- 1Key Laboratory of Vegetation Restoration and Management of Degraded Ecosystems, South China Botanical Garden, Chinese Academy of Sciences, Guangzhou, China
- 2College of Life Science, University of Chinese Academy of Sciences, Beijing, China
- 3Guangdong Provincial Key Laboratory of Applied Botany, South China Botanical Garden, Chinese Academy of Sciences, Guangzhou, China
- 4College of Natural Resources and Environment, South China Agricultural University, Guangzhou, China
Global warming could increase leaf transpiration and soil evaporation, which can potentially cause water deficit to plants. As valves, leaf stomata can control plant water loss and carbon gain, particularly under water stress conditions. To investigate the responses of stomata to elevated temperature in Schima superba and Syzygium rehderianum, two co-occurring subtropical forest dominant tree species, functional traits related to gas exchange, stomatal anatomy, and drought resistance were measured under control and warming environment (ca. 2°C higher). We found that leaf water potential at both predawn and midday significantly decreased for the two species grown under warming conditions compared with those grown in the control environment. Warming resulted in significant decrease of stomatal size in S. rehderianum, but had no obvious effect on that of S. superba. By contrast, stomatal density of S. superba significantly decreased under warming conditions, while non-significant change was observed for S. rehderianum. In addition, warming significantly reduced photosynthetic rate, stomatal conductance, and stomatal sensitivity to leaf water potential of S. superba, but had non-significant effects on those of S. rehderianum. Overall, our results demonstrated that, confronting water deficit caused by elevated temperature, the two co-occurring subtropical tree species responded differently through the adjustment of stomatal morphology and photosynthetic function. Consequently, S. rehderianum was able to maintain similar carbon assimilation as under control environment, while S. superba showed a decrease in carbon gain that might bring adverse effect on its dominancy in subtropical forest community under future climate change.
Introduction
Recent predictions of global climate change suggest that temperature would continue to increase in the future 100 years (Christensen et al., 2007; Intergovernmental Panel on Climate Change [IPCC], 2014). Regardless of future changes in precipitation, increased temperature would increase soil evaporation and leaf transpiration, thus resulting in potential water stress on plants. Over the last decade, field observational studies across forests worldwide have documented changes in forest structure and tree mortality, which have been attributed to climate warming and severe droughts (Adams et al., 2009; Williams et al., 2012; Anderegg et al., 2013; Zhou et al., 2013; McIntyre et al., 2015). However, the physiological mechanisms that govern the growth and survival of plants by climate warming are not well understood. It is known that stomata can function as valves to control the balance of water loss and carbon gain in plants, which primary determine key physiological processes of plants (Farquhar et al., 1980; Luomala et al., 2005). Thus, a better understanding of stomatal response to climate warming may provide deeper insights into the acclimation and adaptation of plants under global climate change scenarios.
It has been shown that stomatal traits are strongly affected by elevated temperature. Recently studies have indicated that plants acclimate to a warmer environment by adjusting their optimum for growth and photosynthesis (Way and Yamori, 2014; Scafaro et al., 2017; Drake et al., 2018), and the relationship between stomatal conductance and water potential and vapor pressure deficit (VPD; Mott and Peak, 2010; Marchin et al., 2016; Urban et al., 2017). But understanding of the effects of elevated temperature on anatomical traits of stomata, which are linked to gas exchange traits, is limited for tropical and subtropical trees. In general, plants may respond quickly to short-term temperature changes by adjusting the openness/closure of the stomatal pores (Zhou et al., 2010). By contrast, long-term (annual to decadal) environmental changes such as climate warming may affect stomatal aperture size, stomatal density, and the pattern of stomatal distribution in leaves (McElwain et al., 1999; Yan et al., 2017). As a result, the change in stomatal density may modify the number of sites available for gas exchange per unit leaf area, while change in stomatal size may modify stomatal conductance by changing stomatal pore area through which CO2 can enter the leaf (Maherali et al., 2002; Franks and Farquhar, 2007). Although it has been shown that elevated temperature may have effects on both stomatal size and density (Beerling and Chaloner, 1993; McElwain et al., 1999; Yan et al., 2017), the results have been quite variable. For instance, in contrast to increases of leaf stomatal density in response to warming (Fraser et al., 2009; Hill et al., 2014), decreases in stomatal density were also observed (Luomala et al., 2005). Besides, some studies showed no change in stomatal density upon warming treatment (Zheng et al., 2013; Kivimäenpää et al., 2017). Similarly, warming had diverse effects on stomatal size across species, such that either increases (Ferris et al., 1996; Zheng et al., 2013), decreases (Zhang et al., 2010), or no change (Apple et al., 2000; Hill et al., 2014) in stomatal size were reported. Therefore, in parallel to investigating the change in stomatal size and density under elevated temperature condition, monitoring the change in key physiological processes such as gas exchange and drought resistance may allow for a more comprehensive understanding of plant response and adaptation to warming.
In this study, we selected two co-occurring dominant species (Schima superba and Syzygium rehderianum) from a subtropical forest in southern China. As found in our previous studies at the same site (e.g., Zhu et al., 2013; Li et al., 2015), the canopy of S. superba can reach 8–10 m, while S. rehderianum is a shrub-like species that usually grows 3–5 m high in this forest. Open top chamber (OTC) facilities were employed such that treated plants experienced ca. 2°C higher atmosphere temperature compared with the control plants. Stomatal anatomical traits (stomatal size and density) were determined, in parallel to measurements of physiological traits that are related to gas exchange [photosynthetic rate, stomatal conductance, and water use efficiency (WUE)] and plant water status (leaf water potential at predawn and midday). Specifically, we asked: (i) how would warming affect stomatal anatomy of these two co-occurring dominant tree species in subtropical forests and (ii) would these two species respond differently to warming in terms of controlling water balance and carbon assimilation through stomatal regulation?
Materials and Methods
Study Site
This study was carried out at the Dinghushan Biosphere Reserve (23° 09′N–23° 11′N, 112° 30′E–112° 33′E; DBR), with an area of 1155 ha. DBR is located in the middle of Guangdong Province in southern China. It is characterized by a typical subtropical monsoon climate, with mean annual temperature ca. 21°C, ranging from 12.6°C in the coldest month (January) to 28.0°C in the warmest month (July). The mean annual precipitation is ca. 1900 mm, of which about 80% occurs in the wet season (through April to September).
Experimental Design and Treatments
Six pools were constructed using bricks and concrete. The pools were 3 m × 3 m in area, 0.8 m in depth, and 0.1 m high above the ground. Three of the six pools were sealed by transparent glass to form OTCs (3 m in height), and the rest were used as controls. Both control pools and OTCs receive only ambient precipitation. The chambers were heated by forced air blown over closed-loop resistance wires surrounding the OTCs at 1.5 m in height to simulate warming environment. Sensors were installed inside and outside the OTCs to monitor air temperature (HMP 155A, Vaisala, Finland), soil profile temperatures (CT 109, Campbell, United States), and volumetric soil water content (CS616, Campbell, United States) at 5 cm depth. Each meteorological observation was connected with a data logger (CR1000, Campbell, United States) to record data every 1 h. The chambers were heated year-round, both day and night, resulting in ca. 2°C higher of air temperature in the OTCs compared with the non-heated control pools.
Seedlings (1-year-old) of S. superba and S. rehderianum were collected, with six individuals of each study species transplanted into each of the OTCs and the control pools. Functional traits were measured after 1 year warming treatment.
Predawn and Midday Leaf Water Measurements
We determined plant water status by measuring leaf water potential at predawn (Ψpd) and midday (Ψmd) using a pressure chamber (PMS, Corvallis, OR, United States). Measurements were carried out between 06:30 and 07:30 for Ψpd and between 13:00 and 14:00 for Ψmd. A minimum of nine fully expanded mature leaves from three individuals per species in each OTC or the control pool were sampled for leaf water potential measurements.
Gas Exchange Measurement
Leaf gas exchange was measured between 9:00 and 11:00 using a portable photosynthesis system equipped with a CO2 injector (Li6400, Li-Cor, Lincoln, NE, United States). Based on preliminary trials, the photosynthetic photon flux density was set at 1500 μmol m-2s-1 to ensure that light-saturated photosynthesis rates were reached for the two study species. Ambient CO2 was maintained at 400 μmol mol-1 and leaf temperature at 25°C for all measurements. Before data were recorded, leaves were exposed to the above conditions for about 15 min to allow photosynthetic parameters to stabilize (Zotz and Mikona, 2003). Similar to the sampling way for leaf water potential measurements, nine fully expanded mature leaves from three individuals per species in each OTC or the control pool were selected, for the quantification of CO2 assimilation rate (An) and stomatal conductance (gs). Leaf-level WUE was calculated as WUE = An/Tr, where Tr is the leaf transpiration rate.
To test the relationship between stomatal conductance and leaf water potential, stomatal conductance, and VPD, nine fully expanded mature leaves from three individuals per species in each OTC or the control pool were selected and marked. Photon flux density and ambient CO2 concentration were set at 1500 μmol m-2s-1 and 400 μmol mol-1, respectively, and the measurement was carried out at their respective ambient conditions (control plants at control temperature and warmed plants at elevated temperature). We periodically measured gas exchange of all the marked leaves from 9:00 to 14:00 at an interval of an hour, each rotation the same marked leaves were measured at three times. Simultaneously, we cut leaves near the marked leaves to measured leaf water potential of each individual at each rotation.
Leaf Anatomic Traits’ Measurement
Stomatal anatomic traits were measured following the protocols described by Carins Murphy et al. (2012). In brief, cuticles were stained with diluted (c. 0.1%) aqueous toluidine blue, rinsed, mounted on microscope slides, covered with a cover slip, gently pressured with fine-point tweezers, and then immediately observed under a light microscope (YS100, Nikon, Tokyo, Japan). Stomatal density (D) and guard cell width (W) and length (L) were measured using an image analysis software (OPTPro 2012 4.0, Optec XTS20, Chongqing Optec Instrument, China). Stomatal size (S) was defined as guard length (L) multiplied by the total width (2W) of the guard cell pair (Franks et al., 2009).
Statistical Analysis
Trait values of the control and warming-treated plants of the two study species were compared using nested ANOVA. The difference in stomatal conductance to leaf water potential sensitivity was tested using analysis of covariance (ANCOVA). All statistical analyses were performed using SPSS 13.0 (SPSS Inc., Chicago, IL, United States).
Results
Environmental Variables
The monthly air and soil temperatures at the warming environment were distinctly higher than those at the control environment. The mean soil temperature at 5 cm in depth was 1.14°C higher in the warming environment than in the control environment (Figure 1A, P < 0.01). The mean soil moisture was not significantly lower in the warming environment than control environment (Figure 1A, P > 0.05). Upon the warming treatment, the mean, maximum, and minimum monthly air temperatures were 2.53, 2.90, and 1.83°C higher in the warming environment than those in the control environment, respectively (Figure 1B, P < 0.01). During gas exchange measurement, the mean, maximum, and minimum air temperatures were 2.35, 3.12, and 1.17°C higher in the warming environment than those in the control environment, respectively (Figure 1C, P < 0.05).
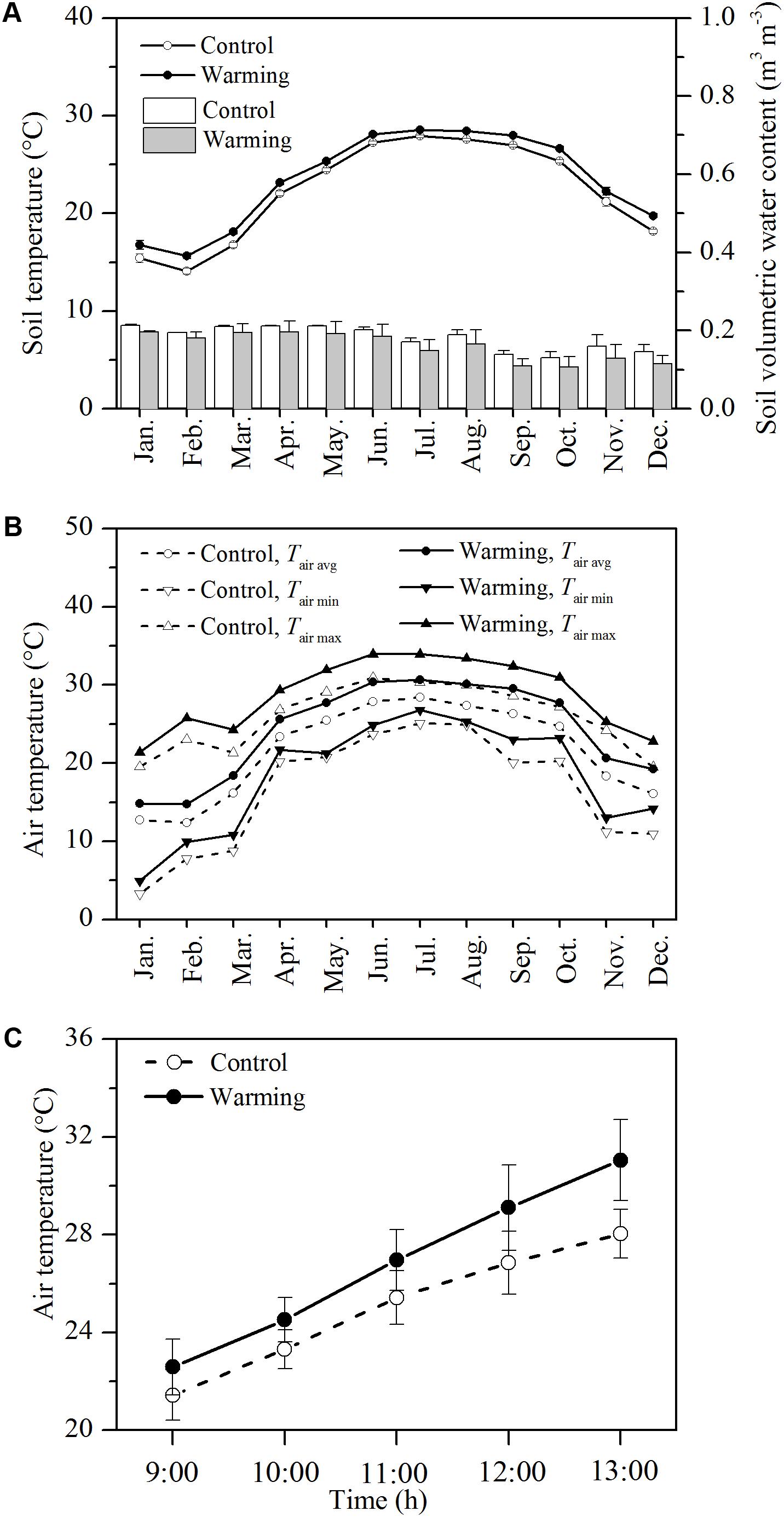
FIGURE 1. Monthly mean soil temperature (lines) and volumetric soil water content (A), daily mean air temperature during gas exchange measurement (B), monthly maximum, minimum, and mean air temperature (C) in the control and warming OTCs. Values are mean ± SD (n = 3 OTCs).
Predawn and Midday Leaf Water Potential
Leaf water potential at predawn and midday of the warming plants was significantly lower (more negative) than plants grown in control condition for both S. superba and S. rehderianum (P < 0.05; Figure 2).
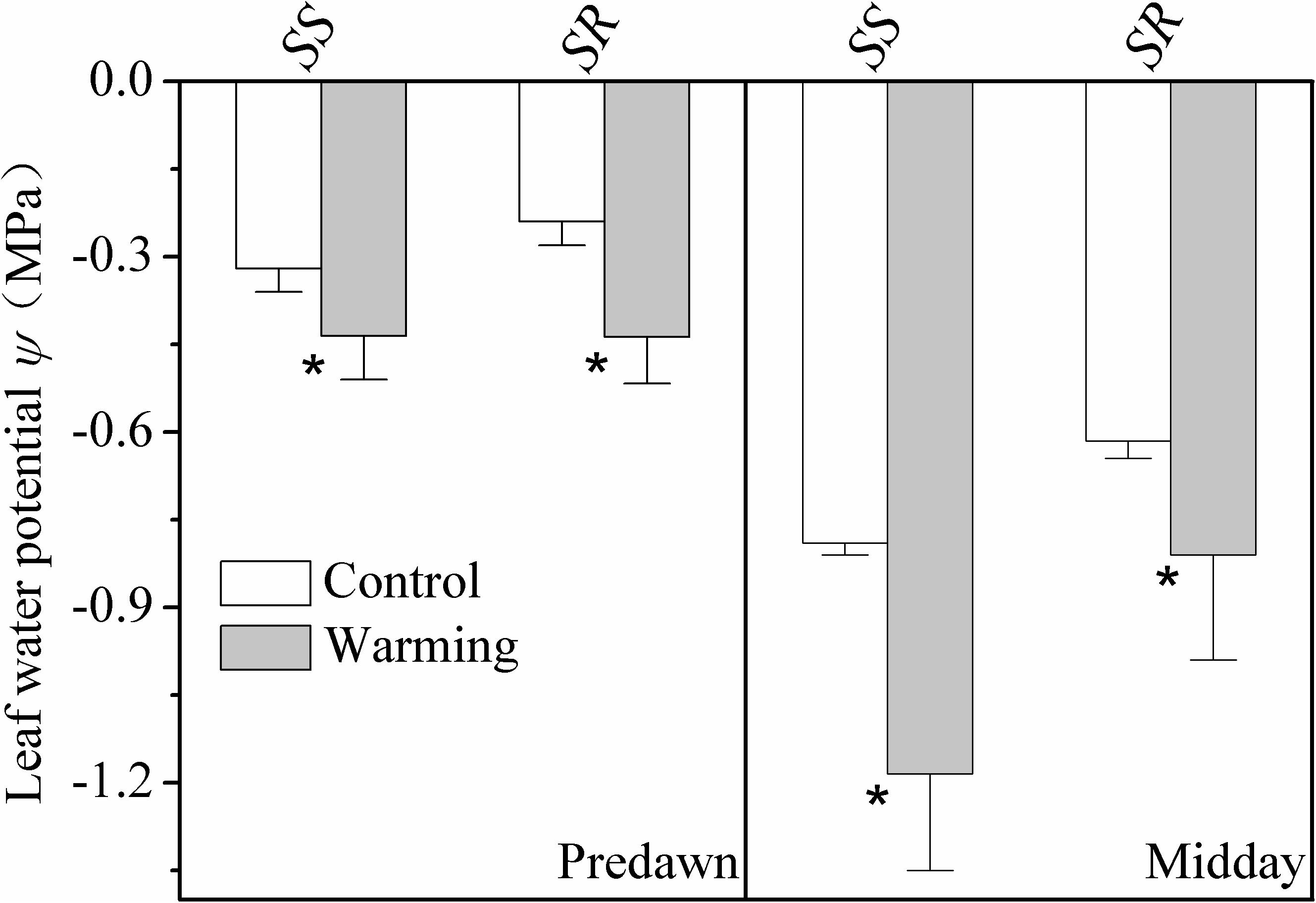
FIGURE 2. Leaf water potential at predawn (Ψpd) and midday (Ψmd) for the two study species grown in both control and warming environments. Values are mean ± SD (n = 9 individual trees). ∗Indicates the significant difference at P < 0.05 (nested ANOVA). SS and SR represent Schima superba and Syzygium rehderianum, respectively.
Stomatal Size and Density
Warming resulted in significant decrease (P < 0.05) of stomatal size in S. rehderianum, but had no obvious effect (P > 0.05) on that of S. superba (Figure 3A). By contrast, stomatal density of S. superba significantly decreased (P < 0.05) under warming conditions, while non-significant change was observed for S. rehderianum (Figure 3B).
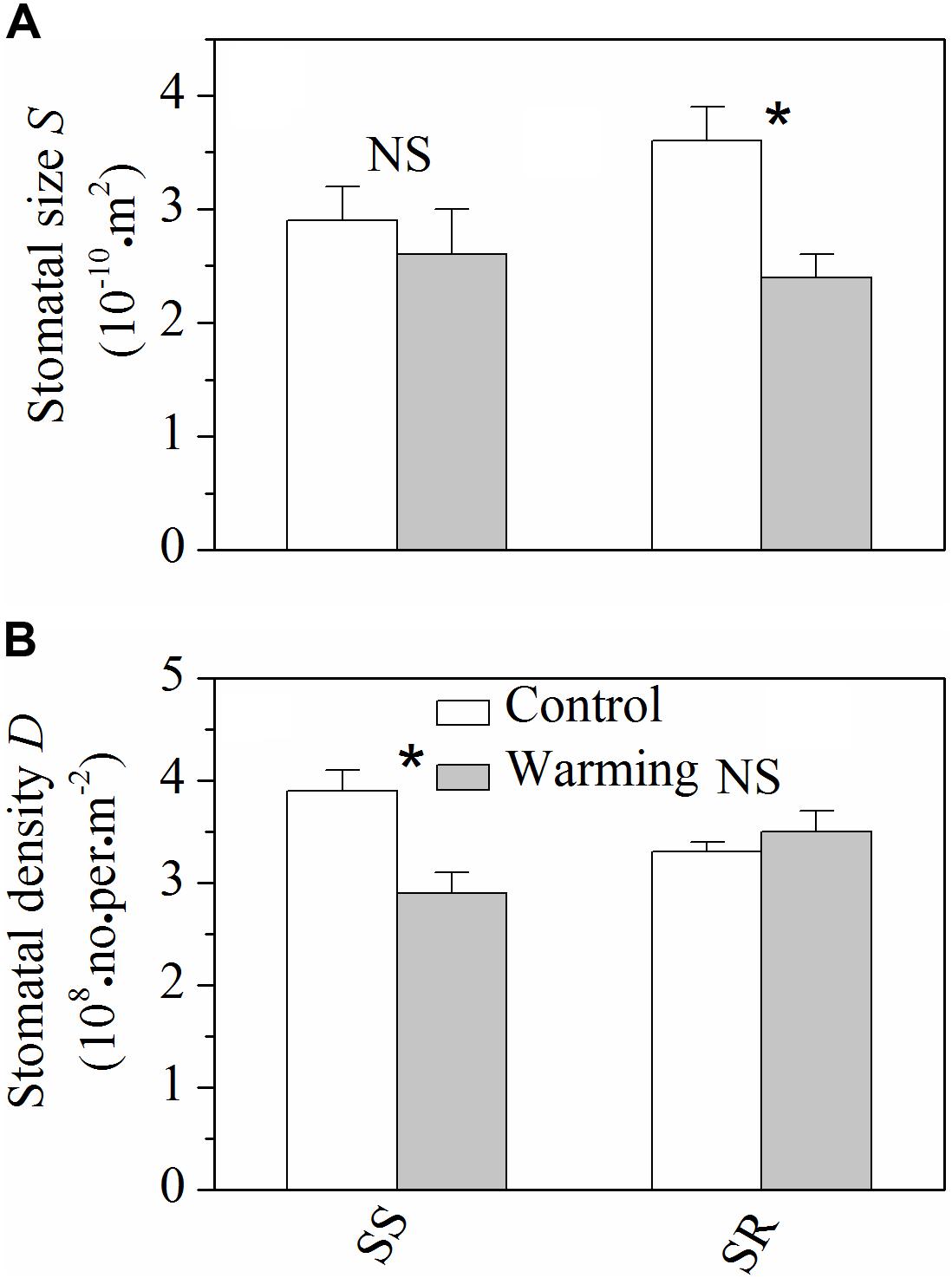
FIGURE 3. (A) Stomatal size (S) and (B) stomatal density (D) for the two study species grown in both control and warming environments. Values are mean ± SD (n = 9 trees). NS and ∗Indicate no significant difference and significant difference at P < 0.05 (nested ANOVA), respectively. SS and SR represent Schima superba and Syzygium rehderianum, respectively.
Leaf Gas Exchange
For gas exchange characteristics, warming caused significant decreases (P < 0.05) in photosynthetic rate, stomatal conductance, and WUE in S. superba, while no significant (P > 0.05) effects of warming on those of S. rehderianum were observed (Figure 4).
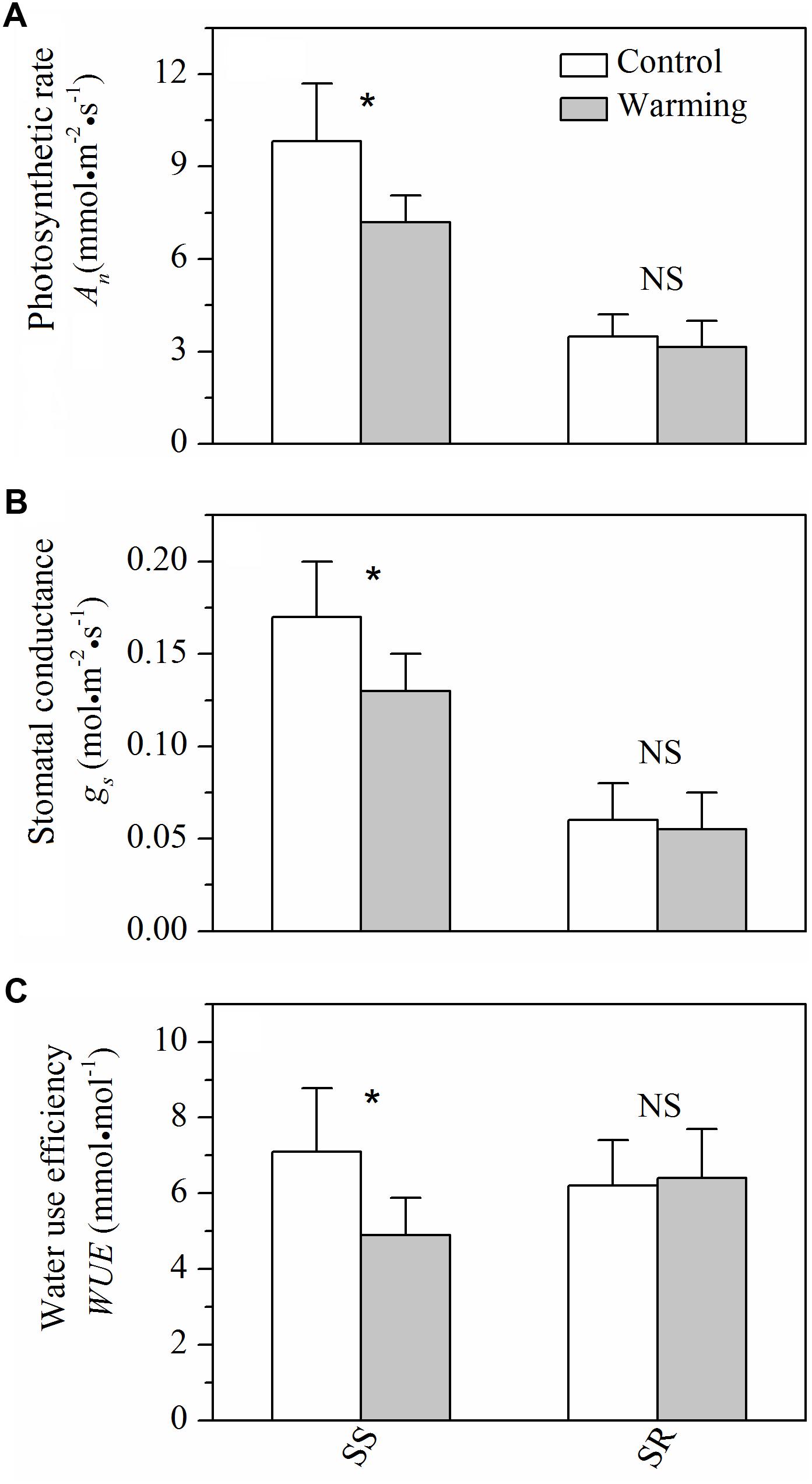
FIGURE 4. (A) Light-saturated photosynthetic rate (An), (B) stomatal conductance (gs), and (C) water use efficiency (WUE) for the two study species grown in both control and warming environments. Values are mean ± SD (n = 9 trees). NS and ∗Indicate no significant difference and significant difference at P < 0.05 (nested ANOVA), respectively. SS and SR represent Schima superba and Syzygium rehderianum, respectively.
To detect the sensitivity of stomatal conductance to leaf water potential and VPD, we fitted the relationship between stomatal conductance, leaf water potential, and VPD with linear models. We found that, for the two species, stomatal conductance was positively correlated with leaf water potential and negatively correlated with VPD both in warming and control conditions. However, both stomatal sensitivities to leaf water potential and to VPD were significantly higher (P < 0.05) in control than in warming environment for S. superba, as indicated by a steeper slope of the regression line, while the difference of stomatal sensitivity to leaf water potential and VPD was not significant (P > 0.05) for S. rehderianum (Figure 5).
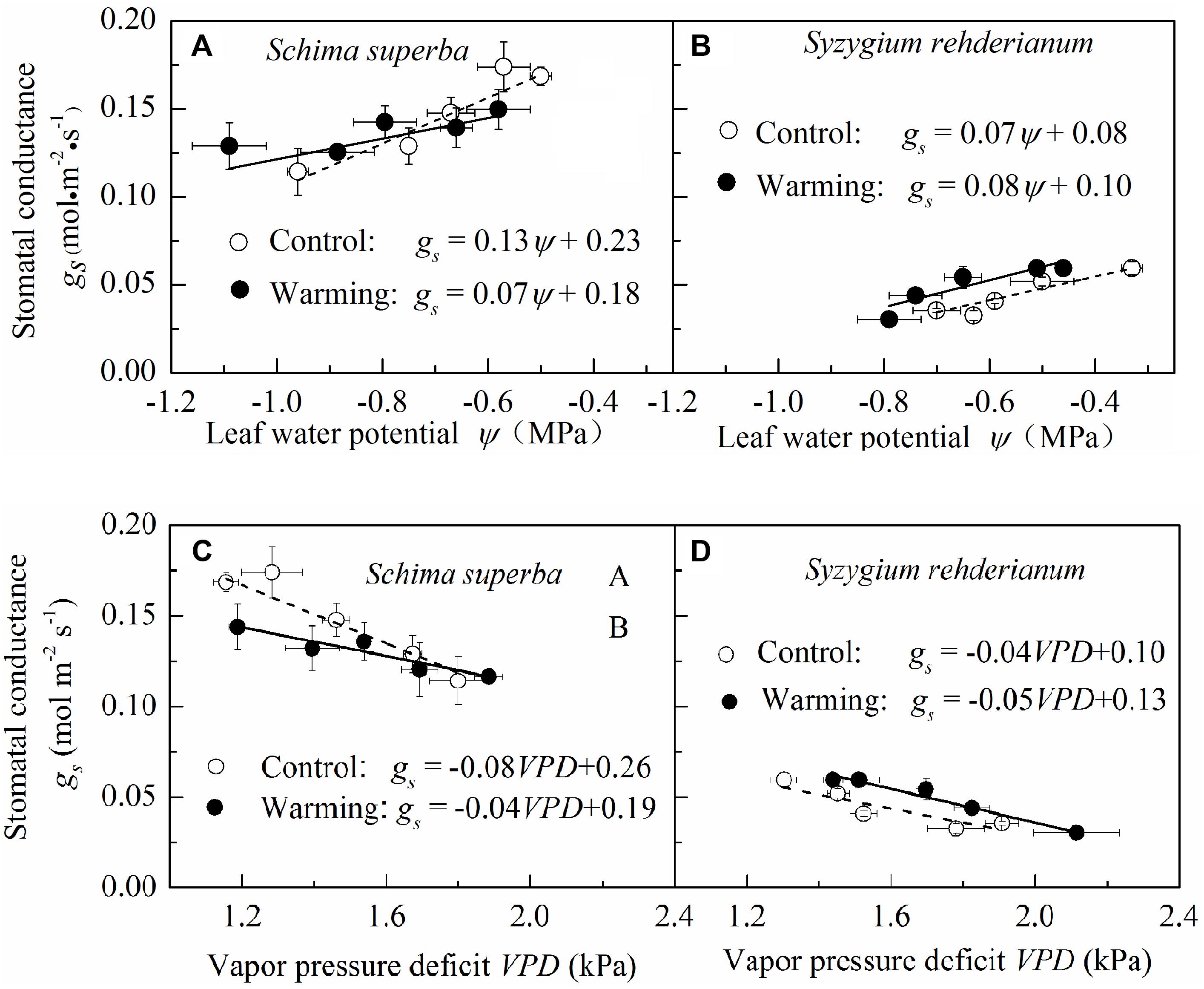
FIGURE 5. Relationship between stomatal conductance and leaf water potential (A,B) and vapor pressure deficit (C,D) for the two study species grown in both control and warming environments. Values are mean ± SE (n = 9 trees). Slope of stomatal conductance to leaf water potential represents stomatal sensitivity to leaf water potential. Open circle (○) and filled circle (•) represent control and warming environments, respectively.
Discussion
We found in this study that the two species showed contrasting stomatal responses to elevated temperature. Warming significantly affected stomatal morphology (stomatal size or density) and its sensitivity to water deficit in both study species. S. superba employed a conservative strategy by reducing stomatal density but keeping a constant stomatal size, which can potentially prevent excessive water loss, but at the cost of reducing carbon gain (i.e., decrease in photosynthetic rate). By contrast, S. rehderianum was able to balance water loss and carbon gain through reducing stomatal size without affecting stomatal density, thus allowing the maintenance of photosynthetic rate under warming conditions.
Warming Induced Changes in Stomatal Anatomic and Gas Exchange Traits
In general, plants with lower stomatal density would prevent water from excessive loss (Hepworth et al., 2015), but leaf stomatal conductance and photosynthetic rate might be reduced correspondingly (Xu and Zhou, 2008; Yoo et al., 2010; Upadhyay et al., 2013). For example, leaves with lower stomatal density have fewer pores through which CO2 can enter, resulting in potential lower stomatal conductance and photosynthesis rates (Tanaka et al., 2013). Furthermore, smaller size of stomata would decrease CO2 diffusion into the leaf (Parkhurst, 1994), because its conductance is proportional to the square of the effective radius of the pore (Maherali et al., 2002). However, smaller size of stomatal would allow plants to respond to environment change more promptly, thus potentially enhancing CO2 assimilation (Hetherington and Woodward, 2003; Franks et al., 2009). Such an adjustment is advantageous especially under water deficit conditions. For instance, Spence et al. (1986) reported that smaller size of stomata may allow the pores remaining open under drought stress, leading to the maintenance of photosynthesis. Interestingly, Hughes et al. (2017) showed that the decrease of both stomatal density and size in Hordeum vulgare reduced stomatal conductance and photosynthetic rate under well-watered conditions, but displayed non-significant effect under drought stress. Therefore, it is likely that the advantage of smaller size of stomata counteracted the negative effect on plant photosynthesis caused by lower stomatal density. In the present study, warming caused water deficit in both species, and we observed decreases of stomatal conductance and photosynthetic rate in S. superba, which might be due to the decrease of stomatal density under warming conditions; whereas S. rehderianum was able to maintain stomatal conductance and photosynthetic rate, probably owing to its reduced stomatal size in response to warming.
Apart from the adjustment of stomatal structure to warming, the two species may have different optimum growth temperatures, because S. superba is a canopy tree, while S. rehderianum is a shrub-like species growing in the understory. Therefore, the different ecological niche (e.g., canopy height) might result in different light sensitivity of the two species during the ontogeny, which might be one of the reasons for the observed contrasting responses in photosynthetic rate of the two species to warming (Way and Yamori, 2014; Slot and Winter, 2017a,b, 2018). In addition, warming might have different effects on Rubisco content (Scafaro et al., 2017) and mesophyll conductance (Qiu et al., 2017), leading to different changes in photosynthetic rate for different plants, which deserves further investigation for the two study species.
We noted that although the two species responded differently to warming through the adjustment of stomatal morphology and photosynthetic rates, non-significant differences in plant height and base diameter for the two species were observed after 1 year warming treatment (Supplementary Figure S1). On the one hand, both S. superba and S. rehderianum are slow-growing species with a slow “return on investment” (Zhu et al., 2013), and hence, a longer term warming treatment might be needed to investigate the effects on growth for the two species. On the other hand, as shown in a number of studies, warming might cause a decrease of dark respiration rate (Yamori et al., 2014; Slot and Winter, 2017a), which in turn results in less carbon loss in maintenance processes and more carbon can be retained for growth. This also needs to be investigated in future studies.
Warming Induced Changes in Stomatal Sensitivity to Leaf Water Potential and Vapor Pressure Deficit
Stomatal sensitivity to leaf water potential and VPD reflects the balance of carbon gain and water use in plants (Fredeen and Sage, 1999; Buckley, 2005; Martínez-Vilalta and Garcia-Forner, 2017). High stomatal sensitivity to leaf water potential and VPD indicates that plants can effectively regulate their stomata aperture according to leaf water status. Such a strategy represents an advantage of plants in adaptation to water deficit, in which plants can fully open stomata when water status is well and close them when water status is unfavorable (Williams and Ehleringer, 2000; Addington et al., 2004; McDowell et al., 2008). By contrast, plants with low stomatal sensitivity to leaf water potential and VPD may have advantage when water condition is favorable, but readily suffer from water stress because they lack the “smart” regulation manner in preventing excessive water loss (Dai et al., 1992; Domec and Johnson, 2012; Klein, 2014). We found, in the present study, a significant decrease of stomatal sensitivity to leaf water potential and VPD in S. superba, but non-significant change in that of S. rehderianum. Our results indicate that stomatal adjustment in S. rehderianum is more advantageous than that of S. superba under water deficit environment caused by elevated temperature.
Conclusion
Our study clearly demonstrated that, S. rehderianum and S. superba, the two co-occurring subtropical forest dominant tree species showed deferential responses to elevated temperature in terms of stomatal structure and photosynthetic function. The decreased stomatal size and constant stomatal density of S. rehderianum allowed the maintenance of carbon assimilation rate, WUE, and high stomatal sensitivity to leaf water potential, while the converse was true for S. superba, which may, in a long term, imperil its dominancy in this subtropical forest community under future climate change.
Author Contributions
GW and QY conceived the idea. GW and LH conducted the experiments. GW analyzed the data. QY, HL, and GW wrote the manuscript. All authors helped in drafting the manuscript and gave essential input to the work.
Funding
This work was funded by the National Natural Science Foundation of China (31570405), the National Basic Research Program of China (2013CB956704), Strategic Priority Research Program of the Chinese Academy Sciences (XDA13020500), and the CAS/SAFEA International Partnership Program for Creative Research Teams.
Conflict of Interest Statement
The authors declare that the research was conducted in the absence of any commercial or financial relationships that could be construed as a potential conflict of interest.
Acknowledgments
We are grateful to the reviewers for their insightful and constructive suggestions on earlier versions of this article.
Supplementary Material
The Supplementary Material for this article can be found online at: https://www.frontiersin.org/articles/10.3389/fpls.2018.00467/full#supplementary-material
References
Adams, H. D., Guardiola-Claramonte, M., Barron-Gafford, G. A., Villegas, J. C., Breshears, D. D., Zou, C. B., et al. (2009). Temperature sensitivity of drought-induced tree mortality portends increased regional die-off under global-change-type drought. Proc. Natl. Acad. Sci. U.S.A. 106, 7063–7066. doi: 10.1073/pnas.0901438106
Addington, R. N., Mitchell, R. J., Oren, R., and Donovan, L. A. (2004). Stomatal sensitivity to vapor pressure deficit and its relationship to hydraulic conductance in Pinus palustris. Tree Physiol. 24, 561–569. doi: 10.1093/treephys/24.5.561
Anderegg, W. R., Kane, J. M., and Anderegg, L. D. (2013). Consequences of widespread tree mortality triggered by drought and temperature stress. Nat. Clim. Change 3, 30–36. doi: 10.1038/nclimate1635
Apple, M. E., Olszyk, D. M., Ormrod, D. P., Lewis, J., Southworth, D., and Tingey, D. T. (2000). Morphology and stomatal function of Douglas fir needles exposed to climate change: elevated CO2 and temperature. Int. J. Plant Sci. 161, 127–132. doi: 10.1086/314237
Beerling, D. J., and Chaloner, W. G. (1993). The impact of atmospheric CO2 and temperature changes on stomatal density: observation from Quercus robur Lammas leaves. Ann. Bot. 71, 231–235. doi: 10.1006/anbo.1993.1029
Buckley, T. N. (2005). The control of stomata by water balance. New Phytol. 168, 275–292. doi: 10.1111/j.1469-8137.2005.01543.x
Carins Murphy, M. R., Jordan, G. J., and Brodribb, T. J. (2012). Differential leaf expansion can enable hydraulic acclimation to sun and shade. Plant Cell Environ. 35, 1407–1418. doi: 10.1111/j.1365-3040.2012.02498.x
Christensen, J. H., Hewitson, B., Busuioc, A., Chen, A., Gao, X., Held, R., et al. (2007). Regional Climate Projections. Climate Change 2007: The Physical Science Basis. Contribution of Working Group I to the Fourth Assessment Report of the Intergovernmental Panel on Climate Change. Cambridge: Cambridge University Press, 847–940.
Dai, Z., Edwards, G. E., and Ku, M. S. B. (1992). Control of photosynthesis and stomatal conductance in Ricinus communis L. (Castor Bean) by leaf to air vapor pressure deficit. Plant Physiol. 99, 1426–1434. doi: 10.1104/pp.99.4.1426
Domec, J. C., and Johnson, D. M. (2012). Does homeostasis or disturbance of homeostasis in minimum leaf water potential explain the isohydric versus anisohydric behavior of Vitis vinifera L. cultivars? Tree Physiol. 32, 245–248. doi: 10.1093/treephys/tps013
Drake, J. E., Tjoelker, M. G., Vårhammar, A., Medlyn, B. E., Reich, P. B., Leigh, A., et al. (2018). Trees tolerate an extreme heatwave via sustained transpirational cooling and increased leaf thermal tolerance. Glob. Change Biol. 1–13. doi: 10.1111/gcb.14037
Farquhar, G. D., Caemmerer, S., and Berry, J. A. (1980). A biochemical model of photosynthetic CO2 assimilation in leaves of C3 species. Planta 149, 78–90. doi: 10.1007/BF00386231
Ferris, R., Nijs, I., Behaeghe, T., and Impens, I. (1996). Elevated CO2 and temperature have different effects on leaf anatomy of perennial ryegrass in spring and summer. Ann. Bot. 78, 489–497. doi: 10.1006/anbo.1996.0146
Franks, P. J., Beerling, D. J., and Berner, R. A. (2009). Maximum leaf conductance driven by CO2 effects on stomatal size and density over geologic time. Proc. Natl. Acad. Sci. U.S.A. 106, 10343–10347. doi: 10.1073/pnas.0904209106
Franks, P. J., and Farquhar, G. D. (2007). The mechanical diversity of stomata and its significant in gas-exchange control. Plant Physiol. 143, 78–87. doi: 10.1104/pp.106.089367
Fraser, L. H., Greenall, A., Carlyle, C., Turkington, R., and Friedman, C. R. (2009). Adaptive phenotypic plasticity of Pseudoroegneria spicata: response of stomatal density, leaf area and biomass to changes in water supply and increased temperature. Ann. Bot. 103, 769–775. doi: 10.1093/aob/mcn252
Fredeen, A. L., and Sage, R. F. (1999). Temperature and humidity effects on branchlet gas-exchange in white spruce: an explanation for the increase in transpiration with branchlet temperature. Trees 14, 161–168. doi: 10.1007/s004680050220
Hepworth, C., Adams, T. D., Hunt, L., Cameron, D. D., and Gray, J. E. (2015). Manipulating stomatal density enhances drought tolerance without deleterious effect on nutrient uptake. New Phytol. 2, 336–341. doi: 10.1111/nph.13598
Hetherington, A. M., and Woodward, F. I. (2003). The role of stomata in sensing and driving environmental change. Nature 424, 901–908. doi: 10.1038/nature01843
Hill, K. E., Guerin, G. R., Hill, R. S., and Watling, J. R. (2014). Temperature influences stomatal density and maximum potential water loss through stomata of Dodonaea viscosa subsp. Angustissima along a latitude gradient in southern Australia. Aust. J. Bot. 62, 657–665. doi: 10.1071/BT14204
Hughes, J., Hepworth, C., Dutton, C., Dunn, J. A., Hunt, L., Stephens, J., et al. (2017). Reducing stomatal density in barley improve drought tolerance without impacting on yield. Plant Physiol. 174, 776–787. doi: 10.1104/pp.16.01844
Intergovernmental Panel on Climate Change [IPCC] (2014). Climate Change 2014-Impacts, Adaptation and Vulnerability. Part B: Regional Aspects. Cambridge University Press Summary for policymakers. In: Climate Change 2014: Impacts, Adaptation, and Vulnerability. Contribution of Working Group II to the Fifth Assessment Report of the Intergovernmental Panel on Climate Change, eds B. V. Field, C. B. Dokken, D. J. Mach, K. J. Mastrandrea, M. D. Bilir, T. E. Chatterjee et al. (Cambridge: Cambridge University Press), 1–32.
Kivimäenpää, M., Sutinen, S., Valolahti, H., Häikiö, E., Riikonen, J., Kasurinen, A., et al. (2017). Warming and elevated ozone differently modify needle anatomy of Norway. Rev. Can. Rech. For. 47, 488–499. doi: 10.1139/cjfr-2016-0406
Klein, T. (2014). The variability of stomatal sensitivity to leaf water potential across tree species indicates a continuum between isohydric and anisohydric behaviours. Funct. Ecol. 28, 1313–1320. doi: 10.1111/1365-2435.12289
Li, R., Zhu, S. D., Chen, H. Y. H., John, R., Zhou, G., Zhang, D., et al. (2015). Are functional traits a good predictor of global change impacts on tree species abundance dynamics in a subtropical forest? Ecol. Lett. 18, 1181–1189. doi: 10.1111/ele.12497
Luomala, E. M., Laitinen, K., Sutinen, S., Kellomäki, S., and Vapaavuori, E. (2005). Stomatal density, anatomy and nutrient concentrations of Scots pine needles are affected by elevated CO2 and temperature. Plant Cell Environ. 28, 733–749. doi: 10.1111/j.1365-3040.2005.01319.x
Maherali, H., Reid, C. D., Polley, H. W., Johnson, H. B., and Jachson, R. B. (2002). Stomatal acclimation over a subambient to elevated CO2 gradient in a C3/C4 grassland. Plant Cell Environ. 25, 557–566. doi: 10.1046/j.1365-3040.2002.00832.x
Marchin, R. M., Broadhead, A. A., Bostic, L. E., Dunn, R. R., and Hoffmann, W. A. (2016). Stomatal acclimation to vapor pressure deficit doubles transpiration of small tree seedlings with warming. Plant Cell Environ. 39, 2221–2234. doi: 10.1111/pce.12790
Martínez-Vilalta, J., and Garcia-Forner, N. (2017). Water potential regulation, stomatal behaviour and hydraulic transport under drought: deconstructing the iso/anisohydric concept. Plant Cell Environ. 40, 962–976. doi: 10.1111/pce.12846
McDowell, N., Pockman, W. T., Allen, C. D., Breshears, D. D., Cobb, N., Kolb, T., et al. (2008). Mechanisms of plant survival and mortality during drought: why do some plants survive while others succumb to drought. New Phytol. 178, 719–739. doi: 10.1111/j.1469-8137.2008.02436.x
McElwain, J. C., Beerling, D. J., and Woodward, F. I. (1999). Fossil plants and global warming at the Triassic-Jurassic boundary. Science 285, 1386–1390. doi: 10.1126/science.285.5432.1386
McIntyre, P. J., Thorne, J. H., Dolanc, C. R., Flint, A. L., Flint, L. E., Kelly, M., et al. (2015). Twentieth-century shifts in forest structure in California: denser forests, smaller trees, and increased dominance of oaks. Proc. Natl. Acad. Sci. U.S.A. 112, 1458–1463. doi: 10.1073/pnas.1410186112
Mott, K. A., and Peak, D. (2010). Stomatal responses to humidity and temperature in darkness. Plant Cell Environ. 33, 1084–1090. doi: 10.1111/j.1365-3040.2010.02129.x
Parkhurst, D. F. (1994). Diffusion of CO2 and other gases inside leaves. New Phytol. 126, 449–479. doi: 10.1111/j.1469-8137.1994.tb04244.x
Qiu, C., Ethier, G., Pepin, S., Dubé, P., Desjardins, Y., and Gosselin, A. (2017). Persistent negative temperature response of mesophyll conductance in red raspberry (Rubus idaeus L.) leaves under both high and low vapour pressure deficits: a role for abscisic acid? Plant Cell Environ. 40, 1940–1959. doi: 10.1111/pce.13071
Scafaro, A. P., Xiang, S., Long, B. M., Bahar, N. A., Weerasinghe, L. K., Creek, D., et al. (2017). Strong thermal acclimation of photosynthesis in tropical and temperate wet-forest tree species: the importance of altered Rubisco content. Glob. Change Biol. 23, 2783–2800. doi: 10.1111/gcb.13566
Slot, M., and Winter, K. (2017a). In situ temperature relationships of biochemical and stomatal controls of photosynthesis in four lowland tropical tree species. Plant Cell Environ. 40, 3055–3068. doi: 10.1111/pce.13071
Slot, M., and Winter, K. (2017b). Photosynthetic acclimation to warming in tropical forest tree seedlings. J. Exp. Bot. 68, 2275–2284. doi: 10.1093/jxb/erx071
Slot, M., and Winter, K. (2018). High tolerance of tropical sapling growth and gas exchange to moderate warming. Funct. Ecol. 32, 599–611. doi: 10.1111/1365-2435.13001
Spence, R. D., Wu, H., Sharpe, P. J. H., and Clark, K. G. (1986). Water stress effects on guard cell anatomy and the mechanical advantage of the epidermal cells. Plant Cell Environ. 9, 197–202. doi: 10.1111/1365-3040.ep11611639
Tanaka, Y., Sugano, S. S., Shimada, T., and Hara-Nishimura, I. (2013). Enhancement of leaf photosynthetic capacity through increased stomatal density in Arabidopsis. New Phytol. 198, 757–764. doi: 10.1111/nph.12186
Upadhyay, R. K., Soni, D. K., Singh, R., Dwivedi, U. N., Pathre, U. V., and Nath, P. (2013). SIERF36, an EAR-motif-containing ERF gene from tomato, alters stomatal density and modulates photosynthesis and growth. J. Exp. Bot. 64, 3237–3247. doi: 10.1093/jxb/ert162
Urban, J., Ingwers, M. W., McGuire, M. A., and Teskey, R. O. (2017). Increase in leaf temperature opens stomata and decouples net photosynthesis from stomatal conductance in Pinus taeda and Populus deltoides x nigra. J. Exp. Bot. 68, 1757–1767. doi: 10.1093/jxb/erx052
Way, D. A., and Yamori, W. (2014). Thermal acclimation of photosynthesis: on the importance of adjusting our definitions and accounting for thermal acclimation of respiration. Photosynth. Res. 119, 89–100. doi: 10.1007/s11120-013-9873-7
Williams, A. P., Allen, C. D., Macalady, A. K., Griffin, D., Woodhouse, C. A., Meko, D. M., et al. (2012). Temperature as a potent driver of regional forest drought stress and tree mortality. Nat. Clim. Change 3, 292–297. doi: 10.1038/nclimate1693
Williams, D. G., and Ehleringer, J. R. (2000). Intra- and interspecific variation for summer precipitation use in pinyon-juniper woodlands. Ecol. Monogr. 70, 517–537.
Xu, Z., and Zhou, G. (2008). Responses of leaf stomatal density to water status and its relationship with photosynthesis in a grass. J. Exp. Bot. 59, 3317–3325. doi: 10.1093/jxb/ern185
Yamori, W., Hikosaka, K., and Way, D. A. (2014). Temperature response of photosynthesis in C3, C4, and CAM plants: temperature acclimation and temperature adaptation. Photosynth. Res. 119, 101–117. doi: 10.1007/s11120-013-9874-6
Yan, W., Zhong, Y., and Shangguan, Z. (2017). Contrasting responses of leaf stomatal characteristics to climate change: a considerable challenge to predict carbon and water cycles. Glob. Change Biol. 104, 145–157. doi: 10.1111/gcb.13654
Yoo, C. Y., Pence, H. E., Jin, J. B., Miura, K., Gosney, M. J., Hasegawa, P. M., et al. (2010). The Arabidopsis GTL1 transcription factor regulates water use efficiency and drought tolerance by modulating stomatal density via transrepression of SDD1. Plant Cell 22, 4128–4141. doi: 10.1105/tpc.110.078691
Zhang, L. R., Niu, H. S., Wang, S. P., Li, Y. N., and Zhao, X. Q. (2010). Effects of temperature increase and grazing on stomatal density and length of four alpine Kobresia meadow species, Qinghai-Tibetan Plateau. Acta Ecol. Sin. 30, 6961–6969.
Zheng, Y., Xu, M., Hou, R., Shen, R., Qiu, S., and Ouyang, Z. (2013). Effects of experimental warming on stomatal traits in leaves of maize (Zea may L.). Ecol. Evol. 3, 3095–3111. doi: 10.1002/ece3.674
Zhou, G., Peng, C., Li, Y., Liu, S., Zhang, Q., Tang, X., et al. (2013). A climate change-induced threat to the ecological resilience of a subtropical monsoon evergreen broad-leaved forest in Southern China. Glob. Change Biol. 19, 1197–1210. doi: 10.1111/gcb.12128
Zhou, H. H., Chen, Y. N., Li, W. H., and Chen, Y. P. (2010). Photosynthesis of Populus euphratica in relation to groundwater depths and high temperature in arid environment, northwest China. Photosynthetica 48, 257–268. doi: 10.1007/s11099-010-0032-5
Zhu, S. D., Song, J. J., Li, R. H., and Ye, Q. (2013). Plant hydraulics and photosynthesis of 34 woody species from different successional stages of subtropical forests. Plant Cell Environ. 36, 879–891. doi: 10.1111/pce.12024
Keywords: functional traits, gas exchange, subtropical tree species, warming, water deficit
Citation: Wu G, Liu H, Hua L, Luo Q, Lin Y, He P, Feng S, Liu J and Ye Q (2018) Differential Responses of Stomata and Photosynthesis to Elevated Temperature in Two Co-occurring Subtropical Forest Tree Species. Front. Plant Sci. 9:467. doi: 10.3389/fpls.2018.00467
Received: 11 December 2017; Accepted: 23 March 2018;
Published: 10 April 2018.
Edited by:
Boris Rewald, Universität für Bodenkultur Wien, AustriaReviewed by:
Martijn Slot, Smithsonian Tropical Research Institute, PanamaJosef Urban, Mendel University in Brno, Czechia
Copyright © 2018 Wu, Liu, Hua, Luo, Lin, He, Feng, Liu and Ye. This is an open-access article distributed under the terms of the Creative Commons Attribution License (CC BY). The use, distribution or reproduction in other forums is permitted, provided the original author(s) and the copyright owner are credited and that the original publication in this journal is cited, in accordance with accepted academic practice. No use, distribution or reproduction is permitted which does not comply with these terms.
*Correspondence: Qing Ye, cXllQHNjYmcuYWMuY24=