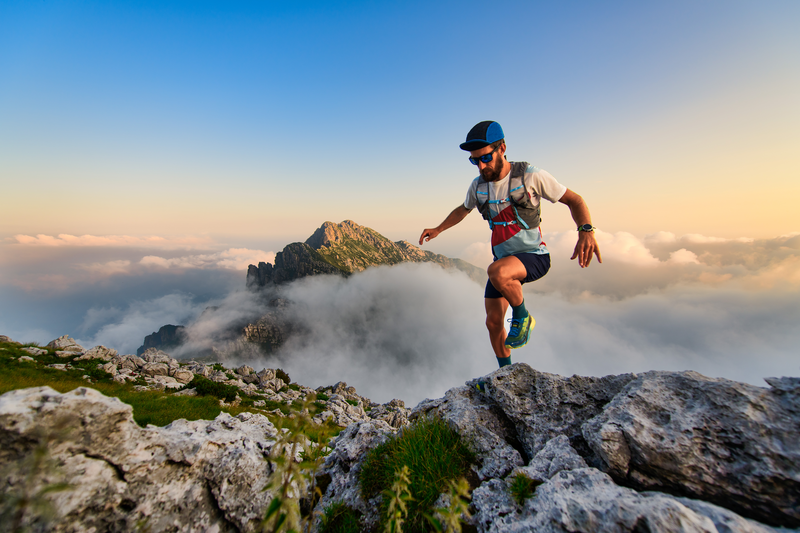
94% of researchers rate our articles as excellent or good
Learn more about the work of our research integrity team to safeguard the quality of each article we publish.
Find out more
REVIEW article
Front. Plant Sci. , 28 November 2017
Sec. Plant Genetics and Genomics
Volume 8 - 2017 | https://doi.org/10.3389/fpls.2017.02041
Polycomb group (PcG) proteins within the polycomb repressive complex 1 (PRC1) and PRC2 are significant epigenetic regulatory factors involved in important cellular and developmental processes in eukaryotes. In Arabidopsis, LIKE HETEROCHROMATIN PROTEIN 1 (LHP1), also known as TERMINAL FLOWER 2, has been proposed as a plant specific subunit of PRC1 that could bind the trimethylated lysine 27 of histone H3 (H3K27me3), which is established by PRC2 and is required for a functional plant PcG system. LHP1 not only interacts with PRC1 to catalyze monoubiquitination at lysine 119 of histone H2A but also functions with PRC2 to establish H3K27me3. This review is about the interaction of LHP1 with PRC1 and PRC2, in which LHP1 may act as a bridge between the two. Meantime, this review highlights that LHP1 could act as an activator and a repressor of transcription.
Eukaryotic genomic DNA is packaged in the nucleus around histone octamers into nucleosomes forming the chromatin structure, consisting of DNA, RNA, histones and non-histone proteins. Each nucleosome contains a stretch of DNA wrapped around an octamer of histone proteins comprising two molecules each of the four core histones H2A, H2B, H3, and H4 (Luger et al., 1997). Each histone has a protrudent N-terminal and/or C-terminal tail and can be subjected to covalent modification by acetylation, methylation, phosphorylation, and other enzymatic reactions (Feng and Shen, 2014; Shi et al., 2014). Covalent modifications of DNA and histones play critical roles in regulating chromatin structure and gene expression, mainly by altering its compaction and shaping the three-dimensional topology of the genome (Mozgova and Hennig, 2015).
The evolutionarily conserved Polycomb group (PcG) proteins broadly found in many multicellular organisms participate in one of the earliest epigenetic regulatory mechanisms. They were originally identified in Drosophila spp. as repressors of homeotic genes (Lewis, 1978). PcG proteins form multiprotein Polycomb Repressive Complexes PRC1 and PRC2, which both have functions in the epigenetic repression of gene expression via histone modifications (Bemer and Grossniklaus, 2012; Molitor and Shen, 2013; Grossniklaus and Paro, 2014; Mozgova and Hennig, 2015; Xiao and Wagner, 2015). PRC2 catalyzes histone H3 lysine-27 trimethylation (H3K27me3), forming a docking site for PRC1, which subsequently recognizes/reads the H3K27me3 mark and further mediates deposition of downstream H2A monoubiquitination (H2Aub1) to repress gene expression (Simon and Kingston, 2013; Mozgova and Hennig, 2015; Xiao and Wagner, 2015). Accordingly, PRC1 activity was proposed to stabilize the repression of H3K27me3 marked loci. However, in recent years there have been numerous reports challenging this model. The relationships between PRC1 and PRC2 components may be far more complex than initially anticipated. PRC1 can be recruited independent of, or even prior to, H3K27me3 deposition, which indicates that PRC1 may act as a docking point for PRC2 and subsequent spreading of H3K27me3 marks at some target genes (Kim et al., 2012; Yang et al., 2013; Calonje, 2014; Molitor et al., 2014; Shen et al., 2014; Merini and Calonje, 2015). For example, during post-germinative repression of seed maturation genes, PRC1 acts upstream of PRC2, since H2Aub preceded H3K27me3 (Yang et al., 2013; Calonje, 2014; Molitor et al., 2014). To date, these possible mechanisms are unclear, especially in plants. In this review, we summarize the recent findings of the role of PcG protein, LHP1, during various developmental programs in plants and highlight that LHP1 could act as an activator and a repressor of transcription.
Up to the present, many plant LIKE HETEROCHROMATIN PROTEIN1 (LHP1) homologs have been identified (Zemach et al., 2006; Mimida et al., 2007; Exner et al., 2009; Hennig and Derkacheva, 2009). However, in addition to Arabidopsis, the current studies of LHP1 are limited to the differences in protein expression profile, but not in-depth study of gene expression, functions, and evolution of plant LHP1. This review, the focus has been on the character of LHP1 in Arabidopsis. LHP1 was initially identified in screens for inflorescence meristem function and was named TERMINAL FLOWER 2 (Larsson et al., 1998; Kotake et al., 2003). LHP1 is a single-copy gene encoding a functional homolog of Pc, despite its structural homology with the protein of metazoan HETEROCHROMATIN PROTEIN 1 (HP1) (Gaudin et al., 2001; Kotake et al., 2003). The Arabidopsis LHP1 gene that encodes an ∼45 kDa protein composed of 445 amino acids is composed of six exons and five introns and localized to chromosome 5 (AT5G17690). LHP1 protein structure is highly evolutionarily conserved and possesses significant homology to HP1, which contains two major structural domains: a chromo domain (CD) and a chromo shadow domain (CSD) (Gaudin et al., 2001; Libault et al., 2005; Zemach et al., 2006). However, HP1 is capable of binding to heterochromatic regions of the genome and binds to H3K9me2/3, whereas LHP1 usually localizes to euchromatin and is needed for maintenance of gene silencing in euchromatin, but not in heterochromatin, which has an affinity for H3K9me3 and H3K27me3 marks (Libault et al., 2005; Nakahigashi et al., 2005). The chromo domain is essential for ensuring the function of the LHP1 protein. Disruption in the functional chromo domain caused a phenotype similar to the lhp1 null mutant and H3K27me3 could not be recognized (Exner et al., 2009). The CSD is also very important for the function of LHP1, which has a potential protein–protein interaction site (Cowieson et al., 2000). LHP1 possesses five K-R/K-X-R/K classical nuclear localization signals (NLSs) (Dingwall and Laskey, 1991). Four NLS are located in the N-terminal end of the protein, while one single motif is present in the C-terminal end.
PRC2s are highly conserved between animals and plants (Chen and Rasmuson-Lestander, 2009; He et al., 2013). For example, the four PRC2 core components in Arabidopsis contain three homologs of the histone methyltransferase enhancer of zeste E(z), namely, CURLY LEAF (CLF), SWINGER (SWN), and MEDEA (MEA) (Grossniklaus et al., 1998; Chanvivattana et al., 2004; Schubert et al., 2006), three homologs of the suppressor of zeste: EMBRYONIC FLOWER 2 (EMF2), FERTILIZATION INDEPENDENT SEED 2 (FIS2) and VERNALIZATION 2 (VRN2) (Luo et al., 1999; Gendall et al., 2001; Yoshida et al., 2001), a single homolog of the Drosophila ESC WD-40 protein: FERTILIZATION INDEPENDENT ENDOSPERM (FIE) (Ohad et al., 1999), and five homologs of p55: MULTICOPY SUPPRESSOR OF IRA 1–5 (MSI1–5) (Table 2). Until now, only MSI1 has been demonstrated as part of the PRC2 complex (Hennig et al., 2005). In contrast, the existence of plant PRC1 complex was debated for a long time, and PRC1 compositions are drastically diverged in plants compared to animals (Molitor and Shen, 2013). In plants, PRC1 contains two RING1 homologs (AtRING1a and AtRING1b), three Psc/PCGF homologs (AtBMI1a, AtBMI1b, and AtBMI1c, Xu and Shen, 2008; Bratzel et al., 2010; Chen et al., 2010), and two other plant-specific proteins EMBRYONIC FLOWER1 (EMF1) and LHP1 (Gaudin et al., 2001; Calonje et al., 2008) (Table 1).
Yeast two-hybrid and pull-down assays showed that AtRING1a, AtBMI1a, AtBMI1b, and AtBMI1c directly interact with LHP1 (Xu and Shen, 2008; Bratzel et al., 2010; Chen et al., 2010). The PRC1 component EMF1 also directly interacts with LHP1 (Wang et al., 2014), indicating that LHP1 is a core component of PRC1 and can be present in several PRC1-like complexes in Arabidopsis. In addition, genetic evidence also demonstrated physical interactions between AtRING1 and LHP1. The single lhp1-/- mutant shows pleiotropic phenotypes, including small, narrow and upward-curled rosette leaves, reduced plant height (more than 50% reduction), early flowering, a terminal flower structure, and smaller and fewer siliques (Larsson et al., 1998; Gaudin et al., 2001; Kotake et al., 2003). Atring1a-/-Atring1b-/-lhp1-/- mutants have more pronounced phenotypes: the plants are extremely small with very few sessile leaves and completely arrested inflorescences (Xu and Shen, 2008). In addition, a recent report showed that LHP1 co-purifies with PRC2, and LHP1 can directly bind MSI1 in vitro and can be co-immunoprecipitated with MSI1 and EMF2 in vivo, showing a complex interaction between LHP1 and EMF–PRC2. The LHP1–PRC2 interactions are, therefore, likely facilitating recruitment of PRC2 to target genes and LHP1 and PRC2 are closely integrated in Arabidopsis (Derkacheva et al., 2013; Wang et al., 2016). LHP1 may act as a bridge between PRC1 and PRC2 as it could directly interact with members of the two.
Accurate flowering time is critical for the reproductive success of plants. FLOWERING LOCUS T (FT) plays a central role in promoting flowering, and the leaf vein is the site where various flowering-regulatory genes converge to regulate FT mRNA expression (Turck et al., 2008). Compared to wild-type, loss-of-function lhp1 mutants exhibit a photoperiod-independent early flowering phenotype. Overexpression of LHP1 (35S:LHP1) showed little effect on flowering time, and ft mutations completely suppressed the early flowering of lhp1. Molecular analyses of the mutants indicated that LHP1 controls flowering time primarily by recognizing and binding to H3K27me3 and directly interacts with FT chromatin repression of FT expression. Studies have demonstrated a high correlation between LHP1 and H3K27me3 deposition, and in the lhp1 background, H3K27me3 deposition was globally reduced (Turck et al., 2007; Veluchamy et al., 2016). However, increasing LHP1 does not delay the timing of floral transition in wild-type Arabidopsis (Takada and Goto, 2003). LHP1 mRNA is widely expressed in all wild-type tissues, but in mature leaves, LHP1 mRNA is only expressed in the vascular tissue (Kotake et al., 2003; Takada and Goto, 2003). EMF1, LHP1, and a histone H3 lysine-4 demethylase form a distinct PcG complex, termed EMF1c, directly represses the FT expression in the vascular tissue before dusk and at night (Wang et al., 2014). In the long-day pathway, CONSTANS (CO) directly activates FT expression and disrupts EMF1 binding to FT chromatin in leaf veins, specifically at dusk (Takada and Goto, 2003). EMF1-PcG complex plays a role downstream of the photoperiod pathway to control FT expression in the phloem. The vascular EMF1c complex integrates several flowering-regulatory pathways to synchronize flowering time to environmental cues. In addition, in vernalization and the autonomous pathway, LHP1 is part of a multiprotein complex with PRC2 to re-establish H3K27me3 in the repression of FLC expression.
Roots are essential organs for absorption, utilization of water and mineral nutrients. The phytohormone auxin plays vital roles for successful growth and development of plants. Altered auxin levels rapidly induce changes in gene expression and cause a change in phenotype or function. In the lhp1 mutant, auxin levels are lower than in wild-type. To investigate the mechanism, the auxin biosynthetic pathways have been studied. The results showed that the expression of auxin biosynthesis related YUCCA genes are down-regulated, resulting in low levels of auxin in the lhp1 mutant. Moreover, LHP1 was found to directly regulates genes in the auxin biosynthetic pathway (Rizzardi et al., 2011). This suggests that LHP1 is involved in auxin biosynthesis through positive regulation of YUCCA genes. In the Arabidopsis root, SCARECROW (SCR), of a transcription factor of plant-specific GRAS family, is required for the first cell division in the roots. The lhp1 mutant has a premature middle cortex phenotype, which is similar to the scr mutant. ChIP-PCR was performed with LHP1 and SCR and demonstrated that LHP1 and SCR act together to suppress premature middle cortex formation (Cui and Benfey, 2009). Previous research showed that the phytohormone gibberellin (GA) plays a key role in middle cortex formation and in the scr mutant. GA suppressed the premature middle cortex phenotype, but the GA biosynthesis inhibitor paclobutrazol (Pac) enhanced the premature middle cortex phenotype (Paquette and Benfey, 2005). Comparing the lhp1 mutant and wild-type roots after treatment with GA or with Pac, middle cortex formation was completely suppressed by GA and Pac caused middle cortex formation in the lhp1 mutants but in none of the wild-type roots (Cui and Benfey, 2009). These results indicated that LHP1 and SCR suppress premature middle cortex formation by a similar mechanism, in which GA has a dominant role.
Generally, polycomb group proteins have been known as transcriptional repressors, which play crucial roles in the maintenance of the transcriptionally silenced state for proper cell differentiation in animals and plants. However, recent studies showed that LHP1 could also be an activator of transcription by differential expression analysis of LHP1-target genes in lhp1 mutants versus wild-types (Veluchamy et al., 2016). By comparing H3K27me3 and RNA Pol II ChIP-Seq results, LHP1 and H3K27me3 deposition are highly positively correlated while LHP1 and RNA Pol II occupancy are negatively correlated. Furthermore, in the lhp1 mutant, it was found that the majority of LHP1 targets were hypo-methylated. These results were consistent with the function of LHP1 as a transcriptional repressor. In addition, more than one-third of LHP1 disturbed target genes were down-regulated in the lhp1 mutant, suggesting that LHP1 can function as a positive regulator of a set of genes (Veluchamy et al., 2016). Rizzardi et al. (2011) research showed that LHP1 exerts a positive role in transcriptional activation of the YUCCA genes.
LHP1 interacts with different proteins in different cell types to perform distinct functions, and to date, several proteins interacting with LHP1 in Arabidopsis have been identified. For example, it has been confirmed by yeast two hybridization, pull down and BiFc methods, that LHP1 directly interacts with leaf development regulators ASYMMETRIC LEAVES 1 (AS1) and AS2 in vitro and in vivo. The wild-type Col-0, as1-1, as2-1, and lhp1 single mutants produced smaller rosette leaves, while the as1-1 lhp1 and as2-1 lhp1 double mutants produced much smaller rosette leaves (Li et al., 2016). This genetic evidence also showed that there is a interaction between LHP1 and AS1/AS2. Beside the direct interaction between AS1/AS2 and LHP, SHORT VEGETATIVE PHASE (SVP), a MADS box transcription factor, can recruit LHP1 to the SEPALLATA 3 (SEP3) gene and repress SEP3 transcription, consequently preventing premature differentiation of floral meristems (Liu et al., 2009). Enhancer of LHP1 (Eol1), a gene related to yeast Chromosome transmission fidelity 4 (Ctf4), recruits LHP1-PRC2 to maintain H3K27me3 levels in dividing cells in Arabidopsis (Zhou et al., 2017b). CYCLOPHILIN71 (CYP71), which controls leaf differentiation and leaf shape establishment, also has been identified to interact with LHP1, and both the chromo and CSDs in LHP1 are required for the interaction (Li and Luan, 2011). Loss of AtCYP71 function results in dwarfism and distorted, small leaves, and delayed overall development. In addition, the double mutant, lhp1 cyp71, showed more severe phenotypes than the single mutants. This genetic evidence indicates that CYP71 and LHP1 synergistically control plant development (Li and Luan, 2011). In addition, in the context of DNA replication, LHP1 interacts with two DNA polymerase (pol) catalytic subunits, INCURVATA2 (ICU2, a subunit of DNA pol alpha) and EARLY IN SHORT DAYS 7 (ESD7, a subunit of DNA pol epsilon) (Barrero et al., 2007; del Olmo et al., 2010). In vitro, LHP1 was reported to interact with the GAGA factor BASIC PENTACYSTEINE6 (BPC6) and was recruited to GAGA motifs (Hecker et al., 2015). The most recent research indicates that LHP1-INTERACTING FACTOR2 (LIF2), with three RNA-recognition motifs (RRMs), is involved in Arabidopsis thaliana cell fate and stress responses and has been shown to interact with LHP1 mediated by the conserved CSD. Interestingly, this interaction links chromatin dynamics, RNA processing, and developmental plasticity together (Latrasse et al., 2011; Molitor et al., 2016). There is evidence that LHP1 interacts with the APOLO long non-coding RNA during the dynamic regulation of chromatin three-dimensional conformation in response to auxin (Ariel et al., 2014). Veluchamy et al. (2016) showed that LHP1 can play a role in the modulation of global chromatin architecture as genome topology was globally altered in the lhp1 background.
In summary, these data strongly suggest that the roles of LHP1 are more complex than once previously thought. LHP1 interacts with several proteins in several pathways to perform its distinct functions. Firstly, it recognizes H3K27me3 in a classic PcG-mediated transcriptional repression pathway. Secondly, transcription factors interact with LHP1 and recruit it to specific DNA elements to initiate gene silencing. Thirdly, through interacting with a replication complex, LHP1 possibly form a high-order complex with polymerase to recruit other PRC2 components to targets after DNA replication. However, more recent research findings argue against the classic PcG-mediated transcriptional repression pathway. For example, LHP1 was originally proposed to be the counterpart of Pc, responsible for recruitment of PRC1 to H3K27me3 catalyzed by PRC2, but recent studies showed that H3K27me3 modifications also require LHP1 (Liu et al., 2009; Derkacheva et al., 2013) and LHP1 coordinates with CLF to regulate H3K27me3 modification and target gene repression. In addition, both CLF and LHP1 are involved in spreading of H3K27me3 marks (Wang et al., 2016). In the classic PcG pathway, PRC2-mediated H3K27me3 recruits PRC1 that in turn monoubiquitinates H2A. Recent study, however, showed that LHP1 is not required for H2AK121ub marking and PRC2 activity is dispensable to establish H2AK121ub marks at most genes (Zhou et al., 2017a). It is obvious that in PRC1-mediated gene repression, the roles of these LHP1 interacting proteins have yet been fully understood. In addition, it is still unclear that in which context they are recruited to specific genes to carry out their functions. Moreover, only a few LHP1 interacting proteins have been identified. Thus, further in depth researches are needed to identify more LHP1-binding proteins and their functions.
Generally, LHP1 is known to act as a transcriptional repressor, both in plants and animals. Recently, growing evidences show that LHP1 can also act as a activator. For example, LHP1 positive regulates YUCC4 expression (Rizzardi et al., 2011), LHP1 exerts a positive role in environmental stimuli (Veluchamy et al., 2016) and LHP1 interacts with GmPHD6 to form a transcriptional activation complex to regulate genes for salt tolerance (Wei et al., 2017). At present, although the study of plant LHP1 as an activator is limited, studies have long been shown that HP, a homologs of LHP1, can act as activators. For example, HP1 has been reported involvement in the activation of several euchromatic genes in Drosophila. By immunostaining experiments using an HP1 antibody, it found that HP1 is associated with developmental and heat shock-induced puffs on polytene chromosomes. And HP1 is positively involved in Hsp70 gene activity (Piacentini et al., 2003). Mammalian HP1 homologs together with dimethylated H3K9 also associate with many active transcription units (Vakoc et al., 2005). In addition, the mammalian HP1γ and Drosophila melanogaster HP1α, involving in positive euchromatic gene regulation (Piacentini et al., 2003; Cryderman et al., 2005; Vakoc et al., 2005; Fanti and Pimpinelli, 2008; Smallwood et al., 2012; Eissenberg and Elgin, 2014). In Drosophila, HP1α positively regulates euchromatic gene expression through RNA transcript association and interaction with hnRNPs (Piacentini et al., 2003, 2009; De Lucia et al., 2005; Vermaak and Malik, 2009; Piacentini and Pimpinelli, 2010). HP1c, a homolog of HP1, appears to be linked to transcription activity rather than repression in Drosophila (De Wit et al., 2007). Regardless of the positive and negative effects of HP1, are determined by its interacting proteins. The activator and repressor activities require distinct protein domains for different DNA–protein, RNA–protein, or protein–protein interactions. HP1 has different domains that should confer it to the necessary structural flexibility required for multiple functional roles. Plant LHP1 is able to perform several of the functions carried out by the HETEROCHROMATIN PROTEIN1 family in animals. However, the molecular mechanisms underlying its dual role on gene expression remain to be elucidated.
JF wrote this manuscript and JL provide assistance.
The authors declare that the research was conducted in the absence of any commercial or financial relationships that could be construed as a potential conflict of interest.
This article was partially supported by the Program from Postdoctoral Foundation of Guangxi Academy of Agricultural Sciences (GNKB2016025), Guangxi Natural Science Foundation (2016GXNSFCA380020), Bagui Scholar Fund of Guangxi ([2013]3), the Science and Technology Development Fund of Guangxi Academy of Agricultural Sciences (2015YT95).
Ariel, F., Jegu, T., Latrasse, D., Romero-Barrios, N., Christ, A., Benhamed, M., et al. (2014). Noncoding transcription by alternative RNA polymerases dynamically regulates an auxin-driven chromatin loop. Mol. Cell 55, 383–396. doi: 10.1016/j.molcel.2014.06
Barrero, J. M., González-Bayón, R., del Pozo, J. C., Ponce, M. R., and Micol, J. L. (2007). INCURVATA2 encodes the catalytic subunit of DNA Polymer-ase alpha and interacts with genes involved in chromatin-mediated cellular memory in Arabidopsis thaliana. Plant Cell 19, 2822–2838. doi: 10.1105/tpc.107.054130
Bemer, M., and Grossniklaus, U. (2012). Dynamic regulation of Polycomb group activity during plant development. Curr. Opin. Plant Biol. 15, 523–529. doi: 10.1016/j.pbi.2012.09.006
Bratzel, F., López-Torrejón, G., Koch, M., Del Pozo, J. C., and Calonje, M. (2010). Keeping cell identity in Arabidopsis requires PRC1 RING-finger homologs that catalyze H2A monoubiquitination. Curr. Biol. 20, 1853–1859. doi: 10.1016/j.cub.2010.09.046
Calonje, M. (2014). PRC1 marks the difference in plant PcG repression. Mol. Plant. 7, 459–471. doi: 10.1093/mp/sst150
Calonje, M., Sanchez, R., Chen, L., and Sung, Z. R. (2008). EMBRYONIC FLOWER1 participates in polycomb group-mediated AG gene silencing in Arabidopsis. Plant Cell 20, 277–291. doi: 10.1105/tpc.106.049957
Chanvivattana, Y., Bishopp, A., Schubert, D., Stock, C., Moon, Y. H., Sung, Z. R., et al. (2004). Interaction of Polycomb-group proteins controlling flowering in Arabidopsis. Development 131, 5263–5276. doi: 10.1242/dev.01400
Chen, D., Molitor, A., Liu, C., and Shen, W. H. (2010). The Arabidopsis PRC1-like ring-finger proteins are necessary for repression of embryonic traits during vegetative growth. Cell Res. 20, 1332–1344. doi: 10.1038/cr.2010.151
Chen, S., and Rasmuson-Lestander, A. (2009). Regulation of the Drosophila engrailed gene by Polycomb repressor complex 2. Mech. Dev. 126, 443–448. doi: 10.1016/j.mod.2009.01.004
Cowieson, N. P., Partridge, J. F., Allshire, R. C., and McLaughlin, P. J. (2000). Dimerisation of a chromo shadow domain and distinctions from the chromodomain as revealed by structural analysis. Curr. Biol. 10, 517–525. doi: 10.1016/S0960-9822(00)00467-X
Cryderman, D. E., Grade, S. K., Li, Y., Fanti, L., Pimpinelli, S., and Wallrath, L. L. (2005). Role of Drosophila HP1 in euchromatic gene expression. Dev. Dyn. 232, 767–774. doi: 10.1002/dvdy.20310
Cui, H., and Benfey, P. N. (2009). Interplay between SCARECROW, GA and LIKE HETEROCHROMATIN PROTEIN 1 in ground tissue patterning in the Arabidopsis root. Plant J. 58, 1016–1027. doi: 10.1111/j.1365-313X.2009.03839.x
De Lucia, F., Ni, J. Q., Vaillant, C., and Sun, F. L. (2005). HP1 modulates the transcription of cell-cycle regulators in Drosophila melanogaster. Nucleic Acids Res. 33, 2852–2858. doi: 10.1093/nar/gki584
De Wit, E., Greil, F., and van Steensel, B. (2007). High-resolution mapping reveals links of HP1 with active and inactive chromatin components. PLOS Genet. 3:e38. doi: 10.1371/journal.pgen.0030038
del Olmo, I., Lopez-Gonzalez, L., Martin-Trillo, M. M., Martinez-Zapater, J. M., Pineiro, M., and Jarillo, J. A. (2010). EARLY IN SHORT DAYS 7(ESD7) encodes the catalytic subunit of DNA polymerase epsilon and is required for flowering repression through a mechanism involving epigenetic gene silencing. Plant J. 61, 623–636. doi: 10.1111/j.1365-313X.2009.04093.x
Derkacheva, M., Steinbach, Y., Wildhaber, T., Mozgova, I., Mahrez, W., Nanni, P., et al. (2013). Arabidopsis MSI1 con-nects LHP1 to PRC2 complexes. EMBO J. 32, 2073–2085. doi: 10.1038/emboj.2013.145
Dingwall, C., and Laskey, R. A. (1991). Nuclear targeting sequences–a consensus? Trends Biochem Sci. 16, 478–481.
Eissenberg, J. C., and Elgin, S. C. R. (2014). HP1a: a structural chromosomal protein regulating transcription. Trends Genet. 30, 103–110. doi: 10.1016/j.tig.2014.01.002
Exner, V., Aichinger, E., Shu, H., Wildhaber, T., Alfarano, P., Caflisch, A., et al. (2009). The chromodomain of LIKE HETEROCHROMATIN PROTEIN 1 is essential for H3K27me3 binding and function during Arabidopsis development. PLOS ONE 4:e5335. doi: 10.1371/journal.pone.0005335
Fanti, L., and Pimpinelli, S. (2008). HP1: a functionally multifaceted protein. Curr. Opin. Genet. Dev. 18, 169–174. doi: 10.1016/j.gde.2008.01.009
Feng, J., and Shen, W. H. (2014). Dynamic regulation and function of histone monoubiquitination in plants. Front. Plant Sci. 5:83. doi: 10.3389/fpls.2014.00083
Gaudin, V., Libault, M., Pouteau, S., Juul, T., Zhao, G., Lefebvre, D., et al. (2001). Mutations in LIKE HETEROCHROMATIN PROTEIN 1 affect flowering time and plant architecture in Arabidopsis. Development 128, 4847–4858.
Gendall, A. R., Levy, Y. Y., Wilson, A., and Dean, C. (2001). The VERNALIZATION 2 gene mediates the epigenetic regulation of vernalization in Arabidopsis. Cell 107, 525–535. doi: 10.1016/S0092-8674(01)00573-6
Grossniklaus, U., and Paro, R. (2014). Transcriptional silencing by polycomb-group proteins. Cold Spring Harb. Perspect. Biol. 6:a019331. doi: 10.1101/cshperspect.a019331
Grossniklaus, U., Vielle-Calzada, J. P., Hoeppner, M. A., and Gagliano, W. B. (1998). Maternal control of embryogenesis by MEDEA, a polycomb group gene in Arabidopsis. Science 280, 446–450. doi: 10.1126/science.280.5362.446
He, C., Huang, H., and Xu, L. (2013). Mechanisms guiding Polycomb activities during gene silencing in Arabidopsis thaliana. Front. Plant Sci. 4:454. doi: 10.3389/fpls.2013.00454
Hecker, A., Brand, L. H., Peter, S., Simoncello, N., Kilian, J., Harter, K., et al. (2015). The Arabidopsis GAGA-binding factor BPC6 recruits PRC1 component LHP1 to GAGA DNA-motifs. Plant Physiol. 168, 1013–1024. doi: 10.1104/pp.15.00409
Hennig, L., Bouveret, R., and Gruissem, W. (2005). MSI1-like proteins: an escort service for chromatin assembly and remodeling complexes. Trends Cell Biol. 15, 295–302. doi: 10.1016/j.tcb.2005.04.004
Hennig, L., and Derkacheva, M. (2009). Diversity of Polycomb group complexes in plants: same rules, different players? Trends Genet. 25, 414–423. doi: 10.1016/j.tig.2009.07.002
Kim, S. Y., Lee, J., Eshed-Williams, L., Zilberman, D., and Sung, Z. R. (2012). EMF1 and PRC2 cooperate to repress key regulators of Arabidopsis development. PLOS Genet. 8:e1002512. doi: 10.1371/journal.pgen.1002512
Kotake, T., Takada, S., Nakahigashi, K., Ohto, M., and Goto, K. (2003). Arabidopsis TERMINAL FLOWER 2 gene encodes a LIKE HETEROCHROMATIN PROTEIN 1 homolog and represses both FLOWERING LOCUS T to regulate flowering time and several floral homeotic genes. Plant Cell Physiol. 44, k555–564. doi: 10.1093/pcp/pcg091
Larsson, A. S., Landberg, K., and Meeks-Wagner, D. R. (1998). The TERMINAL FLOWER 2 (TFL2) gene controls the reproductive transition and meristem identity in Arabidopsis thaliana. Genetics 149, 597–605.
Latrasse, D., Germann, S., Houba-Herin, N., Dubois, E., Bui-Prodhomme, D., Hourcade, D., et al. (2011). Control offlowering and cell fate by LIF2, an RNA binding partner of the polycomb complex component LHP1. PLOS ONE 6:e16592. doi: 10.1371/journal.pone.0016592
Lewis, E. B. (1978). A gene complex controlling segmentation in Drosophila. Nature 276, 565–570. doi: 10.1038/276565a0
Li, H., and Luan, S. (2011). The Cyclophilin AtCYP71 Interacts with CAF-1 and LHP1 and functions in multiple chromatin remodeling processes. Mol. Plant 4, 748–758. doi: 10.1093/mp/ssr036
Li, Z., Li, B., Liu, J., Guo, Z., Liu, Y., Li, Y., et al. (2016). Transcription factors AS1 and AS2 interact with LHP1 to repress KNOX genes in Arabidopsis. J. Integr. Plant Biol. 58, 959–970. doi: 10.1111/jipb.12485
Libault, M., Tessadori, F., Germann, S., Snijder, B., Fransz, P., and Gaudin, V. (2005). The Arabidopsis LHP1 protein is a component of euchromatin. Planta 222, 910–925. doi: 10.1007/s00425-005-0129-4
Liu, C., Xi, W., Shen, L., Tan, C., and Yu, H. (2009). Regulation of floral patterning by flowering time genes. Dev. Cell 16, 711–722. doi: 10.1016/j.devcel.2009.03.011
Luger, K., Mader, A. W., Richmond, R. K., Sargent, D. F., and Richmond, T. J. (1997). Crystal structure of the nucleosome core particle at 2.8 A resolution. Nature 389, 251–260. doi: 10.1038/38444
Luo, M., Bilodeau, P., Koltunow, A., Dennis, E. S., Peacock, W. J., and Chaudhury, A. M. (1999). Genes controlling fertilization-independent seed development in Arabidopsis thaliana. Proc. Natl. Acad. Sci. U.S.A. 96, 296–301. doi: 10.1073/pnas.96.1.296
Merini, W., and Calonje, M. (2015). PRC1 is taking the lead in PcG repression. Plant J. 83, 110–120. doi: 10.1111/tpj.12818
Mimida, N., Kidou, S., and Kotoda, N. (2007). Constitutive expression of two apple (Malus × domestica Borkh.) homolog genes of LIKE HETEROCHROMATIN PROTEIN1 affects flowering time and whole-plant growth in transgenic Arabidopsis. Mol. Genet. Genomics 278, 295–305. doi: 10.1007/s00438-007-0250-0
Molitor, A., and Shen, W. H. (2013). The Polycomb complex PRC1: composition and function in plants. J. Genet. Genomics 40, 231–238. doi: 10.1016/j.jgg.2012.12.005
Molitor, A. M., Bu, Z., Yu, Y., and Shen, W. H. (2014). Arabidopsis AL PHD-PRC1 complexes promote seed germination through H3K4me3-to-H3K27me3 chromatin state switch in repression of seed developmental genes. PLOS Genet. 10:e1004091. doi: 10.1371/journal.pgen.1004091
Molitor, A. M., Latrasse, D., Zytnicki, M., Andrey, P., Houba-Hérin, N., Hachet, M., et al. (2016). The Arabidopsis hnRNP-Q Protein LIF2 and the PRC1 Subunit LHP1 function in concert to regulate the transcription of stress-responsive genes. Plant Cell 28, 2197–2211. doi: 10.1105/tpc.16.00244
Mozgova, I., and Hennig, L. (2015). The polycomb group protein regulatory network. Annu. Rev. Plant Biol. 66, 269–296. doi: 10.1146/annurev-arplant-043014-115627
Nakahigashi, K., Jasencakova, Z., Schubert, I., and Goto, K. (2005). The Arabidopsis heterochromatin protein1 homo-log (TERMINAL FLOWER2) silences genes within the euchromatic region but not genes positioned in heterochromatin. Plant Cell Physiol. 46, 1747–1756. doi: 10.1093/pcp/pci195
Ohad, N., Yadegari, R., Margossian, L., Hannon, M., Michaeli, D., Harada, J. J., et al. (1999). Mutations in FIE, a WD polycomb group gene, allow endosperm development without fertilization. Plant Cell 11, 407–416. doi: 10.1105/tpc.11.3.407
Paquette, A. J., and Benfey, P. N. (2005). Maturation of the ground tissue of the root is regulated by gibberellin and SCARECROW and requires SHORT-ROOT. Plant Physiol. 138, 636–640. doi: 10.1104/pp.104.058362
Piacentini, L., Fanti, L., Berloco, M., Perrini, B., and Pimpinelli, S. (2003). Heterochromatin protein 1 (HP1) is associated with induced gene expression in Drosophila euchromatin. J. Cell Biol. 161, 707–714. doi: 10.1083/jcb.200303012
Piacentini, L., Fanti, L., Negri, R., Del Vescovo, V., Fatica, A., Altieri, F., et al. (2009). Heterochromatin protein 1 (HP1a) positively regulates euchromatic gene expression through RNA transcript association and interaction with hnRNPs in Drosophila. PLOS Genet. 5:e1000670. doi: 10.1371/journal.pgen.1000670
Piacentini, L., and Pimpinelli, S. (2010). Positive regulation of euchromatic gene expression by HP1. Fly (Austin). 4, 299–301. doi: 10.4161/fly.4.4.13261
Rizzardi, K., Landberg, K., Nilsson, L., Ljung, K., and Sundås-Larsson, A. (2011). TFL2/LHP1 is involved in auxin biosynthesis through positive regulation of YUCCA genes. Plant J. 65, 897–906. doi: 10.1111/j.1365-313X.2010.04470.x
Schubert, D., Primavesi, L., Bishopp, A., Roberts, G., Doonan, J., Jenuwein, T., et al. (2006). Silencing by plant polycomb-group genes requires dispersed trimethylation of histone H3 at lysine 27. EMBO J. 25, 4638–4649. doi: 10.1038/sj.emboj.7601311
Shen, L., Thong, Z., Gong, X., Shen, Q., Gan, Y., and Yu, H. (2014). The putative PRC1 RING-finger protein AtRING1A regulates flowering through repressing MADS AFFECTING FLOWERING genes in Arabidopsis. Development 14, 1303–1312. doi: 10.1242/dev.104513
Shi, J., Dong, A., and Shen, W. H. (2014). Epigenetic regulation of rice flowering and reproduction. Front. Plant Sci. 5:803. doi: 10.3389/fpls.2014.00803
Simon, J. A., and Kingston, R. E. (2013). Occupying chromatin: polycomb mechanisms for getting to genomic targets, stopping transcriptional traffic, and staying put. Mol. Cell 49, 808–824. doi: 10.1016/j.molcel.2013.02.013
Smallwood, A., Hon, G. C., Jin, F., Henry, R. E., Espinosa, J. M., and Ren, B. (2012). CBX3 regulates efficient RNA processing genome-wide. Genome Res. 22, 1426–1436. doi: 10.1101/gr.124818.111
Takada, S., and Goto, K. (2003). Terminal flower 2, an Arabidopsis homolog of heterochromatin protein1, counteracts the activation of flowering locus Tby constansin the vascular tissues of leaves to regulate flowering time. Plant Cell 15, 2856–2865. doi: 10.1105/tpc.016345
Turck, F., Fornara, F., and Coupland, G. (2008). Regulation and identity of florigen: FLOWERING LOCUS T moves center stage. Annu. Rev. Plant Biol. 59, 573–594. doi: 10.1146/annurev.arplant.59.032607.092755
Turck, F., Roudier, F., Farrona, S., Martin-Magniette, M. L., Guillaume, E., Buisine, N., et al. (2007). Arabidopsis TFL2/LHP1 specifically associates with genes marked by trimethylation of histone H3 lysine 27. PLOS Genet. 3:e86. doi: 10.1371/journal.pgen.0030086
Vakoc, C. R., Mandat, S. A., Olenchock, B. A., and Blobel, G. A. (2005). Histone H3 lysine 9 methylation and HP1gamma are associated with transcription elongation through mammalian chromatin. Mol. Cell 19, 381–391. doi: 10.1016/j.molcel.2005.06.011
Veluchamy, A., Jégu, T., Ariel, F., Latrasse, D., Mariappan, K. G., Kim, S. K., et al. (2016). LHP1 Regulates H3K27me3 spreading and shapes the Three-dimensional conformation of the Arabidopsis Genome. PLOS ONE 11:e0158936. doi: 10.1371/journal.pone.0158936
Vermaak, D., and Malik, H. S. (2009). Multiple roles for heterochromatin protein 1 genes in Drosophila. Annu. Rev. Genet. 43, 467–492. doi: 10.1146/annurev-genet-102108-134802
Wang, H., Liu, C., Cheng, J., Liu, J., Zhang, L., He, C., et al. (2016). Arabidopsis flower and embryo developmental genes are repressed in seedlings by different combinations of polycomb group proteins in association with distinct sets of Cis-regulatory elements. PLOS Genet. 12:e1005771. doi: 10.1371/journal.pgen.1005771
Wang, Y. Z., Gu, X. F., Yuan, W. Y., Schmitz, R. J., and He, Y. H. (2014). Photoperiodic control of the floral transition through a distinct polycomb repressive complex. Dev. Cell 6, 727–736. doi: 10.1016/j.devcel.2014.01.029
Wei, W., Tao, J. J., Chen, H. W., Li, Q. T., Zhang, W. K., Ma, B., et al. (2017). A histone code reader and a transcriptional activator interact to regulate genes for salt tolerance. Plant Physiol. 175, 1304–1320. doi: 10.1104/pp.16.01764
Xiao, J., and Wagner, D. (2015). Polycomb repression in the regulation of growth and development in Arabidopsis. Curr. Opin. Plant Biol. 23, 15–24. doi: 10.1016/j.pbi.2014.10.003
Xu, L., and Shen, W. H. (2008). Polycomb silencing of KNOX genes confines shoot stem cell niches in Arabidopsis. Curr. Biol. 18, 1966–1971. doi: 10.1016/j.cub.2008.11.019
Yang, C., Bratzel, F., Hohmann, N., Koch, M., Turck, F., and Calonje, M. (2013). VAL- and AtBMI1-mediated H2Aub initiate the switch from embryonic to postgerminative growth in Arabidopsis. Curr. Biol. 23, 1324–1329. doi: 10.1016/j.cub.2013.05.050
Yoshida, N., Yanai, Y., Chen, L., Kato, Y., Hiratsuka, J., Miwa, T., et al. (2001). EMBRYONIC FLOWER2, a novel polycomb group protein homolog, mediates shoot development and flowering in Arabidopsis. Plant Cell 13, 2471–2481. doi: 10.1105/tpc.13.11.2471
Zemach, A., Li, Y., Ben-Meir, H., Oliva, M., Mosquna, A., Kiss, V., et al. (2006). Different domains control the localization and mobility of LIKE HETEROCHROMATIN PROTEIN1 in Arabidopsis nuclei. Plant Cell 18, k133–145. doi: 10.1105/tpc.105.036855
Zhou, Y., Romero-Campero, F. J., Gómez-Zambrano,Á., Turck, F., and Calonje, M. (2017a). H2A monoubiquitination in Arabidopsis thaliana is generally independent of LHP1 and PRC2 activity. Genome Biol. 18, 69. doi: 10.1186/s13059-017-1197-z
Keywords: polycomb group, LHP1, chromatin, histone modification, Arabidopsis thaliana
Citation: Feng J and Lu J (2017) LHP1 Could Act as an Activator and a Repressor of Transcription in Plants. Front. Plant Sci. 8:2041. doi: 10.3389/fpls.2017.02041
Received: 12 July 2017; Accepted: 14 November 2017;
Published: 28 November 2017.
Edited by:
Dao-Xiu Zhou, Université Paris-Sud, FranceReviewed by:
Yuhai Cui, Agriculture and Agri-Food Canada (AAFC), CanadaCopyright © 2017 Feng and Lu. This is an open-access article distributed under the terms of the Creative Commons Attribution License (CC BY). The use, distribution or reproduction in other forums is permitted, provided the original author(s) or licensor are credited and that the original publication in this journal is cited, in accordance with accepted academic practice. No use, distribution or reproduction is permitted which does not comply with these terms.
*Correspondence: Jiang Lu, amlhbmcubHVAc2p0dS5lZHUuY24= Jing Feng, ZmVuZ2ppbmcwNDE0MTQxQDE2My5jb20=
Disclaimer: All claims expressed in this article are solely those of the authors and do not necessarily represent those of their affiliated organizations, or those of the publisher, the editors and the reviewers. Any product that may be evaluated in this article or claim that may be made by its manufacturer is not guaranteed or endorsed by the publisher.
Research integrity at Frontiers
Learn more about the work of our research integrity team to safeguard the quality of each article we publish.