Corrigendum: Factors Affecting Quality and Health Promoting Compounds during Growth and Postharvest Life of Sweet Cherry (Prunus avium L.)
- 1Centre for the Research and Technology of Agro-Environmental and Biological Sciences, University of Trás-os-Montes e Alto Douro, Vila Real, Portugal
- 2Horticulture and Product Physiology, Wageningen University, Wageningen, Netherlands
Sweet cherries are attractive fruits due to their taste, color, nutritional value, and beneficial health effects. Sweet cherry is a highly perishable fruit and all quality attributes and the level of health promoting compounds are affected by growth conditions, picking, packing, transport, and storage. During production, the correct combination of scion × rootstock will produce fruits with higher firmness, weight, sugars, vitamins, and phenolic compounds that boost the fruit antioxidant activity. Orchard management, such as applying drip irrigation and summer pruning, will increase fruit sugar levels and total phenolic content, while application of growth regulators can result in improved storability, increased red coloring, increased fruit size, and reduced cracking. Salicylic acid, oxalic acid, acetylsalicylic acid, and methyl salicylate are promising growth regulators as they also increase total phenolics, anthocyanins, and induce higher activity of antioxidant enzymes. These growth regulators are now also applied as fruit coatings that improve shelf-life with higher antioxidant enzyme activities and total phenolics. Optimizing storage and transport conditions, such as hydro cooling with added CaCl2, chain temperature and relative humidity control, are crucial for slowing down decay of quality attributes and increasing the antioxidant capacity. Application of controlled atmosphere during storage is successful in delaying quality attributes, but lowers ascorbic acid levels. The combination of low temperature storage in combination with modified atmosphere packaging (MAP) is successful in reducing the incidence of fruit decay, while preserving taste attributes and stem color with a higher antioxidant capacity. A new trend in MAP is the use of biodegradable films such as micro-perforated polylactic acid film that combine significant retention of quality attributes, high consumer acceptability, and a reduced environmental footprint. Another trend is to replace MAP with fruit edible coatings. Edible coatings, such as various lipid composite coatings, have advantages in retaining quality attributes and increasing the antioxidant activity (chitosan) and are regarded as approved food additives, although studies regarding consumer acceptance are needed. The recent publication of the sweet cherry genome will likely increase the identification of more candidate genes involved in growing and maintaining health related compounds and quality attributes.
Introduction
Sweet cherry (Prunus avium L.) is one of the most popular table fruits. In 2014, the estimation of the world total cherry production was 2.294 thousand metric tons, with the top three producing countries (Turkey, USA, and Iran) producing 43% (FAO, 2017). Sweet cherry is greatly valued by consumers due to its taste, color, nutritional value, and beneficial health effects. Important quality characteristics of cherry fruits are weight, color, firmness, sweetness, sourness, flavor, and aroma (Crisosto et al., 2006). Sweet cherry is a highly perishable fruit containing significant levels of important nutrients such as potassium, dietary fiber, ascorbic acid, carotenoids, anthocyanins, and phenolic acids with only low caloric content (USDA, 2017). The objective of this review is to provide understanding of the cherry pre-and postharvest changes, and packaging technologies with regard to critical quality indicators and health benefits, thereby updating previous reviews by Predieri et al. (2003), Romano et al. (2006), McCune et al. (2010), and Wani et al. (2014). It also provides a peek on the road forwards with regard to the availability of new technologies. Part of these technologies are related to improving the circumstances in the chain as to gain access to long distance markets. Examples of these are the use of natural compounds and biodegradable films for modified atmosphere packaging (MAP) and edible coatings. The other part is related to improving the properties of the sweet cherry fruit itself; these are presented by showing the recent advances in marker assisted breeding preservation.
Sweet Cherry Quality Factors
Maturity
Fruit maturity is one of the key factors determining overall fruit quality. Cherries should be harvested at the end of the maturation stage when they are fully ripe to ensure a good eating quality (Serrano et al., 2009). Although, the harvest timing varies based on the sweet cherry cultivars, there is harvest window of <5 days for harvesting fruit of optimal quality. The harvest season for sweet cherries is short and labor intensive. Ripe fruit are prone to mechanical damage and cooling directly after harvest is important (Dever et al., 1996; Chauvin et al., 2009).
During early developmental stages, skin color changes, and softening starts, glucose, and fructose accumulate, together with a rapid increase in fruit size. In later development stages, ascorbic acid and anthocyanin accumulate, antioxidant activity increases, and fruit flesh darkens (Serrano et al., 2005). A significant increase of total phenols was obtained in “Pico Negro” cherries during ripening and consequently, an increase in antioxidant activity, which may have beneficial health effects (Serradilla et al., 2012). Early-harvested cherries suffered from low acceptance due to the low sweetness while late-harvested cherries showed low acceptance due to low texture (Chauvin et al., 2009). Nevertheless, higher sensory scores were obtained for late-harvested sweet cherry fruits, especially for skin color and flavor intensity (Chauvin et al., 2009; Serradilla et al., 2012). Harvested cherries show weight loss, changes in the sugar-acid balance, color, softening, and stem browning (Kappel et al., 2002; Bernalte et al., 2003; Alique et al., 2005). During postharvest storage (4 days at 20°C) “Ambrunés” fruits showed a decrease in sugar levels, skin color, firmness, and TA, whereas SSC remained fairly stable, decreasing from 15.2 to 14.8°Brix. Malic acid levels decreased up to 20%, which may indicate that malic acid is an essential substrate for respiration in cherries (Alique et al., 2005).
Assessment of the optimal maturity in the orchard is not straightforward due to the variation in maturity within a tree, but especially within an orchard. Orchard mapping can support growers finding the correct harvest window by sampling°Brix and firmness during growth, just as now is being applied for dry matter assessment per plot for mango (Subedi et al., 2013). This type of quality mapping (Zude-Sasse et al., 2016) may be helpful to scheduling future cherry harvest campaigns.
Color, Firmness, and Water Loss
Cherry fruit quality traits related to consumer purchase decisions are based on external quality attributes such as color, fruit size, stem freshness, absence of defects, and stem length (Predieri et al., 2004). Aroma, flavor, sourness, sweetness, and texture are also essential attributes (Romano et al., 2006; Díaz-Mula et al., 2009). Fruit color is, however, the main quality trait (Mozetič et al., 2004). Color development has been studied as function of cultivar, ripeness stage, and storage conditions (Esti et al., 2002; Gonçalves et al., 2007; Pérez-Sánchez et al., 2010). Large variation exists amongst sweet cherry cultivars grown in Italy with the skin color variation classified as dark—(e.g., “Black Star” and “Moreau”) and light type cultivars (e.g., “Gabbaladri” and “Napoleona Verifica”; Ballistreri et al., 2013). Dark red blush cultivars have a higher consumer preference when comparing with full bright red cherries (Pérez-Sánchez et al., 2010). Color change during maturation is mostly due to an increase in anthocyanin content, specifically, cyanidin-3-O-rutinoside and cyanidin-3-O-glucoside (Mozetič et al., 2004; Gonçalves et al., 2007; Serrano et al., 2009). Transcriptomic studies in cherry fruit maturation have been carried out to elucidate the role of abscisic acid (ABA) and ethylene (Ren et al., 2011) and ripening (Luo et al., 2014). PacNCED1 transcription induced ABA synthesis and accumulates during cherry ripening, which should play a crucial factor in regulation on the sweet cherry fruit ripening (Ren et al., 2011; Luo et al., 2014). The ethylene production via regulation of PacACO1 expression (1-aminocyclopropane-1-carboxylic acid oxidase) might be stimulated with exogenous ABA, which indicates that the ethylene is synthesized by cherries (Ren et al., 2011) to start anthocyanin biosynthesis (Luo et al., 2014).
Positive effects of light on biosynthesis of phenolic compounds, mainly flavonoids are reviewed by Zoratti et al. (2014). Extensive studies have been carried out on the effect of supplemental UV-light treatments to improve anthocyanin levels in sweet cherry skin (Arakawa, 1993; Kataoka et al., 1996). Moreover, the application of UV-C light in postharvest has also been mentioned as a promise tool for extension of fruit shelf life, by delaying fruit senescence, increasing flavonoid content, and antioxidant activity in fruits (Wang et al., 2009; Crupi et al., 2013; Rivera-Pastrana et al., 2013; Li, 2014). The application of blue light emitting diodes (LEDs) to increase anthocyanin levels in cherries could be a reality soon (Arakawa et al., 2017), increasing both color and health promoting potential.
In general, consumers prefer cherries fully ripe based on their dark skin color (Crisosto et al., 2003). However, consumers chose partially ripe over full ripe “Sweetheart” cherries (Chauvin et al., 2009) which indicates the importance of a number of less well understood attribute interactions in consumer acceptance. In general, the chromatic values at harvest are lower at full ripeness stage than at the partially ripe stage. During postharvest storage, a further reduction in lightness (L*) and hue angle (h°) values were observed which indicates that the cherries became darker (Gonçalves et al., 2007). Firmness is related to storability, providing resistance to fruit deterioration and mechanical injury (Esti et al., 2002). Late season cultivars are frequently firmer than early season cultivars (Usenik et al., 2005). Fruit firmness at harvest varies greatly, from 3.3 for “Moreau” to 27 N for “Minnulara” cherries (Ballistreri et al., 2013). Firmness at harvest of 17 sweet cherry cultivars varied between 2.56 and 4.71 N and was judged acceptable for 73–92% of the panelists (Hampson et al., 2014). Softening is due to an increased peroxidase (POD), polyphenoloxidase (PPO), pectinmethylesterase (PME), polygalacturonase (PG), and β-galactosidase (β-Gal) activities. The level of these enzymes increased around 2 to 2.5-fold during a 5-day storage period causing a damage of cell wall components and subsequent softening (Remón et al., 2003).
The fruit cuticle likely plays an important role with respect to postharvest performance. The sweet cherry fruit cuticle is composed of a cutin polymer matrix, which consists essentially of esterified hydroxy- and epoxyhydroxy fatty acids with a chain length of 16 or 18 C-atoms and embedded in cuticular and surface-deposited epicuticular waxes (Peschel et al., 2007). During cherry development, the levels of C16 and C18 monomers decreased (Peschel et al., 2007), but increased during cold storage (Belge et al., 2014). During early sweet cherry fruit development, the cessation of cuticular membrane deposition is due to downregulation of genes involved in cuticular membrane deposition, such as PaATT1, PaCER1, PaGPAT4/8, PaLACS 1, PaLACS2, PaLCR, PaLipase, PaLTPG1, PaWINA, and PaWINB (Alkio et al., 2012). The main function attributed to the fruit cuticle is limiting water permeability, susceptibility to infections, and physiological disorders such as fruit cracking. The fruit cuticle composition is also associated with postharvest water loss from fruit respiration and firmness changes (Lara et al., 2014). Cherries have a high respiration rate which leads to softening that is partly due to water loss. Dehydration during postharvest storage might induce PacNCED1 transcription and the accumulation of ABA resulting in ethylene production and subsequent fruit senescence (Luo et al., 2014). Water loss may lead to an increased susceptibility to infections and mechanical injuries (Esti et al., 2002). During postharvest storage, in particular for longer periods, fungal spoilage can lead to considerable economic losses (Conte et al., 2009; Romanazzi et al., 2009). The development of off-flavors is promoted by bacteria and fungi due to ethanol and acetaldehyde synthesis (Esti et al., 2002).
Taste
Cherry fruit taste attributes, sweetness, and sourness, are important for consumer acceptance. Sweetness can be expressed as soluble solids content (SSC), sourness as titratable acidity (TA) with the ratio (SSC/TA) regarded as overall taste attribute (Guyer et al., 1993; Crisosto et al., 2002). SSC values varied from 12.3 to 23.5°Brix (González-Gómez et al., 2009) and varied from 14.7 to 23.7°Brix for Spanish sweet cherry cultivars (Pérez-Sánchez et al., 2010). SSC values ranged from 18.1 to 19.3 and from 17.8 to 19.8°Brix for Brazilian and Chinese sweet cherries, respectively (Rios de Souza et al., 2014; Wen et al., 2014). TA values ranged from 0.20 in “Lapins” to 0.28 for the Canada Giant cultivars (Vavoura et al., 2015). High TA affects taste when the SSC value is below 16°Brix in “Brooks” or below 13°Brix in “Bing” (Crisosto et al., 2003). The optimal SSC/TA ratio is between 1.5 and 2.0, with SSC values ranging between 17 and 19°Brix (Kappel et al., 1996).
Sweetness and flavor intensity are important parameters for sweet cherry liking (Romano et al., 2006). The most important sensory features are related to the ripening stage, parameters linked with accumulation of organic acids and aromatic alcohols (Serradilla et al., 2012). Several pathways in the biosynthesis of volatiles originate from amino acids, membrane lipids, and carbohydrates contributing for the ripe fruit flavor (Hadi et al., 2013). The aroma profile of sweet cherries is mainly the result of a complex mixture of alcohols, carbonyls, and organic acids (Mattheis et al., 1992; Girard and Koop, 1998; Bernalte et al., 1999; Zhang et al., 2007; Serradilla et al., 2012; Vavoura et al., 2015). Hexanal and ethyl-2-hexenal are the most relevant compounds in the aroma profile of sweet cherries, that together with ethyl-2-hexen-1-ol, are associated with fresh green odors and green notes (Zhang et al., 2007; Serradilla et al., 2012). Benzaldehyde, originates from the enzymatic hydrolysis of amygdalin, is likely the main contributor to the characteristic flavor of sweet cherry (Zhang et al., 2007). Other important contributors are hexanoic acid, described as floral (Pherobase, 2007), and acetic acid, the main volatile acid found in sweet cherry fruits (Serradilla et al., 2012). Significant changes in volatile composition have been observed during ripening of sweet cherry (Zhang et al., 2007; Serradilla et al., 2012). The branched-chain alcohols, 3-methyl-3-buten-1-ol, and 3-methyl-2-buten-1-ol increase during ripening, whereas the organic acids, tetradecanoic and hexadecanoic acid, decrease during ripening, which may be due to reactions between alcohols and organic acids, catalyzed by alcohol acyltransferases. These enzymes seem to play a crucial role in ester biosynthesis (Olías et al., 1995; Serradilla et al., 2012). High variation was recorded in the composition of volatiles amongst four sweet cherry cultivars grown in Spain (Serradilla et al., 2012). “Sweetheart” cherries were mainly characterized by higher levels of organic acids, such as 9-hexadecenoic acid, hexadecanoic acid, and aromatic alcohols and “Ambrunés” cherries by higher sweetness levels and presence of aliphatic alcohols. “Pico Colorado” cherries showed higher levels of hexanal and “Pico Negro” cherries were characterized by higher levels of branched alcohols, mainly 3-methyl-2-buten-1-ol and aromatic aldehydes, such as benzaldehyde. Similar research with four sweet cherry cultivars grown in Greece reported that “Ferrovia” and “Skeena” cherries showed higher levels of carbonyl compounds (2-propanone and 2-hexenal) and alcohols compared to “Lapins” and “Canada” cherries (Vavoura et al., 2015).
Health Promoting Compounds
The role of several classes of health promoting compounds that are present in sweet cherry, lowering the risk of cancer, cardiovascular disease, diabetes, and other inflammatory diseases, has been recognized and is a field of many animal and now increasingly also epidemiological studies (McCune et al., 2010).
Phenolic Compounds
Sweet cherry phenolic compounds exhibit high antioxidant activity (Gonçalves et al., 2004a; Serrano et al., 2005; Kelebek and Selli, 2011; Serra et al., 2011). These compounds consist of flavonoids, flavan-3-ols, and flavonols and non-flavonoid compounds like hydroxycinnamic and hydroxybenzoic acids (Macheix et al., 1990; Gao and Mazza, 1995; Gonçalves et al., 2004a). Phenolic compounds, concentrated in the fruit skin, increase during ripening together with polyphenolic compounds and anthocyanins that color the cherry skin from green to red (Mozetič et al., 2004; Gonçalves et al., 2007). Anthocyanins and flavonols contribute to sensory and organoleptic properties of fruits (Ferretti et al., 2010). Table 1 presents an overview of the levels of phenolic compounds found in several sweet cherry cultivars. The most abundant phenolic compounds are anthocyanins (Mozetič et al., 2002; Gonçalves et al., 2004a; Usenik et al., 2008) such as cyanidin-3-O-rutinoside and cyanidin-3-O-glucoside, peonidin-3-O-rutinoside and glucoside, as well as pelargonidin-3-O-rutinoside (Gonçalves et al., 2007). Anthocyanins have health promoting properties. For instance, cyanidin-3-O-rutinoside slows down absorption of carbohydrates which may help prevent or treat diabetes mellitus (Adisakwattana et al., 2011) and cyanidin-3-O-glucoside showed cardio-protective effects by reducing blood lipid levels (Xia et al., 2005). Several studies reported higher phenolic content (Gonçalves et al., 2004a; Serrano et al., 2009), SSC, TA and antioxidant activity (Serrano et al., 2009) in ripe cherries than in partially ripe. Sweet cherry contains phenolic acids such as hydroxycinnamic acid derivatives (neochlorogenic, p-coumaroylquinic, and chlorogenic acids; Gonçalves et al., 2004a; Usenik et al., 2010; Liu et al., 2011), flavonols (quercetin-3-glucoside, quercetin-3-rutinoside, and kaempferol-3-rutinoside) and flavan-3-ols (catechin and epicatechin), as shown in Table 1 (Gonçalves et al., 2004a; Mozetič et al., 2006; Usenik et al., 2008; Jakobek et al., 2009). Consumption of cherries might induce health benefits such as inhibition of tumor growth (Kang et al., 2003; Serra et al., 2011), inhibition of inflammation (Seeram et al., 2001; Jacob et al., 2003) and protection against neurodegenerative diseases (Kim et al., 2005). These fruits are also considered an excellent source of polyphenols, such as tannins (Tomás-Barberán and Espín, 2001). Tannins can be classified in hydrolysable tannins (gallic acid polymerization) and condensed tannins or proanthocyanidins (catechin polymerization) (Macheix et al., 1990). Tannins are also secondary metabolites that confer astringency and have health-promoting properties (Tomás-Barberán and Espín, 2001; McCune et al., 2010). Tannin content ranged from 32 to 75 mg 100 g−1 of fresh weigh for Serbian sweet cherry cultivars (Prvulović et al., 2012). Sweet cherry extracts might be a serious candidate to prevent oxidative stress-induced disorders like intestinal inflammation disorders and neuronal cell death (Matias et al., 2016).
Vitamins and Carotenoids
Cherries are rich in C, A, E, and K vitamins and carotenoids, specially β-carotene, lutein, and zeaxantine (Ferretti et al., 2010; Leong and Oey, 2012). Ascorbic acid (vitamin C) levels vary between 4 and 7 g kg−1 of fresh weight among seven sweet cherry cultivars grown in Turkey (Demir, 2013), whereas the ascorbate levels for 22 sweet cherry cultivars grown in southern of Italy was considerably lower, varying from 0.034 to 0.260 g kg−1 of fresh weight (Matteo et al., 2016). Carotenoids, such as β-carotene, β-cryptoxanthin, and α-carotene have been reported in sweet cherry fruit (Leong and Oey, 2012; Demir, 2013; Matteo et al., 2016). These compounds are the main precursors of vitamin A and responsible for the red, yellow and orange hues in the cherry skin. β-carotene, β-cryptoxanthin and α-carotene, lycopene, and lutein are present in cherry at levels of 0.02 mg g−1 dry weight (Leong and Oey, 2012). β-carotene, β-cryptoxanthin, and α-carotene, zeaxantine were identified in seven sweet cherry cultivars in small quantities varying from 0.2 to 16 ppm of fresh weight (Demir, 2013). Cherry carotenoids levels are generally quite low, and likely do not present a large contribution to human health.
Nowadays an increasing trend in society is health consumerism, for example functional drinks with cherry extracts rich in bioactive compounds (Sun-Waterhouse, 2011). However, it is important to evaluate the impact of natural ingredients on sensory properties and consumer acceptance, as the optimal combination of taste and health promoting compounds is difficult to achieve (Corbo et al., 2014).
How Do Growth Conditions Affect Fruit Quality and the Level of Health Promoting Compounds?
Scion × Rootstock Interaction
Several studies have shown the influence of the scion × rootstock combination on cherry fruit quality (Schmitt et al., 1989; Facteau et al., 1996; Shackel et al., 1997; Szot and Meland, 2001; Whiting et al., 2005). Fruit firmness varies with scion x-rootstock combination. For instance, “Burlat” cherries are softer when grafted on CAB 11E (clone of Prunus cerasus L.; semi-vigorous) and firmer when grafted on Gisela 5 (P. cerasus × P runus canescens Bois. hybrid; dwarfing) or P. avium (Gonçalves et al., 2006). Fruits grown on PiKu 1 and Weiroot 13 rootstocks had high levels of chlorogenic acid, neochlorogenic acid, p-coumaric acid and quercetin-3-rutinoside compared to F12/1, Gisela 5, Maxma 14, and Weiroot 158 rootstocks (Jakobek et al., 2009). The lowest stone weight (7% of total fruit weight) and highest level of total sugars (250 g kg−1 fresh weight) was observed for “Lapins” on F12/1 rootstock, whereas PiKu 1 rootstock resulted in the highest yield (20 kg tree−1). The Weiroot 13 rootstock promoted the highest content of total organic acids (9 g kg−1 of fresh weight) and the Edabriz rootstock resulted in the highest SSC (15°Brix). “Lapins” grown on the Weiroot 72 rootstock presented higher fruit weight, firmness, SSC, and phenols content (Usenik et al., 2010). Choosing the right rootstock continues to be widely studied due to its importance for productivity (Koc et al., 2013) but the effects on fruit quality are also of increasing interest (Milinović et al., 2016).
Orchard Management
Nowadays, the big challenge in cherry production is to reduce the costs and the labor requirements. This implies the use of the right rootstock and the best canopy architecture. Mechanization of pruning and a fully mechanical harvest system will facilitate achieving higher yield and fruit quality (Lang, 2017).
Summer pruning, which can provide adequate vigor and good light penetration throughout the canopy, and a high leaf area per fruit are important factors for the production of high quality fruits (Whiting and Lang, 2004). Tree location in the orchard and fruit position in the tree are the most important factors that affect fruit weight (Drake and Fellman, 1987; Flore and Layne, 1999). Fruit size and sweetness decrease with increasing plant density, which indicates competition between trees (Eccher and Granelli, 2006). High light intensity increases ascorbic acid content, and high temperature enhances anthocyanin and total phenolic contents (Wang, 2006).
Canopy architectures, such as Y-trellised and vertical, Tall Spindle Axe, Kim Green Bush, Super Slender Axe, and Upright Fruiting Offshoots, have recently been implemented in several orchards (Lang et al., 2017) with the aim to improve the crop loading, the light input, as well as, the increase of health relating compounds content in cherries.
Especially in Mediterranean areas, due to water scarcity, improvement of cherry production under water stress is essential. Several studies evaluated irrigation systems with regard to productivity and fruit quality (Demirtas et al., 2008; Yin et al., 2011; Neilsen et al., 2014). Drip irrigation applied to “Lapins” cherry trees consumed only 21–29% of the irrigation water compared to micro-sprinkler irrigation, enhanced marketable fruit by 7 to 12% and did not impact fruit yield or firmness, color, and size (Yin et al., 2011). Low-frequency drip irrigation increased SSC in “Cristalina” and “Skeena” cherries (Neilsen et al., 2014). However, irrigation levels did not affect SSC, pH, TA, and fruit weight of the cherries of the 900-Ziraat cultivar (Demirtas et al., 2008). Water management techniques, such as regulated deficit irrigation (RDI) and partial rootzone drying (PRD) may enhance fruit taste without reduction of yield (Ripoll et al., 2016). Applying RDI, to “Summit” sweet cherry trees after the crop was harvested did not impact yield but could save water up to 45% without affecting firmness and limiting thinning costs (Marsal et al., 2010). Limiting water supply during cherry tree development, either by drip irrigation or RDI, may help to produce healthier food a more sustainable manner (Nora et al., 2012), and would especially be important taking into account the effects of climate change.
Cherry production depends on the pollinators and utilization of pollinizer cultivar in the orchard. However, in a near future, these can be replaced by artificial precision pollination systems using liquid pollen suspensions applied with electrostatic sprayer technology (Whiting and Das, 2017).
Growth Regulators
The application of calcium and growth regulators improves cherry quality (Table 2). Application of Ca(OH)2 0.7% reduced cracking and fruit size and increased SSC, firmness, and calcium levels in both fruit flesh and skin (Demirsoy and Bilgener, 1998a). Sweet cherry trees sprayed with CaCl2 0.5% once a week from petal fall until 2 weeks before harvest showed higher SSC and higher levels of phenolics, reduced decay, and cuticular fractures, but showed higher weight loss during storage (Vangdal et al., 2008). Preharvest application of ethephon reduced both fruit removal force and fruit flesh firmness at harvest (Elfving et al., 2009). Fruits treated with 20 ppm gibberellic acid (GA3) at the green to straw-yellow stage showed slower softening with lower PG and cellulase activity, but no effects on SSC and β-Gal activity (Choi et al., 2002). GA3 can induce, depending on the cultivar, increased fruit weight and SSC, including delayed ripening and reduced fruit cracking (Usenik et al., 2005). GA3 application increased fruit firmness of “Lapins” and “135-27-17”cherries, but no effect was observed in “Celeste” and “Merpet” fruits (Choi et al., 2002). Similar preharvest applications of GA3 (25 ppm) at the straw-yellow stage of the “0900 Ziraat” cherries showed increased fruit size and firmness (Canli and Orhan, 2009). Applications of GA3 (30 and 60 mg L−1) to cherries at the straw-yellow stage of the Regina, Sweetheart and 0900 Ziraat cultivars retarded fruit ripening and preserved flesh firmness. However, these fruits showed a decrease in total phenolics, anthocyanins, and antioxidant activity (Ozkan et al., 2016). Preharvest application of 25, 50, and 100 mg L−1 GA3 and GA3 combined with 0.05, 0.1, and 0.5 mg L−1 22S, 23S-homobrassinolide (HBR) to “Regina” and “Summit” cherries at full bloom and at the beginning of fruit development increased fruit size and decreased SSC (Engin et al., 2016). Fruits treated with only HBR showed higher fruit firmness of the flesh and stem resistance (Engin et al., 2016). At the straw-yellow stage applications of “Noir de Guben” cherries with 50, 100, and 150 ppm benzyladenine (BA) and BA combined with 12.5, 25, and 50 ppm gibberellins (GA4+7) improved size and delayed skin color change (Canli et al., 2015a). BA alone or in combination with GA4+7 improved storability by maintaining firmness during 30 days in cold storage (4°C) (Canli et al., 2015b). GA3 applied at stage I (mesocarp growth consisting of both cell division and elongation) and stage II (endocarp hardening and embryo development) improved final “Bing” fruit weight, but higher fruit weight was also obtained when combined with GA4+7 (Zhang and Whiting, 2011). Application of salicylic acid (SA) and acetylsalicylic acid (ASA) at 0.5 and 1 mM at three key points of fruit development improved fruit weight up to 59%, increased firmness and increased anthocyanin and total carotenoid content (Giménez et al., 2014). Application of 2 mM oxalic acid (OA) increased fruit size up to 23%, increased levels of anthocyanins, flavonols, neochlorogenic, and chlorogenic acids (Martínez-Esplá et al., 2014). The growth regulators application improves final fruit size due to the stimulating cell division (Olmstead et al., 2007). However, the successful application of these compounds is highly dependent on climatic conditions and time of application. Methyl salicylate (MeSA) application increased fruit size, red coloring, firmness, and SSC (Giménez et al., 2015). MeSA also increased total phenolics, anthocyanins, and induced higher activity of antioxidant enzymes such as ascorbate peroxidase, catalase, peroxidase, and superoxide dismutase at harvest (Valverde et al., 2015). Interestingly, MeSA coatings affect postharvest quality of cherry fruits while still attached on the tree. Total phenolic and anthocyanin contents, total antioxidant activity and antioxidant enzymes were higher in MeSA-treated fruits during storage (Valverde et al., 2015), while also delaying postharvest ripening due to less coloring, lower acidity, and less softening in treated fruits after storage (Giménez et al., 2015). Application of preharvest coatings may be an exciting new trend in research that could be instrumental to enable further access to long distance markets as both storability and the levels of health promoting compounds are improved. In Portugal, the cherry growers are currently applying biostimulants based on seaweed and tertiary ammonium compounds (such as glycine betaine), and it is likely that, observing the positive (unpublished) results that have been obtained, that application of these compounds might be common in the near future.
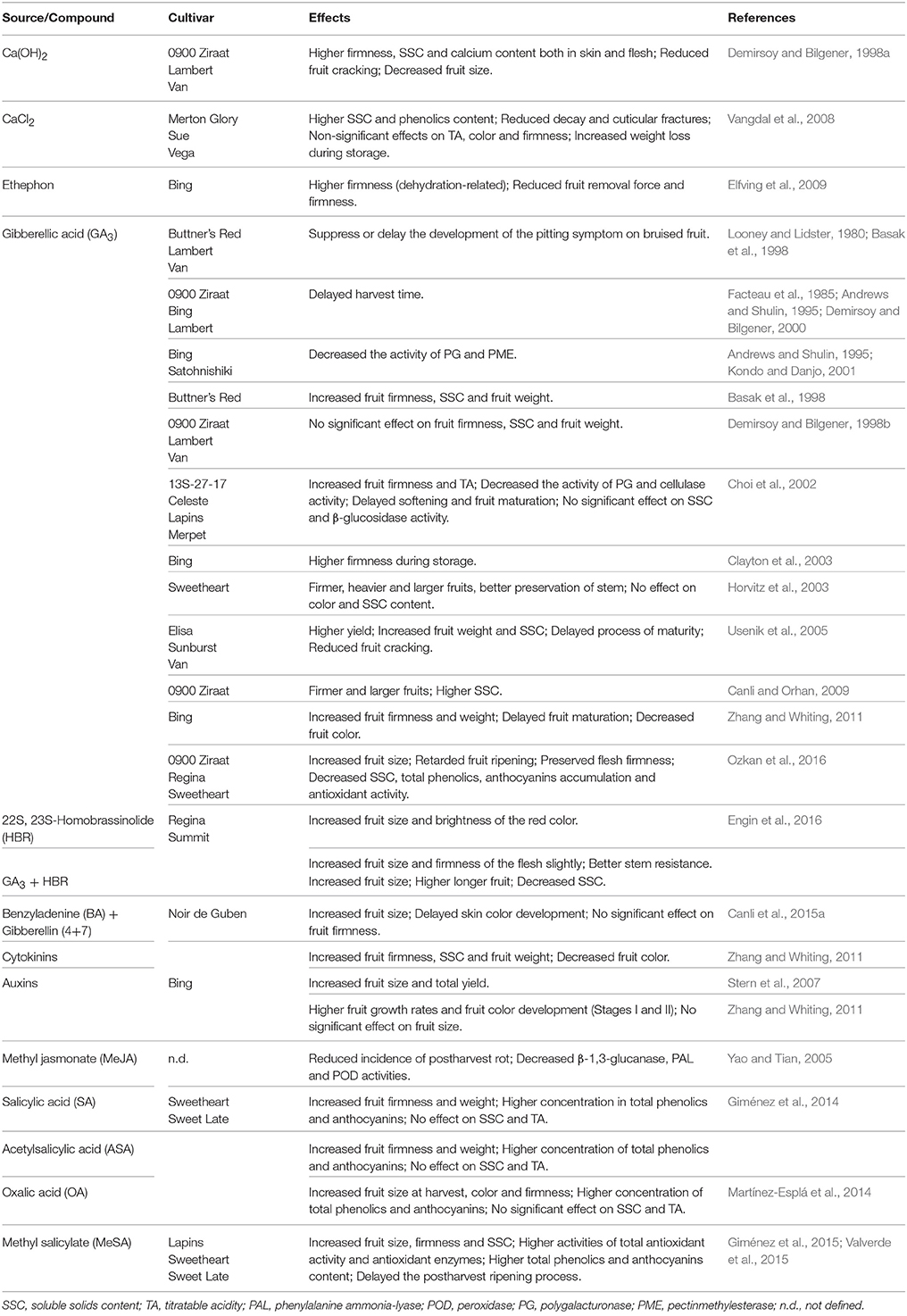
Table 2. Effect of calcium and growth regulators on quality attributes of several sweet cherry cultivars.
How to Maintain Shelf-Life and Health Promoting Compounds in the Chain?
Storage Conditions
Optimization of storage and transport conditions are important tools to extend and preserve shelf life and thus allow long-distance transport to destination markets (Remón et al., 2003; Martínez-Romero et al., 2006). Temperature and relative humidity (RH) are critical factors that influence cherry quality during postharvest storage (Kader, 2001; Romano et al., 2006). According to Bernalte et al. (1999), the optimal temperature to store sweet cherries is 0.5°C at 90% RH. During cold storage, the level of phenolic compounds such as neochlorogenic acid, p-coumaroylquinic acid, chlorogenic acid, rutin, catechin and epicatechin, and the content of anthocyanins such as cyanidin-3-O-rutinoside and peonidin-3-O-rutinoside increased in several sweet cherry cultivars (Gonçalves et al., 2004a,b, 2007; Serrano et al., 2009). The phenolics content in “Canada Giant” and “Ferrovia” cherries increased during at storage at 20°C during 8 days (Goulas et al., 2015), probably due to the condensation of the phenolic compounds by water loss during ripening or by postharvest synthesis (Kalt et al., 1999). However, Esti et al. (2002) observed a decrease of 41–52% of total anthocyanins content after 15 days at 1°C and 95% RH in two sweet cherry cultivars, which may indicate no net anthocyanin biosynthesis.
Hydro-cooling reduced sweet cherry stem browning and surface shriveling, while decay, external color, and SSC were not affected in “Tragana Edessis” and “Mpakirtzeika” cherries after 1-week of cold storage (0°C, 95% RH) (Manganaris et al., 2007). Adding CaCl2 during hydro-cooling reduced the respiration rate, ascorbic acid degradation, and membrane lipid peroxidation. Moreover, total phenolic content, antioxidant capacity, and fruit firmness increased and TA slightly decreased in “Sweetheart” and “Lapins” cherries (Wang Y. et al., 2014). Hydro-cooling can effectively increase quality of cherries by the reduction in temperature of the cherries after harvest, the sooner the better. Hydro-cooling portable equipment that can be installed in the orchard might be a good option (Elansari, 2009).
In order to extend the self-life of sweet cherries, MAP, and controlled atmosphere (CA) storage have been successfully implemented. Optimum CO2 and O2 levels for long time storage have been established at 2–10% for O2 and 5–20% for CO2 (Meheriuk et al., 1997; Remón et al., 2000, 2003; Kupferman and Sanderson, 2001; Tian et al., 2001; Spotts et al., 2002). Lower O2 levels may result in a decrease of volatile aromatic compounds and development of off-flavors (Meheriuk et al., 1995; Remón et al., 2000; Kader, 2002), while CO2 levels higher than 30% have been related to a brown discoloration of the skin (Kader, 1997). High O2 levels (70% O2 + 0% CO2) can inhibited ethanol production, but also induced a rapid decrease in ascorbic acid content and often resulted in browning due to high-O2 injury (Tian et al., 2004). Super atmospheric O2 packaging (100 kPa O2), in polypropylene film during 10 days at 4°C and 70% RH delayed respiration rate of cherries, inhibited ethylene production and PPO and POD activities and maintained firmness, soluble proteins and SSC for the first 4 days of storage. However, ascorbic acid content was lower compared to control cherries (Wang S. et al., 2014). MAP combined with low temperature storage reduces the incidence of fruit decay while preserving SSC, TA, and stem color. Fruits also displayed higher antioxidant capacity, firmness and, a brighter colored skin (Alique et al., 2003; Remón et al., 2003; Padilla-Zakour et al., 2004; Lara et al., 2015). High CO2 levels and cold storage both favor net anthocyanin synthesis as anthocyanin biosynthesis is induced during the postharvest cold storage of several red fruits (Remón et al., 2000; Conte et al., 2009). This increase may be due to inhibition of PPO activity promoted by CO2-enriched atmospheres, since changes in fruit color occurred due to oxidation of phenols by PPO (Vamos-Vigyazó, 1981). MAP and CA stored sweet cherry fruits at 5% O2 combined with 10% CO2 at 1°C reduced PPO and POD activity, prevented flesh browning, decreased fruit decay and promoted firmness retention. Weight loss, pitting, stem visual appearance, and PG and PPO activity, of “0900 Ziraat” cherries were lower when applying modified atmosphere-modified humidity packaging (MA/MH) using 0.20 μm low-density polyethylene during 8 days at 0°C and a 2-day shelf-life (Özkaya et al., 2015). Certainly, MAP can be useful for sweet cherry shelf-life extension, but large scale implementation in practice is limited as it is expensive and somewhat risky due to possible gas mixture mismanagement and fungal growth.
New Frontiers for Sweet Cherry Quality Improvements
Natural Compounds
Natural compounds have been applied to improve the shelf life of cherries. Application of 1 mM SA or ASA or OA on cherries stored for 20 days at 2°C showed lower acidity, less color changes and firmness loss and maintained higher levels of health promoting compounds and antioxidant activity (Valero et al., 2011). Immersion in 30 mM β-aminobutyric acid (BABA) for 10 min reduced respiration rate, weight loss and increased TA levels, total phenolics, sugars and ascorbic acid of “Hongdeng” cherries during 5 days at 20°C. Moreover, BABA-treated cherries showed higher ascorbate peroxidase, catalase, glutathione reductase, and superoxide dismutase activities (Wang et al., 2016). The use of these compounds is considered safe and environmentally friendly, resulting in extending storability of sweet cherry with increased health-promoting properties. Currently, these postharvest treatments have only been applied at the research level, but the future is promising for application in practice.
Biodegradable Films for MAP and Edible Coatings
Preservation technologies have emerged using biodegradable films for MAP (Giacalone and Chiabrando, 2013; Koutsimanis et al., 2014a,b). Biodegradable films have a reduced environmental footprint (Almenar et al., 2012) and delay color changes, softening and loss of acidity (Giacalone and Chiabrando, 2013). Micro-perforated polylactic acid (PLA) film inhibited fungal growth, maintained brightness, skin color, firmness, SSC, and decreased fruit weight loss in sweet cherry when compared with macro-perforated bags. Cherries stored in PLA packages showed better appearance, texture, flavor, and overall acceptability (Koutsimanis et al., 2014a). This packaging technology may become practice (Koutsimanis et al., 2014b), but depends on the balance between (less) waste production and (higher) price for these type of films (Tharanathan, 2003; Vroman and Tighzert, 2009).
Edible coating is a promising technology in the preservation of fruits and vegetables quality (Dhall, 2013). Edible fruit coatings can also provide an alternative to modified atmosphere storage and act as carriers of health promoting compounds. Bioactive compounds, such as antioxidants, antimicrobials, probiotics, flavors, and nutraceutical substances, can be transported from the coating onto the fruit skin by diffusion, enhancing shelf life, and nutritional quality (Quirós-Sauceda et al., 2014). Edible coatings may reduce respiration and transpiration rates, reduce firmness loss, and limit decay (Velickova et al., 2013). Locust bean gum, shellac, polysorbate 80, glycerol, and beeswax coatings applied to “Burlat” cherries showed lower weight loss, less bruising injury, less surface pitting and lower flesh firmness loss resulting in a prolonged shelf-life (Rojas-Argudo et al., 2005). Chitosan is an antimicrobial compound that induces fungal defense-related enzymes and enhances the level of phenolic compounds (Liu et al., 2007). Chitosan-coated sweet cherries showed lower water loss, delayed color changes, higher total phenolics, flavonoid, and anthocyanin levels (Petriccione et al., 2014). Misir et al. (2014) reviewed the application of aloe vera gels as an edible coating for fresh fruits to enhance the postharvest life and quality attributes of, amongst others, sweet cherries. Aloe vera gel reduced weight loss and lowered the respiration rate, delayed color changes, softening, and TA losses in cherry fruit compared to control fruits (Martínez-Romero et al., 2006). According to the European Directive (ED European and Parliament Council Directive N 95/2/EC, 1995; ED European Parliament and Council Directive N 98/72/EC, 1998) and USA regulations (FDA, 2016), edible coatings can be classified as food products, food ingredients or food additives. This allows for application of edible coatings in practice, although more studies are needed in order to improve functionality and consumer acceptance.
Marker Assisted Breeding
The recent publication of the huge and complex sweet cherry genome (Shirasawa et al., 2017) will allow for identification of candidate genes, signal transduction-, and metabolic pathways, which can have a relevant role in fruit quality (Carrasco et al., 2013). Sweet cherry linkage maps have been constructed to facilitate identification and characterization of quantitative trait loci (QTLs) associated with quality traits (Olmstead et al., 2008; Guajardo et al., 2015). QTLs have been identified for fruit weight and firmness (Campoy et al., 2015), fruit size (Zhang et al., 2010; Franceschi et al., 2013; Rosyara et al., 2013), skin and flesh fruit color (Sooriyapathirana et al., 2010), and fruit cracking tolerance (Balbontín et al., 2013). About 133 genes are putatively involved in fruit texture, color, flavor, and chilling injury resistance (Ogundiwin et al., 2009). Moreover, in sweet cherry, exocarp-specific transcripts associated with epidermis development (from flowering to maturity) and stress responses (Alkio et al., 2014) were established. In stone fruit breeding programs, marker-assisted selection (MAS) is an important biotechnological tool, although its use is currently limited due to the polygenic nature of traits linked to fruit quality (Salazar et al., 2014). Genotyping by sequencing (GBS) generates more saturated genetic maps, thus facilitating the identification of (more) accurate QTLs and the discovery of single nucleotide polymorphisms (SNPs) (Guajardo et al., 2015; Salazar et al., 2017), with focus mainly on finding sweet cherry quality QTLs and not on finding QTLs that affect the level of health promoting compounds. The use of DNA information, as clustered regularly interspaced short palindromic repeats (CRISPR) along with CRISPR-associated protein 9 (Cas9) system (CRISPR/Cas9), appears to be relevant in understanding genetic variation, inheritance, genomic organization, and phenotypic performance, contributing to the development of new cultivars of Rosaceous crop breeding (Peace, 2017). Although, the CRISPR/Cas9 gene-editing technique has not yet been used in cherry, it has been effectively used to confer resistance against citrus canker (Peng et al., 2017), regulating ethylene synthesis (Ripening inhibitor gene) in tomato (Ito et al., 2015) and in the efficient knockout of the L-idonate dehydrogenase gene (IdnDH), involved in the tartaric acid pathway in grape (Ren et al., 2016).
Taking this into account, it is expected that CRISPR/CAS gene editing will be instrumental with regard to improving fruit quality and the level of health-related compounds.
Concluding Remarks and Future Perspectives
We are at the beginning of acquiring knowledge to understand the variation in health-related compounds throughout the fruit maturation and the postharvest phase. How environmental factors, dose and time of preharvest compounds application affects tree performance and consequently fruit quality is at the moment largely unknown. Further research should be aimed to develop cultivar-specific strategies that improves both fruit quality and fruit nutritional characteristics, without significantly affecting yield. Optimizing cherry quality attributes needs a better understanding of the interaction between genetics, environmental factors, and plant growth regulators to be able to gain full benefit of new preservation technologies. The incorporation of bioactive compounds may provide advantages in food preservation and contributes to the development of functional foods. Application of bioactive compounds and encapsulation with edible coatings are promising techniques that also need further investigation, also with regard to diminishing off flavors. New cultivars with improved characteristics such as fruit size and color, anthocyanin biosynthesis, and cracking resistance are needed in order to produce cultivars with exceptional quality, high consumer appeal, well adapted to local growing and future climatic conditions. With the recent advancements in marked assisted breeding, especially now that the draft sweet cherry genome has been published, exciting times await.
Author Contributions
SC did the literature research, drafted the manuscript and made tables. RS, AS, and BG helped writing the final manuscript and all authors approved the final manuscript.
Funding
This work is supported by: European Investment Funds by FEDER/COMPETE/POCI—Operational Competitiveness and Internationalization Programme, under the Project POCI-01-0145-FEDER-006958 and National Funds by FCT—Portuguese Foundation for Science and Technology, under the project UID/AGR/04033/2013; INTERACT project -“Integrative Research in Environment, Agro-Chains and Technology,” no. NORTE-01-0145-FEDER-000017, in its line of research entitled ISAC-P2, co-financed by the European Regional Development Fund (ERDF) through NORTE 2020 (North Regional Operational Program 2014/2020) and “CherryCrackLess”—Cherry cracking & mitigation strategies: toward their understanding using a functional metabolomic approach (PTDC/AGR-PRO/7028/2014). SC acknowledge the financial support provided by the FCT-Portuguese Foundation for Science and Technology (SFRH/BD/52541/2014), under the Doctoral Programme “Agricultural Production Chains—from fork to farm” (PD/00122/2012).
Conflict of Interest Statement
The authors declare that the research was conducted in the absence of any commercial or financial relationships that could be construed as a potential conflict of interest.
The reviewer DO and handling Editor declared their shared affiliation.
References
Adisakwattana, S., Yibchok-Anun, S., Charoenlertkul, P., and Wongsasiripat, N. (2011). Cyanidin-3-rutinoside alleviates postprandial hyperglycemia and its synergism with acarbose by inhibition of intestinal α-glucosidase. J. Clin. Biochem. Nutr. 49, 36–41. doi: 10.3164/jcbn.10-116
Alique, R., Martinez, M. A., and Alonso, J. (2003). Influence of the modified atmosphere packaging on shelf life and quality of “Navalinda” sweet cherry. Eur. Food Res. Technol. 217, 416–420. doi: 10.1007/s00217-003-0789-x
Alique, R., Zamorano, J. P., Martínez, M. A., and Alonso, J. (2005). Effect of heat and cold treatments on respiratory metabolism and shelf-life of sweet cherry, type picota cv. Ambrunés Postharvest Biol. Technol. 35, 153–165. doi: 10.1016/j.postharvbio.2004.07.003
Alkio, M., Jonas, U., Sprink, T., Nocker, S., and Knoche, M. (2012). Identification of putative candidate genes involved in cuticle formation in Prunus avium (sweet cherry) fruit. Ann. Bot. 110, 101–112. doi: 10.1093/aob/mcs087
Alkio, M., Jonas, U., Declercq, M., Van Nocker, S., and Knoche, M. (2014). Transcriptional dynamics of the developing sweet cherry (Prunus avium L.) fruit: sequencing, annotation and expression profiling of exocarp-associated genes. Hort. Res. 1:11. doi: 10.1038/hortres.2014.11
Almenar, E., Siddiq, M., and Merkel, C. (2012). “Packaging for processed food and the environment,” in Handbook of Food Process Design, ed M.S. Rahman and J. Ahmed (Oxford: Blackwell), 1369–1405.
Andrews, P. K., and Shulin, L. (1995). Cell wall hydrolytic enzyme activity during development of nonclimacteric sweet cherry (Prunus avium L.) fruit. J. Hortic. Sci. 70, 561–567. doi: 10.1080/14620316.1995.11515327
Arakawa, O., Pungpomin, P., An, S., and Tanaka, N. (2017). “Effect of blue light on red color development and anthocyanin accumulation of sweet cherry,” in 8th International Cherry Symposium in Yamagata (Yamagata), 136.
Arakawa, O. (1993). Effect of ultraviolet light on anthocyanin synthesis in light colored sweet cherry, cv. Sato Nishiki. J. Jpn. Soc. Hortic. Sci. 62, 543–546. doi: 10.2503/jjshs.62.543
Balbontín, C., Ayala, H., Bastías, R. M., Tapia, G., Ellena, M., Torres, C., et al. (2013). Cracking in sweet cherries: a comprehensive review from a physiological, molecular, and genomic perspective. Chil. J. Agric. Res. 73, 66–72. doi: 10.4067/S0718-58392013000100010
Ballistreri, G., Continella, A., Gentile, A., Amenta, M., Fabroni, S., and Rapisarda, P. (2013). Fruit quality and bioactive compounds relevant to human health of sweet cherry (Prunus avium L.) cultivars grown in Italy. Food Chem. 140, 630–638. doi: 10.1016/j.foodchem.2012.11.024
Basak, A., Rozpara, E., and Grzyb, Z. (1998). Use of bioregulators to reduce sweet cherry tree growth and to improve fruit quality. Acta Hortic. 468, 719–723. doi: 10.17660/ActaHortic.1998.468.91
Belge, B., Llovera, M., Comabella, E., Gatius, F., Guillén, P., Graell, J., et al. (2014). Characterization of cuticle composition after cold storage of “Celeste” and “Somerset” sweet cherry fruit. J. Agric. Food Chem. 62, 8722–8729. doi: 10.1021/jf502650t
Bernalte, M. J., Hernandez, M. T., Vidal-Aragon, M. C., and Sabio, E. (1999). Physical, chemical, flavor and sensory characteristics of two sweet cherry varieties grown in Valle del Jerte (Spain). J. Food Qual. 22, 403–416. doi: 10.1111/j.1745-4557.1999.tb00173.x
Bernalte, M. J., Sabio, E., Hernández, M. T., and Gervasini, C. (2003). Influence of storage delay on quality of “Van” sweet cherry. Postharvest Biol. Technol. 28, 303–312.doi: 10.1016/S0925-5214(02)00194-1
Campoy, J. A., Dantec, L. L., Barreneche, T., Dirlewanger, E., and Quero-García, J. (2015). New insights into fruit firmness and weight control in sweet cherry. Plant Mol. Biol. Rep. 33, 783–796. doi: 10.1007/s11105-014-0773-6
Canli, F. A., and Orhan, H. (2009). Effects of preharvest gibberellic acid applications on fruit quality of “0900 Ziraat” sweet cherry. HortTecnology 19, 127–129.
Canli, F. A., Pektas, M., and Ercisli, S. (2015a). Benzyladenine and gibberellin applications improve fruit weight and delay maturity of sweet cherry. Erwerbs Obstbau 57, 71–75. doi: 10.1007/s10341-015-0231-0
Canli, F. A., Sahin, M., Ercisli, S., Yilmaz, O., Temurtas, N., and Pektas, M. (2015b). Harvest and postharvest quality of sweet cherry are improved by pre-harvest benzyladenine and benzyladenine plus gibberellin applications. J. Appl. Bot. Food 88, 255–258. doi: 10.5073/JABFQ.2015.088.037
Carrasco, B., Meisel, L., Gebauer, M., Garcia-Gonzales, R., and Silva, H. (2013). Breeding in peach, cherry and plum: from a tissue culture, genetic, transcriptomic and genomic perspective. Biol. Res. 46, 219–230. doi: 10.4067/S0716-97602013000300001
Chauvin, M. A., Whiting, M., and Ross, C. F. (2009). The influence of harvest time on sensory properties and consumer acceptance of sweet cherries. HortTechnology 19, 748–754.
Choi, C., Wiersma, P. A., Toivonen, P., and Kappel, F. (2002). Fruit growth, firmness and cell wall hydrolytic enzyme activity during development of sweet cherry fruit treated with gibberellic acid (GA3). J. Hortic. Sci. Biotechnol. 77, 615–621. doi: 10.1080/14620316.2002.11511547
Clayton, M., Biasi, W. V., Agar, I. T., Southwick, S. M., and Mitcham, E. J. (2003). Postharvest quality of “Bing” cherries following preharvest treatment with hydrogen cyanamide, calcium ammonium nitrate, or gibberellic acid. HortScience 38, 407–411.
Conte, A., Scrocco, C., Lecce, L., Mastromatteo, M., and Del Nobile, M. A. (2009). Ready-to-eat cherries: study on different packaging systems. Innov. Food Sci. Emerg. 10, 564–571. doi: 10.1016/j.ifset.2008.12.005
Corbo, M. R., Bevilacqua, A., Petruzzi, L., Casanova, F. P., and Sinigaglia, M. (2014). Functional beverages: the emerging side of functional foods commercial trends, research, and health implications. Compr. Rev. Food Sci. Food Saf. 13, 1192–1206. doi: 10.1111/1541-4337.12109
Crisosto, C. H., Crisosto, G. M., and Ritenour, M. A. (2002). Testing the reliability of skin colour as an indicator of quality for early season Brooks (Prunus avium L.) cherry. Postharvest Biol. Technol. 24, 147–154. doi: 10.1016/S0925-5214(01)00190-9
Crisosto, C. H., Crisosto, G. M., and Metheney, P. (2003). Consumer acceptance of “Brooks” and “Bing” cherries is mainly dependent on fruit SSC and visual skin color. Postharvest Biol. Technol. 28, 159–167.doi: 10.1016/S0925-5214(02)00173-4
Crisosto, C. H., Crisosto, G., and Neri, F. (2006). Understanding tree fruit quality based on consumer acceptance. Acta Hortic. 712, 183–190. doi: 10.17660/ActaHortic.2006.712.18
Crupi, P., Pichierri, A., Basile, T., and Antonacci, D. (2013). Postharvest stilbenes and flavonoids enrichment of table grape cv Redglobe (Vitis vinifera L.) as affected by interactive UV-C exposure and storage conditions. Food Chem. 141, 802–808. doi: 10.1016/j.foodchem.2013.03.055
Demir, T. (2013). Determination of carotenoid, organic acid and sugar content in some sweet cherry cultivars grown in Sakarya, Turkey. J. Food Agric. Environ. 11, 73–75.
Demirsoy, L., and Bilgener, S. (1998a). The effects of preharvest calcium hydroxide applications on cracking in “0900 Ziraat,” “Lambert” and “Van” sweet cherries. Acta Hortic. 468, 657–662.
Demirsoy, L., and Bilgener, S. (1998b). The effect of preharvest chemical applications on cracking and fruit quality in 0900 Ziraat, Lambert and Van sweet cherries varieties. Acta Hortic. 468, 663–670. doi: 10.17660/ActaHortic.1998.468.83
Demirsoy, L., and Bilgener, S. (2000). The effect of chemical applications on cuticular and epidermal properties of some sweet cherry cultivars with respect to fruit cracking susceptibility. Turk. J. Agric. For. 24, 541–550.
Demirtas, C., Erturk, U., Yazgan, S., Candogan, B. N., and Soylu, A. (2008). Effects of different irrigation levels on the vegetative growth, flower bud formation and fruit quality of sweet cherry in western part of Turkey. J. Food Agric. Environ. 6, 168–172.
Dever, M. C., MacDonald, R. A., Cliff, M. A., and Lane, W. D. (1996). Sensory evaluation of sweet cherry cultivars. Hortscience 31, 150–153.
Dhall, R. K. (2013). Advances in edible coatings for fresh fruits and vegetables: a Review. Crit. Rev. Food Sci. Nutr. 53, 435–450. doi: 10.1080/10408398.2010.541568
Díaz-Mula, H. M., Castillo, S., Martinez-Romero, D., Valero, D., Zapata, P. J., Guillen, F., et al. (2009). Sensory, nutritive and functional properties of sweet cherry as affected by cultivar and ripening stage. Food Sci. Technol. Int. 15, 535–543. doi: 10.1177/1082013209351868
Drake, S. R., and Fellman, J. K. (1987). Indicators of maturity and storage quality of Rainier sweet cherry. Hortscience 22, 283–285.
Eccher, T., and Granelli, G. (2006). Fruit quality and yield of different apple cultivars as affected by tree density. Acta Hortic. 712, 535–540. doi: 10.17660/ActaHortic.2006.712.66
Elansari, A. M. (2009). Design of portable forced-air precooling cooling system. J. Saudi Soc. Agric. Sci. 8, 1–15.
Elfving, D. C., Auvil, T. D., Castillo, F., Drake, S. R., Kunzel, H., Kupferman, E. M., et al. (2009). Effects of preharvest applications of ethephon and 1-MCP to “Bing” sweet cherry on fruit removal force and fruit quality. J. Am. Pomol. Soc. 63, 84–100.
Engin, H., Gokbayrak, Z., and Sakaldas, M. (2016). Effects of 22S, 23S-homobrassinolide and gibberellic acid on occurrence of physiological disorders and fruit quality of ‘Summit’ and ‘Regina’ sweet cherries. Erwerbs Obstbau 58, 203–210. doi: 10.1007/s10341-016-0273-y
Esti, M., Cinquanta, L., Sinesio, F., Moneta, E., and Di Matteo, M. (2002). Physiochemical and sensory fruit characteristics of two sweet cherry cultivars after cool storage. Food Chem. 76, 399–405. doi: 10.1016/S0308-8146(01)00231-X
ED European Parliament and Council Directive, N 95/2/EC (1995). On Food Additive Other than Colours and Sweeteners. Available online at: https://www.fsai.ie/uploadedFiles/95_2_EC.pdf
ED European Parliament and Council Directive N 98/72/EC (1998). On Food Additive Other than Colours and Sweeteners. Available online at: http://eur-lex.europa.eu/legal-content/EN/TXT/PDF/?uri=CELEX:31998L0072&from=EN
Facteau, T. J., Rowe, K. E., and Chesnut, N. E. (1985). Response patterns of gibberellic acid-treated sweet cherry fruit at different soluble solids levels and leaf/fruit ratios. Sci. Hortic. 27, 257–262. doi: 10.1016/0304-4238(85)90030-5
Facteau, T. J., Chestnut, N. E., and Rowe, K. (1996). Tree, fruit size, and yield of Bing sweet cherry as influenced by rootstock, replant area, and training system. Sci. Hortic. 67, 13–26. doi: 10.1016/S0304-4238(96)00943-0
FAO (2017). Cherry Production. United Nations: Statistical Database-Agriculture, Food and Agricultural Organisation (FAO).
Food Drug Administration (FDA). (2016). CFR Title 21: Foods and Drugs, CFR Part 172: Food Additives Permitted for Direct Addition to Food for Human Consumption CFR, Subpart, C. Coatings, Films and Related Substances. Silver Spring, MD. Available online at: http://www.accessdata.fda.gov/scripts/cdrh/cfdocs/cfCFR/CFRSearch.cfm
Ferretti, G., Bacchetti, T., Belleggia, A., and Neri, D. (2010). Cherry antioxidants: from farm to Ttble. Molecules 15, 6993–7005. doi: 10.3390/molecules15106993
Flore, J. A., and Layne, D. R. (1999). Photoassimilate production and distribution in cherry. Hortscience 34, 1015–1019.
Franceschi, P. D., Stegmeir, T., Cabrera, A., Van Der Knaap, E., Rosyara, U. R., Sebolt, A. M., et al. (2013). Cell number regulator genes in Prunus provide candidate genes for the control of fruit size in sweet and sour cherry. Mol. Breed. 32, 311–326. doi: 10.1007/s11032-013-9872-6
Gao, L., and Mazza, G. (1995). Characterization, quantification and distribution of anthocyanins and colorless phenolics in sweet cherry. J. Agric. Food Chem. 43, 343–346. doi: 10.1021/jf00050a015
Giacalone, G., and Chiabrando, V. (2013). Modified atmosphere packaging of sweet cherries with biodegradable films. Int. Food Res. J. 20, 1263–1268.
Giménez, M. J., Valverde, J. M., Valero, D., Guillén, F., Martínez-Romero, D., Serrano, M., et al. (2014). Quality and antioxidant properties on sweet cherries as affected by preharvest salicylic and acetylsalicylic acids treatments. Food Chem. 160, 226–232. doi: 10.1016/j.foodchem.2014.03.107
Giménez, M. J., Valverde, J. M., Valero, D., Díaz-Mula, H. M., Zapata, P. J., Serrano, S., et al. (2015). Methyl salicylate treatments of sweet cherry trees improve fruit quality at harvest and during storage. Sci. Hortic. 197, 665–673. doi: 10.1016/j.scienta.2015.10.033
Girard, B., and Koop, T. G. (1998). Physicochemical characteristics of selected sweet cherry cultivars. J. Agric. Food Chem. 46, 471–476. doi: 10.1021/jf970646j
Gonçalves, B., Landbo, A. K., Knudsen, D., Silva, A. P., Moutinho-Pereira, J., and Rosa, E. (2004a). Effect of ripeness and postharvest storage on the phenolic profiles of cherries (Prunus avium L.). J. Agric. Food Chem. 52, 523–530. doi: 10.1021/jf030595s
Gonçalves, B., Landbo, A. K., Let, M., Silva, A. P., Rosa, E., and Meyer, A. S. (2004b). Storage affects the phenolic profiles and antioxidant activities of cherries (Prunus avium L.) on human low-density lipoproteins. J. Sci. Food Agric. 84, 1013–1020. doi: 10.1002/jsfa.1752
Gonçalves, B., Moutinho-Pereira, J., Santos, A., Silva, A. P., Bacelar, E., Correia, C., et al. (2006). Scion-rootstock interaction affects the physiology and fruit quality of sweet cherry. Tree Physiol. 26, 93–104. doi: 10.1093/treephys/26.1.93
Gonçalves, B., Silva, A. P., Moutinho-Pereira, J., Bacelar, E., Rosa, E., and Meyer, A. S. (2007). Effect of ripeness and postharvest storage on the evolution of colour and anthocyanins in cherries (Prunus avium L.). Food Chem. 103, 976–984. doi: 10.1016/j.foodchem.2006.08.039
González-Gómez, D., Lozano, M., Fernández-Leóna, M. F., Bernalte, M. J., Ayuso, M. C., and Rodríguez, A. B. (2009). Sweet cherry phytochemicals: identification and characterization by HPLC-DAD/ESI-MS in six sweet-cherry cultivars grown in Valle del Jerte (Spain). J. Food Compost. Anal. 23, 533–539. doi: 10.1016/j.jfca.2009.02.008
Goulas, V., Minas, I. S., Kourdoulas, P. M., Lazaridou, A., Molassiotis, A. N., Gerothanassis, I. P., et al. (2015). 1H NMR metabolic fingerprinting to probe temporal postharvest changes on qualitative attributes and phytochemical profile of sweet cherry fruit. Front. Plant Sci. 6:959. doi: 10.3389/fpls.2015.00959
Guajardo, V., Solís, S., Sagredo, B., Gainza, F., Muñoz, C., Gasic, K., et al. (2015). Construction of high density sweet cherry (Prunus avium L.) linkage maps using microsatellite markers and SNPs detected by genotyping-by-sequencing (GBS). PLoS ONE 10:e0127750. doi: 10.1371/journal.pone.0127750
Guyer, D. E., Sinha, N. K., Tung-Sung, C., and Cash, J. N. (1993). Physicochemical and sensory characteristics of selected Michigan sweet cherry (Prunus avium L.) cultivars. J. Food Qual. 16, 355–370. doi: 10.1111/j.1745-4557.1993.tb00121.x
Hadi, M. A. M., Zhang, F., Wu, F., Zhou, C., and Tao, J. (2013). Advances in fruit aroma volatile research. Molecules 18, 8200–8229. doi: 10.3390/molecules18078200
Hampson, C. R., Stanich, K., MacKenzie, D. L., Herbert, L., Lu, R., Li, J., et al. (2014). Determining the optimum firmness for sweet cherries using Just-About-Right sensory methodology. Postharvest Biol. Technol. 91, 104–111. doi: 10.1016/j.postharvbio.2013.12.022
Horvitz, S., Godoy, C., López Camelo, A. F., Yommi, A., and Godoy, C. (2003). Application of gibberelic acid to “Sweetheart” sweet cherries: effects on fruit quality at harvest and during cold storage. Acta Hortic. 628, 311–316. doi: 10.17660/ActaHortic.2003.628.37
Ito, Y., Nishizawa-Yokoi, A., Endo, M., Mikami, M., and Toki, S. (2015). CRISPR/Cas9-mediated mutagenesis of the RIN locus that regulates tomato fruit ripening. Biochem. Biophys. Res. Commun. 467, 76–82. doi: 10.1016/j.bbrc.2015.09.117
Jacob, R. A., Spinozzi, G. M., Simon, V. A., Kelley, D. S., Prior, R. L., and Hess-Pierce, B. (2003). Consumption of cherries lowers plasma urate in healthy women. J. Nutr. 133, 1826–1829.
Jakobek, L., Marijan, S., Vóca, S., Šindrak, Z., and Dobričević, N. (2009). Flavonol and phenolic acid composition of sweet cherries (cv. Lapins) produced on six different vegetative rootstocks. Sci. Hortic. 123, 23–28. doi: 10.1016/j.scienta.2009.07.012
Kader, A. (1997). “A summary of CA requirements and recommendations for fruits,” in Proceedings of 7th International Controlled Atmosphere Research Conference, ed A. A. Kader (Davis, CA), 3–10.
Kader, A. (2001). Recent advances and future research needs in postharvest technology of fruits. Bull. Int. Inst. Refrigeration. LXXXI, 3–14.
Kader, A. (2002). Postharvest Technology of Horticultural Crops, 3rd Edn. University of California, Agriculture and Natural Resources, Publication. 3311, 535.
Kalt, W., Forney, C. F., Martin, A., and Prior, R. L. (1999). Antioxidant capacity, vitamin C, phenolics and anthocyanins after fresh storage of small fruits. J. Agric. Food Chem. 47, 4638–4644. doi: 10.1021/jf990266t
Kang, S. Y., Seeram, N. P., Nair, M. G., and Bourquin, L. D. (2003). Tart cherry anthocyanins inhibit tumor development in ApcMin mice and reduce proliferation of human colon cancer cells. Cancer Lett. 194, 13–19. doi: 10.1016/S0304-3835(02)00583-9
Kappel, F., Fisher-Fleming, B., and Hogue, E. (1996). Fruit characteristics and sensory attributes of an ideal sweet cherry. Hortscience 31, 443–446.
Kappel, F., Toivonen, P., McKenzie, D. L., and Stan, S. (2002). Storage characteristics of new sweet cherry cultivars. Hortic. Sci. 37, 139–143.
Kataoka, I., Beppu, K., Sugiyama, A., and Taira, T. (1996). Enhancement of coloration of “Satohnishiki” sweet cherry fruit by postharvest irradiation with ultraviolet radiation. Environ. Control Biol. 34, 313–319.
Kelebek, H., and Selli, S. (2011). Evaluation of chemical constituents and antioxidant activity of sweet cherry (Prunus avium L.) cultivars. Int. J. Food Sci. Technol. 46, 2530–2537. doi: 10.1111/j.1365-2621.2011.02777.x
Kim, D. O., Heo, H. J., Kim, Y. J., Yang, H. S., and Lee, C. Y. (2005). Sweet and sour cherry phenolics and their protective effects on neuronal cells. J. Agric. Food Chem. 53, 9921–9927. doi: 10.1021/jf0518599
Koc, A., Celik, Z., Akbulut, M., Bilgener, S., Ercisli, S., Gunes, M., et al. (2013). Morphological characterization of cherry rootstock candidates selected from central and east black sea regions in Turkey. Sci. World J. 2013:916520. doi: 10.1155/2013/916520
Kondo, S., and Danjo, C. (2001). Cell wall polysaccharide metabolism during fruit development in sweet cherry “Satohnishiki” as affected by gibberellic acid. J. Jpn. Soc. Hortic. Sci. 70, 178–184. doi: 10.2503/jjshs.70.178
Koutsimanis, G., Harte, J., and Almenar, E. (2014a). Freshness maintenance of cherries ready for consumption using convenient, microperforated, bio-based packaging. J. Sci. Food Agric. 95, 972–982. doi: 10.1002/jsfa.6771
Koutsimanis, G., Harte, J., and Almenar, E. (2014b). Development and evaluation of a new packaging system for fresh produce: a case study on fresh cherries under global supply chain conditions. Food Bioprocess Technol. 68, 55–669. doi: 10.1007/s11947-014-1437-0
Kupferman, E., and Sanderson, P. (2001). Temperature Management and Modified Atmosphere Packing to Preserve Sweet Cherry Quality. Tree Fruit Research and Extension Center, Washington State University, 1–9.
Lang, G. A., Wilkinson, T., and Larson, J. (2017). “Insights for orchard design and management using intensive sweet cherry canopy architectures on dwarfing to semi-vigorous rootstocks,” in 8th International Cherry Symposium in Yamagata (Yamagata), 57.
Lang, G. A. (2017). “The cherry industries in the USA: current trends and future perspectives,” in 8th International Cherry Symposium in Yamagata (Yamagata), 24.
Lara, I., Belge, B., and Goulao, L. F. (2014). The fruit cuticle as a modulator of postharvest quality. Postharvest Biol. Technol. 87, 103–112. doi: 10.1016/j.postharvbio.2013.08.012
Lara, I., Camats, J. C., Comabella, E., and Ortiz, A. (2015). Eating quality and health-promoting properties of two sweet cherry (Prunus avium L.) cultivars stored in passive modified atmosphere. Food Sci. Technol. Int. 21, 133–144. doi: 10.1177/1082013213518544
Leong, S. Y., and Oey, I. (2012). Effects of processing on anthocyanins, carotenoids and vitamin C in summer fruits and vegetables. Food Chem. 133, 1577–1587. doi: 10.1016/j.foodchem.2012.02.052
Li, S. (2014). Transcriptional control of flavonoid biosynthesis: fine-tuning of the MYB-bHLH-WD40(MBW) complex. Plant Signal. Behav. 9:e27522. doi: 10.4161/psb.27522
Liu, J., Tian, S., Meng, X., and Xu, Y. (2007). Effects of chitosan on control of postharvest diseases and physiological responses of tomato fruit. Postharvest Biol. Technol. 44, 300–306. doi: 10.1016/j.postharvbio.2006.12.019
Liu, Y., Liu, X., Zhong, F., Tian, R., Zhang, K., Zhang, X., et al. (2011). Comparative study of phenolic compounds and antioxidant activity in different species of cherries. J. Food Sci. 76, 633–638. doi: 10.1111/j.1750-3841.2011.02150.x
Looney, N. E., and Lidster, P. D. (1980). Some growth regulator effects on fruit quality, mesocarp composition, and susceptibility to postharvest surface marking of sweet cherries. J. Am. Soc. Hortic. Sci. 105, 130–134.
Luo, H., Dai, S., Ren, J., Zhang, C., Ding, Z., Li, Z., et al. (2014). The role of ABA in the maturation and postharvest life of a nonclimacteric sweet cherry fruit. J. Plant Growth Regul. 33, 373–383. doi: 10.1007/s00344-013-9388-7
Macheix, J. J., Fleuriet, A., and Billot, J. (1990). Fruit Phenolics. Boca Raton, FL: CRC Press. 378.
Manganaris, G. A., Ilias, I. F., Vasilakasis, M., and Mignani, I. (2007). The effect of hydrocooling on ripening related quality attributes and cell wall physicochemical properties of sweet cherry fruit (Prunus avium L.). Int. J. Refrig. 30, 1386–1392. doi: 10.1016/j.ijrefrig.2007.04.001
Marsal, J., Lopez, G., del Campo, J., Mata, M., Arbones, A., and Girona, J. (2010). Postharvest regulated deficit irrigation in “Summit” sweet cherry: fruit yield and quality in the following season. Irrig. Sci. 28:181. doi: 10.1007/s00271-009-0174-z
Martínez-Esplá, A., Zapata, P. J., Valero, D., García-Viguera, C., Castillo, S., and Serrano, M. (2014). Preharvest application of oxalic acid increased fruit size, bioactive compounds, and antioxidant capacity in sweet cherry cultivars (Prunus avium L.). J. Agric. Food Chem. 62, 3432–3437. doi: 10.1021/jf500224g
Martínez-Romero, D., Alburquerque, N., Valverde, J. M., Guillén, F., Castillo, S., Valero, D., et al. (2006). Postharvest sweet cherry quality and safety maintenance by aloe vera treatment: a new edible coating. Postharvest Biol. Technol. 39, 93–100. doi: 10.1016/j.postharvbio.2005.09.006
Matias, A. A., Rosado-Ramos, R., Nunes, L. S., Figueira, I., Serra, A. T., Bronze, M. R., et al. (2016). Protective effect of a (poly)phenol-rich extract derived from sweet cherries culls against oxidative cell damage. Molecules 21, 1–16. doi: 10.3390/molecules21040406
Matteo, A. D., Russo, R., Graziani, G., Ritieni, A., and Vaio, C. D. (2016). Characterization of autochthonous sweet cherry cultivars (Prunus avium L.) of southern Italy for fruit quality, bioactive compounds and antioxidant activity. J. Sci. Food Agric. 97, 2782–2794. doi: 10.1002/jsfa.8106
Mattheis, J. P., Buchanan, D. A., and Fellman, J. K. (1992). Volatile compounds emitted by sweet cherries (Prunus avium cv. Bing) during fruit development and ripening. J. Agric. Food Chem. 40, 471–474. doi: 10.1021/jf00015a022
McCune, L. M., Kubota, C., Stendell-Hollis, N. R., and Thomson, C. A. (2010). Cherries and health: a review. Crit. Rev. Food Sci. Nutr. 51, 1–12. doi: 10.1080/10408390903001719
Meheriuk, M., Girard, B., Moyls, L., Beveridge, H. J. T. D., McKenzie, L., and Harrison, J. (1995). Modified atmosphere packaging of “Lapins” sweet cherry. Food Res. Int. 3, 239–244.
Meheriuk, M., McKenzie, B., Girard, B., Molys, A. L., Weintraub, S., and Hocking, R. (1997). Storage of “Sweetheart” cherries in sealed plastic film. J. Food Qual. 20, 189–198.
Milinović, B., Dragović-Uzelac, V., Kazija, D. H., Jelačić, T., Vujević, P., Čiček, D., et al. (2016). Influence of four different dwarfing rootstocks on phenolic acids and anthocyanin composition of sweet cherry (Prunus avium L.) cvs. ‘Kordia’ and ‘Regina’. J. Appl. Bot. Food Qual. 89, 29–37. doi: 10.5073/JABFQ.2016.089.004
Misir, J., Brishti, F. H., and Hoque, M. M. (2014). Aloe vera gel as a novel edible coating for fresh fruits: a review. Am. J. Food Sci. Technol. 2, 93–97. doi: 10.12691/ajfst-2-3-3
Mozetič, B., Trebše, P., and Hribar, J. (2002). Determination and quantification of anthocyanins and hydroxycinnamic acids in different cultivars of sweet cherries (Prunus avium L.) from Nova Gorica Region (Slovenia). Food Tech. Biotechnol. 40, 207–212.
Mozetič, B., Trebše, P., Simčič, M., and Hribar, J. (2004). Changes of anthocyanins and hydroxycinnamic acids affecting the skin colour during maturation of sweet cherries (Prunus avium L.). Swiss Soc. Food Sci. Technol. 37, 123–128. doi: 10.1016/S0023-6438(03)00143-9
Mozetič, B., Simčič, M., and Trebše, P. (2006). Anthocyanins and hydroxycinnamic acids of lambert compact cherries (Prunus avium L.) after cold storage and 1-methylcyclopropene treatment. Food Chem. 97, 302–309. doi: 10.1016/j.foodchem.2005.04.018
Neilsen, G. H., Neilsen, D., Kappel, F., and Forge, T. (2014). Interaction of irrigation and soil management on sweet cherry productivity and fruit quality at different crop loads that simulate those occurring by environmental extremes. HortScience 49, 215–220.
Nora, L., Dalmazo, G. O., Nora, F., and Rombaldi, C. (2012). “Controlled water stress to improve fruit and vegetable postharvest quality,” in Water Stress, ed Prof. Ismail Md. Mofizur Rahman (InTech.). Available online at: http://www.intechopen.com/books/water-stress
Ogundiwin, E. A., Peace, C. P., Gradziel, T. M., Parfitt, D. E., Bliss, F. A., and Crisosto, C. H. (2009). A fruit quality gene map of Prunus. BMC Genomics 10:587. doi: 10.1186/1471-2164-10-587
Olías, J. M., Sanz, C., Ríos, J. J., and Pérez, A. G. (1995). “Substrates specificity of alcohol acyltransferase from strawberry and banana fruits,” in Fruit Flavors: Biogenesis, Characterization and Authentication eds R. L. Rouseff and M. M. Leahy (Washington, DC: American Chemical Society), 134–141.
Olmstead, J., Iezzoni, A., and Whiting, M. D. (2007). Genotypic differences in sweet cherry fruit size result from differences in fruit cell number. J. Am. Soc. Hortic. Sci. 132, 697–703.
Olmstead, J., Sebolt, A., Cabrera, A., Sooriyapathirana, S., Hammar, S., Iriarte, G., et al. (2008). Construction of an intra-specific sweet cherry (Prunus avium L.) genetic linkage map and synteny analysis with the Prunus reference map. Tree Genet. Genomes 4, 897–910. doi: 10.1007/s11295-008-0161-1
Ozkan, Y., Ucar, M., Yildiz, K., and Ozturkc, B. (2016). Pre-harvest gibberellic acid (GA3) treatments play an important role on bioactive compounds and fruit quality of sweet cherry cultivars. Sci. Hortic. 211, 358–362. doi: 10.1016/j.scienta.2016.09.019
Özkaya, O., Sener, A., Sarida,ş, M. A., Ünal, Ü., Valizadeh, A., and Dündar, Ö. (2015). Influence of fast cold chain and modified atmosphere packaging storage on postharvest quality of early season-harvest sweet cherries. J. Food Process. Preserv. 39, 2119–2128. doi: 10.1111/jfpp.12455
Padilla-Zakour, O. I., Tandon, K. S., and Wargo, J. M. (2004). Quality of modified atmosphere packaged “Hedelfingen” and “Lapins” sweet cherries (Prunus avium L.). HortTechnol. 14, 331–337.
Peace, C. P. (2017). DNA-informed breeding of rosaceous crops: promises, progress and prospects. Hort. Res. 4:17006. doi: 10.1038/hortres.2017.6
Peng, A., Chen, S., Lei, T., Xu, L., He, Y., Wu, L., et al. (2017). Engineering canker resistant plants through CRISPR/Cas9-targeted editing of the susceptibility gene CsLOB1 promoter in citrus. Plant Biotechnol. J. 15, 1509–1519. doi: 10.1111/pbi.12733
Pérez-Sánchez, R., Gómez-Sánchez, M. A., and Morales-Corts, M. R. (2010). Description and quality evaluation of sweet cherries cultured in Spain. J. Food Qual. 33, 490–506. doi: 10.1111/j.1745-4557.2010.00339.x
Peschel, S., Franke, R., Schreiber, L., and Knoche, M. (2007). Composition of the cuticle of developing sweet cherry fruit. Phytochemistry 68, 1017–1025. doi: 10.1016/j.phytochem.2007.01.008
Petriccione, M., De Sanctis, F., Pasquariello, M. S., Mastrobuoni, F., Rega, P., Scortichini, M., et al. (2014). The effect of chitosan coating on the quality and nutraceutical traits of sweet cherry during postharvest life. Food Bioprocess. Technol. 8, 394–408. doi: 10.1007/s11947-014-1411-x
Pherobase (2007). Available from: http://www.pherobase.com/database/floral-compounds/floral-taxa-compounds-fg-Carboxylic%20Esters.php
Predieri, S., Dris, R., Sekse, L., and Rapparini, F. (2003). Influence of environmental factors and orchard management on yield and quality of sweet cherry. J. Food Agric. Environ. 1, 263–266.
Predieri, S., Dris, R., and Rapparini, F. (2004). Influence of growing conditions on yield and quality of cherry: II. fruit quality. J. Food Agric. Environ. 2, 307–309.
Prvulović, D., Popović, M., Malenčić, D., Ljubojević, M., Barać, G., and Ognjanov, V. (2012). Phenolic Content and Antioxidant Capacity of Sweet and Sour Cherries. Studia UBB Chemia, LVII 175–181.
Quirós-Sauceda, A. E., Ayala-Zavala, J. F., Olivas, G. I., and González-Aguilar, G. A. (2014). Edible coatings as encapsulating matrices for bioactive compounds: a review. J. Food Sci. Technol. 51, 1674–1685. doi: 10.1007/s13197-013-1246-x
Remón, S., Ferrer, A., Marquina, P., Burgos, J., and Oria, R. (2000). Use of modified atmospheres to prolong the postharvest life of “Burlat” cherries at two different degrees of ripeness. J. Sci. Food Agric. 80, 1545–1552. doi: 10.1002/1097-0010(200008)80:10<1545::AID-JSFA680>3.0.CO;2-X
Remón, S., Venturini, M. E., Lopez-Buesa, P., and Oria, R. (2003). Burlat cherry quality after long range transport: optimisation of packaging conditions. Innov. Food Sci. Emerg. 4, 425–434. doi: 10.1016/S1466-8564(03)00058-4
Ren, J., Chen, P., Dai, S. J., Li, P., Li, Q., Ji, K., et al. (2011). Role of abscisic acid and ethylene in sweet cherry fruit maturation: molecular aspects. N. Z. J. Crop Hortic. Sci. 3, 161–174. doi: 10.1080/01140671.2011.563424
Ren, C., Liu, X., Zhang, Z., Wang, Y., Duan, W., Li, S., et al. (2016). CRISPR/Cas9-mediated efficient targeted mutagenesis in Chardonnay (Vitis vinifera L.). Sci. Rep. 6:32289. doi: 10.1038/srep32289
Rios de Souza, V., Pimenta Pereira, P. A., da Silva, T. L. T., de Oliveira Lima, L. C., Pio, R., and Queiroz, F. (2014). Determination of bioactive compounds, antioxidant activity and chemical composition of Brazilian blackberry, red raspberry, strawberry, blueberry and sweet cherry fruits. Food Chem. 156, 362–368. doi: 10.1016/j.foodchem.2014.01.125
Ripoll, J., Urban, L., Brunel, B., and Bertin, N. (2016). Water deficit effects on tomato quality depend on fruit developmental stage and genotype. J. Plant Physiol. 190, 26–35. doi: 10.1016/j.jplph.2015.10.006
Rivera-Pastrana, D. M., Gardea, A. A., Yahia, E. M., Martìnez-Tèllez, M. A., and Gonzàles-Aguilar, G. A. (2013). Effect of UV-C irradiation and low temperature storage on bioactive compounds, antioxidant enzymes and radical scavenging of papaya fruit. J. Food Sci. Technol. 51, 3821–3829. doi: 10.1007/s13197-013-0942-x
Rojas-Argudo, C., Pérez-Gago, M. B., and del Río, M. A. (2005). Postharvest quality of coated cherries cv. Burlat as affected by coating composition and solids content. Food Sci. Technol. Int. 11, 417–424. doi: 10.1177/1082013205060180
Romanazzi, G., Nigro, F., and Ippolito, A. (2009). Effectiveness of a short hyperbaric treatment to control postharvest decay of sweet cherries and table grapes. Postharvest Biol. Technol. 49, 440–442. doi: 10.1016/j.postharvbio.2008.01.021
Romano, G. S., Cittadini, E. D., Pugh, B., and Schouten, R. (2006). Sweet cherry quality in the horticultural production chain. Stewart Postharvest Rev. 6, 1–9. doi: 10.2212/spr.2006.6.2
Rosyara, U. R., Bink, M. C. A. M., Weg, E., Zhang, G., Wang, D., Sebolt, A., et al. (2013). Fruit size QTL identification and the prediction of parental QTL genotypes and breeding values in multiple pedigreed populations of sweet cherry. Mol. Breed. 32, 875–887. doi: 10.1007/s11032-013-9916-y
Salazar, J. A., Ruiz, D., Campoy, J. A., Sánchez-Pérez, R., Crisosto, C. H., Martínez- García, P. J., et al. (2014). Quantitative trait loci (QTL) and mendelian trait loci (MTL) analysis in Prunus: a breeding perspective and beyond. Plant. Mol. Biol. Rep. 32, 1–18. doi: 10.1007/s11105-013-0643-7
Salazar, J. A., Pacheco, I., Shinya, P., Zapata, P., Silva, C., Aradhya, M., et al. (2017). Genotyping by sequencing for SNP-based linkage analysis and identification of QTLs linked to fruit quality traits in Japanese Plum (Prunus salicina Lindl.). Front. Plant Sci. 8:476. doi: 10.3389/fpls.2017.00476
Schmitt, E. R., Duhme, F., and Schmid, P. P. S. (1989). Water relations in sweet cherries (Prunus avium L.) on sour cherry rootstocks (Prunus cerasus L.) of different compatibility. Sci. Hortic. 39, 189–200. doi: 10.1016/0304-4238(89)90132-5
Seeram, N. P., Momin, R. A., Nair, M. G., and Bourquin, L. D. (2001). Cyclooxygenase inhibitory and antioxidant cyanidin glycosides in cherries and berries. Phytomedicine 8, 362–369. doi: 10.1078/0944-7113-00053
Serra, A. T., Duarte, R. O., Bonze, M. R., and Duarte, C. M. M. (2011). Identification of bioactive response in traditional cherries from Portugal. Food Chem. 125, 318–325. doi: 10.1016/j.foodchem.2010.07.088
Serradilla, M. J., Martín, A., Ruiz-Moyano, S., Hernández, A. M., López-Corrales, M., and Córdoba, M. G. (2012). Physicochemical and sensorial characterisation of four sweet cherry cultivars grown in Jerte Valley (Spain). Food Chem. 133, 1551–1559. doi: 10.1016/j.foodchem.2012.02.048
Serrano, M., Guillen, F., Martínez-Romero, D., Castillo, S., and Valero, D. (2005). Chemical constituents and antioxidant activity of sweet cherry at different ripening stages. J. Agric. Food Chem. 53, 2741–2745. doi: 10.1021/jf0479160
Serrano, M., Díaz-Mula, H. M., Zapata, P. J., Castillo, S., Guillén, F., and Martínez-Romero, D. (2009). Maturity stage at harvest determines the fruit quality and antioxidant potential after storage of sweet cherry cultivars. J. Agric. Food Chem. 57, 3240–3246. doi: 10.1021/jf803949k
Shackel, K. A., Ahmadi, H., Biasi, W., Buchner, R., Goldhamer, D., Gurusinghe, S., et al. (1997). Plant water status as an index of irrigation need in deciduous fruit trees. HortTechnology 7, 23–29.
Shirasawa, K., Isuzugawa, K., Ikenaga, M., Saito, Y., Yamamoto, T., Hirakawa, H., et al. (2017). The genome sequence of sweet cherry (Prunus avium) for use in genomics-assisted breeding. DNA Res. 24, 499–508. doi: 10.1093/dnares/dsx020
Sooriyapathirana, S. S., Khan, A., Sebolt, A. M., Wang, D., Bushakra, J. M., Allan, A. C., et al. (2010). QTL analysis and candidate gene mapping for skin and flesh color in sweet cherry fruit (Prunus avium L.). Tree Genet. Genomes 6, 821–832. doi: 10.1007/s11295-010-0294-x
Spotts, R. A., Cervantes, L. A., and Facteau, T. J. (2002). Integrated control of brown rot of sweet cherry fruit with a pre-harvest fungicide, a postharvest yeast, modified atmosphere packaging, and cold storage temperature. Postharvest Biol. Technol. 24, 251–257. doi: 10.1016/S0925-5214(01)00155-7
Stern, R. A., Flaishman, M., Applebaum, S., and Ben-Arie, R. (2007). Effect of synthetic auxins on fruit development of “Bing” cherry (Prunus avium L.). Sci. Hortic. 114, 275–280.doi: 10.1016/j.scienta.2007.07.010
Subedi, P., Walsh, K., and Purdy, P. (2013). Determination of optimum maturity stages of mangoes using fruit spectral signatures. Acta Hortic. 992, 521–527. doi: 10.17660/ActaHortic.2013.992.64
Sun-Waterhouse, D. (2011). The development of fruit-based functional foods targeting the health and wellness market: a review. Int. J. Food Sci. Technol. 46, 899–920. doi: 10.1111/j.1365-2621.2010.02499.x
Szot, I., and Meland, M. (2001). Influence of rootstocks on size distribution and fruit quality of sweet cherry cultivars. Int. Agrophys. 15, 207–214.
Tharanathan, R. N. (2003). Biodegradable films and composite coatings: past, present and future. Trends Food Sci. Technol. 14, 71–78. doi: 10.1016/S0924-2244(02)00280-7
Tian, S. P., Fan, Q., Xu, Y., Wang, Y. S., and Jiang, A. (2001). Evaluation of the use of high carbon dioxide concentrations and cold storage to control Monilinia fructicola on sweet cherries. Postharvest Biol. Technol. 22, 53–60. doi: 10.1016/S0925-5214(00)00177-0
Tian, S. P., Jiang, A. L., Xu, Y., and Wang, Y. S. (2004). Responses of physiology and quality of sweet cherry fruit to different atmosphere in storage. Food Chem. 87, 43–49. doi: 10.1016/j.foodchem.2003.10.014
Tomás-Barberán, F. A., and Espín, J. C. (2001). Phenolic compounds and related enzymes as determinants of quality in fruits and vegetables. J. Sci. Food Agric. 81, 853–876. doi: 10.1002/jsfa.885
USDA-U.S. Department of Agriculture (2017). Nutrient values and weights – Fruits 09070 Sweet Cherry. National Nutrient Database for Standard Reference. The National Agricultural Library. Available online at: http://ndb.nal.usda.gov
Usenik, V., Kastelec, D., and Stampar, F. (2005). Physicochemical changes of sweet cherry fruits related to application of gibberellic acid. Food Chem. 90, 663–671. doi: 10.1016/j.foodchem.2004.04.027
Usenik, V., Fabčič, J., and Štampar, F. (2008). Sugars, organic acids, phenolic composition and antioxidant activity of sweet cherry (Prunus avium L.). Food Chem. 107, 185–192. doi: 10.1016/j.foodchem.2007.08.004
Usenik, V., Fajt, N., Mikulic-Petkovsek, M., Slatnar, A., Stampar, F., and Veberic, R. (2010). Sweet cherry pomological and biochemical characteristics influenced by rootstock. J. Agric. Food Chem. 58, 4928–4933. doi: 10.1021/jf903755b
Valero, D., Díaz-Mula, H. M., Zapata, P. J., Castillo, S., Guillén, F., Martínez-Romero, D., et al. (2011). Postharvest treatments with salicylic acid, acetylsalicylic acid or oxalic acid delayed ripening and enhanced bioactive compounds and antioxidant capacity in sweet cherry. J. Agric. Food Chem. 59, 5483–5489. doi: 10.1021/jf200873j
Valverde, J. M., Giménez, M. J., Valero, D., Martínez-Romero, D., and Serrano, M. (2015). Methyl salicylate treatments of sweet cherry trees increase antioxidant systems in fruit at harvest and during storage. Postharvest Biol. Technol. 109, 106–113. doi: 10.1016/j.postharvbio.2015.06.011
Vamos-Vigyazó, L. (1981). Polyphenol oxidase and peroxidase in fruits and vegetables. Crit. Rev. Food Sci. Nutr. 49, 127–140.
Vangdal, E., Hovland, K. L., Børve, J., Sekse, L., and Slimestad, R. (2008). Foliar application of calcium reduces postharvest decay in sweet cherry by various mechanisms. Acta Hortic. 768, 143–148. doi: 10.17660/ActaHortic.2008.768.16
Vavoura, M. V., Badeka, A. V., Kontakos, S., and Kontominas, M. G. (2015). Characterization of four popular sweet cherry cultivars grown in Greece by volatile compound and physicochemical data analysis and sensory evaluation. Molecules 20, 1922–1940. doi: 10.3390/molecules20021922
Velickova, E., Winkelhausen, E., Kuzmanova, S., Alves, V. D., and Moldao-Martins, M. (2013). Impact of chitosan-beeswax edible coatings on the quality of fresh straw-berries (Fragaria ananassa cv. Camarosa) under commercial storage conditions. LWT Food Sci. Technol. 52, 80–92. doi: 10.1016/j.lwt.2013.02.004
Vroman, I., and Tighzert, L. (2009). Biodegradable polymers. Materials 2, 307–344. doi: 10.3390/ma2020307
Wang, C., Chen, C., and Wang, S. (2009). Changes of flavonoid content and antioxidant capacity in blueberries after illumination with UV-C. Food Chem. 117, 426–431. doi: 10.1016/j.foodchem.2009.04.037
Wang, S., Chen, Y., Xu, Y., Wu, J., Xiao, G., and Fu, M. (2014). Super atmospheric O2 packaging maintains postharvest quality of cherry (Prunus avium L.) fruit. J. Food Process Preserv. 38, 2037–2046. doi: 10.1111/jfpp.12181
Wang, Y., Xie, X., and Long, L. E. (2014). The effect of postharvest calcium application in hydro-cooling water on tissue calcium content, biochemical changes, and quality attributes of sweet cherry fruit. Food Chem. 160, 22–30. doi: 10.1016/j.foodchem.2014.03.073
Wang, L., Zhang, H., Jina, P., Guo, X., Li, Y., Fan, C., et al. (2016). Enhancement of storage quality and antioxidant capacity of harvested sweet cherry fruit by immersion with b-aminobutyric acid. Postharvest Biol. Technol. 118, 71–78. doi: 10.1016/j.postharvbio.2016.03.023
Wang, S. Y. (2006). Effect of pre-harvest conditions on antioxidant capacity in fruits. Acta Hortic. 712, 229–305. doi: 10.17660/ActaHortic.2006.712.33
Wani, A. A., Singh, P., Gul, K., Wani, M. H., and Langowski, H. C. (2014). Sweet cherry (Prunus avium): critical factors affecting the composition and shelf life. Food Pack. Shelf Life 1, 86–99. doi: 10.1016/j.fpsl.2014.01.005
Wen, Y. Q., He, F., Zhu, B. Q., Lan, Y. B., Pan, Q. H., Li, C. Y., et al. (2014). Free and glycosidically bound aroma compounds in cherry (Prunus avium L.). Food Chem. 152, 29–36. doi: 10.1016/j.foodchem.2013.11.092
Whiting, M., and Das, P. (2017). “A precision pollination system for sweet cherry yield security,” in 8th International Cherry Symposium in Yamagata (Yamagata), 61.
Whiting, M. D., and Lang, G. A. (2004). Bing sweet cherry on the dwarfing rootstock Gisela 5: thinning affects fruit quality and vegetative growth but not CO2 exchange. J. Am. Soc. Hortic. Sci. 129, 407–415.
Whiting, M. D., Lang, G., and Ophardt, D. (2005). Rootstock and training system affect sweet cherry growth, yield and fruit quality. HortScience 40, 582–586.
Xia, M., Hou, M., Zhu, H., Ma, J., Tang, Z., Wang, Q., et al. (2005). Anthocyanins induce cholesterol efflux from mouse peritoneal macrophages. The role of peroxisome proliferators-activated receptor Ó-Liver X receptor α-ABCA1 pathway. J. Biol. Chem. 280, 36792–36801. doi: 10.1074/jbc.M505047200
Yao, H., and Tian, S. (2005). Effects of pre- and post-harvest application of salicylic acid or methyl jasmonate on inducing disease resistance of sweet cherry fruit in storage. Postharvest Biol. Technol. 35, 253–262. doi: 10.1016/j.postharvbio.2004.09.001
Yin, X., Seavert, C. F., and Roux, J. (2011). Responses of irrigation water use and productivity of sweet cherry to single-lateral drip Irrigation and ground covers. Soil Sci. 176, 39–47. doi: 10.1097/SS.0b013e3182009dbf
Zhang, C., and Whiting, M. D. (2011). Improving “Bing” sweet cherry fruit quality with plant growth regulators. Sci. Hortic. 127, 341–346.doi: 10.1016/j.scienta.2010.11.006
Zhang, G., Sebolt, A. M., Sooriyapathirana, S. S., Wang, D., Bink, M. C. A. M., Olmstead, J. W., et al. (2010). Fruit size QTL analysis of an F1 population derived from a cross between a domesticated sweet cherry cultivar and a wild forest sweet cherry. Tree Genet. Genomes 6, 25–36. doi: 10.1007/s11295-009-0225-x
Zhang, X., Jiang, Y. M., Peng, F. T., He, N. B., Li, Y. J., and Zhao, D. C. (2007). Changes of aroma components in “Hongdeng” sweet cherry during fruit development. Agric. Sci. China 6, 1376–1382. doi: 10.1016/S1671-2927(07)60186-2
Zoratti, L., Karppinen, K., Escobar, A. L., Häggman, H., and Jaakola, L. (2014). Light-controlled flavonoid biosynthesis in fruits. Front. Plant Sci. 5:534, doi: 10.3389/fpls.2014.00534
Keywords: growth conditions, quality indicators, phenolic compounds, new preservation technologies, breeding for quality
Citation: Correia S, Schouten R, Silva AP and Gonçalves B (2017) Factors Affecting Quality and Health Promoting Compounds during Growth and Postharvest Life of Sweet Cherry (Prunus avium L.). Front. Plant Sci. 8:2166. doi: 10.3389/fpls.2017.02166
Received: 02 October 2017; Accepted: 08 December 2017;
Published: 19 December 2017.
Edited by:
Cai-Zhong Jiang, United States Department of Agriculture, United StatesReviewed by:
Dario Stefanelli, Department of Environment and Primary Industries, Victoria, AustraliaDavid Obenland, United States Department of Agriculture, United States
Copyright © 2017 Correia, Schouten, Silva and Gonçalves. This is an open-access article distributed under the terms of the Creative Commons Attribution License (CC BY). The use, distribution or reproduction in other forums is permitted, provided the original author(s) or licensor are credited and that the original publication in this journal is cited, in accordance with accepted academic practice. No use, distribution or reproduction is permitted which does not comply with these terms.
*Correspondence: Sofia Correia, sofiacorreia@utad.pt