- 1School of Biological Sciences, University of Reading, Reading, United Kingdom
- 2School of Pharmacy, University of Reading, Reading, United Kingdom
Plants are important resources in healthcare and for producing pharmaceutical drugs. Pharmacological and phytochemical characterization contributes to both the safe use of herbal medicines and the identification of leads for drug development. However, there is no recent assessment of the proportion of plants used in ethnomedicine that are characterized in this way. Further, although it is increasingly apparent that plants used in ethnomedicine belong to preferred phylogenetic lineages, it is not known how this relates to the focusing of research effort. Here we identify species and lineages rich in ethnomedicinal use and develop methods to describe how well they are known pharmacologically and/or phytochemically. We find 50% of plant species of the family Leguminosae used in ethnomedicine in Brazil, a geographical area where plants are an important part of healthcare, have been the focus of either phytochemical screening or testing for biological activity. Plant species which have more use reports are studied significantly more often (p < 0.05). Considering the taxonomic distribution of use, 70% of genera that include species with ethnomedicinal use have been studied, compared to 19% of genera with no reported use. Using a novel phylogenetic framework, we show that lineages with significantly greater numbers of ethnomedicinal species are phylogenetically over-dispersed within the family, highlighting the diversity of species used. “Hotnode clades” contain 16% of species but 46% of ethnomedicinally-used species. The ethnomedicinal species in hotnode clades have more use reports per species (p < 0.05), suggesting they are more frequently used. They are also more likely to be characterized pharmacologically and/or phytochemically. Research focus has followed traditional use by these measures, at least for these Brazilian plants, yet ethnomedicinal species yielding candidate drugs, raising public health concerns and more intensively studied lie outside of the hotnode clades.
Introduction
Plants are the primary health care resource in many communities around the world (Bannerman et al., 1983). They also serve as resources for the development of biomedicines, and traditionally-used species have contributed significantly to the development of biomedical drugs (Newman et al., 2000; Fabricant and Farnsworth, 2001; Cragg and Newman, 2013). It has been argued that ethnomedicinal use can be used to focus bioprospecting, addressing the decline in leads (Nwaka and Hudson, 2006) by provisioning new chemical entities or combinations of metabolites for testing (Gurib-Fakim, 2006; Sucher, 2013; Skirycz et al., 2016). Whether this is true or not, investigation of plants used in ethnomedicine is needed because public health may be compromised by ongoing use of some plants raises concerns (Jäger, 2015). Given these twin drivers of research, it might be expected that traditionally used plants are known rather well. However, there is a lack of knowledge about how well plants in general have been characterized; there are no explicit studies to investigate whether plants of ethnomedicinal importance are well known, and no recent data are available. The most cited source regarding the deficit of knowledge of plants comes from Verpoorte (1998), cited in Verpoorte (2000) and Fabricant and Farnsworth (2001). Verpoorte (1998) estimated that 6% of all plant species were screened for biological activity, and 15% evaluated phytochemically, but did not consider whether species were used in ethnomedicine. His estimates were made by compiling a count of the number of species in the NAPRALERT database against an estimate of total number of species.
Humans make direct use of only a proportion of the plant species around them, and it has long been known that plants with medicinal use are usually found more frequently in some families than in others (Moerman, 1991; Moerman et al., 1999). Phylogenetic methods are emerging as useful tools to describe these patterns (Saslis-Lagoudakis et al., 2011, 2012; Ernst et al., 2016). The well-known association of phytochemistry with taxonomy (Gibbs, 1974) is also increasingly better understood in terms of phylogeny (Wink, 2013). If lineages rich in species with ethnomedicinal use are those that have metabolites of interest, phylogenetic methods predict bioprospecting potential (Saslis-Lagoudakis et al., 2011; Ernst et al., 2016). Direct tests of phylogenetic structuring of phytochemistry for groups of ethnomedicinal and bioprospecting interest show that some (e.g., Rønsted et al., 2008) but not all (e.g., Grimbs et al., 2017) reveal phylogenetic signal. Nevertheless, it seems probable that close relatives of species of interest might yield larger quantities, or interesting variants, of valuable compounds.
The Leguminosae is one of the largest plant families (Lewis et al., 2005). With many documented uses, the family is over-utilized for medicine in some regions (Korea and Ecuador) but under-utilized in others (North America) (Moerman et al., 1999). Close-related species share similar secondary metabolites that could be of interest (Wink, 2003) and phylogenetic structure in the distribution of secondary metabolites has been well documented for the family (Wink, 2013). In Brazil, the Leguminosae comprises c. 2,800 species in more than 200 genera (Flora of Brazil 2020 in Construction, 2016). The species diversity, widespread distribution, and many reported uses (Souza and Hawkins, 2017), and the availability of phylogenetic information (Legume Phylogeny Working Group [LPWG], 2013) have prompted us to select the family as a case study. We focus on Brazil since its vast biodiversity is a potential source of new medicines as well as a source of primary health care: it is estimated that 66% of the population has no access to commercial drugs (Mazzari and Prieto, 2014).
The objective of this study was to provide a contemporary estimate of the extent to which the plants used in ethnomedicine have been investigated phytochemically and/or pharmacologically and relate this to the ethnomedicinal importance of the plant species and their lineages. Ethnomedicinal importance was estimated from the frequency of use reports in our existing compilation of ethnomedicinal Leguminosae in Brazil (Souza and Hawkins, 2017; www.ewedb.com). To identify ethnomedicinally important lineages, we made a phylogenetic study of the medicinal uses of our target group, the Leguminosae. Until now, phylogenetic investigations of ethnomedicinal plants have considered global floras (Halse-Gramkow et al., 2016), whole floras (e.g., Saslis-Lagoudakis et al., 2012) or genera throughout their range (e.g., Saslis-Lagoudakis et al., 2011; Ernst et al., 2016). As well as being the first study focused at family level, this study is also the first to study the frequency of reported use per species, in order to elucidate the phylogenetic relationships of ethnomedicinally important plants. Ultimately, we present a set of measures that show how research effort on plants with ethnomedicinal use, and their relatives, is focused.
Materials and Methods
Ethnomedicinal Use of Legumes in Brazil
Published ethnomedicinal use data compiled for the Leguminosae of Brazil (Souza and Hawkins, 2017; www.ewedb.com) were used for this study. Publications citing ethnomedicinal uses of Leguminosae species in Brazil were identified using online citation indices. We also used direct searches of unindexed journals, including the following journals not indexed by PubMed: Acta Botanica Brasilica, Flovet, Revista Brasileira de Biociencias, Revista Brasileira de Farmacognosia, Revista Brasileira de Plantas Medicinais, and Rodriguesia. Herbarium data was extracted from the online list of herbarium and biological collections from Brazil, Species Link1. The file was exported to excel format and a search using the keywords “medicinal” and “uses” (in Portuguese) was conducted to select only the specimens with ethnomedicinal information. Generic and species names followed the Flora of Brazil (Brazilian Flora, 2020), and were corrected (standardized) using the Plantminer R script (Carvalho et al., 2010).
Pharmacology of Species Used in Brazil
Pharmacological data associated with the Brazilian ethnomedicinal species, and for all genera, whether used or not, were sought online using online citation indices including “Web of Science” (WoS). Search terms included the species name and commonly used synonyms, together with “pharmacolog∗”. The number of results from WoS for each species was recorded, together with publications related to pharmacological studies. As for ethnomedicinal use, names were standardized to follow the Flora of Brazil (Brazilian Flora, 2020).
Analysis
From our surveys, we compiled data describing the presence or absence of use reports (citation of ethnomedicinal use in the literature) at species and generic level, and the number of use reports at species level. We scored whether each species was the subject of pharmacological research, and record research effort for each species as the number of published studies that investigate each species. We performed Spearman’s Rank Correlation test at species level to determine whether species with more use reports were the focus of more pharmacological research effort.
To understand the distribution of ethnomedicinal species in the family, a phylogenetic tree for the Leguminosae in Brazil was reconstructed at genus level, with one species per genus being sampled. Sequences from the DNA marker MatK were compiled from the Tree of Legumes (Legume Phylogeny Working Group [LPWG], 2013) when present, and from publicly available sequences on Genbank. All accession numbers are detailed in Supplementary Table S1. In total, 203 genera were sampled (92.7% of the total flora). Sequences were aligned using MUSCLE alignment available in Geneious 8.0 and adjustments were made manually. Sequence data was analyzed under the maximum-likelihood (ML) criterion, under the GRTGAMMA model as implemented in RAxML 7.2.8 (Stamatakis, 2014). Unsampled genera and species were added manually as polytomies using the R package PHYTOOLS and the function add.species.to.genus to generate a species tree (Revell, 2012). To make the tree ultrametric, we used the function chronos from the package APE (Paradis et al., 2004). The phylogenetic hypothesis obtained was compared to the Tree of Legumes (Legume Phylogeny Working Group [LPWG], 2013).
Having confirmed our phylogeny was congruent with the existing hypothesis for the Leguminosae, the phylogenetic structure of ethnomedicinal uses was investigated using tools widely used in community phylogenetics (Pearse et al., 2014). We calculated the Net Relatedness Index (NRI) and Nearest Taxon Index (NTI) (Webb, 2000; Webb et al., 2002), with the functions ses.mpd and ses.mntd from the PICANTE package (Kembel et al., 2010). The chosen null model was “taxa.labels”, which shuffles the distance matrix labels across all taxa included in distance matrix with 999 runs. The NRI and NTI values were obtained by multiplying the MPD (Mean phylogenetic distance) and MNTD (Mean Nearest Taxon Distance) values by -1. The NRI value represents the average distance between a species and all other species with the same characteristic (in our case ethnomedicinal use). Higher values of NRI (negative for MPD) indicates a phylogenetic clustering of a sample, while negative values (positive for MPD) show an overdispersion of the studied taxa. Similarly, NTI (and MNTD) values can indicate clustering or dispersion (Figure 1). According to Webb (2000), NRI values describe a deep relationship in the phylogeny, which here might be evidence for clustering or overdispersal at tribal or sub familial levels. On the other hand, NTI describes relatedness in terminal clades, in our case clustering or overdispersion of congeneric ethnomedicinal plants (see also Mazel et al., 2016). Phylogenetic structure was investigated for the ethnomedicinal species compared to the whole Leguminosae flora in Brazil. “Hotnode clades” (as described by Saslis-Lagoudakis et al., 2012) were sought using the NODESIG function in also in R (R Core Team, 2015) adapted by Abellán et al. (2016).
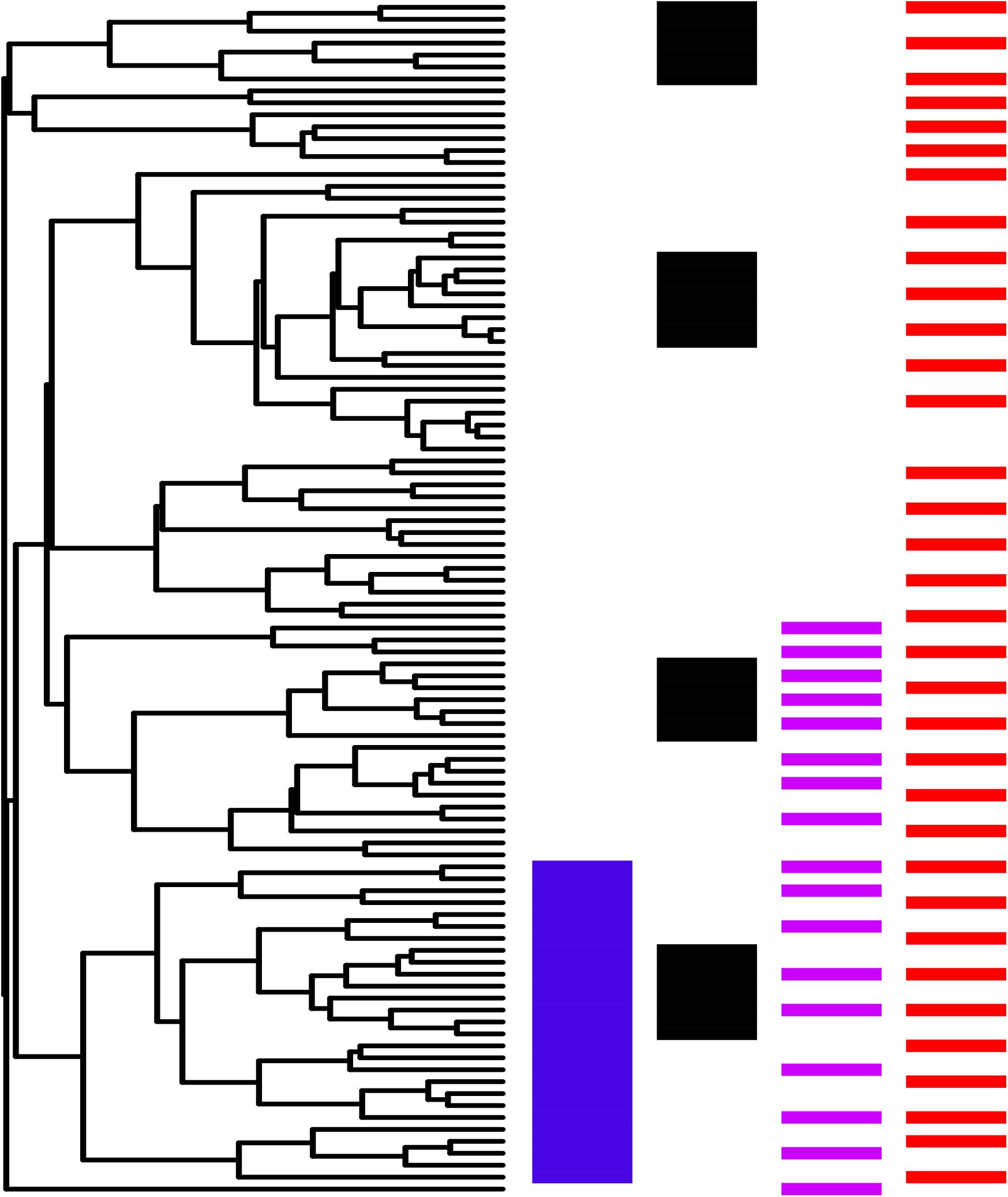
FIGURE 1. Schematic representation of scenarios of relatedness. Clustering and overdispersion are inferred from MPD and MNTD values (and their related NRI and NTI indices respectively). MPD describes relationships that occurred on deep nodes of the phylogeny, while MNDT reflects relationships at shallower nodes. Combined values can be used to infer different scenarios. Blue, phylogenetic clustering, MPD and MNTD positive; black, overdispersion of clustering, MPD negative and MNTD positive; pink, clustering of overdispersion, MPD positive and MNTD negative; red, phylogenetic overdispersion, MPD negative and MNTD negative. The phylogenetic tree was generated with the function “rtree” from the package ape using R.
The phylogenetic framework was used to address the following questions:
1. Are there more species that have been the focus of pharmacological study within the lineages that are hotnode clades for ethnomedicinal use?
2. Is intensity of use of species for ethnomedicine greater in the lineages that are in hotnode clades for ethnomedicinal use
3. Is the intensity of research effort greater within hotnode clades?
4. Do hotnode clades yield more drugs?
Intensity of ethnomedicinal use was estimated based on the number of citations/use reports in the database. Intensity of research effort was measured by counting the number of reported studies (publications) for each species. The intensity of research effort was compared for the species ethnomedicinally-used for medicine inside and outside of the hotnode clades. A Wilcoxon test was used to determine whether there are significantly more pharmacological studies outside of hotnode clades than would be expected if pharmacological effort was homogeneous.
To assess whether traditional use coincides with development of commercial drugs we extracted the list of genera yielding FDA-approved and clinical-trial drugs (drug genera) from the recent large-scale study of Zhu et al. (2011) and recorded the number of genera found in Brazil. We also recorded whether these genera were present in hotnode clades.
All underlying research data is available from the corresponding author.
Results
Data Description
There were 286 species (10% of the total legume flora) in 104 genera (48% of the total legume genera) reported to have ethnomedicinal use (Figure 2). The pharmacological review identified 98 genera that had been screened (43% of genera). There were 143 ethnomedicinally-used species (50% of the species used) that had been the focus of research described in 3047 publications abstracted by the Web of Science. The ethnomedicinally-used species that had been screened are found in 77 genera, thus 72% of genera with ethnomedicinal use had been the focus of pharmacological study. A much lower percentage of the 118 genera without any ethnomedicinal use had been studied (22 genera, 19%). The Spearman’s Rank Correlation showed significant positive correlation between use and pharmacological study at the species level (p < 0.05; ρ = 0.36).
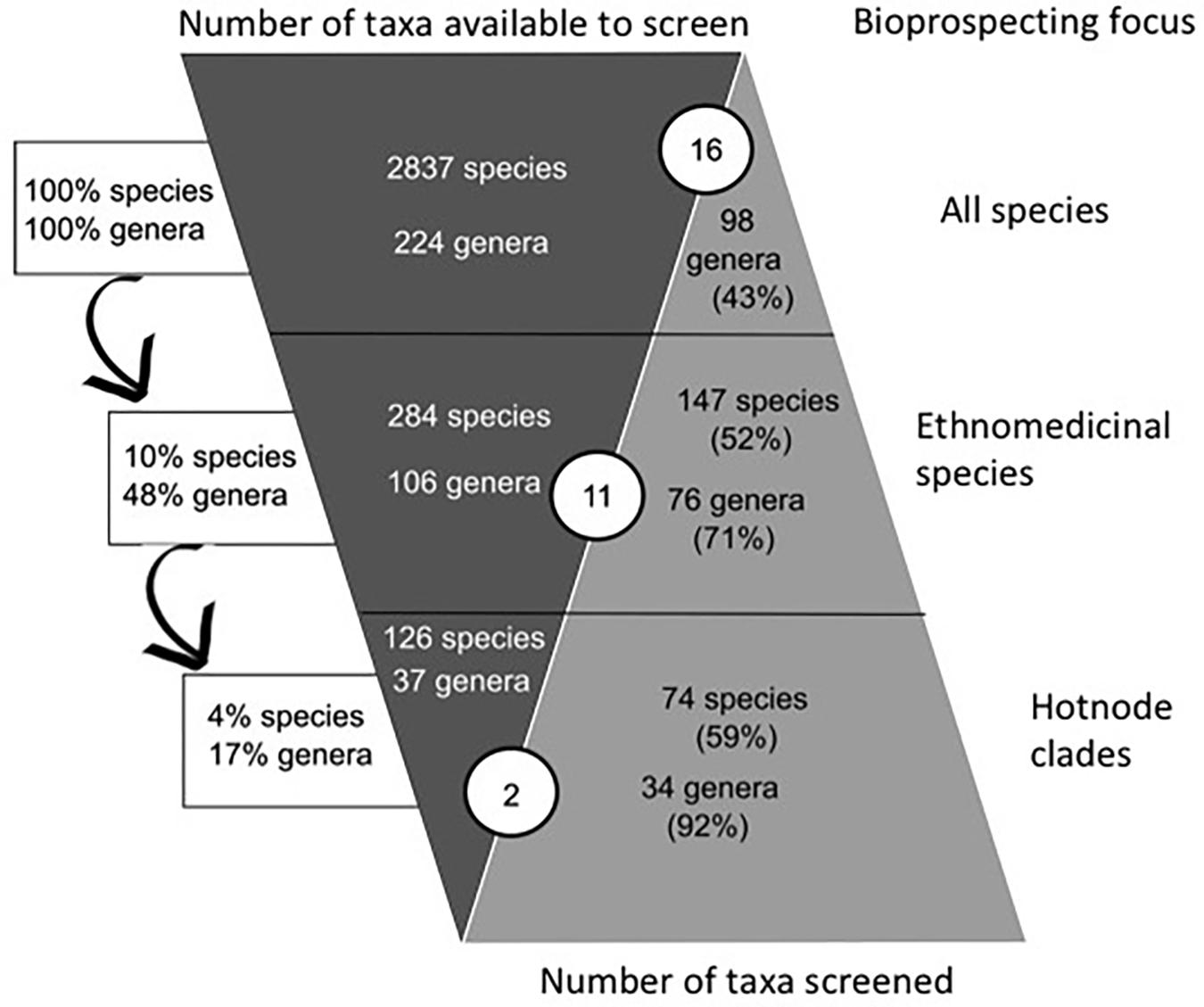
FIGURE 2. Comparison of ethnomedicinal selection and pharmacological research effort. The left “column” (dark gray) shows the decreasing numbers of potential leads, if focus is on taxa (middle row) or lineages (bottom row) with ethnomedicinal use. This column shows that a focus on ethnomedicinal hotnode clades reduces the size of the species pool from 2837 species in total to 126 species that have ethnomedicinal use and are inside a hotnode clade. The arrows show the proportions of total flora represented in the samples, for example, the 126 species with ethnomedicinal use in the bottom row represent 4% of all species. This is 4% of all species. The right “column” (light gray) shows the proportion of these taxa that have been screened according to our survey. If focus is on the whole flora, there are 98 genera that have been investigated pharmacologically (43% of all genera). Considering the ethnomedicinally important lineages (bottom row), there are 34 genera with ethnomedicinal use and have been characterized pharmacologically that belong to hotnode clades (92% of the genera with ethnomedicinal use and in hot modes). The circled numbers in blue show the number of species that have yielded drugs approved for use or clinical trial by the FDA. As focus on ethnomedicinally important groups increases, there is a concomitant increase in research effort, but fewer drugs approved for use or clinical trial by the FDA.
Phylogenetic Analysis
The phylogeny we reconstructed (Supplementary File S1) was congruent with current hypotheses of relationship for the Leguminosae. The investigation of the phylogenetic structure of ethnomedicinal species for medicine shows an over-dispersed structure for overall ethnomedicinal use (NRI and NTI < 0, p < 0.05) (Figure 3). The same pattern of overdispersal was found for therapeutic applications considered separately (Supplementary Table S2). The Nodesig analysis showed 126 ethnomedicinal species (44% of species with at least one use report) were inside hotnode clades, but only 16% of the total flora (Figure 2 and Table 1).
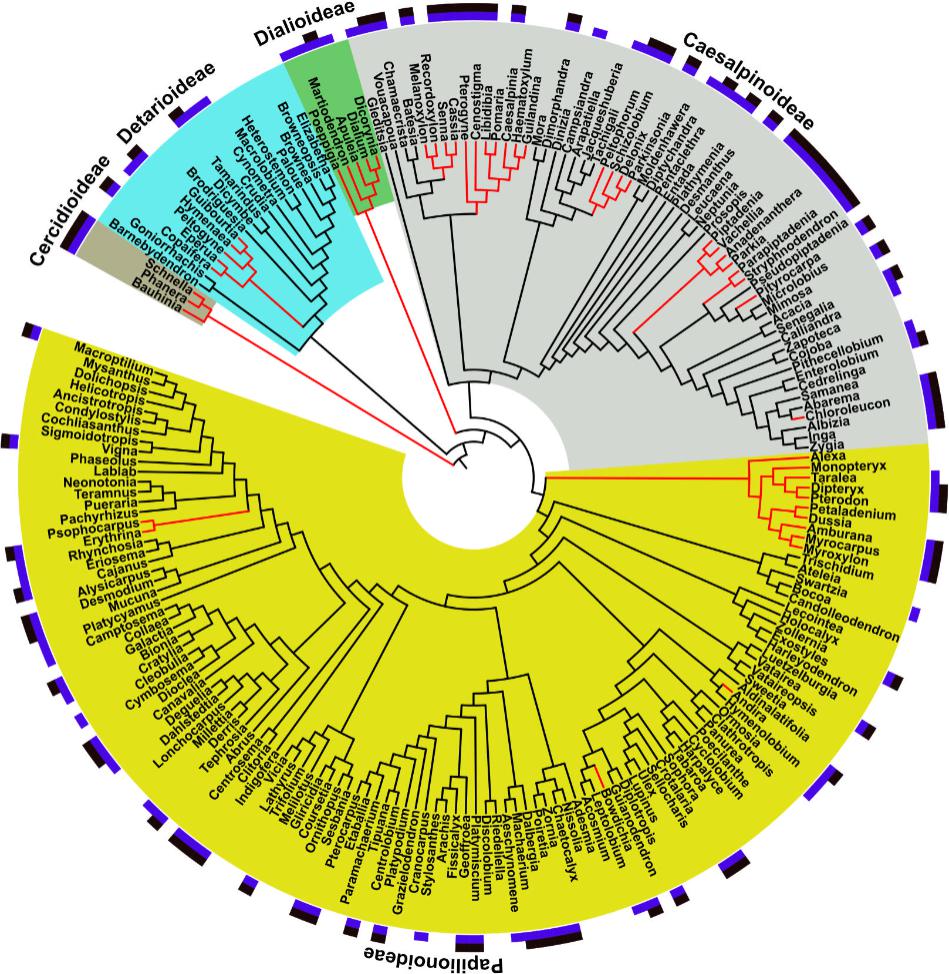
FIGURE 3. Distribution of medicinal uses and pharmacological studies in the Leguminosae phylogeny and “Hotnode clades” for ethnomedicinal use. Block colors at the tips: purple, ethnomedicinal use for that genus; black, pharmacological studies for that genus. Branch colors: red branches, genera present in hotnode clades for species with ethnomedicinal use. Clade colors: yellow, grey, green, light blue and brown indicate membership of subfamilies. Figure prepared using iTOL online (Letunic and Bork, 2016).
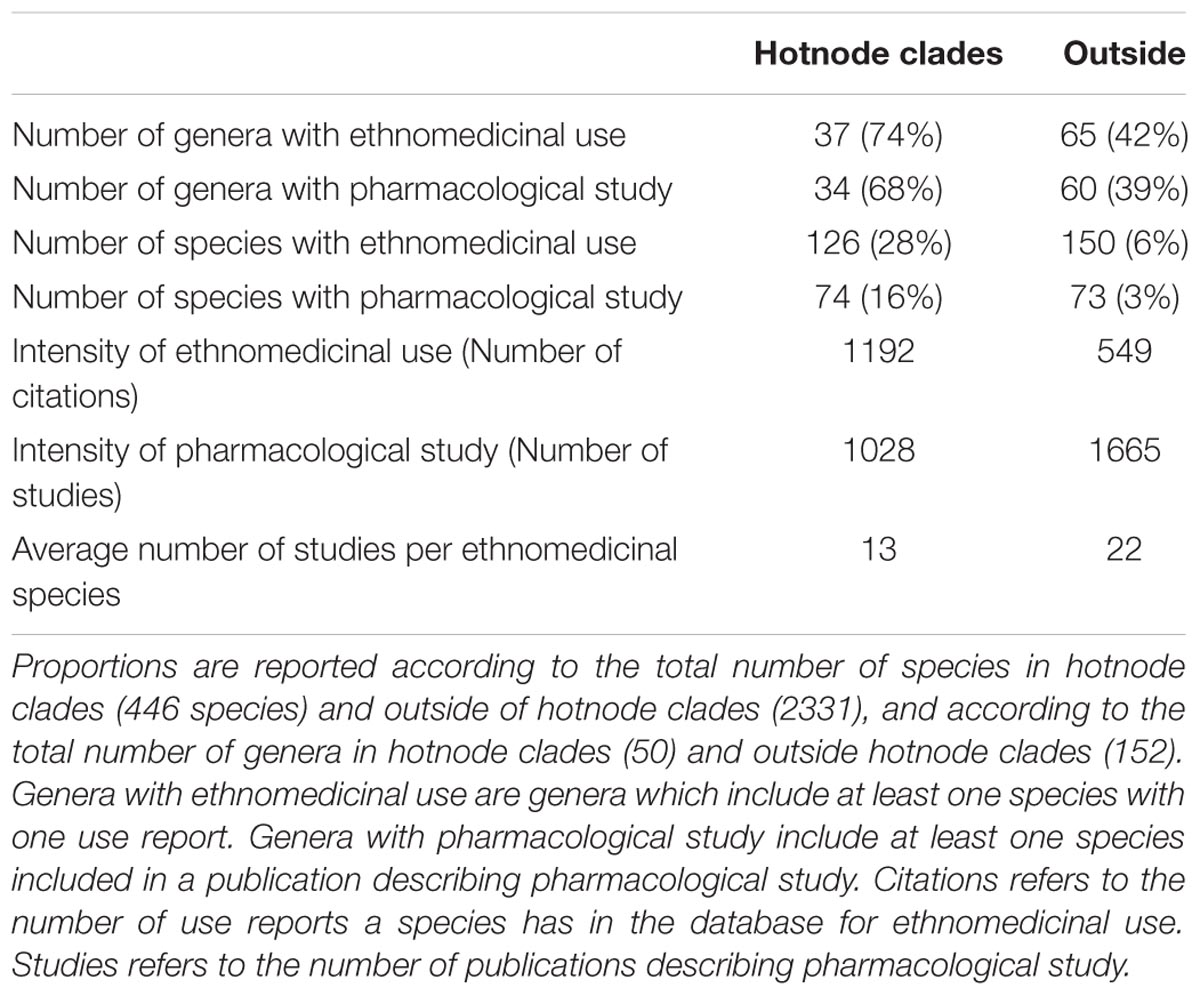
TABLE 1. Characterization of “hotnode clades” in terms of absolute numbers and proportions of species and studies.
1. There are more species that have been the focus of pharmacological study within the lineages that are hotnode clades for ethnomedicinal use. Of the 126 hotnode clade species with ethnomedicinal use, 74 (16% of all hotnode clade species) were the focus of at least one pharmacological study. A lower number, 73 species (3% of all non-hotnode clade species) of the 150 ethnomedicinal species outside hotnode clades had been screened (Table 1) revealing a preference for screening ethnomedicinal species that are in hotnode clades.
2. The intensity of ethnomedicinal use of species for medicine is greater in the lineages that are hotnode clades for ethnomedicinal use. Species with ethnomedicinal use present in the hotnode clades were cited almost twice as often as the ones outside the hotnode clades (p < 0.05; Table 1).
3. The intensity of research effort is not significantly greater for ethnomedicinal species inside hotnode clades. Although there were numerically more species from hotnodes screened, no significant difference was found for the intensity of screening between ethnomedicinal species inside and ethnomedicinal species outside of hotnode clades. In fact, ethnomedicinal species outside hotnode clades are more studied. Figure 4 shows on a phylogeny how intensity of use and intensity of study are distributed.
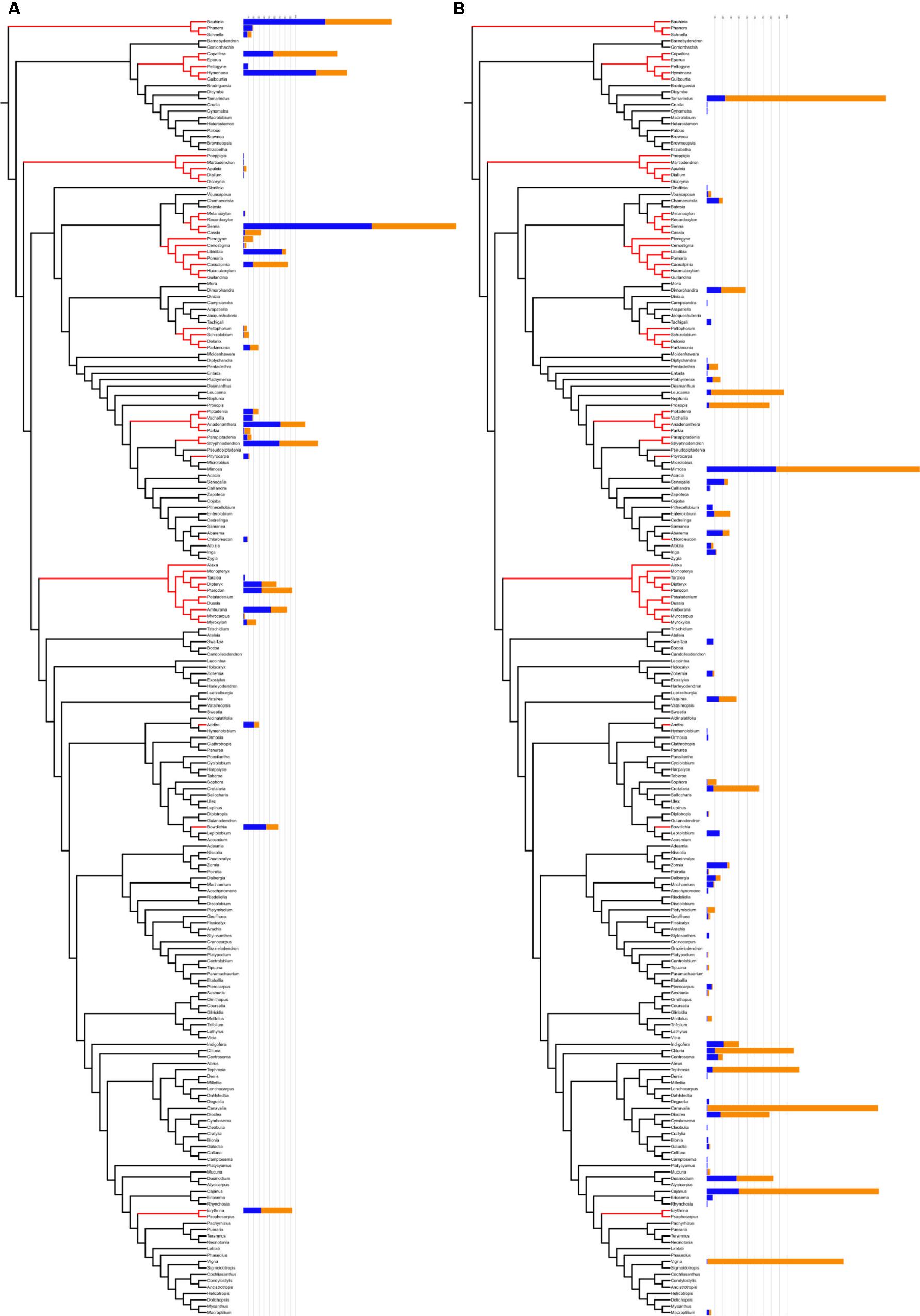
FIGURE 4. Comparison between hotnode clades (A) and other nodes (B) in relation to number of citations (blue) and number of pharmacological studies (orange). Hotnode clades are calculated based on the distribution of recorded use of species. Species in hotnode clades have significantly more use reports (p < 0.05). Figure prepared using iTOL online (Letunic and Bork, 2016).
4. Hotnode clades yield fewer drugs approved by FDA for use or clinical trials. We identified 16 genera found in Brazil that include species (not necessarily from Brazil) that have yielded drugs approved for use or clinical trial by the FDA. We found that 11/14 of these genera have species with ethnomedicinal use in Brazil, and that only 2/11 of these genera belong to hotnode clades. Table 2 shows the therapeutic applications recorded for all Brazilian species belonging to these genera, and indicates whether each genus belong to a hotnode.
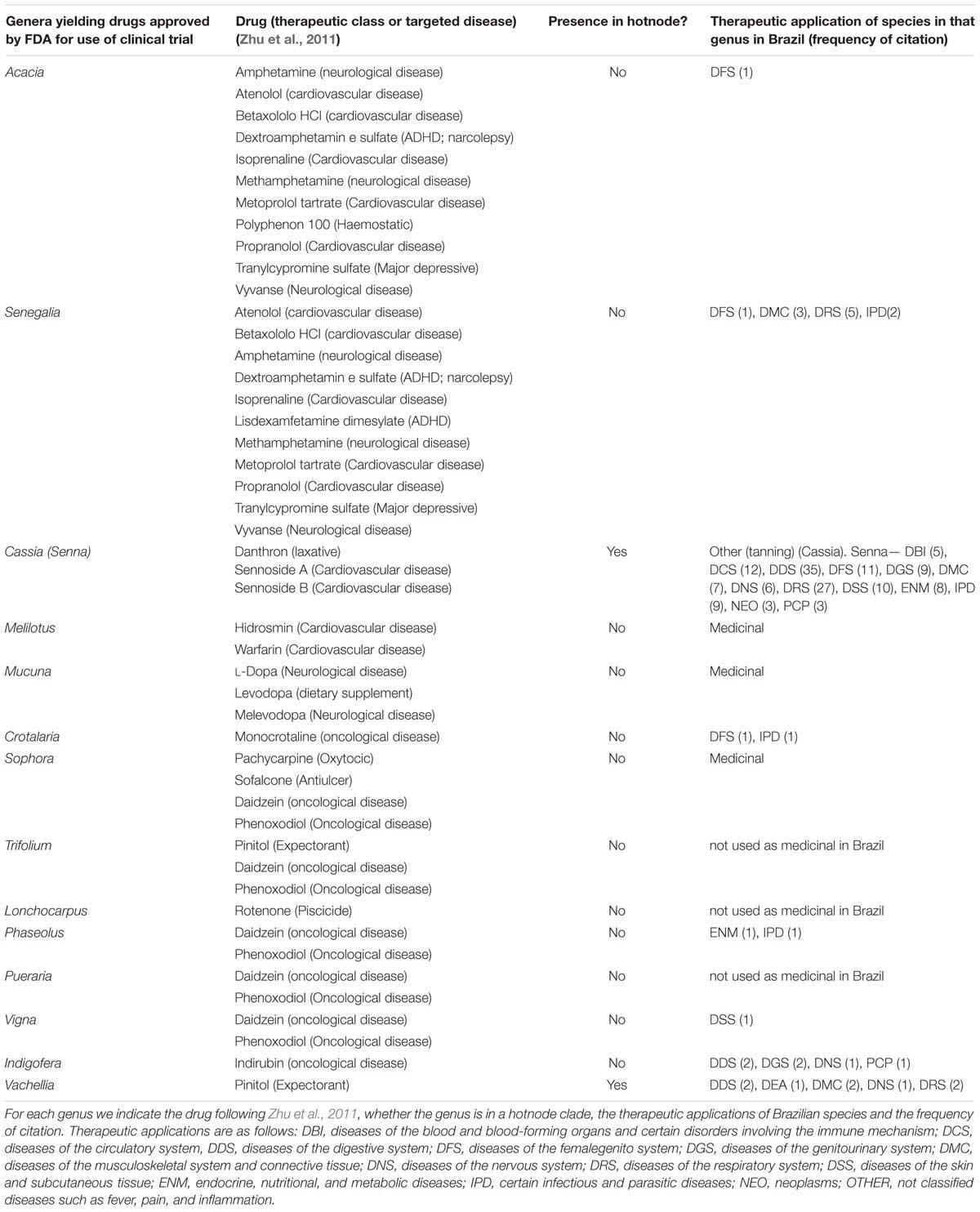
TABLE 2. The 16 genera found in Brazil that include species (not necessarily from Brazil) that have yielded drugs approved for use or clinical trial by the FDA and their ethnomedicinal use, if any, in Brazil.
Discussion
This study reveals that half of the species of Leguminosae used in ethnomedicine in Brazil have been the focus of at least one phytochemical or pharmacological study. Despite the importance of ethnomedicinal plants in bioprospecting and local health care, the frequently cited global estimates of 9% of plants screened do not consider whether plants are used in ethnomedicine or not (Fabricant and Farnsworth, 2001). Although ethnomedicinal use is not taken into account, and this figure is now more than 15 years old, it probably remains our best estimate of how well known are the properties of ethnomedicinally important plants. Our study is an original insight into this question, and shows that at least for some ethnomedicinally important plant lineages in some parts of the world, there is now considerable scientific knowledge of the properties of plants. The consideration of the relationship between pharmacological study and intensity of ethnomedicinal use we present here is a timely first step towards a data-driven evaluation of how ethnomedicine might inform bioprospecting, or direct research into the health risks associated with local plant-based healthcare.
A wider audit is needed to determine whether our estimate of 50% of plants screened applies beyond the geographical and taxonomic limits of our study. Investment in natural products research informed by traditional knowledge has fluctuated (Koehn and Carter, 2005), and while there may be many factors at play, Brazil has invested in realizing the value of local and indigenous knowledge of indigenous plant resources through pharmacological research (Dutra et al., 2016). This might suggest that Brazilian plants could be rather well known compared to plants in other biodiversity hotspots. However, our findings should be considered in the light of Brazilian government policy to integrate herbal medicines into their healthcare system. Because of the huge number of plant species used medicinally in Brazil, the Health Ministry policy focused efforts on elucidating the efficacy and safety of 71 promoted ethnomedicinal plant species (Ministério da Saude, 2009; SUS, 2009; Mazzari and Prieto, 2014). It might be expected that there have been fewer resources directed towards characterization of non-list species, though seven of the listed species belong to family Leguminosae. That many species are found outside of Brazil and we did not limit our survey of research effort to Brazilian research groups might increase likelihood of screening of plants. The family Leguminosae is a rather well-known plant family taxonomically, so it will be interesting to see whether it is better-known than other families. Our study might serve as a start point from which an evolutionarily-informed audit of scientific knowledge of ethnomedicinal plants could be developed.
The approach taken here goes far beyond counting the number of species used in ethnomedicine and recording whether these plants are the focus of pharmacological studies. We use a phylogenetic framework to identify evolutionary lineages that are particularly important in ethnomedicine, and record whether species in those lineages are more often screened than other ethnomedicinal plants. A first step was the phylogenetic characterization of ethnomedicinal species of Leguminosae in Brazil plants, and we revealed novel patterns with significant implications for bioprospecting. Previous phylogenetic studies reported clustering of species with ethnomedicinal use, both for whole floras (Saslis-Lagoudakis et al., 2012), and when species level phylogenies are used to interrogate the patterns of plant use in genera (Saslis-Lagoudakis et al., 2011; Ernst et al., 2016). Our study is the first focused at the level of a plant family, and also the first to recover a signal of phylogenetic overdispersal (Table 1). Phylogenetically-informed bioprospecting until now has been concerned with clustering, whether recovered using D-statistics (Rønsted et al., 2008), Pagel’s lambda (Cámara-Leret et al., 2017), or community phylogenetic methods (Saslis-Lagoudakis et al., 2011, 2012, 2014; Yessoufou et al., 2015; Ernst et al., 2016; Halse-Gramkow et al., 2016). Overdispersal challenges the established use of phylogeny as a predictive tool to inform ethnopharmacological screening, but here we show that hotnode clades can still be identified and lineages of interest highlighted (Figure 2). Bioactivity of plant species is related to the presence of secondary metabolites that mediate interactions of plant species with pathogens and herbivores, other plants, and pollinators (Bourgaud et al., 2001; Edris, 2007; Heil, 2008; Huang et al., 2012). While some metabolites in the legumes, such as flavonoids, triterpenes, and pinitol, are of broad occurrence and can be found in diverse groups, others are limited in their phylogenetic distribution (Wink, 2013). The pattern of overdispersal we find here might result from the adoption of multiple lineages with different secondary metabolites for ethnomedicine. The interplay of chemical and phylogenetic diversity underscores that the hierarchical level of the study is important in devising and interpreting any study. Saslis-Lagoudakis et al. (2012) indicated in a study across all Angiosperms that plants of the Leguminosae were included in cross-cultural hotnode clades. However, in this study at the family level, overdispersal reveals phylogenetically non-random use, comprising overdispersed lineages that we hypothesis here are selected for their diverse chemistries. Many more studies are needed to determine how general these emerging patterns are, and whether they apply to less species-rich families. In the context of what might be overdispersed clusters (Figure 1) a fresh view of priorities for pharmacological research effort can be made.
Our data show how pharmacological studies are distributed relative to hotnode clades. Although hotnode clades comprise only 14% of total species they include almost half of the ethnomedicinal species. As well as being evolutionary hotspots for ethnomedicinal species, overall the hotnode clades encompass significantly more use reports. These same hotnode clades also include more than half of the ethnomedicinally used species that have been the focus of pharmacological investigation. Our study corroborates the view that ethnomedicinal use guides pharmacological research (Gurib-Fakim, 2006), showing that close relatives of ethnomedicinal plants are also the focus of study. Using a phylogenetic framework we highlight here how just a small part of the phylogeny concentrates both traditional knowledge and pharmacological research, whilst overall the diversity of ethnomedicinal species outside of hotnode clades are relatively poorly known.
Emphasis on few lineages would benefit bioprospecting if valuable leads are more likely to be encountered in hotnode clades. A total of 35 species belonging to 25 genera in family Leguminosae, 14 of which are found in Brazil, yield 44 of the products approved for clinical trial by the FDA (Zhu et al., 2011). Only two genera of the 15, Senna and Vachellia, fall into a Brazilian hotnode clade. This is in complete contrast to the findings of Saslis-Lagoudakis et al. (2012), who found a significant association between approval and membership of hotnode clades. The discrepancy might be attributed to the different hierarchical level of study, or to the choice of Leguminosae as a study group. Whilst the whole Leguminosae falls into a hotnode clade in the study of three floras (Saslis-Lagoudakis et al., 2012), focusing the study on the family means that lineages within the Leguminosae are discriminated. We suggest here that our hotnode clades include plants of mild action, whilst clinical investigation has prioritized species of relatively high toxicity. It is known, for example, that species of family Leguminosae used as contraceptives or abortifacients show high toxicity and yield anticancer therapeutics (Leonti et al., 2017). To illustrate the relationship between mild action and frequent use, we can contrast uses of species of two genera, selected here because they are of similar size and exemplify genera inside and outside of hotnode clades, Crotalaria and Copaifera. Crotalaria is represented by 42 species in Brazil, five of which are used based on 15 use reports. Use of these species in Brazilian ethnomedicine is for very specific applications, including as abortifacients or vermifuges. Crotalaria species contain the pyrrolizidine alkaloid (PA) monocrotaline, which has been investigated as an anti-tumor agent (Kusuma et al., 2014). In contrast, the 26 species of Copaifera in Brazil, known to be of mild effect, have 70 use reports for six species. Crotalaria, unlike Copaifera, does not belong to a hotnode clade but is nested in a clade with Sophora (1 species, one use report), Ulex (1 species, not used), Sellocharis (1 species, not used) and Lupinus (31 species, none used), all plants with PAs (Wink, 2013). Copaifera and its close relatives in the hotnode clade (Eperua, Hymenea; Figure 2) contain diterpene resins with antimicrobial activity. These data exemplify the infrequent traditional use of toxic species and their relatives, compared to the species of mild effect in common use. If novel drug leads from the Leguminosae are developed for their cytotoxicity, then bioprospecting effort should be focused on species outside of hotnode clades. In the case of Crotalaria, ultimately monocrotaline was shown to be a hepatocarcinogen which causes pulmonary hypertension and veno-occlusive disease (e.g., Knutsen et al., 2017). Although many plants of high toxicity do not ultimately become commercialized therapeutics, the potential of more toxic products might explain the predominance of plants outside of hotnode clades in the FDA lists (Table 2). We find that although fewer ethnomedicinal species outside of hotnode clades are studied, but when they are there are more studies published. This finding might further support the view that these plants are toxic or have potential as leads.
Whether or not novel leads remain to be discovered, there are compelling reasons to characterize plants that represent different lineages in ethnomedicine. Lack of knowledge may be problematic because health risks associated with ethnomedicinal plant use may be unreported (Jäger, 2015). Studies on safety, and especially well-conducted clinical trials, are rare in Brazil (Dutra et al., 2016). Mazzari and Prieto (2014) highlighted little attention, in the Brazilian context, to the safety of herbal medicines. We show studies characterizing plants are most often of commonly used species from hotnode clades. For example, Brazilian species belonging to the Leguminosae genera Stryphnodendron (Costa et al., 2013), Erythrina (Dias et al., 2013), and Pterodon (Dutra et al., 2016), including plants used extensively in local medicine, have been the focus of recent pharmacological and toxicological survey without substantiating concerns. Stryphnodendron adstringens and Erythrina mulungu are listed by the Brazilian Health Ministry as priority species, and are found in our hotnode clades; Caesalpinia ferrea and Bauhinia variegata are also found in hotnode clades and are listed by the Brazilian Health Ministry. Plants outside of the hotnode clades are particularly poorly known, and it is notable that the only plant of family Leguminosae in the Brazilian Health Ministry list that is not either a common and global agricultural food or fodder plant or in a hot node is Dalbergia subcymosa, a plant with few reported uses in women’s medicine and relatively poorly known from a pharmacological or phytochemical perspective. Phylogenetic methods could be used in tandem with ethnobotanical databases to predict the health risks associated with uncharacterized plant species. Here we show that lineages rich in ethnomedicinal use have more ethnomedicinal species that are the focus of pharmacological studies, showing effort is directed towards characterizing closely-related, most-used species. Just as for bioprospecting purposes, it could be argued that phylogenetically-isolated species should be consciously included in future research. Plants outside of hotnode clades may raise more concerns for the safety of the users of traditional medicine, though they are less used.
Our study considered the presence or absence of ethnomedicinal use, and the presence or absence of phytochemical or pharmacological studies. We also considered intensity of ethnomedicinal use, where the number of use reports served as a proxy for intensity of use. Research effort was recorded by recording the number of published studies we found in our online searches. We are confident in our estimation of presence of ethnomedicinal use, since we make a thorough survey of literature and herbarium records (Souza and Hawkins, 2017). We consider number of use reports to be a reasonable estimate of ethnomedicinal intensity of use, though in the case of flowers, fruits, and seeds, their infrequent use may be their seasonal availability may reduce the number of reports (Benko-Iseppon and Crovella, 2010). Counting papers to estimate research effort may be more flawed. Firstly, it is difficult to make a complete search when publications might use a range of very broad or specific key words. Further, the actual studies may be more revealing than the fact that they exist. Although many pharmacological studies were found for the ethnomedicinal species, the quality and significance of the studies were not easily assessed. Many low impact studies use meaningless bioassays, and report differences between in vitro and in vivo experiments that are a consequence of data quality (Gertsch, 2009). Finally, we explore only ethnomedicinal use and phylogeny as potential factors contributing to the selection of a species for phytochemical or pharmacological studies. Other factors may be important, for example the availability of plentiful plant material (Atanasov et al., 2015); we note the species that are the most intensively screened often have food or fodder use, for example Canavalia species are used as food for cattle. Existing protocols and emerging interest may create a snowball effect, so that many studies are made of species of already proven interest, or of their close relatives.
Brazil is a megadiverse country with a rich history of plant use for medicine, but this knowledge and natural resource is yet to be transformed into products. Although ultimately the regulatory and research infrastructure together determine the success or failure of the drug development pipeline, here we consider aspects at the interface between traditional knowledge and biodiversity. This study shines a light on the phylogenetic distribution of ethnomedicinal plants in family Leguminosae from Brazil. In highlighting an overdispersed structure for the first time it draws attention to the complex nature of plant selection for ethnomedicinal use. It also highlights a connection between lineages of importance for ethnomedicine and those that have become the focus of ethnopharmacological research, raising questions about the drivers of selection of species for screening in pharmacological studies. Much has been written about means devising rational, data-driven selection of plants for screening, for example by inferring useful phytochemical composition from ethnobotanical, chemosystematic or ecological information (Coley et al., 2003; Tan et al., 2006; Douwes et al., 2008; de Albuquerque, 2010; Saslis-Lagoudakis et al., 2011; Albuquerque et al., 2012), or from phylogeny (Rønsted et al., 2008; Saslis-Lagoudakis et al., 2012; Grace et al., 2015; Yessoufou et al., 2015; Ernst et al., 2016). Ultimately, our study demonstrates a method to identify the plant lineages that are most important ethnomedicinally. As research into natural products grows, this framework provides novel insights into how knowledge of ethnomedicinal plants is distributed and might be used.
Author Contributions
ENFS and JAH designed the study. All authors contributed to the analysis of the results and to the writing of the manuscript.
Funding
The present work was possible thanks to Coordenação de Aperfeiçoamento de Pessoal de Nível Superior (CAPES-Brazil) and the Program “Science Without Borders” scholarship for ENFS, BEX:7595-13-1.
Conflict of Interest Statement
The authors declare that the research was conducted in the absence of any commercial or financial relationships that could be construed as a potential conflict of interest.
Supplementary Material
The Supplementary Material for this article can be found online at: https://www.frontiersin.org/articles/10.3389/fpls.2018.00834/full#supplementary-material
Footnotes
References
Abellán, P., Carrete, M., Anadón, J. D., Cardador, L., and Tella, J. L. (2016). Non-random patterns and temporal trends (1912–2012) in the transport, introduction and establishment of exotic birds in Spain and Portugal. Divers. Distrib. 22, 263–273. doi: 10.1111/ddi.12403
Albuquerque, U. P., Ramos, M. A., and Melo, J. G. (2012). New strategies for drug discovery in tropical forests based on ethnobotanical and chemical ecological studies. J. Ethnopharmacol. 140, 197–201. doi: 10.1016/j.jep.2011.12.042
de Albuquerque, U. P. (2010). Implications of ethnobotanical studies on bioprospecting strategies of new drugs in semi-arid regions. Open Complement. Med. J. 2, 21–23. doi: 10.2174/1876391X01002020021
Atanasov, A. G., Waltenberger, B., Pferschy-Wenzig, E. M., Linder, T., Wawrosch, C., Uhrin, P., et al. (2015). Discovery and resupply of pharmacologically active plant-derived natural products: a review. Biotechnol. Adv. 33, 1582–1614. doi: 10.1016/j.biotechadv.2015.08.001
Bannerman, R., Burton, J., and Chen, W. (1983). Traditional Medicine and Health Care Coverage: A Reader for Health Administrators and Practitioners. Geneva: World Health Organization.
Benko-Iseppon, A. M., and Crovella, S. (2010). Ethnobotanical bioprospection of candidates for potential antimicrobial drugs from Brazilian plants: state of art and perspectives. Curr. Protein Pept. Sci. 11, 189–194. doi: 10.2174/138920310791112129
Bourgaud, F., Gravot, A., Milesi, S., and Gontier, E. (2001). Production of plant secondary metabolites: a historical perspective. Plant Sci. 161, 839–851. doi: 10.1016/S0168-9452(01)00490-3
Cámara-Leret, R., Faurby, S., Macía, M. J., Balslev, H., Göldel, B., Svenning, J.-C., et al. (2017). Fundamental species traits explain provisioning services of tropical American palms. Nat. Plants 3:16220. doi: 10.1038/nplants.2016.220
Carvalho, G. H., Cianciaruso, M. V., and Batalha, M. A. (2010). Plantminer: a web tool for checking and gathering plant species taxonomic information. Environ. Model. Softw. 25, 815–816. doi: 10.1016/j.envsoft.2009.11.014
Coley, P. D., Heller, M. V., Aizprua, R., Araúz, B., Flores, N., Correa, M., et al. (2003). Using ecological criteria to design plant collection strategies for drug discovery. Front. Ecol. Environ. 1:421–428. doi: 10.1890/1540-92952003001[0421:UECTDP]2.0.CO;2
Costa, M. A., Palazzo de Mello, J. C., Kaneshima, E. N., Ueda-Nakamura, T., Dias Filho, B. P., Audi, E. A., et al. (2013). Acute and chronic toxicity of an aqueous fraction of the stem bark of Stryphnodendron adstringens (Barbatimão) in rodents. Evid. Based Complement. Alternat. Med. 2013:841580. doi: 10.1155/2013/841580
Cragg, G. M., and Newman, D. J. (2013). Natural products: a continuing source of novel drug leads. Biochim. Biophys. Acta Gen. Subj. 1830, 3670–3695. doi: 10.1016/j.bbagen.2013.02.008
Dias, S. A., Neves, A. E. O., Ferraz, A. D. B. F., Picada, J. N., and Pereira, P. P. (2013). Neuropharmacological and genotoxic evaluation of ethanol extract from Erythrina falcata leaves, a plant used in Brazilian folk medicine. Rev. Bras. Farmacogn. 23, 335–341. doi: 10.1590/S0102-695X2013005000015
Douwes, E., Crouch, N. R., Edwards, T. J., and Mulholland, D. A. (2008). Regression analyses of southern African ethnomedicinal plants: informing the targeted selection of bioprospecting and pharmacological screening subjects. J. Ethnopharmacol. 119, 356–364. doi: 10.1016/j.jep.2008.07.040
Dutra, R. C., Campos, M. M., Santos, A. R., and Calixto, J. B. (2016). Medicinal plants in Brazil: pharmacological studies, drug discovery, challenges and perspectives. Pharmacol. Res. 112, 4–29. doi: 10.1016/j.phrs.2016.01.021
Edris, A. E. (2007). Pharmaceutical and therapeutic potentials of essential oils and their individual volatile constituents: a review. Phytother. Res. 21, 308–323. doi: 10.1002/ptr.2072
Ernst, M., Saslis-Lagoudakis, C. H., Grace, O. M., Nilsson, N., Simonsen, H. T., Horn, J. W., et al. (2016). Evolutionary prediction of medicinal properties in the genus Euphorbia L. Sci. Rep. 6:30531. doi: 10.1038/srep30531
Fabricant, D. S., and Farnsworth, N. R. (2001). The value of plants used in traditional medicine for drug discovery. Environ. Health Perspect. 109(Suppl. 1), 69–75. doi: 10.1289/ehp.01109s169
Flora of Brazil 2020 in Construction (2016). Jardim Botânico do Rio de Janeiro. Available at: http://floradobrasil.jbrj.gov.br/
Gertsch, J. (2009). How scientific is the science in ethnopharmacology? Historical perspectives and epistemological problems. J. Ethnopharmacol. 122, 177–183. doi: 10.1016/j.jep.2009.01.010
Gibbs, R. D. (1974). Chemotaxonomy of Flowering Plants. Vols I-IV. Montreal: McGill-Queen’s University Press. doi: 10.2307/j.ctt1w0ddx8
Grace, O. M., Buerki, S., Symonds, M. R., Forest, F., van Wyk, A. E., Smith, G. F., et al. (2015). Evolutionary history and leaf succulence as explanations for medicinal use in aloes and the global popularity of Aloe vera. BMC Evol. Biol. 15:29. doi: 10.1186/s12862-015-0291-7
Grimbs, A., Shrestha, A., Rezk, A. S., Grimbs, S., Hakeem Said, I., Schepker, H., et al. (2017). Bioactivity in rhododendron: a systemic analysis of antimicrobial and cytotoxic activities and their phylogenetic and phytochemical origins. Front. Plant Sci. 8:551. doi: 10.3389/fpls.2017.00551
Gurib-Fakim, A. (2006). Medicinal plants: traditions of yesterday and drugs of tomorrow. Mol. Aspects Med. 27, 1–93. doi: 10.1016/j.mam.2005.07.008
Halse-Gramkow, M., Ernst, M., Rønsted, N., Dunn, R. R., and Haris Saslis-Lagoudakis, C. (2016). Using evolutionary tools to search for novel psychoactive plants. Plant Genet. Resourc. 14, 246–255. doi: 10.1017/S1479262116000344
Heil, M. (2008). Indirect defence via tritrophic interactions. New Phytol. 178, 41–61. doi: 10.1111/j.1469-8137.2007.02330.x
Huang, M., Sanchez-Moreiras, A. M., Abel, C., Sohrabi, R., Lee, S., Gershenzon, J., et al. (2012). The major volatile organic compound emitted from Arabidopsis thaliana flowers, the sesquiterpene (E)-β-caryophyllene, is a defense against a bacterial pathogen. New Phytol. 193, 997–1008. doi: 10.1111/j.1469-8137.2011.04001.x.
Jäger, A. K. (2015). “Medicinal plant research: a reflection on translational tasks,” in Ethnopharmacology, eds M. Heinrich and A. K. Jager (Hoboken, NJ: Wiley-Blackwell), 11–16.
Kembel, S. W., Cowan, P. D., Helmus, M. R., Cornwell, W. K., Morlon, H., Ackerly, D. D., et al. (2010). Picante: R tools for integrating phylogenies and ecology. Bioinformatics 26, 1463–1464. doi: 10.1093/bioinformatics/btq166
Knutsen, H. K., Alexander, J., Barregård, L., Bignami, M., Brüschweiler, B., Ceccatelli, S., et al. (2017). Risks for human health related to the presence of pyrrolizidine alkaloids in honey, tea, herbal infusions and food supplements. EFSA J. 15:4908. doi: 10.2903/j.efsa.2017.4908
Koehn, F. E., and Carter, G. T. (2005). The evolving role of natural products in drug discovery. Nat. Rev. Drug Discov. 4, 206–220. doi: 10.1038/nrd1657
Kusuma, S. S., Tanneeru, K., Didla, S., Devendra, B. N., and Kiranmayi, P. (2014). Antineoplastic activity of monocrotaline against hepatocellular carcinoma. Anticancer Agents Med. Chem. 14, 1237–1248. doi: 10.2174/1871520614666140715085907
Legume Phylogeny Working Group [LPWG] (2013). Legume phylogeny and classification in the 21st century: progress, prospects and lessons for other species-rich clades. Taxon 62, 217–248. doi: 10.12705/622.8
Leonti, M., Stafford, G. I., Cero, M. D., Cabras, S., Castellanos, M. E., Casu, L., et al. (2017). Reverse ethnopharmacology and drug discovery. J. Ethnopharmacol. 198, 417–431. doi: 10.1016/j.jep.2016.12.044
Letunic, I., and Bork, P. (2016). Interactive tree of life (iTOL) v3: an online tool for the display and annotation of phylogenetic and other trees. Nucleic Acids Res. 44, W242–W245. doi: 10.1093/nar/gkw290
Lewis, G., Schrire, B., Mackinder, B., and Lock, M. (eds). (2005). Legumes of the World. Kew: Royal Botanic Gardens.
Mazel, F., Davies, T. J., Gallien, L., Renaud, J., Groussin, M., Münkemüller, T., et al. (2016). Influence of tree shape and evolutionary time-scale on phylogenetic diversity metrics. Ecography 39, 913–920. doi: 10.1111/ecog.01694
Mazzari, A. L., and Prieto, J. M. (2014). Herbal medicines in Brazil: pharmacokinetic profile and potential herb-drug interactions. Front. Pharmacol. 5:162. doi: 10.3389/fphar.2014.00162
Moerman, D. E. (1991). The medicinal analysis flora of native north America: an analysis. J. Ethnopharmacol. 31, 1–42. doi: 10.1016/0378-8741(91)90141-Y
Moerman, D. E., Pemberton, R. W., Kiefer, D., and Berlin, B. (1999). A comparative analysis of five medicinal floras. J. Ethnobiol. 19, 49–67.
Newman, D. J., Cragg, G. M., and Snader, K. M. (2000). The influence of natural products upon drug discovery. Nat. Prod. Rep. 17, 215–234. doi: 10.1039/a902202c
Nwaka, S., and Hudson, A. (2006). Innovative lead discovery strategies for tropical diseases. Nat. Rev. Drug Discov. 5, 941–955. doi: 10.1038/nrd2144
Paradis, E., Claude, J., and Strimmer, K. (2004). APE: analyses of Phylogenetics and evolution in R language. Bioinformatics 20, 289–290. doi: 10.1093/bioinformatics/btg412
Pearse, W. D., Purvis, A., Cavender-Bares, J., and Helmus, M. R. (2014). “Metrics and models of community phylogenetics,” in Modern Phylogenetic Comparative Methods and their Application in Evolutionary Biology, ed. L. Garamszegi (Berlin: Springer), 451–464.
Revell, L. J. (2012). phytools: an R package for phylogenetic comparative biology (and other things). Methods Ecol. Evol. 3, 217–223. doi: 10.1111/j.2041-210X.2011.00169.x
Rønsted, N., Savolainen, V., Mølgaard, P., and Jäger, A. K. (2008). Phylogenetic selection of Narcissus species for drug discovery. Biochem. Syst. Ecol. 36, 417–422. doi: 10.1016/j.bse.2007.12.010
Saslis-Lagoudakis, C. H., Hawkins, J. A., Greenhill, S. J., Pendry, C. A., Watson, M. F., Tuladhar-douglas, W., et al. (2014). The evolution of traditional knowledge?: environment shapes medicinal plant use in Nepal The evolution of traditional knowledge?: environment shapes medicinal plant use in Nepal. Proced. R. Soc. B 281:20132768. doi: 10.1098/rspb.2013.2768
Saslis-Lagoudakis, C. H., Klitgaard, B. B., Forest, F., Francis, L., Savolainen, V., Williamson, E. M., et al. (2011). The use of phylogeny to interpret cross-cultural patterns in plant use and guide medicinal plant discovery: an example from Pterocarpus (Leguminosae). PLoS One 6:e22275. doi: 10.1371/journal.pone.0022275
Saslis-Lagoudakis, C. H., Savolainen, V., Williamson, E. M., Forest, F., Wagstaff, S. J., Baral, S. R., et al. (2012). Phylogenies reveal predictive power of traditional medicine in bioprospecting. Proc. Natl. Acad. Sci. U.S.A. 109, 15835–15840. doi: 10.1073/pnas.1202242109
Ministério da Saude (2009). Relação Nacional de Plantas Medicinais de Interesse ao SUS (RENISUS). Brazil: Ministério da Saude.
Skirycz, A., Kierszniowska, S., Méret, M., Willmitzer, L., and Tzotzos, G. (2016). Medicinal bioprospecting of the amazon rainforest: a modern Eldorado? Trends Biotechnol. 34, 781–790. doi: 10.1016/j.tibtech.2016.03.006
Souza, E. N. F., and Hawkins, J. A. (2017). Comparison of herbarium label data and published medicinal use: herbaria as an underutilized source of ethnobotanical information. Econ. Bot. 71, 1–12. doi: 10.1007/s12231-017-9367-1
Stamatakis, A. (2014). RAxML version 8: a tool for phylogenetic analysis and post-analysis of large phylogenies. Bioinformatics 30, 1312–1313. doi: 10.1093/bioinformatics/btu033
Sucher, N. J. (2013). The application of Chinese medicine to novel drug discovery. Expert Opin. Drug Discov. 8, 21–34. doi: 10.1517/17460441.2013.739602
Tan, G., Gyllenhaal, C., and Soejarto, D. (2006). Biodiversity as a source of anticancer drugs. Curr. Drug Targets 7, 265–277. doi: 10.2174/138945006776054942
Verpoorte, R. (1998). Exploration of nature’s chemodiversity: the role of secondary metabolites as leads in drug development. Drug Discov. Today 3, 232–238. doi: 10.1016/S1359-6446(97)01167-7
Verpoorte, R. (2000). Pharmacognosy in the new millennium: leadfinding and biotechnology. J. Pharm. Pharmacol. 52, 253–262. doi: 10.1211/0022357001773931
Webb, C. O. (2000). Exploring the phylogenetic structure of ecological communities: an example for rain forest trees. Am. Nat. 156, 145–155. doi: 10.1086/303378
Webb, C. O., Ackerly, D. D., McPeek, M. A., and Donoghue, M. J. (2002). Phylogenies and community ecology. Annu. Rev. Ecol. Syst. 33, 475–505. doi: 10.1146/annurev.ecolsys.33.010802.150448
Wink, M. (2003). Evolution of secondary metabolites from an ecological and molecular phylogenetic perspective. Phytochemistry 64, 3–19. doi: 10.1016/S0031-9422(03)00300-5
Wink, M. (2013). Evolution of secondary metabolites in legumes (Fabaceae). S. Afr. J. Bot. 89, 164–175. doi: 10.1016/j.sajb.2013.06.006
Yessoufou, K., Daru, B. H., and Muasya, A. M. (2015). Phylogenetic exploration of commonly used medicinal plants in South Africa. Mol. Ecol. Resourc. 15, 405–413. doi: 10.1111/1755-0998.12310
Keywords: ethnopharmacology, Brazil, bioprospecting, leguminosae, traditional use, ethnobotany
Citation: Souza ENF, Williamson EM and Hawkins JA (2018) Which Plants Used in Ethnomedicine Are Characterized? Phylogenetic Patterns in Traditional Use Related to Research Effort. Front. Plant Sci. 9:834. doi: 10.3389/fpls.2018.00834
Received: 28 December 2017; Accepted: 29 May 2018;
Published: 20 June 2018.
Edited by:
Wanchai De-Eknamkul, Chulalongkorn University, ThailandReviewed by:
Guilherme Corrêa De Oliveira, Instituto Tecnológico Vale, BrazilGary Ivan Stafford, University of Pretoria, South Africa
Copyright © 2018 Souza, Williamson and Hawkins. This is an open-access article distributed under the terms of the Creative Commons Attribution License (CC BY). The use, distribution or reproduction in other forums is permitted, provided the original author(s) and the copyright owner are credited and that the original publication in this journal is cited, in accordance with accepted academic practice. No use, distribution or reproduction is permitted which does not comply with these terms.
*Correspondence: Estevão N. F. Souza, estevaonfsouza@gmail.com