- 1State Key Laboratory of Soil and Sustainable Agriculture, Institute of Soil Science, Chinese Academy of Sciences, Nanjing, China
- 2Division of Horticultural Sciences, Institute of Crop Science and Resource Conservation, University of Bonn, Bonn, Germany
- 3School of Agriculture and Food, Faculty of Veterinary and Agricultural Sciences, The University of Melbourne, Melbourne, VIC, Australia
Elevated atmospheric CO2 (eCO2) enhances the yield of vegetables and could also affect their nutritional quality. We conducted a meta-analysis using 57 articles consisting of 1,015 observations and found that eCO2 increased the concentrations of fructose, glucose, total soluble sugar, total antioxidant capacity, total phenols, total flavonoids, ascorbic acid, and calcium in the edible part of vegetables by 14.2%, 13.2%, 17.5%, 59.0%, 8.9%, 45.5%, 9.5%, and 8.2%, respectively, but decreased the concentrations of protein, nitrate, magnesium, iron, and zinc by 9.5%, 18.0%, 9.2%, 16.0%, and 9.4%. The concentrations of titratable acidity, total chlorophyll, carotenoids, lycopene, anthocyanins, phosphorus, potassium, sulfur, copper, and manganese were not affected by eCO2. Furthermore, we propose several approaches to improving vegetable quality based on the interaction of eCO2 with various factors, including species, cultivars, CO2 levels, growth stages, light, O3 stress, nutrient, and salinity. Finally, we present a summary of the eCO2 impact on the quality of three widely cultivated crops, namely, lettuce, tomato, and potato.
Introduction
The atmospheric CO2 concentration has increased from 280 μmol mol-1 before the industrial revolution to 408 μmol mol-1 now1 (March 2018) mainly due to fossil fuel combustion and deforestation. It is predicted to reach 1000 μmol mol-1 by the end of this century (IPCC, 2014). Elevated CO2 (eCO2) can promote net photosynthetic rates of plants and thus plant productivity and yield (Long et al., 2004). It also enhances plant tolerance to environmental stresses via increased soluble sugars, antioxidants, and root exudates (Drake et al., 2011; Huang and Xu, 2015). Therefore, eCO2 has been widely used as a gas fertilizer in greenhouse vegetable cultivation, particularly in recent decades as greenhouse technologies have improved (Mortensen, 1987; Bisbis et al., 2018) and the demand for vegetables is continuously increasing (Supplementary Figure S1).
Generally, eCO2 (700–1000 μmol mol-1) can promote the yield of vegetables (Gruda and Tanny, 2014). The sources of CO2 have changed from traditional straw bales and organic soils to relatively pure CO2 from industrial waste or CO2 generators (Gruda, 2005). Elevated CO2 has frequently been demonstrated to increase the yield of various crops, including vegetables (Kimball, 1983; Long et al., 2004). Elevated CO2 (from 355 to 800–900 μmol mol-1) increased the yield of lettuce, carrot, and parsley by 18%, 19%, and 17%, respectively (Mortensen, 1994). Optimizing other environmental factors with eCO2 further increased plant productivity and yield (Kirschbaum, 2011). Elevated CO2 (900 μmol mol-1) with additional light (ambient + 100 μmol m-2 s-1 photosynthetically active radiation or PAR) increased the early yield of tomato and pepper by 15% and 11%, respectively (Fierro et al., 1994). Elevated CO2 (600–700 μmol mol-1) increased the average root dry mass of sugar beet by 26% in high N availability (10 mM NO3-) and by 12% in 1 mM NO3- (Demmers-Derks et al., 1998). More examples of yield benefits for other vegetable crops are reviewed by Gruda (2005).
However, there is less information on the effect of CO2 concentration on the nutritional quality of vegetables (Gruda, 2005; Moretti et al., 2010). The effect of eCO2 on vegetable quality has been briefly reviewed (Idso and Idso, 2001; Gruda, 2005; Moretti et al., 2010; Bisbis et al., 2018). However, these reviews mainly focus on limited parameters of quality affected by various environmental factors. A comprehensive review of recent studies explaining and targeting the key role of the effect of eCO2 on vegetable quality is lacking. To address this knowledge gap, we conducted a meta-analysis on the eCO2 effect and its interaction with factors besides eCO2 on the quality of vegetables, and more specifically of three widely cultivated vegetables: lettuce, tomato, and potato. This information is critical for vegetable nutrition and food security under future climate change.
Methodology
Data Collection
A literature search was conducted for publications between 1990 and 2018 using the following databases: Web of Knowledge, Scopus, ScienceDirect, and Google Scholar. The keywords used were “vegetable,” “vegetable quality,” “quality,” “elevated carbon dioxide,” “eCO2,” “CO2 enrichment,” “FACE,” and “climate change.” The environmental factors “CO2 level,” “CO2 concentration,” “light intensity,” “light quality,” “temperature,” “heat stress,” “chilling stress,” “O3,” and “salinity” and the name of a particular vegetable were also used as keywords. The references cited in the obtained references were also collected. Strawberry, which is categorized as a vegetable in some countries, and potato, which is considered a vegetable as it is rich in ascorbic acid but regarded as staple food due to the large amount of accumulated starch, were included in the database search. The vegetables were classified as root vegetables, stem vegetables, leafy vegetables, and fruit vegetables. Root vegetables included carrot, radish, sugar beet, and turnip; stem vegetables included broccoli, celery, celtuce, Chinese kale, ginger, onion, potato, and scallion; leafy vegetables included cabbage, Chinese cabbage, chives, fenugreek, Hongfengcai, lettuce, oily sowthistle, palak, and spinach; fruit vegetables included cucumber, hot pepper, strawberry, sweet pepper, and tomato. As the common names of several vegetables are not commonly known worldwide, their Latin names are shown here: Chinese kale (Brassica oleracea L. var. alboglabra), fenugreek (Trigonella foenum-graecum L.), hongfengcai or guanyinxian (Gynura bicolor L.), oily sowthistle (Sonchus oleraceus L.), palak (Beta vulgaris L. var. allgreen), and celtuce (Lactuca sativa L. var. augustana).
Fifty-six journal articles and one conference article published in English and meeting the following criteria were included in this analysis: (1) the ambient CO2 for plant growth (rather than storage) was ≥200 and ≤450 μmol-1, while that of eCO2 was between 540 and 1200 μmol-1; (2) measurements of nutritional quality of vegetables were collected, including soluble sugar, organic acid, protein, nitrate, antioxidants, and minerals (see Tables in Supplementary Information). If a study involved several species, cultivars, growth stages, or was conducted for several years or under various CO2 levels, all the observations were regarded to be independent and included in the database. The data extracted from each study were the means and the replicate number of the measurements under both ambient CO2 and eCO2. For the values that cannot be directly extracted from tables and text, i.e., data in figures, the height of the columns in figures was measured to estimate the observation using ImageJ (version 1.51a, National Institutes of Health, United States).
Statistical Analysis
The significant level for comparing the effect of CO2 on nutritional quality, shown in Tables in Supplementary Information, was at p < 0.05. If no multiple comparisons were performed in the references, a two-tailed t-test was used to indicate the significance of the effect of eCO2 on vegetable quality based on the means, standard error/deviation, and the number of replicates using Microsoft Excel 2016. If only the minimal and maximal values (i.e., the range) of the treated CO2 concentration were given in a study, the treated CO2 concentration was estimated as their average, making it possible to calculate the ratio of concentration of eCO2 to ambient CO2 (Tables in Supplementary Information).
A meta-analysis was conducted to assess the effect of CO2 on vegetable quality on the well-reported variables, i.e., concentrations of fructose, glucose, sucrose, total soluble sugar, titratable acidity, total protein, nitrate, total antioxidant capacity, total phenols, total flavonoids, ascorbic acid, total chlorophyll, chlorophyll a, chlorophyll b, carotenoids, lycopene, anthocyanins, phosphorus (P), potassium (K), calcium (Ca), magnesium (Mg), sulfur (S), iron (Fe), manganese (Mn), copper (Cu), and zinc (Zn), following the methods described by Wang et al. (2012). The effect size metric was the response ratio:
where R, E, and A are the response ratio, and the means of quality response under eCO2 and ambient CO2, respectively. The technique of the natural logarithm-transformed ratio (ln R) was used for analysis to reduce biases toward increases (Hedges et al., 1999; Jablonski et al., 2002). Meta-analytic studies normally weigh the effect size by the reciprocal of their variance, which gives greater weight to experiments with greater precision. The effect size can also be weighted by the number of replicates (van Groenigen et al., 2011; Lam et al., 2012; Loladze, 2014). However, many eCO2 studies did not report the variation and sample size of the measurements. Therefore, the unweighted method (Loladze, 2014) was used in this work. The means and 95% confidence intervals of effect size were determined using the nonparametric bootstrap method (5,000 iterations) using the package bootES in R software (version 3.3.2) (Kirby and Gerlanc, 2013). The CO2 effect was considered as significant when the 95% confidence intervals did not overlap with zero. The effect size was back-transformed to ordinary percentage change to ease interpretation.
The potential publication bias in the meta-analysis was assessed based on the correlation of effect size (ln R) and the sample sizes/replicates for each study and each measurement regardless of the observations without reporting the number of replicates (Wang et al., 2012; Loladze, 2014). The funnel-shaped and symmetrical cloud of points (Supplementary Figure S2) indicates the absence of any significant publication bias (Egger et al., 1997).
Results and Discussion
Effect of eCO2 on Soluble Sugar and Acidity
Elevated CO2 promotes soluble sugar accumulation in the edible parts of vegetables. The increased CO2 fixation under eCO2 promotes the synthesis of triose phosphate in leaves (Long et al., 2004), which can be further transformed into other carbohydrates, e.g., glucose, fructose, and sucrose. Our meta-analysis showed that eCO2 increased the concentrations of glucose by 13.2%, fructose by 14.2%, sucrose by 3.7% (at p = 0.07), and total soluble sugar by 17.5% in terms of all vegetables (Figure 1). The increment of total soluble sugar in leaf (an organ for carbohydrate synthesis) under eCO2 was the greatest (36.2%) among all the classes of vegetables. The increment can reach 38–188% in the leaves of Chinese cabbage and 16–53% in the leaves of oily sowthistle (Jin et al., 2009). Compared to leafy vegetables, the increments of total soluble sugar were less in fruit and root vegetables, and were 8.5% and 16.3%, respectively. This indicates that the synthesized carbohydrates in leaves cannot be fully translocated to fruits as well as to roots, although one needs to be cautious regarding the species variation. For example, eCO2 (950 μmol mol-1) increased total soluble sugar in strawberry fruits by 20% relative to 350 μmol mol-1 (Wang and Bunce, 2004). Similarly, the total soluble sugar was increased by 13% in radish and 20% in turnip under 1,000 μmol mol-1 CO2 compared to 400 μmol mol-1 control (Azam et al., 2013).
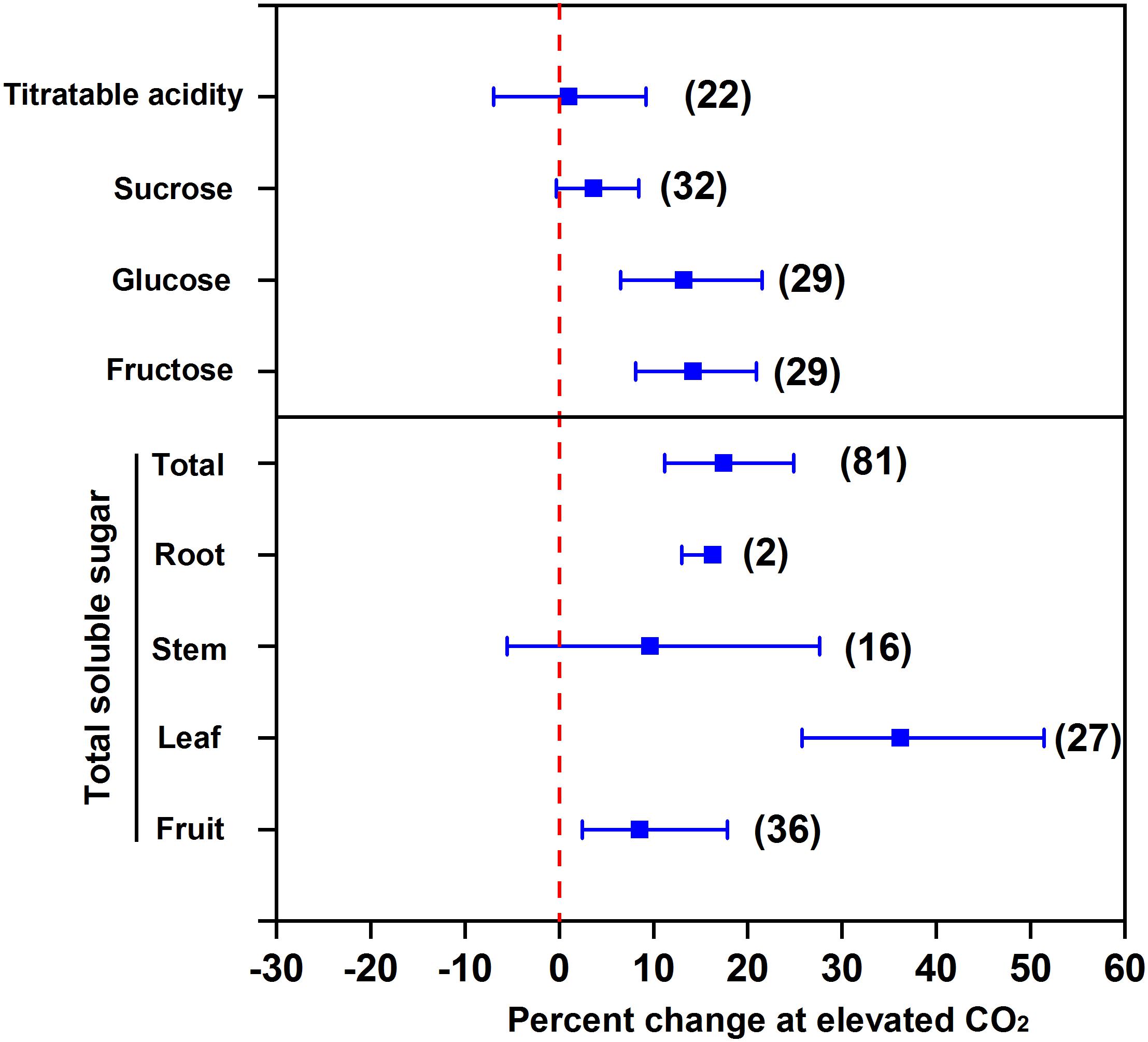
FIGURE 1. Effect of elevated CO2 on the concentrations of soluble sugar and acidity in vegetables. Data are means of percent change with 95% confidence intervals (indicated with error bars) under elevated CO2 compared to ambient CO2. The number of observations is in parentheses.
However, eCO2 does not affect the total soluble sugar in stem vegetables (Figure 1). It is possible that eCO2 promotes the transformation of soluble sugar to lignin, which counteracts the carbon transformation toward soluble sugar accumulation (Liu et al., 2018). Elevated CO2 was found to even decrease soluble sugar concentration in stem vegetables, e.g., celery (Jin et al., 2009). Likewise, we found that eCO2 had no effect on titratable acidity (Figure 1), which indicates that eCO2 promotes the transformation of fixed CO2 to soluble sugar to a greater extent than that to organic acids (Wang and Bunce, 2004) and thus allows a greater sugar-to-acid ratio and a stronger taste of vegetables.
Effect of eCO2 on Nitrogenous Compounds
Our meta-analysis showed that eCO2 decreased the protein concentration in vegetables (9.5%), specifically 10.5% for fruit vegetables, 12.6% for stem vegetables, and 20.5% for root vegetables (Figure 2). However, no significant effect was observed for leafy vegetables. Since leafy vegetables generally contain a greater concentration of nitrate, eCO2 may promote N assimilation in leaves (Stitt and Krapp, 1999). For example, eCO2 increased the N concentration in the inner leaves of lettuce cv. “Batavia Rubia Munguía” noninoculated with arbuscular mycorrhizal fungi to a greater extent than the outer leaves (Baslam et al., 2012). Moreover, eCO2 limits the uptake of nitrogen and the synthesis of nitrogenous compounds of vegetables to a lesser extent than that of other crops (mainly grain crops) (9.5% vs. 10–15%) (Taub et al., 2008; Loladze, 2014), probably because N deficiency is more common for grain crop cultivation in soils compared to vegetable cultivation.
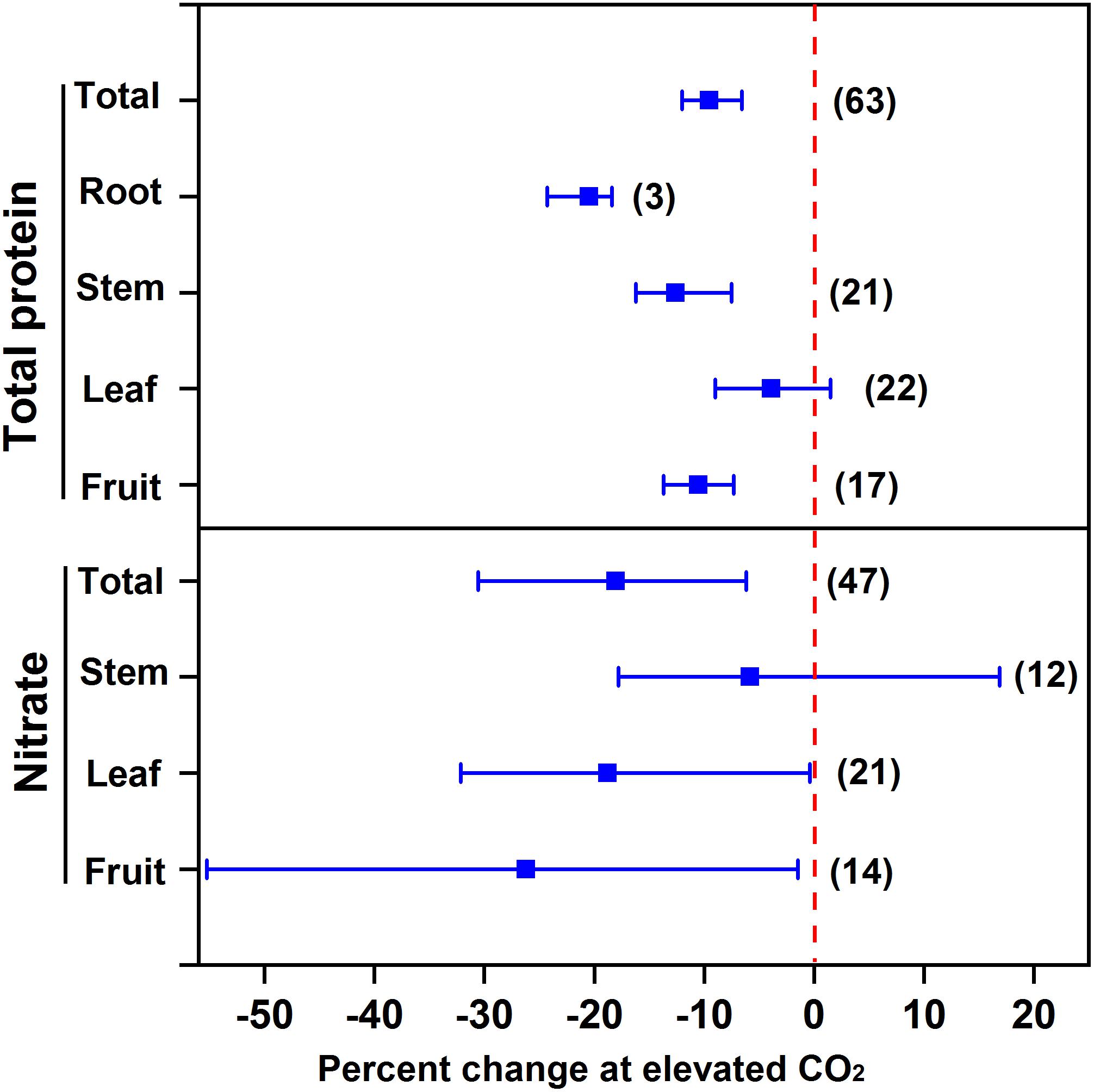
FIGURE 2. Effect of elevated CO2 on the concentrations of total protein and nitrate in vegetables. Data are means of percent change with 95% confidence intervals (indicated with error bars) under elevated CO2 compared to ambient CO2. The number of observations is in parentheses.
On the other hand, eCO2 may alleviate the potential toxicity from nitrate intake for human beings. Overall, eCO2 decreased the nitrate concentration of all vegetables by 18.0% (Figure 2). Specifically, eCO2 decreased the nitrate concentration in fruit and leafy vegetables by 26.2% and 18.8%, respectively. This indicates that eCO2 promotes the nitrate assimilation to a greater extent than nitrate uptake. Bloom et al. (2010) found that eCO2 inhibited nitrate assimilation of wheat and Arabidopsis initially and then reduced nitrate uptake, which further limited N assimilation progressively. The big variation in the effect of eCO2 on nitrate concentration might be due to its distinct impact among species. For example, eCO2 could have greatly decreased the nitrate concentration in cucumber (Tang et al., 2018), but greatly increased that in tomato (Wei et al., 2018). This is probably why the overall eCO2 effect on stem vegetables was not significant. Elevated CO2 was found to sharply increase the nitrate accumulation in celtuce but decrease that in celery (Jin et al., 2009).
Furthermore, it appears that eCO2 affected the components of free amino acid in lettuce (Miyagi et al., 2017), potato (Högy and Fangmeier, 2009), and sweet pepper (Piñero et al., 2017a,b). This suggests that eCO2 has different effects on the metabolic process of amino acids, whose mechanisms are unclear till now.
Effect of eCO2 on Antioxidants
Overall, eCO2 promotes the accumulation of antioxidants in vegetables, thus improving vegetable quality. Our results showed that eCO2 increased total antioxidant capacity, total phenols, total flavonoids, ascorbic acid, and chlorophyll b by 59.0%, 8.9%, 45.5%, 9.5%, and 42.5%, respectively, indicating an improvement of beneficial compounds in vegetables (Figure 3). The greatest increase in total antioxidant capacity (72.5%) as well as the greatest increase in ascorbic acid (15.3%) were both observed in leafy vegetables among different types of vegetables (Figure 3). It is reasonable to predict that the increased soluble sugar as precursors can increase the synthesis and accumulation of antioxidants (Wang et al., 2003; Jaafar et al., 2012; Becker and Kläring, 2016). For example, compared to 200 μmol mol-1 CO2 control, 1,000 μmol mol-1 CO2 is supposed to promote sugar accumulation and subsequently phenol synthesis in lettuce leaf (Becker and Kläring, 2016). On the other hand, eCO2 is thought to promote NADPH synthesis, such that eCO2 can enhance the plants’ capability of diverting NADPH to maintain a higher concentration of antioxidants, e.g., glutathione and ascorbate, to counteract damage from ozone (O3) (Rao et al., 1995) or other stresses (Xu et al., 2015).
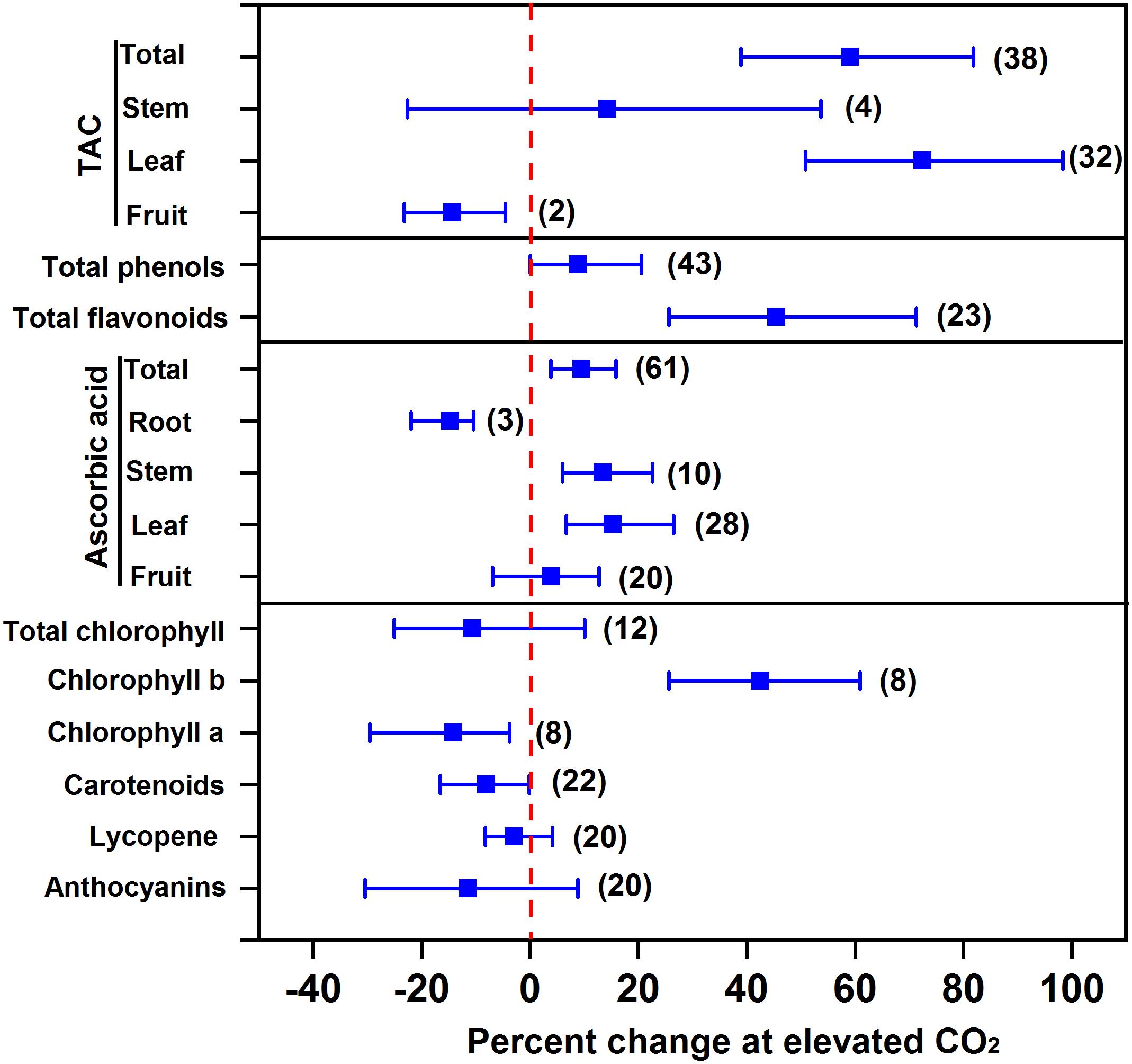
FIGURE 3. Effect of elevated CO2 on the concentrations of antioxidants in vegetables. Data are means of percent change with 95% confidence intervals (indicated with error bars) under elevated CO2 compared to ambient CO2. The number of observations is in parentheses. TAC, total antioxidant capacity.
In contrast, eCO2 had no significant effect on the accumulation of pigments like total chlorophyll, carotenoids, lycopene, and anthocyanins, and even decreased the concentration of total antioxidant capacity in fruit vegetables, ascorbic acid in root vegetables, chlorophyll a, and carotenoids by 14.4%, 14.8%, 14.1%, and 8.1%, respectively. Elevated CO2 can decrease the photorespiration of plants when grown in high light intensity. This may consequently decrease the formation of oxygen radicals, thus reducing antioxidant metabolism (Pérez-López et al., 2018). A recent study on the transcript profile of genes in carrots found that eCO2 could affect antioxidant accumulation (i.e., ascorbic acid) through a complex process, involving the synthesis, recycling, and degradation of ascorbic acid (Wu et al., 2017).
Effect of eCO2 on Minerals
Our results showed that eCO2 decreased the concentrations of Mg, Fe, and Zn by 9.2%, 16.0%, and 9.4%, respectively, whilst it maintained the concentrations of P, K, S, Cu, and Mn of vegetables as a whole (Figure 4). The decrease in Fe concentration was the greatest in leafy vegetables (31.0%), followed by fruit vegetables (19.2%) and root vegetables (8.2%), whereas the decrease in Zn concentration was 18.1% in both fruit and root vegetables and 10.7% in stem vegetables. The decrease in Fe was greater than that in wheat (5.1%) and rice (5.2%) (Myers et al., 2014) or in C3 plants (10%) (Loladze, 2014). As Fe and Zn are important for human nutrition, particularly for children (Myers et al., 2014), their deficiency in vegetables under future CO2 climates should not be neglected.
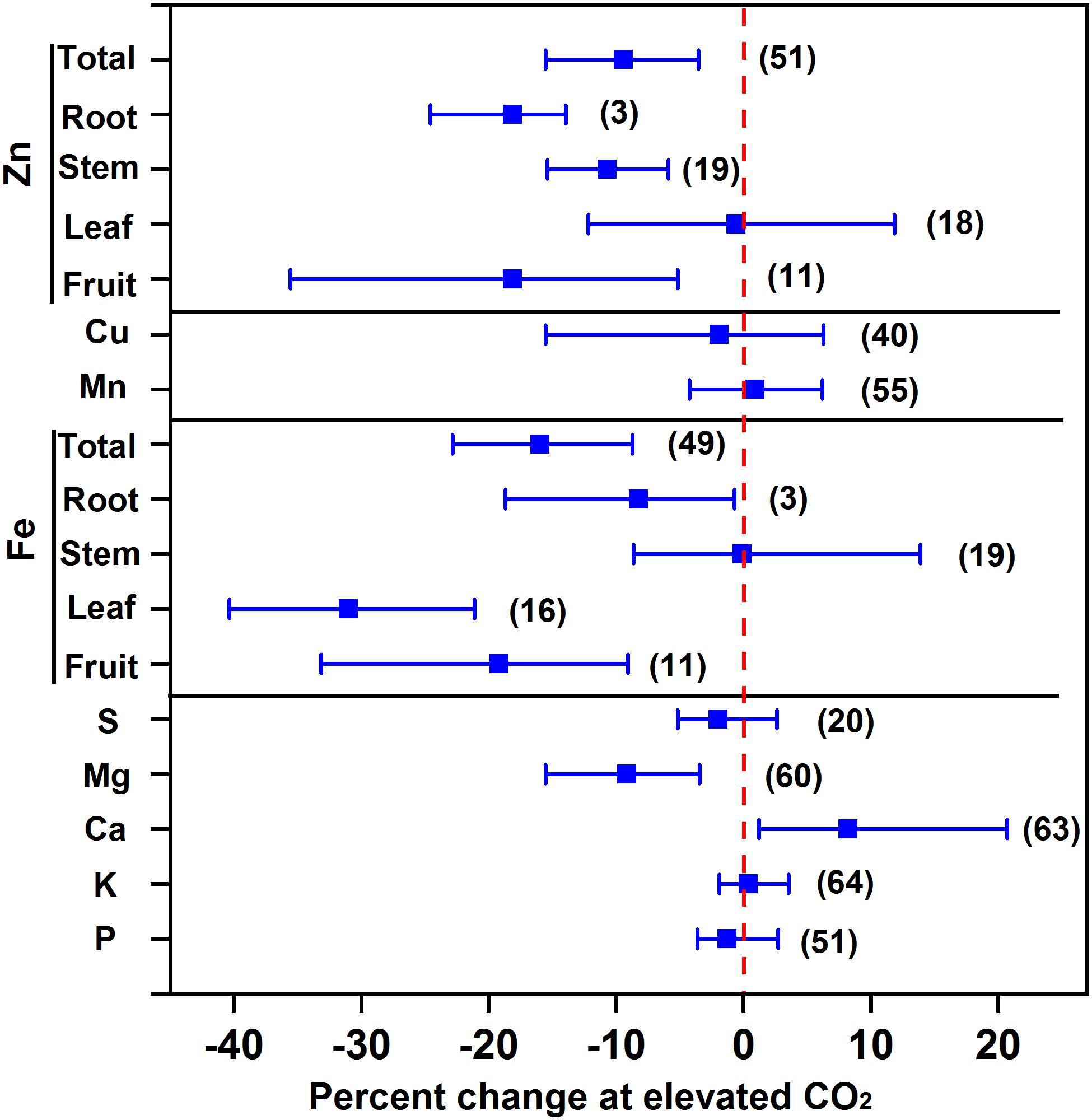
FIGURE 4. Effect of elevated CO2 on the concentrations of minerals in vegetables. Data are means of percent change with 95% confidence intervals (indicated with error bars) under elevated CO2 compared to ambient CO2. The number of observations is in parentheses.
Several studies reported that eCO2 decreased the mineral concentration by a dilution effect (Fangmeier et al., 2002; Högy and Fangmeier, 2009; Loladze, 2014) or restricted transpiration (McDonald et al., 2002). For example, recent reviews demonstrated that eCO2 decreased all the mineral concentrations in grain crops (Loladze, 2014; Myers et al., 2014), suggesting that the decrease in mineral concentration is not specifically regulated by certain metabolic processes but by a dilution effect due to the increased biomass. However, Figure 4 shows that the Ca concentration of vegetables was increased by 8.2% under eCO2, indicating that neither the dilution effect nor the limitation of transpiration can explain the greater accumulation of Ca in vegetables. The inhibition of fruit enlargement resulting from other environmental factors (e.g., N deficiency) may increase Ca storage in fruits (Dong et al., 2018; Tang et al., 2018).
Interactions of eCO2 With Other Factors
As mentioned above, the effects of eCO2 on vegetable quality vary among various types of vegetables and environments. However, limited data are available for a robust meta-analysis on the interaction of eCO2 with several factors. Therefore, we qualitatively summarize the limited studies here involving the following factors: plant cultivar, CO2 level, growth stage, light, O3 stress, nutrient, and salinity.
Plant Cultivar
Elevated CO2 generally increased the total flavonoids in some onion cultivars with a greater ability of flavonoid accumulation like cv. “Choesty” but had no effect on other cultivars with less constitutive flavonoid accumulation like cv. “Fineleaf” (Thompson et al., 2004). Elevated CO2 potentially promoted the accumulation of antioxidative compounds to a greater extent in cultivars richer in the constitutive accumulation of antioxidants (Pérez-López et al., 2015a). By contrast, eCO2 enhanced the glycoalkaloid concentration in potato cv. “Russet Burbank” to a greater extent than cv. “Norland,” but their abilities of producing glycoalkaloid were similar (Nitithamyong et al., 1999). Elevated CO2 led to a greater nitrate accumulation in the leaves of pigmented lettuce cv. “Blonde of Paris Batavia” in nonsaline conditions and decreased that in the leaves of cv. “Oak Leaf,” which possesses a similar ability of nitrate accumulation (Pérez-López et al., 2015a). CO2 effects on mineral concentrations can differ between lettuce cultivars (cv. “Batavia Rubia Munguía” vs. cv. “Maravilla de Verano”) (Baslam et al., 2012). In general, the constitutive differences of compound accumulation in tissues might contribute to the intraspecific variation in response to eCO2.
CO2 Level
Higher CO2 results in greater photosynthetic rates initially but often photosynthetic acclimation, i.e., down regulation of net photosynthetic rates in the long term (Long et al., 2004; Kirschbaum, 2011), which indicates that increases in CO2 concentration can substantially affect vegetable quality. Elevated CO2 (by 600 μmol mol-1 above ambient CO2) enhanced the concentrations of fructose, glucose, and sucrose, but reduced the concentrations of malic acid, citric acid, and quinic acid in strawberry fruits to a greater extent than an elevation of CO2 of 300 μmol mol-1 (Wang and Bunce, 2004), indicating greater carbohydrate accumulation and less organic acid transformation from carbohydrates in strawberry under higher CO2 concentration. On the other hand, compared to ambient CO2, a lower CO2 elevation (550 μmol mol-1) increased the concentrations of fructose and glucose in potato at maturity to a greater extent than a higher CO2 elevation (680 μmol mol-1) (Högy and Fangmeier, 2009), indicating an inhibition of carbohydrate synthesis under long-term higher CO2 exposure. Interestingly, the reverse was true for total glycoalkaloid concentration in potato fruits (Högy and Fangmeier, 2009), implying that the effect of CO2 on carbon metabolism varies with the direction of shifts. Similarly, 550 μmol mol-1 CO2 decreased the concentrations of phenols and flavonoids, and the total antioxidant capacity of tomato fruits to a greater extent than 700 μmol mol-1, but it increased the concentration of ascorbic acid to a greater degree than that under 700 μmol mol-1 (Mamatha et al., 2014). Differences in product quality among different CO2 levels were also reported in other studies (Levine and Paré, 2009; Ren et al., 2014; Fu et al., 2015).
Growth Stage
Elevated CO2 had increased the sucrose concentration of tomato fruits to a greater extent at the early fruiting stage than that at the later fruiting stage (Islam et al., 1996). The same pattern was noticed in terms of the concentrations of soluble sugars and organic acids in grapes (Bindi et al., 2001). The minimal impact on sugar accumulation at the later growth stage may be attributed to the long-term CO2 exposure when less carbon can be fixed and translocated to fruits. Tomato fruits are usually harvested when their color meets certain standards (Zhang et al., 2014). However, eCO2 has been found to increase the synthesis of color-related pigments in tomatoes to a lesser extent than the synthesis of soluble sugar and total solids (Islam et al., 1996; Zhang et al., 2014), resulting in a mismatch of the fruit color with its maturity. This means that producers may have delayed harvesting the fruits under eCO2 based on the standards set for ambient CO2. Elevated CO2 thus increases soluble sugar accumulation as the sugar concentration is continuously increasing from the green to the red stage of tomato (Winsor et al., 1962). This might also explain the decrease in the concentrations of organic acids (Islam et al., 1996) and ascorbic acid (Khan et al., 2013) in tomato fruits at the red stage under eCO2, because the concentrations of organic acids and ascorbic acid were generally increased when tomato reaches its maturity but decreased after ripening (Islam et al., 1996). Elevated CO2 might promote soluble sugar and fiber accumulation in tomato cv. “Eureka” by accelerating its maturity as well (Khan et al., 2013).
Elevated CO2 generally increased N concentration in the leaves of spinach and fenugreek at 40 days after CO2 exposure, but N concentration declined at 80 days after CO2 exposure (Jain et al., 2007), indicating that eCO2 probably promotes N assimilation of these leafy vegetables at the early stage and this is consistent with a study on barley seedlings (Robredo et al., 2011), but N assimilation is limited due to photosynthetic acclimation at the later stage (Taub et al., 2008).
Light
Plants grown in moderate vs. low light can generate more ATP and NADPH for carbon fixation, whereas high vs. moderate light may cause photoinhibition resulting from excessive light intensity that produces greater amounts of reactive oxygen species (Pérez-López et al., 2018). Elevated CO2 can improve vegetable quality under certain light intensities. For example, both eCO2 (800 μmol mol-1) and high light intensity (800 μmol m-2 s-1) decreased nitrate accumulation in spinach leaves independently and interactively, resulting in the lowest nitrate concentration in the combination of eCO2 with high light intensity (Proietti et al., 2013). A combination of eCO2 (700 vs. 400 μmol mol-1) and high light intensity (700 vs. 400 μmol m-2 s-1) increased the total antioxidant capacity of both cultivars of lettuce relative to ambient CO2 and high light intensity (Pérez-López et al., 2015b). However, this combination could decrease the total antioxidant capacity in both cultivars observed in another study with the same growth conditions (Pérez-López et al., 2018), indicating that the total antioxidant capacity can be very sensitive to environmental factors. Similarly, eCO2 decreased the concentrations of ascorbic acid and capsaicin to a greater extent under high light intensity (463 vs. 233 μmol m-2 s-1) (Li et al., 2017). In terms of light quality, increases in CO2 concentration (from 450 to 1200 μmol mol-1) generally improved the concentration of phenols, anthocyanin, and flavonoids in RB20 (ratio of red LED light to blue light, 80%/20%) to a greater extent than that in RB40 (red/blue, 60%/40%), with the same light intensity at 250 μmol m-2 s-1 (Ren et al., 2014). Compared to ambient CO2 without ultraviolet-B radiation, the combination of eCO2 (700 vs. 350 μmol mol-1) with ultraviolet-B radiation enhanced the concentrations of soluble sugar, ascorbic acid, and lycopene in tomato fruits (to be the best for ultraviolet-B radiation at 1.2 kJ m-2) (Li et al., 2007).
O3 Stress
Increases in ground-level O3 also contribute to global warming and other aspects of environmental change (IPCC, 2014). O3 is easily absorbed through plant stomata, and it induces the formation of reactive oxygen species and free radicals damaging the components of plant cells and inhibits plant growth (Kumari et al., 2013). Elevated CO2 may alleviate the adverse effect of O3 through decreasing stomatal conductance, and thus O3 uptake (Kumari et al., 2013). Therefore, under O3-stressed conditions, eCO2 promotes carbon fixation and improves the downstream metabolic process related to vegetable quality, e.g., promoting carbohydrate synthesis, including soluble sugar in the leaves of palak (Kumari et al., 2013) and tubers of potato (Kumari and Agrawal, 2014). In addition, eCO2 can reduce the chlorophyll destruction in leaves under O3 stress and enhance the activity of antioxidant systems in the leaves of palak, including activities of ascorbate peroxidase (Kumari et al., 2013). By contrast, the concentrations of K, Ca, Na, Fe, and Zn in tubers of potato decreased under eCO2 and high O3 relative to ambient CO2 and ambient O3 (Kumari and Agrawal, 2014), thereby decreasing tuber quality. The interaction between eCO2 and O3 has not been detected in quality-related parameters, e.g., soluble sugar, organic acid, and ascorbic acid, in another study on potato (Donnelly et al., 2001).
Nutrient
Nutrient availability also influences the effect of eCO2 on product quality. Low nutrient availability limits the eCO2 effect on plant photosynthetic rates (Arp, 1991; Gruda and Tanny, 2015), probably resulting in less carbon available for synthesizing secondary compounds. For example, eCO2 reduced the concentrations of soluble solids, soluble sugar, and lycopene in tomato fruits in normal N availability where less carbon was fixed, while it promoted their concentration in higher N availability (Helyes et al., 2012). Similarly, eCO2 decreased the total antioxidant capacity and antioxidant compounds of strawberry in low N availability to a greater extent than that in high N (Sun et al., 2012). However, eCO2 increased the concentrations of aliphatic glucosinolates of Chinese kale under N supply at 5 and 10 mmol L-1 N, but decreased their concentrations at 20 mmol L-1 N with unknown mechanisms (La et al., 2009). On the other hand, low nutrient availability exacerbates the uptake and assimilation of the nutrient itself under eCO2, as evidenced by a greater decrease in grain protein concentration under low N availability (Taub et al., 2008). The trend is also true for vegetables. For example, eCO2 decreased the total N concentration in the bolting stem of Chinese kale in high N supply to a lesser extent than that in low N (La et al., 2009).
Interestingly, N form also interacts with eCO2 and hence affects the product quality of Chinese cabbage (Reich et al., 2016) and sweet pepper (Piñero et al., 2017b). Specifically, eCO2 (800 vs. 420 μmol mol-1) increased P concentration and maintained S concentration in shoots of 20-day-old Chinese cabbage in 1.88 mM NH4NO3 at a temperature of 15/12°C (day/night), while it maintained P concentration and decreased S concentration in plants supplied with 3.75 mM NO3- (Reich et al., 2016). Elevated CO2 generally increased the concentrations of chlorophyll a, chlorophyll b, protein, P, Ca, Mg, Mn, Cu, and cadaverine in fruits of sweet pepper when supplied with 10 mM NO3- and 2 mM NH4+, but decreased these parameters under 12 mM NO3- (Piñero et al., 2017b). Although NH4+ can be toxic when supplied at high levels for vegetable growth (Schjoerring et al., 2002; Roosta and Schjoerring, 2008), eCO2 appears to improve the nutritional quality of vegetables when supplied N source partly using NH4+.
Salinity Stress
Saline water (such as 5 dS m-1) is widely used in greenhouse vegetable cultivation in some countries like Israel and there are interactive effects between eCO2 and salinity on vegetable quality (Mizrahi and Pasternak, 1985; Li et al., 1999). Elevated CO2 increased the productivity and yield of tomato under saline conditions (7 dS m-1); however, the quality parameters—total soluble sugar, total soluble solids, and acidity—remained stable (Li et al., 1999). In contrast, eCO2 increased nitrate accumulation in the leaves of pigmented lettuce cv. “Blonde of Paris Batavia” in saline conditions (200 mmol L-1 NaCl) to a lesser extent than that in nonsaline conditions (Pérez-López et al., 2015a), thus benefiting lettuce quality.
Elevated CO2 reduced anthocyanin synthesis in the leaves of pigmented lettuce cv. “Oat leaf” to a greater extent in saline conditions than nonsalinity (Pérez-López et al., 2015a). However, eCO2 tended to increase the concentration of reduced ascorbate in cv. “Oat leaf” under saline conditions (Pérez-López et al., 2015a). Therefore, eCO2 might enhance the synthesis of some components of antioxidants rather than decreasing the concentrations of all antioxidants (Figure 3). The increase in total antioxidant activity under eCO2 was also observed under saline conditions for both lettuce cultivars in another study (Pérez-López et al., 2013).
Apart from the above factors, the interactive effect of eCO2 with temperature (Sun et al., 2012) and water availability (Wei et al., 2018) has also been investigated.
Recommendations for Optimizing eCO2 Benefits
Several approaches can be considered to combine eCO2 with other factors to enhance the nutritional quality of vegetables: (1) selecting vegetable species or cultivars that possess greater ability in carbon fixation and synthesis of required quality-related compounds; (2) optimizing other environmental factors (e.g., moderate CO2 concentrations, moderate light intensity, increased N availability, or increased fertilization of Fe or Zn) to promote carbon fixation and nutrient uptake interactively when growing plants under eCO2; (3) harvesting vegetable products earlier in cases of over maturity and reduced benefit of eCO2 to vegetative growth; and (4) combining eCO2 with mild environmental stress (e.g., ultraviolet-B radiation or salinity) in instances when this enhances vegetable quality and might counteract the dilution effect or direct metabolic pathways toward the synthesis of health-beneficial compounds. However, one needs to be cautious that it is less likely to improve all the parameters of nutritional quality simultaneously. An improvement of quality might result in yield penalty.
Effects of Elevated CO2 on Quality of Some Specific Crops
Lettuce
Lettuce is one of the most preferred vegetables worldwide for its taste, flavor, and richness in healthy compounds. Therefore, the effects of eCO2 and its interactions with other factors on vegetable quality have received more attention in lettuce than other vegetables (Pérez-López et al., 2013, 2015a,b, 2018; Becker and Kläring, 2016; Sgherri et al., 2017). Elevated CO2 potentially enhances the taste of lettuce indicated by increasing soluble sugar accumulation by 27.1% (n = 18, meta-analysis), as demonstrated in several studies (Jin et al., 2009; Baslam et al., 2012; Pérez-López et al., 2015a; Becker and Kläring, 2016). More specifically, eCO2 increased the soluble sugar concentration in the outer layer of the leaves of cv. “Maravilla de Verano” to a greater extent than that of the inner layer (Baslam et al., 2012), whereas there was no similar promotion by eCO2 if plants were grown in a high light intensity of 700 μmol m-2 s-1 (Pérez-López et al., 2015b). The protein concentration was decreased by 5.6% under eCO2 (n = 12, meta-analysis at p = 0.07), indicating a decreased nutritional value. By contrast, the meta-analytic results showed that eCO2 increased ascorbic acid and total antioxidant capacity by 7.1% (n = 18) and 82.0% (n = 23), respectively, indicating an improvement of lettuce quality. However, the responses of healthy compounds in lettuce to eCO2, including phenolic acid, flavonoid, ascorbic acid, and pigments, are not consistent among growth conditions or studies (Supplementary Table S4). For example, eCO2 greatly promoted ascorbic acid concentration (Jin et al., 2009), but not always (Baslam et al., 2012; Pérez-López et al., 2015a,b). In general, (1) lettuce plants received more benefits from eCO2 on its antioxidant metabolism under salinity stress or high light intensity (Pérez-López et al., 2013, 2015a) and (2) eCO2 promoted the accumulation of antioxidative compounds in cv. “Oak Leaf” to a greater extent than that of cv. “Blonde of Paris Batavia” (Pérez-López et al., 2015a,b).
Tomato
The effect of eCO2 on tomato fruit quality has also received much attention in research. Our analysis indicates that eCO2 enhances tomato quality, and likely taste, by increasing the concentrations of fructose, glucose, and total soluble sugars (14.7% using meta-analysis, n = 24) in tomato fruits (Islam et al., 1994; Behboudian and Tod, 1995; Li et al., 2007; Zhang et al., 2014). This effect was reduced for fruits of the first several harvests (Islam et al., 1994; Zhang et al., 2014) and under moderate N supply (Helyes et al., 2012) when fewer carbohydrates were accumulated. Compared with soluble sugar, total soluble solids and organic acids were increased to a lesser extent (Behboudian and Tod, 1995; Zhang et al., 2014), probably due to the less transformation from increased sugar. Generally, eCO2 increased the concentration of ascorbic acid by 18.5% (n = 12, meta-analysis). In contrast, the effect of eCO2 on lycopene concentration is variable (Supplementary Table S4), perhaps due to the sensitivity of lycopene synthesis to temperature (Krumbein et al., 2006), and thus our meta-analysis (n = 18) found no significant effect of eCO2 on lycopene concentration. Together, these results indicate that more research on the interactive effects of eCO2 and other growth conditions on tomato fruit quality is needed.
Potato
The program Changing Climate and Potential Impacts on Potato Yield and Quality (CHIP) funded by the European Commission pursues a comprehensive exploration of the effects of eCO2 on potato quality (De Temmerman et al., 2002). Their results showed that the eCO2 effect on the nutritional and processing quality of potato can be variable (Högy and Fangmeier, 2009). Elevated CO2 increased the concentrations of soluble sugars and starch, and maintained the concentrations of organic acids when the growth condition is suitable for a greater yield in general, resulting in a higher risk of browning and increased acrylamide production when fried (Donnelly et al., 2001; Kumari and Agrawal, 2014). Otherwise, the reducing sugar concentration can be decreased (Kumari and Agrawal, 2014). The decreased citrate concentration led to a higher risk of discoloration but resulted in better taste (Högy and Fangmeier, 2009). The protein and Zn concentration in tubers was lower (13.1%, n = 18 and 10.7%, n = 19, respectively, from meta-analysis) under eCO2 and thus reduced the nutritive value of tubers as shown in several studies (Donnelly et al., 2001; Fangmeier et al., 2002; Heagle et al., 2003; Högy and Fangmeier, 2009) except when eCO2 recovered plant growth from intense stresses (Kumari and Agrawal, 2014). The total glycoalkaloid and α-chaconine concentration under eCO2 was decreased (Vorne et al., 2002; Högy and Fangmeier, 2009), remained stable (Donnelly et al., 2001), or increased (Nitithamyong et al., 1999). The judgment of the glycoalkaloids also differs as their decreases can be regarded as positive in terms of its toxicity but as negative in terms of a worse taste (Högy and Fangmeier, 2009). In conclusion, potato quality under eCO2 generally should be assessed in terms of the corresponding parameters and the needs of customers.
Summary
Several studies have been conducted in recent decades on the effects of eCO2 on vegetable quality, including parameters related to taste, flavor, nutritive value, and industrial processing. These studies show that eCO2 can promote the accumulation of soluble sugar including glucose and fructose, and the accumulation of antioxidants including ascorbic acid, total phenols, and total flavonoids, but reduce the levels of protein, nitrate, Mg, Fe, and Zn in products. In practice, it is advisable to enhance vegetable quality by (1) selecting species or cultivars that respond well to eCO2; (2) providing optimal environments together with eCO2; (3) harvesting vegetables earlier than standards set at ambient CO2; and (4) combining with moderate environmental stresses. The promotion by the increased carbon fixation and thus the precursor, dilution effect, stress induction, and limitation by transpiration or N assimilation can generally explain the shifts of vegetable quality under eCO2. However, research is still required to reveal the underlying physiological and molecular mechanisms more specifically.
Author Contributions
JD, NG, and XL conceived and designed the review structure. JD collected references, analyzed the data, and wrote the paper. NG, XL, SL, and ZD revised the paper.
Funding
The authors thank the National Science and Technology Support Program of China (2014BAD14B04), the Strategic Priority Research Program of the Chinese Academy of Sciences (XDB15030300), and the Frontier Project of Knowledge Innovation Program of Institute of Soil Science, Chinese Academy of Sciences (ISSASIP1635) for funding support.
Conflict of Interest Statement
The authors declare that the research was conducted in the absence of any commercial or financial relationships that could be construed as a potential conflict of interest.
Acknowledgments
We thank Dr. Wenying Chu, Aihua Wang, and Ying Tang (Institute of Soil Science, CAS) for helpful discussion.
Supplementary Material
The Supplementary Material for this article can be found online at: https://www.frontiersin.org/articles/10.3389/fpls.2018.00924/full#supplementary-material
Footnotes
References
Arp, W. (1991). Effects of source-sink relations on photosynthetic acclimation to elevated CO2. Plant Cell Environ. 14, 869–875. doi: 10.1111/j.1365-3040.1991.tb01450.x
Azam, A., Khan, I., Mahmood, A., and Hameed, A. (2013). Yield, chemical composition and nutritional quality responses of carrot, radish and turnip to elevated atmospheric carbon dioxide. J. Sci. Food Agric. 93, 3237–3244. doi: 10.1002/jsfa.6165
Baslam, M., Garmendia, I., and Goicoechea, N. (2012). Elevated CO2 may impair the beneficial effect of arbuscular mycorrhizal fungi on the mineral and phytochemical quality of lettuce. Ann. Appl. Biol. 161, 180–191. doi: 10.1111/j.1744-7348.2012.00563.x
Becker, C., and Kläring, H.-P. (2016). CO2 enrichment can produce high red leaf lettuce yield while increasing most flavonoid glycoside and some caffeic acid derivative concentrations. Food Chem. 199, 736–745. doi: 10.1016/j.foodchem.2015.12.059
Behboudian, M. H., and Tod, C. (1995). Postharvest attributes of ‘Virosa’ tomato fruit produced in an enriched carbon dioxide environment. Hortic. Sci. 30, 490–491.
Bindi, M., Fibbi, L., and Miglietta, F. (2001). Free air CO2 enrichment (FACE) of grapevine (Vitis vinifera L.): II. Growth and quality of grape and wine in response to elevated CO2 concentrations. Eur. J. Agron. 14, 145–155. doi: 10.1016/S1161-0301(00)00093-9
Bisbis, M. B., Gruda, N., and Blanke, M. (2018). Potential impacts of climate change on vegetable production and product quality–a review. J. Clean. Prod. 170, 1602–1620. doi: 10.1016/j.jclepro.2017.09.224
Bloom, A. J., Burger, M., Asensio, J. S. R., and Cousins, A. B. (2010). Carbon dioxide enrichment inhibits nitrate assimilation in wheat and Arabidopsis. Science 328, 899–903. doi: 10.1126/science.1186440
De Temmerman, L., Hacour, A., and Guns, M. (2002). Changing climate and potential impacts on potato yield and quality ‘CHIP’: introduction, aims and methodology. Eur. J. Agron. 17, 233–242. doi: 10.1016/S1161-0301(02)00063-1
Demmers-Derks, H., Mitchell, R. A. C., Mitchell, V. J., and Lawlor, D. W. (1998). Response of sugar beet (Beta vulgaris L.) yield and biochemical composition to elevated CO2 and temperature at two nitrogen applications. Plant Cell Environ. 21, 829–836. doi: 10.1046/j.1365-3040.1998.00327.x
Dong, J., Xu, Q., Gruda, N., Chu, W., Li, X., and Duan, Z. (2018). Elevated and super-elevated CO2 differ in their interactive effects with nitrogen availability on fruit yield and quality of cucumber. J. Sci. Food Agric. doi: 10.1002/jsfa.8976 [Epub ahead of print].
Donnelly, A., Lawson, T., Craigon, J., Black, C. R., Colls, J. J., and Landon, G. (2001). Effects of elevated CO2 and O3 on tuber quality in potato (Solanum tuberosum L.). Agric. Ecosyst. Environ. 87, 273–285. doi: 10.1016/S0167-8809(01)00144-X
Drake, J. E., Gallet-Budynek, A., Hofmockel, K. S., Bernhardt, E. S., Billings, S. A., Jackson, R. B., et al. (2011). Increases in the flux of carbon belowground stimulate nitrogen uptake and sustain the long-term enhancement of forest productivity under elevated CO2. Ecol. Lett. 14, 349–357. doi: 10.1111/j.1461-0248.2011.01593.x
Egger, M., Smith, G. D., Schneider, M., and Minder, C. (1997). Bias in meta-analysis detected by a simple, graphical test. BMJ 315, 629–634. doi: 10.1136/bmj.315.7109.629
Fangmeier, A., De Temmerman, L., Black, C., Persson, K., and Vorne, V. (2002). Effects of elevated CO2 and/or ozone on nutrient concentrations and nutrient uptake of potatoes. Eur. J. Agron. 17, 353–368. doi: 10.1016/S1161-0301(02)00071-0
Fierro, A., Gosselin, A., and Tremblay, N. (1994). Supplemental carbon dioxide and light improved tomato and pepper seedling growth and yield. Hortscience 29, 152–154.
Fu, Y., Shao, L., Liu, H., Li, H., Zhao, Z., Ye, P., et al. (2015). Unexpected decrease in yield and antioxidants in vegetable at very high CO2 levels. Environ. Chem. Lett. 13, 473–479. doi: 10.1007/s10311-015-0522-6
Gruda, N. (2005). Impact of environmental factors on product quality of greenhouse vegetables for fresh consumption. Crit. Rev. Plant Sci. 24, 227–247. doi: 10.1080/07352680591008628
Gruda, N., and Tanny, J. (2014). “Protected Crops,” in Horticulture: Plants for People and Places: Production Horticulture, Vol. 1, eds G. R. Dixon and D. E. Aldous (Dordrecht: Springer), 327–405. doi: 10.1007/978-94-017-8578-5_10
Gruda, N., and Tanny, J. (2015). Protected Crops-Recent Advances, Innovative Technologies and Future Challenges. Leuven: International Society for Horticultural Science (ISHS), 271–278. doi: 10.17660/ActaHortic.2015.1107.37
Heagle, A. S., Miller, J. E., and Pursley, W. A. (2003). Growth and yield responses of potato to mixtures of carbon dioxide and ozone. J. Environ. Qual. 32, 1603–1610. doi: 10.2134/jeq2003.1603
Hedges, L. V., Gurevitch, J., and Curtis Peter, S. (1999). The meta-analysis of response ratios in experimental ecology. Ecology 80, 1150–1156. doi: 10.1890/0012-9658(1999)080[1150:TMAORR]2.0.CO;2
Helyes, L., Lugasi, A., Neményi, A., and Pék, Z. (2012). The simultaneous effect of elevated CO2-level and nitrogen-supply on the fruit components of tomato. Acta Aliment 41, 265–271. doi: 10.1556/AAlim.41.2012.2.13
Högy, P., and Fangmeier, A. (2009). Atmospheric CO2 enrichment affects potatoes: 2. Tuber quality traits. Eur. J. Agron. 30, 85–94. doi: 10.1016/j.eja.2008.07.006
Huang, B., and Xu, Y. (2015). Cellular and molecular mechanisms for elevated CO2-regulation of plant growth and stress adaptation. Crop Sci. 55, 1–20. doi: 10.2135/cropsci2014.07.0508
Idso, S. B., and Idso, K. E. (2001). Effects of atmospheric CO2 enrichment on plant constituents related to animal and human health. Environ. Exp. Bot. 45, 179–199. doi: 10.1016/S0098-8472(00)00091-5
IPCC (2014). Climate Change 2014: Synthesis Report. Contribution of Working Groups I, II and III to the Fifth Assessment Report of the Intergovernmental Panel on Climate Change, eds Core Writing Team, R. K. Pachauri, and L. A. Meyer. Geneva: IPCC, 40–54.
Islam, M., Matsui, T., and Yoshida, Y. (1994). Effects of carbon dioxide enrichment on acid invertase and sugar concentration in developing tomato fruit. Environ. Control Biol. 32, 245–251. doi: 10.2525/ecb1963.32.245
Islam, S. M., Matsui, T., and Yoshida, Y. (1996). Effect of carbon dioxide enrichment on physico-chemical and enzymatic changes in tomato fruits at various stages of maturity. Sci. Hortic. 65, 137–149. doi: 10.1016/0304-4238(95)00867-5
Jaafar, H. Z. E., Ibrahim, M. H., and Karimi, E. (2012). Phenolics and flavonoids compounds, phenylanine ammonia lyase and antioxidant activity responses to elevated CO2 in Labisia pumila (Myrisinaceae). Molecules 17, 6331–6347. doi: 10.3390/molecules17066331
Jablonski, L. M., Wang, X., and Curtis, P. S. (2002). Plant reproduction under elevated CO2 conditions: a meta-analysis of reports on 79 crop and wild species. New Phytol. 156, 9–26. doi: 10.1046/j.1469-8137.2002.00494.x
Jain, V., Pal, M., Raj, A., and Khetarpal, S. (2007). Photosynthesis and nutrient composition of spinach and fenugreek grown under elevated carbon dioxide concentration. Biol. Plant. 51, 559–562. doi: 10.1007/s10535-007-0122-9
Jin, C., Du, S., Wang, Y., Condon, J., Lin, X., and Zhang, Y. (2009). Carbon dioxide enrichment by composting in greenhouses and its effect on vegetable production. J. Plant Nutr. Soil Sci. 172, 418–424. doi: 10.1002/jpln.200700220
Khan, I., Azam, A., and Mahmood, A. (2013). The impact of enhanced atmospheric carbon dioxide on yield, proximate composition, elemental concentration, fatty acid and vitamin C contents of tomato (Lycopersicon esculentum). Environ. Monit. Assess. 185, 205–214. doi: 10.1007/s10661-012-2544-x
Kimball, B. A. (1983). Carbon dioxide and agricultural yield: an assemblage and analysis of 430 prior observations. Agron. J. 75, 779–788. doi: 10.2134/agronj1983.00021962007500050014x
Kirby, K. N., and Gerlanc, D. (2013). BootES: an R package for bootstrap confidence intervals on effect sizes. Behav. Res. 45, 905–927. doi: 10.3758/s13428-013-0330-5
Kirschbaum, M. U. (2011). Does enhanced photosynthesis enhance growth? Lessons learned from CO2 enrichment studies. Plant Physiol. 155, 117–124. doi: 10.1104/pp.110.166819
Krumbein, A., Schwarz, D., and Kläring, H. P. (2006). Effects of environmental factors on carotenoid content in tomato (Lycopersicon esculentum L. Mill.) grown in a greenhouse. J. Appl. Bot. Food Qual. 80, 160–164.
Kumari, S., and Agrawal, M. (2014). Growth, yield and quality attributes of a tropical potato variety (Solanum tuberosum L. cv Kufri chandramukhi) under ambient and elevated carbon dioxide and ozone and their interactions. Ecotoxicol. Environ. Saf. 101, 146–156. doi: 10.1016/j.ecoenv.2013.12.021
Kumari, S., Agrawal, M., and Tiwari, S. (2013). Impact of elevated CO2 and elevated O3 on Beta vulgaris L.: pigments, metabolites, antioxidants, growth and yield. Environ. Pollut. 174, 279–288. doi: 10.1016/j.envpol.2012.11.021
La, G., Fang, P., Teng, Y., Li, Y., and Lin, X. (2009). Effect of CO2 enrichment on the glucosinolate contents under different nitrogen levels in bolting stem of Chinese kale (Brassica alboglabra L.). J. Zhejiang Univ. Sci. B 10, 454–464. doi: 10.1631/jzus.B0820354
Lam, S. K., Chen, D., Norton, R., Armstrong, R., and Mosier, A. R. (2012). Nitrogen dynamics in grain crop and legume pasture systems under elevated atmospheric carbon dioxide concentration: a meta-analysis. Glob. Change Biol. 18, 2853–2859. doi: 10.1111/j.1365-2486.2012.02758.x
Levine, L. H., and Paré, P. W. (2009). Antioxidant capacity reduced in scallions grown under elevated CO2 independent of assayed light intensity. Adv. Space Res. 44, 887–894. doi: 10.1016/j.asr.2009.06.017
Li, F., Wang, J., Chen, Y., Zou, Z., Wang, X., and Yue, M. (2007). Combined effects of enhanced ultraviolet-B radiation and doubled CO2 concentration on growth, fruit quality and yield of tomato in winter plastic greenhouse. Front. Biol. China 2:414–418. doi: 10.1007/s11515-007-0063-x
Li, J. H., Sagi, M., Gale, J., Volokita, M., and Novoplansky, A. (1999). Response of tomato plants to saline water as affected by carbon dioxide supplementation. I. Growth, yield and fruit quality. J. Hortic. Sci. Biotechnol. 74, 232–237. doi: 10.1080/14620316.1999.11511100
Li, X., Kang, S., Li, F., Zhang, X., Huo, Z., Ding, R., et al. (2017). Light supplement and carbon dioxide enrichment affect yield and quality of off-season pepper. Agron. J. 109, 2107–2118. doi: 10.2134/agronj2017.01.0044
Liu, J., Feng, K., Wang, G., Xu, Z., Wang, F., and Xiong, A. (2018). Elevated CO2 induces alteration in lignin accumulation in celery (Apium graveolens L.). Plant Physiol. Biochem. 127, 310–319. doi: 10.1016/j.plaphy.2018.04.003
Loladze, I. (2014). Hidden shift of the ionome of plants exposed to elevated CO2 depletes minerals at the base of human nutrition. eLife 3:e02245. doi: 10.7554/eLife.02245
Long, S. P., Ainsworth, E. A., Rogers, A., and Ort, D. R. (2004). Rising atmospheric carbon dioxide: plants FACE the Future. Annu. Rev. Plant Biol. 55, 591–628. doi: 10.1146/annurev.arplant.55.031903.141610
Mamatha, H., Srinivasa Rao, N. K., Laxman, R. H., Shivashankara, K. S., Bhatt, R. M., and Pavithra, K. C. (2014). Impact of elevated CO2 on growth, physiology, yield, and quality of tomato (Lycopersicon esculentum Mill) cv. Arka Ashish. Photosynthetica 52, 519–528. doi: 10.1007/s11099-014-0059-0
McDonald, E. P., Erickson, J. E., and Kruger, E. L. (2002). Can decreased transpiration limit plant nitrogen acquisition in elevated CO2? Funct. Plant Biol. 29, 1115–1120. doi: 10.1071/FP02007
Miyagi, A., Uchimiya, H., and Kawai-Yamada, M. (2017). Synergistic effects of light quality, carbon dioxide and nutrients on metabolite compositions of head lettuce under artificial growth conditions mimicking a plant factory. Food Chem. 218, 561–568. doi: 10.1016/j.foodchem.2016.09.102
Mizrahi, Y., and Pasternak, D. O. V. (1985). Effect of salinity on quality of various agricultural crops. Plant Soil 89, 301–307. doi: 10.1007/BF02182249
Moretti, C. L., Mattos, L. M., Calbo, A. G., and Sargent, S. A. (2010). Climate changes and potential impacts on postharvest quality of fruit and vegetable crops: a review. Food Res. Int. 43, 1824–1832. doi: 10.1016/j.foodres.2009.10.013
Mortensen, L. M. (1987). Review: CO2 enrichment in greenhouses. Crop responses. Sci. Hortic. 33, 1–25. doi: 10.1016/j.scitotenv.2016.07.030
Mortensen, L. M. (1994). Effects of elevated CO2 concentrations on growth and yield of eight vegetable species in a cool climate. Sci. Hortic. 58, 177–185. doi: 10.1016/0304-4238(94)90149-X
Myers, S. S., Zanobetti, A., Kloog, I., Huybers, P., Leakey, A. D. B., Bloom, A. J., et al. (2014). Increasing CO2 threatens human nutrition. Nature 510, 139–142. doi: 10.1038/nature13179
Nitithamyong, A., Vonelbe, J. H., Wheeler, R. M., and Tibbitts, T. W. (1999). Glycoalkaloids in potato tubers grown under controlled environments. Am. J. Potato Res. 76, 337–343. doi: 10.1007/BF02910006
Pérez-López, U., Miranda-Apodaca, J., Lacuesta, M., Mena-Petite, A., and Muñoz-Rueda, A. (2015a). Growth and nutritional quality improvement in two differently pigmented lettuce cultivars grown under elevated CO2 and/or salinity. Sci. Hortic. 195, 56–66. doi: 10.1016/j.scienta.2015.08.034
Pérez-López, U., Miranda-Apodaca, J., Muñoz-Rueda, A., and Mena-Petite, A. (2013). Lettuce production and antioxidant capacity are differentially modified by salt stress and light intensity under ambient and elevated CO2. J. Plant Physiol. 170, 1517–1525. doi: 10.1016/j.jplph.2013.06.004
Pérez-López, U., Miranda-Apodaca, J., Muñoz-Rueda, A., and Mena-Petite, A. (2015b). Interacting effects of high light and elevated CO2 on the nutraceutical quality of two differently pigmented Lactuca sativa cultivars (Blonde of Paris Batavia and Oak Leaf). Sci. Hortic. 191, 38–48. doi: 10.1016/j.scienta.2015.04.030
Pérez-López, U., Sgherri, C., Miranda-Apodaca, J., Micaelli, F., Lacuesta, M., Mena-Petite, A., et al. (2018). Concentration of phenolic compounds is increased in lettuce grown under high light intensity and elevated CO2. Plant Physiol. Biochem. 123, 233–241. doi: 10.1016/j.plaphy.2017.12.010
Piñero, M. C., Pérez-Jiménez, M., López-Marín, J., and del Amor, F. M. (2017a). Fruit quality of sweet pepper as affected by foliar Ca applications to mitigate the supply of saline water under a climate change scenario. J. Sci. Food Agric. 98, 1071–1078. doi: 10.1002/jsfa.8557
Piñero, M. C., Otálora, G., Porras, M. E., Sánchez-Guerrero, M. C., Lorenzo, P., Medrano, E., et al. (2017b). The form in which nitrogen is supplied affects the polyamines, amino acids, and mineral composition of sweet pepper fruit under an elevated CO2 concentration. J. Agric. Food Chem. 65, 711–717. doi: 10.1021/acs.jafc.6b04118
Proietti, S., Moscatello, S., Giacomelli, G. A., and Battistelli, A. (2013). Influence of the interaction between light intensity and CO2 concentration on productivity and quality of spinach (Spinacia oleracea L.) grown in fully controlled environment. Adv. Space Res. 52, 1193–1200. doi: 10.1016/j.asr.2013.06.005
Rao, M. V., Hale, B. A., and Ormrod, D. P. (1995). Amelioration of ozone-induced oxidative damage in wheat plants grown under high carbon dioxide (role of antioxidant enzymes). Plant Physiol. 109, 421–432. doi: 10.1104/pp.109.2.421
Reich, M., van den Meerakker, A. N., Parmar, S., Hawkesford, M. J., and De Kok, L. J. (2016). Temperature determines size and direction of effects of elevated CO2 and nitrogen form on yield quantity and quality of Chinese cabbage. Plant Biol. 18, 63–75. doi: 10.1111/plb.12396
Ren, J., Guo, S.-S., Xin, X.-L., and Chen, L. (2014). Changes in volatile constituents and phenols from Gynura bicolor DC grown in elevated CO2 and LED lighting. Sci. Hortic. 175, 243–250. doi: 10.1016/j.scienta.2014.06.023
Robredo, A., Pérez-López, U., Miranda-Apodaca, J., Lacuesta, M., Mena-Petite, A., and Muñoz-Rueda, A. (2011). Elevated CO2 reduces the drought effect on nitrogen metabolism in barley plants during drought and subsequent recovery. Environ. Exp. Bot. 71, 399–408. doi: 10.1016/j.envexpbot.2011.02.011
Roosta, H. R., and Schjoerring, J. K. (2008). Effects of nitrate and potassium on ammonium toxicity in cucumber plants. J. Plant Nutr. 31, 1270–1283. doi: 10.1080/01904160802135050
Schjoerring, J. K., Husted, S., Mäck, G., and Mattsson, M. (2002). The regulation of ammonium translocation in plants. J. Exp. Bot. 53, 883–890. doi: 10.1093/jexbot/53.370.883
Sgherri, C., Pérez-López, U., Micaelli, F., Miranda-Apodaca, J., Mena-Petite, A., Muñoz-Rueda, A., et al. (2017). Elevated CO2 and salinity are responsible for phenolics-enrichment in two differently pigmented lettuces. Plant Physiol. Biochem. 115, 269–278. doi: 10.1016/j.plaphy.2017.04.006
Stitt, M., and Krapp, A. (1999). The interaction between elevated carbon dioxide and nitrogen nutrition: the physiological and molecular background. Plant Cell Environ. 22, 583–621. doi: 10.1046/j.1365-3040.1999.00386.x
Sun, P., Mantri, N., Lou, H., Hu, Y., Sun, D., Zhu, Y., et al. (2012). Effects of elevated CO2 and temperature on yield and fruit quality of strawberry (Fragaria × ananassa Duch.) at two levels of nitrogen application. PLoS One 7:e41000. doi: 10.1371/journal.pone.0041000
Tang, Y., Dong, J., Li, X., Gruda, N., and Duan, Z. (2018). Interactive effects of elevated carbon dioxide and nitrogen availability on fruit quality of cucumber (Cucumis sativus L.). J. Integr. Agric.
Taub, D. R., Miller, B., and Allen, H. (2008). Effects of elevated CO2 on the protein concentration of food crops: a meta-analysis. Glob. Change Biol. 14, 565–575. doi: 10.1111/j.1365-2486.2007.01511.x
Thompson, L., Peffley, E., Green, C., Pare, P., and Tissue, D. (2004). “Biomass, flavonol levels and sensory characteristics of Allium cultivars grown hydroponically at ambient and elevated CO2,” in Proceedings of the 34th International Conferences on Environmental Systems (ICES), Colorado Springs, Colorado. doi: 10.4271/2004-01-2300
van Groenigen, K. J., Osenberg, C. W., and Hungate, B. A. (2011). Increased soil emissions of potent greenhouse gases under increased atmospheric CO2. Nature 475, 214–218. doi: 10.1038/nature10176
Vorne, V., Ojanperä, K., De Temmerman, L., Bindi, M., Högy, P., Jones, M., et al. (2002). Effects of elevated carbon dioxide and ozone on potato tuber quality in the European multiple-site experiment ‘CHIP-project’. Eur. J. Agron. 17, 369–381. doi: 10.1016/S1161-0301(02)00072-2
Wang, D., Heckathorn, S. A., Wang, X., and Philpott, S. M. (2012). A meta-analysis of plant physiological and growth responses to temperature and elevated CO2. Oecologia 169, 1–13. doi: 10.1007/s00442-011-2172-0
Wang, S. Y., and Bunce, J. A. (2004). Elevated carbon dioxide affects fruit flavor in field-grown strawberries (Fragaria × ananassa Duch). J. Sci. Food Agric. 84, 1464–1468. doi: 10.1002/jsfa.1824
Wang, S. Y., Bunce, J. A., and Maas, J. L. (2003). Elevated carbon dioxide increases contents of antioxidant compounds in field-grown strawberries. J. Agric. Food Chem. 51, 4315–4320. doi: 10.1021/jf021172d
Wei, Z., Du, T., Li, X., Fang, L., and Liu, F. (2018). Interactive effects of elevated CO2 and N fertilization on yield and quality of tomato grown under reduced irrigation regime. Front. Plant Sci. 9:328. doi: 10.3389/fpls.2018.00328
Winsor, G. W., Davies, J. N., and Massey, D. M. (1962). Composition of tomato fruit. IV.—Changes in some constituents of the fruit walls during ripening. J. Sci. Food Agric. 13, 141–145. doi: 10.1002/jsfa.2740130301
Wu, X., Sun, S., Xing, G., Wang, G., Wang, F., Xu, Z., et al. (2017). Elevated carbon dioxide altered morphological and anatomical characteristics, ascorbic acid accumulation, and related gene expression during taproot development in carrots. Front. Plant Sci. 7:2026. doi: 10.3389/fpls.2016.02026
Xu, Z., Jiang, Y., and Zhou, G. (2015). Response and adaptation of photosynthesis, respiration, and antioxidant systems to elevated CO2 with environmental stress in plants. Front. Plant Sci. 6:701. doi: 10.3389/fpls.2015.00701
Keywords: antioxidants, climate change, elevated carbon dioxide, environmental factors, greenhouse vegetables, mineral, protein, soluble sugar
Citation: Dong J, Gruda N, Lam SK, Li X and Duan Z (2018) Effects of Elevated CO2 on Nutritional Quality of Vegetables: A Review. Front. Plant Sci. 9:924. doi: 10.3389/fpls.2018.00924
Received: 25 February 2018; Accepted: 11 June 2018;
Published: 15 August 2018.
Edited by:
Scott Alan Heckathorn, University of Toledo, United StatesReviewed by:
Gaurav Zinta, Shanghai Center for Plant Stress Biology (CAS), ChinaCristina Sgherri, Università degli Studi di Pisa, Italy
Copyright © 2018 Dong, Gruda, Lam, Li and Duan. This is an open-access article distributed under the terms of the Creative Commons Attribution License (CC BY). The use, distribution or reproduction in other forums is permitted, provided the original author(s) and the copyright owner(s) are credited and that the original publication in this journal is cited, in accordance with accepted academic practice. No use, distribution or reproduction is permitted which does not comply with these terms.
*Correspondence: Xun Li, eGxpQGlzc2FzLmFjLmNu Zengqiang Duan, enFkdWFuQGlzc2FzLmFjLmNu