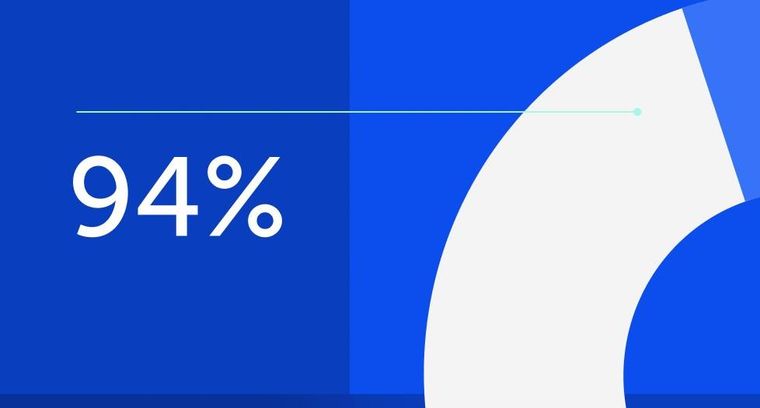
94% of researchers rate our articles as excellent or good
Learn more about the work of our research integrity team to safeguard the quality of each article we publish.
Find out more
ORIGINAL RESEARCH article
Front. Plant Sci., 05 June 2018
Sec. Plant Physiology
Volume 9 - 2018 | https://doi.org/10.3389/fpls.2018.00742
In dioecious woody plants, females often make a greater reproductive effort than male individuals at the cost of lower growth rate. We hypothesized that a greater reproductive effort of female compared with male Taxus baccata individuals would be associated with lower female photochemical capacity and higher activity of antioxidant enzymes. Differences between the genders would change seasonally and would be more remarkable under nutrient deficiency. Electron transport rate (ETRmax), saturation photosynthetic photon flux corresponding to maximum electron transport rate (PPFsat), quantum yield of PSII photochemistry at PPFsat (ΦPPFsat), and chlorophyll a fluorescence and activity of antioxidant enzymes were determined in needles of T. baccata female and male individuals growing in the experiment with or without fertilization. The effects of seasonal changes and fertilization treatment on photochemical parameters, photosynthetic pigments concentration, and antioxidant enzymes were more pronounced than the effects of between-sexes differences in reproductive efforts. Results showed that photosynthetic capacity expressed as ETRmax and ΦPPFsat and photosynthetic pigments concentrations decreased and non-photochemical quenching of fluorescence (NPQ) increased under nutrient deficiency. Fertilized individuals were less sensitive to photoinhibition than non-fertilized ones. T. baccata female and male individuals did not differ in photochemical capacity, but females showed higher maximum quantum yield of PSII photochemistry (Fv/Fm) than males. The activity of guaiacol peroxidase (POX) was also higher in female than in male needles. We concluded that larger T. baccata female reproductive effort compared with males was not at the cost of photochemical capacity, but to some extent it could be due to between-sexes differences in ability to protect the photosynthetic apparatus against photoinhibition with antioxidants.
The main advantages of dioecious species over hermaphroditic are complete exclusion of the risk of self-pollination (Darwin, 1892; Charlesworth and Charlesworth, 1978) and optimization of resource allocation in both male and female functions (Maynard-Smith, 1978; Charnov, 1982). However, this mating system is relatively rare; only 6% of angiosperms are dioecious (Renner and Ricklefs, 1995; Renner, 2014). One of the reasons for the small number of dioecious species is reduced seed dispersal because only half of individuals (females) produces diaspores (Heilbuth et al., 2001). Moreover, females usually invest in greater reproductive effort over a longer period compared to males (Garbarino et al., 2015; Vessella et al., 2015; Matsushita et al., 2016). In woody dioecious species this greater investment by females in reproduction effort is often linked to lower growth rates than in males supporting the costs of reproduction hypothesis (e.g., Obeso, 2002; Leigh et al., 2006). Sexual differences in reproductive effort, stress response or different environment requirements may cause dioecious species to be more sensitive to environmental changes (Rozas et al., 2009; Chen et al., 2014). Sex-related differences frequently arise or are greater under stressful conditions (such as drought and thermal stress), indicating a greater sensitivity of females to poorer habitat conditions (Zhang et al., 2010; Iszkuło et al., 2011b; Zhao et al., 2011; DeSoto et al., 2016). Females have higher reproductive effort and are adapted to higher-resource microsites compared with males (Vessella et al., 2015). A consequence is that environmental stress caused by less-than-optimal light, nutrition, or water conditions often favors maleness (reviewed by Korpelainen, 2007).
Females often have a greater intensity of gas exchange in comparison to males (Dawson and Ehleringer, 1993; Obeso, 2002; Montesinos et al., 2012) and increase photosynthesis by increasing leaf area (Wallace and Rundel, 1979; Meagher, 1992; Kohorn, 1994). Nevertheless, this is not clear whether between-sexes differences in net CO2 assimilation rates result from dark photosynthetic processes or photochemistry. We suggest, therefore, that differences in photochemical capacity between sexes would be related to their reproductive efforts, in agreement with the optimal resource use hypothesis of Bloom et al. (1985). However, the cost-benefit balance of sex-related differences in photosynthesis and photoprotection in leaves of dioecious plants has been poorly studied to date (Juvany et al., 2014). Previous studies indicate that males and females might not differ in maximum quantum yield of photosystem II (PSII) photochemistry (Fv/Fm) but males had enhanced photoprotection. Nevertheless, differences in photochemical efficiency and capacity between males and females under nutrient deficiency are not fully understood (Juvany et al., 2014). Photosynthesis is the main source of damaging reactive oxygen species (ROS) (Foyer and Shigeoka, 2011). Therefore, enzymatic and non-enzymatic antioxidant systems are required to maintain intracellular ROS pools at low levels (Ratajczak et al., 2015) and mitigate potentially harmful reactions caused by enhanced oxidative load (Foyer and Shigeoka, 2011). Enzymatic antioxidant systems in photosynthetic processes involve the action of superoxide dismutase, peroxidases, catalases, and reductases (Ding et al., 2016). Superoxide dismutase (SOD) is involved in the detoxification of superoxide anion () resulting in molecular oxygen (O2) or the formation of hydrogen peroxide (H2O2). The latter is subsequently reduced by catalase and peroxidases to water with the generation of monodehydroascorbate. These antioxidants not only have specific protective functions in photosynthesis, but are also associated with redox signaling during this process (Foyer and Noctor, 2011; Benzarti et al., 2012). It is expected that female plants would show higher levels of antioxidants because of their higher metabolic activity, in particular, their higher activity of photosynthesis. The metabolic rate increases steeply in relation to reproductive effort in females. This increase in metabolism results in higher production of ROS, which may initiate oxidative stress (Zinta et al., 2016). Therefore, a highly active antioxidant system is necessary to maintain homeostasis in the cells (Foyer and Shigeoka, 2011). Reduction of oxidative stress may underlie the trade-off between reproduction and survival of females.
Taxus baccata is a suitable model for studying dioecious species because males and females are known to respond differently to environmental conditions. Males of T. baccata grow taller (Iszkuło et al., 2009), show greater radial growth than females after the beginning of sexual maturity (Cedro and Iszkuło, 2011; Iszkuło et al., 2011a), and females have lower N concentration in needles, especially when undergoing intensive shoot elongation and radial growth (Nowak-Dyjeta et al., 2017). T. baccata is threatened by extinction due to its low tolerance of a range of environmental stresses and its intensive exploitation in the past (Thomas and Polwart, 2003; Iszkuło et al., 2016; Kýpetová et al., 2018).
Our overarching hypothesis was that greater reproductive effort of T. baccata females compared with males would be reflected in their (1) lower photosynthetic capacity presented by lower quantum yield of PSII photochemistry at the saturation level of PPF (ΦPPFsat), lower Fv/Fm and lower apparent maximum electron transport rate (ETRmax), (2) higher energy losses as heat assessed with non-photochemical quenching of fluorescence (NPQ), and (3) higher activity of antioxidant enzymes. An additional hypothesis was that fertilization effects on photochemistry and antioxidant activity would be more pronounced than differences between sexes. Moreover, we suggest that the differences between male and female individuals would be reduced under higher nutrient availability.
The experiment was conducted at the Institute of Dendrology, Polish Academy of Sciences in Kornik, Poland. Rooted shoots of T. baccata were used in the experiment. In 2012, fifty shoots were collected and rooted from 20 trees (10 males and 10 females) of T. baccata growing in the Kornik Arboretum (two genders x 10 individuals x 50 shoots = 1,000 plants). Cuttings of similar size were taken from the middle part of each crown, growing in similar, partially-shaded light conditions. Individuals were grown in 5-liter pots under 2-m-high scaffolding with shading net to produce a 50% reduction in full sunlight. The degree of light reduction was confirmed by measurements of relative photosynthetic photon flux density using a line quantum sensor (Apogee Inc.) following the methods of Messier and Puttonen (1995). The soil for the pots was obtained from a natural broadleaved forest, similar to the typical habitat of the study species. Soil (10% of the soil volume) was added from a stand of T. baccata to ensure natural mycorrhizal inoculation. In March 2013, seedlings were randomly divided into two blocks containing both genders, and then within each block two fertilization treatments were established. The fertilized group of seedlings received 6 g per liter of Osmocote Exact 5-6 M (ICL, Israel) in March 2014 and 2015, whereas non-fertilized seedlings were grown without any fertilizer. The fertilizer contained 15% N, 9% P, 12% K, 2.5% MgO, and microelements. Needles were sampled over the two following years every 4 months: (1) in March, at the end of the flowering period before the beginning of growth; (2) in June when mass allocation to aboveground organs reaches a maximum, at the end of height growth; (3) in September, at the end of the mass allocation to roots and other parts of a plant but while females are investing resources in maturing seeds and arils; (4) in December, when plants are dormant. At each sampling point, 1-year needles from 24 seedlings were used for all analysis (3 seedlings x 2 fertilization treatments x 2 genders x 2 blocks). Needles were taken from the same shoot from the top of individuals for all analyses.
During the experiment, air temperature and relative air humidity were recorded each hour using four EL-USB-2+ data loggers (EasyLog, Inc.). Monthly mean (Tmean), monthly minimum (Tmin), and maximum (Tmax) values of air temperatures and monthly mean air relative humidity (RH) over the sampling period are shown in Table 1. The difference between the highest and lowest values of Tmean in June 2014 and December 2015 was 20.3°C. During the experiment, Tmax was in June 2015 and Tmin was in December 2015 (Tmax−Tmin = 58°C). Mean air relative humidity was highest in January 2015 and it attained the minimum in June 2014 (RHmax−RHmin = 33.9%).
Chlorophyll a fluorescence was measured in needles using a Fluorescence Monitoring System (FMS 2, Hansatech, Norfolk, UK) operating in an online mode. Collected 1-year needles were wrapped in moist paper, enclosed in Eppendorf tubes, and brought to the laboratory. Prior to fluorescence measurements, needles were dark adapted for 30 min at air temperature around 22°C (21–23°C) in the laboratory, arranged tightly side-by-side, stuck on self-adhesive transparent tape to fill the entire aperture of the factory-provided clip. The mean air temperature of fluorescence measurements was 22°C (21–24°C, min.-max.), monitored using a thermocouple installed in the leaf clip. Using a light-tight chamber containing a light source inserted onto the leaf clip, the needles were exposed to modulated light at 0.05 μmol quanta m−2 s−1. After reading minimum fluorescence F0, a saturating 0.7 s pulse of light (PPF = 15.3 mmol m−2 s−1) was delivered to induce a maximum fluorescence (Fm). Maximum quantum yield of PSII photochemistry was calculated as Fv/Fm, where Fv = Fm-F0.
Subsequently, to generate light response curves of PSII quantum yield (ΦPSII) needles in the clip were illuminated with actinic light using an inbuilt halogen lamp. The intensity of actinic light corresponding to values indicated by the software was measured prior to the experiment using a light sensor inserted in the leaf-clip in the position of the needles. Up to 12 levels of actinic light of increasing intensity were used, and for each level, after a stable steady state fluorescence (Fs) was reached, 0.7 s saturating pulse was delivered and maximum light-adapted fluorescence (F'm) was determined. Quantum yield of PSII was calculated as: ΦPSII = (F'm–Fs)/F'm (Genty et al., 1989). At each actinic light level, non-photochemical quenching of fluorescence (NPQ) was calculated as: NPQ = (Fm−F'm)/F'm (Maxwell and Johnson, 2000). The course of fluorescence and all the measured parameters were constantly monitored to ensure stable Fs values after changing actinic illumination levels before a saturating pulse was applied. Usually, stabilization time of Fs took 1.5–3.5 min.
For each light level, the apparent rates of photosynthetic electron transport (ETR) were calculated as: ETR = α * ΦPSII * PPF*0.5 (α—needle absorptance, Maxwell and Johnson, 2000). Assumptions were made that the excitation energy is partitioned equally between the two photosystems (hence the factor of 0.5; Maxwell and Johnson, 2000). Leaf absorptance may differ among plants depending on species and adaptation to microclimate conditions. In our study, it was calculated using the model by Evans (1993), which is based on total chlorophyll content in leaf.
The maximum apparent rate of photosynthetic electron transport of PSII (ETRmax) and the saturation level of photosynthetic photon flux density (PPFsat) in Equation (1) were derived by fitting the functions of Ye et al. (2013):
where α is the initial slope, β is the extent of dynamic down-regulation of PSII, and γ is defined as a saturation term of light response curve for photosynthetic electron transport rate (ETR-PPF), PPF is photosynthetic photon flux.
PPFsat is calculated from the Equation (2):
ETRmax has been defined as ETR at PPFsat and is derived from the Equation (3):
To estimate quantum yield of PSII photochemistry at saturating PPF (ΦPPFsat), the exponential rise to maximum function was fitted to light curves of ΦPSII (Rascher et al., 2000; Robakowski, 2005) as in Equation (4).
where a, b, c, d, m are independent parameters.
The PPF vs. NPQ curves were fitted with the exponential function in the Equation (5):
Light curves of NPQ are considered to rise to infinity (Maxwell and Johnson, 2000). Therefore, NPQ was calculated at the arbitrary value of 345 μmol m−2 s−1 of actinic light (NPQ345) and used in estimating the effects of time, fertilization, and sex on energy losses as heat. Light curves of ΦPSII, ETR, and NPQ were fitted with the above functions to derive all parameters using the non-linear estimation of Levenberg-Marquardt in Statistica 13.1 (Tulsa, USA).
The one-year needles (40–50 mg of fresh weight) were used for spectrophotometric analyses of chlorophyll and total carotenoids contents. They were cut into 2 mm pieces and incubated in 5 ml of 100% dimethylsulfoxide (DMSO) saturated with CaCO3 to avoid pheophytization, in a water bath at 60°C until the solution became translucent (~5 h). The absorbance of the extract was measured at 665, 648, and 470 nm. Chlorophyll a, b, and total carotenoids contents were calculated using the formulae given by Barnes et al. (1992).
The whole one-year needles were used for analysis of all enzymes. All extraction procedures were conducted at 4°C. Samples were ground in liquid nitrogen and homogenized in 50 mM sodium phosphate buffer, pH 7.0, containing 0.2 mM EDTA, and 20% polyvinylpolypyrrolidone (PVPP) and were incubated for 1 h in the cold. The homogenates were filtered through two layers of cheesecloth and centrifuged at 4°C at 20,000 g for 20 min. The supernatant was desalted on a Sephadex G25 (Sigma-Aldrich) standard column according to Helmerhorst and Stokes (1980). Analysis of enzyme activity was performed in the cytosolic fraction.
The superoxide dismutase (SOD, EC. 1.15.11) activity was determined using the method of Giannopolitis and Ries (1977) by measuring its ability to inhibit the photochemical reduction of 4-nitro blue tetrazolium chloride (NBT), as described in Pukacka and Pukacki (2000). One unit (1U) of SOD activity was defined as the amount of enzyme required to cause 50% inhibition of the rate of NBT reduction (U mg−1 protein). The 3 ml reaction mixture contained 50 mM phosphate buffer (pH 7.8), 1.3 mM riboflavin, 0.1 mM dithiothreitol (DTT), 63 mM NBT, and 50 μl enzyme extract. The mixture was illuminated in glass test tubes. A non-irradiated reaction mixture served as a control.
Guaiacol peroxidase and catalase were determined according to Chance and Maehly (1955). Total guaiacol peroxidase (POX, EC. 1.11.1.7) activity was measured by the oxidation of guaiacol at 470 nm (ε = 26.6 mM−1 cm−1). The reaction mixture contained 1 ml of 100 mM potassium phosphate buffer at pH 7.0, 1 ml of 0.089 mM guaiacol, 1 ml of 200 mM H2O2, and 50 μl of enzyme extract. The reaction was initiated by adding H2O2. Controls were made for the background absorbance at 470 nm, without H2O2 (one control) and without guaiacol (second control). The reaction was initiated by adding H2O2. POX activity was expressed as nkat min−1 mg. protein−1.
For catalase (CAT, EC 1.11.1.6) activity, the reaction mixture contained 1 ml of 0.1 M phosphate buffer pH 7.0, 1 ml of 30 mM H2O2, and 50 μl of enzyme extract. The catalase activity was determined by the decrease in absorbance at 240 nm. The reaction was initiated by adding H2O2. Controls were made for the background absorbance at 240 nm, without H2O2 (one control) and without enzyme extract (second control). CAT activity was expressed as mmol H2O2 min−1 mg. protein−1.
Cytosolic ascorbate peroxidase (APX, EC.1.11.1.11) activity was measured by following the decrease in absorbance at 290 nm due to ascorbic acid (ASA) oxidation for 5–10 min. according to Nakano and Asada (1981). The reaction mixture contained: 1 ml of 0.68 mM ASA, 0.1 mM EDTA in 0.1 M phosphate buffer at pH 7.0, 1 ml of 4 mM H2O2, and 50 μl of the enzyme extract. The reaction was initiated by adding H2O2. A control was made for the low, non-enzymatic oxidation of ASA by H2O2. APX activity was expressed as nmol ASA min−1 mg. protein−1.
The protein content of crude enzyme extracts was estimated according to Bradford (1976), using bovine serum albumin as a standard.
Prior to analyses, all data were tested for normality using the Shapiro-Wilk's test and homogeneity of variance with the Levene's test. Results were analyzed with the full-factorial three way analysis of variance with gender, fertilization group, and time of sampling as the sources of fixed effects and with blocks as the source of random effect. When interactions occurred, Student t-test was applied to test differences between means. Means were considered to differ statistically at P < 0.05. Data were presented as means with standard errors (SE). All analyses were done using JMP 12 software (SAS Institute Inc., Cary, NC, 1989-2007). Figures were prepared with SigmaPlot v. 14 (Systat Software, Inc., San Jose California USA).
Curves of ΦPSII and ETR vs. PPF in needles of fertilized T. baccata individuals run above the curves in needles of non-fertilized individuals except for the initial part of the curves at low PPF (Figures 1A,B). Significant differences in mean values of ΦPSII, ETR, and NPQ between fertilization treatments were observed beginning from 208 μmol m−2 s−1 of fluorescence induction light. The photosynthetic capacity of non-fertilized plants estimated by the mean value of ETRmax was about 30% lower compared with fertilized ones. Non-fertilized individuals lost more needle absorbed energy as heat reflecting the higher NPQ values in non-fertilized individuals except for when NPQ was low (up to 208 μmol m−2 s−1) and high (above 800 μmol m−2 s−1) PPF of induction light (Figure 1C). There were not significant differences between the sexes in ΦPSII, ETR, and NPQ vs. PPF.
Figure 1. Light response curves of needles of T. baccata individuals growing with (brown solid line) or without fertilization (dark blue dotted line): (A) Photosystem II (PSII) quantum yield (ΦPSII), (B) Apparent electron transfer rate (ETR), and (C) Non-photochemical quenching of fluorescence (NPQ) vs. photosynthetic photon flux (PPF). The fluorescence measurements were conducted in June 2015. The equation used for non-linear fitting together were as follows: (A) , (B) (C) NPQ = m+a(1−e(−bPPF)). The values of adjusted coefficients of determination and probability were described by ≥ 0.98 with P < 0.001 for all used equations. Data are means with standard errors (SE, n = 12). Asterisks indicate statistical differences between means at *0.05 > P ≥ 0.01, **0.01 > P ≥ 0.001, P < 0.001***.
All parameters based on chlorophyll a fluorescence underwent significant seasonal changes and differed significantly between fertilization treatments (Table 2, Supplementary file—Table 1). Generally, fertilized individuals had higher values of ETRmax, ΦPPFsat, PPTsat, and Fv/Fm (Figures 2A–D) and lower values of NPQ345 (Figure 2E) compared with non-fertilized individuals. The mean values±SE of ETRmax for fertilized and non-fertilized individuals were 121 ± 3 and 83 ± 2 μmol m−2 s−1, respectively. The differences in this parameter between fertilization treatments were significant before the appearance of the secondary sex characteristics. The mean values±SE of ETRmax for female and male individuals were 101 ± 3 and 102 ± 3 μmol m−2 s−1, respectively. Value of ΦPPFsat was increased by fertilization (0.250 ± 0.10) compared with non-fertilized plants (0.199 ± 0.10) (Table 2; Figures 2A,B). Significant interaction between the sampling date (time) and fertilization treatment indicates that the differences between the fertilization treatments were modified by seasonal changes of ETRmax, ΦPPFsat, and NPQ345 (Figure 2). Interestingly, females had the significantly higher values of Fv/Fm compared with males (Table 2, Figure 3A). Fv/Fm increased from September 2013 to the first appearance of cones in September 2014. Then, this parameter stabilized at the higher in female and lower level in male needles (Figure 3A). In September 2013, Fv/Fm attained the lowest value of 0.747 ± 0.012 in needles of non-fertilized male individuals, and in September 2014 the highest value of 0.862 ± 0.003 in needles of fertilized female plants. The effect of time and the interactions: time x fertilization and time x sex were significant indicating that seasonal changes modified effects of the experimental treatments (Table 2). Significant differences between the sexes within the same date of fluorescence measurements were found in September 2013, December 2014, and in March 2015 (Figure 3A). Fv/Fm < 0.8 indicating photoinhibition or PSII down-regulation was more often observed in non-fertilized males compared to females. When data from the whole experiment were pooled, Fv/Fm of non-fertilized males was 0.788 ± 0.006, non-fertilized females 0.813 ± 0.004, fertilized males 0.804 ± 0.003, and fertilized females 0.827 ± 0.004.
Table 2. ANOVA results for chlorophyll a fluorescence light curves: ETRmax, apparent maximum electron transport rate; ΦPPFsat, quantum yield of PSII photochemistry at the saturation value of photosynthetic photon flux; Fv/Fm, maximum quantum yield of PSII photochemistry; NPQ345, non-photochemical quenching of fluorescence at PPF = 345 μmol m−2 s−1; PPFsat, saturation photosynthetic photon flux corresponding to maximum electron transport rate.
Figure 2. Changes in time in photosynthetic parameters in needles of T. baccata individuals growing with (brown solid line) or without fertilization (dark blue dotted line): (A) Apparent maximum electron transport rate (ETRmax); (B) Quantum yield of PSII photochemistry at the saturation value of photosynthetic photon flux (ΦPPFsat); (C) Maximum quantum yield of PSII photochemistry (Fv/Fm); (D) Saturation photosynthetic photon flux corresponding to maximum electron transport rate (PPFsat); and (E) Non-photochemical quenching of fluorescence at PPF = 345 μmol m−2 s−1 (NPQ345). Data are means with standard errors (SE, n = 12). Asterisks indicate statistical differences between means at P < 0.05.
Figure 3. Changes with time in photochemistry in needles of T. baccata male (black solid line) and female (red dotted line) individuals: (A) Maximum quantum yield of PSII photochemistry (Fv/Fm); (B) Chlorophyll a/b ratio; (C) Needle absorptance (α); (D) ascorbate peroxidase (1 nmol ASA min−1 mg. protein−1, APX); and (E) guaiacol peroxidase (nkat min−1 mg. protein−1, POX). Data are means with standard errors (SE, n = 12). Asterisks indicate statistical differences between means with P < 0.05.
A significant decrease in LMA was detected in January 2015 in both fertilized and non-fertilized individuals (Figure 4A). Fertilized plants had higher LMA (198.5 ± 5.3 g m−2) than non-fertilized ones (183.7 ± 4.3). Total needle chlorophyll concentration changed over time (Figure 4B). The lowest values were observed in March 2014 (4.3 ± 0.3 mg g−1), the highest in September (10.8 ± 0.4). In fertilized plants total chlorophyll concentration was on average 9.1 ± 0.3 and in non-fertilized ones 7.6 ± 0.3 (Table 3). The trend of seasonal changes in carotenoids concentration was similar to that of total chlorophyll concentration (Figure 4D). There were higher carotenoids concentration in fertilized (1.81 ± 0.04 mg g−1) compared with non-fertilized individuals (1.59 ± 0.05) (Table 3, Figure 4D). The time-course of needle absorptance (α) increased till September 2014 and after a remarkable depletion in January 2015 it was stable except for a small decline in non-fertilized individuals in September 2015 (Figure 4E). Plants differed significantly between fertilization treatments showing higher α in needles of fertilized (0.960 ± 0.002) compared with non-fertilized ones (0.947 ± 0.002). There was also a significant interaction between time and fertilization in LMA, needle absorptance, and photosynthetic pigments concentrations (including chlorophyll a/b ratio; Table 3). Fertilized individuals generally had higher values of analyzed parameters than non-fertilized ones (Figure 4).
Figure 4. Changes in time of leaf parameters in needles of T. baccata individuals growing with (brown solid line) or without fertilization (dark blue dotted line): (A) Leaf mass-to-area ratio (LMA); (B) Total chlorophyll concentration (mg g−1); (C) Chlorophyll a/b ratio; (D) Carotenoids concentration (mg g−1); and (E) Needle absorptance. Data are means with standard errors (SE, n = 12). Asterisks indicate statistical differences between means with P < 0.05.
Table 3. ANOVA results for leaf mass-to-area ratio (LMA, g m−2), total chlorophyll concentration (mg g−1), chlorophyll a to b ratio, carotenoids (mg g−1), needle absorptance (α).
No significant differences between sexes were found in the variables measured (Table 3, Figure 4), although there was a significant interaction between sex and time for chlorophyll a/b ratio and needle absorptance (Table 3, Figures 3B,C).
Superoxidase dismutase (SOD) activity was neither influenced by fertilization nor by sex, however underwent significant changes over time (Table 4, Figure 5). The mean values of SOD for fertilized and non-fertilized individuals were 96.9 ± 5.8 U mg protein−1 and 96.3 ± 5.1 and for male and female individuals 101.7 ± 6.1 and 91.6 ± 4.7, respectively. The activity of catalase (CAT) and ascorbate peroxidase (APX) changed significantly with time and were generally higher in the fertilized (67.1 ± 3.2 mmol H2O2 min−1 mg. protein−1 and 234.1 ± 11.4 nmol ASA min−1 mg. protein−1) compared to non-fertilized plants (57.7 ± 2.6 and 177.1 ± 10.9) except for September 2015 when non-fertilized plants had the higher level of activity of both enzymes (Table 4, Figure 5). There was a significant interaction between time and fertilization in the case of APX but not between time and sex (Table 4, Figure 3D). APX activities increased up to March 2015 and abruptly decreased in September 2015 (Figure 3D). Guaiacol peroxidase (POX) activities were influenced by sex and were higher in females (540.7 ± 30.7 nkat min−1 mg. protein−1) compared with males (451.8 ± 22.8) except for June 2014 and March 2015 (Figure 3E, Supplementary file—Table 1).
Table 4. ANOVA results for total enzyme activities of: CAT, catalase; APX, ascorbate peroxidase; POX, guaiacol peroxidase; SOD, superoxide dismutase.
Figure 5. Changes with time in antioxidant systems in needles of T. baccata individuals growing with (brown solid line) or without fertilization (dark blue dotted line) in total activities of: (A) superoxide dismutase (U mg. protein−1, SOD); (B) catalase (1 mmol H2O2 min−1 mg. protein−1, CAT); (C) ascorbate peroxidase (1 nmol ASA min−1 mg. protein−1, APX); and (D) guaiacol peroxidase (nkat min−1 mg. protein−1, POX). Data are means with standard errors (SE, n = 12). Asterisks indicates statistical differences between means with P < 0.05.
The results of our study show that the effects of fertilization treatment and seasonal changes on photochemical parameters, photosynthetic pigments' concentrations, and antioxidant enzymes activity overrode between-sexes differences in reproductive efforts except for Fv/Fm and guaiacol peroxidase activity (POX). Additionally, the significant interaction between time and sex effects indicates that an effect of sex on the chlorophyll a/b ratio and needle absorptance was modified by seasonal changes. Therefore, we conclude that the greater reproductive efforts of female T. baccata individuals were not at the cost of their photosynthetic capacity expressed as ETRmax and ΦPPFsat. Our results are partially consistent with those of Mitchell (1998) who found that T. baccata females and males did not differ in photosynthetic leaf traits. Moreover, they are in agreement with the photosynthetic responses of Silene latifolia genders growing under low levels of light, water, and nutrients (Gehring and Monson, 1994). However, our findings are not consistent with earlier results of other authors who indicate that females show greater reproductive efforts than males at the expense of photosynthetic performance (Gehring and Monson, 1994; Obeso, 2002; Wheelwright and Logan, 2004; Xu et al., 2008).
Contrary to our first hypothesis, higher Fv/Fm in needles of female individuals indicates that they had a potentially higher capacity of light absorption, but it also means that they had been adapted to the low light environment and were thus more threatened by photoinhibition when exposed to high light compared with males (Maxwell and Johnson, 2000; Wyka et al., 2007). Our results are in agreement with the earlier findings of Xu et al. (2008) and Morales et al. (2016) suggesting that females are generally more sensitive to photoinhibition than males.
In our experiment, the male and female individuals were grown in the same light conditions, therefore lower values of Fv/Fm in T. baccata males compared with females suggest that males are evolutionarily adapted to a higher light environment. Females of this species are usually smaller and grow for longer under the shade of neighboring trees' crowns compared with males who are tall and better illuminated (Iszkuło et al., 2009). Moreover, females adapted to lower light environments might develop a different strategy of photoprotection than males. In our study, the PSII down-regulation in males did not significantly decrease their photosynthetic capacity compared with females, thus we state that it plays a photoprotective role as has been found in other conifer species (Adams et al., 2004). These results were confirmed by the enzyme activity data since the activity of POX was higher in female than in male needles, suggesting that females had a more efficient mechanism for scavenging free radicals (Sharma et al., 2012). This confirms our third hypothesis. In contrast to our results Mitchell (1998) did not find differences in Fv/Fm between T. baccata males and females growing in sun. Moreover, Juvany et al. (2014) also did not find differences in Fv/Fm between Pistacia lentiscus sexes, but NPQ values in female were higher than in male leaves indicating better photoprotection in female leaves. However, Xu et al. (2008) found higher Fv/Fm in male than in female Populus cathayana leaves only when they were exposed to drought. The differences between sexes in photochemistry and ability for photoprotection found in our study suggest that results depend on species and conditions of growth.
All of the mechanisms which help avoid photodamage, and which repair photodamage, have the potential to decrease the net CO2 assimilation rates and growth, and can be regarded as costs of photoinhibition (Raven, 2011). Mechanisms of photoprotection have been developed in the course of evolution of oxygenic photosynthesis, which ensures flexible light energy utilization in a variety of plant habitats (Ruban, 2015). In our experiment conducted under artificial shading, there was no durable or severe photoinhibition even in winter, but was replaced by PSII down-regulation, especially in non-fertilized males. Although the presented results do not allow us to evaluate fully the differences between T. baccata sexes in costs of photoprotection, the higher activity of POX and APX in female needles suggests that T. baccata females made greater efforts for protection against ROS than males. In addition, antioxidant enzymes also have an antagonistic effect on auxin activity (Sofo et al., 2004). This can explain to some extent the slower growth of T. baccata females compared with males.
Our findings corroborate the earlier results which indicate that fertilized young plants show higher photosynthesis and they are less affected by photoinhibition than non-fertilized ones (Nunes et al., 1993). Fertilization increased photosynthetic capacity of T. baccata expressed as ETRmax and ΦPPFsat. This result is consistent with the general trend in conifers that indicates nitrogen concentration is positively correlated with photosynthetic capacity (Gough et al., 2004a). However, in the long term, a positive fertilization effect on photosynthesis can disappear as in Pinus taeda L. (Gough et al., 2004b). Improved photosynthetic capacity of fertilized conifers such as P. taeda, Pinus sylvestris, and Abies alba has been shown to be due to more efficient and enhanced capacity of electron transport as it was in our study (Kellomäki and Wang, 1997; Lavigne et al., 2001) and/or greater carboxylation capacity (Kellomäki and Wang, 1997; Maier et al., 2002; Robakowski et al., 2003).
In our experiment, non-fertilized female and male T. baccata individuals displayed lower photochemical parameters, lower total chlorophyll and carotenoid concentrations, and higher activities of antioxidant enzymes (POX, APX, SOD) than fertilized individuals (hypothesis 4). This is consistent with earlier findings indicating that Mg-deficiency decreased concentrations of photosynthetic pigments and increased the activities of antioxidants (Laing et al., 2000; Tewari et al., 2006; Chou et al., 2011; Tang et al., 2012). In our study, under nutrient deficiency, a reduction of ETR resulted from a decrease in ΦPSII, and to a lesser extent to a decrease in α which was directly dependent on needle chlorophyll concentration. When plants are stressed by nutrient deficiency or excessive light, the acceptor side of PSII is overreduced, and the plants produce ROS near PSII. A delay of electron transport between PSII and PSI is due to their separation from the stroma thylakoids and the grana, respectively. This delay of electron transport promotes ROS generation such as the free radicals O·−2 and OH·− and non-radicals like H2O2 and 1O2 (Das and Roychoudhury, 2014; Yoshioka-Nishimura, 2016). Our results have shown that non-fertilized T. baccata individuals having lower ETRmax at lower values of PPFsat, lower Fv/Fm and higher NPQ345 along with higher activity of CAT, APX, and POX compared with fertilized individuals were more sensitive to photoinhibition induced by nutrients deficiency and low winter temperatures in December. This indicates that the antioxidant system is active and maintains the electron flow balance (Adams et al., 2004; Huseynova, 2012). Higher NPQ345 has also indicated that in non-fertilized individuals the higher amount of energy was dissipated as heat than in fertilized individuals, and the cost of this photoprotective mechanism, expressed as energy losses, can be greater under nutrient deficiency.
We did not find any significant interaction between the gender and fertilization. This indicates that both sexes reacted in a similar way to the stress conditions caused by nutrients deficiency. Male and female individuals of T. baccata acclimated to nutrients deficiency by lowering photosynthetic capacity and needle pigments concentrations. Total chlorophyll and carotenoids concentrations in needles changed seasonally and were lower without fertilization, but were independent of sex. However, in contrast to our results, Zarek (2015) found that there were higher concentrations of total chlorophyll and carotenoids in needles of adult female trees of T. baccata compared with males in autumn and in winter. This discrepancy may result from the fact that we used young individuals, and not mature trees. This confirms the conclusions from earlier research that long-term studies are needed to elucidate the responses of the sexes to multiple stressors (Suzuki et al., 2014; Juvany and Munné-Bosch, 2015; Retuerto et al., 2018). Female costs, in terms of carbon, may be at least partially compensated by increasing photosynthetic rates or by the photosynthesis of non-matured cones or fruits. We measured chlorophyll a fluorescence of a cross section of green T. baccata arils. The values of Fv/Fm ranging from 0.78 to 0.80 are lower than the optimum value of 0.84, but this suggests that green arils are able to photosynthesize and compensate to some extent the female reproductive effort. However, photosynthesis by green arils cannot cover construction and maintenance of non-photosynthetic seed and red arils. In a similar way, photosynthetic activity of reproductive structures in the dioecious Neotropical tree Ocotea tenera was insufficient to pay the high costs of reproduction borne by females (Wheelwright and Logan, 2004).
In conclusion, our results indicate that females of T. baccata have similar photosynthetic capacity to males and the higher reproductive efforts of females are not at the expense of their photosynthetic capacity. The maintenance of photosynthetic capacity and higher reproductive efforts of T. baccata females were at the cost of antioxidants production. The higher reproductive efforts of females did not decrease their photosynthetic capacity suggesting that the between-sexes differences in Fv/Fm and antioxidants activity found in our study resulted from the adaptation of females and males to different growth environments.
Study conception and design: PR, EP-K, and GI; acquisition of data: PR, EP-K, ER, and MR; analysis, interpretation of data, and drafting of manuscript: PR, ER, EP-K, and GI; critical revision: PT and Z-PY; final version: PR, EP-K, ER, PT, Z-PY, and GI.
The project was financed by the Polish National Science Centre awarded based on the decision number DEC-2012/07/B/NZ9/01314.
The authors declare that the research was conducted in the absence of any commercial or financial relationships that could be construed as a potential conflict of interest.
We would like to thank Stanisław Zymon and Kinga Nowak for help during rooting shoots and the field work.
The Supplementary Material for this article can be found online at: https://www.frontiersin.org/articles/10.3389/fpls.2018.00742/full#supplementary-material
ETR, apparent electron transport rate (μmol m−2 s−1); ETRmax, maximum value of ETR; Fv/Fm, maximum quantum yield of photosystem II photochemistry; Fm, maximum fluorescence yield; F'm, maximum fluorescence in the light; F0, minimum fluorescence yield; Fs, steady-state fluorescence; NPQ, non-photochemical quenching of fluorescence; PPF, photosynthetic photon flux (μmol m−2 s−1); PPFsat, saturating level of photosynthetic photon flux; PSII, photosystem II; ΦPSII, quantum yield of PSII photochemistry; ΦPPFsat, quantum yield of PSII photochemistry at the saturation level of PPF; SOD, superoxide dismutase; POX, guaiacol peroxidase; CAT, catalase; APX, ascorbate peroxidase.
Adams, W. W., Zarter, C. R., Ebbert, V., and Demmig-Adams, B. (2004). Photoprotective strategies of overwintering evergreens. Bioscience 54, 41–49. doi: 10.1641/0006-3568(2004)054[0041:PSOOE]2.0.CO;2
Barnes, J. D., Balaguer, L., Manrique, E., and Elvir, S. (1992). A Reappraisal of the use of DMSO for the extraction and determination of chlorophylls a and b in lichens and higher plants. Environ. Exp. Bot. 32, 85–100. doi: 10.1016/0098-8472(92)90034-Y
Benzarti, M., Rejeb, K. B., Debez, A., Messedi, D., and Abdelly, C. (2012). Photosynthetic activity and leaf antioxidative responses of Atriplex portulacoides subjected to extreme salinity. Acta Physiol. Plant. 34, 1679–1688. doi: 10.1007/s11738-012-0963-5
Bloom, A. J., Chapin, F. S., and Mooney, H. A. (1985). Resource limitation in plants – an economic analogy. Ann. Rev. Ecol. Syst. 16, 363–392. doi: 10.1146/annurev.es.16.110185.002051
Bradford, M. M. (1976). A rapid and sensitive method for the quantitation of microgram of protein utilizing the principle of protein-dye binding. Anal. Biochem. 72, 248–254. doi: 10.1016/0003-2697(76)90527-3
Cedro, A., and Iszkuło, G. (2011). Do females differ from males of European yew (Taxus baccata L.) in dendrochronological analysis? Tree Ring Res. 67, 3–11. doi: 10.3959/2009-9.1
Chance, B., and Maehly, A. C. (1955). “Assay of catalases and peroxidases,” in Methods in Enzymology, eds S. P. Collowick and N. O. Kapplan (New York, NY: Academic Press), 764–765.
Charlesworth, B., and Charlesworth, D. (1978). A model for the evolution of dioecy and gynodioecy. Am. Nat. 112, 975–997. doi: 10.1086/283342
Charnov, E. L. (1982). The Theory of Sex Allocation. Princeton Monograph in Population Biology, 18. New Jersey, NJ: Princeton University Press.
Chen, L., Dong, T., and Duan, B. (2014). Sex-specific carbon and nitrogen partitioning under N deposition in Populus cathayana. Trees 28, 793–806. doi: 10.1007/s00468-014-0992-3
Chou, T. S., Chao, Y. Y., Huang, W. D., Hong, C. Y., and Kao, C. H. (2011). Effect of magnesium deficiency on antioxidant status and cadmium toxicity in rice seedlings. J. Plant Physiol. 168, 1021–1030. doi: 10.1016/j.jplph.2010.12.004
Darwin, C. (1892). The Effects of Cross and Self Fertilisation in the Vegetable Kingdom. New York, NY: D. Appleton.
Das, K., and Roychoudhury, A. (2014). Reactive oxigen species (ROS) and response of antioxidants as ROS-scavengers during environmental stress in plants. Front. Environ. Sci. 2:53. doi: 10.3389/fenvs.2014.00053
Dawson, T. E., and Ehleringer, J. R. (1993). Gender-specific physiology, carbon isotope discrimination, and habitat distribution in boxelder, Acer negundo. Ecology 74, 798–815. doi: 10.2307/1940807
DeSoto, L., Olano, J. M., and Rozas, V. (2016). Secondary growth and carbohydrate storage patterns differ between sexes in Juniperus thurifera. Front. Plant Sci. 7:723. doi: 10.3389/fpls.2016.00723
Ding, X., Jiang, Y., Hao, T., Jin, H., Zhang, H., He, L., et al. (2016). Yu effects of heat shock on photosynthetic properties, antioxidant enzyme activity, and downy mildew of cucumber (Cucumis sativus L.) PLoS ONE 11:e0152429. doi: 10.1371/journal.pone.0152429
Evans, R. J. (1993). Photosynthetic acclimation and nitrogen partitioning within a lucerne canopy. II. Stability through time and comparison with a theoretical optimum. Aust. J. Plant Physiol. 20, 69–82. doi: 10.1071/PP9930069
Foyer, C. H., and Noctor, G. (2011). Ascorbate and glutathione: the heart of the redox hub. Plant Physiol. 155, 2–18. doi: 10.1104/pp.110.167569
Foyer, C. H., and Shigeoka, S. (2011). Understanding oxidative stress and antioxidant functions to enhance photosynthesis. Plant Physiol. 155, 93–100. doi: 10.1104/pp.110.166181
Garbarino, M., Weisberg, P. J., Bagnara, L., and Urbinati, C. (2015). Sex-related spatial segregation along environmental gradients in the dioecious conifer, Taxus baccata. For. Ecol. Manag. 358, 122–129. doi: 10.1016/j.foreco.2015.09.009
Gehring, J. L., and Monson, R. K. (1994). Sexual differences in gas exchange and response to environmental stress in dioecious Silene latifolia (Caryophyllaceae). Am. J. Bot. 81, 166–174. doi: 10.2307/2445630
Genty, B., Briantais, J. M., and Baker, N. R. (1989). The relationship between the quantum yield of photosynthetic electron transport and quenching of chlorophyll fluorescence. Biochem. Biophys. Acta 990, 87–92.
Giannopolitis, C. N., and Ries, S. K. (1977). Superoxide dismutases: I. Occurrence in higher plants. Plant Physiol. 59, 309–314. doi: 10.1104/pp.59.2.309
Gough, C. M., Seiler, J. R., and Maier, C. A. (2004a). Short term effects of fertilization on loblolly pine (Pinus taeda L.) physiology. Plant Cell Environ. 27, 876–886. doi: 10.1111/j.1365-3040.2004.01193.x
Gough, C. M., Seiler, J. R., Johnsen, K. H., and Sampson, D. A. (2004b). Seasonal photosynthesis in fertilized and nonfertilized loblolly pine. For. Sci. 50, 1–9.
Heilbuth, J. C., Ilves, K. L., and Otto, S. P. (2001). The consequences of dioecy for seed dispersal: modeling the seed-shadow handicap. Evolution 55, 880–888. doi: 10.1554/0014-3820(2001)055[0880:TCODFS]2.0.CO;2
Helmerhorst, E., and Stokes, G. B. (1980). Microcentrifuge desalting: a rapid, quantitative method for desalting small amounts of protein. Anal. Biochem. 104, 130–135. doi: 10.1016/0003-2697(80)90287-0
Huseynova, I. M. (2012). Photosynthetic characteristics and enzymatic antioxidant capacity of leaves from wheat cultivars exposed to drought. Biochim. Biophys. Acta 1817, 1516–1523. doi: 10.1016/j.bbabio.2012.02.037
Iszkuło, G., Jasinska, A. K., and Sobierajska, K. (2011a). Dendroecological differences between Taxus baccata males and females in comparison with monoecious Abies alba. Dendrobiology 65, 55–61.
Iszkuło, G., Jasinska, A. K., Giertych, M. J., and Boratynski, A. (2009). Do secondary sexual dimorphism and female intolerance to drought influence the sex ratio and extinction risk of Taxus baccata? Plant Ecol. 200, 229–240. doi: 10.1007/s11258-008-9447-5
Iszkuło, G., Jasinska, A. K., Romo Díez, À. M., Tomaszewski, D., and Szmyt, J. (2011b). The greater growth rate of male over female of the dioecious tree Juniperus thurifera only in worse habitat conditions. Dendrobiology 66, 15–24.
Iszkuło, G., Pers-Kamczyc, E., Nalepka, D., Rabska, M., Walas, Ł., and Dering, M. (2016). Postglacial migration dynamics helps to explain current scattered distribution of Taxus baccata. Dendrobiology 76, 81–89. doi: 10.12657/denbio.076.008
Juvany, M., and Munné-Bosch, S. (2015). Sex-related differences in stress tolerance in dioecious plants: a critical appraisal in a physiological context. J. Exp. Bot. 66, 6083–6092. doi: 10.1093/jxb/erv343
Juvany, M., Müller, M., Pintó-Marijuan, M., and Munné-Bosch, S. (2014). Sex-related differences in lipid peroxidation and photoprotection in Pistacia lentiscus. J. Exp. Bot. 65, 1039–1049. doi: 10.1093/jxb/ert446
Kellomäki, S., and Wang, K. Y. (1997). Effects of long-term CO2 and temperature elevation on crown nitrogen distribution and daily photosynthetic performance of Scots pine. For. Ecol. Manag. 99, 309–326. doi: 10.1016/S0378-1127(97)00059-5
Kohorn, L. (1994). Shoot morphology and reproduction in jojoba - advantages of sexual dimorphism. Ecology 75, 2384–2394. doi: 10.2307/1940892
Korpelainen, H. (2007). Labile sex expression in plants. Biol. Rev. 73, 157–180. doi: 10.1111/j.1469-185X.1997.tb00028.x
Kýpetová, M., Walas, Ł., Jaloviar, P., and Iszkuło, G. (2018). Influence of herbivory pressure on the growth rate and needle morphology of Taxus baccata L. juveniles. Dendrobiology 79, 10–19. doi: 10.12657/denbio.079.002
Laing, W., Greer, D., Sun, O., Beets, P., Alison Lowe, A., and Payn, T. (2000). Physiological impacts of Mg deficiency in Pinus radiata: growth and photosynthesis. New Phytol. 146, 47–57. doi: 10.1046/j.1469-8137.2000.00616.x
Lavigne, M. B., Little, C. H., and Major, J. E. (2001). Increasing the sink: source balance enhances photosynthetic rate of 1-year-old balsam fir foliage by increasing allocation of mineral nutrients. Tree Physiol. 21, 417–426. doi: 10.1093/treephys/21.7.417
Leigh, A., Cosgrove, M. J., and Nicotra, A. B. (2006). Reproductive allocation in a gender dimorphic shrub: anomalous female investment in Gynatrix pulchella? J. Ecol. 94, 1261–1271. doi: 10.1111/j.1365-2745.2006.01164.x
Maier, C. A., Johnsen, K. H., Butnor, J., Kress, L. W., and Anderson, P. H. (2002). Branch growth and gas exchange in 13-year-old loblolly pine (Pinus taeda) trees in response to elevated carbon dioxide concentration and fertilization. Tree Physiol. 22, 1093–1106. doi: 10.1093/treephys/22.15-16.1093
Matsushita, M., Takao, M., and Makita, A. (2016). Sex-different response in growth traits to resource heterogeneity explains male-biased sex ratio. Acta Oecol. 75, 8–14. doi: 10.1016/j.actao.2016.06.009
Maxwell, K., and Johnson, G. N. (2000). Chlorophyll fluorescence – a practical guide. J. Exp. Bot. 51, 659–668. doi: 10.1093/jexbot/51.345.659
Meagher, T. R. (1992). The quantitative genetics of sexual dimorphism in Silene latifolia (Caryophyllaceae). I. Genetic Variation. Evolution 46:445. doi: 10.2307/2409863
Messier, C., and Puttonen, P. (1995). Spatial and temporal variation in the Bight environment of developing Scots pine stands: the basis for a quick and efficient method of characterizing Bight. Can. J. For. Res. 25, 343–354. doi: 10.1139/x95-038
Mitchell, A. K. (1998). Acclimation of Pacific yew (Taxus bervifolia) foliage to sun and shade. Tree Physiol. 18, 749–757.
Montesinos, D., Villar-Salvador, P., García-Fayos, P., and Verdú, M. (2012). Genders in Juniperus thurifera have different functional responses to variations in nutrient availability. New Phytol. 193, 705–712. doi: 10.1111/j.1469-8137.2011.03982.x
Morales, M., Pintó-Marijuan, M., and Munné-Bosch, S. (2016). Seasonal, sex- and plant size-related effects on photoinhibition and photoprotection in the dioecious Mediterranean dwarf palm, Chamaerops humilis Front. Plant. Sci. 7:1116. doi: 10.3389/fpls.2016.01116
Nakano, Y., and Asada, K. (1981). Hydrogen peroxide is scavenged by ascorbate specific peroxidases in spinach chloroplast. Plant Cell Physiol. 22, 887–892.
Nowak-Dyjeta, K., Giertych, M. J., Thomas, P., and Iszkuło, G. (2017). Males and females of Juniperus communis L. and Taxus baccata L. show different seasonal patterns of nitrogen and carbon content in needles. Acta Physiol. Plant. 39:191. doi: 10.1007/s11738-017-2489-3
Nunes, M. A., Ramalho, J. D. C., and Dias, M. A. (1993). Effect of nitrogen supply on the photosynthetic performance of leaves from coffee plants exposed to bright light. J. Exp. Bot. 44, 893–899. doi: 10.1093/jxb/44.5.893
Obeso, J. R. (2002). The costs of reproduction in plants. New Phytol. 155, 321–348. doi: 10.1046/j.1469-8137.2002.00477.x
Pukacka, S., and Pukacki, P. M. (2000). Seasonal changes in antioxidant level of Scots pine (Pinus sylvestris L.) needles exposed to industrial pollution. II. Enzymatic scavengers activities. Acta Physiol. Plant. 22, 457–464. doi: 10.1007/s11738-000-0089-z
Rascher, U., Liebieg, M., and Lüttge, U. (2000). Evaluation of instant light-response curves of chlorophyll fluorescence parameters obtained with a portable chlorophyll fluorometer on site in the field. Plant Cell Environ. 23, 1397–1405. doi: 10.1046/j.1365-3040.2000.00650.x
Ratajczak, E., Małecka, A., Bagniewska-Zadworna, A., and Kalemba, E. M. (2015). The production, localization and spreading of reactive oxygen species contributes to the low vitality of long-term stored common beech (Fagus sylvatica L.) seeds. J. Plant Physiol. 174, 147–156. doi: 10.1016/j.jplph.2014.08.021
Raven, J. A. (2011). The cost of photoinhibition. Physiol. Plant. 142, 87–104. doi: 10.1111/j.1399-3054.2011.01465.x
Renner, S. S. (2014). The relative and absolute frequencies of angiosperm sexual systems: dioecy, monoecy, gynodioecy, and an updated online database. Am. J. Bot. 101, 1588–1596. doi: 10.3732/ajb.1400196
Renner, S. S., and Ricklefs, R. E. (1995). Dioecy and its correlates in the flowering plants. Am. J. Bot. 82, 596–606.
Retuerto, R., Sánchez Vilas, J., and Varga, S. (2018). Sexual dimorphism in response to stress. Environ. Exp. Bot. 146, 1–4. doi: 10.1016/j.envexpbot.2017.12.006
Robakowski, P. (2005). Susceptibility to low-temperature photoinhibition in three conifers differing in successional status. Tree Physiol. 25, 1151–1160. doi: 10.1093/treephys/25.9.1151
Robakowski, P., Montpied, P., and Dreyer, E. (2003). Plasticity of morphological and physiological traits in response to different levels of irradiance in seedlings of silver fir (Abies alba Mill). Trees 17, 431–441. doi: 10.1007/s00468-003-0257-z
Rozas, V., DeSoto, L., and Olano, J. M. (2009). Sex-specific, age-dependent sensitivity of tree-ring growth to climate in the dioecious tree Juniperus thurifera. New Phytol. 182, 687–697. doi: 10.1111/j.1469-8137.2009.02770.x
Ruban, A. V. (2015). Evolution under the sun: optimizing light harvesting in photosynthesis. J. Exp. Bot. 66, 7–23. doi: 10.1093/jxb/eru400
Sharma, P., Jha, A. B., Dubey, R. S., and Pessarakli, M. (2012). Reactive oxygen species, oxidative damage, and antioxidative defense mechanism in plants under stressful conditions. J. Bot. 2012:217037. doi: 10.1155/2012/217037
Sofo, A., Dichio, B., Xiloyannis, C., and Masia, A. (2004). Effects of different irradiance levels on some antioxidant enzymes and on malondialdehyde content during rewatering in olive tree. Plant Sci. 166, 293–302. doi: 10.1016/j.plantsci.2003.09.018
Suzuki, N., Rivero, R. M., Shulaev, V., Blumwald, E., and Mittler, R. (2014). Abiotic and biotic stress combinations. New Phytol. 203, 32–43. doi: 10.1111/nph.12797
Tang, T., Li, Y., and Chen, L. S. (2012). Magnesium deficiency–induced impairment of photosynthesis in leaves of fruiting Citrus reticulata trees accompanied by up-regulation of antioxidant metabolism to avoid photo-oxidative damage J. Plant Nutr. Soil Sci. 175, 784–793. doi: 10.1002/jpln.201100329
Tewari, R. K., Kumar, P., and Sharma, P. N. (2006). Magnesium deficiency induced oxidative stress and antioxidant responses in mulberry plants. Sci. Hortic. 108, 7–14. doi: 10.1016/j.scienta.2005.12.006
Thomas, P. A., and Polwart, A. (2003). Taxus baccata L. J. Ecol. 91, 489–524. doi: 10.1046/j.1365-2745.2003.00783.x
Vessella, F., Salis, A., Scirè, M., Piovesan, G., and Schirone, B. (2015). Natural regeneration and gender-specific spatial pattern of Taxus baccata in an old-growth population in Foresta Umbra (Italy). Dendrobiology 73, 75–90. doi: 10.12657/denbio.073.008
Wallace, C. S., and Rundel, P. W. (1979). Sexual dimorphism and resource allocation in male and female shrubs of Simmondsia chinensis. Oecologia 44, 34–39.
Wheelwright, N. T., and Logan, B. A. (2004). Previous-year reproduction reduces photosynthetic capacity and slows lifetime growth in females of a neotropical tree. Proc. Natl. Acad. Sci. U.S.A. 101, 8051–8055. doi: 10.1073/pnas.0402735101
Wyka, T., Robakowski, P., and Zytkowiak, R. (2007). Leaf acclimation to contrasting irradiance in juvenile evergreen and deciduous trees. Tree Physiol. 27, 1293–1306. doi: 10.1093/treephys/27.9.1293
Xu, X., Peng, G., Wu, C., Korpelainen, H., and Li, C. (2008). Drought inhibits photosynthetic capacity more in females than in males of Populus cathayana. Tree Physiol. 28, 1751–1759. doi: 10.1093/treephys/28.11.1751
Ye, Z. P., Robakowski, P., and Suggett, D. J. (2013). A mechanistic model for the light response of photosynthetic electron transport rate based on light harvesting properties of photosynthetic pigment molecules. Planta 237, 837–847. doi: 10.1007/s00425-012-1790-z
Yoshioka-Nishimura, M. (2016). “Quality control of photosystem II role of structural changes of thylakoid membranes and FtsH proteases in high light tolerance and recovery from photoinhibition” in Handbook of Photosynthesis, ed M. Pessarakli (Boca Raton, FL; London; New York, NY: Taylor and Francis; CRC Press), 229–240.
Zarek, M. (2015). Seasonal fluctuations of photosynthetic pigments content in Taxus baccata needles. Dendrobiology 76, 13–24. doi: 10.12657/denbio.076.002
Zhang, S., Chen, F., Peng, S., Ma, W., Korpelainen, H., and Li, C. (2010). Comparative physiological, ultrastructural and proteomic analyses reveal sexual differences in the responses of Populus cathayana under drought stress. Proteomics 10, 2661–2677. doi: 10.1002/pmic.200900650
Zhao, H., Xu, X., Zhang, Y., Korpelainen, H., and Li, C. (2011). Nitrogen deposition limits photosynthetic response to elevated CO2 differentially in a dioecious species. Oecologia 165, 41–54. doi: 10.1007/s00442-010-1763-5
Keywords: apparent electron transportation rate, carotenoids, chlorophyll a fluorescence, dioecious plants, antioxidants, English yew, photosynthesis
Citation: Robakowski P, Pers-Kamczyc E, Ratajczak E, Thomas PA, Ye Z-P, Rabska M and Iszkuło G (2018) Photochemistry and Antioxidative Capacity of Female and Male Taxus baccata L. Acclimated to Different Nutritional Environments. Front. Plant Sci. 9:742. doi: 10.3389/fpls.2018.00742
Received: 21 February 2018; Accepted: 15 May 2018;
Published: 05 June 2018.
Edited by:
Francisco Javier Cejudo, Universidad de Sevilla, SpainReviewed by:
Fernando José Cebola Lidon, Universidade Nova de Lisboa, PortugalCopyright © 2018 Robakowski, Pers-Kamczyc, Ratajczak, Thomas, Ye, Rabska and Iszkuło. This is an open-access article distributed under the terms of the Creative Commons Attribution License (CC BY). The use, distribution or reproduction in other forums is permitted, provided the original author(s) and the copyright owner are credited and that the original publication in this journal is cited, in accordance with accepted academic practice. No use, distribution or reproduction is permitted which does not comply with these terms.
*Correspondence: Emilia Pers-Kamczyc, ZXBrQG1hbi5wb3puYW4ucGw=
Disclaimer: All claims expressed in this article are solely those of the authors and do not necessarily represent those of their affiliated organizations, or those of the publisher, the editors and the reviewers. Any product that may be evaluated in this article or claim that may be made by its manufacturer is not guaranteed or endorsed by the publisher.
Research integrity at Frontiers
Learn more about the work of our research integrity team to safeguard the quality of each article we publish.