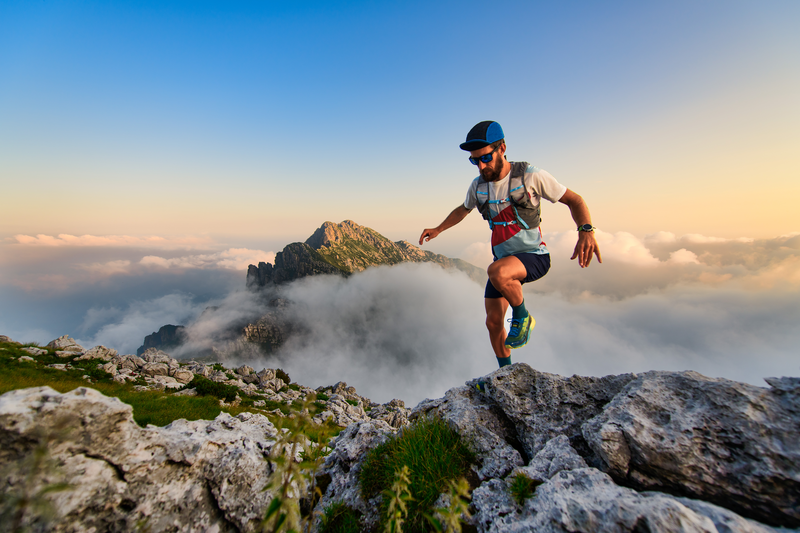
94% of researchers rate our articles as excellent or good
Learn more about the work of our research integrity team to safeguard the quality of each article we publish.
Find out more
MINI REVIEW article
Front. Plant Sci. , 09 August 2017
Sec. Plant Metabolism and Chemodiversity
Volume 8 - 2017 | https://doi.org/10.3389/fpls.2017.01390
Withania somnifera, commonly known as Ashwagandha an important medicinal plant largely used in Ayurvedic and indigenous medicine for over 3,000 years. Being a medicinal plant, dried powder, crude extract as well as purified metabolies of the plant has shown promising therapeutic properties. Withanolides are the principal metabolites, responsible for the medicinal properties of the plant. Availability and amount of particular withanolides differ with tissue type and chemotype and its importance leads to identification characterization of several genes/ enzymes related to withanolide biosynthetic pathway. The modulation in withanolides can be achieved by controlling the environmental conditions like, different tissue culture techniques, altered media compositions, use of elicitors, etc. Among all the in vitro techniques, hairy root culture proved its importance at industrial scale, which also gets benefits due to more accumulation (amount and number) of withanolides in roots tissues of W. somnifera. Use of media compostion and elicitors further enhances the amount of withanolides in hairy roots. Another important modern day technique used for accumulation of desired secondary metabolites is modulating the gene expression by altering environmental conditions (use of different media composition, elicitors, etc.) or through genetic enginnering. Knowing the significance of the gene and the key enzymatic step of the pathway, modulation in withanolide contents can be achieved upto required amount in therapeutic industry. To accomplish maximum productivity through genetic enginnering different means of Withania transformation methods have been developed to obtain maximum transformation efficiency. These standardized transformation procedues have been used to overexpress/silence desired gene in W. somnifera to understand the outcome and succeed with enhanced metabolic production for the ultimate benefit of human race.
Withania somnifera (Ashwagandha; Solanaceae family) is one of the most recognized and studied medicinal plants due to its wide distribution all around the world. W. somnifera has been used for over 3,000 years in indigenous medicine (Ayurvedic) system (Scartezzini and Speroni, 2000; Kumar and Kalonia, 2007; Tuli and Sangwan, 2009; Singh et al., 2015b). Several studies collectively provide metabolic insight of more than 200 primary and secondary metabolic components of W. somnifera. Significance of Withania in therapeutic world has been recognized due to maximum accumulation and diversified form of withanolide. All the identified variants of withanolides became interesting for researchers due to their beneficial effects for human body (Figure 1A; Kumar et al., 2007; Kulkarni and Dhir, 2008; Sharada et al., 2008; Mirjalili et al., 2009; Singh et al., 2010; Dar et al., 2015).
Figure 1. (A) Some important therapeutic uses of Withania somnifera with few proposed mode of actions (Dar et al., 2015); (B) Possible metabolic pathway for withanolides and glycowithanolides (withanosides) production (Senthil et al., 2010; Dhar et al., 2013; Sabir et al., 2013; Singh et al., 2015b) [GA-3P, glyceraldehyde-3-phosphate; MEP, 2-methyl- D-erythritol 4-phosphate; DMAPP, dimethylalyl pyrophosphate; IPP, isopentenyl pyrophosphate; IPPI, isopentenyl diphosphate isomerise; HMG-CoA, 3-hydroxy-3-methylglutaryl-coenzyme A; DXP, 1-deoxy-D-xylulose 5-phosphate; MVAPK, mevalonate phosphate kinase; MVAPP, diphosphomevalonate decarboxylase; CDP-ME, 4-diphospho-cytidyl-2-methyl-D-erythritol; CMS, 4-(cytidine-5-diphospho)-2-C-tmethyl-Derythritol synthase; CMK, 4-(cytidine-5-diphospho)-2-C-methyl-D-erythritol kinase, CDP-MEP, 2-C-methyl- D-erythritol-2-phosphate; MCS, 2-C-methyl-D-erythritol-2,4-cyclodiphosphate synthase; HDS, Hydroxy methyl butenyl 4- diphosphate synthase; HMBPP, Hydroxy methyl butenyl 4-diphosphate; HDR, Hydroxy methyl butenyl 4-diphosphate reductase].
Biosynthesis of metabolites could be improved effectively through genetic engineering, which requires full information of all the genes/enzymes involve in biosynthetic pathway. Using the limited reports available on genes as well as enzymes of W. somnifera, researchers have prosposed possible metabolic pathway for the synthesis of different withanolides (Figure 1B; Senthil et al., 2010; Dhar et al., 2013; Sabir et al., 2013). Genes, enzymes as well as metabolites of respective metabolic pathway show differential pattern of expression according to the plant part, age, season, and other environmental factors. Optimization of various tissue culture techniques become very important to explore W. somnifera at different aspects, as plants obtained from fileds are not enough for all in vitro studies. Therefore, efficient tissue culture techniques like, micropropogation, regeneration, organogenesis, hairy root production, etc. have been established. Also, development of transgenic plants has been considered as the most economical way to improve the yield of therapeutic metabolites on large scale.
Present review recognizes the importance of W. somnifera and disscuss in detail genes/enzymes involved in the biosynthesis of secondary metabolites. The review also includes the significance of in vitro techniques in order to modulate the productivity of W. somnifera according to the desired final product. Suitable combinations of these findings create a very cooperative setting to modulate expression profile of various genes using different circumstances, results in synthesis of various secondary metabolites of W. somnifera.
Withanolide biosynthesis involves the key upstream metabolic step of isoprenogenesis using isoprenoid as precursor. Isoprenogenesis is known to proceeds through two different independent pathways; mevalonic acid (MVA) and methylerythritol phosphate (MEP; also called deoxyxylulose pathway, DOXP) pathway (Chaurasiya et al., 2007; Sangwan et al., 2007). These pathways occur in cytosol and plastid, respectively and ultimately synthesizes the 30 carbon compound (triterpenoids), 24-methylene cholesterol (Figure 1B). Till date, complete information of whole withanolide biosynthesis pathway is not available. However, combination of several studies provide an overview of pathway illustrating several enzymatic steps (Mirjalili et al., 2009; Senthil et al., 2010; Chaurasiya et al., 2012; Gupta et al., 2013a,b, 2015; Dhar et al., 2015). Enzymatic steps of MVA and MEP pathways has been prescribed through the first transcriptome analysis of the plant (Senthil et al., 2010), which keeps improving with advancement in techniques (Gupta et al., 2013b, 2015; Senthil et al., 2015). These analyses reveal numbers of tissue specific unique sequences, differentially expressed genes related to biosynthesis of secondary metabolites.
Genes involved in biosynthesis of withanolides are Δ14-sterol reductase (EC 1.3.1.70), 1-deoxy-D-xylulose-5-phosphate reducto-isomerase/reductase (DXR; EC 1.1.1.267), 1-deoxy-D-xylulose-5-phosphate synthase (DXS; EC 2.2.1.7), 2-C-methyl-D-erythritol 2,4-cyclodiphosphate synthase (MEcPP synthase, IspF, EC 4.6.1.12), 2-C-methyl-D-erythritol 4-phosphate cytidylyl transferase (EC 2.7.7.60), 3-hydroxy-3-methylglutaryl-coenzymeA reductase (HMGR; EC 1.1.1.34), 4-diphosphocytidyl-2-C-methyl-D-erythritol kinase (EC 2.7.1.148), 4-hydroxy-3-methylbut-2-enyldiphosphate reductase (EC 1.17.1.2), 4-hydroxy-3-methylbut-2-enyldiphosphate synthase (HMB-PPS, IspG, EC 1.17.7.1), acetyl-CoA acetyltransferase (ACT, EC 2.3.1.9), C-5-sterol desaturase (C5SD, EC 1.14.19.20), cycloartenol C-24 methyltransferase (EC 2.1.1.142), cycloartenol synthase (CAS; EC 5.4.99.8), cycloeucalenol cycloisomerase (EC 5.5.1.9), cytochrome-P450s reductase (CPR, EC 1.6.2.4), farnesyl diphosphate synthase (FPPS, EC 2.5.1.10), geranyl diphosphate synthase (GPPS, EC 2.5.1.1), geranyl-geranyl diphosphate synthase (GGPPS, EC 2.5.1.29), glycosyltransferases (GT, EC 2.4.-), hydroxymethyl glutaryl-CoA synthase (HMGS, EC 2.3.3.10), isopentenyl diphosphate isomerase (IPPI, EC 5.3.3.2), methyltransferase (MT, EC 2.1.1.), mevalonate diphosphosphate decarboxylase (EC 4.1.1.33), mevalonate kinase (MVAK, EC 2.7.1.36), obtusifoliol 14-demethylase (EC 1.14.13.70), phosphomevalonate kinase (EC 2.7.4.2), squalene synthase (SQS, EC 2.5.1.21), squalene monooxygenase/epoxidase (SQE, 1.14.14.17), sterol Δ7 reductase (DWF, EC 1.3.1.21), etc. (Senthil et al., 2010, 2015; Gupta et al., 2013b, 2015).
To understand the interactions of various molecular network in entirety, Dhar et al. (2015) and Singh et al. (2015b) summarized the available information of some in vitro studies with respect to regulation of pathway genes required for withanolide accumulation.
Among a number of enzymes, SQS and SQE are considered as an important enzymes in the biosynthesis of triterpenoids. Considering this, Gupta et al. (2012) characterize isoforms of SQS gene, while, Razdan et al. (2013) perform characterization and promoter analysis of SQE gene from W. somnifera. To confirm the functional activity, both studies also involved the cloning, expression and purification of gens/enzymes in E. coli. Genes encoding DXS, DXR and HMGR enzymes expressed their importance by catalyzing the key regulatory step of the isoprenoid biosynthesis. These genes revealed tissue specific, chemotype specific and modulated expression while exposed to SA, MeJA, as well as MI (Akhtar et al., 2013; Gupta et al., 2013c).
Few members of sterol-GT (SGT) gene family of W. somnifera, have been recognized and characterized (Sharma et al., 2007; Madina et al., 2007a,b; Chaturvedi et al., 2012). SGTs are responsible for diversified glycosylation of sterols (including withanolides). The identified SGTs expressed different level of expression in different tissues as well as under different stress conditions, to proove their physiological importance (Sharma et al., 2007; Chaturvedi et al., 2011, 2012). Purified SGTs showed broad substrate specificity for sugar acceptor but not for the sugar donor (Madina et al., 2007a,b). Similar pattern of glycosylation was observed by Singh et al. (2013) during functional characterization of flavonoid-GT gene from W. somnifera.
Relation among few pathway genes, withanolides accumulation with morphogenic transition has been studied by Sabir et al. (2013). In vitro tissues belongs to different stages of organogenesis (rhizogenesis and shoot organogenesis) were used for the experiment. Accumulation of major withanolides and expression of HMGR, FPP synthase (FPPS), SQS, SQE, cycloaretenol synthase (CAS), GTs were analyzed at different morphogenic transition states.
Detailed study on four-CYP450 has been performed by Srivastava et al. (2015) to illustrate involvement of these enzyme in some specialized secondary metabolite (withanolides). The expression profiles of these CYPs showed chemotype-specific and tissue-specific variation, as well as variation in response to physiological and developmental factors. To expand the understanding of expression of genes in relation to withanolide biosynthetic pathway, Pal et al. (2016) perform experiments with different concentrations of fertilizers on fresh twigs of W. somnifera. Treated twigs related to highest accumulation of withaferin-A has been selected to analyse expression pattern of CYPs, allene oxide cyclases (AOCs) and few other pathway related genes.
Numerous, campylotropous, whitish, disk shaped seeds are found inside red or orange colored fruit (berry) of W. somnifera. Earlier reports mentioned high dormancy with poor seed viability (Khanna et al., 2013; Viji et al., 2013), also seeds of W. somnifera showed low and erratic germination with heterogeneous seedlings (Vashistha et al., 2010) having higher mortality rate of seedlings under field conditions (Khanna et al., 2013). The problems with seed germination of W. somnifera (in vitro and in field) guided the researchers toward finding of simple techniques with optimized conditions, in order to get faster and more germination rate. These conditions include nutrient medium, light conditions and condition of seeds, etc. These findings will help nursery workers and poor farmers interested in developing mass planting stock.
Soaking of seeds in water, diluted sodium hypochlorite, nitrate solutions (of potassium, ammonium, cobalt, sodium, calcium and zinc), has been suggested to soften the hard seed coat of W. somnifera (Kattimani and Reddy, 2001; Vashistha et al., 2010). Improved germination has been observed at 25 ± 2°C and 16-h-light/8-h-dark photoperiod with the light intensity of 3,000 lux (Kambizi et al., 2006; Khanna et al., 2013; Viji et al., 2013). In addition to these conditions, incision on seed coat and few pre-incubation conditions (dark or 15°C) increases the germination percentage (Pandey et al., 2013; Viji et al., 2013; Kumar et al., 2016).
Seedlings, embryos, cotyledon, epicotyl, hypocotyl, petiole, leaves, nodes, internodes, stem, shoot tips and roots have been used in different experiments for callus induction, adventitious root induction, regeneration, differentiation, flower induction, and fruit setting (Sharada et al., 2008; Supe et al., 2011; Singh et al., 2017). Composition of gelling matrix was standardized for encapsulation of shoot tips of W. somnifera along with optimization of media composition (or soilrite) for conversion of encapsulated shoot tips into plantlets (Singh et al., 2006). Most studies with optimized in vitro tissue culture conditions of W. somnifera have been briefly summarized recently by Singh et al. (2017).
The ultimate goal of different studies on W. somnifera is to provide maximum and better plant material for therapeutic purpose. These involes standardization of phytochemical analysis of different types of tissues obtained from different region and accession of W. somnifera, for accumulation of therapeutic metabolites (Table 1). On the basis of difference in available withanolides, W. somnifera has been divided into various chemotypes (accessions). Differences in chemo-profile of some selected chemotypes have been documented in several studies (Dhar et al., 2006; Kumar et al., 2007; Scartezzini et al., 2007; Bhatia et al., 2013).
Table 1. Different conditions/situation in order to accumulate therapeutically important metabolites of W. somnifera.
Variation persist in accumulation of withanolides due to plant parts, developmental stages (Praveen and Murthy, 2010; Dhar et al., 2013), plant part obtained from different types of cultures (Sharada et al., 2007; Singh et al., 2017) of W. somnifera. These studies establish relationship between morphology/condition of plant tissue and withanolide contents. Sivanandhan et al., 2012a,b, 2013b,c, 2014a,b, 2015a; Singh et al., 2017) used in vitro grown plants in different studies to develop adventitious roots, multiple shoots, shoot suspension culture, cell suspension culture, flowers, and fruits using different growth conditions. These developed tissues were harvested to extract different combinations of withanolides.
Based on different studies, Singh et al. (2017) summarized effects of in vitro conditions on accumulation of withanolides. These studies involving organ and callus culture, cell suspension culture and Agrobacterium tumefaciens as well as A. rhizogene mediated transformation. Different conditions of these techniques resulted in modulated accumulation of different withanolides, some of which related to modulated gene expression pattern.
Hairy root cultures are a promising approach of bioprocess engineering for large scale production of valuable plant secondary metabolites. There are several reports available in order to modulate quantity of withanolides in hairy roots culture using A. rhizogenes mediated transformation (Pawar and Maheshwari, 2004; Bandyopadhyay et al., 2007; Murthy et al., 2008; Saravanakumar et al., 2012; Sivanandhan et al., 2013a, 2015b). It has been reported that different factors like carbohydrates (Doma et al., 2012), inorganic supplements (Praveen and Murthy, 2013), seaweed extracts (Gracilaria edulis and Sargassum wightii; Sivanandhan et al., 2015b), hormones, elicitation (like, chitosan, JA, SA; Chaudhuri et al., 2009; Doma et al., 2012; Sivanandhan et al., 2013a), etc. modulate biogeneration of withanolides in hairy root cultures.
Difference in hairy root emergence was observed illustrating resistance or susceptibility of W. somnifera toward different strains of A. rhizogenes (Pawar and Maheshwari, 2004; Bandyopadhyay et al., 2007; Saravanakumar et al., 2012) as well as transformation efficiency of different explants used for the experiment (Murthy et al., 2008; Saravanakumar et al., 2012). Leaves proved to be more appropriate for infection by different strains of A. rhizogene, since used as explant in various studies (Ray et al., 1996; Bandyopadhyay et al., 2007; Chaudhuri et al., 2009; Doma et al., 2012; Saravanakumar et al., 2012; Praveen and Murthy, 2013; Sil et al., 2015; Thilip et al., 2015). Recenlty, Pandey et al. (2015) induced hairy root from leaf explants of W. somnifera expressing sterol glucosyltransferase gene (clone-4) using A. rhizogenes. The transgenic hairy roots were observed to accumulate higher amount of withanolide-A when subjected to elicitation (salicylic acid and methyl jasmonate).
Numerous studies have helped in developing efficient methods for regeneration of W. somnifera, while only few reports are available for genetic transformation for this medicinal plant (Singh et al., 2017). Altered expressions of genes related to biosynthetic pathway, ultimately modulate quantity of plant secondary metabolites, which are of therapeutic importance. Ray and Jha (1999) infected leaves of in vitro grown plants (two genotypes) with wild type nopaline and octopine strains of A. tumefaciens. Different types of galls obtained due to different levels of virulence on the two genotypes. Two principle withanolides, withanolide D and withaferin A extracted from shooty teratoma cultures in higher amount, while, withanolide D alone was detected in rooty teratomas.
Pandey et al. (2010) performed successful A. tumefaciens mediated transformation with 1.67 efficiency using non-virulent strain. Leaves excised from 1-5-nodes of both in situ and in vitro grown 30 to 90-day-old seedlings of different accessions of W. somnifera were used for the study. LBA4404 containing the binary vector pIG121Hm showed more gus expression in second and third leaves of 75 day old seedlings. Leaf explants ultrasonicated at 47 KHz ± 6% for 10 s showed higher gus expression as compared to directly infected explants. The protocol was used to analyse in vivo enzymatic action of one SGT (WsSGTL1) of W. somnifera by Saema et al. (2015, 2016). RNAi silencing (Saema et al., 2015) as well as overexpression (Saema et al., 2016) of WsSGTL1 gene has been achieved in transgenic W. somnifera. As expected, reduction in the level of glycosylated products observed in transgenic with silenced WsSGTL1 transcript. However, transgenics with overexpressing WsSGTL1 showed early and enhanced growth, increased production of glycosterols, and glycowithanolides. These transgenics displayed biotic (Spodoptera litura) and abiotic (cold) stress tolerance as well as recovery after cold stress along with improved photosynthetic performance.
Patel et al. (2014) established A. tumefaciens mediated transformation, microprojectile bombardment and microprojectile bombardment assisted agroinfection. Apical and nodal explants obtained from multiplied culture after in vitro seed germination were used as explants. Modified vector pCAMBIA1301 used to confirm transgene expression. Pre-cultured explants were bombarded and immediately infected with A. tumefaciens for microprojectile bombardment assisted agroinfection. The transformation efficiencies achieved were 3.86, 3.62, and 8.71%, through A. tumefaciens mediated, microprojectile bombardment and with the combination of both, respectively.
The protocol (Patel et al., 2014) used to overexpress of WsSQS in W. somnifera (Patel et al., 2015). Grover et al. (2013) also transformed leaves and shoots of 4-6-weeks old seedlings with A. tumefaciens (GV3101 harboring pBI121H) containing SQS from W. somnifera. Transgenics were confirmed with enhance expression of WsSQS transcript and its enzymatic activity. Higher amount of different withanolides observed in transgenics to proove the involvement of SQS with enhanced withanolide biosynthesis.
Nodal explants of 3-month old filed grown plants were used to develop transformation protocol of W. somnifera by Sivanandhan et al. (2015a) with 10% efficiency. These explant were found as an ideal tissue for the production of higher number of multiple shoots, hence adopted for the production transgenics. Explants were precultured (6-days) to obtain maximum transformation efficiency using Agrobacterium suspension (strain LBA4404 harboring pCAMIBA2301) at 0.2 OD600. The transformation frequency increased significantly with wounded nodal explants subjected to a sonication (10 s, longer treatment affected the viability of regenerating cells). Maximum transformation efficiency of 10.6% was observed by Mishra et al. (2015) using nodal explants infected with A. tumefaciens strain GV3101 harboring pIG121Hm. Explants were pre-cultured on MS supplemented with TDZ for 2 days and infected with Agrobacterium (0.2 OD600) for 20 min and co-cultivated for 48 h at 22°C.
Virus induced gene silencing methods was adopted by several researchers to achive fast and efficient characterization of genes related to withanolide biosynthesis. Using this technology, successful silencing of SQS (Singh et al., 2015a), WsDWF-5 (Gupta et al., 2015) and three-WsSGTLs genes (Singh et al., 2016) were achived in W. somnifera. Ws-SQS silenced plants revealed positive and negative affects on expression of upstream asnd downstream pathway genes, which ultimately reduces the accumulation of phytosterols. Silencincing of WsDWF-5 was observed with reduced accumulation of withanolide while, 3-WsSGTLs gene silencing found associated with enhanced level of different withanolides and reduced level of glycowithanolides. Increased expression of other upstream genes of withanolide biosynthesis pathway also relates with the supressed activity of WsSGTLs, which leads to reduced tolerance toward biotic stress.
W. somnifera is of great importance in lots of medical conditions due to abundance of diversified therapeutic secondary metabolites (withanolides). Significance of the plant leads researchers to identify the best suitable way to enhace plant productivity according to increasing demands. In order to complete the requirement, complete information related to metabolites, their biosynthesis (pathway genes/enzymes) and effect of different factors (composition of soil/media, elicitors etc.) is essential. Under the influence of significance of biosynthetic pathway, related genes/enzymes and external factors, this review describes all analyzed combinations of molecular and/or in vitro techniques that modifies the accumulation of desired metabolites. Several environmental factors like, soil/media composition, different types of elicitors/stresses etc. affect the withanolide biosynthesis by regulation of gene expression pattern. A lot of investigations included in this review that analyse withanolide accumulation through different types of in vitro culture techniques, like, micropropagation, organogenesis, hairy root production etc. Combination of optimized in vitro techniques and information of pathway gene/enzyme are of great interest these days. Such combination of genetic transformation and optimized in vitro conditions provides much better productivity in terms of metabolite accumulation. The present review describes that there are a lot more combinations available and need to utilize in order to achieve best productivity, to make it easily accessible for the progress of medical industry.
VP and WA collected literature and wrote the manuscript, PM and NA critically evaluated the manuscript. All authors approved the manuscript.
The authors declare that the research was conducted in the absence of any commercial or financial relationships that could be construed as a potential conflict of interest.
Authors acknowledge Director, CSIR-National Botanical Research Institute, as well as Head of Division, Banaras Hindu University, for providing research facilities. VP is thankful to Dr. D S Kothari Post-Doctoral Fellowship. WA is thankful for UGC-MAN-JRF-SRF.
SA, salicylic acid; MeJA, methyl jasmonate; MI, mechanical injury; ABA, abscisic acid; JA, jasmonic acid; Ws, Withania somnifera; GA3, gibberellic acid; YE, yeast extract.
Ahuja, A., Kaur, D., Sharada, M., Kumar, A., Suri, K. A., and Dutt, P. (2009). Glycowithanolides accumulation in in vitro shoot cultures of Indian ginseng (Withania somnifera Dunal). Nat. Prod. Commun. 4, 479–482.
Akhtar, N., Gupta, P., Sangwan, N. S., Sangwan, R. S., and Trivedi, P. K. (2013). Cloning and functional characterization of 3-hydroxy-3-methylglutaryl coenzyme A reductase gene from Withania somnifera: an important medicinal plant. Protoplasma 250, 613–622. doi: 10.1007/s00709-012-0450-2
Alam, N., Hossain, M., Khalil, M. I., Moniruzzaman, M., Sulaiman, S. A., and Gan, S. H. (2011). High catechin concentrations detected in Withania somnifera (ashwagandha) by high performance liquid chromatography analysis. BMC Complement. Altern. Med. 11:65. doi: 10.1186/1472-6882-11-65
Bandyopadhyay, M., Jha, S., and Tepfer, D. (2007). Changes in morphological phenotypes and withanolide composition of Ri-transformed roots of Withania somnifera. Plant Cell Rep. 26, 599–609. doi: 10.1007/s00299-006-0260-0
Bharti, S. K., Bhatia, A., Tewari, S. K., Sidhu, O. P., and Roy, R. (2011). Application of HR-MAS NMR spectroscopy for studying chemotype variations of Withania somnifera (L.) Dunal. Magn. Reson. Chem. 49, 659–667. doi: 10.1002/mrc.2817
Bhatia, A., Bharti, S. K., Tewari, S. K., Sidhu, O. P., and Roy, R. (2013). Metabolic profiling for studying chemotype variations in Withania somnifera (L.) Dunal fruits using GC–MS and NMR spectroscopy. Phytochemistry 93, 105–115. doi: 10.1016/j.phytochem.2013.03.013
Bolleddula, J., Fitch, W., Vareed, S. K., and Nair, M. G. (2012). Identification of metabolites in Withania somnifera fruits by liquid chromatography and high-resolution mass spectrometry. Rapid Commun. Mass Spectrom. 26, 1277–1290. doi: 10.1002/rcm.6221
Chakraborty, N., Banerjee, D., Ghosh, M., Pradhan, P., Gupta, N. S., Acharya, K., et al. (2013). Influence of plant growth regulators on callus mediated regeneration and secondary metabolites synthesis in Withania somnifera (L.) Dunal. Physiol. Mol. Biol. Plants 19, 117–125. doi: 10.1007/s12298-012-0146-2
Chatterjee, S., Srivastava, S., Khalid, A., Singh, N., Sangwan, R. S., Sidhu, O. P., et al. (2010). Comprehensive metabolic fingerprinting of Withania somnifera leaf and root extracts. Phytochemistry 71, 1085–1094. doi: 10.1016/j.phytochem.2010.04.001
Chaturvedi, P., Mishra, M., Akhtar, N., Gupta, P., Mishra, P., and Tuli, R. (2012). Sterol glycosyltransferases-identification of members of gene family and their role in stress in Withania somnifera. Mol. Biol. Rep. 39, 9755–9764. doi: 10.1007/s11033-012-1841-3
Chaturvedi, P., Misra, P., and Tuli, R. (2011). Sterol glycosyltransferases–the enzymes that modify sterols. Appl. Biochem. Biotechnol. 165, 47–68. doi: 10.1007/s12010-011-9232-0
Chaudhuri, K., Das, S., Bandyopadhyay, M., Zalar, A., Kollmann, A., Jha, S., et al. (2009). Transgenic mimicry of pathogen attack stimulates growth and secondary metabolite accumulation. Transgenic Res. 18, 121–134. doi: 10.1007/s11248-008-9201-8
Chaurasiya, N. D., Gupta, V. K., and Sangwan, R. S. (2007). Leaf ontogenic phase-related dynamics of withaferin A and withanone biogenesis in Ashwagandha (Withania somnifera Dunal.) – An important medicinal herb. J. Plant Biol. 50, 508–513. doi: 10.1007/BF03030691
Chaurasiya, N. D., Sangwan, N. S., Sabir, F., Misra, L., and Sangwan, R. S. (2012). Withanolide biosynthesis recruits both mevalonate and DOXP pathways of isoprenogenesis in ashwagandha Withania somnifera L. (Dunal). Plant Cell Rep. 31, 1889–1897. doi: 10.1007/s00299-012-1302-4
Chaurasiya, N. D., Uniyal, G. C., Lal, P., Misra, L., Sangwan, N. S., Tuli, R., et al. (2008). Analysis of withanolides in root and leaf of Withania somnifera by HPLC with photodiode array and evaporative light scattering detection. Phytochem. Anal. 19, 148–154. doi: 10.1002/pca.1029
Choudhary, M. I., Yousuf, S., Nawaz, S. A., Ahmed, S., and Atta-ur-Rahman (2004). Cholinesterase inhibiting withanolides from Withania somnifera. Chem. Pharm. Bull. 52, 1358–1361. doi: 10.1248/cpb.52.1358
Dar, N. J., Hamid, A., and Ahmad, M. (2015). Pharmacologic overview of Withania somnifera, the Indian Ginseng. Cell. Mol. Life Sci. 72, 4445–4460. doi: 10.1007/s00018-015-2012-1
Dewir, Y. H., Chakrabarty, D., Lee, S. H., Hahn, E. J., and Paek, K. Y. (2010). Indirect regeneration of Withania somnifera and comparative analysis of withanolides in in vitro and greenhouse grown plants. Biol. Plantarum. 54, 357–360. doi: 10.1007/s10535-010-0063-6
Dhar, N., Rana, S., Bhat, W. W., Razdan, S., Pandith, S. A., Khan, S., et al. (2013). Dynamics of withanolide biosynthesis in relation to temporal expression pattern of metabolic genes in Withania somnifera (L.) Dunal, a comparative study in two morpho-chemovariants. Mol. Biol. Rep. 40, 7007–7016. doi: 10.1007/s11033-013-2820-z
Dhar, N., Rana, S., Razdan, S., Bhat, W. W., Hussain, A., Dhar, R. S., et al. (2014). Cloning and functional characterization of three branch point oxidosqualene cyclases from Withania somnifera (L.) dunal. J. Biol. Chem. 289, 17249–17267. doi: 10.1074/jbc.M114.571919
Dhar, N., Razdan, S., Rana, S., Bhat, W. W., Vishwakarma, R., and Lattoo, S. K. (2015). Decade of molecular understanding of withanolide biosynthesis and in vitro studies in Withania somnifera (L.) Dunal, prospects and perspectives for pathway engineering. Front. Plant Sci. 6:1031. doi: 10.3389/fpls.2015.01031
Dhar, R. S., Khan, S., Khajuria, R. K., and Bedi, Y. S. (2016). Dynamics of squalene content in different tissues of Ashwagandha (Withania somnifera L. Dunal) during its growth phases. Ind. Crops Prod. 84, 375–380. doi: 10.1016/j.indcrop.2016.02.003
Dhar, R. S., Verma, V., Suri, K. A., Sangwan, R. S., Satti, N. K., Kumar, A., et al. (2006). Phytochemical and genetic analysis in selected chemotypes of Withania somnifera. Phytochemistry 67, 2269–2276. doi: 10.1016/j.phytochem.2006.07.014
Doma, M., Abhayankar, G., Reddy, V. D., and Kavi Kishor, P. B. (2012). Carbohydrate and elicitor enhanced withanolide (withaferin A and withanolide A) accumulation in hairy root cultures of Withania somnifera (L.). Indian J. Exp. Biol. 50, 484–490.
Furmanowa, M., Gajdzis-Kuls, D., Ruszkowska, J., Czarnocki, Z., Obidoska, G., Sadowska, A., et al. (2001). In vitro propagation of Withania somnifera and isolation of withanolides with immunesuppressive activity. Planta Med. 67, 146–149. doi: 10.1055/s-2001-11494
Ganzera, M., Choudhary, M. I., and Khan, I. A. (2003). Quantitative HPLC analysis of withanolides in Withania somnifera. Fitoterapia 74, 68–76. doi: 10.1016/S0367-326X(02)00325-8
Grover, A., Samuel, G., Bisaria, V. S., and Sundar, D. (2013). Enhanced withanolide production by overexpression of squalene synthase in Withania somnifera. J. Biosci. Bioeng. 115, 680–685. doi: 10.1016/j.jbiosc.2012.12.011
Gupta, M., Srivastava, P. K., Shikha, N. A., and Tewari, S. K. (2016). Use of a bioaugmented organic soil amendment in combination with gypsum for Withania somnifera growth on sodic soil. Pedosphere 26, 299–309. doi: 10.1016/S1002-0160(15)60044-3
Gupta, N., Sharma, P., Santosh Kumar, R. J., Vishwakarma, R. K., and Khan, B. M. (2012). Functional characterization and differential expression studies of squalene synthase from Withania somnifera. Mol. Biol. Rep. 39, 8803–8812. doi: 10.1007/s11033-012-1743-4
Gupta, P., Agarwal, A. V., Akhtar, N., Sangwan, R. S., Singh, S. P., and Trivedi, P. K. (2013c). Cloning and characterization of 2-C-methyl-D-erythritol-4-phosphate pathway genes for isoprenoid biosynthesis from Indian ginseng, Withania somnifera. Protoplasma 250, 285–295. doi: 10.1007/s00709-012-0410-x
Gupta, P., Agarwal, A. V., Akhtar, N., Sangwan, R. S., Singh, S. P., and Trivedi, P. K. (2013a). Cloning and characterization of 2-C-methyl-D-erythritol-4-phosphate pathway genes for isoprenoid biosynthesis from Indian ginseng, Withania somnifera. Protoplasma 250, 285–295. doi: 10.1007/s00709-012-0410-x
Gupta, P., Akhtar, N., Tewari, S. K., Sangwan, R. S., and Trivedi, P. K. (2011). Differential expression of farnesyl diphosphate synthase gene from Withania somnifera in different chemotypes and in response to elicitors. Plant Growth Regul. 65, 93–100. doi: 10.1007/s10725-011-9578-x
Gupta, P., Goel, R., Agarwal, A. V., Asif, M. H., Sangwan, N. S., Sangwan, R. S., et al. (2015). Comparative transcriptome analysis of different chemotypes elucidates withanolide biosynthesis pathway from medicinal plant Withania somnifera. Sci. Rep. 5:18611. doi: 10.1038/srep18611
Gupta, P., Goel, R., Pathak, S., Srivastava, A., Singh, S. P., Sangwan, R. S., et al. (2013b). De novo assembly, functional annotation and comparative analysis of Withania somnifera leaf and root transcriptomes to identify putative genes involved in the withanolides biosynthesis. PLoS ONE 8:e62714. doi: 10.1371/journal.pone.0062714
Johny, L., Conlan, X., Cahill, D., and Adholeya, A. (2015). In vitro and in situ screening systems for morphological and phytochemical analysis of Withania somnifera germplasms. Plant Cell Tissue Organ Cult. 120, 1191–1202. doi: 10.1007/s11240-014-0673-3
Kambizi, L., Adebola, P. O., and Afolayan, A. J. (2006). Effects of temperature, pre-chilling and light on seed germination of Withania somnifera; a high value medicinal plant. South Afr. J. Bot. 72, 11–14. doi: 10.1016/j.sajb.2005.03.001
Kattimani, K. N., and Reddy, Y. N. (2001). A note on influence of pre-sowing seed treatments on growth and root yield of Ashwagandha. Karnataka J. Agri. Sci. 14, 846–848.
Khanna, P. K., Kumar, A., Chandra, R., and Verma, V. (2013). Germination behaviour of seeds of Withania somnifera (L.) Dunal, a high value medicinal plant. Physiol. Mol. Biol. Plants 19, 449–454. doi: 10.1007/s12298-013-0169-3
Kulkarni, S. K., and Dhir, A. (2008). Withania somnifera: an Indian ginseng. Prog. Neuropsychopharmacol. Biol. Psychiatry 32, 1093–1105. doi: 10.1016/j.pnpbp.2007.09.011
Kumar, A., and Kalonia, H. (2007). Protective effect of Withania somnifera Dunal on the behavioral and biochemical alterations in sleep-disturbed mice (Grid over water suspended method). Indian J. Exp. Biol. 45, 524–528.
Kumar, A., Kaul, M. K., Bhan, M. K., Khanna, P. K., and Suri, K. A. (2007). Morphological and chemical variation in 25 collections of the Indian medicinal plant, Withania somnifera (L.) Dunal (Solanaceae). Genet. Res. Crop Evol. 54, 655–660. doi: 10.1007/s10722-006-9129-x
Kumar, B., Yadav, R., Singh, S. C., and Singh, H. P. (2016). Seed germination behaviour of Withania spp. under different temperature regimes. J. Crop Improv. 30, 287–292. doi: 10.1080/15427528.2016.1151849
Kumar, M. S., Vinothkumar, D., Kumar, S., Aslam, A., and Shajahan, A. (2011). The phytochemical constituents of Withania somnifera and Withania obtusifolia by GCMS analysis. Int. J. Pharm. Phytochem. Res. 3, 31–34.
Madina, B. R., Sharma, L. K., Chaturvedi, P., Sangwan, R. S., and Tuli, R. (2007a). Purification and physico-kinetic characterization of 3beta-hydroxy specific sterol glucosyltransferase from Withania somnifera (L) and its stress response. Biochim. Biophys. Acta 1774, 392–402. doi: 10.1016/j.bbapap.2006.12.009
Madina, B. R., Sharma, L. K., Chaturvedi, P., Sangwan, R. S., and Tuli, R. (2007b). Purification and characterization of a novel glucosyltransferase specific to 27beta-hydroxy steroidal lactones from Withania somnifera and its role in stress responses. Biochim. Biophys. Acta 1774, 1199–1207. doi: 10.1016/j.bbapap.2007.06.015
Mirjalili, M. H., Moyano, E., Bonfill, M., Cusido, R. M., and Palazón, J. (2009). Steroidal lactones from Withania somnifera, an ancient plant for novel medicine. Molecules 14, 2373–2393. doi: 10.3390/molecules14072373
Mishra, S., Bansal, S., Mishra, B., Sangwan, R. S., Asha Jadaun, J. S., and Sangwan, N. S. (2016). RNAi and homologous over-expression based functional approaches reveal Triterpenoid Synthase Gene-Cycloartenol Synthase is involved in downstream Withanolide Biosynthesis in Withania somnifera. PLoS ONE 11:e0149691. doi: 10.1371/journal.pone.0149691
Mishra, S., Bansal, S., Sangwan, R. S., and Sangwan, N. S. (2015). Genotype independent and efficient Agrobacterium-mediated genetic transformation of the medicinal plant Withania somnifera Dunal. J. Plant Biochem. Biotechnol. 25, 191–198. doi: 10.1007/s13562-015-0324-8
Misra, L., Lal, P., Sangwan, R. S., Sangwan, N. S., Uniyal, G. C., and Tuli, R. (2005). Unusually sulfated and oxygenated steroids from Withania somnifera. Phytochemistry 66, 2702–2707. doi: 10.1016/j.phytochem.2005.10.001
Misra, L., Mishra, P., Pandey, A., Sangwan, R. S., Sangwan, N. S., and Tuli, R. (2008). Withanolides from Withania somnifera roots. Phytochemistry 69, 1000–1004. doi: 10.1016/j.phytochem.2007.10.024
Murthy, H. N., and Praveen, N. (2013). Carbon sources and medium pH affects the growth of Withania somnifera (L.) Dunal adventitious roots and withanolide A production. Nat. Prod. Res. 27, 185–189. doi: 10.1080/14786419.2012.660691
Murthy, H. N., Dijkstra, C., Anthony, P., White, D. A., Davey, M. R., Power, J. B., et al. (2008). Establishment of Withania somnifera hairy root cultures for the production of withanolide A. J. Integr. Plant Biol. 50, 975–981. doi: 10.1111/j.1744-7909.2008.00680.x
Namdeo, A. G., Sharma, A., Yadav, K. N., Gawande, R., Mahadik, K. R., Lopez-Gresa, M. P., et al. (2011). Metabolic characterization of Withania somnifera from different regions of India using NMR spectroscopy. Planta Med. 77, 1958–1964. doi: 10.1055/s-0031-1279997
Pal, S., Yadav, A. K., Singh, A. K., Rastogi, S., Gupta, M. M., Verma, R. K., et al. (2016). Nitrogen treatment enhances sterols and withaferin A through transcriptional activation of jasmonate pathway, WRKY transcription factors, and biosynthesis genes in Withania somnifera (L.) Dunal. Protoplasma 254, 389–399. doi: 10.1007/s00709-016-0959-x
Pandey, V., Mishra, M. K., Atri, N., and Misra, P. (2013). In vitro-seed germination of different chemotypes of Withania somnifera. Int. J. Tech. Res. Appl. 1, 1–6.
Pandey, V., Misra, P., Chaturvedi, P., Mishra, M. K., Trivedi, P. K., and Tuli, R. (2010). Agrobacterium tumefaciens-mediated transformation of Withania somnifera (L.) Dunal, an important medicinal plant. Plant Cell Rep. 29, 133–141. doi: 10.1007/s00299-009-0805-0
Pandey, V., Srivastava, R., Akhtar, N., Mishra, J., Mishra, P., and Verma, P. C. (2015). Expression of Withania somnifera steroidal glucosyltransferase gene enhances withanolide content in hairy roots. Plant Mol. Biol. Rep. 34, 681–689. doi: 10.1007/s11105-015-0955-x
Patel, N., Patel, P., Kendurkar, S. V., Thulasiram, H. V., and Khan, B. M. (2015). Overexpression of squalene synthase in Withania somnifera leads to enhanced withanolide biosynthesis. Plant Cell Tissue Organ Cult. 122, 409–420. doi: 10.1007/s11240-015-0778-3
Patel, N., Patel, P., Kumari, U., Kendurkar, S. V., and Khan, B. M. (2014). Microprojectile bombardment assisted agroinfection increases transformation efficiency of Withania somnifera (L.). Res. Biotechnol. 5, 13–24.
Pawar, P. K., and Maheshwari, V. L. (2004). Agrobacterium rhizogenes mediated hairy root induction in two medicinally important members of family Solanaceae. Indian J. Biotechnol. 3, 414–417.
Praveen, N., and Murthy, H. N. (2010). Production of withanolide-A from adventitious root cultures of Withania somnifera. Acta Physiol. Plant 32, 1017–1022. doi: 10.1007/s11738-010-0489-7
Praveen, N., and Murthy, H. N. (2013). Withanolide A production from Withania somnifera hairy root cultures with improved growth by altering the concentrations of macro elements and nitrogen source in the medium. Acta Physiol. Plant. 35, 811–816. doi: 10.1007/s11738-012-1125-5
Praveen, N., Naik, P. M., Manohar, S. H., and Murthy, H. N. (2010). Distribution of withanolides A content in various organs of Withania somnifera (L.) Dunal. Int. J. Pharma Bio Sci. 1, ISSN: 0975–6299.
Raja, G., and Veerakumari, L. (2013). Influence of vermicomposts on the yield and alkaloid content of Withania somnifera. Dunal. Int. J. Adv. Biol. Res. 3, 223–226.
Rana, S., Bhat, W. W., Dhar, N., Pandith, S. A., Razdan, S., Vishwakarma, R., et al. (2014). Molecular characterization of two A-type P450s, WsCYP98A and WsCYP76A from Withania somnifera (L.) Dunal: expression analysis and withanolide accumulation in response to exogenous elicitations. BMC Biotechnol. 14:89. doi: 10.1186/s12896-014-0089-5
Rana, S., Lattoo, S. K., Dhar, N., Razdan, S., Bhat, W. W., Dhar, R. S., et al. (2013). NADPH-cytochrome P450 reductase: molecular cloning and functional characterization of two paralogs from Withania somnifera (L.) dunal. PLoS ONE 8:e57068. doi: 10.1371/journal.pone.0057068
Ray, S., and Jha, S. (1999). Withanolide synthesis in cultures of Withania somnifera transformed with Agrobacterium tumefaciens. Plant Sci. 146, 1–7. doi: 10.1016/S0168-9452(99)00077-1
Ray, S., Ghosh, B., Sen, S., and Jha, S. (1996). Withanolide production by root culture of Withania somnifera transformed with Agrobacterium rhizogenes. Planta Med. 62, 571–573. doi: 10.1055/s-2006-957977
Razdan, S., Bhat, W. W., Dhar, N., Rana, S., Pandith, S. A., Wani, T. A., et al. (2016). Molecular characterization of DWF1 from Withania somnifera (L.) Dunal: its implications in withanolide biosynthesis. J. Plant Biochem. Biotechnol. 26, 52–63. doi: 10.1007/s13562-016-0359-5
Razdan, S., Bhat, W. W., Rana, S., Dhar, N., Lattoo, S. K., Dhar, R. S., et al. (2013). Molecular characterization and promoter analysis of squalene epoxidase gene from Withania somnifera (L.) Dunal. Mol. Biol. Rep. 40, 905–916. doi: 10.1007/s11033-012-2131-9
Sabir, F., Mishra, S., Sangwan, R. S., Jadaun, J. S., and Sangwan, N. S. (2013). Qualitative and quantitative variations in withanolides and expression of some pathway genes during different stages of morphogenesis in Withania somnifera Dunal. Protoplasma 250, 539–549. doi: 10.1007/s00709-012-0438-y
Saema, S., Rahman, L. U., Niranjan, A., Ahmad, I. Z., and Misra, P. (2015). RNAi-mediated gene silencing of WsSGTL1 in W. somnifera affects growth and glycosylation pattern. Plant Signal. Behav. 10:e1078064. doi: 10.1080/15592324.2015.1078064
Saema, S., Rahman, L. U., Singh, R., Niranjan, A., Ahmad, I. Z., and Misra, P. (2016). Ectopic overexpression of WsSGTL1, a sterol glucosyltransferase gene in Withania somnifera, promotes growth, enhances glycowithanolide and provides tolerance to abiotic and biotic stresses. Plant Cell Rep. 35, 195–211. doi: 10.1007/s00299-015-1879-5
Sangwan, R. S., Chaurasiya, N. D., Lal, P., Misra, L., Uniyal, G. C., Tuli, R., et al. (2007). Withanolide A biogeneration in in vitro shoot cultures of ashwagandha (Withania somnifera DUNAL), a main medicinal plant in Ayurveda. Chem. Pharm. Bull. 55, 1371–1375. doi: 10.1248/cpb.55.1371
Saravanakumar, A., Aslam, A., and Shajahan, A. (2012). Development and optimization of hairy root culture systems in Withania somnifera (L.) Dunal for withaferin-A production. Afr. J. Biotechnol. 11, 16412–16420. doi: 10.5897/AJB11.3867
Scartezzini, P., and Speroni, E. (2000). Review on some plants of Indian traditional medicine with antioxidant activity. J. Ethnopharmacol. 71, 23–43. doi: 10.1016/S0378-8741(00)00213-0
Scartezzini, P., Antognoni, F., Conte, L., Maxia, A., Troìa, A., and Poli, F. (2007). Genetic and phytochemical difference between some Indian and Italian plants of Withania somnifera (L.) Dunal. Nat. Prod. Res. 21, 923–932. doi: 10.1080/14786410701500169
Senthil, K., Jayakodi, M., Thirugnanasambantham, P., Lee, S. C., Duraisamy, P., Purushotham, P. M., et al. (2015). Transcriptome analysis reveals in vitro cultured Withania somnifera leaf and root tissues as a promising source for targeted withanolide biosynthesis. BMC Genomics 16:14. doi: 10.1186/s12864-015-1214-0
Senthil, K., Wasnik, N. G., Kim, Y. J., and Yang, D. C. (2010). Generation and analysis of expressed sequence tags from leaf and root of Withania somnifera (Ashwgandha). Mol. Biol. Rep. 37, 893–902. doi: 10.1007/s11033-009-9696-y
Sharada, M., Ahuja, A., and Vij, S. P. (2008). “Application of biotechnology in Indian ginseng (ashwagandha): progress and prospects,” in Recent Advances in Plant Biotechnology and Its Applications: Prof. Dr. Karl-Hermann Neumann, eds A. Kumar and S. K. Sopory (New Delhi: IK International Pvt Ltd), 560–582.
Sharada, M., Ahuja, A., Suri, K. A., Vij, S. P., Khajuria, R. K., Verma, V., et al. (2007). Withanolide production by in vitro cultures of Withania somnifera and its association with differentiation. Biol. Plant. 51, 161–164. doi: 10.1007/s10535-007-0031-y
Sharma, L. K., Madina, B. R., Chaturvedi, P., Sangwan, R. S., and Tuli, R. (2007). Molecular cloning and characterization of one member of 3beta-hydroxy sterol glucosyltransferase gene family in Withania somnifera. Arch. Biochem. Biophys. 460, 48–55. doi: 10.1016/j.abb.2007.01.024
Sidhu, O. P., Annarao, S., Chatterjee, S., Tuli, R., Roy, R., and Khetrapal, C. L. (2011). Metabolic alterations of Withania somnifera (L.) Dunal fruits at different developmental stages by NMR spectroscop Ahlawat y. Phytochem. Anal. 22, 492–502. doi: 10.1002/pca.1307
Sil, B., Mukherjee, C., Jha, S., and Mitra, A. (2015). Metabolic shift from withasteroid formation to phenylpropanoid accumulation in cryptogein-cotransformed hairy roots of Withania somnifera (L.) Dunal. Protoplasma 252, 1097–1110. doi: 10.1007/s00709-014-0743-8
Singh, A. K., Dwivedi, V., Rai, A., Pal, S., Reddy, S. G., Rao, D. K., et al. (2015a). Virus-induced gene silencing of Withania somnifera squalene synthase negatively regulates sterol and defence-related genes resulting in reduced withanolides and biotic stress tolerance. Plant Biotechnol. J. 13, 1287–1299. doi: 10.1111/pbi.12347
Singh, A. K., Varshney, R., Sharma, M., Agarwal, S. S., and Bansal, K. C. (2006). Regeneration of plants from alginate-encapsulated shoot tips of Withania somnifera (L.) Dunal, a medicinally important plant species. J. Plant Physiol. 163, 220–223. doi: 10.1016/j.jplph.2005.06.013
Singh, G., Sharma, P. K., Dudhe, R., and Singh, S. (2010). Biological activities of Withania somnifera. Ann. Biol. Res. 1, 56–63.
Singh, G., Tiwari, M., Singh, S. P., Singh, S., Trivedi, P. K., and Misra, P. (2016). Silencing of sterol glycosyltransferases modulates the withanolide biosynthesis and leads to compromised basal immunity of Withania somnifera. Sci. Rep. 6:25562. doi: 10.1038/srep25562
Singh, P., Guleri, R., Angurala, A., Kaur, K., Kaur, K., Kaul, S. C., et al. (2017). Addressing challenges to enhance the bioactives of Withania somnifera through organ, tissue, and cell culture based approaches. Biomed Res. Int. 2017:3278494. doi: 10.1155/2017/3278494
Singh, P., Guleri, R., Singh, V., Kaur, G., Kataria, H., Singh, B., et al. (2015b). Biotechnological interventions in Withania somnifera (L.) Dunal. Biotechnol. Genet. Eng. Rev. 19, 1–20. doi: 10.1080/02648725.2015.1020467
Singh, S., Rishi, K., Vishwakarma, R. J., Kumar, S., Sonawane, P. D., and Ruby, K. B. M. (2013). Functional characterization of a flavonoid glycosyltransferase gene from Withania somnifera (Ashwagandha). Appl. Biochem. Biotechnol. 170, 729–741. doi: 10.1007/s12010-013-0230-2
Sivanandhan, G., Arun, M., Mayavan, S., Rajesh, M., Jeyaraj, M., Dev, G. K., et al. (2012a). Optimization of elicitation conditions with methyl jasmonate and salicylic acid to improve the productivity of withanolides in the adventitious root culture of Withania somnifera (L.) Dunal. Appl. Biochem. Biotechnol. 168, 681–696. doi: 10.1007/s12010-012-9809-2
Sivanandhan, G., Arun, M., Mayavan, S., Rajesh, M., Mariashibu, T. S., Manickavasagam, M., et al. (2012b). Chitosan enhances withanolides production in adventitious root cultures of Withania somnifera (L.) Dunal. Ind. Crops Prod. 37, 124–129. doi: 10.1016/j.indcrop.2011.11.022
Sivanandhan, G., Arunachalam, C., Selvaraj, N., Sulaiman, A. A., Lim, Y. P., and Ganapathi, A. (2015b). Expression of important pathway genes involved in withanolides biosynthesis in hairy root culture of Withania somnifera upon treatment with Gracilaria edulis and Sargassum wightii. Plant Physiol. Biochem. 91, 61–64. doi: 10.1016/j.plaphy.2015.04.007
Sivanandhan, G., Dev, G. K., Jeyaraj, M., Rajesh, M., Arjunan, A., Muthuselvam, M., et al. (2013a). Increased production of withanolide A, withanone, and withaferin A in hairy root cultures of Withania somnifera (L.) Dunal elicited with methyl jasmonate and salicylic acid. Plant Cell Tissue Organ Cult. 114, 121–129. doi: 10.1007/s11240-013-0297-z
Sivanandhan, G., Dev, G. K., Jeyaraj, M., Rajesh, M., Muthuselvam, M., Selvaraj, N., et al. (2013b). A promising approach on biomass accumulation and withanolides production in cell suspension culture of Withania somnifera (L.) Dunal. Protoplasma 250, 885–898. doi: 10.1007/s00709-012-0471-x
Sivanandhan, G., Kapil Dev, G., Theboral, J., Selvaraj, N., Ganapathi, A., and Manickavasagam, M. (2015a). Sonication, vacuum infiltration and thiol compounds enhance the Agrobacterium-mediated transformation frequency of Withania somnifera (L.) Dunal. PLoS ONE 10:e0124693. doi: 10.1371/journal.pone.0124693
Sivanandhan, G., Rajesh, M., Arun, M., Jeyaraj, M., Dev, G. K., Arjunan, A., et al. (2013c). Effect of culture conditions, cytokinins, methyl jasmonate and salicylic acid on the biomass accumulation and production of withanolides in multiple shoot culture of Withania somnifera (L.) Dunal using liquid culture. Acta Physiol. Plant 35, 715–728. doi: 10.1007/s11738-012-1112-x
Sivanandhan, G., Selvaraj, N., Ganapathi, A., and Manickavasagam, M. (2014a). Enhanced biosynthesis of withanolides by elicitation and precursor feeding in cell suspension culture of Withania somnifera (L.) Dunal in shake-flask culture and bioreactor. PLoS ONE 9:e104005. doi: 10.1371/journal.pone.0104005
Sivanandhan, G., Selvaraj, N., Ganapathi, A., and Manickavasagam, M. (2014b). Improved production of withanolides in shoot suspension culture of Withania somnifera (L.) Dunal by seaweed extracts. Plant Cell Tissue Organ Cult. 119, 221–225. doi: 10.1007/s11240-014-0521-5
Sivanandhan, G., Theboral, J., Dev, G. K., Selvaraj, N., Manickavasagam, M., and Ganapathi, A. (2015c). Effect of carbon and nitrogen sources on in vitro flower and fruit formation and withanolides production in Withania somnifera (L.) Dunal. Indian J. Exp. Biol. 53, 177–183.
Srivastava, P., Tiwari, N., Yadav, A. K., Kumar, V., Shanker, K., Verma, R. K., et al. (2008). Simultaneous quantification of withanolides in Withania somnifera by a validated high-performance thin-layer chromatographic method. J. AOAC Int. 91, 1154–1161.
Srivastava, S., Sangwan, R. S., Tripathi, S., Mishra, B., Narnoliya, L. K., Misra, L. N., et al. (2015). Light and auxin responsive cytochrome P450s from Withania somnifera Dunal: cloning, expression and molecular modelling of two pairs of homologue genes with differential regulation. Protoplasma 252, 1421–1437. doi: 10.1007/s00709-015-0766-9
Subbaraju, G. V., Vanisree, M., Rao, C. V., Sivaramakrishna, C., Sridhar, P., Jayaprakasam, B., et al. (2006). Ashwagandhanolide, a bioactive dimeric thiowithanolide isolated from the roots of Withania somnifera. J. Nat. Prod. 69, 1790–1792. doi: 10.1021/np060147p
Supe, U., Dhote, F., and Roymon, M. G. (2011). A review on micropropagation of Withania somnifera–A medicinal plant. J Agricult. Technol. 7, 1475–1483.
Thilip, C., Raju, C. S., Varutharaju, K., Aslam, A., and Shajahan, A. (2015). Improved Agrobacterium rhizogenes-mediated hairy root culture system of Withania somnifera (L.) Dunal using sonication and heat treatment. 3 Biotech 5, 949–956. doi: 10.1007/s13205-015-0297-2
Tuli, R., and Sangwan, R. S. (2009). Ashwagandha (Withania somnifera): A model Indian Medicinal Plant (1st Edn.). New Delhi: CSIR-National Botanical Research Institute.
Vashistha, R. K., Chaturvedi, A. K., Butola, J. S., and Nautiyal, M. C. (2010). Evaluation of emergence and vigour of Ashwagandha (Withania somnifera Dunal) seedlings under the influence of Sodium Hypochlorite (NaHClO). Biol. Forum- An Intl. J. 2, 30–34.
Viji, M. O., Mathew, M. M., and Parvatham, R. (2013). Effects of light intensity and imbibition frequency of in vivo and in vitro propagated seeds of Withania somnifera (L.) Poshita on germination. Int. J. Curr. Microbiol. App. Sci. 2, 354–360.
Keywords: Withania somnifera, Ashwagandha, metabolites, withanolides, tissue culture, differentiation, and transformation
Citation: Pandey V, Ansari WA, Misra P and Atri N (2017) Withania somnifera: Advances and Implementation of Molecular and Tissue Culture Techniques to Enhance Its Application. Front. Plant Sci. 8:1390. doi: 10.3389/fpls.2017.01390
Received: 06 March 2017; Accepted: 26 July 2017;
Published: 09 August 2017.
Edited by:
Chang-Jun Liu, Brookhaven National Laboratory, United StatesCopyright © 2017 Pandey, Ansari, Misra and Atri. This is an open-access article distributed under the terms of the Creative Commons Attribution License (CC BY). The use, distribution or reproduction in other forums is permitted, provided the original author(s) or licensor are credited and that the original publication in this journal is cited, in accordance with accepted academic practice. No use, distribution or reproduction is permitted which does not comply with these terms.
*Correspondence: Pratibha Misra, cHJhdGliaGFmbG9yYUB5YWhvby5jb20=
Neelam Atri, bmVlbGFtbW12MTRAZ21haWwuY29t
Disclaimer: All claims expressed in this article are solely those of the authors and do not necessarily represent those of their affiliated organizations, or those of the publisher, the editors and the reviewers. Any product that may be evaluated in this article or claim that may be made by its manufacturer is not guaranteed or endorsed by the publisher.
Research integrity at Frontiers
Learn more about the work of our research integrity team to safeguard the quality of each article we publish.