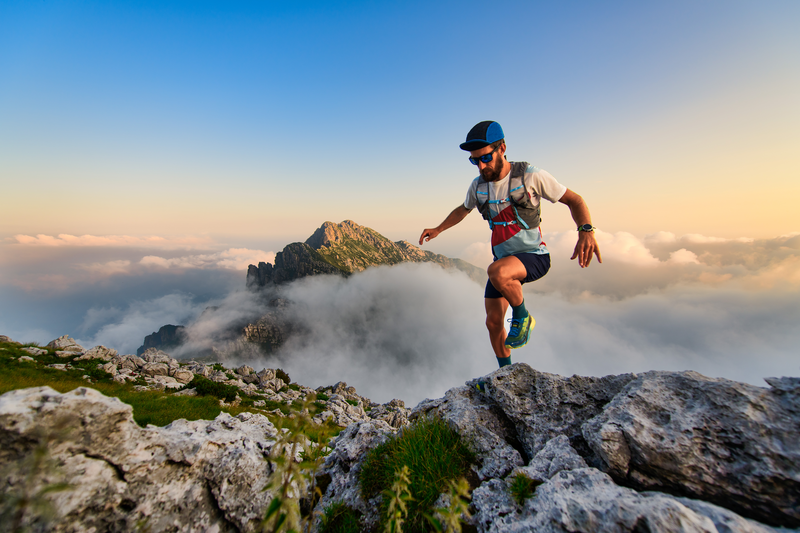
95% of researchers rate our articles as excellent or good
Learn more about the work of our research integrity team to safeguard the quality of each article we publish.
Find out more
ORIGINAL RESEARCH article
Front. Plant Sci. , 13 February 2018
Sec. Agroecology
Volume 9 - 2018 | https://doi.org/10.3389/fpls.2018.00165
Water and fertilizers affect the nitrogen (N) and phosphorus (P) acquisition and allocation among organs in dominant species in natural vegetation on the semiarid Loess Plateau. This study aimed to clarify the N and P accumulation and N:P ratio at organ and plant level of a local legume species mixed with a grass species under varying water and fertilizer supplies, and thus to fully understand the requirements and balance of nutrient elements in response to growth conditions change of native species. The N and P concentration in the organ (leaf, stem, and root) and plant level of Lespedeza davurica (C3 legume), were examined when intercropped with Bothriochloa ischaemum (C4 grass). The two species were grown outdoors in pots under 80, 60, and 40% of soil water field capacity (FC), -NP, +N, +P, and +NP supply and the grass:legume mixture ratios of 2:10, 4:8, 6:6, 8:4, 10:2, and 12:0. The three set of treatments were under a randomized complete block design. Intercropping with B. ischaemum did not affect N concentrations in leaf, stem and root of L. davurica, but reduced P concentration in each organ under P fertilization. Only leaf N concentration in L. davurica showed decreasing trend as soil water content decreased under all fertilization and mixture proportion treatments. Stems had the lowest, while roots had the highest N and P concentration. As the mixture proportion of L. davurica decreased under P fertilization, P concentration in leaf and root also decreased. The N concentration in L. davurica at the whole plant level was 11.1–17.2%. P fertilization improved P concentration, while decreased N:P ratio in L. davurica. The N:P ratios were less than 14.0 under +P and +NP treatments. Our results implied that exogenous N and P fertilizer application may change the N:P stoichiometry and influence the balance between nutrients and organs of native dominant species in natural grassland, and P element should be paid more attention when considering rehabilitating degraded grassland via fertilization application in semiarid Loess Plateau region.
Plant growth and productivity in terrestrial ecosystems are limited by N and P (Aerts and Chapin, 2000; Güsewell, 2004; Vitousek et al., 2010; Dijkstra et al., 2016). Plant N demand is largely determined by photosynthetic N use efficiency, which varies among species because of leaf morphology or photosynthesis differences (Lambers and Poorter, 1992; Garnier et al., 1995). The variation in the requirements of P is related to internal phosphate recycling rates or related metabolic pathways differences among plants (Vance et al., 2003; Chen et al., 2013). N and P contents in plants are jointly affected by plant intrinsic properties, carbon synthesis characteristics and meteorological and pedologic factors (Li et al., 2007). In terrestrial ecosystems, N and P availability is important for community function and vegetation composition, and N:P ratios changing in plants may influence the competition balance among species in their communities (Güsewell et al., 2002; Agren, 2004; van der Hoek et al., 2004; Han et al., 2005; Fujita et al., 2010). The growth and productivity performance of species is affected by N and P fertilization, because plants need to maintain a certain N:P stoichiometric balance to function properly at the specific conditions (Fujita et al., 2010). Consequently, it is critical to determine the N and P requirements of each species in order to minimize interspecific competition and increase yield in their mixtures (Isaac et al., 2012; Neugschwandtner and Kaul, 2015, 2016).
High exploration of the root system for water and nutrient acquisition is required for improving N and P uptake efficiency when soil nutrients are scarce (Hinsinger et al., 2011a; Isaac et al., 2012). In limited nutrient environments, two or more species growing under intercropping or mixture plantations, may increase N and P acquisition and use efficiency, because such systems are supposed to enhance mutual positive interactions between plants (Zhang and Li, 2003; Li et al., 2007; Hinsinger et al., 2011a,b). In that respect, the cereal-legume intercropping system have advantages in growth, yield and resource use efficiency compared to respective sole cropping, because of their complementarity and facilitation in using light, water, and nutrition (Zhang and Li, 2003; Li et al., 2007; Hinsinger et al., 2011a; Betencourt et al., 2012; Xia et al., 2013; Tang et al., 2014; Neugschwandtner and Kaul, 2014, 2015). Although the improved acquisition of N under the cereal-legume intercropping is well known, only few recent studies indicated that similar effects may occur for P (Hinsinger et al., 2011a; Betencourt et al., 2012; Xia et al., 2013). In some dry environments, like the semi-arid Loess Plateau of China, the soil environment is hostile for plant growth as water deficits often occur and the soil erosion causes losses of N and P (Meng et al., 2008; Lü and Han, 2010; Liu et al., 2013; Niu et al., 2016). To understand the acquisition and allocation of limiting N and P in plants is useful to determine their adaptation strategy to the environment, and find appropriate measures to increase their productivity and improve their function in the region.
Native dominant species have great eco-adaptation to regional climatic and soil environment, and play an important role in ecological conservation and services in the region. Understanding the N and P relations in these species under different water and nutrient (i.e., N and P) availability is critical to clarify their co-existence mechanisms, and to choose appropriate measures to improve their productivity in natural grassland. In the natural grassland community of the semi-arid Loess Plateau of China, there are two co-dominant perennial species, the C4 grass Bothriochloa ischaemum and the C3 legume subshrub Lespedeza davurica. When they are grown in mixtures under different water and fertilizer levels, biomass production and water use efficiency are higher than when grown alone (Xu et al., 2013). The different mixtures and levels of water and fertilizer supply, particularly N and P combined, affect the N and P concentration in different organs of the grass B. ischaemum (Xu et al., 2016). Here, we report a study aimed to examine the N:P stoichiometry of the matched legume species L. davurica, from both the organ and whole plant level in response to soil water, mixture proportions and fertility changing. Our aims were to clarify the N and P accumulation and N:P ratio in L. davurica at organ and plant level, and examine the effects of soil water and N and P fertilizers on N:P stoichiometry in L. davurica intercropped with B. ischaemum in various mixture proportions.
The two co-dominant perennial species, B. ischaemum and L. davurica were used in this study. Their seeds were previously collected from a natural grassland community and tested for germination prior to this study. Seed collection was made at the Ansai Research Station, Ansai County, Shaanxi Province, China (36°51′30″N, 109°19′23″E). Plants were grown in cylindrical plastic pots 20 cm diameter, 30 cm depth, filled with 9.0 kg of the soil at a bulk density approx.1.2 g cm-3. The experiment was conducted at the nursery of the State Key Laboratory of Soil Erosion and Dryland Farming on the Loess Plateau, Yangling, Shaanxi Province, China (34°12′N, 108°7′E, altitude 530 m a.s.l.). The soil was a sandy-loam, collected from the upper 20 cm of a field site at the station. The soil gravimetric water content at field capacity (FC) and wilting point in the pot was 20.0 and 4.0%, respectively. The FC value (g H2O 100 g dry soil-1) was obtained using Wilcox method (Duan et al., 2010). Referring to world reference base for soil resources (FAO, 2006), the loess soil (called Huangmiantu locally) is classified as Calcaric Cambisol (Qiu et al., 2012). The organic matter of the soil was 2.7 g kg-1, total N content 0.17 g kg-1, total P content 0.63 g kg-1 and total K 19.7 g kg-1. The soil pH value was 8.5, and the content of total CaCO3 was 103 mg kg-1. The extractable N, P and K were 11.2, 6.55 and 94.8 mg kg-1, respectively. The soil was amended with or without N and/or P fertilizer as per fertility treatment. An open-end plastic pipe (2.0 cm diameter) was inserted inside each pot adjacent to the inner wall to supply water at 10 cm height from the bottom of each pot. The seeds were germinated on filter paper moistened with deionized water, in covered Petri-dishes, at room temperature for 7 days, before transplanting into the pots. Germinated seeds of each species were sown in 12 equally spaced dibbles in each pot on March 31, 2010. The scheme of plant arrangement within the pots was same as described in Xu et al. (2011). After emergence, seedlings were thinned to one plant per dibble (i.e., 12 plants per pot), and all pots were initially well watered during the seedling establishment. The soil surface of each pot was covered with 40 g of perlite to reduce soil evaporation. During the rainy days, the pots were covered by rainout shelter.
The treatments consisted of (1) six mixture proportions of B. ischaemum: L. davurica: 12:0, 10:2, 8:4, 6:6, 4:8, and 2:10. The mixture proportions were designed using a replacement series design (Connolly et al., 2001), in which 12 plants per pot of L. davurica (L) were replaced by B. ischaemum (B); (2) four fertilization treatments: non-NP (-NP), N supplied at 0.025 g kg-1 soil (+N), P supplied at 0.1 g kg-1 soil (+P) and combined N (0.025 g kg-1) and P (0.1 g kg-1) (+NP). N and P were applied as urea (CON2H4) and KH2PO4, respectively, and the amount was about 123 kg ha-1 (N) and 225 kg ha-1 (P), respectively; and (3) three soil water regimes: 16% (80% FC), 12% (60% FC), and 8% (40% FC). No other nutrients were applied as a base fertilization. The water treatments were imposed to match the natural grassland soil water content, because previous research has showed that the soil gravimetric water content in the natural grassland of the Loess Plateau region varies between 6 and 20% (Chen et al., 2008; Jiao et al., 2013). The mixture proportions, fertilization treatments and soil water regimes were arranged as a randomized complete block design. Each treatment had six replications (three in each harvest).
There were two harvests. The first sampling was made on June 21, 2010, 82 days after sowing (DAS), when plants of B. ischaemum were at 5th leaf stage and plants of L. davurica were branching. This sampling was made to calibrate the watering regimes only. Three pots for each mixture proportion (totally 18 pots) were harvested and the fresh biomass production of the two species was measured as reference. Then the three soil water content (SWC) regimes were induced by withholding watering and regularly weighing of the pots until they reached 80% FC, 60% FC, and 40% FC, respectively.
The second sampling was made on October 19, when the plants reached maturity at 202 DAS. This sampling was made to measure plant biomass by separating the shoots and roots. The roots were then washed to remove the soil. The leaves, stems and roots from each plant were separated and dried at 75°C for 48 h in an air-drying oven. In some treatments, L. davurica had seeds and ears, and which were combined into stem biomass and element analysis. The dry weight of each pot was combined as one biomass sample for each species.
The chemical properties of the soil used in the study were performed using standard soil test procedures from the Chinese Ecosystem Research Network (Editorial Committee, 1996) and the Soil Science Society of China (1999). Soil pH value was determined in a 1:2.5 soil/water slurry using a Delta 320 pH meter (Mettler-Toledo, Shanghai, China). Soil CaCO3 content was determined by using the Chittick apparatus (Dreimanis, 1962). Soil organic matter content was determined by wet digestion with a mixture of potassium dichromate and concentrated sulfuric acid. Soil total nitrogen content was measured via the semi-micro Kjeldahl method and a Kjeltec System 1026 Distilling Unit. Soil total phosphorus content was determined colorimetrically samples went through wet digestion with H2SO4+ HClO4. Total potassium was analyzed by the sodium hydroxide melting method with flame photometers. Soil available nitrogen content was determined with a micro-diffusion technique after samples went through alkaline hydrolysis. Soil available phosphorus was determined by the Olsen method (ISSCAS, 1978). Soil available potassium was measured in 1.0 mol L-1 NH4OAc extracts by flame photometry.
N concentration in leaves, stems (including ears) and roots were determined using the Auto-Kjeldahl method (Kjektec System 2300 Distilling Unit, Foss, Sweden). Total P concentration in leaves, stems (including ears) and roots were analyzed using a molybdate/stannous chloride method after H2SO4–H2O2–HF digestion quantified by reference to a national standard material with a known P concentration (TU1901 UV spectrophotometer, China) (Qin et al., 2016). Plant tissues were grounded to a fine powder using a ring mill through 100-mesh. Total plant N and P concentration was determined by the summation of the N and P amount in each organ and then divided by the total biomass production. The N:P concentration ratio was calculated as the N concentration divided by the P concentration.
Differences in the mean N and P concentration and N:P concentration ratio for each organ and whole plant for each treatment (soil water regime, mixture proportion or fertilization, mixture vs. monoculture) were determined using the Tukey LSD analysis of SPSS Statistics17.0 (IBM, United States). Differences were considered significant for all statistical tests at p ≤ 0.05. The interactive effects of mixture proportion, fertilizer supply and soil water regime on N and P concentration and N:P ratio in each organ and whole plant was analyzed using a three-way ANOVA through GLM (general liner models) in SPSS.
The mean annual temperature at the State Key Laboratory of Soil Erosion and Dryland Farming on the Loess Plateau, Yangling, China was 13.0°C with a maximum mean temperature of 26.7°C in July and a minimum temperature of -1.5°C in January. Mean annual precipitation was 650 mm.
Leaf N concentration in L. davurica decreased as soil water content decreased under all fertilization and mixture proportion treatments. Stem N concentration under 40% FC was higher than those under 80% FC and 60% FC soil water regimes. Root N concentration was higher in monocultures than in mixtures, especially at SWC of 40% FC. Under each treatment (i.e., soil water regime, fertilization, mixture proportion), stem had significantly lower while root had significantly higher (p ≤ 0.05) N concentration (Figure 1). Fertilization had no effects on stem N concentration, and the interactions of SWC × fertilization on leaf and root N concentration, and the interaction of SWC × mixture proportion on leaf N concentration of L. davurica were not significant (Table 1).
FIGURE 1. Leaf, stem, and root N concentration of Lespedeza davurica (L) mixed with Bothriochloa ischaemum (B) at various mixture proportions under three soil water regimes [HSWC: 80% FC (field capacity); MSWC: 60% FC; LSWC: 40% FC] and four fertilization treatments (–NP, +N, +P, +NP). BiLj (i, j = 0, 2, 4, 6, 8, 10, 12; i+j = 12) means the plant numbers of B. ischaemum to L. davurica in their mixtures. Error bars are LSD (p < 0.05).
TABLE 1. P-values of three-way ANOVA to evaluate the effect of soil water content (SWC), fertilization treatment (FT), mixture proportion (MP) and their interactions on N and P concentration in leaves, stems, roots and whole plants of Lespedeza davurica intercropped with Bothriochloa ischaemum.
The leaf P concentration of L. davurica were higher under monoculture than under mixtures (p < 0.05), and showed a decreasing trend as its ratio in the mixture decreased (Figure 2). P fertilizer (+P and +NP treatments) significantly improved leaf P concentration across mixture proportions and soil water regimes (Figure 2). For each SWC, fertilization or mixture proportion treatment, the significantly highest P concentration was found in the roots and the lowest in the stems. The interaction of SWC and fertilization did not have effects on P concentration in both leaf and stem. The interactions from SWC and mixture proportion, and SWC × mixture proportion × fertilization interaction did not affect leaf P concentration. P concentration in root was significantly affected by SWC, mixture proportion or fertilization, and their combined interactions (Table 1).
FIGURE 2. Leaf, stem, and root P concentration of L. davurica (L) mixed with B. ischaemum (B) at various mixture proportions under three soil water regimes [HSWC: 80% FC (field capacity); MSWC: 60% FC; LSWC: 40% FC] and four fertilization treatments (–NP, +N, +P, +NP). BiLj (i, j = 0, 2, 4, 6, 8, 10, 12; i+j = 12) means the plant numbers of B. ischaemum to L. davurica in their mixtures. Error bars are LSD (p < 0.05).
P fertilization decreased the N:P ratio in each organ of L. davurica under all water regimes in monocultures or mixtures (Figure 3). When N was applied (+N and +NP treatments), N:P ratio increased gradually as the mixture proportion of L. davurica decreased. The N:P ratio in the stem was higher while in the root it was lower under all soil water regime, mixture proportion and fertilization treatments. Soil water content had no effect on stem N:P ratio, and its interaction with fertilization did not have effects on N:P ratio in leaf and stem (Table 1). No significant effects of the interactions from SWC and mixture proportion, and interaction of SWC, mixture proportion and fertilization on leaf N:P ratio were detected.
FIGURE 3. Leaf, stem, and root N:P ratios of L. davurica (L) mixed with B. ischaemum (B) at various mixture proportions under three soil water regimes [HSWC: 80% FC (field capacity); MSWC: 60% FC; LSWC: 40% FC] and four fertilization treatments (–NP, +N, +P, +NP). BiLj (i, j = 0, 2, 4, 6, 8, 10, 12; i+j = 12) means the plant numbers of B. ischaemum to L. davurica in their mixtures. Error bars are LSD (p < 0.05).
At the whole plant level, the N concentration in L. davurica was 11.1–17.2%. The P concentration had a tendency to decrease when the proportion of L. davurica in the mixtures decreased, especially under P fertilizer supply (+P or +NP) treatments (Figure 4). P fertilizer also significantly increased P concentration in L. davurica for each mixture proportion and soil water regime. Only in a few cases, the values of N:P ratio were bigger than 16.0, and all appeared in -NP and +N fertilization treatments. Under P fertilizer supply (+P or +NP) treatments, the N:P ratio concentration had a tendency to increase when the proportion of L. davurica in the mixtures decreased, and as the N:P ratio concentration were smaller than 16.0, and lower than the values under -NP and +N treatments for each mixture proportion and soil water regime (Figure 4). SWC, fertilization treatment, mixture proportion, and their interaction significantly affected N and P concentration and N:P ratio in L. davurica (Table 1).
FIGURE 4. Whole plant N concentration, P concentration and N:P ratio of L. davurica (L) mixed with B. ischaemum (B) at various mixture proportions under three soil water regimes [HSWC: 80% FC (field capacity); MSWC: 60% FC; LSWC: 40% FC] and four fertilization treatments (–NP, +N, +P, +NP). BiLj (i, j = 0, 2, 4, 6, 8, 10, 12; i+j = 12) means the plant numbers of B. ischaemum to L. davurica in their mixtures. Error bars are LSD (p < 0.05).
In the single plant level, N and P acquired by L. davurica under all treatments were mainly allocated to roots (Figures 5, 6). The average amount across mixture proportions and water regimes of N allocation in leaf, stem and root were 3.71–8.15, 3.49–6.08, and 11.68–13.96 mg plant-1, respectively. The mean amount of P in leaf, stem and root of single plant was 0.22–0.71, 0.18–0.43, and 0.93–1.67 mg plant-1 across all mixture proportions and water regimes, respectively. Under P fertilizer (+P and +NP treatments), P amount in leaf, stem and root was significantly improved. P fertilization significantly increased the N amount in leaf and stem (Figures 5, 6).
FIGURE 5. N allocation in leaf, stem and root of L. davurica (L) mixed with B. ischaemum (B) at various mixture proportions under three soil water regimes [HSWC: 80% FC (field capacity); MSWC: 60% FC; LSWC: 40% FC] and four fertilization treatments (–NP, +N, +P, +NP). BiLj (i, j = 0, 2, 4, 6, 8, 10, 12; i+j = 12) means the plant numbers of B. ischaemum to L. davurica in their mixtures. Error bars are LSD (p < 0.05).
FIGURE 6. P allocation in leaf, stem and root of L. davurica (L) mixed with B. ischaemum (B) at various mixture proportions under three soil water regimes [HSWC: 80% FC (field capacity); MSWC: 60% FC; LSWC: 40% FC] and four fertilization treatments (–NP, +N, +P, +NP). BiLj (i, j = 0, 2, 4, 6, 8, 10, 12; i+j = 12) means the plant numbers of B. ischaemum to L. davurica in their mixtures. Error bars are LSD (p < 0.05).
Individual plant biomass production of B. ischaemum decreased as its proportion increased in the mixtures, and the monoculture had the smallest values (Supplementary Figure S1). Soil water content significantly affected biomass production of B. ischaemum, regardless of mixing proportions and fertilizer treatments. Across mixture proportions including monoculture, the averaged biomass production under 40% FC was significantly lower (p < 0.05) than under 80% FC and 60% FC, and there was no significant differences between the latter two (Supplementary Figure S2). Single plant biomass production of L. davurica averagely increased about 10% under fertilizer applications (+N, +P, or +NP treatments). The averaged root biomass in the whole plant accounted for more than 38% across mixture proportions and fertilizations (Supplementary Figure S3). Leaf biomass accounted for 20.8–27.1% of the whole plant of L. davurica in the experiment, and showed obvious decreasing trend as its proportion decreased in the mixture under +NP treatment.
Legume and grass growing together is a common phenomenon in natural community. However, less attention has been given to nutrient behavior in different organs in their mixtures. Together with the sister paper, the studies provides the evidences of the N:P stoichiometric changes with mixture proportions in grass-forage mixture systems of native species in semiarid Loess Plateau (Xu et al., 2016). N and P concentration in different organs of the legume L. davurica varied with the SWC and fertilization when mixed with B. ischaemum, but their changing patterns were different. Leaf and stem N concentration of L. davurica did not vary significantly in response to mixture proportions, which may be associated to the low N requirements of this leguminous specie or it can maintain relatively stable N concentration in both organs under different conditions (Fujita et al., 2010; Sardans et al., 2012). In the grass-legume mixtures, it is likely that the grass absorbed N that was released by the legume root and nodule root system, resulting in a more efficient use of nutrients (Hauggaard-Nielsen and Jensen, 2005; Chen et al., 2013; Neugschwandtner and Kaul, 2014, 2015). Here N concentration in the roots of L. davurica under sole cropping was higher than under intercropping, indicating that mixture plantation decreased N enrichment in roots of L. davurica (Figure 1). It also may be caused by strong N acquisition of the neighboring shallow-root grass species B. ischaemum, whose fibrous root system intermingled with the legume roots and acquired the legume-derived N (Xu et al., 2016; Dhamala et al., 2017).
P concentrations in different organs of L. davurica under intercropping decreased compared with its monoculture, especially under +P or +NP treatments. There were two likely processes that may influence P concentration in L. davurica, as its proportion decreased in the mixtures: the first was that P fertilization tended to increase P availability, and the second was that limited P resources from fertilizer and soil was equally used by each single plant of L. davurica. Therefore, the increased numbers in the mixtures would decrease the P reallocation, limiting plant P uptake and P concentration in organs of L. davurica (Mi et al., 2015). Similar results were reported for the soybean-citrus intercropping, where P uptake and distribution amongst the organs of soybean decreased compared with their respective monoculture (Zhou et al., 2006). This contrasts with what was expected that P acquisition would be improved in both legumes and cereals under their mixtures because soil rhizosphere pH decreased, and P activities in the soils and roots (e.g., rhizosphere soil acid phosphatase activities) and rhizosphere soil P concentration increased (Inal et al., 2007; Betencourt et al., 2012). Unfortunately, we did not measure soil rhizosphere pH and phosphatase activity in the soils and roots in this study. P concentration in different organs or whole plant level of L. davurica decreased as its numbers decreased in the mixtures, implying that intraspecific competition intensification may increase its P uptake.
The higher N or P concentrations in the roots than in other organs and the higher N or P concentrations in the leaves than in the stems of L. davurica, could be explained by competition between the plant organs for N and P demand for growth, and the advantage that an organ may have for being close to the nutrient source. This is because nutrients are allocated to distant plant organs, after the demand of the adjacent organs to the nutrient source are met (Yang et al., 2014). However, this contradicts the findings from shrubs, where nutrient concentration in the roots increased in parallel with their neighbors, the stem and the leaves (Yang et al., 2014). Nutrient allocation is also driven by the plant and organ demand for N-rich proteins and P-rich RNAs (Vrede et al., 2004). Additional N and P allocation to the leaves are important to maintain leaf photosynthesis and photosynthate remobilization (Li et al., 2010). In dry environments (or dry years), a high N concentration in the leaves may provide adaptation to drought and competition by exploiting greater light availability while also increasing water use efficiency (Wright et al., 2003; Li et al., 2010; Yang et al., 2014).
Leaf N:P concentration ratio was higher than 16.0 under -NP and +N treatments, indicating a P limitation (Koerselman and Meuleman, 1996; Tessier and Raynal, 2003). Across most treatments (e.g., soil water regime, mixture proportion and fertilization), N:P ratio in leaf and root was significantly lower than 14.0, indicating a N limitation (Craine et al., 2008; Li et al., 2010). At whole plant level, the N:P ratio values were between 14.0 and 16.0 under -NP and +N treatments, while significantly smaller than 14.0 under +P and +NP treatments. These suggest that the species may have low P requirements, and mixture and fertilization changed the P accumulation in L. davurica (Zhou et al., 2006; Hinsinger et al., 2011a). Normally, N:P ratio is higher in structural organs than in metabolically active organs, as indicated here root had significantly lower N:P ratio (Xiao et al., 2004; Kerkhoff et al., 2006; Xu et al., 2013).
Our previous paper showed that the aggressivity values of B. ischaemum were bigger than 0 in their mixtures, which meant that B. ischaemum was the dominant while L. davurica the dominated species in their mixtures. In the study, the pot depth was about 30 cm, and the main soil depth for their root distribution was in 0–20 cm soil depth, which would strengthen the interaction between their root systems (Xu et al., 2015). This would explain why P facilitation may occur rather than competition between the two-species under intercropping. It is likely, that L. davurica was the preferred user of this pool when intercropped with B. ischaemum in comparison with respective monoculture (Li et al., 2008; Xu et al., 2013). Another reason could be that strong competition between them for accessing the same soil horizons because both are native species whose roots mainly distribute in the top soil layer and the small pots may restrict root distribution and proliferation (Li et al., 2006; Zhou et al., 2006; Hinsinger et al., 2011a).
Water is the main factor limiting plant growth and production in arid and semiarid region on the Loess Plateau of China. Nutrient uptake and concentrations in plants would be reduced by drought due to decreased transpiration flow and root growth, and lower soil nutrient mobility (Neugschwandtner and Kaul, 2015, 2016). In the specific water condition, rational fertilization is favorable for plant growth and production (Shan and Chen, 1993; Xu et al., 2016). Here, we found that single plant biomass production of L. davurica increased about 10% under N and/or P fertilization treatments, while the effects were mixture ratio-specific (Supplementary Figure S3). The N and P concentration in organ and whole plant level generally increased as N and/or P fertilizer were applied, while they were not exactly the same for all treatments. Along or with N, P fertilization could not only increased biomass production, but also nutrient concentration in each organ of L. davurica across mixture proportions and soil water regimes. For example, compared with 40%FC soil regime, single plant biomass production of L. davurica under 80%FC increased 35.8% at 10:2 of B. ischaemum to L. davurica after +NP treatment, while N concentration in leaf and whole plant level decreased 10 and 12%, respectively (Figures 1, 4, 5). In the same mixture ratio, compared with 80%FC, the single plant biomass production of L. davurica under 40% FC soil regime decreased about 27% after N fertilization (i.e., +N treatment), but N concentration in whole plant level increased about 10.7% (Figures 1, 5). All these suggested that P fertilization played an important function in improving biomass production and increasing nutrient concentrations in different organs of the legume, and also implied that the improvement of biomass production after fertilization may possess “dilution effect” on nutrient concentration in plant, besides “enrichment effect” (Craine et al., 2008; Neugschwandtner and Kaul, 2015; Li et al., 2016).
On the semiarid Loess Plateau of China, wild and native plant species experience the harsh environment with variable soil water and infertile soil nutrient conditions. Artificial fertilization was normally applied in order to increase their growth, and thus to improve vegetation coverage, accelerate successional process and mitigate desertification in the region. Results showed that P applied alone or combined with N, affected not only the biomass production, but also the N and P accumulation in each component of L. davurica in the mixtures, suggesting that P element should not be neglected when considering rehabilitating degraded grassland through fertilizer application. N and/or P fertilizers significantly affected N and P concentrations at both organ and whole plant levels, and the effects were closely related with mixture proportions, implying that exogenous fertilization may change the N:P stoichiometry of native dominant species in natural grasslands, and such effects may depend on their relative abundance in the community. Our results also provided clearly evidence of inconsistent changes of N:P stoichiometry in the organ and whole plant level under same treatment, suggesting that the organ differences should be considered when studying nutrient absorption and cycling between or among plant species. Because B. ischaemum and L. davurica are perennial species, and here we only reported the nutrient behaviors during the establishment period in their controlled mixtures, it is worthy and necessary to test their nutrient requirements as growth years extended, especially in the field natural conditions. Further research also needs to be taken on the nutrient cycling and balance at the specific mixture proportion in response to water and fertilization treatments to clarify the detailed mechanisms behind.
BX and YC conceived and designed the experiments. WX, ZW, and ZC performed the experiments. WX, ZW, ZC, and JP analyzed the data. BX and JP contributed reagents/materials/analysis tools. BX and YC wrote the paper.
This work was financially supported by the National Natural Science Foundation of China (41071339, 41371509, and 41771553) and Hundred Talents Program of the Chinese Academy of Sciences (A315021449).
The authors declare that the research was conducted in the absence of any commercial or financial relationships that could be construed as a potential conflict of interest.
We are grateful to the three anonymous referees and the associate editor for their insightful comments on previous versions of the manuscript.
The Supplementary Material for this article can be found online at: https://www.frontiersin.org/articles/10.3389/fpls.2018.00165/full#supplementary-material
Aerts, R., and Chapin, F. III. (2000). The mineral nutrition of wild plants revisited: a re-evaluation of processes and patterns. Adv. Ecol. Res. 30, 1–7.
Agren, G. I. (2004). The C: N: P stoichiometry of autotrophs – theory and observations. Ecol. Lett. 7, 185–191. doi: 10.1111/j.1461-0248.2004.00567.x
Betencourt, E., Duputel, M., Colomb, B., Desclaux, D., and Hinsinger, P. (2012). Intercropping promotes the ability of durum wheat and chickpea to increase rhizosphere phosphorus availability in a low P soil. Soil Biol. Biochem. 46, 181–190. doi: 10.1016/j.soilbio.2011.11.015
Chen, H., Shao, M., and Li, Y. (2008). The characteristics of soil water cycle and water balance on steep grassland under natural and simulated rainfall conditions in the Loess Plateau of China. J. Hydrol. 360, 242–251. doi: 10.1016/j.jhydrol.2008.07.037
Chen, Y. L., Dunbabin, V. M., Diggle, A. J., Siddique, K. H. M., and Rengel, Z. (2013). Phosphorus starvation boosts carboxylate secretion in P-deficient genotypes of Lupinus angustifolius with contrasting root structure. Crop Pasture Sci. 64, 588–599. doi: 10.1071/CP13012
Connolly, J., Goma, H. C., and Rahim, K. (2001). The information content of indicators in intercropping research. Agric. Ecosyst. Environ. 87, 191–207. doi: 10.1016/S0167-8809(01)00278-X
Craine, J. M., Morrow, C., and Stock, W. D. (2008). Nutrient concentration ratios and co-limitation in South African grasslands. New Phytol. 179, 829–836. doi: 10.1111/j.1469-8137.2008.02513.x
Dhamala, N. R., Rasmussen, J., Carlsson, G., Søegaard, K., and Eriksen, J. (2017). N transfer in three-species grass-clover mixtures with chicory, ribwort plantain or caraway. Plant Soil 413, 217–230. doi: 10.1007/s11104-016-3088-6
Dijkstra, F. A., Carrillo, Y., Aspinwall, M. J., Maier, C., Canarini, A., Tahaei, H., et al. (2016). Nitrogen and phosphorus use efficiencies of four tree species in response to variable water and nutrient supply. Plant Soil 406, 187–199. doi: 10.1007/s11104-016-2873-6
Dreimanis, A. (1962). Quantitative gasometric determination of calcite and dolomite by using Chittick apparatus. J. Sediment. Petrol. 32, 520–529.
Duan, X., Xie, Y., Liu, G., Gao, X., and Lu, H. (2010). Field capacity in black soil region, Northeast China. Chin. Geogr. Sci. 20, 406–413. doi: 10.1007/s11769-010-0414-4
Editorial Committee (1996). Soil Physical and Chemical Analysis Description of Soil Profiles. Beijing: Standards Press of China.
FAO (2006). World Reference Base for Soil Resources 2006: A Framework for International Classification, Correlation and Communication. World Soil Resources Report, No. 103. Rome: FAO.
Fujita, Y., de Ruiter, P. C., Wassen, M. J., and Heil, G. W. (2010). Time-dependent, species-specific effects of N:P stoichiometry on grassland plant growth. Plant Soil 334, 99–112. doi: 10.1007/s11104-010-0495-y
Garnier, E., Gobin, O., and Poorter, H. (1995). Nitrogen productivity depends on photosynthetic nitrogen use efficiency and on nitrogen allocation within the plant. Ann. Bot. 76, 667–672. doi: 10.1006/anbo.1995.1145
Güsewell, S. (2004). N:P ratios in terrestrial plants: variation and functional significance. New Phytol. 164, 243–266. doi: 10.1111/j.1469-8137.2004.01192.x
Güsewell, S., Koerselman, W., and Verhoeven, J. T. A. (2002). Time-dependent effects of fertilization on plant biomass in floating fens. J. Veg. Sci. 13, 705–718. doi: 10.1111/j.1654-1103.2002.tb02098.x
Han, W., Fang, J., Guo, D., and Zhang, Y. (2005). Leaf nitrogen and phosphorus stoichiometry across 753 terrestrial plant species in China. New Phytol. 168, 377–385. doi: 10.1111/j.1469-8137.2005.01530.x
Hauggaard-Nielsen, H., and Jensen, E. S. (2005). Facilitative root interactions in intercrops. Plant Soil 274, 237–250. doi: 10.1007/s11104-004-1305-1
Hinsinger, P., Betencourt, E., Bernard, L., Brauman, A., Plassard, C., Shen, J., et al. (2011a). P for two, sharing a scarce resource: soil phosphorus acquisition in the rhizosphere of intercropped species. Plant Physiol. 156, 1078–1086. doi: 10.1104/pp.111.175331
Hinsinger, P., Brauman, A., Devau, N., Gérard, F., Jourdan, C., Laclau, J. P., et al. (2011b). Acquisition of phosphorus and other poorly mobile nutrients by roots. Where do plant nutrition models fail? Plant Soil 348, 29–61. doi: 10.1007/s11104-011-0903-y
Inal, A., Gunes, A., Zhang, F., and Cakmak, I. (2007). Peanut/maize intercropping induced changes in rhizosphere and nutrient concentrations in shoots. Plant Physiol. Biochem. 45, 350–356. doi: 10.1016/j.plaphy.2007.03.016
Isaac, M. E., Hinsinger, P., and Harmand, J. M. (2012). Nitrogen and phosphorus economy of a legume tree-cereal intercropping system under controlled conditions. Sci. Total Environ. 434, 71–78. doi: 10.1016/j.scitotenv.2011.12.071
ISSCAS (1978). Institute of Soil Science, Chinese Academy Science. Physical and Chemical Analysis Methods of Soils. Shanghai: Shanghai Science Technology Press.
Jiao, F., Wen, Z. M., and An, S. S. (2013). Soil water storage capacity under chronosequence of revegetation in Yanhe watershed on the Loess Plateau, China. Springerplus 2(Suppl. 1):S15. doi: 10.1186/2193-1801-2-S1-S15
Kerkhoff, A. J., Fagan, W. F., Elser, J. J., and Enquist, B. J. (2006). Phylogenetic and growth form variation in the scaling of nitrogen and phosphorus in the seed plants. Am. Nat. 168, E103–E122. doi: 10.1086/507879
Koerselman, W., and Meuleman, A. F. M. (1996). The vegetation N:P ratio: a new tool to detect the nature of nutrient limitation. J. Appl. Ecol. 33, 1441–1450. doi: 10.2307/2404783
Lambers, H., and Poorter, H. (1992). Inherent variation in growth rate between higher plants: a search for physiological causes and ecological consequences. Adv. Ecol. Res. 23, 188–261. doi: 10.1016/S0065-2504(08)60148-8
Li, A., Guo, D., Wang, Z., and Liu, H. (2010). Nitrogen and phosphorus allocation in leaves, twigs, and fine roots across 49 temperate, subtropical and tropical tree species: a hierarchical pattern. Funct. Ecol. 24, 224–232. doi: 10.1111/j.1365-2435.2009.01603.x
Li, B., Li, Y. Y., Wu, H. M., Zhang, F. F., Li, C. J., Li, X. X., et al. (2016). Root exudates drive interspecific facilitation by enhancing nodulation and N2 fixation. Proc. Nat. Acad. Sci. U.S.A. 113, 6496–6501. doi: 10.1073/pnas.1523580113
Li, H., Shen, J., Zhang, F., Clairotte, M., Drevon, J. J., Le Cadre, E., et al. (2008). Dynamics of phosphorus fractions in the rhizosphere of common bean (Phaseolus vulgaris L.) and durum wheat (Triticumturgidum durum L.) grown in monocropping and intercropping systems. Plant Soil 312, 139–150. doi: 10.1007/s11104-007-9512-1
Li, L., Li, S. M., Sun, J. H., Zhou, L. L., Bao, X. G., Zhang, H. G., et al. (2007). Diversity enhances agricultural productivity via rhizosphere phosphorus facilitation on phosphorus-deficient soils. Proc. Nat. Acad. Sci. U.S.A. 104, 11192–11196. doi: 10.1073/pnas.0704591104
Li, L., Sun, J., Zhang, F., and Guo, T. (2006). Root distribution and interactions between intercropped species. Oecologia 147, 280–290. doi: 10.1007/s00442-005-0256-4
Liu, Z. P., Shao, M. A., and Wang, Y. Q. (2013). Spatial patterns of soil total nitrogen and soil total phosphorus across the entire Loess Plateau region of China. Geoderma 19, 67–78. doi: 10.1016/j.geoderma.2012.12.011
Lü, X. T., and Han, X. G. (2010). Nutrient resorption responses to water and nitrogen amendment in semi-arid grassland of Inner Mongolia, China. Plant Soil 327, 481–491. doi: 10.1007/s11104-009-0078-y
Meng, Q., Fu, B., Tang, X., and Ren, H. (2008). Effects of land use on phosphorus loss in the hilly area of the Loess Plateau, China. Environ. Monit. Assess. 139, 195–204. doi: 10.1007/s10661-007-9826-8
Mi, Z., Huang, Y., Gan, H., Zhou, W., Flynn, D. F. B., and He, J. S. (2015). Leaf P increase outpaces leaf N in an Inner Mongolia grassland over 27 years. Biol. Lett. 11:20140981. doi: 10.1098/rsbl.2014.0981
Neugschwandtner, R. W., and Kaul, H. P. (2014). Sowing ratio and N fertilization affect yield and yield components of oat and pea in intercrops. Field Crops Res. 155, 159–163. doi: 10.1016/j.fcr.2013.09.010
Neugschwandtner, R. W., and Kaul, H. P. (2015). Nitrogen uptake, use and utilization efficiency by oat-pea intercrops. Field Crops Res. 179, 113–119. doi: 10.1016/j.fcr.2015.04.018
Neugschwandtner, R. W., and Kaul, H. P. (2016). Concentrations and uptake of macronutrients by oat and pea in intercrops in response to N fertilization and sowing ratio. Arch. Agron. Soil Sci. 62, 1236–1249. doi: 10.1080/03650340.2016.1147648
Niu, F., Duan, D., Chen, J., Xiong, P., Zhang, H., Wang, Z., et al. (2016). Eco-physiological responses of dominant species to watering in a natural grassland community on the semi-arid Loess Plateau of China. Front. Plant Sci. 7:663. doi: 10.3389/fpls.2016.00663
Qin, J., Xi, W., Rahmlow, A., Kong, H., Zhang, Z., and Shangguan, Z. (2016). Effects of forest plantation types on leaf traits of Ulmus pumila and Robinia pseudoacacia on the Loess Plateau, China. Ecol. Eng. 97, 416–425. doi: 10.1016/j.ecoleng.2016.10.038
Qiu, L., Zheng, F., and Yin, R. (2012). SWAT-based runoff and sediment simulation in a small watershed, the loessial hilly-gullied region of China: capabilities and challenges. Int. J. Sediment Res. 27, 226–234. doi: 10.1016/S1001-6279(12)60030-4
Sardans, J., Rivas-Ubach, A., and Penuelas, J. (2012). The C:N:P stoichiometry of organisms and ecosystems in a changing world: a review and perspectives. Perspect. Plant Ecol. 14, 33–47. doi: 10.1016/j.ppees.2011.08.002
Shan, L., and Chen, G. L. (1993). Theory and Practice of Dryland Farming on the Loess Plateau. Beijing: Chinese Science Press.
Soil Science Society of China (1999). Soil Physical and Chemical Analysis. Beijing: Agricultural Science and Technology.
Tang, X., Bernard, L., Brauman, A., Daufresne, T., Deleporte, P., Desclaux, D., et al. (2014). Increase in microbial biomass and phosphorus availability in the rhizosphere of intercropped cereal and legumes under field conditions. Soil Biol. Biochem. 75, 86–93. doi: 10.1016/j.soilbio.2014.04.001
Tessier, J. T., and Raynal, D. J. (2003). Use of nitrogen to phosphorus ratios in plant tissue as an indicator of nutrient limitation and nitrogen saturation. J. Appl. Ecol. 40, 523–534. doi: 10.1046/j.1365-2664.2003.00820.x
van der Hoek, D., van Mierlo, A. J. E. M., and van Groenendael, J. M. (2004). Nutrient limitation and nutrient-driven shifts in plant species composition in a species-rich fen meadow. J. Veg. Sci. 15, 389–396. doi: 10.1111/j.1654-1103.2004.tb02276.x
Vance, C. P., Uhde-Stone, C., and Allan, D. (2003). Phosphorus acquisition and use: critical adaptations by plants for securing a nonrenewable resource. New Phytol. 157, 423–447. doi: 10.1046/j.1469-8137.2003.00695.x
Vitousek, P. M., Porder, S., Houlton, B. Z., and Chadwick, O. A. (2010). Terrestrial phosphorus limitation: mechanisms, implications, and nitrogen–phosphorus interactions. Ecol. Appl. 20, 5–15. doi: 10.1890/08-0127.1
Vrede, T., Dobberfuhl, D. R., Kooijman, S. A. L. M., and Elser, J. J. (2004). Fundamental connections among organism C:N:P stoichiometry, macromolecular composition, and growth. Ecology 85, 1217–1229. doi: 10.1890/02-0249
Wright, I. J., Reich, P. B., and Westoby, M. (2003). Least-cost input mixtures of water and nitrogen for photosynthesis. Am. Nat. 161, 98–111.
Xia, H. Y., Wang, Z. G., Zhao, J. H., Sun, J. H., Bao, X. G., Christie, P., et al. (2013). Contribution of interspecific interactions and phosphorus application to sustainable and productive intercropping systems. Field Crop Res. 154, 53–64. doi: 10.1016/j.fcr.2013.07.011
Xiao, Y., Li, L., and Zhang, F. (2004). Effect of root contact on interspecific competition and N transfer between wheat and fababean using direct and indirect 15N techniques. Plant Soil 262, 45–54. doi: 10.1023/B:PLSO.0000037019.34719.0d
Xu, B. C., Gao, Z. J., Wang, J., Xu, W. Z., and Huang, J. (2015). Morphological changes in roots of Bothriochloa ischaemum intercropped with Lespedeza davurica following phosphorus application and water stress. Plant Biosyst. 149, 298–306. doi: 10.1080/11263504.2013.823132
Xu, B. C., Gao, Z. J., Wang, J., Xu, W. Z., Palta, A. J., and Chen, Y. L. (2016). N:P ratio of the grass Bothriochloa ischaemum mixed with the legume Lespedeza davurica under varying water and fertilizer supplies. Plant Soil 400, 67–79. doi: 10.1007/s11104-015-2714-z
Xu, B. C., Xu, W. Z., Gao, Z. J., Wang, J., and Huang, J. (2013). Biomass production, relative competitive ability and water use efficiency of two dominant species in semiarid Loess Plateau under different water supply and fertilization. Ecol. Res. 28, 781–792. doi: 10.1007/s11284-013-1061-x
Xu, B. C., Xu, W. Z., Huang, J., Shan, L., and Li, F. M. (2011). Biomass allocation, relative competitive ability and water use efficiency of two dominant species in semiarid Loess Plateau under water stress. Plant Sci. 181, 644–651. doi: 10.1016/j.plantsci.2011.03.005
Yang, X., Tang, Z., Ji, C., Liu, H., Ma, W., Mohhamot, A., et al. (2014). Scaling of nitrogen and phosphorus across plant organs in shrubland biomes across Northern China. Sci. Rep. 4:5448. doi: 10.1038/srep05448
Zhang, F. S., and Li, L. (2003). Using competitive and facilitative interactions in intercropping systems enhances crop productivity and nutrient-use efficiency. Plant Soil 248, 305–312. doi: 10.1023/A:1022352229863
Keywords: intercropping, mixture proportion, soil water, N:P ratio, P fertilization
Citation: Xu B, Xu W, Wang Z, Chen Z, Palta JA and Chen Y (2018) Accumulation of N and P in the Legume Lespedeza davurica in Controlled Mixtures with the Grass Bothriochloa ischaemum under Varying Water and Fertilization Conditions. Front. Plant Sci. 9:165. doi: 10.3389/fpls.2018.00165
Received: 10 October 2017; Accepted: 30 January 2018;
Published: 13 February 2018.
Edited by:
Urs Feller, University of Bern, SwitzerlandReviewed by:
João Coutinho, University of Trás-os-Montes and Alto Douro, PortugalCopyright © 2018 Xu, Xu, Wang, Chen, Palta and Chen. This is an open-access article distributed under the terms of the Creative Commons Attribution License (CC BY). The use, distribution or reproduction in other forums is permitted, provided the original author(s) and the copyright owner are credited and that the original publication in this journal is cited, in accordance with accepted academic practice. No use, distribution or reproduction is permitted which does not comply with these terms.
*Correspondence: Bingcheng Xu, YmN4dUBtcy5pc3djLmFjLmNu Yinglong Chen, eWluZ2xvbmdjaGVuQGhvdG1haWwuY29t; eWluZ2xvbmcuY2hlbkB1d2EuZWR1LmF1
Disclaimer: All claims expressed in this article are solely those of the authors and do not necessarily represent those of their affiliated organizations, or those of the publisher, the editors and the reviewers. Any product that may be evaluated in this article or claim that may be made by its manufacturer is not guaranteed or endorsed by the publisher.
Research integrity at Frontiers
Learn more about the work of our research integrity team to safeguard the quality of each article we publish.