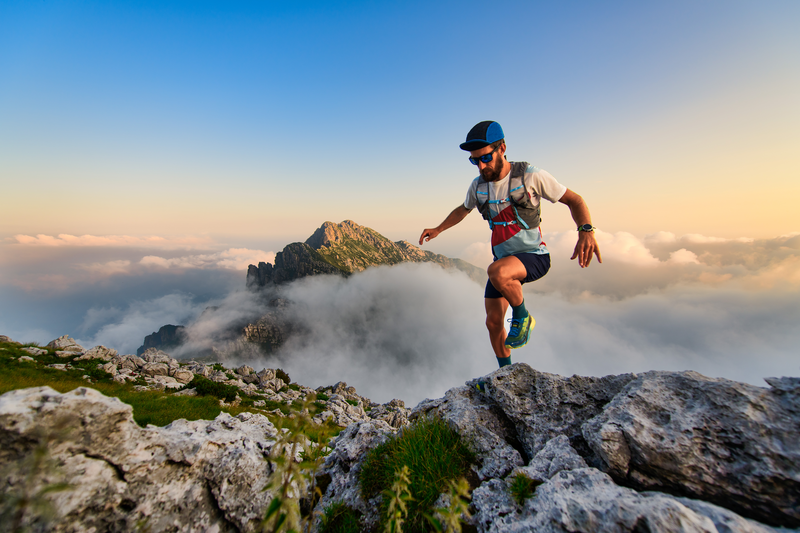
95% of researchers rate our articles as excellent or good
Learn more about the work of our research integrity team to safeguard the quality of each article we publish.
Find out more
ORIGINAL RESEARCH article
Front. Plant Sci. , 10 August 2017
Sec. Plant Abiotic Stress
Volume 8 - 2017 | https://doi.org/10.3389/fpls.2017.01372
Soybean is an important and staple oilseed crop worldwide. Salinity stress has adverse effects on soybean development periods, especially on seed germination and post-germinative growth. Improving seed germination and emergence will have positive effects under salt stress conditions on agricultural production. Here we report that NaCl delays soybean seed germination by negatively regulating gibberellin (GA) while positively mediating abscisic acid (ABA) biogenesis, which leads to a decrease in the GA/ABA ratio. This study suggests that fluridone (FLUN), an ABA biogenesis inhibitor, might be a potential plant growth regulator that can promote soybean seed germination under saline stress. Different soybean cultivars, which possessed distinct genetic backgrounds, showed a similar repressed phenotype during seed germination under exogenous NaCl application. Biochemical analysis revealed that NaCl treatment led to high MDA (malondialdehyde) level during germination and the post-germinative growth stages. Furthermore, catalase, superoxide dismutase, and peroxidase activities also changed after NaCl treatment. Subsequent quantitative Real-Time Polymerase Chain Reaction analysis showed that the transcription levels of ABA and GA biogenesis and signaling genes were altered after NaCl treatment. In line with this, phytohormone measurement also revealed that NaCl considerably down-regulated active GA1, GA3, and GA4 levels, whereas the ABA content was up-regulated; and therefore ratios, such as GA1/ABA, GA3/ABA, and GA4/ABA, are decreased. Consistent with the hormonal quantification, FLUN partially rescued the delayed-germination phenotype caused by NaCl-treatment. Altogether, these results demonstrate that NaCl stress inhibits soybean seed germination by decreasing the GA/ABA ratio, and that FLUN might be a potential plant growth regulator that could promote soybean seed germination under salinity stress.
Salinity is one of the most important abiotic stresses in the world. It has adverse effects on almost all development stages during the plant life-cycle, including seed germination, seedling establishment and development, vegetative and reproductive growth, and crop survival and yield (Zhu, 2016). More than 45 million hectares of agricultural land have been damaged by salt worldwide, and NaCl stress is an important salinity stress (Munns and Tester, 2008). Over the past few decades, numerous studies have demonstrated that high soil salinity content significantly affects crop growth and development through many diverse pathways, including water stress, nutritional disorders, ion toxicity, oxidative stress, alterations to metabolic processes, cell membrane disorganization, and reduced cell expansion and division (Hasegawa et al., 2000; Munns, 2002; Zhu, 2002, 2016; Teige et al., 2004; Hanin et al., 2016). Therefore, the physiological and molecular mechanisms through which plants adapt to salt stress need further investigating and the results could lead to improvements in agricultural production worldwide, especially on saline land.
Soybean (Glycine max (L.) Merrill) is an important and staple oil crop worldwide, and is used as a plant oil and protein resource by humans. However, salinity stress has significantly decreased soybean yield by inhibiting seed germination and post-germinative growth. Several key regulators, including GmSALT3, GmMYB76, GmMYB92, GmMYB177, GmbZIP132, and MiR172C, have been documented, and their related-mechanisms were investigated (Liao et al., 2008a,b; Guan et al., 2014; Wang et al., 2014; Kan et al., 2016; Li et al., 2016; Liu et al., 2016). High and uniform germination and emergence in the field are the key determinants of soybean yield, especially under salt stress conditions. Salinity represses soybean seed germination (Zhang et al., 2014; Kan et al., 2016), but the precise mechanisms underlying the inhibition effect of salt on soybean seed germination are only partially understood. Further, potential plant growth regulators need to be developed to promote soybean seed germination and emergence under salinity stress conditions.
Seed germination and post-germinative growth are critical stages during the plant life-cycle. The phytohormone abscisic acid (ABA) delays seed germination whereas gibberellin (GA) promotes this process, and both hormones are key regulators involved in seed germination processes (Finkelstein et al., 2008; Graeber et al., 2012; Nee et al., 2016; Shu et al., 2016b). Consistently, mutants with dysfunctional ABA and GA biosynthesis, and catabolic or signaling transduction pathways always show an altered germination phenotype. In Arabidopsis, the ABA biosynthesis mutants nced6, nced5, nced3, and aba2 (Seo et al., 2006; Frey et al., 2012), and ABA signaling mutants abi3 (Koornneef et al., 1989), abi4 (Shu et al., 2013), and abi5 (Piskurewicz et al., 2008) had a faster germination phenotype compared to wild type, whereas overexpression of Nicotiana ABA2 or Arabidopsis ABI4 resulted in a delayed-germination phenotype (Frey et al., 1999; Shu et al., 2013). However, the seeds of GA-deficiency mutants, ga1 and ga2, failed to germinate unless there was an exogenous supply of GA (Lee et al., 2002; Shu et al., 2013), while the mutants that are defective in GA2-oxidases (GA2ox), which deactivate bioactive GA, have a faster germination phenotype (Yamauchi et al., 2007). Further, the ratio between the levels of active GA/ABA is also a key determinant of seed germination (Shu et al., 2015, 2016b; Meng et al., 2016). Taken together, both ABA and GA levels, along with signaling play important roles in the regulation of seed germination.
Several abiotic stress conditions, including drought and salinity, lead to the up-regulation of ABA, which in turn elicits diverse adaptive responses in plants (Zhu, 2002, 2016). Osmotic stress–induced ABA accumulation is largely dependent on the activation of biosynthesis and the inhibition of degradation pathways (Zhu, 2002). Previous studies revealed that applying a salt treatment up-regulated the transcription levels of ZEP and NCED genes, which encode the key enzymes involved in the ABA biosynthesis pathway (Xiong et al., 2002; Galle et al., 2013). Furthermore, some ABA biosynthetic mutants such as aba2 seeds germinate earlier than the wild type under salt stress conditions (Gonzalez-Guzman et al., 2002), and ABA2 transcript can also be upregulated by prolonged salt treatments (Lin et al., 2007). On the other hand, salinity negatively regulates GA biosynthesis during the seed germination and seedling development stages (Shakirova et al., 2003; Horvath et al., 2015; Zhang et al., 2016). A recent study showed that ABA and GA biosynthesis were also involved in seed heteromorphism in Suaeda salsa under salt stress conditions (Li et al., 2015). However, the relationship between salt stress and GA still needs further investigation, especially during the soybean seed germination processes.
This study shows that exogenous NaCl treatment inhibits soybean seed germination by mediating the ABA and GA biogenesis pathways, and importantly, our evidences demonstrate that an ABA biogenesis inhibitor, fluridone (FLUN), might be a potential plant growth regulator that could be used to enhance soybean seed germination under salinity stress conditions. The quantitative Real-Time Polymerase Chain Reaction (qRT-PCR) analysis revealed that the transcription levels of ABA and GA biogenesis genes were altered by NaCl application. Consistently, further phytohormone quantification revealed that NaCl remarkably down-regulated active GA1, GA3, and GA4 levels, whereas ABA content was up-regulated; and consequently the ratios for GA/ABA (including GA1/ABA, GA3/ABA, and GA4/ABA) were significantly decreased. Taken together, it can be concluded that salt stress delays soybean seed germination by negatively regulating GA while positively mediating ABA biogenesis and that this consequently leads to a reduction in the GA/ABA ratios. Last but not least, FLUN can be used as a potential chemical to promote soybean seed germination and post-germinative growth under salinity stress conditions.
We first tested the effect of exogenous NaCl treatment on soybean seed germination. The germination analysis showed that NaCl delays the seed germination processes in cultivar ND-12 (Figures 1A,B). The germination rate of the NaCl-treated soybean seeds was about two to three-fold less than the control (CK) during the germination processes (Figure 1B). Consistently, the analysis of several post-germinative growth parameters, including the length of radicle (Figure 1E) and the fresh weights of germinated seeds (Figure 1F), also supported that the delayed-germination phenotype was caused by the NaCl treatment (Figures 1A,B). To further confirm the inhibition effect of NaCl on soybean seed germination processes, we tested another cultivar, HD-19, which is genetically distinct from the ND-12 cultivar. The results revealed that NaCl treatment also inhibited HD-19 seed germination processes (Figures 1C,D), and the post-germinative growth analysis showed that post-germinative growth was also reduced by NaCl (Figures 1G,H). Exogenous NaCl application had a remarkable inhibitory effect on radicle length and the fresh weights of germinated seeds (Figures 1G,H).
FIGURE 1. NaCl inhibits soybean seed germination. Healthy soybean seeds (cultivars ND12 and HD19) were incubated on two layers of filter paper in Petri dishes. The concentration of NaCl used was 150 mM, and the equivalent ultrapure water was employed as control (CK). (A) The representative images (36 h after sowing) are shown for cultivar ND12. (B) Quantitative analysis of germination rates is shown for ND12. (C) The representative images (36 h after sowing) are shown for cultivar HD19. (D) Quantitative analysis of germination rates is shown for HD19. (E,F) Radicle length and fresh weight of germinated soybean seeds were measured for cultivar ND12. (G,H) Radicle length and fresh weight of germinated soybean seeds were measured for cultivar HD19. Bar = 10 mm. The average percentages of four repeats ± standard error were shown. Student’s t-test assay was employed for statistical analysis, and ∗∗ difference is significant at the 0.01 level.
Subsequently, we also analyzed the germination rate of the black soybean cultivar, C-103. The results revealed that NaCl application inhibited C-103 seed germination processes (Supplementary Figures S1A,B), and significantly reduced the radicle length and fresh weights of germinated seeds (Supplementary Figures S1C,D), which was similar to the analysis of HD-19 and ND-12 cultivars. These results demonstrated that exogenous NaCl treatment delays soybean seed germination processes.
In order to test the cell oxidative status after treatment with exogenous NaCl, oxidative stress parameters, including malondialdehyde (MDA) content, and catalase (CAT), superoxide dismutase (SOD), and peroxidase (POD) activities, were investigated during the seed germination processes. The results show that after exogenous NaCl treatment, the MDA content significantly increased, especially from 72 h to 94 h after imbibition (Figure 2A), which suggested that, during soybean seed germination and post-germinative growth, NaCl treatment increased the toxic stress in cells and inhibited both processes. Furthermore, the CAT, SOD, and POD activities also increased after NaCl application (Figures 2B–D). The increase in those enzymes suggested that NaCl treatment caused oxidative stress in cells during seed germination.
FIGURE 2. Quantification of MDA (malondialdehyde) content, activities of superoxide dismutase (SOD), catalase (CAT), and peroxidase (POD) during soybean seed germination after NaCl application. (A) Effect of NaCl on change of MDA content during seed imbibition. (B–D) Activities of CAT, SOD, and POD after exogenous NaCl treatment during soybean seed germination. Soybean cultivar ND12 seeds were employed and the concentration of NaCl used was 150 mM. Student’s t-test assay was employed for statistical analysis, and ∗∗ difference is significant at the 0.01 level.
Previous studies have demonstrated that ABA and GA play key roles in seed germination (Graeber et al., 2012; Shu et al., 2016b). Therefore, we further investigated the missing links between the inhibitory effect of NaCl on soybean seed germination and the effects on the GA and ABA pathways. Consequently, we explored the transcription patterns of ABA and GA biogenesis and signaling genes in soybean seeds during the imbibition process using a time-course analysis. Then we further analyzed the ABA and active GA content during seed imbibition after NaCl treatment.
The qRT-PCR results showed that the transcript level of the ABA biosynthesis genes: GmNCED9, GmNCED5, and GmAAO, were significantly up-regulated by NaCl-treatment during seed imbibition compared to the CK (Figure 3A). The NaCl treatment enhanced GmNCED9 expression at 6 h after imbibition, whereas NaCl-induced GmNCED5 transcription was also detected at 3 and 6 h after sowing (Figure 3A). Higher GmAAO transcription levels were maintained, especially at 9 h after imbibition (Figure 3A). We next investigated the transcription levels of genes involved in the ABA signaling pathway. Figure 3B shows that the exogenous NaCl application considerably enhanced ABA signaling by increasing GmABI4 and GmABI5 transcription because GmABI4 and GmABI5 are key positive regulators in the ABA signaling pathway (Shu et al., 2016b). Taken together, the transcription pattern analysis revealed that ABA content and signaling were possibly enhanced by exogenous NaCl treatment during soybean seed germination.
FIGURE 3. Gene transcription pattern analysis during the course of seed imbibition. Soybean cultivar ND12 dry seeds and imbibed seeds (0, 3, 6, 9 h after imbibition) were used for total RNA extraction. Three biological replications were performed. (A) Abscisic acid (ABA) biosynthesis genes, GmNCED9, GmNCED5, and GmAAO. (B) ABA signaling positive regulator genes, GmABI5 and GmABI4. (C) Gibberellin (GA) biosynthesis genes, GmGA3ox1 and GmKAO. (D) GA inactivate gene, GmGA2ox8. (E) negative regulator gene in GA signaling transduction pathway, GmRGL. Three replications were performed and similar results were obtained. The concentration of NaCl used was 150 mM. Student’s t-test assay was employed for statistical analysis, and asterisks indicate statistically significant differences from CK at different time points (∗P < 0.05; ∗∗P < 0.01). Primer pairs of the marker genes are listed in Supplementary Table S1.
Subsequently, we further examined the expression pattern of the key genes involved in GA biogenesis and the signaling pathways. The qRT-PCR assay showed that after NaCl treatment the level of GA biosynthesis genes GmGA3ox1 and GmKAO decreased, especially at 9 h after sowing, compared to the CK (Figure 3C). On the other hand, GA inactive gene GmGA2ox8 expression increased during seed imbibition (Figure 3D). Furthermore, GmRGL transcription, a gene that belongs to the DELLA family and represses seed coat rupture during seed germination (Piskurewicz and Lopez-Molina, 2009), was up-regulated considerably during imbibition after NaCl-treatment (Figure 3E). Taken together, these analyses revealed that exogenous NaCl probably negatively regulated GA biogenesis and signaling during soybean seed germination.
The qRT-PCR data described above hinted us that NaCl may mediate ABA and GA biogenesis by regulating the expression of the metabolic genes for both phytohormones. Consequently, the endogenous ABA and active GA levels during imbibition were quantified. The results indicated that NaCl significantly decreased active GA1, GA3, and GA4 levels (Figures 4A–C). In contrast, the ABA content in NaCl-treated soybean seed during imbibition remarkably increased (Figure 4D), which meant that the GA1/ABA, GA3/ABA, and GA4/ABA ratios were down-regulated (Figure 4E). The active GA/ABA ratio is a key factor during seed germination (Finkelstein et al., 2008; Shu et al., 2016a,b). Therefore, the measurement of both ABA and GA supported the transcription analysis and the phenotype description. Altogether, all the results suggest that exogenous NaCl treatment positively regulates ABA biogenesis, but negatively mediates GA biosynthesis.
FIGURE 4. NaCl decreases the ratios between active GA and ABA, including GA1/ABA, GA3/ABA, and GA4/ABA. Soybean seeds were incubated at 25°C and treated with NaCl. Equivalent ultrapure water was added as control (CK). Dry seeds and 6-h imbibed seeds were used to determine the concentration of endogenous active GA1, GA3, and GA4. The results showed that NaCl treatment decreases active GA1 (A), GA3 (B), and GA4 (C), while ABA content was increased after NaCl application (D). The ratios of GA1/ABA, GA3/ABA, and GA4/ABA (E) were also shown. The concentration of NaCl used was 150 mM. Student’s t-test assay was employed for statistical analysis, and ∗ difference is significant at the 0.05 level, ∗∗ difference is significant at the 0.01 level.
To further confirm that NaCl enhances ABA biogenesis while impairs GA biogenesis during soybean seed germination, we next tested the responsiveness of soybean seeds to FLUN, an ABA biosynthesis inhibitor, and paclobutrazol (PAC), a GA biosynthesis inhibitor, during seed imbibition.
We also investigated the effect of the FLUN and PAC treatments on soybean seed germination. The results showed that FLUN promotes soybean seed germination, whereas PAC delays imbibition and germination (Figures 5A,B). The effects on post-germination growth parameters, such as radicle length (Figure 5C), were also similar to the germination results. The NaCl-treatment clearly promotes ABA biosynthesis during soybean seed germination, and FLUN blocks the ABA biosynthesis pathway. Therefore, we hypothesized that FLUN may be able to rescue the delayed-germination phenotype caused by enhanced ABA biogenesis. The germination analysis showed that FLUN could partially restore the delayed-germination phenotype seen when soybean seeds are treated with NaCl (Figures 6A,B). As shown in Figure 6B, although the seed germination rate of NaCl treatment was similar to NaCl+FLUN treatment within 24 h imbibition, the germination rate of NaCl+FLUN was higher than that of NaCl, but lower than that of CK between 36 to 60 h of imbibition (Figures 6B). This indicates that exogenous application of FLUN partially rescued salt stress inhibition of seed germination, which was also observed in Figure 6C based on radicle length. In other words, some other factors might be involved in salt stress-induced delay of seed germination (Figures 6C). On the other hand, we also found that NaCl+PAC mimics the inhibition effect caused by NaCl treatment (Figures 7A,B), and the post-germinative growth parameters further supported this (Figure 7C). Taken together, these results demonstrated that NaCl delays the soybean seed germination process by promoting ABA biosynthesis while impairing GA biosynthesis.
FIGURE 5. Gibberellin (GA) biogenesis inhibitor PAC, and ABA biogenesis inhibitor fluridone (FLUN) regulates soybean seed germination oppositely. (A,B) Soybean seeds (cultivar ND12) were incubated on two layers of filter paper in Petri dishes at 25°C and treated with 100 nM FLUN and10 μM PAC. Quantitative analysis of germination rates is shown in (B). Radicle length (C) of germinated seeds were measured under FLUN and PAC treatments and CK. The average length and fresh weight of four repeats are shown. Student’s t-test assay was employed for statistical analysis. ∗∗ Difference is significant at the 0.01 level, while ∗ difference is significant at the 0.05 level. Bar = 10 mm.
FIGURE 6. Fluridone partially rescues the repressed-germination phenotype resulting from NaCl stress. (A) and (B) Soybean cultivar ND12 seeds were incubated on two layers of filter paper in Petri dishes at 25°C and treated with 150 mM NaCl and 100 nM FLUN + 150 mM NaCl, respectively. Quantitative analysis of germination rates is shown in (B). The representative images (36 h after sowing) are shown (A). Radicle length of germinated seeds were measured (C). Student’s t-test assay was employed for statistical analysis. ∗∗ Difference is significant at the 0.01 level, while ∗ difference is significant at the 0.05 level. When performed the statistical analysis, the data of NaCl treatment were used as the baseline in (B), while the data of CK were used as baseline in (C). Bar = 10 mm.
FIGURE 7. The effect of PAC+NaCl combination treatment on soybean seed germination, Soybean cultivar ND12 seeds were used. (A–B) Soybean seeds were incubated on two layers of filter paper in Petri dishes at 25°C and treated with 150 mM NaCl and 100 nM PAC + 150 mM NaCl, respectively. Quantitative analysis of germination rates is shown in (B). The representative images (36 h after sowing) are shown (A). Radicle length of germinated seeds were measured (C). The CK data were used as baseline when performed the statistical analysis. Student’s t-test assay was employed for statistical analysis. ∗∗ Difference is significant at the 0.01 level, while ∗ difference is significant at the 0.05 level. Bar = 10 mm.
The large tracts of saline land that exist worldwide significantly constrain the development of sustainable agriculture, and on the other hand, they could also be used to improve food security after reclamation. However, the modification of salt-affected soils is very expensive and time-consuming. Consequently, increased plant salt resistance during different growth stages may provide a lower cost alternative for modern agriculture. Therefore, potential plant growth regulators should be explored further. In this study, we dissected the mechanisms through which salt repress soybean seed germination, and further showed that FLUN might be a potential plant growth regulator that could be used to promote soybean seed germination under salinity stress.
It is well-known that salt inhibits soybean seed germination and post-germinative growth, which eventually leads to decreases in yield (Hamayun et al., 2015). Previous investigations and the present study showed that NaCl treatment caused cellular oxidative stress (Figure 2; Liang et al., 2003; Zhu, 2016; Farhangi-Abriz and Torabian, 2017), and the level of cellular oxidative pressure is further associated with the activity of seed germination (Ma et al., 2017). In the past decade, soybean salt tolerance-related quantitative trait loci and some key proteins have been identified over the past few decades (Xu et al., 2011; Guan et al., 2014; Kan et al., 2015). However, the detailed molecular mechanisms underlying the inhibition-effect of salt on soybean seed germination and post-germinative growth are not fully understood, especially the relationship between salt effects and the ABA and GA pathways. In this study, the seed germination phenotype description (Figure 1 and Supplementary Figure S1), the biochemical analysis (Figure 2), the gene transcription pattern analysis (Figure 3), and the phytohormones measurement results (Figure 4) all demonstrated that NaCl stress positively regulated ABA biogenesis, but negatively mediated the GA biosynthesis pathways. This led to a decrease in the GA and ABA ratios (including GA1/ABA, GA3/ABA, and GA4/ABA). Importantly, we further have shown that FLUN might be a potential plant growth regulator that could promote soybean seed germination under salinity stress (Figure 6).
FLUN blocks ABA biosynthesis and disrupts plant photosynthesis (Stetsenko et al., 2015). It is also an aquatic herbicide that is usually used to control invasive plants (Gamble and Mullet, 1986; Sun et al., 2012). However, recent studies have revealed that FLUN is also involved in other processes, such as the regulation of plant drought stresses responses, plant development control, and even human disease control. It is well known that both heavy metals (including Cu2+, Cd2+, and Hg2+) and heat stress stop maize seed germination, but FLUN can significantly alleviate thermodormancy and rescue the delayed-germination phenotype caused by heavy metals (Deng et al., 2016). Furthermore, applying FLUN to tobacco leaves can promote NtEXGT expression and further increases plant drought resistance (Kuluev et al., 2017). It has also been recently demonstrated that FLUN stimulated Vellozia sp. seed germination in the dark (Vieira et al., 2017). Furthermore, FLUN has potential as a candidate drug for the treatment of acute neosporosis in vivo (Ybanez et al., 2016) and as a new anti-inflammatory drug (Magnone et al., 2013). These recent studies have demonstrated that FLUN possesses many different biological functions in both plants and animals.
Salinity inhibits soybean development across the whole life-cycle, which finally leads to decreased yields. Seed germination and emergence in the field are key crop yield determinants. However, large parts of the world are covered in saline soil, which significantly limits soybean seed germination and yield. Therefore, enhancing soybean seed germination under salt stress conditions will be of major benefit to agriculture worldwide. In this study, we showed that applying FLUN can rescue the delayed-germination phenotype caused by NaCl treatment. Consequently, FLUN can be a potential plant growth regulator that could be used to promote soybean seed germination under salt stress. Last but not least, it is noted that NaCl treatment caused oxidative stress in soybean seed cells (Figure 2), thus it is valuable to test whether and how FLUN enhances soybean seed germination under salt stress through activation of the cellular antioxidant systems.
Soybean cultivars employed in the present investigation were Hedou-19 (HD-19), Nandou-12 (ND-12) and C-103. HD-19 and ND-12 (yellow seed coat in color) and C-103 (black seed coat in color) are the prevailing soybean cultivars in Southwestern China, and are breeded by Nanchong Academy of Agricultural Sciences, Sichuan Province, China. These three genotypes are distinct regards to the genetic background. These variates were grown in Science and Technology Campus, Sichuan Agricultural University, and were harvested at the same time. The elite soybean seeds were chosen for further investigation.
For each petri dishes, 20 soybean seeds were incubated on two layers of medium-speed qualitative filter papers in Petri Dishes (diameter is 9 cm). Then, 11 mL distilled water, NaCl (at 150 mM), 100 nM FLUN or 10 μM paclobutrazol (PAC) were add to each plate according to the experiment requirements. Four replications (Petri Dishe) were performed. The plates were incubated at 25°C in incubator (Sanyo Versatile Environmental Teat Chamber MLR-350H). Germination rates were considered when the radicle broke through the seed coat. The germination percentages were counted at with time-course analysis.
After 60 h of imbibition, the radicles will be cut off, and the length and fresh weight of radicles per 20 seeds were measured. The Image J software was employed to measure the length of radicles. For each germination test, three experimental replications were performed. The average germination percentage ± SE (standard error) of triplicate experiments was calculated. NaCl (product number 7647-14-5), FLUN (product number 45511), and PAC (product number 33371) were ordered from Sigma–Aldrich Company Ltd., United States.
After NaCl treatment, MDA content and the activities of SOD, CAT, and POD were detected during soybean seed germination processes with time-course pattern. The method published elsewhere were employed for the quantification (Yang et al., 2010).
Imbibition seeds were collected at different time points after sowing and frozen in liquid nitrogen quickly. Total RNA preparation, first-strand cDNA synthesis and qRT-PCR assay were performed as our previously described (Shu et al., 2013). Quantitative PCR was performed using the SsoFastTM EvaGreen Supermix (Bio-Rad). Each 10 μL reaction comprised 2 μL template, 5 μL SsoFastTM EvaGreen Supermix, 0.5 μL (10 μM) of each primer and 2 μL Dnase-free ddH2O. The transcription pattern of genes involved in ABA/GA biosynthesis and signaling transduction pathways including GmNCED9, GmNCED5, GmAAO, GmABI4, GmABI5, and GmGA3ox1, GmKAO, GmGA2ox8, and GmRGL were investigated. Three biological replicates were performed for each experiment. Primer sequences for qRT-PCR are shown in Supplemental Table S1.
For analysis of ABA content in soybean seeds, the previously protocol we used (Shu et al., 2013) were employed in this study. Firstly, the seeds were ground to powder in liquid nitrogen, and 300 mg of seeds powder was homogenized and extracted for 24 h in methanol containing D6-ABA (OIChemIm Co. Ltd.) as an internal standard. Purification was performed with an Oasis Max solid phase extract cartridge (Waters) and eluted with 5% formic acid in methanol. Subsequently, the elution was dried and reconstituted, and it was then injected into a liquid chromatography–tandem mass spectrometry system consisting of an Acquity ultra performance liquid chromatograph (Acquity UPLC; Waters) and a triple quadruple tandem mass spectrometer (Quattro Premier XE; Waters). Three biological replications were performed.
The endogenous gibberellins were determined according to our method described previously (Chen et al., 2011). Soybean seeds (400 mg) were frozen in liquid nitrogen, ground to fine powder, and extracted with 80% (v/v) methanol. GA d2 isotope standards were added to plant samples before grinding. The crude extracts were purified by reversed-phase solid-phase extraction, ethyl ether extraction, and derivatization. The resulting mixture was injected into capillary electrophoresis-mass spectrometry (CE-MS) for quantitative analysis. Three biological replications were performed.
The data including germination rates, fresh weight and radicle length of germinated seeds, gene expression data, and phytohormones quantification results were analyzed using Student’s t-test. Different baselines were employed according to different requirements, and the detailed information was presented in the figure legends.
KS designed the research. YQ, FC, YM, XL, HS, WZ, JDi, JDu, JL, FY, QW, WL, TY, XW, and YF performed the experiments. KS and WY analyzed the data and wrote the manuscript.
This work was supported by Funding of Department of Education Sichuan Province (16ZB0040), National Key Research and Development Program of China (2017YFD0201306), and China Postdoctoral Science Foundation funded project (2014M552377 and 2016T90868).
The authors declare that the research was conducted in the absence of any commercial or financial relationships that could be construed as a potential conflict of interest.
We thank Ms. Chunyan Liu and Liang Yu (Sichuan Agricultural University) for the technical assistance.
The Supplementary Material for this article can be found online at: http://journal.frontiersin.org/article/10.3389/fpls.2017.01372/full#supplementary-material
FIGURE S1 | NaCl inhibits soybean seed germination. Cultivars C-103 seeds was incubated on two layers of filter paper in Petri dishes. The concentration of NaCl used was 150 mM, and the equivalent ultrapure water was employed as control (CK). (A) The representative images (36 h after sowing) are shown. (B) Quantitative analysis of germination rates is shown. (C,D) Radicle length and fresh weight of germinated soybean seeds were measured. Bar = 10 mm. The average percentages of four repeats ±standard error were shown. Student’s t-test assay was employed for statistical analysis. ∗∗ Difference is significant at the 0.01 level.
Chen, M. L., Huang, Y. Q., Liu, J. Q., Yuan, B. F., and Feng, Y. Q. (2011). Highly sensitive profiling assay of acidic plant hormones using a novel mass probe by capillary electrophoresis-time of flight-mass spectrometry. J. Chromatogr. B Analyt. Technol. Biomed. Life Sci. 879, 938–944. doi: 10.1016/j.jchromb.2011.03.003
Deng, B., Yang, K., Zhang, Y., and Li, Z. (2016). Can heavy metal pollution defend seed germination against heat stress? Effect of heavy metals (Cu2+, Cd2+ and Hg2+) on maize seed germination under high temperature. Environ. Pollut. 216, 46–52. doi: 10.1016/j.envpol.2016.05.050
Farhangi-Abriz, S., and Torabian, S. (2017) Antioxidant enzyme and osmotic adjustment changes in bean seedlings as affected by biochar under salt stress. Ecotoxicol. Environ. Saf. 137, 64–70. doi: 10.1016/j.ecoenv.2016.11.029
Finkelstein, R., Reeves, W., Ariizumi, T., and Steber, C. (2008). Molecular aspects of seed dormancy. Annu. Rev. Plant Biol. 59, 387–415. doi: 10.1146/annurev.arplant.59.032607.092740
Frey, A., Audran, C., Marin, E., Sotta, B., and Marion-Poll, A. (1999). Engineering seed dormancy by the modification of zeaxanthin epoxidase gene expression. Plant Mol. Biol. 39, 1267–1274. doi: 10.1023/A:1006145025631
Frey, A., Effroy, D., Lefebvre, V., Seo, M., Perreau, F., Berger, A., et al. (2012). Epoxycarotenoid cleavage by NCED5 fine-tunes ABA accumulation and affects seed dormancy and drought tolerance with other NCED family members. Plant J. 70, 501–512. doi: 10.1111/j.1365-313X.2011.04887.x
Galle, A., Csiszar, J., Benyo, D., Laskay, G., Leviczky, T., Erdei, L., et al. (2013). Isohydric and anisohydric strategies of wheat genotypes under osmotic stress: biosynthesis and function of ABA in stress responses. J. Plant Physiol. 170, 1389–1399. doi: 10.1016/j.jplph.2013.04.010
Gamble, P. E., and Mullet, J. E. (1986). Inhibition of carotenoid accumulation and abscisic acid biosynthesis in fluridone-treated dark-grown barley. Eur. J. Biochem. 160, 117–121. doi: 10.1111/j.1432-1033.1986.tb09947.x
Gonzalez-Guzman, M., Apostolova, N., Belles, J. M., Barrero, J. M., Piqueras, P., Ponce, M. R., et al. (2002). The short-chain alcohol dehydrogenase ABA2 catalyzes the conversion of xanthoxin to abscisic aldehyde. Plant Cell 14, 1833–1846. doi: 10.1105/tpc.002477
Graeber, K., Nakabayashi, K., Miatton, E., Leubner-Metzger, G., and Soppe, W. J. (2012). Molecular mechanisms of seed dormancy. Plant Cell Environ. 35, 1769–1786. doi: 10.1111/j.1365-3040.2012.02542.x
Guan, R., Qu, Y., Guo, Y., Yu, L., Liu, Y., Jiang, J., et al. (2014). Salinity tolerance in soybean is modulated by natural variation in GmSALT3. Plant J. 80, 937–950. doi: 10.1111/tpj.12695
Hamayun, M., Hussain, A., Khan, S. A., Irshad, M., Khan, A. L., Waqas, M., et al. (2015). Kinetin modulates physio-hormonal attributes and isoflavone contents of Soybean grown under salinity stress. Front. Plant Sci. 6:377. doi: 10.3389/fpls.2015.00377
Hanin, M., Ebel, C., Ngom, M., Laplaze, L., and Masmoudi, K. (2016). New insights on plant salt tolerance mechanisms and their potential use for breeding. Front. Plant Sci. 7:1787. doi: 10.3389/fpls.2016.01787
Hasegawa, P. M., Bressan, R. A., Zhu, J. K., and Bohnert, H. J. (2000). Plant cellular and molecular responses to high salinity. Annu. Rev. Plant Physiol. Plant Mol. Biol. 51, 463–499. doi: 10.1146/annurev.arplant.51.1.463
Horvath, E., Csiszar, J., Galle, A., Poor, P., Szepesi, A., and Tari, I. (2015). Hardening with salicylic acid induces concentration-dependent changes in abscisic acid biosynthesis of tomato under salt stress. J. Plant Physiol. 183, 54–63. doi: 10.1016/j.jplph.2015.05.010
Kan, G., Ning, L., Li, Y., Hu, Z., Zhang, W., He, X., et al. (2016). Identification of novel loci for salt stress at the seed germination stage in soybean. Breed. Sci. 66, 530–541. doi: 10.1270/jsbbs.15147
Kan, G., Zhang, W., Yang, W., Ma, D., Zhang, D., Hao, D., et al. (2015). Association mapping of soybean seed germination under salt stress. Mol. Genet. Genomics 290, 2147–2162. doi: 10.1007/s00438-015-1066-y
Koornneef, M., Hanhart, C. J., Hilhorst, H. W., and Karssen, C. M. (1989). In vivo inhibition of seed development and reserve protein accumulation in recombinants of abscisic acid biosynthesis and responsiveness mutants in Arabidopsis thaliana. Plant Physiol. 90, 463–469. doi: 10.1104/pp.90.2.463
Kuluev, B., Mikhaylova, E., Berezhneva, Z., Nikonorov, Y., Postrigan, B., Kudoyarova, G., et al. (2017). Expression profiles and hormonal regulation of tobacco NtEXGT gene and its involvement in abiotic stress response. Plant Physiol. Biochem. 111, 203–215. doi: 10.1016/j.plaphy.2016.12.005
Lee, S., Cheng, H., King, K. E., Wang, W., He, Y., Hussain, A., et al. (2002). Gibberellin regulates Arabidopsis seed germination via RGL2, a GAI/RGA-like gene whose expression is up-regulated following imbibition. Genes Dev. 16, 646–658. doi: 10.1101/gad.969002
Li, W., Wang, T., Zhang, Y., and Li, Y. (2016). Overexpression of soybean miR172c confers tolerance to water deficit and salt stress, but increases ABA sensitivity in transgenic Arabidopsis thaliana. J. Exp. Bot. 67, 175–194. doi: 10.1093/jxb/erv450
Li, W., Yamaguchi, S., Khan, M. A., An, P., Liu, X., and Tran, L. S. (2015). Roles of gibberellins and abscisic acid in regulating germination of Suaeda salsa dimorphic seeds under salt stress. Front. Plant Sci. 6:1235. doi: 10.3389/fpls.2015.01235
Liang, Y. C., Chen, Q., Liu, Q., Zhang, W. H., and Ding, R. X. (2003). Exogenous silicon (Si) increases antioxidant enzyme activity and reduces lipid peroxidation in roots of salt-stressed barley (Hordeum vulgare L.). J. Plant Physiol. 160, 1157–1164. doi: 10.1078/0176-1617-01065
Liao, Y., Zhang, J. S., Chen, S. Y., and Zhang, W. K. (2008a). Role of soybean GmbZIP132 under abscisic acid and salt stresses. J. Integr. Plant Biol. 50, 221–230. doi: 10.1111/j.1744-7909.2007.00593.x
Liao, Y., Zou, H. F., Wang, H. W., Zhang, W. K., Ma, B., Zhang, J. S., et al. (2008b). Soybean GmMYB76, GmMYB92, and GmMYB177 genes confer stress tolerance in transgenic Arabidopsis plants. Cell Res. 18, 1047–1060. doi: 10.1038/cr.2008.280
Lin, P. C., Hwang, S. G., Endo, A., Okamoto, M., Koshiba, T., and Cheng, W. H. (2007). Ectopic expression of ABSCISIC ACID 2/GLUCOSE INSENSITIVE 1 in Arabidopsis promotes seed dormancy and stress tolerance. Plant Physiol. 143, 745–758. doi: 10.1104/pp.106.084103
Liu, Y., Yu, L., Qu, Y., Chen, J., Liu, X., Hong, H., et al. (2016). GmSALT3, which confers improved soybean salt tolerance in the field, increases leaf Cl- exclusion prior to Na+ exclusion but does not improve early vigor under salinity. Front. Plant Sci. 7:1485. doi: 10.3389/fpls.2016.01485
Ma, Q., Kang, J., Long, R., Zhang, T., Xiong, J., Zhang, K., et al. (2017). Comparative proteomic analysis of alfalfa revealed new salt and drought stress-related factors involved in seed germination. Mol. Biol. Rep. 44, 261–272. doi: 10.1007/s11033-017-4104-5
Magnone, M., Scarfi, S., Sturla, L., Guida, L., Cuzzocrea, S., Di Paola, R., et al. (2013). Fluridone as a new anti-inflammatory drug. Eur. J. Pharmacol. 720, 7–15. doi: 10.1016/j.ejphar.2013.10.058
Meng, Y., Chen, F., Shuai, H., Luo, X., Ding, J., Tang, S., et al. (2016). Karrikins delay soybean seed germination by mediating abscisic acid and gibberellin biogenesis under shaded conditions. Sci. Rep. 6:22073. doi: 10.1038/srep22073
Munns, R. (2002). Comparative physiology of salt and water stress. Plant Cell Environ. 25, 239–250. doi: 10.1046/j.0016-8025.2001.00808.x
Munns, R., and Tester, M. (2008). Mechanisms of salinity tolerance. Annu. Rev. Plant Biol. 59, 651–681. doi: 10.1146/annurev.arplant.59.032607.092911
Nee, G., Xiang, Y., and Soppe, W. J. (2016). The release of dormancy, a wake-up call for seeds to germinate. Curr. Opin. Plant Biol. 35, 8–14. doi: 10.1016/j.pbi.2016.09.002
Piskurewicz, U., Jikumaru, Y., Kinoshita, N., Nambara, E., Kamiya, Y., and Lopez-Molina, L. (2008). The gibberellic acid signaling repressor RGL2 inhibits Arabidopsis seed germination by stimulating abscisic acid synthesis and ABI5 activity. Plant Cell 20, 2729–2745. doi: 10.1105/tpc.108.061515
Piskurewicz, U., and Lopez-Molina, L. (2009). The GA-signaling repressor RGL3 represses testa rupture in response to changes in GA and ABA levels. Plant Signal. Behav. 4, 63–65.
Seo, M., Hanada, A., Kuwahara, A., Endo, A., Okamoto, M., Yamauchi, Y., et al. (2006). Regulation of hormone metabolism in Arabidopsis seeds: phytochrome regulation of abscisic acid metabolism and abscisic acid regulation of gibberellin metabolism. Plant J. 48, 354–366. doi: 10.1111/j.1365-313X.2006.02881.x
Shakirova, F., Sakhabutdinova, A., Bezrukova, M., Fatkhutdinova, R., and Fatkhutdinova, D. (2003). Changes in the hormonal status of wheat seedlings induced by salicylic acid and salinity. Plant Sci. 164, 317–322. doi: 10.1016/S0168-9452(02)00415-6
Shu, K., Chen, Q., Wu, Y., Liu, R., Zhang, H., Wang, P., et al. (2016a). ABI4 mediates antagonistic effects of abscisic acid and gibberellins at transcript and protein levels. Plant J. 85, 348–361. doi: 10.1111/tpj.13109
Shu, K., Liu, X. D., Xie, Q., and He, Z. H. (2016b). Two faces of one seed: hormonal regulation of dormancy and germination. Mol. Plant 9, 34–45. doi: 10.1016/j.molp.2015.08.010
Shu, K., Meng, Y. J., Shuai, H. W., Liu, W. G., Du, J. B., Liu, J., et al. (2015). Dormancy and germination: How does the crop seed decide? Plant Biol. 17, 1104–1112. doi: 10.1111/plb.12356
Shu, K., Zhang, H., Wang, S., Chen, M., Wu, Y., Tang, S., et al. (2013). ABI4 regulates primary seed dormancy by regulating the biogenesis of abscisic acid and gibberellins in Arabidopsis. PLoS Genet. 9:e1003577. doi: 10.1371/journal.pgen.1003577
Stetsenko, L. A., Vedenicheva, N. P., Likhnevsky, R. V., and Kuznetsov, V. V. (2015). Influence of abscisic acid and fluridone on the content of phytohormones and polyamines and the level of oxidative stress in plants of Mesembryanthemum crystallinum L. under salinity. Izv. Akad. Nauk. Ser. Biol. 42, 134–144.
Sun, K., Gao, B., Ro, K. S., Novak, J. M., Wang, Z., Herbert, S., et al. (2012). Assessment of herbicide sorption by biochars and organic matter associated with soil and sediment. Environ. Pollut. 163, 167–173. doi: 10.1016/j.envpol.2011.12.015
Teige, M., Scheikl, E., Eulgem, T., Doczi, R., Ichimura, K., Shinozaki, K., et al. (2004). The MKK2 pathway mediates cold and salt stress signaling in Arabidopsis. Mol. Cell 15, 141–152. doi: 10.1016/j.molcel.2004.06.023
Vieira, B. C., Bicalho, E. M., Munne-Bosch, S., and Garcia, Q. S. (2017). Abscisic acid regulates seed germination of Vellozia species in response to temperature. Plant Biol. 19, 211–216. doi: 10.1111/plb.12515
Wang, Y., Wang, L., Zou, Y., Chen, L., Cai, Z., Zhang, S., et al. (2014). Soybean miR172c targets the repressive AP2 transcription factor NNC1 to activate ENOD40 expression and regulate nodule initiation. Plant Cell 26, 4782–4801. doi: 10.1105/tpc.114.131607
Xiong, L., Lee, H., Ishitani, M., and Zhu, J. K. (2002). Regulation of osmotic stress-responsive gene expression by the LOS6/ABA1 locus in Arabidopsis. J. Biol. Chem. 277, 8588–8596. doi: 10.1074/jbc.M109275200
Xu, X., Fan, R., Zheng, R., Li, C., and Yu, D. (2011). Proteomic analysis of seed germination under salt stress in soybeans. J. Zhejiang Univ. Sci. B 12, 507–517. doi: 10.1631/jzus.B1100061
Yamauchi, Y., Takeda-Kamiya, N., Hanada, A., Ogawa, M., Kuwahara, A., Seo, M., et al. (2007). Contribution of gibberellin deactivation by AtGA2ox2 to the suppression of germination of dark-imbibed Arabidopsis thaliana seeds. Plant Cell Physiol. 48, 555–561. doi: 10.1093/pcp/pcm023
Yang, F., Wang, Y., and Miao, L. F. (2010). Comparative physiological and proteomic responses to drought stress in two poplar species originating from different altitudes. Physiol. Plant. 139, 388–400. doi: 10.1111/j.1399-3054.2010.01375.x
Ybanez, R. H., Leesombun, A., Nishimura, M., Matsubara, R., Kojima, M., Sakakibara, H., et al. (2016). In vitro and in vivo effects of the phytohormone inhibitor fluridone against Neospora caninum infection. Parasitol. Int. 65, 319–322. doi: 10.1016/j.parint.2016.03.009
Zhang, W. J., Niu, Y., Bu, S. H., Li, M., Feng, J. Y., Zhang, J., et al. (2014). Epistatic association mapping for alkaline and salinity tolerance traits in the soybean germination stage. PLoS ONE 9:e84750. doi: 10.1371/journal.pone.0084750
Zhang, Y., Lan, H., Shao, Q., Wang, R., Chen, H., Tang, H., et al. (2016). An A20/AN1-type zinc finger protein modulates gibberellins and abscisic acid contents and increases sensitivity to abiotic stress in rice (Oryza sativa). J. Exp. Bot. 67, 315–326. doi: 10.1093/jxb/erv464
Zhu, J. K. (2002). Salt and drought stress signal transduction in plants. Annu. Rev. Plant Biol. 53, 247–273. doi: 10.1146/annurev.arplant.53.091401.143329
Keywords: salinity, seed germination, abscisic acid, gibberellin, soybean
Citation: Shu K, Qi Y, Chen F, Meng Y, Luo X, Shuai H, Zhou W, Ding J, Du J, Liu J, Yang F, Wang Q, Liu W, Yong T, Wang X, Feng Y and Yang W (2017) Salt Stress Represses Soybean Seed Germination by Negatively Regulating GA Biosynthesis While Positively Mediating ABA Biosynthesis. Front. Plant Sci. 8:1372. doi: 10.3389/fpls.2017.01372
Received: 22 May 2017; Accepted: 24 July 2017;
Published: 10 August 2017.
Edited by:
Vicent Arbona, Jaume I University, SpainCopyright © 2017 Shu, Qi, Chen, Meng, Luo, Shuai, Zhou, Ding, Du, Liu, Yang, Wang, Liu, Yong, Wang, Feng and Yang. This is an open-access article distributed under the terms of the Creative Commons Attribution License (CC BY). The use, distribution or reproduction in other forums is permitted, provided the original author(s) or licensor are credited and that the original publication in this journal is cited, in accordance with accepted academic practice. No use, distribution or reproduction is permitted which does not comply with these terms.
*Correspondence: Kai Shu, a3NodUBzaWNhdS5lZHUuY24= Wenyu Yang, bXNzaXlhbmd3eUBzaWNhdS5lZHUuY24=
†These authors have contributed equally to this work.
Disclaimer: All claims expressed in this article are solely those of the authors and do not necessarily represent those of their affiliated organizations, or those of the publisher, the editors and the reviewers. Any product that may be evaluated in this article or claim that may be made by its manufacturer is not guaranteed or endorsed by the publisher.
Research integrity at Frontiers
Learn more about the work of our research integrity team to safeguard the quality of each article we publish.