- 1Plant Breeding Institute, Christian-Albrechts-University of Kiel, Kiel, Germany
- 2Lehrfach Variationsstatistik, Christian-Albrechts-University of Kiel, Kiel, Germany
The species Beta vulgaris encompasses wild and cultivated members with a broad range of phenological development. The annual life cycle is commonly found in sea beets (ssp. maritima) from Mediterranean environments which germinate, bolt, and flower within one season under long day conditions. Biennials such as the cultivated sugar beet (B. vulgaris ssp. vulgaris) as well as sea beets from northern latitudes require prolonged exposure to cold temperature over winter to acquire floral competence. Sugar beet is mainly cultivated for sugar production in Europe and is likely to have originated from sea beet. Flowering time strongly affects seed yield and yield potential and is thus a trait of high agronomic relevance. Besides environmental cues, there are complex genetic networks known to impact life cycle switch in flowering plants. In sugar beet, BTC1, BvBBX19, BvFT1, and BvFT2 are major flowering time regulators. In this study, we phenotyped plants from a diversity Beta panel encompassing cultivated and wild species from different geographical origin. Plants were grown under different day length regimes with and without vernalization. Haplotype analysis of BTC1, BvBBX19, BvFT1, and BvFT2 was performed to identify natural diversity of these genes and their impact on flowering. We found that accessions from northern latitudes flowered significantly later than those from southern latitudes. Some plants did not flower at all, indicating a strong impact of latitude of origin on life cycle. Haplotype analysis revealed a high conservation of the CCT-, REC-, BBX-, and PEBP-domains with regard to SNP occurrence. We identified sequence variation which may impact life cycle adaptation in beet. Our data endorse the importance of BTC1 in the domestication process of cultivated beets and contribute to the understanding of distribution and adaption of Beta species to different life cycle regimes in response to different environments. Moreover, our data provide a resource for haplotypes identified for the major floral regulators in beet.
Introduction
To ensure reproductive and therewith evolutionary success, flowering plants have developed different life cycles. Sea beets (Beta vulgaris L. ssp. maritima) are wild relatives of sugar beet (B. vulgaris L. ssp. vulgaris). Annual sea beets from Mediterranean environments germinate, bolt, and flower within one season under long days, whereas most sea beets from northern latitudes are biennial. They need prolonged exposure to cold temperatures (typically during winter) to acquire a floral competent state. Besides, there are perennial sea beets mostly from Northern Europe which exhibit an iteroparous life cycle (Hautekèete et al., 2002). While iteroparous beets revert to vegetative growth after reproduction, annual and biennial beets are semelparous and die after reproduction (Hautekèete et al., 2001). The onset of floral transition in beets is indicated by the elongation of the main shoot which is commonly referred to as “bolting.” In sugar beet cultivation, early bolting (without vernalization) is a trait of high agronomic relevance because it causes severe yield loss. The genetic control of photoperiodic flowering has been elucidated in the model plant Arabidopsis thaliana and many of the identified genes are structurally conserved in all known plants (Capovilla et al., 2014; Pajoro et al., 2014; Blümel et al., 2015).
In beet, major components of the photoperiodic pathway have been identified. The bolting locus BOLTING TIME CONTROL 1 (BTC1) determines the annual life (Pin et al., 2012). BTC1 was identified as a pseudo-response regulator (PRR) gene, sharing sequence homology with the PSEUDO RESPONSE REGULATOR 7 (PRR7) gene from A. thaliana. It encodes for a protein carrying a response regulator receiver (REC) and a CONSTANS, CONSTANS-Like, and TOC1 (CCT) domain. Beets carrying the dominant BTC1 allele mainly reveal an annual growth habit such as most sea beet genotypes, while beets carrying the recessive btc1 allele exhibit a biennial life cycle (Pin et al., 2012). Two FLOWERING LOCUS T (FT) genes, BvFT1, and BvFT2 which are homologous to the Arabidopsis FT, were discovered acting downstream of BTC1 (Pin et al., 2010). BvFT1 and BvFT2, both belonging to the phosphatidylethanolamine-binding protein (PEBP) gene family, have evolved antagonistic functions. While BvFT2 promotes flowering and is required for floral development, BvFT1 acts as a floral repressor. Pin et al. (2012) proposed a model for life cycle control in beet with BTC1 acting upstream of BvFT1 and BvFT2. In annual beets, the dominant BTC1 allele represses BvFT1 and concurrently activates BvFT2 to induce bolting and flowering. On the contrary, in biennial beets the expression of the recessive btc1 allele is increasing gradually to a decreasing expression of BvFT1 during vernalization, enabling the promotion of BvFT2 expression to initiate flowering. The recent discovery of another bolting time regulator BvBBX19 encoding for a DOUBLE B-BOX TYPE ZINC FINGER protein extended the model for bolting time regulation in beet (Dally et al., 2014). BvBBX19 is diurnally regulated and acts epistatically over BTC1 upstream of BvFT1 and BvFT2. Interestingly, BTC1 transcription was reduced in BvBBX19 mutants suggesting a physical interaction of both proteins to jointly regulate BvFT1 and BvFT2 (Dally et al., 2014). In addition to those major regulators, several CONSTANS-LIKE (COL) genes have been detected, differing by their zinc-finger (B-Box) and CCT domains (Chia et al., 2008; Dally et al., 2014). To date, only BvCOL1 has been functionally characterized by overexpression in Arabidopsis (Chia et al., 2008) but it was excluded as a functional ortholog of CO due to non-typical expression profile. Hébrard et al. (2013) compared gene expression and DNA methylation profiles of bolting-resistant and bolting-sensitive beet genotypes after vernalization and determined 169 differentially expressed genes and 111 differentially methylated regions as putative bolting loci. The SBT-9/BR1 locus was discovered to control bolting resistance after winter (Pfeiffer et al., 2014), where a homolog of the Arabidopsis CLEAVAGE AND POLYADENYLATION SPECIFIC FACTOR 73-I (CPSF73-I) was identified as the most promising candidate gene (Tränkner et al., 2016). Recently, Tränkner et al. (2017) proposed that two QTL contribute to variation in seasonal bolting. Besides SBT-9/BR1, the SBT-4 locus was elucidated to majorly control seasonal bolting and BvFT2 was suggested as a candidate gene.
The adaptation to different environments is of central importance for the evolutionary success in flowering plants. In Beta species, adaptation to different geographical regions is processed through the evolution of different life cycles (Hautekèete et al., 2002). It was suggested that the domestication of sugar beet involved the selection of a rare partial loss-of-function allele of BTC1, which alters the plant's response to long day conditions (Pin et al., 2012). A BTC1 haplotype analysis of a large number of Beta accessions and cultivars revealed eleven haplotypes divided into two classes, “annuals” (BTC1d−k) and “biennials” (btc1a−c). These two classes mainly differ by six non-synonymous single-nucleotide polymorphisms (SNPs) as well as a large insertion (~28 kb) within the promoter of biennial btc1 alleles (Pin et al., 2012). Intriguingly, vast majority of cultivated beets carry the recessive btc1a allele while sea beets mainly exhibited BTC1 alleles from the “annual” class.
In contrast, information about BvBBX19, BvFT1, and BvFT2 haplotypes and their abundance among wild and cultivated species is lacking so far. This study aims to understand the role of the four major Beta flowering time regulators BTC1, BvFT1, BvFT2, and BvBBX19 on the adaptation to different environments. We assumed that sequence variations within the coding region of these genes have a major impact on phenological development. Consequently, a non-random distribution of haplotypes across accessions from different geographical origin was expected. Moreover, we reasoned that life cycle changes follow a latitudinal cline. For this purpose, 29 Beta accessions from different geographical origin were grown under standardized conditions and the onset of bolting was recorded. The coding regions of BTC1, BvFT1, BvFT2, and BvBBX19 were sequenced from all accessions and found high variation within BTC1, whereas sequence variation among the other genes was low. A relationship between haplotype variation and life cycle regime could be established. Cultivated beets carry similar combinations of their BTC1, BvBBX19, BvFT1, and BvFT2 haplotypes while sea beets displayed a much higher heterogeneity. These results demonstrate that haplotype variations of flowering time regulator genes are main drivers of the adaptive evolution of Beta species and the domestication of cultivated beet.
Materials and Methods
Plant Material, Growth Conditions, and Phenotypic Analysis
Beta accessions were selected based on geographical diversity and expected bolting characteristics (annual and biennial; Table 1). Seeds were sown in 9 cm2 pots and plants were grown and phenotyped in a climate chamber with 10 plants per accession under different experimental conditions: 22 h of light, 20°C [experiment 1 (E1)], 16 h of light, 20°C [experiment 2 (E2)], and 22 h of light, 20°C interrupted by a cold treatment at 4°C for 3 months [experiment 3 (E3)]. Plants were watered every second day. In experiment 3 plants were fertilized twice, after 119 days directly before vernalization as well as after 210 days directly after vernalization with PERIMOR. The light intensity was held at 315 μmol m−2s−1 and the humidity was about 70%. Bolting (BBCH 51) and flowering (BBCH 60) was recorded according to Meier et al., 1993). Without vernalization, 16 weeks after sowing, plants were classified as annual (bolting) or biennial (non-bolting; experiment 1 and 2). Plants which did not bolt 16 weeks after vernalization were classified as “never bolting” (experiment 3).
Molecular Analysis
The coding region of the flowering time genes BvBBX19, BTC1, BvFT1, and BvFT2 was amplified by PCR. Primers and PCR conditions are listed in Supplementary Tables 1, 2. In silico prediction of the coding gene structures of BTC1, BvBBX19, BvFT1, and BvFT2 and primer positions are indicated in Supplementary Figure 1. DNA was isolated from leaves using the CTAB method (Rogers and Bendich, 1985) with slight modifications. PCRs were performed for single plants and PCR products of all plants of the same accession were diluted to an equal concentration and pooled. Sanger sequencing of all pools was performed at the Institute of Clinical Molecular Biology (IKMB, CAU Kiel). Sequence analysis was done with the CLC Main Workbench 6.9 (CLC bio, Aarhus, Denmark) and the DNASTAR Lasergene SeqMan Pro (DNASTAR Inc., Madison, USA) program packages. Allelic haplotypes were defined by aligning obtained sequences of the amplified fragments and checking for single nucleotide polymorphisms (SNPs) and insertion/deletion polymorphisms. Pooled sequences were blasted against the beet reference sequence (KWS2320Refseq0.9) (Dohm et al., 2013) using the BLASTN function of the CLC Main Workbench 6.9. All SNP positions were numbered beginning with the translation start site. The evaluation of SNPs and their positions was performed according to the IUPAC code (Johnson, 2010; Supplementary Table 3). Polymorphisms were categorized as synonymous (no impact on the amino acid sequence) or non-synonymous.
Statistical Analysis
The software R (R Development Core Team, 2015) was used for statistical analysis. The data evaluation started with the definition of an appropriate statistical mixed model (Laird and Ware, 1982). The data were assumed to be normally distributed and to be heteroscedastic due to the different levels of environments (experiments) and latitude. These assumptions are based on a graphical residual analysis. The statistical model included a pseudo factor (Schaarschmidt and Vaas, 2009), consisting of the actual factors experiment (E1, E2, E3), latitude (21°N-60°N) and varieties (sea beet, sugar beet, table beet, fodder beet, and leaf beet). This pseudo factor was necessary because the actual factors are not orthogonal. The genotype was regarded as a random factor. Based on this model, multiple contrast tests (Bretz et al., 2011) were conducted in order to compare the several levels of (i) variety, (ii) latitude, and (iii) experiment, respectively. Moreover, a further statistical model was established using latitude and experiment as covariates instead of the pseudo factor. On the basis of this model, an analysis of covariance (ANCOVA) was conducted (Cochran, 1957), resulting in (three) different linear regression functions with the same slope.
Results
Large Phenotypic Variation for Flowering Time in Species of the Genus Beta
We chose 29 accessions from different geographical origin to represent the genetic diversity of the species B. vulgaris (Table 1). Of each accession, 10 plants were grown in a climate chamber under three different environmental conditions. The onset of bolting was assessed as beginning of elongation of the main stem (BBCH51) after Meier et al., 1993). In experiment 1 and 2, plants were held under 22 and 16 h of light, respectively. In the third experiment, the same day/night regime as in experiment 1 was applied but biennial accessions were subjected to another 12 weeks of cold treatment (4°C). We uncovered annual and biennial bolting behavior in both wild and cultivated accessions (Figure 1, Supplementary Table 4).
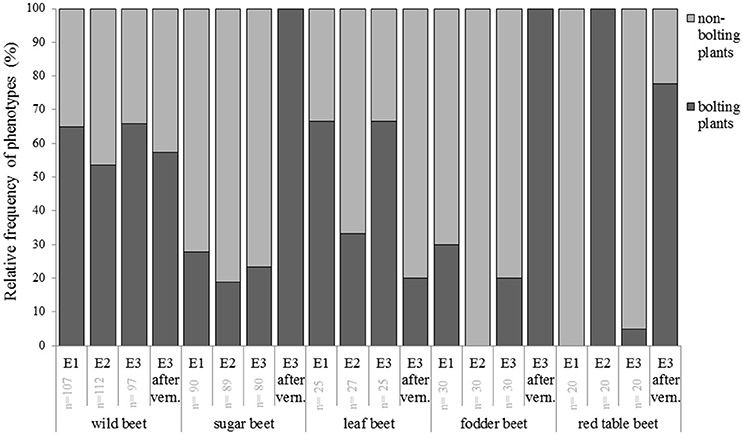
Figure 1. Phenological development of cultivated beet under three different environments (experiment 1–3). Bolting was determined as the beginning of shoot elongation (BBCH51; Meier et al., 1993). Plants were grown in pods in a climate chamber and kept under three different LD conditions: 22 h light, 20°C (E1), 16 h light, 20°C (E2), 22 h light, 20°C, interrupted by 12 weeks of 4°C (E3). The number of cultivars used (n) is indicated in light gray. In E1 and E2 “non-bolting” means that plants did not bolt until 16 weeks after sowing. In E3 “non-bolting” means that plants did not bolt until the end of the experiment (16 weeks after vernalization).
There was a clear tendency for earlier bolting before vernalization under 22 h of light (experiment 1 and 3). On average, annual plants bolted 10 days earlier as when grown under 16 h of light. Six accessions were classified as annual (or segregating for annual and biennial) under 22 h of light while they behaved as biennials under 16 h of light (Figures 2A–C, Supplementary Table 4). The earliest accession, 080437 from Pakistan (31°N), bolted 19 days after sowing when grown under 22 h of light. Under 16 h of light it bolted after 27 days. The earliest accession under 16 h of light was 080468 from Egypt (27°N), which bolted 23 days after sowing. Contrary, 080538 from Great Britain (54°N) was the latest accession under 22 h of light which bolted 69 days after sowing. Interestingly, in experiment 3 only seven out of 10 plants bolted before vernalization, but the remaining three bolted after vernalization. When grown under 16 h of light, accession 080538 performed a biennial life cycle. There was considerable phenotypic variation within accessions under the same experimental conditions. The sea beet accessions 080260 (52°N), 080538 (54°N), 100539 (51°N), and 112787 (46°N) from northern latitudes segregated into annual and biennial plants (22 h of light). Similarly, the cultivated beet accessions 080384 (39°N), 080394 (32°N), and 080396 (39°N) from southern latitudes segregated for bolting under 22 h light (Supplementary Table 4).
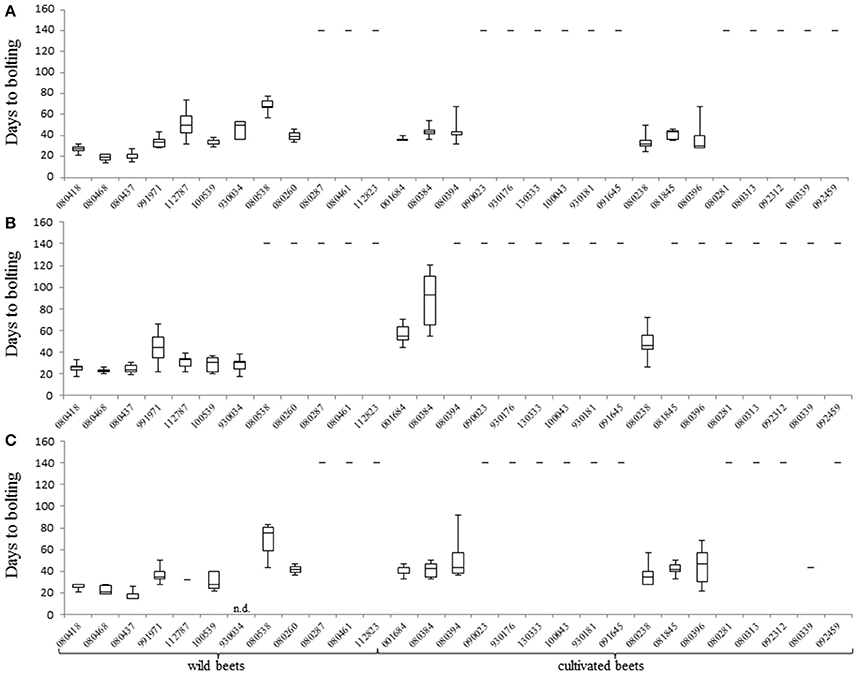
Figure 2. Bolting time measurements for all accessions under different environmental conditions. (A) Days to bolting (DTB) of plants of accessions that bolted before vernalization when held in experiment 1 (22 h light/2 h dark). (B) DTB of plants of accessions when held in experiment 2 (16 h light/8 h dark). (C) DTB of plants of accessions when held in experiment 3 (22 h light/2 h dark). Bolting was determined as DTB after sowing without vernalization. Plants were grown in pods in a climate chamber and kept under LD conditions at 20°C, 315 μmol m−2s−1 and 70% humidity. Plants are separated by wild beet accessions and cultivated beet accessions. For plants of accessions that did not bolt without vernalization the value of days to bolting was set to 140 days. Error bars represent the standard error of the mean (SEM).
We reasoned that the phenological development of Beta genotypes depends on latitude of origin. To test this hypothesis, an analysis of covariances (Cochran, 1957) with data from experiment 1–3 was conducted. This analysis revealed three different linear regression functions with the same slope (Figure 3), suggesting that accessions from southern latitudes of origin flowered earlier than those from northern latitudes. As all regression functions revealed the same slope, we concluded that all environments exert a similar effect of latitude on days to bolting. Additionally, our data show that accessions from northern latitudes exhibited a tendency toward biennial bolting (Supplementary Table 4). Surprisingly, not all cultivated beets displayed a biennial behavior. The leaf beet accession 080238 from Iraq (33°N) revealed an annual life cycle under all experimental conditions (without vernalization). Similar as some sea beet accessions, it bolted earlier under 22 h light (35 days), than under 16 h light (50 days). Moreover, the sugar beet accession 080384 from Turkey (39°N) segregated for bolting and non-bolting before vernalization, while bolting plants had a strong tendency toward early flowering in all experiments. After vernalization, sugar and fodder beets bolted within 26–61 days. However, one leaf beet accession from Italy (42°N) and one table beet accession from Russia (60°N) segregated into bolting and “never bolting” after vernalization. Also, the sea beet accessions 080287, 080461, 112823, and 112787 from Ireland (53°N), Denmark (56°N), Great Britain (54°N), and France (46°N) exhibited a tendency toward “never bolting” (Supplementary Table 4).
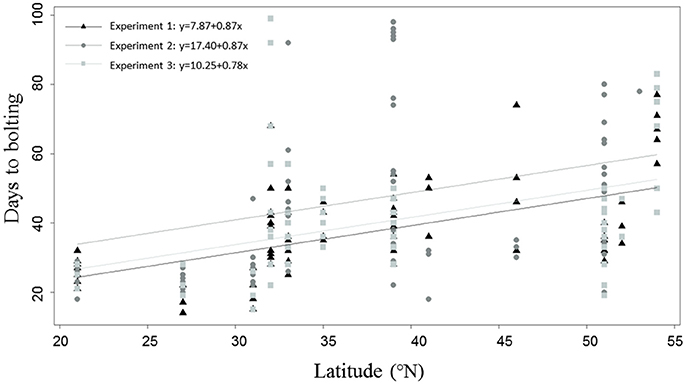
Figure 3. Days to bolting on a latitudinal cline (°N). Bolting plants from experiment 1 are depicted as black triangles. Bolting plants from experiment 2 are depicted as dark gray, filled circles and bolting plants from experiment 3 are depicted as light gray, filled boxes. The statistical model included experiment and latitude as covariates and an analysis of covariances (ANCOVA) was conducted, resulting in different linear regression functions for all environments with the same slope. Plants were grown in pods in a climate chamber and kept under LD conditions (either 16 h of light/8 h dark, 20°C (experiment 2) or 22 h of light/2 h dark (experiment 1 and 3), 20°C, 315 μmol m−2s−1 and 70% humidity). Non-bolting plants are not included in the analysis.
Haplotype Variation of Four Major Flowering Time Regulators
Next, we aimed to link sequence variations and phenological development. For haplotyping, the coding regions of BTC1, BvBBX19, BvFT1, and BvFT2 were sequenced because they had been identified as major constituents of the bolting time regulatory pathway in beet (Pin et al., 2010, 2012; Dally et al., 2014). We sequenced pooled PCR products from single plants of an accession grown in experiment 1. If a pooled DNA sample turned out to be a mixture of different sequences or if segregation into bolting and non-bolting plants was detected, single plants were sequenced.
First, we sequenced the BTC1 coding region (2,367 bp) for each accession and compared it to the reference sequence (Pin et al., 2012). Twenty-five out of 27 SNPs have already been described by Pin et al. (2012) whereas two additional polymorphisms (exon 8 nt2 and exon 9 nt29) turned out to be new (Table 2). Four polymorphic nucleotides are located within the sequence encoding the CCT- and the REC-domain (two in each domain). One of these (exon 3, nt351) represents a non-synonymous mutation from Asparagine to Lysine (Pin et al., 2012). In total, 1 different BTC1 haplotypes were identified among 29 accessions. Of these, three haplotypes have been unknown so far (BTC1l, BTC1m, BTC1n), the remaining eight haplotypes have already been described by Pin et al. (2012) (Table 2). As expected, most of the cultivated (biennial) beet accessions carried the btc1a haplotype (Supplementary Tables 5, 9), which has already been attributed as “biennial” btc1 haplotype (btc1a−c; Pin et al., 2012).
Second, we sequenced the coding region of the BvBBX19 gene (588bp). Sequence variation was much lower as observed for BTC1. We identified one non-synonymous and three synonymous polymorphisms. As expected, none of the accession carried the EMS mutations which had been published by Dally et al. (2014). Interestingly, only one synonymous SNP was located within the region coding for the B-Box-domains. Taken together, all polymorphisms gave rise to seven haplotypes (BvBBX19a−g) across all accessions analyzed in this study (Table 3). The non-synonymous SNP was present only in two haplotypes (BvBBX19f,g). These haplotypes occurred in all leaf beet- and several sea beet accessions, but only in one fodder beet- and one sugar beet accession (Supplementary Table 6). Intriguingly, all accessions with the BvBBX19for BvBBX19ghaplotypes originate from southern latitudes (21–42°N).
Third, we analyzed the coding region of the floral repressor BvFT1 (540 bp). In total, we found one synonymous as well as four non-synonymous SNPs. Additionally, an insertion of 3 bp in exon 1 was identified which occurred only in two accessions. These polymorphisms could be assembled to eight haplotypes (BvFT1a−h; Table 4). Four of the five polymorphisms are located outside the PEBP -domain region. Only two accessions house a polymorphism within the PEBP coding region (exon 4 nt57, haplotype BvFT1c; Supplementary Table 7), indicating a high conservation of this domain.
Fourth, the BvFT2 gene was studied and two non-synonymous polymorphisms giving rise to four haplotypes (BvFT2a−d; Table 5) were identified. One SNP is located within the PEBP-domain region (exon 4 nt39). Remarkably, those haplotypes with the PEBP domain mutation (BvFT2b and BvFT2c) were only present in sea beet accessions from northern latitudes (39–56°N). In contrast, BvFT2a and BvFT2d are highly abundant in cultivated Beta accessions (Supplementary Table 8).
Relation between Haplotype Variation and Life Cycle Regime
We anticipated a link between haplotype variation and life cycle regime which in turn depends on the geographical origin of an accession. First, we looked for a reciprocal relation between haplotypes and phenological development under long day conditions (experiment 1; Supplementary Table 9). As a general rule, cultivated beets which mainly exhibited a biennial life cycle displayed low genetic variation. In the majority, they carried similar combinations of their BTC1, BvBBX19, BvFT1, and BvFT2 haplotypes. Most cultivated sugar beet accessions (090023, 130333, 091645, 100043, and 930176) revealed the “biennial” btc1a and BvBBX19a haplotypes, respectively. Moreover, seven out of nine sugar beet accessions, as well as both red table beet accessions displayed either the BvFT1a and BvFT2a or the BvFT1a and BvFT2d haplotype combination. Sea beets displayed a much higher heterogeneity (between and within accessions) regarding their BvBBX19, BvFT1, and BvFT2 haplotypes whereas most accessions were fixed for only one BTC1 haplotype (exceptions: 991971, 080538, 081845), despite a high sequence variation within this gene across all accessions. Noteworthy, the new BTC1m haplotype only occurred in sea beet accessions from higher latitudes (21.0-39.0°N).
Discussion
We examined 29 Beta accessions from different geographical origins including wild and cultivated beets for phenotypic plasticity under different photoperiodic conditions. Further, the haplotypes of four flowering time regulators, BTC1, BvBBX19, BvFT1, and BvFT2 were analyzed to uncover the relationship between haplotype variation and life cycle adaptation. We found a general southward shift toward earlier flowering. Plants from northern latitudes flowered considerably later or did not flower at all, pointing at a strong coherence of life cycle and geographical origin. Besides, our data revealed a high conservation of the important protein domains (CCT-, REC-, BBX-, and PEBP) for all genes emphasizing their evolutionary relevance for life cycle adaptation in Beta species. Withal, haplotype analysis of BvBBX19, BvFT1, and BvFT2 displayed only a few polymorphisms when compared with the high SNP frequency in BTC1. While most cultivated beets carried similar haplotype combinations of BTC1, BvBBX19, BvFT1, and BvFT2, sea beets displayed much higher heterogeneity. Our findings display several new haplotypes of beet's major floral regulators and connect these to different life cycle regimes.
To warrant evolutionary success of a flowering plant, the adaptation to different climates concomitant with life cycle control is of utmost importance. There are several environmental factors which impact phenotypic plasticity of a flowering plant, such as temperature and photoperiod (Andrés and Coupland, 2012). Species of the genus Beta have evolved different life cycles over the years allowing adaptation to a broad spectrum of latitudes. Besides annuality, beets have evolved biennial and perennial life cycles (Hautekèete et al., 2002), which makes this species an ideal species to study life cycle adaptation. However, prior to this study there was only scant knowledge about the relationship between plant phenology and geographical origin in correlation with genetic diversity of known flowering time genes. What are possible explanations for this relationship particularly with regard to vernalization requirement?
For sea beets (B. vulgaris spp. maritima) a genetically based latitudinal gradient for flowering time along Western European coasts has been shown, together with heritability for flowering time and vernalization requirement. Van Dijk et al. (1997) and Boudry et al. (2002) have demonstrated that differences in life cycle due to vernalization requirement seem to be an adaptive response to season length and spring temperatures in particular latitudes. Van Dijk et al. (1997) sampled seeds from 93 sea beet populations situated along a latitudinal cline around the French coast and in adjacent regions and examined flowering behavior and the relationship between latitude and vernalization requirement under greenhouse conditions. They found that the more southern the origin of plants is the less vernalization is required. While the frequency of flowering phenotypes without vernalization requirement is very high in coastal and inland populations around the Mediterranean, most plants from the Atlantic coast are not able to flower without vernalization. The authors suggested that flowering time in southern parts is controlled by warm temperatures and day length, whereas northern populations have a strong requirement for vernalization. The general importance of vernalization requirement was further emphasized by Van Dijk (2009) who showed that a shortened cold period leads to a complete inhibition of flowering which can be compensated by an artificial increase of photoperiod. Similar observations were obtained by Boudry et al. (2002), who investigated a smaller number of sea beet populations but a higher number of plants along a similar north-south cline of France as Van Dijk et al. (1997). The authors applied several cold regimes to plants of different age over 3 years in the glasshouse and in the field and found that plants from northern origins exhibit a greater requirement for vernalization. Intriguingly they detected that the northernmost population exhibited a lower reproductive success than other populations and thus seemed to be not well adapted. They hypothesized that these findings may underlie inbreeding depression and a lack of genetic variability in small rather isolated populations and that the evolutionary equilibrium has not been reached since the last ice age.
The general aspects of early flowering in wild population have been reviewed by Charnov and Schaffer (1973) who hypothesized that selection for earlier flowering and therewith reproduction underlies a decreased probability of survival. In case of sea beets, a high mortality pressure often occurs especially for inland populations in disturbed environments along roadsides or in sunflower fields, where they are under heavy selective pressure (Boudry et al., 1993). These so-called weed beets are a result of accidental cross-fertilization between ruderal wild beets and crop lineages, mainly found close to seed production fields. The selection for early flowering and a short life history based on high mortality pressure has been suggested for weed beet populations in sugar beet production areas (Van Dijk and Desplanque, 1999).
Even though several studies covered a higher number of populations and plants compared to our study, their observations mainly focus on sea beets from the Mediterranean and the Atlantic coast of France. Our Beta panel, by contrast, displays a broader range of genotypes from different geographical origin and it includes several cultivated beets. We detected a southward shift toward earlier flowering and a complete absence of vernalization requirement of Beta accessions from southern latitudes which is in line with previous studies from Boudry et al. (2002) and Van Dijk et al. (1997). Our findings show that Beta accessions from northern hemispheres flowered later or did not flower at all, hinting at a major effect of vernalization requirement. By contrast, accessions from southern latitudes flowered earlier, especially wild beets. This result is in line with a general observation that in southern latitudes flowering is mediated mainly by spring temperatures, while in northern latitudes winter chilling is a limiting factor (Tooke and Battey, 2010). In our study, three cultivated accessions from southern latitudes (080384, 39°N; 080394, 32°N; and 080396, 39°N) segregated into annual and biennial plants which can be explained by cross-fertilization with sea beets that suffered high mortality pressure as demonstrated by Boudry et al. (1993) and Van Dijk and Desplanque (1999). The very early flowering phenotypes we observed for some accessions from southern latitudes could be the result of southern climate conditions or environmental instability as discussed by Hautekèete et al. (2009).
How can the phenological development of Beta genotypes be explained by genetic variation? In a changing climate, early flowering will be selected for in long day plants (Van Dijk and Hautekèete, 2007). The direct effect of climate change on phenology in sea beets was recently demonstrated by Van Dijk and Hautekèete (2014). They sampled seeds from 73 sea beet populations on Mediterranean and European Atlantic coasts in 2 different years (1989 and 2009) and grew the plants under greenhouse conditions. As a result of natural selection within 20 years, the southern populations shifted toward later flowering, whereas the northern populations flowered earlier. The authors conclude that their findings are based on genetic changes in sensitivity to environmental cues, such as increased temperature over the years. Thus, evidence for genetic change imparting flowering phenology has been given, but the genetic reasons remained in the dark. Today we know that vernalization requirement is a key component of flowering time regulation.
In A. thaliana, the MADS-box gene Flowering Locus C (FLC) plays a central role in regulating vernalization response (Michaels and Amasino, 1999; Sheldon et al., 2000). In B. vulgaris, a vernalization-responsive FLC homolog, FLC-LIKE 1 (BvFL1) has been identified and a conserved function as a floral repressor was suggested after genetic complementation in Arabidopsis (Reeves et al., 2007). Hébrard et al. (2013) detected RNA methylation of the BvFL1 mRNA after vernalization which seemed to indicate its role in vernalization response. However, a recent study clearly demonstrated that RNAi-mediated down-regulation of BvFL1 did not reveal any major effect on bolting without or after vernalization. Moreover, over-expression of BvFL1 only led to a 1 week delay in bolting after vernalization, suggesting that BvFL1 is not a major regulator of vernalization response in beet (Vogt et al., 2014).
Evidently, vernalization requirement is under control of the bolting locus B (Abegg, 1936; Van Dijk et al., 1997; Boudry et al., 2002; Van Dijk, 2009; Pin et al., 2012). Pin et al. (2012) had cloned the BTC1 bolting gene from the B locus and demonstrated for the first time that natural allelic variation of a single gene impacts life cycle variation of beet. The authors suggested that life cycle adaptation results from haplotype diversity of BTC1 which alters the plant's response to long day conditions. Interestingly, only one of the non-synonymous SNPs (exon 3, nt351) was located within the sequence encoding for the CCT-domain. This polymorphism was only present in cultivated beets which carry the btc1a haplotype, indicating a potential target during domestication. In our study eleven BTC1 haplotypes from which three were unknown to date were identified, suggesting a high genetic diversity of the chosen material. Our findings are in line with those of Pin et al. (2012) in a manner that “annual” BTC1 haplotypes primarily occurred in sea beets from southern regions, whereas “biennial” btc1 haplotypes were mainly found in cultivated beets from northern regions. Interestingly, we identified two sea beet accessions from northern latitudes (080287 from Ireland and 080461 from Denmark) which showed a biennial life cycle under all experimental conditions although these genotypes carried an “annual” BTC1 haplotype.
Apart from BTC1, three more genes (BvBBX19, BvFT1, and BvFT2) are major flowering time regulators associated with life cycle adaptation in beet (Pin et al., 2010; Dally et al., 2014). For BvBBX19, it was shown that polymorphisms derived from EMS mutagenesis turned an annual to a biennial beet (Dally et al., 2014). In our study we focused on natural variation within these flowering time genes. We detected four polymorphisms within the coding region of BvBBX19 resulting in seven haplotypes. One SNPs (exon 4, nt59) results in a non-synonymous mutation, while another one (exon 2, nt69) causes a synonymous mutation within the B-Box coding domain. The haplotypes BvBBX19f−g, harboring the non-synonymous mutation, were found exclusively in accessions from southern latitudes. Interestingly, these haplotypes appear in both, wild and cultivated beets and, with one exception, all of these accessions segregated for bolting when grow under 22 h of light. The remaining two SNPs resulted in synonymous mutations which are located outside the B-Box coding domain.
Recently, BvFT2 was proposed as a candidate gene for seasonal bolting time at the SBT-4 locus (Tränkner et al., 2017). We identified two and five polymorphisms within BvFT2 and BvFT1, respectively. Interestingly, for each gene we found one non-synonymous SNP within the PEBP-domain coding sequence. BvFT2 haplotypes which carried this SNP (BvFT2b and BvFT2c) were only present in sea beets from northern latitudes (39–56°N) which primarily exhibited a biennial life cycle. In addition, the BvFT1f haplotype, which displayed a 3bp insertion in the coding region of the PEBP domain, was only identified in the two southernmost sea beet accessions (080437 and 080418) from Pakistan and India which exhibited very early bolting phenotypes. We hypothesize that this insertion may impair the repressing function of BvFT1 in these plants, thus enabling early flowering. Overall, BvFT2 displayed the highest sequence conservation which underpins its importance as a floral integrator. This is in line with other studies where FT functional orthologs that induce flowering are highly conserved in diverse species, such as the rice FT ortholog Heading date3 (Hd3a; Tamaki et al., 2007), SINGLE FLOWER TRUSS (SFT) from tomato (Lifschitz et al., 2006) or CENTRORADIALIS8 (ZCN8) from Zea mays (Lazakis et al., 2011; Meng et al., 2011).
In conclusion, our findings show that geographical origin impacts life cycle adaptation of Beta genotypes. We found that vernalization requirement is absent in sea beet accessions from southern latitudes. A comparison of sequence variation of main flowering time genes between wild and cultivated beets exhibited a general tendency for increased sequence heterogeneity in sea beets. This can be explained by domestication and breeding which resulted in reduced genetic variation within these genes, indicative for selective sweeps. The BvFT1f haplotype which was found in the two southernmost sea beet accessions is of great interest for further studies, because it may represent an example for evolutionary genetic change to enable a short life history due to high mortality pressure in disturbed areas as suggested by Van Dijk and Desplanque (1999). Moreover, the new BvFT2 and BvBBX19 haplotypes may serve as novel resource for beet breeding to broaden the variation for bolting resistance even after winter which is necessary to breed winter beets (Jung and Müller, 2009).
Author Contributions
NH: planned, conducted, and analyzed all experiments and drafted and wrote the manuscript; MH: helped with statistical analyses; ND: participated in designing the study and helped to draft the manuscript; CJ: participated in the design of the study and revised the manuscript. All authors read and approved the final manuscript.
Funding
This study was supported by the International Max Planck Research School for Evolutionary Biology at the Christian-Albrechts-University Kiel and by the DFG Priority Program SPP1530. We gratefully acknowledge financial support by the federal state of Schleswig-Holstein within the funding program Open Access Publikationsfonds.
Conflict of Interest Statement
The authors declare that the research was conducted in the absence of any commercial or financial relationships that could be construed as a potential conflict of interest.
Acknowledgments
We would like to thank Monika Bruisch, Brigitte Neidhard-Olf, and Hilke Jensen for technical assistance and Jay Jethva for helping with phenotyping. We thank Prof. Hinrich Schulenburg and Prof. Arne Nolte for helpful discussions.
Supplementary Material
The Supplementary Material for this article can be found online at: https://www.frontiersin.org/articles/10.3389/fpls.2017.02211/full#supplementary-material
Supplementary Figure 1. In silico prediction of the coding gene structures of BTC1, BvBBX19, BvFT1, and BvFT2. Exons are depicted by cross-striped boxes. Conserved regions encoding for protein domains (REC., CCT-, BB1-, BB2-, and PEPB-domain) are indicated below the exonic structures. 3′- and 5′ UTRs are indicated by arrows above the exons. Identified non-synonymous polymorphisms are indicated by red arrows and synonymous polymorphisms are indicated by blue arrows.
Supplementary Table 1. List of primer combinations and PCR conditions used for sequence analysis.
Supplementary Table 2. List of markers including primer sequences used in this study for PCR and SANGER sequencing.
Supplementary Table 3. IUPAC code for incomplete nucleic acid specification (Johnson, 2010).
Supplementary Table 4. Phenotypic data from three different experiments. Plants were classified as annual (bolting within 16 weeks after sowing) or biennial (bolting only after vernalization). Plants that did not bolt 16 weeks after vernalization were classified as never bolting. Plants were grown and phenotyped in a climate chamber and kept under LD conditions with a light intensity of 900 μE.
Supplementary Table 5. BTC1 haplotypes of all plants from the Beta panel. The coding region was sequenced. The position of the SNPs is given relative to the translation start site according to the regarding exons. For each accession, PCR products of 10 plants were pooled and sequenced for haplotype analysis. In case of sequence heterogeneity, all single plants were sequenced and haplotypes were assembled which could result in more than one haplotypes per accession. The nomenclature of polymorphisms was given according to the IUPAC code (Johnson, 2010).
Supplementary Table 6. BvBBX19 haplotypes of all plants from the Beta panel. The coding region was sequenced. The position of the SNPs is given relative to the translation start site according to the regarding exons. For each accession, PCR products of 10 plants were pooled and sequenced for haplotype analysis. In case of sequence heterogeneity, all single plants were sequenced and haplotypes were assembled which could result in more than one haplotypes per accession.
Supplementary Table 7. BvFT1 haplotypes of all plants from the Beta panel. The coding region was sequenced. The position of the SNPs is given relative to the translation start site according to the regarding exons. For each accession, PCR products of 10 plants were pooled and sequenced for haplotype analysis. In case of sequence heterogeneity, all single plants were sequenced and haplotypes were assembled which could result in more than one haplotypes per accession.
Supplementary Table 8. BvFT2 haplotypes of all plants from the Beta panel. The coding region was sequenced. The position of the SNPs is given relative to the translation start site according to the regarding exons. For each accession, PCR products of 10 plants were pooled and sequenced for haplotype analysis. In case of sequence heterogeneity, all single plants were sequenced and haplotypes were assembled which could result in more than one haplotypes per accession.
Supplementary Table 9. Complete list of all haplotype combinations and phenotypic data. The table also comprises information on growth type and latitude of origin. Rows shaded in light gray indicate accessions which were non-bolting without vernalization under all environments. Cells shaded in dark gray indicate accessions which revealed a non-bolting phenotype under 16 h of light (experiment 2) but an annual phenotype or mixed phenotype (annual + biennial) under 22 h light (experiment 1+3) before vernalization.
References
Abegg, F. A. (1936). A genetic factor for the annual habit in beets and linkage relationship. J. Agric. Res. 53, 493–511.
Andrés, F., and Coupland, G. (2012). The genetic basis of flowering responses to seasonal cues. Nat. Rev. Genet. 13, 627–639. doi: 10.1038/nrg3291
Blümel, M., Dally, N., and Jung, C. (2015). Flowering time regulation in crops — what did we learn from Arabidopsis? Curr. Opin. Biotechnol. 32, 121–129. doi: 10.1016/j.copbio.2014.11.023
Boudry, P., McCombie, H., and Van Dijk, H. (2002). Vernalization requirement of wild beet Beta vulgaris ssp. maritima: among population variation and its adaptive significance. J. Ecol. 90, 693–703. doi: 10.1046/j.1365-2745.2002.00704.x
Boudry, P., Mörchen, M., Saumitou-Laprade, P., Vernet, P., and Van Dijk, H. (1993). The origin and evolution of weed beets: consequences for the breeding and release of herbicide-resistant transgenic sugar beets. Theor. Appl. Genet. 87, 471–478. doi: 10.1007/BF00215093
Bretz, F., Maurer, W., and Hommel, G. (2011). Test and power considerations for multiple endpoint analyses using sequentially rejective graphical procedures. Stat. Med. 30, 1489–1501. doi: 10.1002/sim.3988
Capovilla, G., Schmid, M., and Pose, D. (2014). Control of flowering by ambient temperature. J. Exp. Bot. 66, 59–69. doi: 10.1093/jxb/eru416
Charnov, E. L., and Schaffer, W. M. (1973). Life-history consequences of natural selection: Cole's result revisited. Am. Nat. 107, 791–793. doi: 10.1086/282877
Chia, T. Y. P., Müller, A., Jung, C., and Mutasa-Göttgens, E. S. (2008). Sugar beet contains a large CONSTANS-LIKE gene family including a CO homologue that is independent of the early-bolting (B) gene locus. J. Exp. Bot. 59, 2735–2748. doi: 10.1093/jxb/ern129
Cochran, W. G. (1957). Analysis of covariance - its nature and uses. Biometrics 13, 261–281. doi: 10.2307/2527916
Dally, N., Xiao, K., Holtgräwe, D., and Jung, C. (2014). The B2 flowering time locus of beet encodes a zinc finger transcription factor. Proc. Natl. Acad. Sci. U.S.A. 111, 10365–10370. doi: 10.1073/pnas.1404829111
Dohm, J. C., Minoche, A. E., Holtgräwe, D., Capella-Gutierrez, S., Zakrzewski, F., Tafer, H., et al. (2013). The genome of the recently domesticated crop plant sugar beet (Beta vulgaris). Nature 505, 546–549. doi: 10.1038/nature12817
Hautekèete, N. C., Piquot, Y., and Van Dijk, H. (2001). Investment in survival and reproduction along a semelparity–iteroparity gradient in the Beta species complex. J. Evol. Biol. 14, 795–804. doi: 10.1046/j.1420-9101.2001.00322.x
Hautekèete, N. C., Piquot, Y., and Van Dijk, H. (2002). Life span in Beta vulgaris ssp maritima: the effects of age at first reproduction and disturbance. J. Ecol. 90, 508–516. doi: 10.1046/j.1365-2745.2002.00688.x
Hautekèete, N. C., Van Dijk, H., Piquot, Y., and Teriokhin, A. (2009). Evolutionary optimization of life-history traits in the sea beet Beta vulgaris subsp. maritima: comparing model to data. Acta Oecol. 35, 104–116. doi: 10.1016/j.actao.2008.08.004
Hébrard, C., Trap-Gentil, M. V., Lafon-Placette, C., Delaunay, A., Joseph, C., Lefebvre, M., et al. (2013). Identification of differentially methylated regions during vernalization revealed a role for RNA methyltransferases in bolting. J. Exp. Bot. 64, 651–663. doi: 10.1093/jxb/ers363
Johnson, A. D. (2010). An extended IUPAC nomenclature code for polymorphic nucleic acids. Bioinformatics 26, 1386–1389. doi: 10.1093/bioinformatics/btq098
Jung, C., and Müller, A. E. (2009). Flowering time control and applications in plant breeding. Trends Plant Sci. 14, 563–573. doi: 10.1016/j.tplants.2009.07.005
Laird, N. M., and Ware, J. H. (1982). Random-effects models for longitudinal data. Biometrics 38, 963–974. doi: 10.2307/2529876
Lazakis, C. M., Coneva, V., and Colasanti, J. (2011). ZCN8 encodes a potential orthologue of Arabidopsis FT florigen that integrates both endogenous and photoperiod flowering signals in maize. J. Exp. Bot. 62, 4833–4842. doi: 10.1093/jxb/err129
Lifschitz, E., Eviatar, T., Rozman, A., Shalit, A., Goldshmidt, A., Amsellem, Z., et al. (2006). The tomato FT gene triggers conserved systemic flowering signals that regulate termination and substitute for light and photoperiodic stimuli. Comp. Biochem. Physiol. Mol. Integr. Physiol. 143, S166–S167. doi: 10.1073/pnas.0601620103
Meier, U., Bachmann, L., Buhtz, E., Hack, H., Klose, R., Märländer, B., et al. (1993). Phänologische entwicklungsstadien der Beta-Rüben (Beta vulgaris L.). codierung und beschreibung nach der erweiterten BBCH-skala mit abbildungen. Nachrichtenblatt des Deutschen Pflanzenschutzdienstes. Braunschweig: Biologische Bundesanstalt für Land- und Forstwirtschaft.
Meng, X., Muszynski, M. G., and Danilevskaya, O. N. (2011). The FT-Like ZCN8 gene functions as a floral activator and is involved in photoperiod sensitivity in maize. Plant Cell 23, 942–960. doi: 10.1105/tpc.110.081406
Michaels, S. D., and Amasino, R. M. (1999). FLOWERING LOCUS C encodes a novel MADS domain protein that acts as a repressor of flowering. Plant Cell 11, 949–956. doi: 10.1105/tpc.11.5.949
Pajoro, A., Biewers, S., Dougali, E., Leal Valentim, F., Mendes, M. A., Porri, A., et al. (2014). The (r)evolution of gene regulatory networks controlling Arabidopsis plant reproduction: a two-decade history. J. Exp. Bot. 65, 4731–4745. doi: 10.1093/jxb/eru233
Pfeiffer, N., Tränkner, C., Lemnian, I., Grosse, I., Müller, A. E., Jung, C., et al. (2014). Genetic analysis of bolting after winter in sugar beet (Beta vulgaris L.). Theor. Appl. Genet. 127, 2479–2489. doi: 10.1007/s00122-014-2392-x
Pin, P. A., Benlloch, R., Bonnet, D., Wremerth-Weich, E., Kraft, T., Gielen, J. J. L., et al. (2010). An antagonistic pair of FT homologs mediates the control of flowering time in sugar beet. Science 330, 1397–1400. doi: 10.1126/science.1197004
Pin, P. A., Zhang, W., Vogt, S. H., Dally, N., Büttner, B., Schulze-Buxloh, G., et al. (2012). The role of a pseudo-response regulator gene in life cycle adaptation and domestication of beet. Curr. Biol. 22, 1095–1101. doi: 10.1016/j.cub.2012.04.007
R Development Core Team (2015). R: A Language and Environment for Statistical Computing. Vienna: R Foundation for Statistical Computing.
Reeves, P. A., He, Y., Schmitz, R. J., Amasino, R. M., Panella, L. W., and Richards, C. M. (2007). Evolutionary conservation of the FLOWERING LOCUS C-mediated vernalization response: evidence from the sugar beet (Beta vulgaris). Genetics 176, 295–307. doi: 10.1534/genetics.106.069336
Rogers, S. O., and Bendich, A. J. (1985). Extraction of DNA from milligram amounts of fresh, herbarium and mummified plant tissues. Plant Mol. Biol. 5, 69–76. doi: 10.1007/BF00020088
Schaarschmidt, F., and Vaas, L. (2009). Analysis of trials with complex treatment structure using multiple contrast tests. HortScience 44, 188–195. Available online at: https://apps.webofknowledge.com/full_record.do?product=WOS&search_mode=GeneralSearch&qid=3&SID=C5jsmsNqPRtFmSnwLWo&page=1&doc=1
Sheldon, C. C., Rouse, D. T., Finnegan, E. J., Peacock, W. J., and Dennis, E. S. (2000). The molecular basis of vernalization: the central role of FLOWERING LOCUS C (FLC). Proc. Natl. Acad. Sci. U.S.A. 97, 3753–3758. doi: 10.1073/pnas.97.7.3753
Tamaki, S., Matsuo, S., Wong, H. L., Yokoi, S., and Shimamoto, K. (2007). Hd3a protein is a mobile flowering signal in rice. Science 316, 1033–1036. doi: 10.1126/science.1141753
Tooke, F., and Battey, N. H. (2010). Temperate flowering phenology. J. Exp. Bot. 61, 2853–2862. doi: 10.1093/jxb/erq165
Tränkner, C., Lemnian, I. M., Emrani, N., Pfeiffer, N., Tiwari, S. P., Kopisch-Obuch, F. J., et al. (2016). A detailed analysis of the BR1 locus suggests a new mechanism for bolting after winter in sugar beet (Beta vulgaris L.). Front. Plant Sci. 7:1662. doi: 10.3389/fpls.2016.01662
Tränkner, C., Pfeiffer, N., Kirchhoff, M., Kopisch-Obuch, F. J., van Dijk, H., Schilhabel, M., et al. (2017). Deciphering the complex nature of bolting time regulation in Beta vulgaris. Theor. Appl. Genet. 130, 1649–1667. doi: 10.1007/s00122-017-2916-2
Van Dijk, H. (2009). Evolutionary change in flowering phenology in the iteroparous herb Beta vulgaris ssp. maritima: a search for the underlying mechanisms. J. Exp. Bot. 60, 3143–3155. doi: 10.1093/jxb/erp142
Van Dijk, H., and Desplanque, B. (1999). “European Beta: crops and their wild and weedy relatives,” in Plant Evolution in Man-Made Habitats, eds L. W. D. Van Raamsdonk and J. C. M. Den Nijs (Amsterdam: Hugo de Vries Laboratory), 257–270.
Van Dijk, H., and Hautekèete, N. (2007). Long day plants and the response to global warming: rapid evolutionary change in day length sensitivity is possible in wild beet. J. Evol. Biol. 20, 349–357. doi: 10.1111/j.1420-9101.2006.01192.x
Van Dijk, H., and Hautekèete, N. C. (2014). Evidence of genetic change in the flowering phenology of sea beets along a latitudinal cline within two decades. J. Evol. Biol. 27, 1572–1581. doi: 10.1111/jeb.12410
Van Dijk, H., Boudry, P., McCombre, H., and Vernet, P. (1997). Flowering time in wild beet (Beta vulgaris ssp. maritima) along a latitudinal cline. Acta Oecol. 18, 47–60. doi: 10.1016/S1146-609X(97)80080-X
Keywords: Beta vulgaris, ssp. maritima, vernalization, bolting, phenological development
Citation: Höft N, Dally N, Hasler M and Jung C (2018) Haplotype Variation of Flowering Time Genes of Sugar Beet and Its Wild Relatives and the Impact on Life Cycle Regimes. Front. Plant Sci. 8:2211. doi: 10.3389/fpls.2017.02211
Received: 25 August 2017; Accepted: 15 December 2017;
Published: 04 January 2018.
Edited by:
George N. Skaracis, Agricultural University of Athens, GreeceReviewed by:
Christopher M. Richards, National Laboratory for Genetic Resource Preservation (ARS-USDA), United StatesEnrico Biancardi, Consiglio per la Ricerca in Agricoltura e L'analisi Dell'economia Agraria (CREA), Italy
Andreas Katsiotis, Cyprus University of Technology, Cyprus
Copyright © 2018 Höft, Dally, Hasler and Jung. This is an open-access article distributed under the terms of the Creative Commons Attribution License (CC BY). The use, distribution or reproduction in other forums is permitted, provided the original author(s) or licensor are credited and that the original publication in this journal is cited, in accordance with accepted academic practice. No use, distribution or reproduction is permitted which does not comply with these terms.
*Correspondence: Christian Jung, Yy5qdW5nQHBsYW50YnJlZWRpbmcudW5pLWtpZWwuZGU=