- 1School of Agriculture and Environment, The University of Western Australia, Perth, WA, Australia
- 2The UWA Institute of Agriculture, The University of Western Australia, Perth, WA, Australia
- 3CSIRO Agriculture and Food, Wembley, WA, Australia
End-of-season drought or “terminal drought,” which occurs after flowering, is considered the most significant abiotic stress affecting crop yields. Wheat crop production in Mediterranean-type environments is often exposed to terminal drought due to decreasing rainfall and rapid increases in temperature and evapotranspiration during spring when wheat crops enter the reproductive stage. Under such conditions, every millimeter of extra soil water extracted by the roots benefits grain filling and yield and improves water use efficiency (WUE). When terminal drought develops, soil dries from the top, exposing the top part of the root system to dry soil while the bottom part is in contact with available soil water. Plant roots sense the drying soil and produce signals, which on transmission to shoots trigger stomatal closure to regulate crop water use through transpiration. However, transpiration is linked to crop growth and productivity and limiting transpiration may reduce potential yield. While an early and high degree of stomatal closure affects photosynthesis and hence biomass production, a late and low degree of stomatal closure exhausts available soil water rapidly which results in yield losses through a reduction in post-anthesis water use. The plant hormone abscisic acid (ABA) is considered the major chemical signal involved in stomatal regulation. Wheat genotypes differ in their ability to produce ABA under drought and also in their stomatal sensitivity to ABA. In this viewpoint article we discuss the possibilities of exploiting genotypic differences in ABA response to soil drying in regulating the use of water under terminal drought. Root density distribution in the upper drying layers of the soil profile is identified as a candidate trait that can affect ABA accumulation and subsequent stomatal closure. We also examine whether leaf ABA can be designated as a surrogate characteristic for improved WUE in wheat to sustain grain yield under terminal drought. Ease of collecting leaf samples to quantify ABA compared to extracting xylem sap will facilitate rapid screening of a large number of germplasm for drought tolerance.
Introduction
Wheat (Triticum aestivum L.) is the second most important dietary intake grain after rice (FAO, 2013) and the most internationally traded food crop (Foresight, 2011). Average annual global production reached 713 million metric tons in 2013 (FAO, 2015) and around 65% of the produce is used as food (FAO, 2013). By 2050, wheat production has to double to meet the growing global demand for food (Foresight, 2011). Achieving this target, against rising global temperatures and changing patterns of precipitation, will be challenging. Drought is a major abiotic stress reducing wheat yields in many wheat growing areas of the world. Although drought at all wheat growth stages impair crop performance, drought occurring during flowering and grain-filling (terminal drought) is the most detrimental to grain yield.
Terminal drought often occurs in wheat growing regions with Mediterranean-type climatic conditions. These regions are characterized by wet, cold winters and dry, warm summers and wheat growth is low during winter due to low temperature and radiation (Palta and Watt, 2009). The low transpiration demand due to low temperature, low vapour pressure deficit (VPD) and low variability in winter rainfall reduces the occurrence of water stress during vegetative growth. Low and erratic rainfall, increased temperatures and VPD, and evaporative demand in spring and early summer lead to soil water shortage, which often causes crop water deficit after flowering (Turner and Asseng, 2005). Thus, terminal drought is the most significant stress affecting wheat yield (Saini and Aspinall, 1981), but the degree of grain yield reduction depends on the time and rate of development of the crop water deficit (Kobata et al., 1992; Palta et al., 1994). Grain yield in wheat declined by 50% when terminal drought was induced at flowering (Dias de Oliveira et al., 2013). Under extreme terminal drought conditions, wheat yields can fall below 0.5 t/ha (Asseng et al., 2004). Reduced rainfall predicted during autumn may delay sowing until later in the season and could, therefore, further increase the risk of exposure to terminal drought (Farre and Foster, 2010). The impact of water stress on wheat yield is determined by how it affects the physiological processes and conditions in plants, which varies between wheat genotypes (Kramer, 1980).
In water-limited environments, grain yield is a function of water use, water use efficiency (WUE) and harvest index (Passioura, 1983). Hence, terminal drought can be contested to a considerable extent by breeding new varieties with traits that improve WUE (Turner and Asseng, 2005). WUE describes the biomass accumulated per unit of water consumed, and is often used in different levels and units (Turner, 1986; Tambussi et al., 2007). Reduced water uptake will clearly improve WUE, but reduces yield as per Passioura’s equation described above. For improving yield under water limited environment, identifying traits that favor effective use of available water is considered essential (Blum, 2009). This viewpoint article explores the possibilities of exploiting potential genotypic differences in ABA response to soil drying in regulating the use of water to protect yield under terminal drought.
Crop Adaptive Strategies to Combat Terminal Drought
Terminal drought occurs when crops enter their reproductive growth stage (Turner and Begg, 1981). Since wheat is a determinate crop (Atwell et al., 1999), adaptation mechanisms such as reductions in leaf area, tiller number and biomass are no longer feasible under terminal drought. Drought escapism, the ability to complete a lifecycle before severe plant water deficit develops (Kramer, 1980), has been used by crop breeders for earliness (Siddique et al., 1989). However, earliness may reduce yield potential in years where rainfall is plentiful (Turner, 1986). Furthermore, under Mediterranean-type climates, drought escapism should be accompanied by low-temperature tolerance (Kramer, 1980). Under prevailing unpredictable rainfall conditions, adaptive measures to tolerate drought either by postponing or enduring dehydration (Turner, 1986) help to sustain physiological activities and minimize yield loss in instances where rainfall is minimal. Osmotic adjustment to tolerate dehydration has no direct influence on grain yield other than modifying the water extraction pattern (Morgan and Condon, 1986; Serraj and Sinclair, 2002). Furthermore, osmotic adjustment helps plants to keep stomatal open under water stress (Blum et al., 1999), which could rapidly exhaust available soil water and be detrimental to grain filling and yield.
Terminal drought affects grain filling (Fischer and Kohn, 1966; Saini and Aspinall, 1981; Rajala et al., 2009), resulting in shriveled grains (Mitchell et al., 2013). The carbohydrate requirement for grain filling is partly met by current assimilates and partly by the translocation of assimilates stored in vegetative parts. Under terminal drought, the major source of carbon for grain filling is stored assimilates in the tillers (Pheloung and Siddique, 1991; Kobata et al., 1992; Blum, 1998) as photosynthesis will be limited by water stress. The proportion of biomass converted to grain yield is determined mainly by the water used after anthesis (Passioura, 1983). Thus, every extra millimeter of water extracted during grain filling can result in yield advantage (Manschadi et al., 2006; Kirkegaard et al., 2007). Therefore, sustaining water uptake during grain filling is critical for improving grain yield under terminal drought. Plant adaptation strategies such as stomatal closure to regulate water loss and/or root properties to slow down rapid depletion of soil moisture use may lead to yield improvement under terminal drought.
Stomatal Regulation to Control Water Use Under Terminal Drought
More than 90% of water uptake in plants is lost through transpiration (Pei et al., 1998) mainly through diminutive pores in the leaf epidermis called stomata. Leaf transpiration is determined by the leaf-to-air vapor pressure deficit (VPD) and resistance to the movement of water from the leaf to the atmosphere (Farquhar and Sharkey, 1982). Reducing the width of the stomatal opening reduces the ease with which water passes from the plant to the atmosphere (stomatal conductance) and is considered a drought adaptive mechanism (Schmidt, 1983).
Root-To-Shoot Signaling to Regulate Stomata
Stomatal regulation in response to soil dryness implies communication between the roots in the drying soil and the responding leaves. As roots are in direct contact with the drying soil, it has been postulated that roots generate and transmit signals to the leaves such that the stomata respond (Gollan et al., 1986; Passioura, 1988; Blum and Johnson, 1993). The involvement of root signals in controlling stomata has been confirmed by many studies and a vast pool of data supports a chemical signal, the plant hormone abscisic acid (ABA) (Loveys and Kriedemann, 1974; Zhang et al., 1987; Henson et al., 1989b; Zhang and Davies, 1990a; Munns and Sharp, 1993).
Abscisic acid has been strongly advocated as the chemical signal involved in this root-to-shoot communication process, but it has not been confirmed as the sole signal involved. For instance, Munns and King (1988) showed the presence of a different compound in the xylem sap of wheat plants that reduces stomatal conductance and increases leaf ABA concentration. When excised wheat leaves were fed exogenous solutions without ABA, partial stomatal closure was noticed (Dodd, 2013), probably due to the lack of some signals to keep the stomata fully open, possibly other hormones like cytokinin. In recent years, hormone interactions (Acharya and Assmann, 2009) and interactions between hormones and the environment have attracted much interest. Thus, the involvement of other hormones and chemicals like cytokinin, auxins, ethylene, jasmonic acid, salicylic acid, H2O2 and ionic substances has been suggested which can act either as positive (presence or increased concentration causes stomatal closure) or negative (absence or decreased concentration reduces stomatal conductance) signals (Schachtman and Goodger, 2008; Acharya and Assmann, 2009; Wilkinson et al., 2012). Esters of ABA, especially glucose esters, can play a significant role as a root signal (Munns and Sharp, 1993; Sauter et al., 2002). An increase in xylem pH (Davies and Zhang, 1991; Sobeih et al., 2004) has also been considered a root signal or an amplifier of root signal which facilitates the redistribution of sequestrated leaf ABA to reach guard cells.
Another study with grafted Arabidopsis plants with either ABA-deficient stock or scion points to little importance of ABA as a root signal, but emphasized the importance of leaf ABA in stomatal regulation (Christmann et al., 2007). Supplying water directly to leaves of water-stressed plants reverted stomatal closure indicating that hydraulic signals were also involved in stomatal regulation (Comstock, 2002; Christmann et al., 2007). A drop in root water potential, with a net result of decreased soil water potential and water flux, can be considered the signal generator to regulate stomata (Tardieu et al., 1991). No consensus has been reached regarding the root signal that causes stomatal closure when the soil dries. Whatever it may be, ABA concentration in wheat leaves increases in response to water stress (Wright, 1969) and modulates stomatal conductance (Mittelheuser and Van Steveninck, 1969).
Root Distribution in Stomatal Regulation
As stomatal closure under water deficits is in response to the signals generated and transmitted from the roots, root characteristics might play an important role in this signal generation process. Wheat plants regulated stomata in response to drying signals from the roots in the top drying layer of the soil profile even though leaf water status was maintained by unlimited water supply from deeper soil layers (Blum and Johnson, 1993; Saradadevi et al., 2015). These findings were substantiated with increased ABA concentration in barley leaves when more seminal roots were distributed in the dry half of the pots (Martin-Vertedor and Dodd, 2011). This proves that root distribution plays an important role in signal generation and subsequent stomatal regulation. Therefore, under terminal drought conditions in Mediterranean-type regions, a greater root distribution in the drying upper soil layers causes ABA to accumulate in leaves which regulates stomata to conserve water for grain filling. This may help the plant as an early signaling mechanism to regulate stomata and conserve water well before a large part of the root zone has been depleted of water.
ABA Accumulation and Stomatal Regulation
An increased concentration of ABA in leaves associated with reduced stomatal conductance (gs) under water deficits has been confirmed in several studies conducted in various species including wheat (Wright, 1969; Loveys and Kriedemann, 1974; Quarrie and Jones, 1977; Quarrie, 1980; Blackman and Davies, 1985; Zhang et al., 1987; Henson et al., 1989b; Davies and Zhang, 1991; Munns and Sharp, 1993). Leaf ABA as the main driver of stomatal regulation was questioned when several studies in species such as maize demonstrated that xylem ABA increases much earlier than leaf ABA and correlates better with gs than leaf ABA (Blackman and Davies, 1985; Zhang and Davies, 1990a; Tardieu et al., 1992). This is because leaf ABA consists of ABA sequestrated into the mesophyll chloroplast which has no effect on stomatal regulation (Dodd et al., 1996). However, this has not been clearly demonstrated in wheat, probably because few studies have measured xylem sap ABA in wheat under drying soil conditions (Table 1) as a consequence of the difficulty in obtaining xylem sap (Cramer and Lewis, 1993; Munns et al., 1993). In addition, strong correlation between leaf ABA and gs has been demonstrated in wheat (Henson et al., 1989b; Ali et al., 1998; Saradadevi et al., 2014, 2015), unlike in maize or sunflower (Zhang and Davies, 1990b; Tardieu et al., 1992). This does not suggest that xylem ABA has no role in stomatal regulation in wheat. The limited studies that have extracted xylem sap from wheat seedlings by pressuring the whole plant have demonstrated that xylem sap ABA increases with reduction in soil moisture, and turgid wheat leaves reduce gs when fed the collected sap (Munns and King, 1988; Munns et al., 1993). Wheat leaves fed with exogenous ABA also mimicked the effect of water stress by closing their stomata (Mittelheuser and Van Steveninck, 1969; Quarrie and Jones, 1977), confirming the involvement of xylem ABA in the stomatal regulation of wheat. However, the exogenous ABA concentration required to mimic stomatal response was 100 times that of its endogenous ABA (Munns and King, 1988) indicating that other factors act in conjunction with xylem ABA in stomatal closure, such as the presence of other compounds (Munns et al., 1993) or xylem sap pH (Wilkinson and Davies, 1997; Sobeih et al., 2004). Alternatively, leaf ABA may contribute to ABA that reach guard cells in water stressed plants (Cowan et al., 1982; Bahrun et al., 2002), especially in mature plants since stomatal sensitivity to xylem ABA decreases with aging in wheat (Atkinson et al., 1989). Increased accumulation of leaf ABA in non-pressurized plants compared to pressurized wheat plants under similar moisture stress supports the leaf as the major source for ABA at the reproductive stage (Westgate et al., 1996). Flag leaf ABA increases in response to turgor loss and is the source for ABA to the spike (Morgan and King, 1984). Consequently, at least in wheat plants at the reproductive stage, leaf ABA is significant and correlated with gs (Henson et al., 1989b). Evidence from different species including wheat suggests that stomatal regulation can be considered the net result of an integrative response of both root and leaf ABA (Tardieu and Davies, 1993).
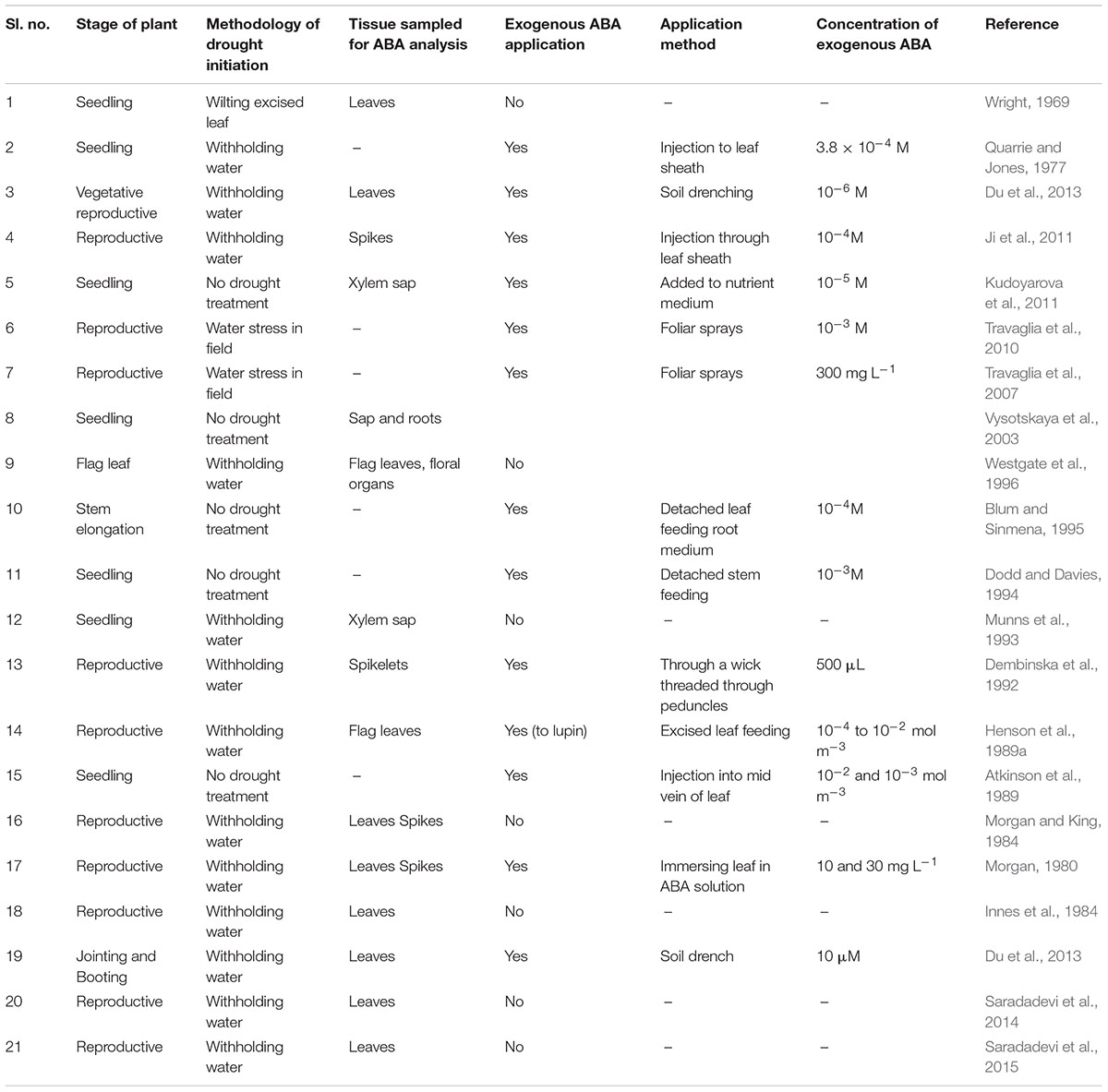
TABLE 1. Examples of previous research conducted in wheat to elucidate the role of ABA under drought.
ABA Regulates Root Hydraulic Resistance: A Trait that Limits Water Flux Through Roots
Water flow through plants is governed by the driving forces and resistance imposed by the conduit (Boyer, 1985). Considerable resistance to water flow through the plant is provided by roots (Newman, 1976). Therefore, resistance to water flow (low conductance) within the root prevents absorption and the supply of water to the shoot even though root growth is sufficient to reach available water within the soil. Water absorbed by roots flows across the root radius to reach xylem (radial pathway) and then follows a longitudinal pathway to the shoot through the xylem (axial pathway). Hence, root hydraulic resistance is a combination of resistances offered by both radial and axial pathways, with radial flow being the greatest constraint (Steudle and Peterson, 1998; Bramley et al., 2009). Root structure and anatomy contributes to the hydraulic properties of roots (Bramley et al., 2009). For instance, small xylem vessels impart larger resistance to water flow through the xylem (Richards and Passioura, 1989). Likewise, the predominant radial pathway adopted affects hydraulic conductance. For example, apoplastic flow is driven by the hydrostatic gradient and involves minimal resistance compared with the symplastic pathway (Steudle and Peterson, 1998). In wheat, significant radial water flow occurs symplastically (Bramley et al., 2009), which is facilitated by the membrane-bound protein, aquaporin. Aquaporin activity can potentially be enhanced by interactions with ABA (Hose et al., 2000). A higher concentration of ABA was observed in wheat roots in association with increased root hydraulic conductance following excision of four out of five seminal roots (Vysotskaya et al., 2003, 2004). This hike in root ABA and subsequent enhancement of root hydraulic conductivity to meet increased transpiration demand is due to the redistribution of ABA from leaf to root (Kudoyarova et al., 2011). Thus, leaf ABA is involved in regulating root hydraulic conductivity, in addition to its role in regulating stomata.
ABA Dynamics in Plants
Abscisic acid is synthesized in apical root cells and also in mesophyll cells in the leaves (Hartung et al., 2002). Plant roots absorb ABA and its conjugates (ABA-glucose ester) from the soil solution (Hartung et al., 2002). Root cells synthesize ABA when their water status is reduced by 50% or more (Hartung et al., 2002). ABA in the root tissues takes both apoplastic and symplastic pathways to reach xylem (Hartung et al., 2002). Xylem ABA acts as an early signal that initiates stomatal regulation (Zhang and Davies, 1990a). As water deficit increases, ABA biosynthesis in leaves is triggered by a reduced leaf water potential or turgor (Westgate et al., 1996). ABA concentration increases in all leaf tissues including guard cells (Harris et al., 1988). Leaf-synthesized ABA is loaded into the phloem and transported to the roots (Slovik et al., 1995; Kudoyarova et al., 2011) either to enter the xylem (Liang et al., 1997) or to be deposited in root tissues (Hartung et al., 2002). During transportation from root to leaves, stem parenchyma cells also contribute to xylem ABA under conditions of high concentration and pH gradient (Sauter and Hartung, 2002). ABA being a weak acid (Hartung and Slovik, 1991), ABA reaching the leaf lamina through xylem gets sequestrated into alkaline compartments of leaf tissues (Cowan et al., 1982; Slovik et al., 1995) depending on the pH gradient between the tissue and xylem (Wilkinson and Davies, 1997). With higher xylem sap pH, ABA sequestration to leaf tissue is reduced or the redistribution of leaf tissue ABA to reach guard cells is favored (Cowan et al., 1982; Popova et al., 2000). In addition, guard cells can synthesize ABA (Bauer et al., 2013).
Abscisic acid also gets degraded to form phaseic acid (PA), which may be further metabolized to dihydrophaseic acid (DPA) (Harrison and Walton, 1975; Creelman and Zeevaart, 1984). Alternatively, ABA conjugates with glucose to form ABA-glucose ester (ABA-GE) which is not active in stomatal regulation (Zeevaart and Creelman, 1988). Esters of ABA are present in the xylem sap of several species (Jeschke et al., 1997; Hansen and Dörffling, 1999; Sauter et al., 2002) and are believed to be involved in root-to-shoot signaling. In wheat, the high-molecular weight compound with anti-transpiration properties in the xylem sap of water-stressed plants is possibly a glucose ester of ABA (Munns and King, 1988; Munns et al., 1993). ABA-GE is capable of releasing free ABA upon hydrolysis by β-glucosidases (Dietz et al., 2000; Lee et al., 2006; Schroeder and Nambara, 2006; Xu et al., 2012). Thus, bulk leaf ABA is the net result of ABA transport through the xylem, its biosynthesis in leaves, degradation and conjugation (Figure 1). To understand the mode-of-action of ABA, ABA biosynthesis, distribution, and degradation, it is critical to first establish reliable tissue sampling techniques to quantify ABA.
Tissue Sampling for ABA Analysis
A strong correlation exists with ABA and gs, but the relationship varies with species and the tissue sampled. The main reason for this discrepancy is that the ABA concentration in leaf tissue or xylem sap does not relate to those reaching guard cells, which acts on stomata (Munns and Sharp, 1993). The most accurate option is to measure ABA nearest to the site of action, which is the guard cells, but this is possible only in some species like Commelina where the epidermis can be stripped easily (Blackman and Davies, 1983) and guard cells can be isolated by killing all other epidermal cells by a low pH treatment (MacRobbie, 1980). Due to this limitation in isolating the epidermis in some species and the laborious process involved in collecting sufficient epidermal tissue for analysis, xylem sap is considered the best option to explain stomatal behavior (Dodd et al., 1996).
Xylem sap sampling is not easy in cereals as it is difficult to collect a sufficient volume of xylem sap to quantify ABA (Dodd and Davies, 1996), especially in wheat and barley (Cramer and Lewis, 1993; Munns et al., 1993). Hence, most studies where xylem sap ABA is measured have been conducted on maize due to the ease of extraction of a large volume of sap (Dodd and Davies, 1996; Table 2). Besides, much attention is needed to get a representative sample as ABA concentration varies with the volume of collected sap (Borel and Simonneau, 2002), the method of collection (Quarrie and Lister, 1983), the point of collection (Netting et al., 2012) and the time of collection (Schurr et al., 1992; Tardieu and Davies, 1992).
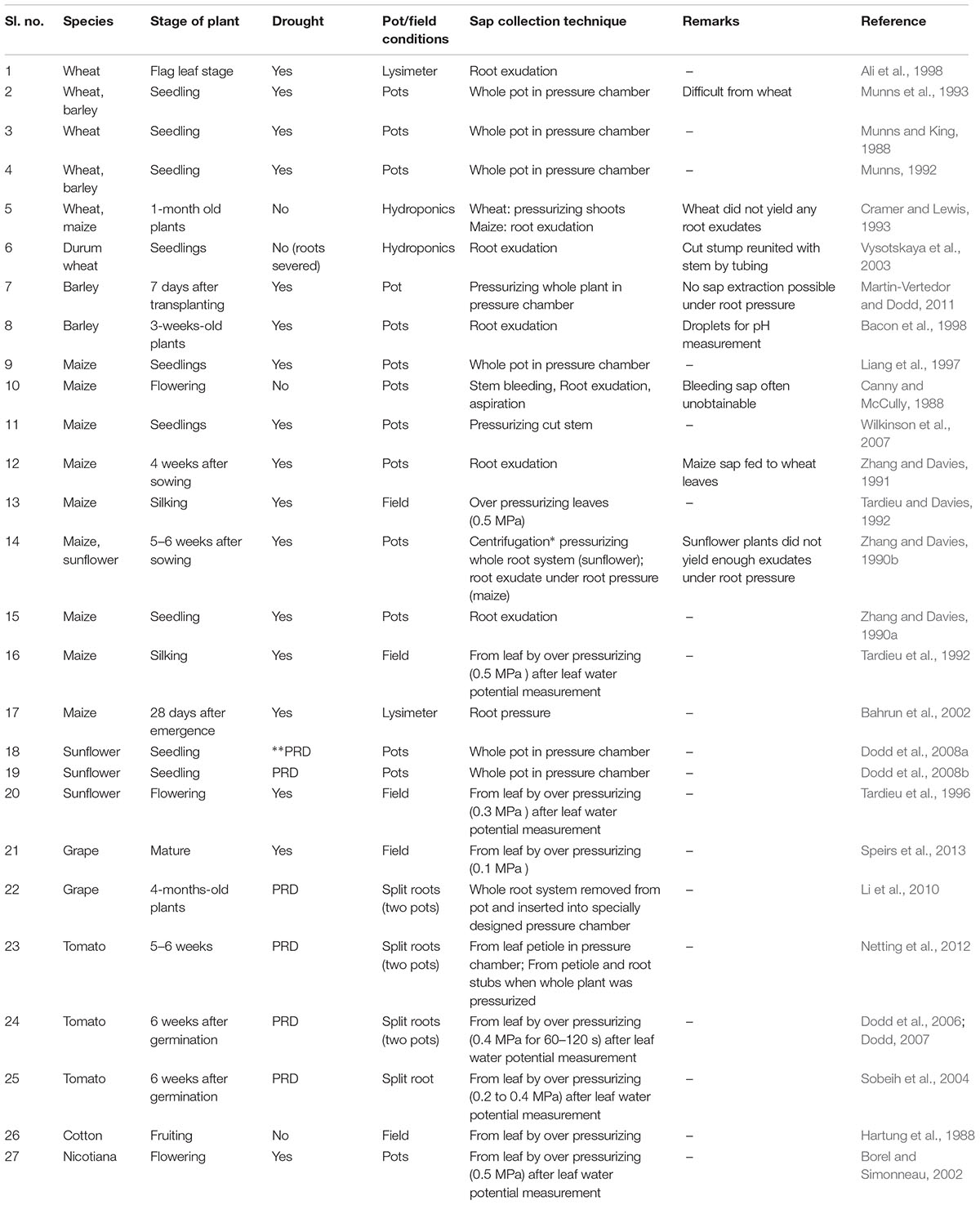
TABLE 2. Summary of the literature showing methodology used to collect xylem sap from different species.
Xylem Sap Sampling Techniques
A commonly used method to collect exuded root xylem sap is from detached root stumps (Zhang and Davies, 1990a; Vysotskaya et al., 2003). Removing the aerial part will cease transpiration, which increases root pressure, thus causing exudation. However, little exudate was collected from wheat grown under hydroponics, even without water stress (Cramer and Lewis, 1993). Root exudation did not yield any sap from barley plants (Martin-Vertedor and Dodd, 2011). Nevertheless, Ali et al. (1998) collected xylem sap from water-stressed wheat plants grown in lysimeters through root exudation. The drawback in exudate collection is that the flux of sap exudation will be much lower due to lack of transpiration pull (Schurr, 1998), which alters the ABA concentration in the sap (Else et al., 1994; Goodger et al., 2005). Information about fluxes and ABA concentration is needed (Schurr, 1998) to account for the changes in stomatal opening. It is ideal to collect the sap when the flow rate is similar to the transpiring rate of an intact plant (Else et al., 1995), but negative pressure in the xylem of transpiring plants makes collection difficult (Schurr, 1998).
The pressurization technique allows sap collection to occur at a similar rate of flux as the intact transpiring plant (Munns et al., 1993), but the ABA concentration may vary due to wounding (Else et al., 1994) and the interrupted flow of signals and ions from phloem to xylem (Schurr, 1998). Pressurization can be applied to extract sap from other plant parts like leaves, but the volume of extraction without water contamination from internal compartments is limited (Dodd, 2007). Some studies have collected xylem sap from wheat by pressurizing the whole root system in a pressure chamber (Table 2). The lack of pressure chambers (Dodd, 2007) suitable for large flowering stage plants limits its applicability to seedlings.
The application of external forces such as a vacuum is another method of collecting xylem sap. As the xylem fluid is under less axial resistance compared to the fluid in surrounding tissues, application of a slight force will separate xylem sap increasing the risk of contamination with other fluids. Applying negative pressure through a vacuum stimulates conditions similar to intact transpiring plants (Freundl et al., 1998). However, few experiments have used this technique to extract xylem sap (Table 2).
Controversy exists regarding which xylem sap sampling procedures best represents ABA concentration in the xylem sap of a transpiring plant. Since the main site of action is the leaves and they are easy to access and abundant (Dodd et al., 1996), and because leaf ABA and gs in wheat are correlated, leaves tissue are the most sampled tissue in ABA studies in wheat (Tables 1, 2).
Genotypic Variation in ABA Accumulation and Stomatal Sensitivity to ABA
Significant genotypic variation in the accumulation of ABA in wheat leaves under water stress has been demonstrated in most of the studies conducted in wheat (Quarrie and Jones, 1977; Henson and Quarrie, 1981; Quarrie, 1981; Ji et al., 2011; Du et al., 2013). Wheat genotypes that accumulate less ABA in their leaves have been associated to drought resistance and those accumulating more ABA have been considered sensitive to drought (Quarrie, 1981; Ji et al., 2011). On the contrary, high leaf ABA accumulating wheat lines demonstrated better WUE for grain yield than low ABA lines (Innes et al., 1984). Genotypic variation in ABA accumulation associated with drought tolerance in pearl millet is more pronounced under well-watered conditions such that genotypes accumulating more ABA under well-watered conditions showed drought tolerance (Kholova et al., 2010). Similarly, in a split-root study, wheat genotype Drysdale, which yielded more than the drought-tolerant line IGW-3262, had higher leaf ABA content under well-watered conditions (Saradadevi et al., 2014, 2015). These contrasting evidences suggest that the ABA-associated drought tolerance is not just through the effect of ABA on stomatal conductance, but other processes that may be affected by ABA (Quarrie and Henson, 1981), such as pollen sterility, translocation of pre-anthesis stored carbohydrates to grain and root hydraulic conductivity.
Wheat genotypes also differ in their stomatal sensitivity to leaf ABA content, as demonstrated by Blum and Sinmena (1995) through a transpiration bioassay. Thus, differences in stomatal sensitivity to ABA concentration could either counteract or uphold the effect of differences in ABA accumulation among genotypes. Despite clear evidence for genotypic variation in ABA accumulation and sensitivity, information on its impact on stomatal regulation and water use to sustain grain yield is very limited. The potential role of ABA in improving WUE is highlighted by the differential performance of four transgenic wheat lines with and without expression of ABA-responsive barley genes (Sivamani et al., 2000). Recently, we found consistent variation between two genotypes (Drysdale and IGW-3262), in their relationship between leaf ABA, stomatal conductance, water use and yield (Saradadevi et al., 2014, 2015, 2017). As genotypic variation for the capacity to accumulate ABA and ABA sensitivity is highly heritable and homogeneity can be achieved within a few generations (Quarrie, 1981; Quarrie and Lister, 1983), it is important to explore the causes of these genotypic differences.
Factors Affecting Genotypic Differences in ABA
In the above mentioned studies demonstrating genotypic variation in ABA accumulation, the relationship between leaf ABA and stomatal conductance was stronger in Drysdale, but weaker in IGW-3262. The observed differences between genotypes in the root density distribution in the upper drying soil layer and ABA catabolism was considered as reasons for the differences in leaf ABA and stomatal behavior between these genotypes (Saradadevi et al., 2015). Under well-watered conditions, leaf ABA concentration in IGW-3262 was found to be fluctuating with the relative humidity, but not in Drysdale. Hence genotypic differences in their sensitivity to environmental factors like relative humidity or VPD (Kholova et al., 2010) can also affect ABA accumulation and degradation.
As ABA is not the sole phytohormone involved in stomatal regulation, but several other phytohormones and/or compounds are also involved, the differences among genotypes in the accumulation and sensitivity to ABA might be due to difference among genotypes in the synthesis and degradation of other phytohormones involved in the process. In addition, several factors determine ABA accumulation and the stomatal response to a given concentration of ABA: including the water status of plants, leaf water potential (Quarrie, 1980), leaf turgor (Morgan and King, 1984), soil water status, pH (Slovik et al., 1995; Hartung et al., 2002), soil compaction (Tardieu et al., 1992), environmental factors such as temperature (Wright, 1969; Dodd and Davies, 1994), relative humidity, light or time of day, changes in water flux through the xylem (Slovik et al., 1995) and previous exposure to ABA flux (Atkinson et al., 1989). In addition, the ABA concentration reaching guard cells at any given time can vary due to sequestration, remobilization and degradation and/or conjugation; these mechanisms are not yet fully understood. Furthermore, ABA is mobile within the plant moving up and down the plant; from roots to leaves through xylem and from leaves to roots through phloem. ABA from leaves is also exported to spikes in wheat. Regulation of this movement and its physiological implications is not yet clear (Seo and Koshiba, 2011). Thus, ABA accumulation in response to drought can be confounded effect of two or more of the above factors. In addition, stomata are controlled by several feedback loops (Raschke, 1975) involving external and internal factors such as light, VPD, intercellular CO2 concentration, leaf turgor and soil water status. Finally, the role of ABA is not limited to stomatal regulation, but is involved in many physiological functions from seed germination, growth (Milborrow, 1967), tiller production (Quarrie and Jones, 1977), root hydraulic conductivity (Davies et al., 1982), cell wall rigidity (Davies et al., 1982), pollen sterility, and the determination of yield (Travaglia et al., 2007). The involvement of ABA in stomatal regulation and drought adaptation is equivocal, but the above suggested intricacies have limited the understanding of the complex mechanism of ABA-mediated plant responses under water stress. Recent advances in ABA-related research explores biochemical, molecular and genetic aspects of ABA signaling like ABA biosynthesis, ABA receptors, their structures, mechanics of binding ABA to receptors, ABA transporters, gene expressions and transcription factors [reviewed by Cutler et al. (2010), Hubbard et al. (2010), Klingler et al. (2010), Weiner et al. (2010) and Sah et al. (2016)]. Rapid advances in genomic aspects of ABA widens the gap between genomic and physiological information available on ABA in relation to drought adaptation and crop improvement (Blum, 2015). Hence attention is required to explore physiological significance of ABA in combating drought and maintaining grain yield.
The Effect of Stomatal Regulation on Yield
The main focus of any wheat breeding program is grain yield improvement. Unfortunately, many favorable plant responses to moisture stress have negative effects on grain yield (Schmidt, 1983; Turner, 1986). For instance, Blum and Johnson (1993) showed a negative impact on grain yield of wheat cultivars with reduced stomatal conductance in response to the dry top soil. This may be due to reduced photosynthesis due to stomatal closure or the adverse effect of ABA on pollen sterility showed in other studies (Morgan and King, 1984).
Stomatal Conductance and Photosynthesis
As stomata serve as a portal through which water exits the leaf and CO2 diffuses into leaf tissue for photosynthesis, stomatal regulation to limit water use during water deficit is at the expense of CO2 diffusion into leaf tissue which subsequently reduces photosynthesis (Chaves et al., 2009). In addition to the reduced CO2 influx, soil water deficits reduce mesophyll conductance of CO2 limiting photosynthesis (Flexas et al., 2004). As the relative water content decreases due to soil water deficit, decreased ATP synthesis and consequent RuBP synthesis causes metabolic limitation of photosynthesis (Lawlor, 2002; Lawlor and Cornic, 2002). Interestingly, the rate of reduction of CO2 assimilation is comparatively less than the reduction in transpiration (Holaday et al., 1992). In well-adapted plants, the stomatal role in controlling photosynthesis is not more than 20% of the total photosynthetic inhibition (Jones, 1998). So the positive effect of reduced transpiration may outweigh the negative effect of decreased photosynthesis under terminal drought conditions. A low canopy conductance to facilitate water availability for uptake during the reproductive stage is proposed as an important trait to maintain grain yield in chickpea under terminal drought conditions (Guóth et al., 2010).
Impact of ABA on Yield
Reduction in grain set due to drought has been associated with increased ABA concentrations in leaves and spikes (Morgan and King, 1984). Yield reduction in response to exogenous ABA application supports the negative impact of ABA on grain set and yield (Morgan, 1980). A reduction in the number of grains per ear in genotypes selected for high leaf ABA levels, even under well-watered conditions, suggests that leaf ABA negatively influences wheat pollination (Innes et al., 1984). Therefore, grain yield reduction is observed when the water deficit coincides with pollen mother cell meiosis and not during later developmental stage (Saini and Aspinall, 1981). Furthermore, ABA-associated pollen sterility is more pronounced in drought-sensitive wheat genotypes compared to drought-tolerant ones (Ji et al., 2011). In a split-root study, where the dry half of the root system contributed to increased leaf ABA while the other half supplied water to maintain leaf water potential, no yield reduction was noticed (Dembinska et al., 1992). This suggests that endogenous ABA is not the sole factor affecting grain set under drought.
Conversely, ABA reportedly has a positive influence on grain yield by affecting the redistribution of carbohydrates from the shoot into wheat grain (Travaglia et al., 2007). Increased grain yield by foliar application of ABA in a wheat field was confirmed by Travaglia et al. (2010). This disagrees with the findings of King and Patrick (1982), where no such involvement of ABA in assimilate transport to grain was observed. No grain yield benefit resulted from drenching soil with exogenous ABA (Du et al., 2013). The lack of consensus among researchers in relation to a positive, negative or neutral influence of ABA on grain yield suggests that the timing at which the water stress occurs is important. Pre-anthesis water stress, particularly during spike development and pollen meiosis, reduced grain number while post-anthesis water stress reduced grain size (Dolferus et al., 2011). This is because high ABA levels during the early reproductive stage affect grain set and reduces grain number while during post-anthesis stages, it promotes grain filling by redistributing reserved carbohydrates to the grain (Liu et al., 2005). In a recent study, when the soil water was exhausted rapidly after anthesis, the wheat cultivar Drysdale maintained a higher grain yield with higher harvest index and grain weight compared to the advanced drought-tolerant line IGW-3262 (Saradadevi et al., 2015). This was mainly because the cultivar Drysdale was more efficient at translocation of assimilate to grain (Saradadevi et al., 2015). Based on the above studies, it appears that ABA negatively affects grain set, but has a positive effect on grain filling by facilitating assimilate partitioning to grain.
Conclusion
Stomatal regulation is an important mechanism that controls water use and maintain grain yield under terminal drought, a abiotic major stress affecting wheat grain yield. The role of ABA in regulating gs under water deficit has been explored extensively in crops such as maize, tomato and sunflower, with limited studies in wheat and no clear consensus on the mechanisms of ABA-mediated stomatal regulation. With contrasting evidence on the relationship between leaf and xylem ABA and stomatal conductance in species like maize and wheat, research should focus on the factors affecting these specific differences and the possibility of exploiting genotypic variation in ABA accumulation as a surrogate characteristic for improving effective water use in wheat to sustain grain yield under terminal drought. Ease of collecting leaf samples to quantify ABA compared to extracting xylem sap will facilitate the rapid screening of a large number of germplasm for drought tolerance.
Author Contributions
RS has performed data acquisition, analysis and interpretation of the data and drafting of the manuscript. RS is the corresponding author of this manuscript. KS and JP conceived the idea, assisted in data interpretation, and critically reviewed and edited the manuscript.
Funding
RS thanks Endeavour Scholarships, the UWA School of Plant Biology and the UWA Institute of Agriculture for funding this project.
Conflict of Interest Statement
The authors declare that the research was conducted in the absence of any commercial or financial relationships that could be construed as a potential conflict of interest.
References
Acharya, B. R., and Assmann, S. M. (2009). Hormone interactions in stomatal function. Plant Mol. Biol. 69, 451–462. doi: 10.1007/s11103-008-9427-0
Ali, M., Jensen, C. R., and Mogensen, V. O. (1998). Early signals in field grown wheat in response to shallow soil drying. Funct. Plant Biol. 25, 871–882. doi: 10.1071/pp98061
Asseng, S., Jamieson, P. D., Kimball, B., Pinter, P., Sayre, K., Bowden, J. W., et al. (2004). Simulated wheat growth affected by rising temperature, increased water deficit and elevated atmospheric CO2. Field Crops Res. 85, 85–102. doi: 10.1016/s0378-4290(03)00154-0
Atkinson, C. J., Davies, W. J., and Mansfield, T. A. (1989). Changes in stomatal conductance in intact ageing wheat leaves in response to abscisic acid. J. Exp. Bot. 40, 1021–1028. doi: 10.1093/jxb/40.9.1021
Atwell, B. J., Kriedemann, P. E., and Turnbull, C. G. N. (eds). (1999). “Water: a limiting factor,” in Plants in Action: Adaptation in Nature, Performance in Cultivation (Melbourne: Macmillian Education Australia Pty Ltd).
Bacon, M. A., Wilkinson, S., and Davies, W. J. (1998). pH-regulated leaf cell expansion in droughted plants is abscisic acid dependent. Plant Physiol. 118, 1507–1515. doi: 10.1104/pp.118.4.1507
Bahrun, A., Jensen, C. R., Asch, F., and Mogensen, V. O. (2002). Drought-induced changes in xylem pH, ionic composition, and ABA concentration act as early signals in field-grown maize (Zea mays L.). J. Exp. Bot. 53, 251–263. doi: 10.1093/jexbot/53.367.251
Bauer, H., Ache, P., Lautner, S., Fromm, J., Hartung, W., Al-Rasheid, K. A. S., et al. (2013). The stomatal response to reduced relative humidity requires guard cell-autonomous ABA synthesis. Curr. Biol. 23, 53–57. doi: 10.1016/j.cub.2012.11.022
Blackman, P. G., and Davies, W. J. (1983). The effects of cytokinins and ABA on stomatal behaviour of maize and Commelina. J. Exp. Bot. 34, 1619–1626. doi: 10.1093/jxb/34.12.1619
Blackman, P. G., and Davies, W. J. (1985). Root to shoot communication in maize plants of the effects of soil drying. J. Exp. Bot. 36, 39–48. doi: 10.1093/jxb/36.1.39
Blum, A. (1998). Improving wheat grain filling under stress by stem reserve mobilisation. Euphytica 100, 77–83. doi: 10.1023/a:1018303922482
Blum, A. (2009). Effective use of water (EUW) and not water-use efficiency (WUE) is the target of crop yield improvement under drought stress. Field Crops Res. 112, 119–123. doi: 10.1016/j.fcr.2009.03.009
Blum, A. (2015). Towards a conceptual ABA ideotype in plant breeding for water limited environments. Funct. Plant Biol. 42, 502–513. doi: 10.1071/FP14334
Blum, A., and Johnson, J. W. (1993). Wheat cultivars respond differently to a drying top soil and a possible non-hydraulic root signal. J. Exp. Bot. 44, 1149–1153. doi: 10.1093/jxb/44.7.1149
Blum, A., and Sinmena, B. (1995). Isolation and characterization of variant wheat cultivars for ABA sensitivity. Plant Cell Environ. 18, 77–83. doi: 10.1111/j.1365-3040.1995.tb00546.x
Blum, A., Zhang, J., and Nguyen, H. T. (1999). Consistent differences among wheat cultivars in osmotic adjustment and their relationship to plant production. Field Crops Res. 64, 287–291. doi: 10.1016/S0378-4290(99)00064-7
Borel, C., and Simonneau, T. (2002). Is the ABA concentration in the sap collected by pressurizing leaves relevant for analysing drought effects on stomata? Evidence from ABA-fed leaves of transgenic plants with modified capacities to synthesize ABA. J. Exp. Bot. 53, 287–296. doi: 10.1093/jexbot/53.367.287
Boyer, J. S. (1985). Water transport. Annu. Rev. Plant Physiol. 36, 473–516. doi: 10.1146/annurev.pp.36.060185.002353
Bramley, H., Turner, N. C., Turner, D. W., and Tyerman, S. D. (2009). Roles of morphology, anatomy, and aquaporins in determining contrasting hydraulic behavior of roots. Plant Physiol. 150, 348–364. doi: 10.1104/pp.108.134098
Canny, M., and McCully, M. (1988). The xylem sap of maize roots: its collection, composition and formation. Funct. Plant Biol. 15, 557–566. doi: 10.1071/pp9880557
Chaves, M. M., Flexas, J., and Pinheiro, C. (2009). Photosynthesis under drought and salt stress: regulation mechanisms from whole plant to cell. Ann. Bot. 103, 551–560. doi: 10.1093/aob/mcn125
Christmann, A., Weiler, E. W., Steudle, E., and Grill, E. (2007). A hydraulic signal in root-to-shoot signalling of water shortage. Plant J. 52, 167–174. doi: 10.1111/j.1365-313X.2007.03234.x
Comstock, J. P. (2002). Hydraulic and chemical signalling in the control of stomatal conductance and transpiration. J. Exp. Bot. 53, 195–200. doi: 10.1093/jexbot/53.367.195
Cowan, I. R., Raven, J. A., Hartung, W., and Farquhar, G. D. (1982). A possible role for abscisic acid in coupling stomatal conductance and photosynthetic carbon metabolism in leaves. Funct. Plant Biol. 9, 489–498. doi: 10.1071/pp9820489
Cramer, M. D., and Lewis, O. A. M. (1993). The influence of nitrate and ammonium nutrition on the growth of wheat (Triticum aestivum) and maize (Zea mays) plants. Ann. Bot. 72, 359–365. doi: 10.1006/anbo.1993.1119
Creelman, R. A., and Zeevaart, J. A. D. (1984). Incorporation of oxygen into abscisic acid and phaseic acid from molecular oxygen. Plant Physiol. 75, 166–169.
Cutler, S. R., Rodriguez, P. L., Finkelstein, R. R., and Abrams, S. R. (2010). Abscisic acid: emergence of a core signaling network. Annu. Rev. Plant Biol. 61, 651–679. doi: 10.1146/annurev-arplant-042809-112122
Davies, W. J., Rodriguez, J. L., and Fiscus, E. L. (1982). Stomatal behaviour and water movement through roots of wheat plants treated with abscisic acid. Plant Cell Environ. 5, 485–493. doi: 10.1111/1365-3040.ep11611847
Davies, W. J., and Zhang, J. (1991). Root signals and the regulation of growth and development of plants in drying soil. Annu. Rev. Plant Physiol. Plant Mol. Biol. 42, 55–76. doi: 10.1146/annurev.pp.42.060191.000415
Dembinska, O., Lalonde, S., and Saini, H. S. (1992). Evidence against the regulation of grain set by spikelet abscisic acid levels in water-stressed wheat. Plant Physiol. 100, 1599–1602. doi: 10.1104/pp.100.3.1599
Dias de Oliveira, E., Bramley, H., Siddique, K. H. M., Henty, S., Berger, J., and Palta, J. A. (2013). Can elevated CO2 combined with high temperature ameliorate the effect of terminal drought in wheat? Funct. Plant Biol. 40, 160–171. doi: 10.1071/FP12206
Dietz, K. J., Sauter, A., Wichert, K., Messdaghi, D., and Hartung, W. (2000). Extracellular β-glucosidase activity in barley involved in the hydrolysis of ABA glucose conjugate in leaves. J. Exp. Bot. 51, 937–944.
Dodd, I. C. (2007). Soil moisture heterogeneity during deficit irrigation alters root-to-shoot signalling of abscisic acid. Funct. Plant Biol. 34, 439–448. doi: 10.1071/FP07009
Dodd, I. C. (2013). Abscisic acid and stomatal closure: a hydraulic conductance conundrum? New Phytol. 197, 6–8. doi: 10.1111/nph.12052
Dodd, I. C., and Davies, W. J. (1994). Leaf growth responses to ABA are temperature dependent. J. Exp. Bot. 45, 903–907. doi: 10.1093/jxb/45.7.903
Dodd, I. C., and Davies, W. J. (1996). The relationship between leaf growth and ABA accumulation in the grass leaf elongation zone. Plant Cell Environ. 19, 1047–1056. doi: 10.1111/j.1365-3040.1996.tb00211.x
Dodd, I. C., Egea, G., and Davies, W. J. (2008a). Abscisic acid signalling when soil moisture is heterogeneous: decreased photoperiod sap flow from drying roots limits abscisic acid export to the shoots. Plant Cell Environ. 31, 1263–1274. doi: 10.1111/j.1365-3040.2008.01831.x
Dodd, I. C., Egea, G., and Davies, W. J. (2008b). Accounting for sap flow from different parts of the root system improves the prediction of xylem ABA concentration in plants grown with heterogeneous soil moisture. J. Exp. Bot. 59, 4083–4093. doi: 10.1093/jxb/ern246
Dodd, I. C., Stikic, R., and Davies, W. J. (1996). Chemical regulation of gas exchange and growth of plants in drying soil in the field. J. Exp. Bot. 47, 1475–1490. doi: 10.1093/jxb/47.10.1475
Dodd, I. C., Theobald, J. C., Bacon, M. A., and Davies, W. J. (2006). Alternation of wet and dry sides during partial rootzone drying irrigation alters root-to-shoot signalling of abscisic acid. Funct. Plant Biol. 33, 1081–1089. doi: 10.1071/FP06203
Dolferus, R., Ji, X., and Richards, R. A. (2011). Abiotic stress and control of grain number in cereals. Plant Sci. 181, 331–341. doi: 10.1016/j.plantsci.2011.05.015
Du, Y. L., Wang, Z. Y., Fan, J. W., Turner, N. C., He, J., Wang, T., et al. (2013). Exogenous abscisic acid reduces water loss and improves antioxidant defence, desiccation tolerance and transpiration efficiency in two spring wheat cultivars subjected to a soil water deficit. Funct. Plant Biol. 40, 494–506. doi: 10.1071/FP12250
Else, M. A., Davies, W. J., Whitford, P. N., Hall, K. C., and Jackson, M. B. (1994). Concentrations of abscisic acid and other solutes in xylem sap from root systems of tomato and castor-oil plants are distorted by wounding and variable sap flow rates. J. Exp. Bot. 45, 317–323. doi: 10.1093/jxb/45.3.317
Else, M. A., Hall, K. C., Arnold, G. M., Davies, W. J., and Jackson, M. B. (1995). Export of abscisic acid, 1-aminocyclopropane-1-carboxylic acid, phosphate, and nitrate from roots to shoots of flooded tomato plants. Accounting for effects of xylem sap flow rate on concentration and delivery. Plant Physiol. 107, 377–384. doi: 10.1104/pp.107.2.377
FAO. (2013). FAO Statistical Year Book 2013 - World Food and Agriculture. Available at: http://www.fao.org/economic/ess/ess-publications/ess-yearbook/en/\#.VIuQ4Mm6a2o
FAO (2015). FAO Statistical Pocketbook 2015. Available at: http://www.fao.org/3/a-i4691e.pdf
Farre, I., and Foster, I. (2010). “Impact of climate change on wheat yields in Western Australia. Will wheat production be more risky in the future?,” in Proceedings of the 15th Agronomy Conference, eds H. Dove and R. A. Culvenor (Christchurch: Food Security from Sustainable Agriculture).
Farquhar, G. D., and Sharkey, T. D. (1982). Stomatal conductance and photosynthesis. Annu. Rev. Plant Physiol. 33, 317–345. doi: 10.1146/annurev.pp.33.060182.001533
Fischer, R. A., and Kohn, G. D. (1966). The relationship of grain yield to vegetative growth and post-flowering leaf area in the wheat crop under conditions of limited soil moisture. Aust. J. Agric. Res. 17, 281–295. doi: 10.1071/AR9660281
Flexas, J., Bota, J., Loreto, F., Cornic, G., and Sharkey, T. D. (2004). Diffusive and metabolic limitations to photosynthesis under drought and salinity in C3 plants. Plant Biol. 6, 269–279.
Foresight (2011). The Future of Food and Farming: Challenges and Choices for Global Sustainability. The Final Project Report. London: The Government Office for Science.
Freundl, E., Steudle, E., and Hartung, W. (1998). Water uptake by roots of maize and sunflower affects the radial transport of abscisic acid and its concentration in the xylem. Planta 207, 8–19. doi: 10.1007/s004250050450
Gollan, T., Passioura, J. B., and Munns, R. (1986). Soil water status affects the stomatal conductance of fully turgid wheat and sunflower leaves. Funct. Plant Biol. 13, 459–464.
Goodger, J. Q. D., Sharp, R. E., Marsh, E. L., and Schachtman, D. P. (2005). Relationships between xylem sap constituents and leaf conductance of well-watered and water-stressed maize across three xylem sap sampling techniques. J. Exp. Bot. 56, 2389–2400. doi: 10.1093/jxb/eri231
Guóth, A., Benyó, D., Csiszár, J., Gallé,Á, Horváth, F., Cseuz, L., et al. (2010). Relationship between osmotic stress-induced abscisic acid accumulation, biomass production and plant growth in drought-tolerant and -sensitive wheat cultivars. Acta Physiol. Plant. 32, 719–727. doi: 10.1007/s11738-009-0453-6
Hansen, H., and Dörffling, K. (1999). Changes of free and conjugated abscisic acid and phaseic acid in xylem sap of drought-stressed sunflower plants. J. Exp. Bot. 50, 1599–1605. doi: 10.1093/jxb/50.339.1599
Harris, M. J., Outlaw, W. H., Mertens, R., and Weiler, E. W. (1988). Water-stress-induced changes in the abscisic acid content of guard cells and other cells of Vicia faba L. leaves as determined by enzyme-amplified immunoassay. Proc. Natl. Acad. Sci. U.S.A. 85, 2584–2588.
Harrison, M. A., and Walton, D. C. (1975). Abscisic acid metabolism in water-stressed bean leaves. Plant Physiol. 56, 250–254. doi: 10.1104/pp.56.2.250
Hartung, W., Radin, J. W., and Hendrix, D. L. (1988). Abscisic acid movement into the apoplastic solution of water-stressed cotton leaves, Role apoplastic pH. Plant Physiol. 86, 908–913. doi: 10.1104/pp.86.3.908
Hartung, W., Sauter, A., and Hose, E. (2002). Abscisic acid in the xylem: where does it come from, where does it go to? J. Exp. Bot. 53, 27–32. doi: 10.1093/jexbot/53.366.27
Hartung, W., and Slovik, S. (1991). Physicochemical properties of plant growth regulators and plant tissues determine their distribution and redistribution: stomatal regulation by abscisic acid in leaves. New Phytol. 119, 361–382. doi: 10.1111/j.1469-8137.1991.tb00036.x
Henson, I. E., Jensen, C. R., and Turner, N. C. (1989a). Leaf gas exchange and water relations of lupins and wheat. I. Shoot responses to soil water deficits. Funct. Plant Biol. 16, 401–413. doi: 10.1071/pp9890401
Henson, I. E., Jensen, C. R., and Turner, N. C. (1989b). Leaf gas exchange and water relations of lupins and wheat. III. Abscisic acid and drought-induced stomatal closure. Funct. Plant Biol. 16, 429–442. doi: 10.1071/pp9890429
Henson, I. E., and Quarrie, S. A. (1981). Abscisic acid accumulation in detached cereal leaves in response to water stress: I. Effects of incubation time and severity of stress. Z. Pflanzenphysiol. 101, 431–438. doi: 10.1016/S0044-328X(81)80082-7
Holaday, S. A., Ritchie, S. W., and Nguyen, H. T. (1992). Effects of water deficit on gas-exchange parameters and ribulose 1,5-bisphosphate carboxylase activation in wheat. Environ. Exp. Bot. 32, 403–410. doi: 10.1016/0098-8472(92)90053-5
Hose, E., Steudle, E., and Hartung, W. (2000). Abscisic acid and hydraulic conductivity of maize roots: a study using cell- and root-pressure probes. Planta 211, 874–882. doi: 10.1007/s004250000412
Hubbard, K. E., Nishimura, N., Hitomi, K., Getzoff, E. D., and Schroeder, J. I. (2010). Early abscisic acid signal transduction mechanisms: newly discovered components and newly emerging questions. Genes Dev. 24, 1695–1708. doi: 10.1101/gad.1953910
Innes, P., Blackwell, R. D., and Quarrie, S. A. (1984). Some effects of genetic variation in drought-induced abscisic acid accumulation on the yield and water use of spring wheat. J. Agric. Sci. 102, 341–351. doi: 10.1017/S0021859600042660
Jeschke, W. D., Holobradá, M., and Hartung, W. (1997). Growth of Zea mays L. plants with their seminal roots only. Effects on plant development, xylem transport, mineral nutrition and the flow and distribution of abscisic acid (ABA) as a possible shoot to root signal. J. Exp. Bot. 48, 1229–1239. doi: 10.1093/jxb/48.6.1229
Ji, X., Dong, B., Shiran, B., Talbot, M. J., Edlington, J. E., Hughes, T., et al. (2011). Control of abscisic acid catabolism and abscisic acid homeostasis is important for reproductive stage stress tolerance in cereals. Plant Physiol. 156, 647–662. doi: 10.1104/pp.111.176164
Jones, H. G. (1998). Stomatal control of photosynthesis and transpiration. J. Exp. Bot. 49, 387–398. doi: 10.1093/jxb/49.Special_Issue.387
Kholova, J., Hash, C. T., Kumar, P. L., Yadav, R. S., Kocova, M., and Vadez, V. (2010). Terminal drought-tolerant pearl millet [Pennisetum glaucum (L.) R. Br.] have high leaf ABA and limit transpiration at high vapour pressure deficit. J. Exp. Bot. 61, 1431–1440. doi: 10.1093/jxb/erq013
King, R. W., and Patrick, J. W. (1982). Control of assimilate movement in wheat. Is abscisic acid involved? Z. Pflanzenphysiol. 106, 375–380. doi: 10.1016/S0044-328X(82)80117-7
Kirkegaard, J. A., Lilley, J. M., Howe, G. N., and Graham, J. M. (2007). Impact of subsoil water use on wheat yield. Aust. J. Agric. Res. 58, 303–315. doi: 10.1071/AR06285
Klingler, J. P., Batelli, G., and Zhu, J.-K. (2010). ABA receptors: the START of a new paradigm in phytohormone signalling. J. Exp. Bot. 61, 3199–3210. doi: 10.1093/jxb/erq151
Kobata, T., Palta, J. A., and Turner, N. C. (1992). Rate of development of postanthesis water deficits and grain filling of spring wheat. Crop Sci. 32, 1238–1242. doi: 10.2135/cropsci1992.0011183X003200050035x
Kramer, P. J. (1980). “Drought, stress, and the origin of adaptations,” in Adaptation of Plants to Water and High Temperature Stress, eds P. J. Kramer and N. C. Turner (New York, NY: John Wiley & Sons), 7–20.
Kudoyarova, G., Veselova, S., Hartung, W., Farhutdinov, R., Veselov, D., and Sharipova, G. (2011). Involvement of root ABA and hydraulic conductivity in the control of water relations in wheat plants exposed to increased evaporative demand. Planta 233, 87–94. doi: 10.1007/s00425-010-1286-7
Lawlor, D. W. (2002). Limitation to photosynthesis in water-stressed leaves: stomata vs. metabolism and the role of ATP. Annal. Bot. 89, 871–885.
Lawlor, D. W., and Cornic, G. (2002). Photosynthetic carbon assimilation and associated metabolism in relation to water deficits in higher plants. Plant Cell Environ. 25, 275–294. doi: 10.1046/j.0016-8025.2001.00814.x
Lee, K. H., Piao, H. L., Kim, H. Y., Choi, S. M., Jiang, F., Hartung, W., et al. (2006). Activation of glucosidase via stress-induced polymerization rapidly increases active pools of abscisic acid. Cell 126, 1109–1120.
Li, B., Feng, Z., Xie, M., Sun, M., Zhao, Y., Liang, L., et al. (2010). Modulation of the root-sourced ABA signal along its way to the shoot in Vitis riparia ×Vitis labrusca under water deficit. J. Exp. Bot. 62, 1731–1741. doi: 10.1093/jxb/erq390
Liang, J., Zhang, J., and Wong, M. H. (1997). How do roots control xylem sap ABA concentration in response to soil drying? Plant Cell Physiol. 38, 10–16.
Liu, F., Jensen, C. R., and Andersen, M. N. (2005). A review of drought adaptation in crop plants: changes in vegetative and reproductive physiology induced by ABA-based chemical signals. Aust. J. Agric. Res. 56, 1245–1252. doi: 10.1071/AR05062
Loveys, B. R., and Kriedemann, P. E. (1974). Internal control of stomatal physiology and photosynthesis. I. Stomatal regulation and associated changes in endogenous levels of abscisic and phaseic acids. Funct. Plant Biol. 1, 407–415. doi: 10.1071/pp9740407
MacRobbie, E. A. C. (1980). Osmotic measurements on stomatal cells of Commelina communis L. J. Membr. Biol. 53, 189–198. doi: 10.1007/BF01868824
Manschadi, A. M., Christopher, J., deVoil, P., and Hammer, G. L. (2006). The role of root architectural traits in adaptation of wheat to water-limited environments. Funct. Plant Biol. 33, 823–837. doi: 10.1071/FP06055
Martin-Vertedor, A. I., and Dodd, I. C. (2011). Root-to-shoot signalling when soil moisture is heterogeneous: increasing the proportion of root biomass in drying soil inhibits leaf growth and increases leaf abscisic acid concentration. Plant Cell Environ. 34, 1164–1175. doi: 10.1111/j.1365-3040.2011.02315.x
Milborrow, B. V. (1967). The identification of (+)-abscisin II [(+)-dormin] in plants and measurement of its concentrations. Planta 76, 93–113. doi: 10.1007/bf00385456
Mitchell, J. H., Rebetzke, G. J., Chapman, S. C., and Fukai, S. (2013). Evaluation of reduced-tillering (tin) wheat lines in managed, terminal water deficit environments. J. Exp. Bot. 64, 3439–3451. doi: 10.1093/jxb/ert181
Mittelheuser, C. J., and Van Steveninck, R. F. M. (1969). Stomatal closure and inhibition of transpiration induced by (RS)-abscisic acid. Nature 221, 281–282. doi: 10.1038/221281a0
Morgan, J. M. (1980). Possible role of abscisic acid in reducing seed set in water-stressed wheat plants. Nature 285, 655–657. doi: 10.1038/285655a0
Morgan, J. M., and Condon, A. G. (1986). Water use, grain yield, and osmoregulation in wheat. Funct. Plant Biol. 13, 523–532. doi: 10.1071/pp9860523
Morgan, J. M., and King, R. W. (1984). Association between loss of leaf turgor, abscisic acid levels and seed set in two wheat cultivars. Funct. Plant Biol. 11, 143–150. doi: 10.1071/pp9840143
Munns, R. (1992). A leaf elongation assay detects an unknown growth inhibitor in xylem sap from wheat and barley. Funct. Plant Biol. 19, 127–135. doi: 10.1071/pp9920127
Munns, R., and King, R. W. (1988). Abscisic acid is not the only stomatal inhibitor in the transpiration stream of wheat plants. Plant Physiol. 88, 703–708. doi: 10.1104/pp.88.3.703
Munns, R., Passioura, J. B., Milborrow, B. V., James, R. A., and Close, T. J. (1993). Stored xylem sap from wheat and barley in drying soil contains a transpiration inhibitor with a large molecular size. Plant Cell Environ. 16, 867–872. doi: 10.1111/j.1365-3040.1993.tb00509.x
Munns, R., and Sharp, R. E. (1993). Involvement of abscisic acid in controlling plant growth in soil of low water potential. Aust. J. Plant Physiol. 20, 425–437. doi: 10.1071/PP9930425
Netting, A. G., Theobald, J. C., and Dodd, I. C. (2012). Xylem sap collection and extraction methodologies to determine in vivo concentrations of ABA and its bound forms by gas chromatography-mass spectrometry (GC-MS). Plant Methods 8:11. doi: 10.1186/1746-4811-8-11
Newman, E. I. (1976). Water movement through root systems. Philos. Trans. R. Soc. Lond. B Biol. Sci. 273, 463–478. doi: 10.1098/rstb.1976.0025
Palta, J., and Watt, M. (2009). “Vigorous crop root systems: form and function for improving the capture of water and nutrients,” in Crop Physiology. Applications for Genetic Improvement and Agronomy, eds V. C. Sadras and D. Calderini (New York, NY: Elsevier). doi: 10.1016/b978-0-12-374431-9.00013-x
Palta, J. A., Kobata, T., Turner, N. C., and Fillery, I. R. (1994). Remobilization of carbon and nitrogen in wheat as influenced by postanthesis water deficits. Crop Sci. 34, 118–124. doi: 10.2135/cropsci1994.0011183X003400010021x
Passioura, J. B. (1983). Roots and drought resistance. Agric. Water Manag. 7, 265–280. doi: 10.1016/0378-3774(83)90089-6
Passioura, J. B. (1988). Root signals control leaf expansion in wheat seedlings growing in drying soil. Funct. Plant Biol. 15, 687–693. doi: 10.1071/pp9880687
Pei, Z.-M., Ghassemian, M., Kwak, C. M., McCourt, P., and Schroeder, J. I. (1998). Role of farnesyltransferase in ABA regulation of guard cell anion channels and plant water loss. Science 282, 287–290. doi: 10.1126/science.282.5387.287
Pheloung, P. C., and Siddique, K. H. M. (1991). Contribution of stem dry matter to grain yield in wheat cultivars. Funct. Plant Biol. 18, 53–64. doi: 10.1071/pp9910053
Popova, L. P., Outlaw, W. H. Jr., Aghoram, K., and Hite, D. R. C. (2000). Abscisic acid – an intraleaf water-stress signal. Physiol. Plant. 108, 376–381. doi: 10.1034/j.1399-3054.2000.t01-1-100406.x
Quarrie, S. A. (1980). Genotypic differences in leaf water potential, abscisic acid and proline concentrations in spring wheat during drought stress. Ann. Bot. 46, 383–394. doi: 10.1093/oxfordjournals.aob.a085929
Quarrie, S. A. (1981). Genetic variability and heritability of drought-induced abscisic acid accumulation in spring wheat. Plant Cell Environ. 4, 147–151. doi: 10.1111/j.1365-3040.1981.tb01036.x
Quarrie, S. A., and Henson, I. E. (1981). Abscisic acid accumulation in detached cereal leaves in response to water stress: II. Effects of leaf age and leaf position. Z. Pflanzenphysiol. 101, 439–446. doi: 10.1016/S0044-328X(81)80083-9
Quarrie, S. A., and Jones, H. G. (1977). Effects of abscisic acid and water stress on development and morphology of wheat. J. Exp. Bot. 28, 192–203. doi: 10.1093/jxb/28.1.192
Quarrie, S. A., and Lister, P. G. (1983). Characterization of spring wheat genotypes differing in drought-induced abscisic acid accumulation. J. Exp. Bot. 34, 1260–1270. doi: 10.1093/jxb/34.10.1260
Rajala, A., Hakala, K., Mäkelä, P., Muurinen, S., and Peltonen-Sainio, P. (2009). Spring wheat response to timing of water deficit through sink and grain filling capacity. Field Crops Res. 114, 263–271. doi: 10.1016/j.fcr.2009.08.007
Raschke, K. (1975). Stomatal action. Annu. Rev. Plant Physiol. 26, 309–340. doi: 10.1146/annurev.pp.26.060175.001521
Richards, R. A., and Passioura, J. B. (1989). A breeding program to reduce the diameter of the major xylem vessel in the seminal roots of wheat and its effect on grain yield in rain-fed environments. Aust. J. Agric. Res. 40, 943–950. doi: 10.1071/AR9890943
Sah, S. K., Reddy, K. R., and Li, J. (2016). Abscisic acid and abiotic stress tolerance in crop plants. Front. Plant Sci. 7:571. doi: 10.3389/fpls.2016.00571
Saini, H. S., and Aspinall, D. (1981). Effect of water deficit on sporogenesis in wheat (Triticum aestivum L.). Annal. Bot. 48, 623–633. doi: 10.1093/oxfordjournals.aob.a086170
Saradadevi, R., Bramley, H., Palta, J. A., Edwards, E., and Siddique, K. H. M. (2015). Root biomass in the upper layer of the soil profile is related to the stomatal response of wheat as the soil dries. Funct. Plant Biol. 43, 62–74. doi: 10.1071/fp15216
Saradadevi, R., Bramley, H., Palta, J. A., and Siddique, K. H. M. (2017). Stomatal behaviour under terminal drought affects post-anthesis water use in wheat. Funct. Plant Biol. 44, 279–289. doi: 10.1071/FP16078
Saradadevi, R., Bramley, H., Siddique, K. H. M., Edwards, E., and Palta, J. A. (2014). Contrasting stomatal regulation and leaf ABA concentrations in wheat genotypes when split root systems were exposed to terminal drought. Field Crops Res. 162, 77–86. doi: 10.1016/j.fcr.2014.02.004
Sauter, A., Dietz, K. J., and Hartung, W. (2002). A possible stress physiological role of abscisic acid conjugates in root-to-shoot signalling. Plant Cell Environ. 25, 223–228. doi: 10.1046/j.1365-3040.2002.00747.x
Sauter, A., and Hartung, W. (2002). The contribution of internode and mesocotyl tissues to root-to-shoot signalling of abscisic acid. J. Exp. Bot. 53, 297–302. doi: 10.1093/jexbot/53.367.297
Schachtman, D. P., and Goodger, J. Q. D. (2008). Chemical root to shoot signaling under drought. Trends Plant Sci. 13, 281–287. doi: 10.1016/j.tplants.2008.04.003
Schmidt, J. W. (1983). Drought resistance and wheat breeding. Agric. Water Manag. 7, 181–194. doi: 10.1016/0378-3774(83)90082-3
Schroeder, J. I., and Nambara, E. (2006). A quick release mechanism for abscisic acid. Cell 126, 1023–1025.
Schurr, U. (1998). Xylem sap sampling—new approaches to an old topic. Trends Plant Sci. 3, 293–298. doi: 10.1016/s1360-1385(98)01275-8
Schurr, U., Gollan, T., and Schulze, E. D. (1992). Stomatal response to drying soil in relation to changes in the xylem sap composition of Helianthus annuus. II. Stomatal sensitivity to abscisic acid imported from the xylem sap. Plant Cell Environ. 15, 561–567. doi: 10.1111/j.1365-3040.1992.tb01489.x
Seo, M., and Koshiba, T. (2011). Transport of ABA from the site of biosynthesis to the site of action. J. Plant Res. 124, 501–507. doi: 10.1007/s10265-011-0411-4
Serraj, R., and Sinclair, T. R. (2002). Osmolyte accumulation: can it really help increase crop yield under drought conditions? Plant Cell Environ. 25, 333–341. doi: 10.1046/j.1365-3040.2002.00754.x
Siddique, K. H. M., Belford, R. K., Perry, M. W., and Tennant, D. (1989). Growth, development and light interception of old and modern wheat cultivars in a Mediterranean-type environment. Aust. J. Agric. Res. 40, 473–487.
Sivamani, E., Bahieldin, A., Wraith, J. M., Al-Niemi, T., Dyer, W. E., Ho, T. D., et al. (2000). Improved biomass productivity and water use efficiency under water deficit conditions in transgenic wheat constitutively expressing the barley HVA1 gene. Plant Sci. 155, 1–9.
Slovik, S., Daeter, W., and Hartung, W. (1995). Compartmental redistribution and long-distance transport of abscisic acid (ABA) in plants as influenced by environmental changes in the rhizosphere —a biomathematical model. J. Exp. Bot. 46, 881–894. doi: 10.1093/jxb/46.8.881
Sobeih, W. Y., Dodd, I. C., Bacon, M. A., Grierson, D., and Davies, W. J. (2004). Long-distance signals regulating stomatal conductance and leaf growth in tomato (Lycopersicon esculentum) plants subjected to partial root-zone drying. J. Exp. Bot. 55, 2353–2363. doi: 10.1093/jxb/erh204
Speirs, J., Binney, A., Collins, M., Edwards, E., and Loveys, B. (2013). Expression of ABA synthesis and metabolism genes under different irrigation strategies and atmospheric VPDs is associated with stomatal conductance in grapevine (Vitis vinifera L. cv Cabernet Sauvignon). J. Exp. Bot. 64, 1907–1916. doi: 10.1093/jxb/ert052
Steudle, E., and Peterson, C. A. (1998). How does water get through roots? J. Exp. Bot. 49, 775–788. doi: 10.1093/jxb/49.322.775
Tambussi, E. A., Bort, J., and Araus, J. L. (2007). Water use efficiency in C3 cereals under Mediterranean conditions: a review of physiological aspects. Ann. Appl. Biol. 150, 307–321. doi: 10.1111/j.1744-7348.2007.00143.x
Tardieu, F., and Davies, W. J. (1992). Stomatal response to abscisic acid is a function of current plant water status. Plant Physiol. 98, 540–545. doi: 10.1104/pp.98.2.540
Tardieu, F., and Davies, W. J. (1993). Integration of hydraulic and chemical signalling in the control of stomatal conductance and water status of droughted plants. Plant Cell Environ. 16, 341–349. doi: 10.1111/j.1365-3040.1993.tb00880.x
Tardieu, F., Katerji, N., Bethenod, O., Zhang, J., and Davies, W. J. (1991). Maize stomatal conductance in the field: its relationship with soil and plant water potentials, mechanical constraints and ABA concentration in the xylem sap. Plant Cell Environ. 14, 121–126. doi: 10.1111/j.1365-3040.1991.tb01378.x
Tardieu, F., Lafarge, T., and Simonneau, T. (1996). Stomatal control by fed or endogenous xylem ABA in sunflower: interpretation of correlations between leaf water potential and stomatal conductance in anisohydric species. Plant Cell Environ. 19, 75–84. doi: 10.1111/j.1365-3040.1996.tb00228.x
Tardieu, F., Zhang, J., Katerji, N., Bethenod, O., Palmer, S., and Davies, W. J. (1992). Xylem ABA controls the stomatal conductance of field-grown maize subjected to soil compaction or soil drying. Plant Cell Environ. 15, 193–197. doi: 10.1111/j.1365-3040.1992.tb01473.x
Travaglia, C., Cohen, A. C., Reinoso, H., Castillo, C., and Bottini, R. (2007). Exogenous abscisic acid increases carbohydrate accumulation and redistribution to the grains in wheat grown under field conditions of soil water restriction. J. Plant Growth Regul. 26, 285–289. doi: 10.1007/s00344-007-9018-3
Travaglia, C., Reinoso, H., Cohen, A., Luna, C., Tommasino, E., Castillo, C., et al. (2010). Exogenous ABA increases yield in field-grown wheat with moderate water restriction. J. Plant Growth Regul. 29, 366–374. doi: 10.1007/s00344-010-9147-y
Turner, N. C. (1986). Crop water deficits: a decade of progress. Adv. Agron Vol. 39, 1–51. doi: 10.1016/s0065-2113(08)60464-2
Turner, N. C., and Asseng, S. (2005). Productivity, sustainability, and rainfall-use efficiency in Australian rainfed Mediterranean agricultural systems. Aust. J. Agric. Res. 56, 1123–1136. doi: 10.1071/AR05076
Turner, N. C., and Begg, J. E. (1981). Plant-water relations and adaptation to stress. Plant Soil 58, 97–131. doi: 10.1007/bf02180051
Vysotskaya, L. B., Arkhipova, T. N., Timergalina, L. N., Dedov, A. V., Veselov, S. Y., and Kudoyarova, G. R. (2004). Effect of partial root excision on transpiration, root hydraulic conductance and leaf growth in wheat seedlings. Plant Physiol. Biochem. 42, 251–255.
Vysotskaya, L. B., Kudoyarova, G. R., Veselov, S., and Jones, H. G. (2003). Unusual stomatal behaviour on partial root excision in wheat seedlings. Plant Cell Environ. 27, 69–77. doi: 10.1046/j.0016-8025.2003.01126.x
Weiner, J. J., Peterson, F. C., Volkman, B. F., and Cutler, S. R. (2010). Structural and functional insights into core ABA signaling. Curr. Opin. Plant Biol. 13, 495–502. doi: 10.1016/j.pbi.2010.09.007
Westgate, M. E., Passioura, J. B., and Munns, R. (1996). Water status and ABA content of floral organs in drought-stressed wheat. Funct. Plant Biol. 23, 763–772. doi: 10.1071/pp9960763
Wilkinson, S., Bacon, M. A., and Davies, W. J. (2007). Nitrate signalling to stomata and growing leaves: interactions with soil drying, ABA, and xylem sap pH in maize. J. Exp. Bot. 58, 1705–1716. doi: 10.1093/jxb/erm021
Wilkinson, S., and Davies, W. J. (1997). Xylem sap pH increase: a drought signal received at the apoplastic face of the guard cell that involves the suppression of saturable abscisic acid uptake by the epidermal symplast. Plant Physiol. 113, 559–573. doi: 10.1104/pp.113.2.559
Wilkinson, S., Kudoyarova, G. R., Veselov, D. S., Arkhipova, T. N., and Davies, W. J. (2012). Plant hormone interactions: innovative targets for crop breeding and management. J. Exp. Bot. 63, 3499–3509. doi: 10.1093/jxb/ers148
Wright, S. T. C. (1969). An increase in the “inhibitor-β” content of detached wheat leaves following a period of wilting. Planta 86, 10–20. doi: 10.1007/bf00385299
Xu, Z.-Y., Lee, K. H., Dong, T., Jeong, J. C., Jin, J. B., Kanno, Y., et al. (2012). A vacuolar β-glucosidase homolog that possesses glucose-conjugated abscisic acid hydrolyzing activity plays an important role in osmotic stress responses in Arabidopsis. Plant Cell 24, 2184–2199. doi: 10.1105/tpc.112.095935
Zeevaart, J. A. D., and Creelman, R. A. (1988). Metabolism and physiology of abscisic acid. Ann. Rev. Plant Physiol. Plant Mol. Biol. 39, 439–473. doi: 10.1146/annurev.arplant.39.1.439
Zhang, J., and Davies, W. J. (1990a). Changes in the concentration of ABA in xylem sap as a function of changing soil water status can account for changes in leaf conductance and growth. Plant Cell Environ. 13, 277–285. doi: 10.1111/j.1365-3040.1990.tb01312.x
Zhang, J., and Davies, W. J. (1990b). Does ABA in the xylem control the rate of leaf growth in soil-dried maize and sunflower plants? J. Exp. Bot. 41, 1125–1132. doi: 10.1093/jxb/41.9.1125
Zhang, J., and Davies, W. J. (1991). Antitranspirant activity in xylem sap of maize plants. J. Exp. Bot. 42, 317–321. doi: 10.1093/jxb/42.3.317
Keywords: abscisic acid, stomatal conductance, water use efficiency, root hydraulic conductivity, grain yield
Citation: Saradadevi R, Palta JA and Siddique KHM (2017) ABA-Mediated Stomatal Response in Regulating Water Use during the Development of Terminal Drought in Wheat. Front. Plant Sci. 8:1251. doi: 10.3389/fpls.2017.01251
Received: 10 February 2017; Accepted: 03 July 2017;
Published: 18 July 2017.
Edited by:
Partha Sarathi Basu, Indian Institute of Pulses Research, IndiaCopyright © 2017 Saradadevi, Palta and Siddique. This is an open-access article distributed under the terms of the Creative Commons Attribution License (CC BY). The use, distribution or reproduction in other forums is permitted, provided the original author(s) or licensor are credited and that the original publication in this journal is cited, in accordance with accepted academic practice. No use, distribution or reproduction is permitted which does not comply with these terms.
*Correspondence: Renu Saradadevi, renusaradadevi@gmail.com