease of activity of proline dehydrogenase. Proline, as an osmotic substance, could maintain the high cytosolic concentration of the cell to increase the ice point. This indicates that H2S could directly or indirectly alleviate chilling injury by increasing the content of proline. Li et al. (2016a) suggested that H2S could improve the tolerance of banana to chilling injury by promoting the activities of the energy metabolism-related enzymes such as H+-ATPase, Ca2+-ATPase, cytochrome C oxidase, and succinate dehydrogenase. In addition, the quality of banana such as firmness was also sustained by H2S under chilling condition (Li et al., 2016a; Table 1). Thus, H2S seemly regulated the content of osmotic substances, sustaining the tolerance to chilling stress. Taken together, it could be seen that the tolerance to chilling injury of fruits was promoted by H2S, which sustained the integrity of the membrane and maintained sufficient energy via regulating activities of enzymes and content of osmotic substances.
Inhibition of Fungal Growth by H2S in Postharvest Horticultural Products
Generally, decay of some perishable horticultural products happens during storage and transportation. The main reason behind decay is the activity of plant fungal pathogens, which is usually promoted by wounds. In recent years, some research has found that H2S, applied as a regulator or fungicide, could alleviate senescence and decay of postharvest horticultural products by inhibiting the growth of fungi. Fu et al. (2014) reported that exogenous H2S (0.5 mM NaHS solution) effectively decreased the decay of fruits such as apple, kiwifruit, pear, sweet orange, mandarin, and tomato by inhibiting the colony growth of Aspergillus niger and Penicillium italicum, which mainly inhibited spore germination, germ tube elongation, and mycelial growth (Table 1). This was possible because H2S induced the accumulation of ROS in A. niger by decreasing the activity of antioxidant enzymes including CAT and SOD (Figure 1B). Contrarily, the investigations in postharvest freshness of horticultural plants suggested that H2S could scavenge ROS accumulation by promoting the activities of CAT, SOD, APX, and GR (Zhang et al., 2011; Hu et al., 2012; Liu et al., 2017). Fu et al. (2014) also attributed the different effects of H2S on microbes and plants to their different tolerances to H2S. Hence, the low level H2S (0.5 mM NaHS solution) seemly induced the accumulation of ROS in fungal in vivo by decreasing the activities of antioxidant enzymes, thereby, inhibiting the growth of fungi during storage (Figure 1B). However, the higher level H2S generally was used as a fungicide to inhibit the growth and infection of fungi. Wu et al. (2018) demonstrated that the spore germination rate, mycelial development diameter, and pathogenicity of Monilinia fructicola were inhibited by H2S, especially 50 mM NaHS solution for 2 h, which alleviated brown rot of peach fruit, caused by M. fructicola. Hu et al. (2014b) found that H2S delayed the shelf life of fresh-cut slices of pear and alleviated rots by inhibiting the growth of A. niger and Penicillium expansum (Table 1). The inhibition of mycelium growth of A. niger and P. expansum on medium depended on the H2S concentrations, which was found totally inhibited at 2 mM NaHS treatment. Moreover, H2S also blocked the decrease of reducing sugar and soluble protein during postharvest storage of pear (Hu et al., 2014b). The role of H2S in inhibiting senescence and decay of fresh-cut sweet potato during postharvest also depended on exogenous H2S concentration, especially on the optimal concentration of 2 mM NaHS (Tang et al., 2014). Interestingly, H2S alleviated the decay of sweet potato via inhibiting the growth of fungal pathogens including Rhizopus nigricans, Mucor rouxianus, and Geotrichum candidum, which were isolated from sweet potato tissue, which was infected by black or soft rot (Tang et al., 2014; Table 1). Consequently, H2S (at the higher concentration) as a fungicide inhibited the growth and infection of fungi during postharvest storage. As mentioned earlier, the low level H2S may be acts as a gaseous regulator to affect the accumulation of ROS, while it appeared it played the role of a fungicide at high concentrations. Furthermore, H2S might simultaneously play both a regulator and fungicide role in inhibiting the growth and infection of fungi. Although H2S could induce the ROS accumulation in vivo to inhibit fungal infection, its role in regulating the content of ROS has still not been extensively reported. Therefore, the detailed mechanisms of H2S in the inhibition of the fungal community need to be studied.
Interaction of H2S and Other Molecules in Postharvest Senescence
In recent studies, the synergistic or antagonistic roles of H2S with other molecules in postharvest senescence of horticultural products were reported. Zhang et al. (2014) reported the synergistic role of H2S and nitric oxide (NO) in alleviating decay and softening of strawberry. Cotreatment with H2S (0.8 mM NaHS solution) and NO (5 μM SNP solution) significantly maintained the quality (crust color and firmness) of strawberry and also inhibited its respiration rate and decay. Moreover, H2S was combined with NO to increase the activities of chitinase and beta-1,3-glucanase, which played vital roles in ameliorating damages caused by pathogen. The lower activities of pectin methylesterase (PME), polygalacturonase (PG), and endo-β-1,4-glucanase (EGase) that were related to softening of fruits were sustained by cotreatment with H2S and NO (Zhang et al., 2014). Based on these results, the authors concluded that the synergistic effect of H2S and NO could significantly delay senescence and alleviate decay of fruits. However, the mechanism of synergistic effect between H2S and NO on senescence of harvested produce has not been extensively reported. In another study, Al Ubeed et al. (2017) found that H2S alleviated the senescence and postharvest deterioration of pak choy by inhibiting the ethylene generation and ethylene action during storage. 1-Methylcyclopropene (1-MCP), as a competitive inhibitor of the action of ethylene, could inhibit the production of endogenous ethylene. Al Ubeed et al. (2018) found that 1-MCP and H2S were equally effective in inhibiting respiration and endogenous ethylene production when pak choy was stored in the absence of exogenous ethylene. H2S also prolonged postharvest senescence and ripening of banana during storage via antagonizing the effect of ethylene (Ge et al., 2017). It is well known that the ripening and senescence of fruits would be accelerated by the generation of ethylene. This is because ethylene accelerates respiration and other biochemical processes such as increasing cell membrane permeability and organic transformation. Thus, H2S, as a signaling molecule, significantly alleviates the senescence and ripening of fruits by interfering with ethylene synthesis during storage of horticultural products. Moreover, H2S also regulated the expression of ethylene synthesis genes and ethylene receptor genes at the transcriptional level. During ripening and senescence of kiwifruit, the expression of genes including AdSAM, AdACS1, AdACS2, AdACO2, and AdACO3, which were related to ethylene synthesis, was inhibited by H2S (Li et al., 2017; Figure 1B). However, during postharvest storage of a banana, H2S upregulated the expression of ethylene receptor genes such as MaETR, MaERS1, and MaERS2, which were at lower levels of expression in the presence of ethylene (Ge et al., 2017; Figure 1B). It has been indicated that H2S regulated the ethylene generation and its signal transduction at the transcriptional level. Higher levels of nutrients such as ascorbic acid, soluble protein, and reducing sugar were sustained by H2S during the postharvest storage of kiwifruit (Li et al., 2017). H2S also alleviated the yellowing and softening of banana peel, which was caused by ethylene and evidently decreased the activity of PG, which was involved in cell wall degradation (Ge et al., 2017). It can be deduced that H2S does not only regulates generation and signal transduction of ethylene, but also alleviates the deterioration of quality caused by ethylene of physiological parameters. To sum up, the synergistic or antagonistic effects of H2S with other molecules might play vital roles in the regulation of senescence and quality of harvested fruits and vegetables.
Senescence-Related Genes Regulated by H2S
Postharvest senescence is a universally phenomenon for horticultural products, and this seriously affects the shelf life and quality of fruits and vegetables during storage. During senescence, the degradation of some proteins and chlorophyll occurs, and this is regulated by certain genes. The role of H2S in regulating senescence-related genes during postharvest storage is discussed in this review. Li et al. (2014) indicated that the relative expressions of BoSGR, BoCLH2, BoPaO, and BoRCCR, which were related to chlorophyll degradation, were downregulated by H2S during postharvest storage of broccoli. In the storage of broccoli, apart from BoSGR and BoRCCR, other chlorophyll degradation-related genes such as BoNYC, BoCLH1, and BoPPH were also downregulated by H2S (Li et al., 2015). Thus, H2S regulated the expression of chlorophyll degradation-related genes to inhibit degradation of chlorophyll, thereby, effectively alleviating the postharvest yellowing of broccoli. In addition, Li et al. (2015) showed that the expression of BoCP3 (cysteine protease gene) and BoLSC807 (aspartic protease gene) was inhibited by H2S, suggesting that H2S inhibited the degradation of proteins during the senescence process in broccoli. Meanwhile, H2S cloud downregulate the expression of the LOX gene, BoLOX1, which catalyzed the hydroperoxidation of polyunsaturated fatty acids during senescence in broccoli (Li et al., 2014). The expressions of MdLOX2 and MdPPO (encoding PPO) were also downregulated by H2S during storage of apples (Zheng et al., 2016). It can be deduced that H2S delayed the senescence of fruits and vegetables by decreasing the expression of lipid peroxidation-related genes during postharvest storage. Generally, the genes that regulated the synthesis and signal transduction of ethylene were classified as senescence-related genes. Li et al. (2015) found that the expression of BoACS2 and BoACS3, which were key enzymes for ethylene generation, were downregulated by H2S during broccoli postharvest storage. Meanwhile, the expressions of ethylene signal transduction genes, MdERS1 (ethylene response sensor 1) and MdETR1 (ethylene receptor 1), were also inhibited by H2S during the storage of apples (Zheng et al., 2016). Therefore, H2S could inhibit the gene expression of ethylene generation and signal transduction, thereby, alleviating the senescence of postharvest horticultural products. Taken together, H2S regulates the expression of senescence-related genes and delays the senescence of horticultural products during storage. However, the signaling transduction pathway of H2S delaying senescence of horticultural products still has not been extensively reported.
Conclusion
Recently, a number of studies have suggested that H2S played a significant role in maintaining the postharvest freshness of horticultural products. Presently, available reports have suggested that H2S promotes postharvest life of horticultural products mainly by increasing the activity of antioxidant enzymes, alleviating chilling stress, inhibiting the growth of fungi, interacting with other molecules, and regulating senescence-related genes. Consequently, H2S, as a gaseous regulator or signaling molecules, may regulate various physiological and biochemical processes during postharvest storage.
For now, the role of H2S, in alleviating the senescence and decreasing quality, is mainly studied based on the antioxidant system and ethylene generation. However, the detailed mechanisms of H2S, as a gaseous regulator to inhibit the growth of fungi and regulate activities of antioxidant enzymes, have not been reported. Meanwhile, the receptor proteins of H2S regulating the senescence of plants have not been clearly reported at present. The interaction of H2S with other molecules may exert significantly roles in the harvested storage of horticultural products, while its in-depth mechanisms have not been reported in detail until now. In addition, the safety of H2S in the food chain, which is important to be considered in the consumption of fruits and vegetables, has also not been reported. Therefore, it is suggested that the future study of H2S in postharvest storage should focus on its molecular mechanisms and its interaction with other molecules. Furthermore, the toxicity of its residues in treated fruits and vegetables should be paid more attention to in future studies.
Author Contributions
WL provided the idea and revised the paper. JH prepared the manuscript. DH and JZ discussed the article structure. HF and BW finished the figures and tables. CW critically evaluated the manuscript. All the authors read and approved the submission of the manuscript.
Funding
This work was supported by the National Natural Science Foundation of China (Nos. 31560563 and 31160398) and Feitian Excellent Talents in Gansu China.
Conflict of Interest Statement
The authors declare that the research was conducted in the absence of any commercial or financial relationships that could be construed as a potential conflict of interest.
References
Aghdam, M. S., Mahmoudi, R., Razavi, F., Rabiei, V., and Soleimani, A. (2018). Hydrogen sulfide treatment confers chilling tolerance in hawthorn fruit during cold storage by triggering endogenous H2S accumulation, enhancing antioxidant enzymes activity and promoting phenols accumulation. Sci. Hortic. 238, 264–271. doi: 10.1016/j.scienta.2018.04.063
Al Ubeed, H. M. S., Wills, R. B. H., Bowyer, M. C., and Golding, J. B. (2018). Comparison of hydrogen sulphide with 1-methylcyclopropene (1-MCP) to inhibit senescence of the leafy vegetable, pak choy. Postharvest Biol. Technol. 137, 129–133. doi: 10.1016/j.postharvbio.2017.11.020
Al Ubeed, H. M. S., Wills, R. B. H., Bowyer, M. C., Vuong, Q. V., and Golding, J. B. (2017). Interaction of exogenous hydrogen sulphide and ethylene on senescence of green leafy vegetables. Postharvest Biol. Technol. 133, 81–87. doi: 10.1016/j.postharvbio.2017.07.010
Ali, B., Qian, P., Sun, R., Farooq, M. A., Gill, R. A., Wang, J., et al. (2015). Hydrogen sulfide alleviates the aluminum-induced changes in Brassica napus as revealed by physiochemical and ultrastructural study of plant. Environ. Sci. Pollut. Res. 22, 3068–3081. doi: 10.1007/s11356-014-3551-y
Cao, S., Shao, J., Shi, L., Xu, L., Shen, Z., Chen, W., et al. (2018). Melatonin increases chilling tolerance in postharvest peach fruit by alleviating oxidative damage. Sci. Rep. 8:806. doi: 10.1038/s41598-018-19363-5
Chen, J., Shang, Y. T., Wang, W. H., Chen, X. Y., He, E. M., Zheng, H. L., et al. (2016). Hydrogen sulfide-mediated polyamines and sugar changes are involved in hydrogen sulfide-induced drought tolerance in Spinacia oleracea seedlings. Front. Plant Sci. 7:1173. doi: 10.3389/fpls.2016.01173
Duan, B., Ma, Y., Jiang, M., Yang, F., Ni, L., and Lu, W. (2015). Improvement of photosynthesis in rice (Oryza sativa L.) as a result of an increase in stomatal aperture and density by exogenous hydrogen sulfide treatment. Plant Growth Regul. 75, 33–44. doi: 10.1007/s10725-014-9929-5
Fang, H., Liu, Z., Long, Y., Liang, Y., Jin, Z., Zhang, L., et al. (2017). The Ca2+/CaM2 binding transcription factor TGA3 elevates LCD expression and H2S production to bolster Cr6+ tolerance in Arabidopsis. Plant J. 91,1038–1050. doi: 10.1111/tpj.13627
Fu, L. H., Hu, K. D., Hu, L. Y., Li, Y. H., Hu, L. B., Yan, H., et al. (2014). An antifungal role of hydrogen sulfide on the postharvest pathogens Aspergillus niger and Penicillium italicum. PLoS One 9:e104206. doi: 10.1371/journal.pone.0104206
Fu, P., Wang, W., Hou, L., and Liu, X. (2013). Hydrogen sulfide is involved in the chilling stress response in Vitis vinifera L. Acta Soc. Bot. Pol. 82:295. doi: 10.5586/asbp.2013.031
Gao, S. P., Hu, K. D., Hu, L. Y., Li, Y. H., Han, Y., Wang, H. L., et al. (2013). Hydrogen sulfide delays postharvest senescence and plays an antioxidative role in fresh-cut kiwifruit. HortScience 48, 1385–1392.
Ge, Y., Hu, K. D., Wang, S. S., Hu, L. Y., Chen, X. Y., Li, Y. H., et al. (2017). Hydrogen sulfide alleviates postharvest ripening and senescence of banana by antagonizing the effect of ethylene. PLoS One 12:e0180113. doi: 10.1371/journal.pone.0180113
Guo, H., Xiao, T., Zhou, H., Xie, Y., and Shen, W. (2016). Hydrogen sulfide: a versatile regulator of environmental stress in plants. Acta Physiol. Plant. 38, 16. doi: 10.1007/s11738-015-2038-x
Harrington, H. M., and Smith, I. K. (1980). Cysteine metabolism in cultured tobacco cells. Plant Physiol. 65, 151–155. doi: 10.1104/pp.65.1.151
Hatzfeld, Y., Maruyama, A., Schmidt, A., Noji, M., Ishizawa, K., and Saito, K. (2000). β-Cyanoalanine synthase is a mitochondrial cysteine synthase-like protein in spinach and Arabidopsis. Plant Physiol. 123, 1163–1172. doi: 10.1104/pp.123.3.1163
Hu, H., Liu, D., Li, P., and Shen, W. (2015). Hydrogen sulfide delays leaf yellowing of stored water spinach (Ipomoea aquatica) during dark-induced senescence by delaying chlorophyll breakdown, maintaining energy status and increasing antioxidative capacity. Postharvest Biol. Technol. 108, 8–20. doi: 10.1016/j.postharvbio.2015.05.003
Hu, H., Shen, W., and Li, P. (2014a). Effects of hydrogen sulphide on quality and antioxidant capacity of mulberry fruit. Int. J. Food Sci. Technol. 49, 399–409. doi: 10.1111/ijfs.12313
Hu, K. D., Wang, Q., Hu, L. Y., Gao, S. P., Wu, J., Li, Y. H., et al. (2014b). Hydrogen sulfide prolongs postharvest storage of fresh-cut pears (Pyrus pyrifolia) by alleviation of oxidative damage and inhibition of fungal growth. PLoS One 9:e85524. doi: 10.1371/journal.pone.0085524
Hu, L. Y., Hu, S. L., Wu, J., Li, Y. H., Zheng, J. L., Wei, Z. J., et al. (2012). Hydrogen sulfide prolongs postharvest shelf life of strawberry and plays an antioxidative role in fruits. J. Agric. Food Chem. 60, 8684–8693. doi: 10.1021/jf300728h
Jia, H., Hu, Y., Fan, T., and Li, J. (2015). Hydrogen sulfide modulates actin-dependent auxin transport via regulating ABPs results in changing of root development in Arabidopsis. Sci. Rep. 5:8251. doi: 10.1038/srep08251
Jin, Z., and Pei, Y. (2016). Hydrogen sulfide: the shutter button of stomata in plants. Sci. China Life Sci. 59, 1187–1188. doi: 10.1007/s11427-016-0265-3
Jin, Z., Wang, Z., Ma, Q., Sun, L., Zhang, L., Liu, Z., et al. (2017). Hydrogen sulfide mediates ion fluxes inducing stomatal closure in response to drought stress in Arabidopsis thaliana. Plant Soil 419, 141–152. doi: 10.1007/s11104-017-3335-5
Jost, R., Berkowitz, O., Wirtz, M., Hopkins, L., Hawkesford, M. J., and Hell, R. (2000). Genomic and functional characterization of the oas gene family encoding O-acetylserine (thiol) lyases, enzymes catalyzing the final step in cysteine biosynthesis in Arabidopsis thaliana. Gene 253, 237–247. doi: 10.1016/S0378-1119(00)00261-4
Kaya, C., Ashraf, M., and Akram, N. A. (2018). Hydrogen sulfide regulates the levels of key metabolites and antioxidant defense system to counteract oxidative stress in pepper (Capsicum annuum L.) plants exposed to high zinc regime. Environ. Sci. Pollut. Res. 25, 12612–12618. doi: 10.1007/s11356-018-1510-8
Khan, M. N., Mobin, M., Abbas, Z. K., and Siddiqui, M. H. (2017). Nitric oxide-induced synthesis of hydrogen sulfide alleviates osmotic stress in wheat seedlings through sustaining antioxidant enzymes, osmolyte accumulation and cysteine homeostasis. Nitric Oxide 68, 91–102. doi: 10.1016/j.niox.2017.01.001
Lai, D., Mao, Y., Zhou, H., Li, F., Wu, M., Zhang, J., et al. (2014). Endogenous hydrogen sulfide enhances salt tolerance by coupling the reestablishment of redox homeostasis and preventing salt-induced K+ loss in seedlings of Medicago sativa. Plant Sci. 225, 117–129. doi: 10.1016/j.plantsci.2014.06.006
Léon, S., Touraine, B., Briat, J. F., and Lobréaux, S. (2002). The AtNFS2 gene from Arabidopsis thaliana encodes a NifS-like plastidial cysteine desulphurase. Biochem. J 366, 557–564. doi: 10.1042/bj20020322
Li, S. P., Hu, K. D., Hu, L. Y., Li, Y. H., Jiang, A. M., Xiao, F., et al. (2014). Hydrogen sulfide alleviates postharvest senescence of broccoli by modulating antioxidant defense and senescence-related gene expression. J. Agric. Food Chem. 62, 1119–1129. doi: 10.1021/jf4047122
Li, T. T., Li, Z. R., Hu, K. D., Hu, L. Y., Chen, X. Y., and Li, Y. H. (2017). Hydrogen sulfide alleviates kiwifruit ripening and senescence by antagonizing effect of ethylene. HortScience 52, 1556–1562. doi: 10.21273/HORTSCI12261-17
Li, Z. G. (2015). Analysis of some enzymes activities of hydrogen sulfide metabolism in plants. Methods Enzymol. 555, 253–269. doi: 10.1016/bs.mie.2014.11.035
Li, D., Limwachiranon, J., Li, L., Du, R., and Luo, Z. (2016a). Involvement of energy metabolism to chilling tolerance induced by hydrogen sulfide in cold-stored banana fruit. Food Chem. 208, 272–278. doi: 10.1016/j.foodchem.2016.03.113
Li, Z. G., Min, X., and Zhou, Z. H. (2016b). Hydrogen sulfide: a signal molecule in plant cross-adaptation. Front. Plant Sci. 7:1621. doi: 10.3389/fpls.2016.01621
Li, Z. R., Hu, K. D., Zhang, F. Q., Li, S. P., Hu, L. Y., Li, Y. H., et al. (2015). Hydrogen sulfide alleviates dark-promoted senescence in postharvest broccoli. HortScience 50, 416–420.
Lin, Y. T., Li, M. Y., Cui, W. T., Lu, W., and Shen, W. B. (2012). Haem oxygenase-1 is involved in hydrogen sulfide-induced cucumber adventitious root formation. J. Plant Growth Regul. 31, 519–528. doi: 10.1007/s00344-012-9262-z
Liu, D., Xu, S., Hu, H., Pan, J., Li, P., and Shen, W. (2017). Endogenous hydrogen sulfide homeostasis is responsible for the alleviation of senescence of postharvest daylily flower via increasing antioxidant capacity and maintained energy status. J. Agric. Food Chem. 65, 718–726. doi: 10.1021/acs.jafc.6b04389
Liu, R., and Lal, R. (2015). Effects of low-level aqueous hydrogen sulfide and other sulfur species on Lettuce (Lactuca sativa) seed germination. Commun. Soil Sci. Plant Anal. 46, 576–587. doi: 10.1080/00103624.2014.998341
Luo, Z., Li, D., Du, R., and Mou, W. (2015). Hydrogen sulfide alleviates chilling injury of banana fruit by enhanced antioxidant system and proline content. Sci. Hortic. 183, 144–151. doi: 10.1016/j.scienta.2014.12.021
Nagasawa, T., Ishii, T., Kumagai, H., and Yamada, H. (1985). D-Cysteine desulfhydrase of Escherichia coli. Purification and characterization. FEBS J. 153, 541–551. doi: 10.1111/j.1432-1033.1985.tb09335.x
Nagasawa, T., Ishii, T., and Yamada, H. (1988). Physiological comparison of D-cysteine desulfhydrase of Escherichia coli with 3-chloro-D-alanine dehydrochlorinase of Pseudomonas putida CR 1-1. Arch. Microbiol. 149, 413–416. doi: 10.1007/BF00425580
Ni, Z. J., Hu, K. D., Song, C. B., Ma, R. H., Li, Z. R., Zheng, J. L., et al. (2016). Hydrogen sulfide alleviates postharvest senescence of grape by modulating the antioxidant defenses. Oxid. Med. Cell. Longev. 2016:4715651. doi: 10.1155/2016/4715651
Papanatsiou, M., Scuffi, D., Blatt, M. R., and García-Mata, C. (2015). Hydrogen sulfide regulates inward-rectifying K+ channels in conjunction with stomatal closure. Plant Physiol. 168, 29–35. doi: 10.1104/pp.114.256057
Papenbrock, J., Riemenschneider, A., Kamp, A., Schulz-Vogt, H. N., and Schmidt, A. (2007). Characterization of cysteine-degrading and H2S-releasing enzymes of higher plants-from the field to the test tube and back. Plant Biol. 9, 582–588. doi: 10.1055/s-2007-965424
Qian, P., Sun, R., Ali, B., Gill, R. A., Xu, L., and Zhou, W. (2014). Effects of hydrogen sulfide on growth, antioxidative capacity, and ultrastructural changes in oilseed rape seedlings under aluminum toxicity. J. Plant Growth Regul. 33, 526–538. doi: 10.1007/s00344-013-9402-0
Reich, M., Shahbaz, M., Prajapati, D. H., Parmar, S., Hawkesford, M. J., and De Kok, L. J. (2016). Interactions of sulfate with other nutrients as revealed by H2S fumigation of Chinese cabbage. Front. Plant Sci. 7:541. doi: 10.3389/fpls.2016.00541
Rennenberg, H. (1983). Cysteine desulfhydrase activity in cucurbit plants: Stimulation by preincubation with L-or D-cysteine. Phytochemistry 22,1557–1560. doi: 10.1016/0031-9422(83)80088-0
Rennenberg, H. (1989). “Synthesis and emission of hydrogen sulfide by higher plants,” in Fraunhofer Institute for Atmospheric Environmental Research, Vol. 393, (Garmisch-Partenkirchen: Federal Republic of Germany), 44–57. doi: 10.1021/bk-1989-0393.ch004
Riemenschneider, A., Wegele, R., Schmidt, A., and Papenbrock, J. (2005). Isolation and characterization of a D-cysteine desulfhydrase protein from Arabidopsis thaliana. FEBS J. 272, 1291–1304. doi: 10.1111/j.1742-4658.2005.04567.x
Scuffi, D., Alvarez, C., Laspina, N., Gotor, C., Lamattina, L., and Garcíamata, C. (2014). Hydrogen sulfide generated by l-cysteine desulfhydrase acts upstream of nitric oxide to modulate abscisic acid-dependent stomatal closure. Plant Physiol. 166, 2065–2076. doi: 10.1104/pp.114.245373
Sekiya, J., Schmidt, A., Wilson, L. G., and Filner, P. (1982). Emission of hydrogen sulfide by leaf tissue in response to L-cysteine. Plant Physiol. 70, 430–436. doi: 10.1104/pp.70.2.430
Sun, Y., Zhang, W., Zeng, T., Nie, Q., Zhang, F., and Zhu, L. (2015). Hydrogen sulfide inhibits enzymatic browning of fresh-cut lotus root slices by regulating phenolic metabolism. Food Chem. 177, 376–381. doi: 10.1016/j.foodchem.2015.01.065
Takahashi, H., Kopriva, S., Giordano, M., Saito, K., and Hell, R. (2011). Sulfur assimilation in photosynthetic organisms: molecular functions and regulations of transporters and assimilatory enzymes. Annu. Rev. Plant Biol. 62, 157–184. doi: 10.1146/annurev-arplant-042110-103921
Tang, J., Hu, K. D., Hu, L. Y., Li, Y. H., Liu, Y. S., and Zhang, H. (2014). Hydrogen sulfide acts as a fungicide to alleviate senescence and decay in fresh-cut sweetpotato. HortScience 49, 938–943.
Wilson, L. G., Bressan, R. A., and Filner, P. (1978). Light-dependent emission of hydrogen sulfide from plants. Plant Physiol. 61, 184–189. doi: 10.1104/pp.61.2.184
Wu, W., Zhang, C., Chen, L., Li, G., Wang, Q., and Shi, J. (2018). Inhibition of hydrogen sulfide and hypotaurine on Monilinia fructicola disease in peach fruit. Acta Hortic. 1194, 257–266. doi: 10.17660/ActaHortic.2018.1194.38
Xie, Y., Lai, D., Mao, Y., Zhang, W., Shen, W., and Guan, R. (2013). Molecular cloning, characterization, and expression analysis of a novel gene encoding L-cysteine desulfhydrase from Brassica napus. Mol. Biotechnol. 54, 737–746. doi: 10.1007/s12033-012-9621-9
Zhang, H., Hu, S. L., Zhang, Z. J., Hu, L. Y., Jiang, C. X., Wei, Z. J., et al. (2011). Hydrogen sulfide acts as a regulator of flower senescence in plants. Postharvest Biol. Technol. 60, 251–257. doi: 10.1016/j.postharvbio.2011.01.006
Zhang, C., Shi, J. Y., Zhu, L. Q., Li, C. L., and Wang, Q. G. (2014). Cooperative effects of hydrogen sulfide and nitric oxide on delaying softening and decay of strawberry. Int. J. Agric. Biol. Eng. 7, 114–122. doi: 10.3965/j.ijabe.20140706.014
Zheng, J. L., Hu, L. Y., Hu, K. D., Wu, J., Yang, F., and Zhang, H. (2016). Hydrogen sulfide alleviates senescence of fresh-cut apple by regulating antioxidant defense system and senescence-related gene expression. HortScience 51, 152–158.
Keywords: hydrogen sulfide (H2S), chilling injury, antioxidant system, antifungal, interactions, senescence-related genes
Citation: Huo J, Huang D, Zhang J, Fang H, Wang B, Wang C and Liao W (2018) Hydrogen Sulfide: A Gaseous Molecule in Postharvest Freshness. Front. Plant Sci. 9:1172. doi: 10.3389/fpls.2018.01172
Received: 10 May 2018; Accepted: 23 July 2018;
Published: 27 August 2018.
Edited by:
Yanjie Xie, Nanjing Agricultural University, ChinaReviewed by:
Yi Han, Hefei University of Technology, ChinaYanxi Pei, Shanxi University, China
Yongbo Liu, Chinese Research Academy of Environmental Sciences, China
Copyright © 2018 Huo, Huang, Zhang, Fang, Wang, Wang and Liao. This is an open-access article distributed under the terms of the Creative Commons Attribution License (CC BY). The use, distribution or reproduction in other forums is permitted, provided the original author(s) and the copyright owner(s) are credited and that the original publication in this journal is cited, in accordance with accepted academic practice. No use, distribution or reproduction is permitted which does not comply with these terms.
*Correspondence: Weibiao Liao, liaowb@gsau.edu.cn