- 1Shanghai Chenshan Plant Science Research Center, Chinese Academy of Sciences, Shanghai Chenshan Botanical Garden, Shanghai, China
- 2College of Life and Environmental Sciences, Shanghai Normal University, Shanghai, China
- 3College of Life Sciences, Shangrao Normal University, Shangrao, China
The East Asian subtropics mostly occupied by evergreen broad-leaved forests (EBLFs), is one of the global diversity centers for evergreen oaks. Evergreen oaks are keystone canopy trees in EBLFs with important ecosystem function and crucial significance for regional biodiversity conservation. However, the species composition and diversity of Asian evergreen oaks are poorly understood. Here, we test whether the four chloroplast markers atpI-atpH, matK, psbA-trnH, and ycf1, can discriminate the two evergreen oak sections in Asia – Cyclobalanopsis and Ilex. Two hundred and seventy-two individuals representing 57 species were scanned and 17 species from other oaks sections were included for phylogenetic reconstruction. The genetic diversity of the Quercus sections was also compared. Overall, we found that universal chloroplast DNA (cpDNA) barcoding markers could resolve two clades in Quercus, i.e., subgenus Cerris (Old World Clade) and subgenus Quercus (New World Clade). The chloroplast markers distinguished the main sections, with few exceptions. Each cpDNA region showed no barcoding gap and none of them provided good resolution at the species level. The best species resolution (27.78%) was obtained when three or four markers were combined and analyzed using BLAST. The high conservation of the cpDNA and complicated evolutionary patterns, due to incomplete lineage sorting, interspecific hybridization and introgressions may hinder the ability of cpDNA markers to discriminate different species. When comparing diversification pattern across Quercus sections (Cyclobalanopsis, Ilex, Cerris, Quercus, and Protobalanus), we found that section Ilex was the most genetically diverse, and section Cyclobalanopsis was lower genetically diverse. This diversification pattern may have resulted from the interplay of the Eurasia Cenozoic tectonic movements, climate changes and different niches of their ancestral lineages.
Introduction
Understanding the biodiversity of ecosystems is critical for revealing the biome assembly and its function in different ecosystems (Hebert et al., 2003; Lahaye et al., 2008; Pitman et al., 2008). Taxonomy based on morphological features has enriched our understanding of biodiversity for over 300 years, and provide an inventory of global biodiversity. However, the morphology based taxonomy system has shortcomings (Hebert et al., 2003; Vilgalys, 2003; Gonzalez et al., 2009; Goldstein and DeSalle, 2011). For example, the key diagnostic traits may be under selection or subjected to parallel evolution providing false information on the identity of the taxa. Complementing techniques such as DNA sequences under neutral evolution have become an efficient way to identify species (Hollingsworth, 2007; CBOL Plant Working Group, 2009; Valentini et al., 2009; Hollingsworth et al., 2016). DNA barcoding has become instrumental in plant research for identification of cryptic species (Bickford et al., 2007; Miwa et al., 2009; Liu et al., 2011), biodiversity assessments (Lahaye et al., 2008; Gonzalez et al., 2009; von Cräutlein et al., 2011; Yan et al., 2015), community phylogeny (Kress et al., 2009, 2010), conservation biology (Laiou et al., 2013; Veldman et al., 2014; Liu et al., 2015; Shapcott et al., 2015), invasive biology (Armstrong and Ball, 2005; Bleeker et al., 2008; Newmaster and Ragupathy, 2009; Van De Wiel et al., 2009), and disease and pest management (Ball and Armstrong, 2006; Lee et al., 2012; Montecchio and Faccoli, 2014; Shapcott et al., 2015). At present, the resolution of several candidate DNA barcodes has been tested in many plant groups (Clerc-Blain et al., 2010; Gao et al., 2010; Liu et al., 2011; Yu et al., 2011; Wang D.Y. et al., 2017). However, there is a challenge for DNA barcoding to discriminate closely related species, especially for those with shallow phylogeny and complex evolutionary history (Newmaster et al., 2008; Chen et al., 2015; Yan et al., 2015), such as species-rich genera, Quercus.
The genus Quercus (Fagaceae) has ca. 400–500 species, which are widely distributed in the warm temperate forests of the Northern Hemisphere (Camus, 1936–1954; Nixon, 1993). Many species of the genus are the canopy or dominant trees in regional EBLFs and play an important role in the function and service of ecosystem (Fang and Yoda, 1991; Nixon, 2006; Petit et al., 2013). The species identification and taxonomy in oaks are notoriously difficult (Manos et al., 1999; Simeone et al., 2013; Hubert et al., 2014), especially for Asian evergreen oaks that are composed of two species-rich sections (Cyclobalanopsis and Ilex) (Denk and Grimm, 2010; Denk et al., 2017). Species of section Cyclobalanopsis are the dominant trees in Asian (sub)tropical EBLFs with ca. 90–120 species (Denk and Grimm, 2010; Denk et al., 2017). Species of section Ilex are widely distributed in Eurasian low-middle latitude habitats comprising ca. 35–36 species (Denk and Grimm, 2010; Simeone et al., 2016). Unfortunately, the wide range of EBLFs in East Asia is now greatly diminished as a result of intensified human activities (Cao and Zhang, 1997; Tang, 2010). At least 1/3 oak species in China are now endangered or threatened due to the severe habitats loss and human activities (Deng et al., 2013a). Therefore, understanding the species composition of East Asian evergreen oak forests is important for future conservation efforts. Although the Flora of China and other regional floristic works had been finished for decades (e.g., Huang et al., 1999; Phengklai et al., 2008), the oak taxonomy and their systematic placement are still not well resolved, in part due to the high level of intra- and inter-species genetic variation. Even the fine anatomy features of the East Asian evergreen oaks, such as leaf epidermal features (Deng et al., 2014, 2017b), pollen morphology (Denk and Grimm, 2009; Deng et al., 2013b; Denk and Tekleva, 2014), and wood anatomy (Zhao et al., 2007) had been comprehensively studied in recent years. But these works do not provide useful diagnostic features to clarify species identity, and suggest paraphyletic evolution of taxonomical traits. Moreover, the interspecies gene flow has blunted genetic integrity and reduced the ability to distinguish some East Asian evergreen oaks (Tamaki and Okada, 2014; An et al., 2017).
Chloroplast DNA sequences have been applied for phylogenetic analysis and DNA barcoding of species of section Ilex in the Mediterranean (Piredda et al., 2011; Simeone et al., 2013) and China (Yang et al., 2017), providing insights into the evolutionary history of oak taxa. However, these studies included limited taxa and geographic regions (Europe or small region of China), therefore did not provide enough information on DNA barcoding for evergreen oaks. For the species-rich section Cyclobalanopsis in East Asia, little is known about evolution of chloroplast genome and phylogeny. So far, only the intergenic region of the chloroplast trnT-trnL was applied to distinguish six species of section Cyclobalanopsis distributed in Japan, and proved to have moderate efficiency (Ohyama et al., 2001). Recent studies using cpDNA show that the species of section Cyclobalanopsis, e.g., Q. glauca (Xu et al., 2015), Q. schottkyana (Jiang et al., 2016), Q. arbutifolia (Xu et al., 2016), and Q. kerrii (Jiang et al., 2018), contain high variable regions to infer population history, but the resolution of cpDNA markers in discriminating section Cyclobalanopsis species is still unknown.
Evergreen broad-leaved forests potentially cover a wide zone of monsoon-dominated regions in East Asia (Fang and Yoda, 1991). This region, as a global biodiversity hotspot, has the world’s richest flora harboring remarkable array of endemic, relic and endangered species (Myers et al., 2000; Yang et al., 2004; López-Pujol et al., 2006), especially with many endemic and endangered oak species (Menitsky, 1984; Luo and Zhou, 2000; Deng et al., 2013a). Effective DNA barcodes for species identification, conservation and resource utilization are extremely necessary in East Asian evergreen oaks. A further DNA barcoding study with comprehensive sampling on species population from the main distribution ranges is needed. In addition, comparing of the genetic diversity patterns across the main lineages in Quercus can provide more information to infer the driving forces which might contribute to their divergence. Furthermore, the knowledge on biodiversity inventory in East Asian subtropics is far from enough, and new species are continuously being identified in recent years (e.g., Xiao et al., 2015; Liang et al., 2016; Jiang et al., 2017; Wang C.W. et al., 2017). DNA barcoding of keystone species in this region can provide crucial insight into the mechanism of plant community assembly and evolutionary trajectory of the East Asian regional biota.
In this study, we comprehensively collected East Asian evergreen oaks aiming to address the following issues: (1) to reveal the discrimination ability of cpDNA barcode markers in East Asian evergreen oaks; (2) to compare the genetic diversity of the main sections in Quercus; (3) to explore the possible applications of cpDNA markers into the taxonomic and phylogenetic approaches. Our study provides insights into the species identity of Asian oaks and important information for biogeographic and population genetics of these unique oaks.
Materials and Methods
Ethics Statement
Sampling of oak species and other Fagaceae plants were granted and supported by National Forestry Bureau of China and Local National Nature Reserves.
Plant Materials
One hundred and forty-seven individuals belonging to 29 species of Quercus section Cyclobalanopsis from East Asia and 125 individuals of the 28 species of section Ilex from East Asia were included in our studies. The sampling range covered the key distribution range of East Asian evergreen oaks in China, Japan, Vietnam, and Nepal. Additionally, individuals of five species of section Ilex from the Mediterranean were analyzed. The final dataset also included four species of section Cerris (18 individuals, from Eurasia), seven species of section Quercus (11 individuals, from North America and Eurasia), five species of the American section Lobatae (7 individuals), and one species (1 individual) of section Protobalanus from western North America. One individual of Lithocarpus henryi was used as outgroup to root the tree of genus Quercus. Sequences of eight species (16 individuals) were downloaded from GenBank. Detailed information of samples used in this study is listed in Supplementary Table S1.
Healthy leaves of each individual were collected and dried instantly in silica gel for DNA extraction. Voucher specimens of each individual were deposited in the Herbarium of the Shanghai Chenshan Botanical Garden (CSH).
DNA Extraction, PCR, and Sequencing Protocols
Genomic DNA was extracted using the modified CTAB method (Doyle and Doyle, 1987). Four cpDNA regions, the psbA-trnH intergenic spacer, a part of the matK gene, the atpI-atpH intergenic spacer and a portion of the ycf1 region were amplified using PCR and bidirectionally sequenced for analyses. PCR reactions were performed according to previously described method (Xu et al., 2015). The primer sequences used to amplify these regions are summarized in Supplementary Table S2. The sequences obtained in this study have been uploaded to GenBank (accession numbers: MH058100-MH059477).
Sequencher 4.01 (Gene Codes Corp., Ann Arbor, MI, United States) was used to assemble and edit sequences. The DNA sequences were aligned using the package Muscle (Edgar, 2004) implemented in MEGA 7.0.211 (Kumar et al., 2016) with subsequent manual adjustment.
DNA Barcoding Analyses
DNA polymorphisms were examined using DnaSP 5.10 (Librado and Rozas, 2009). To evaluate species discrimination success, four widely used methods, genetic distance-based, similarity-based, tree-based and diagnostic method, were applied to the four cpDNA markers and all their possible combinations.
Genetic distance-based method: p-distances of the four plastid regions were calculated in MEGA 7.0.21 (Kumar et al., 2016) (see footnote 1). We measured three parameters to determine intraspecific variation (Meyer and Paulay, 2005; Lahaye et al., 2008). First, we calculated average intraspecific difference between all samples collected. Second, we measured theta (𝜃), which means the average p-distance within each species. Theta eliminates biases resulted by unequal sampling between species. Lastly, we calculated average coalescent depth, which is the maximum intraspecific distance within each species. Average interspecific distance and smallest interspecific distance were used to characterize interspecific divergence (Meier et al., 2008). The distribution of intraspecific versus interspecific variability was compared using DNA barcoding gaps. Differentiations between the intraspecific and interspecific p-distance for each candidate barcode were also compared using the Wilcoxon signed rank tests in R 3.2.3 (R Core Team, 2015). To explore potential species groups, the obtained p-distance matrices for each barcode candidate and all combinations were analyzed using the Automatic Barcode Gap Discovery (ABGD) under default setting (Puillandre et al., 2012). If conspecific individuals were partitioned into the same group without sequences from other species, the species was considered as successfully delimited.
Similarity-based method: Sequences of the four candidate barcodes and all possible combinations were built as 15 local reference databases using NCBI-blast-2.6.0+ (Camacho et al., 2009; Tao, 2010). Each barcode sequence was then queried using the blastn command against its local reference database. When all individuals of a species had a top hit to conspecific individuals, species discrimination was considered successful.
Tree-based method: Bayesian trees were constructed using MrBayes 3.2.6 (Ronquist et al., 2012). Model of substitution was selected by Modeltest 3.7 (Posada and Crandall, 1998) based on the akaike information criterion (AIC). Two parallel Markov Chain Monte Carlo (MCMC) runs were performed for 20 million generations. The trees were sampled every 1,000 generations and inspected in Tracer v1.62. The first 15% trees were discarded as burn-in. Species discrimination was considered as successful only when all the conspecific individuals formed a monophyletic clade.
Diagnostic method: BLOG 2.0 (Barcoding with LOGic) was used for species identification under a Logic Mining method, which identifies the species in terms of location of key diagnostic nucleotides from the training sequences to classify species (Weitschek et al., 2013). Each candidate barcode and combination were tested with a single file input, with 80% of the sequences as the training data, and 20% as the test data. A species was considered as successfully delimited if conspecific individuals were correctly identified in both training and test datasets.
Phylogeographical and Genetic Diversity Analysis
Haplotypes were extracted by DnaSP 5.10 (Librado and Rozas, 2009). To measure the level of genetic variation, variable sites, average pairwise differences per base pair between sequence (nucleotide diversity) (Nei and Li, 1979) and haplotype diversity (Hd) were calculated using DnaSP 5.10 (Librado and Rozas, 2009). The genetic distances (p-distances) of each section were calculated in MEGA 7.0.21 (Kumar et al., 2016) (see footnote 1) for further comparison of genetic diversity. A principal coordinate analysis (PCoA) was performed using GenAlEx 6.5 (Peakall and Smouse, 2012) to illustrate both the similarity between different sections and the genetic diversity of each section.
Results
PCR Success and Sequence Characteristics
The four chloroplast candidate barcodes showed high amplification success rates (100%) at the species level. At individual level, matK, psbA-trnH, and ycf1 had high success rates (92.2–100%) (Table 1), while the atpI-atpH had the lowest success rate, potentially due to the long PCR product that was being amplified.
A total of 1,439 sequences were available for further analysis, of which 1,375 were newly generated in this study. Among the four cpDNA markers, the aligned lengths ranged from 554 bp for the matK to 1,187 bp for the atpI-atpH. The atpI-atpH region showed the highest number of variable sites, while the matK and psbA-trnH had the lowest variable sites (Table 1). The atpI-atpH region also had the highest number of indels (99), followed by ycf1 (43), psbA-trnH (26) and matK (3). Among the four chloroplast loci, psbA-trnH was the highest haplotype diversity, followed by atpI-atpH, ycf1 and matK (in descending order of Hd).
Genetic Divergence Within and Between Species
PsbA-trnH exhibited the highest level of intra-species variation, followed by ycf1, atpI-atpH and matK. When comparing the interspecific genetic divergence among the four candidate barcodes, psbA-trnH region exhibited the highest interspecific divergence, followed by ycf1, atpI-atpH, and matK (Table 1). This analysis demonstrated that psbA-trnH sequences provide the most suitable DNA barcodes.
DNA Barcoding Gap Assessment
The distribution of intraspecific and interspecific variation of four single markers lacked a distinct gap. Among the single locus, psbA-trnH had the highest variation between the distribution range of interspecific and intraspecific distances (Figure 1). Wilcoxon rank sum tests show that the intraspecific distances were always significantly lower than the interspecific distances (Table 2). However, the maximum intraspecific distances of four loci were higher than the minimum interspecific distance (Table 2).

TABLE 2. Comparison of inter- and intraspecific p-distance of the four chloroplast markers and Wilcoxon rank sum test between inter- and intraspecific p-distance.
Species Identification Efficiency of Chloroplast Loci
Rates of species resolution depended on the analytic methods used (Figure 2 and Supplementary Table S3). Overall, BLAST provided the highest species discrimination rates (6.76–27.78%) among single marker or combined markers, followed by distance-based method (6.76–21.62%) and tree-based (0–20.27%) method. BLOG provided the lowest species resolution (1.35–12.16%) overall (Figure 2 and Supplementary Table S3). The diagnostic nucleotide of the highest species identification identified by BLOG is listed in Supplementary Table S4.
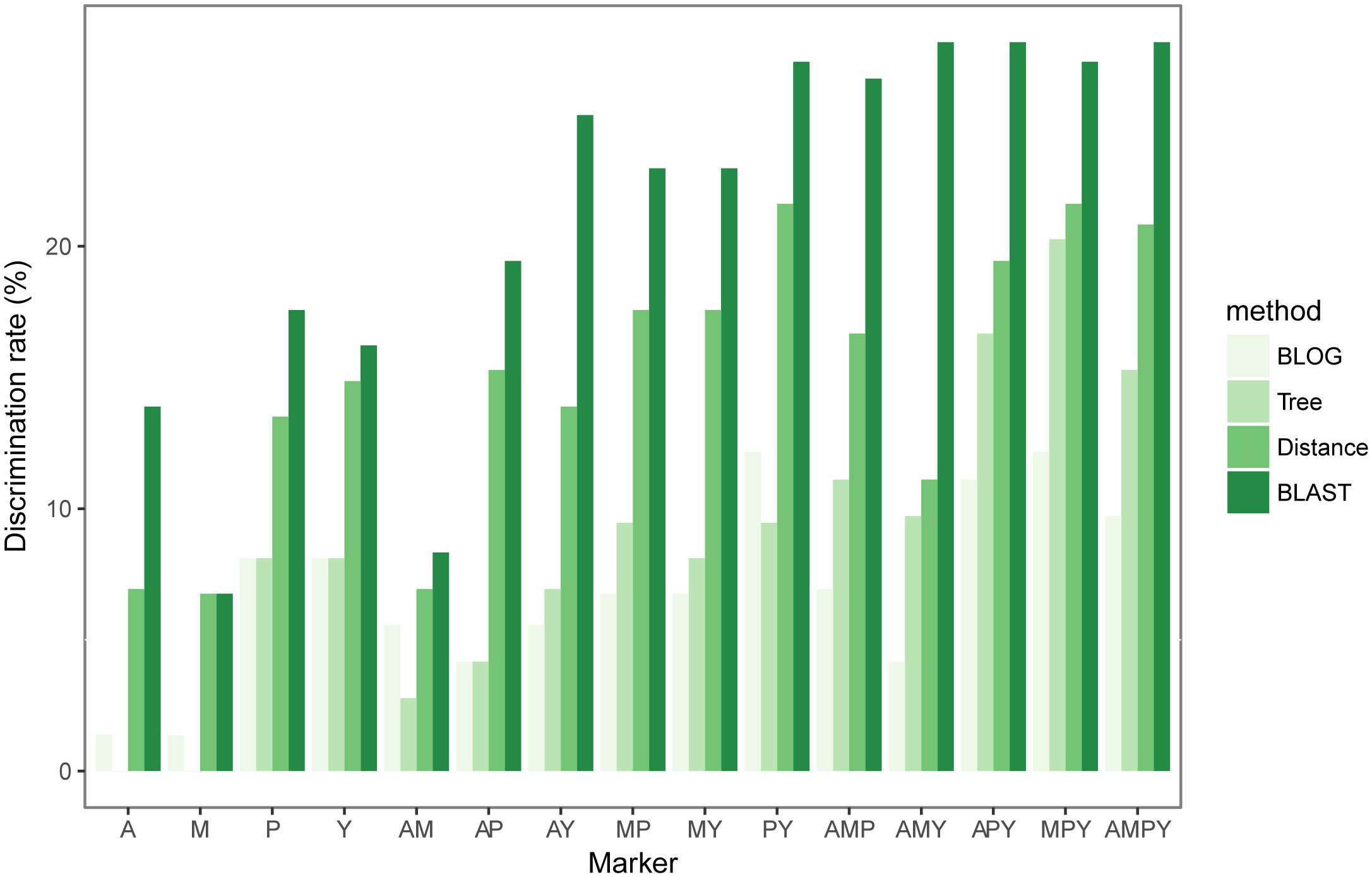
FIGURE 2. Species resolution of the four cpDNA markers and all combinations based on different methods.
Among the four single markers, psbA-trnH region showed the highest species discrimination rate for all methods except the distance-based method. When distance-based method was used, ycf1 exhibited the highest species discrimination rate (Figure 2 and Supplementary Table S3). In general, combinations with more loci could increase the probability that a species is identified correctly. Using three different combinations (atpI-atpH + matK + psbA-trnH, atpI-atpH + matK + ycf1 and atpI-atpH + matK + psbA-trnH + ycf1) with BLAST, they all generated the highest species identification rate (27.78%) (Figure 2 and Supplementary Table S3).
Phylogenetic Relationship
Subgenus Quercus, also known as the New World Clade that includes sections Lobatae, Protobalanus, and Quercus, formed a monophyletic clade (posterior probability = 1.00) (Figure 3). Section Lobatae was the only monophyletic clade (posterior probability = 1.00) inferred in this study. Two lineages were resolved in section Quercus, one with Eurasian species and the other one with the two American species (Figure 3). Section Protobalanus (Q. chrysolepis) was the sister group to the American lineage of section Quercus (Figure 3). However, we did not find strong support for the monophyletic status of subgenus Cerris (the Old World Clade). Species in section Cerris almost formed an monophyletic lineage, except for two individuals. The Mediterranean Q. suber mixed with species of section Ilex and an individual of East Asian Q. variabilis nested in a sister lineage to a section Ilex-Cyclobalanopsis mixed lineage (Figure 3). We also inferred one main clade and 11 small clades in section Cyclobalanopsis. However, we did not find strong support for the monophyletic status of section Cyclobalanopsis (Figure 3). Species within the 11 small Cyclobalanopsis clades were mixed with section Ilex species. In turn, the cpDNA based phylogenetic reconstruction inferred that section Ilex was polyphyletic and its evolutionary history appeared rather complicated compared to the other sections in Quercus (Figure 3).
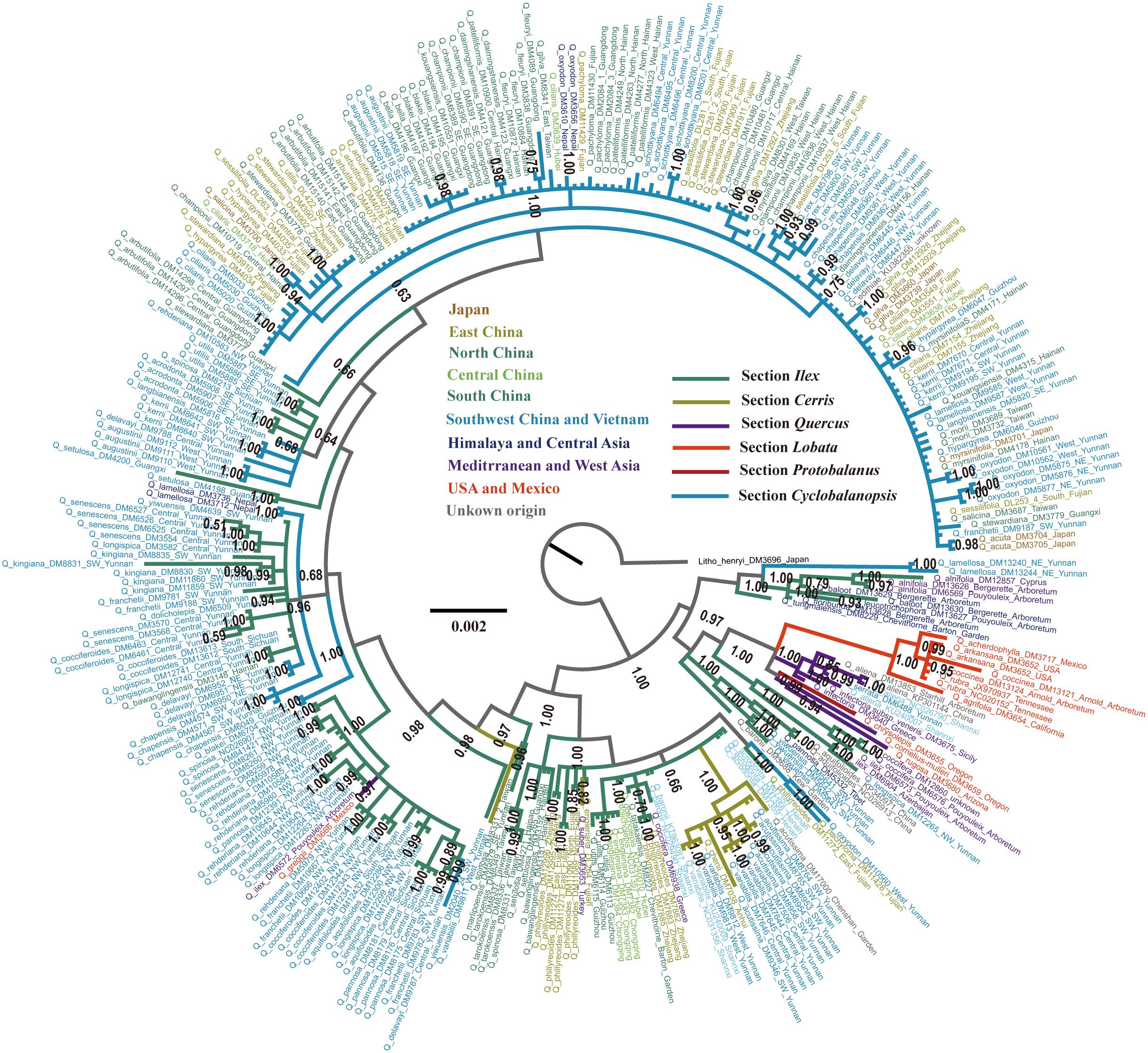
FIGURE 3. BI phylogram of Quercus inferred using combined cpDNA sequences. The tree was rooted using Lithocarpus henryi as the outgroup. Numbers close to the nodes are posterior probability values. Branch colors indicate different sections and colors of individuals indicate their localities with detail indication in the center of this figure.
Similar to the phylogenetic reconstruction results, PCoA using genetic distance among all the six sections (Figure 4) showed that section Cerris is closely to a group of East Asian subtropical species, including Q. acrodonta, Q. baronii, Q. phillyreoides, Q. dolicholepis, and Q. engleriana. Species of section Quercus from Oregon showed close similarity to section Lobatae. The major clade of section Cyclobalanopsis was relatively isolated, with only a few samples from Yunnan, SW China, are closely related to the individuals of section Ilex from the same region.
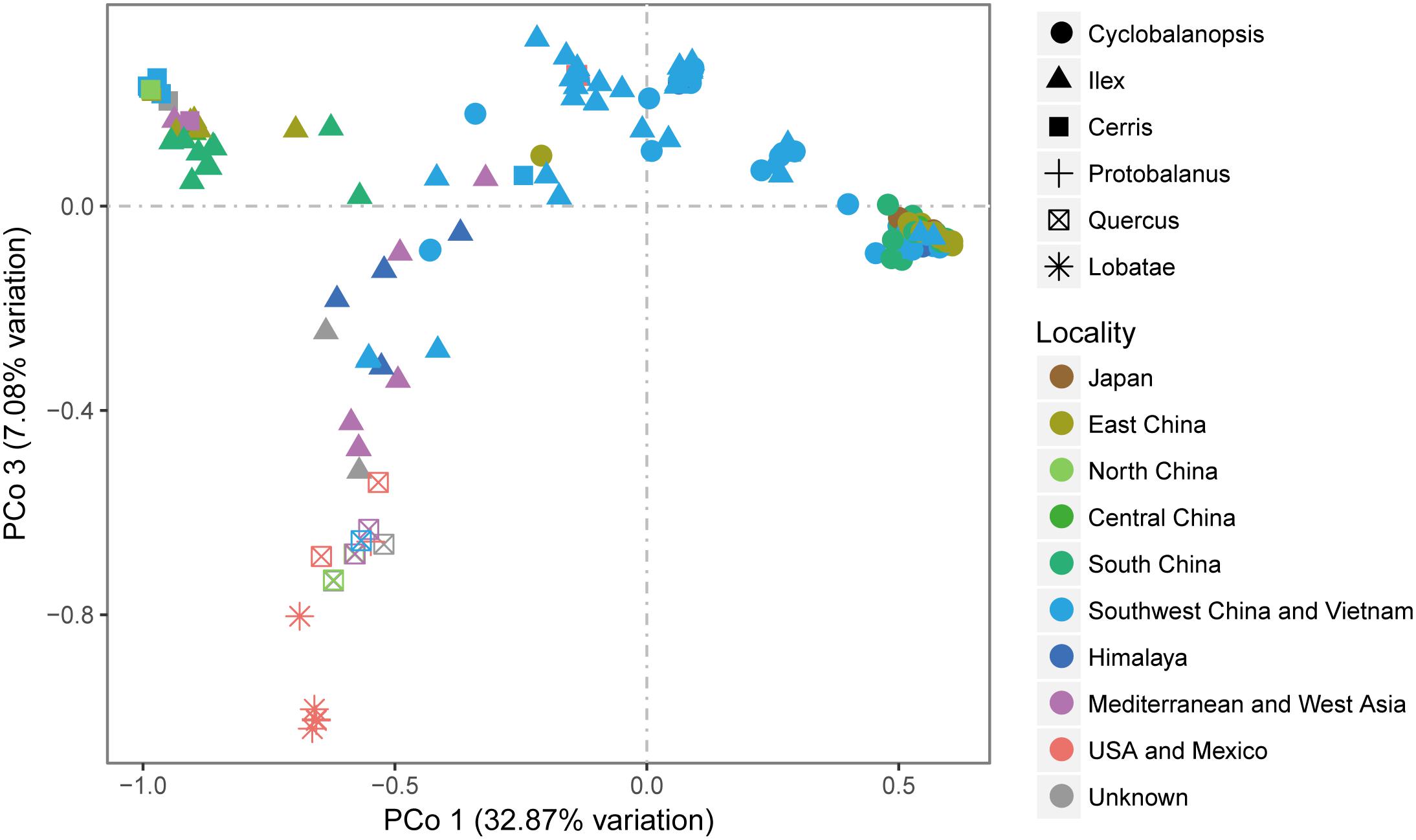
FIGURE 4. PCoA analysis of Quercus species based on the genetic distance obtained from combined cpDNA sequences.
Genetic Diversity Pattern
Section Ilex showed the highest diversity values for all the parameters (Table 3 and Figure 5), and formed three clusters in different quadrants on the PCoA plot (Figure 4). This suggests that section Ilex was the most diverse section in Quercus. Section Quercus was the second diverse section, with the second highest p-distance and haplotype diversity even only having a small number of samples (Table 3 and Figure 5). However, section Cyclobalanopsis with both high number of species and population sampling, yielded low genetic diversity levels, as the p-distance concentrated on low values (Figure 5) and the majority of individuals formed a small cluster in the PCoA analysis (Figure 5). Sections Cerris and Lobatae exhibited low genetic diversity as well (Table 3 and Figure 5). Nonetheless, we could not rule out the possibility that the low diversity might be caused by limited sampling, as we only covered four species and 18 individuals of section Cerris and five species (seven individuals) of section Lobatae.
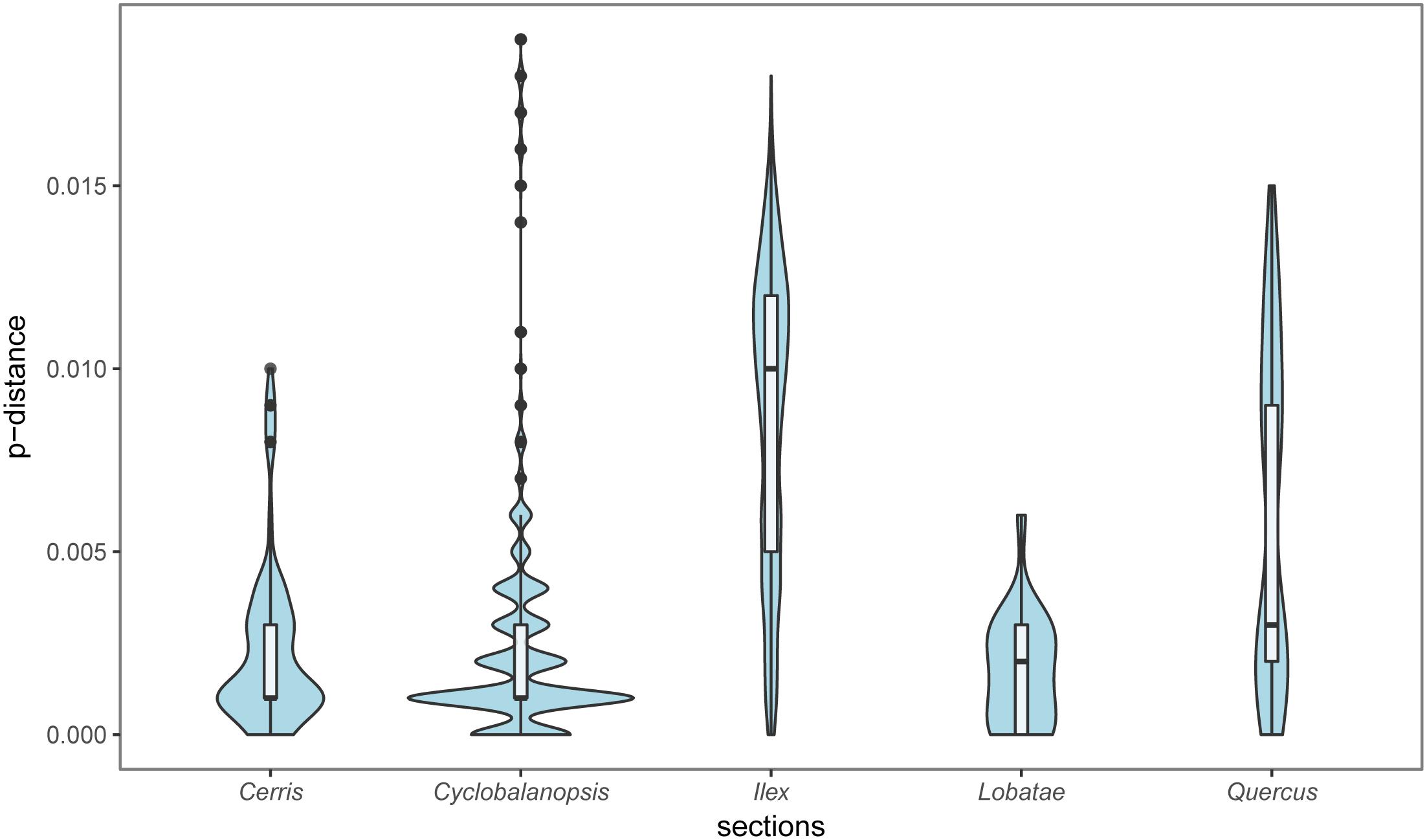
FIGURE 5. Genetic divergence of the five sections of genus Quercus based on the combined cpDNA sequences.
Discussion
The Application of cpDNA Markers on Oak Taxonomy and Systematics
We were able to successfully amplify and sequence the four cpDNA markers at high success rates (over 90%). There were differences in discrimination ability among each cpDNA marker. PsbA-trnH and ycf1 had the highest discrimination ability whereas matK had the lowest. The chloroplast region psbA-trnH had the highest discriminatory power in other plant groups and community phylogeny (Kress et al., 2010; Hollingsworth et al., 2011). Three different combinations (atpI-atpH + matK + psbA-trnH, atpI-atpH + matK + ycf1 and atpI-atpH + matK + psbA-trnH + ycf1) generated the highest species identification rate (27.78%) when used BLAST. Unfortunately, the overall identification success rates are not ideal and even the highest species resolution was below 30%. Meanwhile, none of the four chloroplast regions showed a barcode gap, which is an essential evaluation index for DNA barcodes (Hebert et al., 2003; Meier et al., 2006, 2008). The poor species resolution of universal chloroplast markers has been reported in East Asian oaks (Ohyama et al., 2001; Dong et al., 2015; Yang et al., 2017) and Euro-Mediterranean oaks (Piredda et al., 2011; Simeone et al., 2013), suggesting that these cpDNAs may not be ideal for resolving species identity in closely related oaks.
However, the universal cpDNA markers can provide information on resolving main infrageneric groups in oaks. First, two large clades of oaks, subgenera Cerris and Quercus could be distinguished when using the concatenated marker. Second, section Cerris is almost recognizable among the East Asian oaks, because it formed a monophyletic lineage with only two exceptional individuals falling into different groups. Third, most individuals of section Cyclobalanopsis (84.4%) formed a large lineage that was easily distinguished from section Ilex. Few individuals of section Ilex showed close relationship with few species in section Cyclobalanopsis from SW China. In addition to the concentric rings and scales on the cupule wall, section Cyclobalanopsis and Ilex have distinct morphological traits, such as leaf architecture structures (Zhou et al., 1995; Luo and Zhou, 2002), leaf blade shape and margin teeth (Menitsky, 1984; Huang et al., 1999) that can easily be used to distinguish the two sections.
Chloroplast markers are effective barcodes in discriminating many plant species, such as Myristicaceae (Newmaster et al., 2008), Pedicularis (Yu et al., 2011), Asteraceae (Gao et al., 2010), Carex and Kobresia (Clerc-Blain et al., 2010). However, they have limitations when discriminating closely related species, which is quite common, not only in oaks but also in other plant taxa, such as Salix (Percy et al., 2014), Rhododendron (Yan et al., 2015), Viburnum (Clement and Donoghue, 2012), and Curcuma (Chen et al., 2015). In this study, various cpDNA haplotypes exist within a species and some of these haplotypes are shared interspecifically. Recent phylogeographic studies of the section Ilex from the Himalayas (Feng et al., 2016; Meng et al., 2017) and the pan-Mediterranean (Simeone et al., 2016; Vitelli et al., 2017) also showed similar scenario. Moreover, even using the complete plastid genome sequences for phylogenetic reconstruction, the monophyletic status of species was still rare in section Quercus (Pham et al., 2017). The chloroplast genome may not be an efficient marker for fine-scale DNA barcoding nor for inferring systematics of closely related oak species (Pham et al., 2017). Therefore, either subsets of chloroplast barcode sequences, or the complete chloroplast genome are unlikely to provide confident identification of the source species. All these facts restrict the application of cpDNA sequences on species identification in closely related oaks.
Aside from cpDNA markers, ITS (incl. ITS1, 5.8S, ITS2) has been used as a universal DNA barcode marker for species-level phylogenetic studies (Kress et al., 2005; Hughes et al., 2006). The nrDNA ITS and 5S-IGS have been applied to infer oak phylogeny, which roughly illustrate the phylogeny skeleton of the main lineages in Quercus, however, the deep node phylogeny among sections are not resolved (Manos et al., 1999; Denk and Grimm, 2010). Indeed, orthologous sequences of nrDNA has better resolution than cpDNA at low taxonomical level to infer the phylogeny of oaks due to its biparental inheritance (Simeone et al., 2013). Nonetheless, nrDNA in oaks has paralogous copies and pseudogenes (Mayol and Rosselló, 2001; Bellarosa et al., 2005; Ma, 2006). Incorporating ITS paralogs in plant evolutionary studies is very risky because it can distort the phylogenetic signal (Mayol and Rosselló, 2001; Ma and Zhou, 2005; Feliner and Rosselló, 2007). To avoid incorporating ITS paralogs, PCR products need to be cloned into a vector for sequencing, which is time consuming and costly. Therefore, routine applicability of the ITS for barcoding is difficult.
Even single copy nuclear genes or a small batch of nuclear sequences may be not ideal barcoding makers for precise species identification in oaks, due to limited numbers of informative SNPs (Oh and Manos, 2008; Hubert et al., 2014). Recently, high-throughput markers, such as RAD-seq (Hipp et al., 2014; Cavender-Bares et al., 2015; Deng et al., 2017a,c) have demonstrated their power to successfully resolve the oak phylogeny with rich species and complex evolutionary history. Genome skimming also has great advantages for extending the plant barcode (Li et al., 2015; Coissac et al., 2016; Hollingsworth et al., 2016), allowing for the near-complete assembly of high-copy plastids, mitochondria and ribosomal DNA and possibly a fragmented nuclear genome assembly (Hollingsworth et al., 2016). These advanced technologies offer a promising future for DNA barcoding in oaks and probably other taxonomical difficult groups.
Performance of Different Barcoding Analysis Methods
The different performances of the four methods may reflect the analytical theories implemented in each method. BLAST had the best performance, providing the highest species resolution for all single markers and combinations. BLAST calculates the similarity of sequences, and assume that individuals from a species will be more similar to those from other species (van Velzen et al., 2012). This method also yields higher identification rates in other barcoding studies in Quercus, Curcuma, Rhododendron, and bryophytes (Hassel et al., 2013; Chen et al., 2015; Yan et al., 2015; Yang et al., 2017). The distance-based method (ABGD) automatically finds the distance where the barcode gap is located to partition the data set into candidate species, and can be used even when the intra- and inter-species distances overlap (Puillandre et al., 2012). This explained though intra- and inter-species distances overlap in our dataset, which adds difficulty to discriminate species. Nevertheless, ABGD still provided a relatively high species discrimination rate. Conversely, the tree-based methods and diagnostic methods generate low species identification rate. The tree-based approach is depended on monophyletic status of species. However, we only found few species were resolved as monophyletic clades and successfully identified. The poor resolution of tree-based method is in concordance with other studies (Meier et al., 2006; Little and Stevenson, 2007; Virgilio et al., 2010; Little, 2011). It is worth noting that BLOG has been reported to generate the highest sequence identification success in oaks and other plants (van Velzen et al., 2012; Weitschek et al., 2013; Yang et al., 2017). Actually, in our study BLOG also provides the highest individual identification success (36.23%), but it demonstrated the lowest species identification success, which requires correct identification of all individuals of a species in both training and test sequences. BLOG identifies potential diagnostic nucleotide positions from training sequences and assigns species using logic formulas based on the species-specific (diagnostic) codes (Weitschek et al., 2013). However, the diagnostic codes based on training sequences may not always cover the total variance of all sequences. Meanwhile, the total sequences are divided into training and test datasets in BLOG, as a result there are fewer samples (only one or two) in each dataset. Fewer samples may increase the discrimination rate and lead to a superficially high success rate. Hence, the high success rate produced by BLOG should be checked carefully, whether a species is correctly identified in both training and test dataset.
The Factors That Might Contributed to the Low Resolution of cpDNA in East Asian Evergreen Oaks
Conservation of the Chloroplast Genome
The poor species resolution of the four universal markers might be caused by multiple factors. Low mutation rates of chloroplast genome might lead to the poor resolution among different species. Actually, cpDNA has a low mutation rate, and much lower comparing to nrDNA (Lynch, 1997; Drouin et al., 2008). In theory, taxa with shorter generation time is likely to accumulate more mutations than long-lived species (Martin and Palumbi, 1993; Gaut et al., 1996). For woody plants with long reproductive cycle, their cpDNA mutation rates are much lower than the herbaceous species (Gaut et al., 1996). Generally, oaks have long generation time and oak seedlings need 3–45 years to produce their first acorns (Ducousso et al., 1993; Guyette et al., 2004; Kormanik et al., 2004). Consistent with the theory, the cpDNA substitution rates of oaks (0.19–0.96 × 10-9 s/s/y) (Chen et al., 2012; Xu et al., 2015, 2016; Feng et al., unpublished) are very low, below the average values reported for non-coding regions in other angiosperm lineages (1.2–1.7 × 10-9 s/s/y) (Graur and Li, 2000). Similarly, the low mutation rate of chloroplast genome is also reported in other woody species (Qi et al., 2012; Guo et al., 2014). The low substitution rate of cpDNA of oaks resulted in fewer mutations between different individuals, which partly explains the low species resolution of cpDNA in oaks. However, the low resolution may also be due to selective sweeps in chloroplast genome and past genetic drift (Ellstrand and Elam, 1993; Muir and Filatov, 2007; Percy et al., 2014). Because of the lower effective population size compared to nuclear genome, chloroplast genome may be greatly affected by genetic drift, which reduces the genetic diversity (Birky, 1988; Ellstrand and Elam, 1993; Schaal et al., 1998). As the result, chloroplast genome demonstrates low genetic diversity.
Complicated cpDNA Evolutionary History Involved Introgression and Incomplete Lineage Sorting
Chloroplast is maternally inherited in oaks (Dumolin et al., 1995). As a result, cpDNA is easy to transfer during the hybridization process, especially with asymmetric gene flow in the parental populations. Therefore, the cpDNA phylogeny only reflects the maternal evolutionary history. Oaks are notorious for interspecific hybridization, especially among the species in the same section (Curtu et al., 2007; Neophytou et al., 2011b; Moran et al., 2012; Leroy et al., 2017; McVay et al., 2017). Natural hybridization in East Asian evergreen oaks has been revealed and well demonstrated among sympatric species. In section Cyclobalanopsis, introgression between sympatric species (Q. sessilifolia and Q. acuta distributed in Korea and Japan; Q. austrochinchinensis and Q. kerrii distributed in Southwest China) were fully revealed using morphological traits and molecular markers (Tamaki and Okada, 2014; Song et al., 2015; An et al., 2017). The morphological intermediates have been frequently reported in section Ilex (Huang et al., 1999; Neophytou et al., 2007) and several hybridization zones with a series of morphological transitions have been discovered according to our field observation, indicating frequent interspecies hybridization. Meanwhile, the inconsistent evolutionary patterns between cpDNA and nrDNA were commonly detected in regional phylogeographic and population genetic analysis of section Ilex, which also implied introgression occurs in the Mediterranean and East Asia (Neophytou et al., 2011a,b; Meng et al., 2017; Vitelli et al., 2017). We found that the evolutionary history of the chloroplast genome is net-like rather than dichotomous, which hinders the discriminatory ability to infer the species tree using these maternal markers, especially in a frequent-hybridized taxa (Hollingsworth et al., 2011; Coissac et al., 2016). Moreover, driven by the peculiar paleogeographical histories of the studied regions, plastid genome may reveal geographic differentiation or distribution range of the ancestral lineage instead of the true species phylogeny (Petit et al., 2002; Gugger and Cavender-Bares, 2013; Simeone et al., 2016; Pham et al., 2017; Vitelli et al., 2017). Eurasian evergreen oaks also have strong cpDNA genetic differentiation across different geographical regions instead of species taxonomy. As we found in section Ilex, individuals from Mediterranean-Himalaya, SW China and East China formed independent clusters, respectively. It is likely that active tectonic movements induced uplift of SE Himalaya fringes and adjacent region, which blocked the regional seed-mediated gene flow and resulted in the strong cpDNA geographic differentiation in these oaks.
Another explanation for haplotype sharing between species which located across long distance is the incomplete lineage sorting of the ancestral lineages (Piredda et al., 2011; Simeone et al., 2013). In addition, occasional introgression following the long-distance dispersal events might result in this pattern as well (Lumaret et al., 2002; Toumi and Lumaret, 2010). Therefore, the low cpDNA mutation rates, strong asymmetric interspecies gene flow and incomplete lineage sorting of ancestral polymorphism may have contributed to the low resolution of cpDNA for species delimitation for East Asian evergreen oaks.
Different Genetic Diversity Patterns in Asian Evergreen Lineages
Why Is the cpDNA Phylogeny Non-monophyletic?
Within the subgenus Cerris, our data shows that section Ilex is closely related to both sections Cyclobalanopsis and Cerris. A similar unresolved complex phylogenic relationship within subgenus Cerris was also found in other studies using cpDNA markers (Manos et al., 1999; Simeone et al., 2016). The chloroplast capture events within a genus are mostly due to hybridization (Fehrer et al., 2007; Acosta and Premoli, 2010). However, the hybridization between the extant sections in oaks is extremely rare in the wild (Costello et al., 2011). The non-monophyly of section Ilex plastomes and its close relationship with sections Cyclobalanopsis and Cerris may reflect an ancient introgression or incomplete lineage sorting of the chloroplast genome in the ancestor lineages of subgenus Cerris. In the future, this issue should be subjected to the further study using high-throughput molecular marker (e.g., GBS, RAD-seq) to resolve the species phylogeny. In addition, it needs to recruit multiple species populations of the three sections to reveal the ancient and/or contemporary gene flow among the lineages.
Why the Genetic Diversity in Sections of Quercus Is Different?
Theoretically, the deciduous lineages which are usually fast growing species, may have higher genetic variation, because they have shorter sexual maturation time comparing to evergreen lineages (Zuidema et al., 2009). Generally, it takes at least five or more years for seedlings of evergreen oaks to produce first acorns. But for the deciduous species, this cycle only needs 2–3 years (Wang and Gao, 2005; Wang et al., 2011), such as in Q. serrata, Q. fabri, and Q. aliena. However, the genetic variation patterns in oak sections may not match this theory well, as our study revealed that sections Ilex and Quercus have higher genetic variation, and other sections have lower variation. This result is slightly different to previous reports that sections Ilex, Lobatae and Quercus were the most variable lineages, and section Cerris was the least variable (Simeone et al., 2016). Currently, it is too early to conclude the overall genetic diversity patterns of the genus Quercus, because of the uneven sampling of the main sections.
However, in the well-sampled Asian evergreen sections Cyclobalanopsis and Ilex, we found different patterns of genetic diversity. Trees of section Ilex grow at semi-arid habitats with slow biomass accumulation ratio and long sexual maturation cycle (Mediavilla and Escudero, 2003). All these biological traits of species of section Ilex tend to cause low cpDNA genetic diversity level. However, it was the most diverse lineage based on our study. Interestingly, section Cyclobalanopsis with high number of species and great morphological variation showed low genetic diversity. The different biogeographical histories and adaptabilities to the environment in the two sections may have driven their genetic diversity patterns. Section Cyclobalanopsis was an early derived (at the early Oligocene) section of subgenus Cerris, but their lineage diversification did not occur until the early Miocene and the highest diversification ratio achieved at the mid-late Miocene (Deng et al., 2017a). Instead, section Ilex was later derived at the early-middle Oligocene, but it shows a stepwise diversification pattern since the middle Oligocene onward (Deng et al., 2017c). Therefore, most species in section Cyclobalanopsis are probably younger than those in section Ilex. With longer evolutionary time, more cpDNA mutations may have accumulated, which may explain why the genetic diversity level of section Ilex is much higher than that of section Cyclobalanopsis. Moreover, section Ilex can grow in a wide geographic range with diversified habitats, aside from the prominent geographic barriers, the local adaptation to different environments may have also played a role in boosting the genetic diversity of section Ilex (Du et al., 2016; Feng et al., 2016; Meng et al., 2017; Feng et al., unpublished). In contrast, trees in section Cyclobalanopsis live mainly in warm and humid subtropical habitats (Huang et al., 1999; Deng et al., 2017a). As dominant trees, their large effective population size and the similar habitats may make them less influenced by topographic and climate changes. Shorter divergence time, long-term environment stability and strong gene flow among regional populations, may have altogether resulted in the low genetic diversity of the cpDNA in section Cyclobalanopsis.
Conclusion and Perspective
This study reveals that the cpDNA markers have limited efficiency to identify the Asian evergreen oaks, but these cpDNA markers are still informative to infer the species placement to the main sections of Quercus. The different genetic diversity patterns between the two evergreen oak sections Ilex and Cyclobalanopsis may have been shaped by their spatio-temporal histories and different adaptabilities to environments. The different evolutionary pattern of oaks revealed by the chloroplast and nuclear genomes is most likely due to the historical introgression in the ancestral lineages and recent or on-going gene flow between the closely related species. All these factors reduce the efficiency of cpDNA as species level barcodes. Even single copy nuclear genes or a small batch of nuclear sequences may not be ideal barcoding makers for precise species identification in oaks, due to limited number of informative SNPs. The advanced technologies, such as high-throughput markers (e.g., RAD-seq and GBS) and genome skimming offer a promising future for DNA barcoding in oaks and other taxonomic difficult groups.
Author Contributions
MD conceived and designed the experiments. YX, RL, and MY performed the experiments. MY and JS analyzed the data. MD was responsible for field collections and specimen identification. MY and MD wrote and revised the paper.
Funding
This work was supported by grants from the Shanghai Municipal Administration of Forestation and City Appearances (G162405, G172406, and G162404), Southeast Asia Biodiversity Research Institute, Chinese Academy of Sciences (Y4ZK111B01), National Natural Science Foundation of China (31270267), Science and Technology Basic Work (S&T Basic Work) (2013FY112100), and International Partnership Program of Chinese Academy of Sciences (151111KYSB20170021).
Conflict of Interest Statement
The authors declare that the research was conducted in the absence of any commercial or financial relationships that could be construed as a potential conflict of interest.
Acknowledgments
We thank Xiaolong Jiang, Yigang Song, and Quanjian Li for their assistance with the sampling.
Supplementary Material
The Supplementary Material for this article can be found online at: https://www.frontiersin.org/articles/10.3389/fpls.2018.00569/full#supplementary-material
Abbreviations
cpDNA, chloroplast DNA; EBLF, evergreen broad-leaved forest.
Footnotes
References
Acosta, M. C., and Premoli, A. C. (2010). Evidence of chloroplast capture in South American Nothofagus (subgenus Nothofagus, Nothofagaceae). Mol. Phylogenet. Evol. 54, 235–242. doi: 10.1016/j.ympev.2009.08.008
An, M., Deng, M., Zheng, S. S., Jiang, X. L., and Song, Y. G. (2017). Introgression threatens the genetic diversity of Quercus austrocochinchinensis (Fagaceae), an endangered oak: a case inferred by molecular markers. Front. Plant Sci. 8:229. doi: 10.3389/fpls.2017.00229
Armstrong, K., and Ball, S. (2005). DNA barcodes for biosecurity: invasive species identification. Philos. Trans. R. Soc. Lond. B Biol. Sci. 360, 1813–1823. doi: 10.1098/rstb.2005.1713
Ball, S. L., and Armstrong, K. F. (2006). DNA barcodes for insect pest identification: a test case with tussock moths (Lepidoptera: Lymantriidae). Can. J. For. Res. 36, 337–350. doi: 10.1098/rstb.2005.1713
Bellarosa, R., Simeone, M. C., Papini, A., and Schirone, B. (2005). Utility of ITS sequence data for phylogenetic reconstruction of Italian Quercus spp. Mol. Phylogenet. Evol. 34, 355–370. doi: 10.1016/j.ympev.2004.10014
Bickford, D., Lohman, D. J., Sodhi, N. S., Ng, P. K., Meier, R., Winker, K., et al. (2007). Cryptic species as a window on diversity and conservation. Trends Ecol. Evol. 22, 148–155. doi: 10.1016/j.tree.2006.11.004
Birky, C. W. (1988). “Evolution and variation in plant chloroplast and mitochondrial genomes,” in Plant Evolutionary Biology, eds L. D. Gottlieb and S. K. Jain (Dordrecht: Springer), 23–53.
Bleeker, W., Klausmeyer, S., Peintinger, M., and Dienst, M. (2008). DNA sequences identify invasive alien Cardamine at Lake Constance. Biol. Conserv. 141, 692–698. doi: 10.1016/j.biocon.2007.12.015
Camacho, C., Coulouris, G., Avagyan, V., Ma, N., Papadopoulos, J., Bealer, K., et al. (2009). BLAST+: architecture and applications. BMC Bioinformatics 10:421. doi: 10.1186/1471-2105-10-421
Cao, M., and Zhang, J. (1997). Tree species diversity of tropical forest vegetation in Xishuangbanna, SW China. Biodivers. Conserv. 7, 995–1006. doi: 10.15517/rbt.v59i13212
Cavender-Bares, J., González-Rodríguez, A., Eaton, D. A., Hipp, A. A., Beulke, A., and Manos, P. S. (2015). Phylogeny and biogeography of the American live oaks (Quercus subsection Virentes): a genomic and population genetics approach. Mol. Ecol. Resour. 24, 3668–3687. doi: 10.1111/mec.13269
CBOL Plant Working Group (2009). A DNA barcode for land plants. Proc. Natl. Acad. Sci. U.S.A. 106, 12794–12797. doi: 10.1073/pnas.0905845106
Chen, D. M., Zhang, X. X., Kang, H. H., Sun, X., Yin, S., Du, H. E., et al. (2012). Phylogeography of Quercus variabilis based on chloroplast DNA sequence in East Asia: multiple glacial refugia and mainland-migrated island populations. PLoS One 7:e47268. doi: 10.1371/journal.pone.0047268
Chen, J., Zhao, J., Erickson, D. L., Xia, N., and Kress, W. J. (2015). Testing DNA barcodes in closely related species of Curcuma (Zingiberaceae) from Myanmar and China. Mol. Ecol. Resour. 15, 337–348. doi: 10.1111/1755-0998.12319
Clement, W. L., and Donoghue, M. J. (2012). Barcoding success as a function of phylogenetic relatedness in Viburnum, a clade of woody angiosperms. BMC Evol. Biol. 12:73. doi: 10.1186/1471-2148-12-73
Clerc-Blain, J. L., Starr, J. R., Bull, R. D., and Saarela, J. M. (2010). A regional approach to plant DNA barcoding provides high species resolution of sedges (Carex and Kobresia, Cyperaceae) in the Canadian Arctic Archipelago. Mol. Ecol. Resour. 10, 69–91. doi: 10.1111/j.1755-0998.2009.02725.x
Coissac, E., Hollingsworth, P. M., Lavergne, S., and Taberlet, P. (2016). From barcodes to genomes: extending the concept of DNA barcoding. Mol. Ecol. 25, 1423–1428. doi: 10.1111/mec.13549
Costello, L. R., Hagen, B. W., and Katherine, S. J. (2011). Oaks in the Urban Landscape, Selection, Care and Preservation. Richmond, VA: California University of California.
Curtu, A. L., Gailing, O., and Finkeldey, R. (2007). Evidence for hybridization and introgression within a species-rich oak (Quercus spp.) community. BMC Evol. Biol. 7:218. doi: 10.1186/1471-2148-7-218
Deng, M., Hipp, A., Song, Y. G., Li, Q. S., Coombes, A., and Cotton, A. (2014). Leaf epidermal features of Quercus subgenus Cyclobalanopsis (Fagaceae) and their systematic significance. Bot. J. Linn. Soc. 176, 224–259. doi: 10.1111/boj.12207
Deng, M., Jiang, X. L., Hipp, A. L., Manos, P. S., and Hahn, M. (2017a). Phylogeny and biogeography of East Asian evergreen oaks (Quercus section Cyclobalanopsis; Fagaceae): insights into the Cenozoic history of evergreen broad-leaved forests in subtropical Asia. Mol. Phylogenet. Evol. 119, 170–181. doi: 10.1016/j.ympev.2017.11.003
Deng, M., Jiang, X. L., Song, Y. G., Coombes, A., Yang, X. R., Xiong, Y. S., et al. (2017b). Leaf epidermal features of Quercus Group Ilex (Fagaceae) and their application to species identification. Rev. Palaeobot. Palynol. 237, 10–36. doi: 10.1016/j.revpalbo.2016.11.006
Deng, M., Jiang, X. L., Su, T., Hipp, A., and Zhou, Z. K. (2017c). “Young dispersal from East Asia through the Himalya to the Mediterranean of the Tethys disjunction lineage-Biogeography of Quercus section Ilex (Fagaceae),” in Proceedings of the IUFRO Genetics and Genomics of Fagaceae, Shanghai.
Deng, M., Li, Q. S., Yang, S. T., Xu, J., Song, Y. G., and Li, Q. J. (2013a). “Endangered Oaks in China and the conservation challenges,” in Proceedings of the 5th Global Botanic Gardens Congress, Guangzhou.
Deng, M., Song, Y. G., Li, Q. J., and Li, Q. S. (2013b). Pollen morphology of Quercus subg. Cyclobalanopsis (Fagaceae) and its systematic implication. Guihaia 33, 368–375. doi: 10.5586/asbp.2012.005
Denk, T., and Grimm, G. W. (2009). Significance of pollen characteristics for infageric classification and phylogeny in Quercus (Fagaceae). Int. J. Plant Sci. 170, 926–940.
Denk, T., and Grimm, G. W. (2010). The oaks of western Eurasia: traditional classifications and evidence from two nuclear markers. Taxon 59, 351–366.
Denk, T., Grimm, G. W., Manos, P. S., Deng, M., and Hipp, A. L. (2017). “An updated infrageneric classification of the oaks: review of previous taxonomic schemes and synthesis of evolutionary patterns,” in Oaks Physiological Ecology. Exploring the Functional Diversity of Genus Quercus L, eds E. Gil-Pelegrín, J. J. Peguero-Pina, and D. Sancho-Knapik (Berlin: Springer), 13–38.
Denk, T., and Tekleva, M. V. (2014). Pollen morphology and ultrastructure of Quercus with focus on Group Ilex (=Quercus subgenus Heterobalanus (Oerst.) Menitsky): implications for oak systematics and evolution. Grana 53, 255–282. doi: 10.1080/00173134.2014918647
Dong, W., Xu, C., Li, C., Sun, J., Zuo, Y., Shi, S., et al. (2015). ycf1, the most promising plastid DNA barcode of land plants. Sci. Rep. 5:8348. doi: 10.1038/srep08348
Doyle, J. J., and Doyle, J. L. (1987). A rapid DNA isolation procedure for small quantities of fresh leaf tissue. Phytochem. Bull. 19, 11–15.
Drouin, G., Daoud, H., and Xia, J. (2008). Relative rates of synonymous substitutions in the mitochondrial, chloroplast and nuclear genomes of seed plants. Mol. Phylogenet. Evol. 49, 827–831. doi: 10.1016/j.ympev.2008.09.009
Du, F. K., Hou, M., Wang, W., Mao, K., and Hampe, A. (2016). Phylogeography of Quercus aquifolioides provides novel insights into the Neogene history of a major global hotspot of plant diversity in south-west China. J. Biogeogr. 44, 294–307. doi: 10.1111/jbi.12836
Ducousso, A., Michaud, H., and Lumaret, R. (1993). Reproduction and gene flow in the genus Quercus L. Ann. Sci. For. 50(Suppl.), 91s–106s. doi: 10.1051/forest:19930708
Dumolin, S., Demesure, B., and Petit, R. (1995). Inheritance of chloroplast and mitochondrial genomes in pedunculate oak investigated with an efficient PCR method. Theor. Appl. Genet. 91, 1253–1256. doi: 10.1007/BF00220937
Edgar, R. C. (2004). MUSCLE: multiple sequence alignment with high accuracy and high throughput. Nucleic Acids Res. 32, 1792–1797. doi: 10.1093/nar/gkh340
Ellstrand, N. C., and Elam, D. R. (1993). Population genetic consequences of small population size: implications for plant conservation. Annu. Rev. Ecol. Syst. 24, 217–242. doi: 10.1146/annurev.es.24.110193.001245
Fang, J. Y., and Yoda, K. (1991). Climate and vegetation in China V. Effect of climatic factors on the upper limit of distribution of evergreen broadleaf forest. Ecol. Res. 6, 113–125. doi: 10.1007/bf02353874
Fehrer, J., Gemeinholzer, B., Chrtek, J., and Bräutigam, S. (2007). Incongruent plastid and nuclear DNA phylogenies reveal ancient intergeneric hybridization in Pilosella hawkweeds (Hieracium, Cichorieae, Asteraceae). Mol. Phylogenet. Evol. 42, 347–361. doi: 10.1016/j.ympev.2006.07.004
Feliner, G. N., and Rosselló, J. A. (2007). Better the devil you know? Guidelines for insightful utilization of nrDNA ITS in species-level evolutionary studies in plants. Mol. Phylogenet. Evol. 44, 911–919. doi: 10.1016/j.ympev.2007.01.013
Feng, L., Zheng, Q. J., Qian, Z. Q., Yang, J., Zhang, Y. P., Li, Z. H., et al. (2016). Genetic structure and evolutionary history of three Alpine Sclerophyllous oaks in East Himalaya-Hengduan Mountains and adjacent regions. Front. Plant Sci. 7:1688. doi: 10.3389/fgls.2016.01688
Gao, T., Yao, H., Song, J. Y., Zhu, Y. J., Liu, C., and Chen, S. L. (2010). Evaluating the feasibility of using candidate DNA barcodes in discriminating species of the large Asteraceae family. BMC Evol. Biol. 10:324. doi: 10.1186/1471-2148-10-324
Gaut, B. S., Morton, B. R., McCaig, B. C., and Clegg, M. T. (1996). Substitution rate comparisons between grasses and palms: synonymous rate differences at the nuclear gene Adh parallel rate differences at the plastid gene rbcL. Proc. Natl. Acad. Sci. U.S.A. 93, 10274–10279. doi: 10.1073/pnas.93.19.10274
Goldstein, P. Z., and DeSalle, R. (2011). Integrating DNA barcode data and taxonomic practice: determination, discovery, and description. Bioessays 33, 135–147. doi: 10.1002/bies.201000036
Gonzalez, M. A., Baraloto, C., Engel, J., Mori, S. A., Pétronelli, P., Riéra, B., et al. (2009). Identification of Amazonian trees with DNA barcodes. PLoS One 4:e7483. doi: 10.137/journal.pone.0007483
Graur, D., and Li, W. H. (2000). Fundamentals of Molecular Evolution, 2nd Edn. Sunderland, MA: Sinauer Associates Inc.
Gugger, P. F., and Cavender-Bares, J. (2013). Molecular and morphological support for a Florida origin of the Cuban oak. J. Biogeogr. 40, 632–645. doi: 10.1111/j.1365-2699.2011.02610.x
Guo, X. D., Wang, H. F., Bao, L., Wang, T. M., Bai, W. N., Ye, J. W., et al. (2014). Evolutionary history of a widespread tree species Acer mono in East Asia. Ecol. Evol. 4, 4332–4345. doi: 10.1002/ece3.1278
Guyette, R. P., Muzika, R.-M., Kabrick, J., and Stambaugh, M. C. (2004). A Perspective on Quercus Life History Characteristics and Forest Disturbance. Gen. Tech. Rep. SRS-73. Asheville, NC: Department of Agriculture, 138–142.
Hassel, K., Segreto, R., and Ekrem, T. (2013). Restricted variation in plant barcoding markers limits identification in closely related bryophyte species. Mol. Ecol. Resour. 13, 1047–1057. doi: 10.1111/1755-0998.12074
Hebert, P. D., Cywinska, A., and Ball, S. L. (2003). Biological identifications through DNA barcodes. Proc. R. Soc. B Biol. Sci. 270, 313–321. doi: 10.1098/rspb.2002.2218
Hipp, A. L., Eaton, D. A., Cavender-Bares, J., Fitzek, E., Nipper, R., and Manos, P. S. (2014). A framework phylogeny of the American oak clade based on sequenced RAD data. PLoS One 9:e93975. doi: 10.1371/journal.pone.0093975
Hollingsworth, P. M. (2007). DNA barcoding: potential users. Genomics Soc. Policy 3, 44–47. doi: 10.1186/1746-5354-3-2-44
Hollingsworth, P. M., Graham, S. W., and Little, D. P. (2011). Choosing and using a plant DNA barcode. PLoS One 6:e19254. doi: 10.1371/journal.pone.0019254
Hollingsworth, P. M., Li, D. Z., van der Bank, M., and Twyford, A. D. (2016). Telling plant species apart with DNA: from barcodes to genomes. Philos. Trans. R. Soc. Lond. B Biol. Sci. 371:20150338. doi: 10.1098/rstb.2015.0338
Huang, C., Zhang, Y., and Bartholomew, B. (1999). “Fagaceae,” in Flora of China 4 Cycadaceae through Fagaceae, eds Z. Wu and P. Raven (Beijing: Science Press), 314–400.
Hubert, F., Grimm, G. W., Jousselin, E., Berry, V., Franc, A., and Kremer, A. (2014). Multiple nuclear genes stabilize the phylogenetic backbone of the genus Quercus. Syst. Biodivers. 12, 405–423. doi: 10.1080/14772000.2014.941037
Hughes, C. E., Eastwood, R. J., and Bailey, C. D. (2006). From famine to feast? Selecting nuclear DNA sequence loci for plant species-level phylogeny reconstruction. Philos. Trans. R. Soc. Lond. B Biol. Sci. 361, 211–225. doi: 10.1098/rstb.2005.1735
Jiang, L., Xu, K., Fan, Q., and Peng, H. (2017). A new species of Ilex (Aquifoliaceae) from Jiangxi Province, China, based on morphological and molecular data. Phytotaxa 298, 147–157. doi: 10.11646/phytotaxa.298.2.4
Jiang, X. L., An, M., Zheng, S. S., Deng, M., and Su, Z. H. (2018). Geographical isolation and environmental heterogeneity contribute to the spatial genetic patterns of Quercus kerrii (Fagaceae). Heredity 120, 219–233. doi: 10.1038/s41437-017-0012-7
Jiang, X. L., Deng, M., and Li, Y. (2016). Evolutionary history of subtropical evergreen broad-leaved forest in Yunnan Plateau and adjacent areas: an insight from Quercus schottkyana (Fagaceae). Tree Genet. Genomes 12:104. doi: 10.1007/s11295-016-1063-2
Kormanik, P. P., Sung, S.-J. S., Kormanik, T., Tibbs, T., and Zarnoch, S. J. (2004). Northern Red Oak from Acorns to Acorns in 8 Years or Less. Gen. Tech. Rep. SRS 71. Asheville, NC: Department of Agriculture Forest Service, 555–558.
Kress, W. J., Erickson, D. L., Jones, F. A., Swenson, N. G., Perez, R., Sanjur, O., et al. (2009). Plant DNA barcodes and a community phylogeny of a tropical forest dynamics plot in Panama. Proc. Natl. Acad. Sci. U.S.A. 106, 18621–18626. doi: 10.1073/pnas.0909820106
Kress, W. J., Erickson, D. L., Swenson, N. G., Thompson, J., Uriarte, M., and Zimmerman, J. K. (2010). Advances in the use of DNA barcodes to build a community phylogeny for tropical trees in a Puerto Rican forest dynamics plot. PLoS One 5:e15409. doi: 10.1371/journal.pone.0015409
Kress, W. J., Wurdack, K. J., Zimmer, E. A., Weigt, L. A., and Janzen, D. H. (2005). Use of DNA barcodes to identify flowering plants. Proc. Natl. Acad. Sci. U.S.A. 102, 8369–8374. doi: 10.1073/pnas.0503123102
Kumar, S., Stecher, G., and Tamura, K. (2016). MEGA7: molecular evolutionary genetics analysis version 7.0 for bigger datasets. Mol. Biol. Evol. 33, 1870–1874. doi: 10.1093/molbev/msw054
Lahaye, R., van der Bank, M., Bogarin, D., Warner, J., Pupulin, F., Gigot, G., et al. (2008). DNA barcoding the floras of biodiversity hotspots. Proc. Natl. Acad. Sci. U.S.A. 105, 2923–2928. doi: 10.1073/pnas.0709936105
Laiou, A., Mandolini, L. A., Piredda, R., Bellarosa, R., and Simeone, M. C. (2013). DNA barcoding as a complementary tool for conservation and valorisation of forest resources. Zookeys 365, 197–213. doi: 10.3897/zookeys.365.5670
Lee, W., Koh, S. H., Choi, W. I., Jung, C. S., Kim, I. K., Byun, B. K., et al. (2012). Barcoding forest insect pests in South Korea: constructing a basic endemic species dataset. J. Asia Pac. Entomol. 15, 363–368. doi: 10.1016/j.aspen.2012.01.008
Leroy, T., Roux, C., Villate, L., Bodénès, C., Romiguier, J., Paiva, J. A., et al. (2017). Extensive recent secondary contacts between four European white oak species. New Phytol. 214, 865–878. doi: 10.1111/nph.14413
Li, X. W., Yang, Y., Henry, R. J., Rossetto, M., Wang, Y. T., and Chen, S. L. (2015). Plant DNA barcoding: from gene to genome. Bacteriol. Rev. 90, 157–166. doi: 10.1111/brv.12104
Liang, Y. Y., Liu, J., Huang, Y. S., and Lin, C. R. (2016). Aspidistra erythrocephala sp. nov. (Asparagaceae) from Guangxi, China. Phytotaxa 247, 295–298. doi: 10.1146/phytotaxa.247.4.9
Librado, P., and Rozas, J. (2009). DnaSP v5: a software for comprehensive analysis of DNA polymorphism data. Bioinformatics 25, 1451–1452. doi: 10.1093/bioinformatics/btp187
Little, D. P. (2011). DNA barcode sequence identification incorporating taxonomic hierarchy and within taxon variability. PLoS One 6:e20552. doi: 10.1371/journal.pone.002052
Little, D. P., and Stevenson, D. W. (2007). A comparison of algorithms for the identification of specimens using DNA barcodes: examples from gymnosperms. Cladistics 23, 1–21. doi: 10.1111/j.1096-0031.2006.00126.x
Liu, J., Moller, M., Gao, L. M., Zhang, D. Q., and Li, D. Z. (2011). DNA barcoding for the discrimination of Eurasian yews (Taxus L., Taxaceae) and the discovery of cryptic species. Mol. Ecol. Resour. 11, 89–100. doi: 10.1111/j.1755-0998.2010.02907.x
Liu, J., Yan, H. F., Newmaster, S. G., Pei, N., Ragupathy, S., and Ge, X. J. (2015). The use of DNA barcoding as a tool for the conservation biogeography of subtropical forests in China. Divers. Distrib. 21, 188–199. doi: 10.1111/ddi.12276
López-Pujol, J., Zhang, F. M., and Ge, S. (2006). Plant biodiversity in China: richly varied, endangered, and in need of conservation. Biodivers. Conserv. 15, 3983–4026. doi: 10.1007/s10531-005-3015-2
Lumaret, R., Mir, C., Michaud, H., and Raynal, V. (2002). Phylogeographical variation of chloroplast DNA in holm oak (Quercus ilex L.). Mol. Ecol. 11, 2327–2336. doi: 10.1046/j.1365-294X.2002.01611.x
Luo, Y., and Zhou, Z. (2000). Phytogeography of Quercus subg. Cyclobalanopsis. Acta Bot. Yunnanica 23, 1–16. doi: 10.3969/j.issn.2095-0845.2001.01.001
Luo, Y., and Zhou, Z. K. (2002). Leaf architecture in Quercus subgenus Cyclobalanopsis (Fagaceae) from China. Bot. J. Linn. Soc. 140, 283–295. doi: 10.1046/j.1095-8339.2002.00097.x
Lynch, M. (1997). Mutation accumulation in nuclear, organelle, and prokaryotic transfer RNA genes. Mol. Biol. Evol. 14, 914–925. doi: 10.1093/oxfordjournals.molbev.a025834
Ma, C. L. (2006). Phylogeny and Biogeography of Quercus Sect. Heterobalanus. Ph.D. dissertation, Kunming Institute of Botany, Kunming.
Ma, C. L., and Zhou, Z. K. (2005). Effect of ITS pseudogene on the phylogenetic study of Quercus (Fagaceae) and its revelation on the plant molecular phylogenetics. Acta Bot. Yunnanica 28, 127–132. doi: 10.3969/j.issn.2095-0845.2006.02.007
Manos, P. S., Doyle, J. J., and Nixon, K. C. (1999). Phylogeny, biogeography, and processes of molecular differentiation in Quercus subgenus Quercus (Fagaceae). Mol. Phylogenet. Evol. 12, 333–349. doi: 10.1006/mpev.1999.0614
Martin, A. P., and Palumbi, S. R. (1993). Body size, metabolic rate, generation time, and the molecular clock. Proc. Natl. Acad. Sci. U.S.A. 90, 4087–4091. doi: 10.1073/pnas.90.9.4087
Mayol, M., and Rosselló, J. A. (2001). Why nuclear ribosomal DNA spacers (ITS) tell different stories in Quercus. Mol. Phylogenet. Evol. 19, 167–176. doi: 10.1006/mpev.2001.0934
McVay, J. D., Hipp, A. L., and Manos, P. S. (2017). A genetic legacy of introgression confounds phylogeny and biogeography in oaks. Proc. R. Soc. B Biol. Sci. 284:20170300. doi: 10.1098/rspb.2017.0300
Mediavilla, S., and Escudero, A. (2003). Relative growth rate of leaf biomass and leaf nitrogen content in several Mediterranean woody species. Plant Ecol. 168, 321–332. doi: 10.1093/aob/mcl284
Meier, R., Shiyang, K., Vaidya, G., and Ng, P. K. (2006). DNA barcoding and taxonomy in Diptera: a tale of high intraspecific variability and low identification success. Syst. Biol. 55, 715–728. doi: 10.1080/10635150600969864
Meier, R., Zhang, G., and Ali, F. (2008). The use of mean instead of smallest interspecific distances exaggerates the size of the “barcoding gap” and leads to misidentification. Syst. Biol. 57, 809–813. doi: 10.1080/10635150802406343
Meng, H. H., Su, T., Gao, X. Y., Li, J., Jiang, X. L., Sun, H., et al. (2017). Warm-cold colonization: response of oaks to uplift of the Himalaya-Hengduan Mountains. Mol. Ecol. 26, 3276–3294. doi: 10.1111/mec.14092
Meyer, C. P., and Paulay, G. (2005). DNA barcoding: error rates based on comprehensive sampling. PLoS Biol. 3:e422. doi: 10.1371/journal.pbio.0030422
Miwa, H., Odrzykoski, I. J., Matsui, A., Hasegawa, M., Akiyama, H., Jia, Y., et al. (2009). Adaptive evolution of rbcL in Conocephalum (Hepaticae, bryophytes). Gene 441, 169–175. doi: 10.1016/j.gene.2008.11.020
Montecchio, L., and Faccoli, M. (2014). First record of thousand cankers disease Geosmithia morbida and walnut twig beetle Pityophthorus juglandis on Juglans nigra in Europe. Plant Dis. 98, 696–696. doi: 10.1094/PDIS-10-13-1027-PDN
Moran, E. V., Willis, J., and Clark, J. S. (2012). Genetic evidence for hybridization in red oaks (Quercus sect. Lobatae, Fagaceae). Am. J. Bot. 99, 92–100. doi: 10.3732/ajb.1100023
Muir, G., and Filatov, D. (2007). A selective sweep in the chloroplast DNA of dioecious Silene (Section Elisanthe). Genetics 177, 1239–1247. doi: 10.1534/genetics.107.071969
Myers, N., Mittermeier, R. A., Mittermeier, C. G., Da Fonseca, G. A., and Kent, J. (2000). Biodiversity hotspots for conservation priorities. Nature 403, 853–858. doi: 10.1038/35002501
Nei, M., and Li, W. H. (1979). Mathematical model for studying genetic variation in terms of restriction endonucleases. Proc. Natl. Acad. Sci. U.S.A. 76, 5269–5273. doi: 10.1073/pnas.76.10.5269
Neophytou, C., Aravanopoulos, F. A., Fink, S., and Dounavi, A. (2011a). Interfertile oaks in an island environment. II. Limited hybridization between Quercus alnifolia Poech and Q. coccifera L. in a mixed stand. Eur. J. For. Res. 130, 623–635. doi: 10.1007/s10342-010-0454-4
Neophytou, C., Dounavi, A., Fink, S., and Aravanopoulos, F. A. (2011b). Interfertile oaks in an island environment: I. High nuclear genetic differentiation and high degree of chloroplast DNA sharing between Q. alnifolia and Q. coccifera in Cyprus. A multipopulation study. Eur. J. For. Res. 130, 543–555. doi: 10.1007/s10342-010-0454-4
Neophytou, C. H., Palli, G., Dounavi, A., and Aravanopoulos, F. A. (2007). Morphological differentiation and hybridization between Quercus alnifolia Poech and Quercus coccifera l. (Fagaceae) in Cyprus. Silvae Genet. 56, 271–277. doi: 10.1515/sg-2007-0038
Newmaster, S., Fazekas, A., Steeves, R., and Janovec, J. (2008). Testing candidate plant barcode regions in the Myristicaceae. Mol. Ecol. Resour. 8, 480–490. doi: 10.1111/j.1471-8286.2007.02002.x
Newmaster, S. G., and Ragupathy, S. (2009). Testing plant barcoding in a sister species complex of pantropical Acacia (Mimosoideae. Fabaceae). Mol. Ecol. Resour. 9(Suppl. 1), 172–180. doi: 10.1111/j.1755-0998.2009.02642.x
Nixon, K. (2006). “Globe and neotropical distribution and diversity of oak (genus Quercus) and oak forests,” in Ecology and Conservation of Neotropical Montane Oak Forests, ed. M. Kappella (Berlin: Springer), 3–13.
Nixon, K. C. (1993). Infrageneric classification of Quercus (Fagaceae) and typification of sectional names. Ann. Sci. For. 50(Suppl. 1), 25s–34s. doi: 10.1051/forest:19930701
Oh, S.-H., and Manos, P. S. (2008). Molecular phylogenetics and cupule evolution in Fagaceae as inferred from nuclear CRABS CLAW sequences. Taxon 57, 434–451.
Ohyama, M., Baba, K. I., and Itoh, T. (2001). Wood identification of Japanese Cyclobalanopsis species (Fagaceae) based on DNA polymorphism of the intergenic spacer between trnT and trnL 5’ exon. J. Wood Sci. 47, 81–86. doi: 10.1007/bf00780554
Peakall, R., and Smouse, P. E. (2012). GenAlEx 6.5: genetic analysis in excel. Population genetic software for teaching and research—an update. Mol. Ecol. Resour. 28, 2537–2539. doi: 10.1111/j.1471-8286.2005.01155.x
Percy, D. M., Argus, G. W., Cronk, Q. C., Fazekas, A. J., Kesanakurti, P. R., Burgess, K. S., et al. (2014). Understanding the spectacular failure of DNA barcoding in willows (Salix): does this result from a trans-specific selective sweep? Mol. Ecol. 23, 4737–4756. doi: 10.1111/mec.12837
Petit, R. J., Carlson, J., Curtu, A. L., Loustau, M. L., Plomion, C., González-Rodríguez, A., et al. (2013). Fagaceae trees as models to integrate ecology, evolution and genomics. New Phytol. 197, 369–371. doi: 10.1111/nph.12089
Petit, R. J., Csaikl, U. M., Bordács, S., Burg, K., Coart, E., Cottrell, J., et al. (2002). Chloroplast DNA variation in European white oaks: phylogeography and patterns of diversity based on data from over 2600 populations. For. Ecol. Manage. 156, 5–26. doi: 10.1016/S0378-1127(01)00645-4
Pham, K. K., Hipp, A. L., Manos, P. S., and Cronn, R. C. (2017). A time and a place for everything: phylogenetic history and geography as joint predictors of oak plastome phylogeny. Genome 60, 720–732. doi: 10.1139/gen-2016-0191
Phengklai, C., Santisuk, T., and Larsen, K. (2008). “Fagaceae,” in Flora of Thailand, eds T. Santisuk and K. Larsen (Bangkok: The Forest Herbarium), 179–410.
Piredda, R., Simeone, M. C., Attimonelli, M., Bellarosa, R., and Schirone, B. (2011). Prospects of barcoding the Italian wild dendroflora: oaks reveal severe limitations to tracking species identity. Mol. Ecol. Resour. 11, 72–83. doi: 10.1111/j.1755-0998.2010.02900.x
Pitman, N. C., Mogollón, H., Dávila, N., Ríos, M., García-Villacorta, R., Guevara, J., et al. (2008). Tree community change across 700 km of lowland Amazonian forest from the Andean foothills to Brazil. Biotropica 40, 525–535. doi: 10.1111/j.1744-7429.2008.00424.x
Posada, D., and Crandall, K. A. (1998). Modeltest: testing the model of DNA substitution. Bioinformatics 14, 817–818. doi: 10.1093/bioinformatics/14.9.817
Puillandre, N., Lambert, A., Brouillet, S., and Achaz, G. (2012). ABGD, automatic barcode gap discovery for primary species delimitation. Mol. Ecol. Resour. 21, 1864–1877. doi: 10.1111/j.1365-294X.2011.05239.x
Qi, X. S., Chen, C., Comes, H. P., Sakaguchi, S., Liu, Y. H., Tanaka, N., et al. (2012). Molecular data and ecological niche modelling reveal a highly dynamic evolutionary history of the East Asian Tertiary relict Cercidiphyllum (Cercidiphyllaceae). New Phytol. 196, 617–630. doi: 10.1111/j.1469-8137.2012.04242.x
R Core Team (2015). R: A Language and Environment for Statistical Computing. Vienna: R Foundation for Statistical Computing.
Ronquist, F., Teslenko, M., Van Der Mark, P., Ayres, D. L., Darling, A., Höhna, S., et al. (2012). MrBayes 3.2: efficient Bayesian phylogenetic inference and model choice across a large model space. Syst. Biol. 61, 539–542. doi: 10.1093/sysbio/sys029
Schaal, B., Hayworth, D., Olsen, K. M., Rauscher, J., and Smith, W. (1998). Phylogeographic studies in plants: problems and prospects. Mol. Ecol. 7, 465–474.
Shapcott, A., Forster, P. I., Guymer, G. P., McDonald, W. J., Faith, D. P., Erickson, D., et al. (2015). Mapping biodiversity and setting conservation priorities for SE Queensland’s rainforests using DNA barcoding. PLoS One 10:e0122164. doi: 10.1371/journal.pone.o122164
Simeone, M. C., Grimm, G. W., Papini, A., Vessella, F., Cardoni, S., Tordoni, E., et al. (2016). Plastome data reveal multiple geographic origins of Quercus Group Ilex. PeerJ 4:e1897. doi: 10.7287/peerj.preprints.1615
Simeone, M. C., Piredda, R., Papini, A., Vessella, F., and Schirone, B. (2013). Application of plastid and nuclear markers to DNA barcoding of Euro-Mediterranean oaks (Quercus, Fagaceae): problems, prospects and phylogenetic implications. Bot. J. Linn. Soc. 172, 478–499. doi: 10.1111/boj.12059
Song, Y., Deng, M., Hipp, A. L., and Li, Q. (2015). Leaf morphological evidence of natural hybridization between two oak species (Quercus austrocochinchinensis and Q. kerrii) and its implications for conservation management. Eur. J. For. Res. 134, 139–151. doi: 10.1007/s10342-014-0839-x
Tamaki, I., and Okada, M. (2014). Genetic admixing of two evergreen oaks, Quercus acuta and Q. sessilifolia (subgenus Cyclobalanopsis), is the result of interspecific introgressive hybridization. Tree Genet. Genomes 10, 989–999. doi: 10.1007/s11295-014-0737-x
Tang, C. Q. (2010). Subtropical montane evergreen broad-leaved forests of Yunnan, China: diversity, succession dynamics, human influence. Front. Earth Sci. China 4, 22–32. doi: 10.1007/s11707-009-0057-x
Tao, T. (2010). BLAST® Help. Standalone BLAST Setup for Unix. Bethesda, MD: National Center for Biotechnology Information.
Toumi, L., and Lumaret, R. (2010). Genetic variation and evolutionary history of holly oak: a circum-Mediterranean species-complex [Quercus coccifera L./Q. calliprinos (Webb) Holmboe, Fagaceae]. Plant Syst. Evol. 290, 159–171. doi: 10.1007/s00606-010-0358-2
Valentini, A., Pompanon, F., and Taberlet, P. (2009). DNA barcoding for ecologists. Trends Ecol. Evol. 24, 110–117. doi: 10.1016/j.tree.2008.09.011
Van De Wiel, C., Van Der Schoot, J., Van Valkenburg, J., Duistermaat, H., and Smulders, M. (2009). DNA barcoding discriminates the noxious invasive plant species, floating pennywort (Hydrocotyle ranunculoides Lf), from non-invasive relatives. Mol. Ecol. Resour. 9, 1086–1091. doi: 10.1111/j.1755-0998.2009.02547.x
van Velzen, R., Weitschek, E., Felici, G., and Bakker, F. T. (2012). DNA barcoding of recently diverged species: relative performance of matching methods. PLoS One 7:e30490. doi: 10.1371/journal.pone.0030490
Veldman, S., Otieno, J., Gravendeel, B., van Andel, T., and de Boer, H. (2014). “Conservation of endangered wild harvested medicinal plants: use of DNA barcoding,” in Novel Plant Bioresources, ed. A. Gurib-Fakim (Chichester: John Wiley & Sons), 81–88. doi: 10.1002/9781118460566.ch6
Vilgalys, R. (2003). Taxonomic misidentification in public DNA databases. New Phytol. 160, 4–5. doi: 10.1046/j.1469-8137.2003.00894.x
Virgilio, M., Backeljau, T., Nevado, B., and De Meyer, M. (2010). Comparative performances of DNA barcoding across insect orders. BMC Bioinformatics 11:206. doi: 10.1186/1471-2105-11-206
Vitelli, M., Vessella, F., Cardoni, S., Pollegioni, P., Denk, T., Grimm, G. W., et al. (2017). Phylogeographic structuring of plastome diversity in Mediterranean oaks (Quercus Group Ilex, Fagaceae). Tree Genet. Genomes 13:3. doi: 10.1007/s11295-016-1086-8
von Cräutlein, M., Korpelainen, H., Pietiläinen, M., and Rikkinen, J. (2011). DNA barcoding: a tool for improved taxon identification and detection of species diversity. Biodivers. Conserv. 20, 373–389. doi: 10.1007/s10531-010-9964-0
Wang, C. W., Yang, B. Y., and Jin, X. H. (2017). Herminium motuoensis sp. nov. (Orchidaceae, Orchidoideae), a new species from Tibet, China. Phytotaxa 329, 197–200. doi: 10.11646/phytotaxa.329.2.14
Wang, D. Y., Wang, Q., Wang, Y. L., Xiang, X. G., Huang, L. Q., and Jin, X. H. (2017). Evaluation of DNA barcodes in Codonopsis (Campanulaceae) and in some large angiosperm plant genera. PLoS One 12:e0170286. doi: 10.1371/journal.pone.0170286
Wang, J. X., Liang, S. C., Wei, F., and Li, J. W. (2011). Spatial Pattern of Quercus fabri population in Karst Hills of Kaili in Guizhou. J. Anhui Agric. Sci. 39, 9639–9642. doi: 10.3969/j.issn.0517-6611.2011.16.068
Wang, Z. L., and Gao, X. M. (2005). The regeneration of Quercus aliena var. acuteserrata: acorn status, seedling pool and size structure. J. Northwest A & F Univ. 25, 986–993. doi: 10.4028/www.scientific.net/amm.52-54.949
Weitschek, E., Velzen, R., Felici, G., and Bertolazzi, P. (2013). BLOG 2.0: a software system for character-based species classification with DNA Barcode sequences. What it does, how to use it. Mol. Ecol. Resour. 13, 1043–1046. doi: 10.1111/1755-0998.12073
Xiao, Y., Li, C., Hsieh, T. Y., Tian, D. K., Zhou, J. J., Zhang, D. G., et al. (2015). Eutrema bulbiferum (Brassicaceae), a new species with bulbils from Hunan, China. Phytotaxa 219, 233–242. doi: 10.11646/phytotaxa.219.3.3
Xu, J., Deng, M., Jiang, X. L., Westwood, M., Song, Y. G., and Turkington, R. (2015). Phylogeography of Quercus glauca (Fagaceae), a dominant tree of East Asian subtropical evergreen forests, based on three chloroplast DNA interspace sequences. Tree Genet. Genomes 11:805. doi: 10.1007/s11295-014-0805-2
Xu, J., Jiang, X. L., Deng, M., Westwood, M., Song, Y. G., and Zheng, S. S. (2016). Conservation genetics of rare trees restricted to subtropical montane cloud forests in southern China: a case study from Quercus arbutifolia (Fagaceae). Tree Genet. Genomes 12:90. doi: 10.1007/s11295-016-1048-1
Yan, L. J., Liu, J., Moller, M., Zhang, L., Zhang, X. M., Li, D. Z., et al. (2015). DNA barcoding of Rhododendron (Ericaceae), the largest Chinese plant genus in biodiversity hotspots of the Himalaya-Hengduan Mountains. Mol. Ecol. Resour. 15, 932–944. doi: 10.1111/1755-0998.12353
Yang, J., Vázquez, L., Chen, X., Li, H., Zhang, H., Liu, Z., et al. (2017). Development of chloroplast and nuclear DNA markers for Chinese oaks (Quercus subgenus Quercus) and assessment of their utility as DNA barcodes. Front. Plant Sci. 8:816. doi: 10.3389/fpls.2017.00816
Yang, Y. M., Tian, K., Hao, J. M., Pei, S. J., and Yang, Y. X. (2004). Biodiversity and biodiversity conservation in Yunnan, China. Biodivers. Conserv. 13, 813–826. doi: 10.1023/b:bioc.0000014464.80847.02
Yu, W. B., Huang, P. H., Ree, R. H., Liu, M. L., Li, D. Z., and Wang, H. (2011). DNA barcoding of Pedicularis L.(Orobanchaceae): evaluating four universal barcode loci in a large and hemiparasitic genus. J. Syst. Evol. 49, 425–437. doi: 10.1111/j.1759-6831.2011.00154.x
Zhao, J. F., Feng, D. J., and Lei, Y. F. (2007). Wood study on Quercus and Cyclobalanopsis of Fagaceae in Shaanxi Province. J. Northwest A & F Univ. 35, 196–202. doi: 10.3321/j.issn:1671-9387.2007.10.038
Zhou, Z. K., Wilkinson, H., and Wu, C. Y. (1995). Taxonomical and evolutionary implications of the leaf anatomy and architecture of Quercus L. subgenus Quercus from China. Cathaya 7, 1–34. doi: 10.1046/j.1095-8339.2002.00097.x
Keywords: cpDNA, DNA barcoding, genetic diversity, Quercus, section Cyclobalanopsis, section Ilex
Citation: Yan M, Xiong Y, Liu R, Deng M and Song J (2018) The Application and Limitation of Universal Chloroplast Markers in Discriminating East Asian Evergreen Oaks. Front. Plant Sci. 9:569. doi: 10.3389/fpls.2018.00569
Received: 26 January 2018; Accepted: 11 April 2018;
Published: 08 May 2018.
Edited by:
Rosane Garcia Collevatti, Universidade Federal de Goiás, BrazilReviewed by:
Andrea Cecilia Premoli, Universidad Nacional del Comahue, ArgentinaGlaucia Salles Cortopassi Buso, Embrapa Genetic Resources and Biotechnology, Brazil
Copyright © 2018 Yan, Xiong, Liu, Deng and Song. This is an open-access article distributed under the terms of the Creative Commons Attribution License (CC BY). The use, distribution or reproduction in other forums is permitted, provided the original author(s) and the copyright owner are credited and that the original publication in this journal is cited, in accordance with accepted academic practice. No use, distribution or reproduction is permitted which does not comply with these terms.
*Correspondence: Min Deng, ZGVuZ21pbkBzaWJzLmFjLmNu