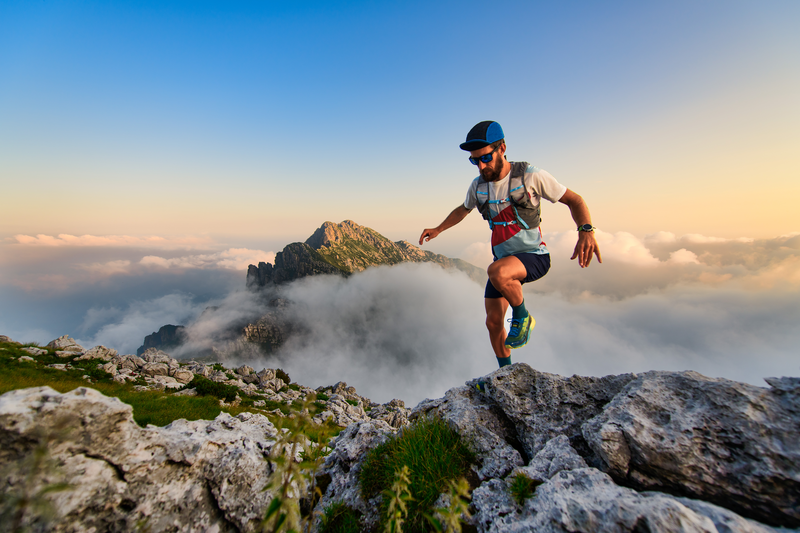
94% of researchers rate our articles as excellent or good
Learn more about the work of our research integrity team to safeguard the quality of each article we publish.
Find out more
REVIEW article
Front. Plant Sci. , 09 May 2017
Sec. Plant Biotechnology
Volume 8 - 2017 | https://doi.org/10.3389/fpls.2017.00750
Plant parasitic nematodes (PPNs) are obligate biotrophic parasites causing serious damage and reduction in crop yields. Several economically important genera parasitize various crop plants. The root-knot, root lesion, and cyst nematodes are the three most economically damaging genera of PPNs on crops within the family Heteroderidae. It is very important to devise various management strategies against PPNs in economically important crop plants. Genetic engineering has proven a promising tool for the development of biotic and abiotic stress tolerance in crop plants. Additionally, the genetic engineering leading to transgenic plants harboring nematode resistance genes has demonstrated its significance in the field of plant nematology. Here, we have discussed the use of genetic engineering for the development of nematode resistance in plants. This review article also provides a detailed account of transgenic strategies for the resistance against PPNs. The strategies include natural resistance genes, cloning of proteinase inhibitor coding genes, anti-nematodal proteins and use of RNA interference to suppress nematode effectors. Furthermore, the manipulation of expression levels of genes induced and suppressed by nematodes has also been suggested as an innovative approach for inducing nematode resistance in plants. The information in this article will provide an array of possibilities to engineer resistance against PPNs in different crop plants.
The word ‘nematode’ comes from the Greek word ‘nema,’ which means thread. Nematodes are thread like, long, cylindrical, sometimes microscopic worms, which can be found in a variety of environments. They belong to a huge phylum of animals called ‘Nematoda’ that comprises of plant and animal parasites, as well as numerous free-living species. They are omnipresent in nature inhabiting in all types of environments and habitats (Ali et al., 2015). However, most of the nematodes are free-living and feed on bacteria, fungi or algae. Some of them invade and parasitize both vertebrates and invertebrates including human beings, thus causing serious health damage and even human death, i.e., guinea worm (Dracunculus medinensis) and intestinal worm (Ascaris lumbricoides) (Decraemer and Hunt, 2006). On the other hand, some of these worms are serious parasites of plants and result in huge crop losses. Phyto-parasitic nematodes count for 7% of total species of the phylum Nematoda from 197 genera and 4300 species that can infect a wide range of economically important crop plants including wheat (Triticum aestivum), potato (Solanum tuberosum), tomato (Solanum lycopersicum), maize (Zea mays), soybean (Glycine max), and sugar beets (Beta vulgaris) (Decraemer and Hunt, 2006).
Most of the nematodes are obligate biotrophic parasites of plants and their infection results in above-ground symptoms in plants like leaf necrosis, chlorosis, plant wilting, stunted growth, and enhanced susceptibility to other pathogens, which mostly occur in patches (Webster, 1995). Penetration of plant parasitic nematodes (PPNs) to the root systems results in the hindrance of translocation of water from roots toward areal plant parts and translocation of nutrients from leaves toward the plant roots. The developing nematodes feed on cell sap and hinder the food supply to the plant physiological systems, which results in stunted growth, yellowing and drooping of leaves. Generally, below-ground symptoms include gall and knot formation in infected roots, root lesions and necrosis and root abbreviations. Figure 1 demonstrates the above- and below-ground symptoms on different plant species. Moreover, in addition to direct damage to crops in the form of yield reduction, these worms serve as disease transmitting vectors for viruses, thereby resulting in crucial economic and social impacts worldwide (Ali et al., 2015).
FIGURE 1. Above-ground and below-ground symptoms from different plant species in response to nematode infections. (A) Soybean plants infected with soybean cyst nematodes (Heterodera glycines) (http://www.extension.umn.edu, reproduced with the permission of Assoc. Prof. Malvick from UMN, US). (B) Infected and uninfected wheat plants with cereal cyst nematode H. avenae (Courtesy Prof. Honglian Li, China, reproduced with permission from Riley et al., 2009). (C–E) Roots of sponge gourd, carrots, and okra infected with root-knot nematode Meloidogyne incognita, respectively. (F) Arabidopsis roots showing development of cysts induced by beet cyst nematode H. schachtii.
The members of family Heteroderidae (Superfamily: Tylenchoidea) are divided into three economically important genera Meloidogyne, Heterodera, and Globodera, which parasitize a huge number of crop plants. These are obligate endo-parasites of host plant roots, enter the plant roots as second stage juvenile larva (J2 larva) and develop specific feeding cells in the plant roots. The members of the genus Meloidogyne, known as root-knot nematodes (RKNs), develop giant cells (Jones and Payne, 1978). On the other hand, the cyst nematodes (CNs) that belong to the genera Heterodera and Globodera induce a very specialized feeding cell called syncytium (plural: Syncytia) (Jones, 1981). Migratory endo-parasitic nematodes are another category that is economically important. These nematodes follow destructive mode of feeding by continuously moving through the cells of root tissues and resulting in enormous tissue necrosis (Moens and Perry, 2009). The important genera from this category of nematodes are Pratylenchus, Radopholus, and Hirschmanniella. Moreover, Aphelenchoides, Ditylenchus, and Anguina are the main genera that infect above-ground plant parts like leaves, stem, and grains, respectively.
In the last two decades, our understanding of plant–nematode interactions has increased significantly. The first genome sequences of two root-knot nematodes species, M. incognita (Abad et al., 2008) and M. hapla (Opperman et al., 2008), have been described, which were significantly different from genome of the free-living nematode Caenorhabditis elegans. Both M. incognita and M. hapla have definite set of proteins that determine the virulence in plant species. The secretomes (set of secreted proteins through the stylets) of different PPNs have demonstrated a number of effector proteins that are involved in compatible plant–nematode interactions (Huang et al., 2003; Bellafiore et al., 2008; Caillaud et al., 2008). In response to infection of various nematodes, plant’s transcriptome resulted in increased metabolic activity in the feeding cells and suppression of defense mechanisms of the plants in most of the cases (Szakasits et al., 2009; Barcala et al., 2010; Kyndt et al., 2012; Ali et al., 2015). Most of these studies revealed considerable progress toward an understanding of plant–nematode interactions under natural conditions. On the other hand, many works have been published in the past two decades regarding the transgenic resistance in model plants, as well as the crop species using natural resistance (R) genes, proteinase inhibitors and RNA interference of nematode effector genes (Bakhetia et al., 2005; Fuller et al., 2008; Gheysen et al., 2010; Atkinson et al., 2012). In this article, we have focused on the most recent literature on PPNs with the emphasis on the use of different conventional and transgenic approaches to manage PPNs in different plant species of economic importance.
The management of PPNs has been a big challenge for the agricultural scientists and farming community. The control of nematodes below the threshold level is very important for agricultural sustainability and food security. Several strategies have been tried for this purpose, which includes management through cultural, chemical, biological, and transgenic means. The cultural practices for nematode management include solarization (Katan, 1981), flooding (MacGuidwin, 1993), fallowing (Brodie and Murphy, 1975), crop rotation (Westphal, 2011; Dababat et al., 2015), cover crops (Kirkegaard and Sarwar, 1998), and a combination of some of these (Singh et al., 2009). Similarly, the utilization of natural host resistance from various crop species is a preferred approach for nematode management because it is environmentally safe and cost-saving option as compared to chemical control (Williamson and Kumar, 2006; Dababat et al., 2016). In chemical control, fumigants, organophosphates, and carbamates have been used for inhibiting nematode populations in the soil. Most of the nematicides are now being banned due to their high cost and health and environment issues (Sorribas et al., 2005). Similarly, currently available organophosphate and carbamate compounds, i.e., oxamyl, fosthiazate, and ethoprophos, are at risk of withdrawal by EU Directive 91/414/EEC due to their hazardous nature (Clayton et al., 2008).
A lot of work has been carried out and going on to find biological antagonistic microorganisms like fungi and bacteria to minimize the effect of pathogens (Kerry, 1980, 1988; Rodriguez-Kabana et al., 1987; Sayre and Walter, 1991; Stirling, 1991; Sikora, 1992; Dababat et al., 2015). Sayre (1986) listed nematode fungal parasites Dactylella oviparasitica, Nematophthora gynophila, and Paecilomyces lilacinus along with a bacterium Pasteuria penetrans as important candidates for management of nematodes. Similarly, a Fusarium oxysporum strain, Fo162, has been shown promising for controlling M. incognita in various studies (Dababat and Sikora, 2007a,b; Martinuz et al., 2012). However, it has been found difficult to develop a biological control agent that is effective worldwide for any plant parasitic nematode. Due to high cost and health hazards, nematicides are losing their value with the passage of time thus paving the way toward the use of nematode resistance crop varieties, biocontrol and transgenic strategies for nematode management.
Recent advancements in biotechnological approaches have made it possible to incorporate and express indigenous and heterologous proteins from one organism to another. This has brought about new era of crop improvements after the advent of so-called “Green Revolution” in the 1960s. Genetic engineering of different crop plants has led to improvement of both quality and quantity of the produce in addition to enhancement of tolerance against various stresses. Several disease and pest resistance genes have been introduced into crop plants through genetic engineering. The important examples in this context are Bt cotton (Gossypium hirsutum) resistant against bollworms (Helicoverpa armigera), herbicide resistance for weed management, salinity tolerance, and rust resistance in wheat. Additionally, genetic engineering has been exceptional for enhancement of nematode resistance in plants. Here, we discuss different ways of engineering resistance genes in crop plants to suppress the nematode infection and populations in the soil below the threshold level.
In the past, several transgenic strategies have been used for enhancement of nematode resistance in plants. The resistance genes from natural resources have been cloned from numerous plant species and could be transferred to other plant species, for instance, Mi gene from tomato for resistance against M. incognita, Hs1pro-1 from sugar beet (Beta vulgaris) against H. schachtii, Gpa-2 from potato against Globodera pallida and Hero A from tomato against G. rostochiensis (reviewed by Fuller et al., 2008). The overexpression of different protease inhibitors (PIs) such as cowpea trypsin inhibitor (CpTI), PIN2, cystatins, and serine proteases has been used for producing nematode resistant plants (Lilley et al., 1999). Another main strategy was the targeted suppression of important nematode effectors in plants using RNA interference (RNAi) approach. Unlike these strategies, some recent researches have suggested that nematode resistance could be enhanced in plants by modifying the expression of particular genes in syncytia (Klink and Matthews, 2009; Ali, 2012; Ali et al., 2013a,b). An overview of various transgenic strategies aimed at nematode resistance is provided in Table 1.
Natural resistance genes could exist in both polygenic manner and single dominant nature. The resistance conferred by host plant single dominant resistance genes, the R genes from plants, interacts specifically with corresponding avirulence (Avr) genes in the nematode, resulting in a so-called ‘gene-for-gene’ interaction. This type of interaction initiates a cascade of defense responses in the plants. A short summary of natural nematode resistance genes is recently given by Fuller et al. (2008) to provide the basis for this kind of resistance in plants.
Several natural host resistance genes have been cloned from some plant species and could be transferred to other plant species. For instance, Mi-1.2 from tomato against M. incognita (Milligan et al., 1998; Williamson, 1998), Hs1pro-1 from Beta procumbens against beet cyst nematode H. schachtii (Cai et al., 1997), Gpa-2 from potato against potato cyst nematode (PCN, Globodera pallida) (van der Vossen et al., 2000) and Hero A from tomato against G. pallida and G. rostochiensis (Sobczak et al., 2005) and Cre loci from Aegilops spp. against cereal cyst nematodes in wheat (Williams et al., 1994; Safari et al., 2005) are some examples that could be used in future to develop cyst nematode resistance in crop plants. Transgenic expression of resistance proteins also induces the expression of PR (pathogenesis related) proteins to establish nematode resistance in plants. The potato roots expressing Hero A gene showed high levels of several salicylic acid (SA)-dependent PR genes in the incompatible interaction with PCN at 3 dpi (Uehara et al., 2010). They confirmed that SA inducible PR-1(P4) was a hallmark for the cultivar resistance conferred by Hero A against PCN and that nematode parasitism resulted in the inhibition of the SA signaling pathway in the susceptible cultivars. Similar effects were found in resistant line of hexaploid wheat carrying Cre2 gene, which showed upregulation of ascorbate peroxidase coding gene in response to cereal cyst nematode (H. avenae) when compared with the expression in the susceptible lines (Simonetti et al., 2010).
Another example is Gro1-4, the constitutive expression of which has increased resistance in potato plants against G. rostochiensis pathotype Ro1 (Paal et al., 2004). Rhg1 is another natural resistance gene identified in soybean against soybean cyst nematode (SCN), H. glycines (Kandoth et al., 2011). A recent study has shown that map-based cloning of a gene at the Rhg4 locus, which is a major quantitative trait locus (QTL), contributing resistance against SCN (Liu et al., 2012). In that study, the Rhg4 mutant was analyzed through transgenic complementation, which revealed that this gene confers resistance in soybean by encoding a serine hydroxymethyltransferase. This was further confirmed through overexpression of serine hydroxymethyltransferase in soybean roots that demonstrated 45% decrease in the number of mature cyst nematode (Matthews et al., 2013). In the same study, overexpression of nine other putative resistance genes (including short chain dehydrogenase, ascorbate peroxidase, lipase, β-1,4-endoglucanase, calmodulin, DREPP membrane protein, and three proteins with unknown function) resulted in more than 50% decrease in the number of adult females in soybean roots. Similarly, Hero A gene gives almost complete resistance (>95%) against root cyst nematode (G. rostochiensis), while it provides around 80% of resistance against G. pallida (Ernst et al., 2002). Transgenic expression of Hs1pro-1 (resistance gene against H. schachtii) from B. procumbens into sugar beet led to nematode resistance, but unluckily was linked with the genes that were negatively correlated with beet yield (Panella and Lewellen, 2007). Moreover, the R genes are typically effective against one or limited range of nematode species/pathotypes. Another limitation with this strategy is the development of different nematode pathotypes, which have the effectors (avr genes) that would not be recognized by the R genes (Jung et al., 1998).
Proteinase inhibitors/PIs are molecules, mostly protein in nature, which inhibit the function of proteinases/proteases released by the pathogens. After the attack of herbivores and wounding, a variety of proteinase inhibitors are produced into the plants. In case of PPNs, these PIs become active against all the four classes of proteinases from nematodes, i.e., serine, cysteine, metalloproteinases, and aspartic. The PIs used for nematode resistance studies are CpTI (Hepher and Atkinson, 1992), sweet potato (Ipomoea batatas) serine PI (sporamin or SpTI-1) (Cai et al., 2003), PIN2 (Vishnudasan et al., 2005), rice (Oryza sativa) cystatin (Oc-IΔD86) (Urwin et al., 1997, 1998), and some others cystatins from maize (Zea mays), taro (Colocasia esculenta), and sunflower (Helianthus annuus) (Fuller et al., 2008; Chan et al., 2015).
The anti-nematode potential of plant PIs was firstly described in transgenic potato expressing the serine PI, the cowpea (Vigna unguiculata) trypsin inhibitor (CpTI) against PCN (G. pallida) (Hepher and Atkinson, 1992). Similarly, the Arabidopsis plants overexpressing cystatin Oc-IΔD86 suppress both the growth and fecundity in H. schachtii and M. incognita (Urwin et al., 1997). Oc-IΔD86 was effective against different nematode species in various plants species (Urwin et al., 1995, 2000, 2003; Vain et al., 1998; Atkinson et al., 2004; Lilley et al., 2004).
Moreover, the combinations of different PIs could be helpful to couple specificity with wide range of resistance. Transgenic expression of two proteinase inhibitors, CpTI and Oc-I1Δ86, as a translational fusion protein in Arabidopsis resulted in an additive effect against G. pallida and H. schachtii (Hepher and Atkinson, 1992; Urwin et al., 1998). Other important proteinase inhibitors are sporamin (SpTI-1) (Cai et al., 2003) and PIN2 (Vishnudasan et al., 2005), which have shown good resistant response in plants.
As compared to other proteinase inhibitors, cystatins from different plant species have met the promise of enhancement of nematode resistance in a variety of crop plants (Urwin et al., 1997, 1998; Chan et al., 2010, 2015; Green et al., 2012; Tripathi et al., 2015; Papolu et al., 2016). Heterologous expression of a taro cystatin established considerable degree of resistance in tomato against M. incognita infection by interference with nematode ability of sex determination and gall formation (Chan et al., 2010). Recently, a dual strategy has been used to control PCN without affecting the soil quality (Green et al., 2012). In the first approach, a peptide was precisely targeted under control of a root tip-specific promoter that disrupts chemoreception of nematodes and suppresses root invasion without a lethal effect in both containment as well as under field trial (Lilley et al., 2011). In addition to this chemoreception disruptive peptide, OcIΔD86 cystatin from rice was incorporated to control the invading larvae that are able to cross the barrier of chemodisruptive peptide. This approach establishes that a combination of these genes offers distinct bases for the transgenic plant resistance to G. pallida without harmful impact on the non-target nematode soil community (Green et al., 2012). This rice cystatin has also shown good control in lily (Lilium longiflorum cv. ‘Nellie White’) against lesion nematode (P. penetrans) (Vieira et al., 2015).
Recently, an anti-feedant maize cystatin and an anti-root invasion synthetic peptide were transformed into plantain (Musa spp., cv. Gonja manjaya), individually and in combination (Tripathi et al., 2015). The field trials of the best transgenic event containing the peptide only demonstrated 186% more yield in addition to 99% control against R. similis, H. multicinctus, and Meloidogyne spp. as compared to non-transgenic control. Moreover, transgenic expression of proteinase inhibitor from maize and synthetic chemodisruptive peptide resulted in enhance yield and nematode resistance in plantain. Similarly, dual overexpression a taro cysteine proteinase inhibitor (CeCPI) and a fungal chitinase (PjCHI-1) in tomato under the control of a synthetic promoter, pMSPOA, (with NOS-like and SP8a elements), had negative effects on reproduction of M. incognita (Chan et al., 2015). This study further revealed that dual gene transformation had more inhibition of nematodes than plants transformed with a single gene. This advocated that the use of gene pyramiding could be employed for developing and improving nematode resistance in plants (Chan et al., 2015; Tripathi et al., 2015, 2017).
Very recently, a modified rice cystatin (Oc-IΔD86) was expressed in the roots of eggplant (S. melongena) under the control of the root-specific promoter, TUB-1 (Papolu et al., 2016). Five putative transformants containing this cystatin exhibited detrimental effects on both the development and the reproduction of M. incognita in eggplant. In that study, a single copy transgenic event showed 78.3% reduction in reproductive success of M. incognita. This concludes that proteinase inhibitors are potential candidates for induction of nematodes resistance in a variety of crop plants to increase crop yield and minimize the damage caused by these parasitic worms.
These proteins could be characterized as anti-nematode proteins because these are directly involved in inhibiting the nematode development on the plants. Lectins, some antibodies, and Bt Cry proteins are some examples of these proteins. The toxicity of lectins is characterized by their ability to obstruct intestinal function of organisms exhibiting or ingesting them (Vasconcelos and Oliveira, 2004). The defense mechanism conditioned by the lectins is vital as several lectins bind with glycans (Peumans and van Damme, 1995). Overexpression of a snowdrop (Galanthus nivalis) lectin GNA driven by cauliflower mosaic virus promoter (CaMV35S) has been exploited to exhibit anti-nematode activity in several plants, i.e., Arabidopsis, oilseed rape (Brassica napus), and potato, in response to RKNs, CNs and lesion nematodes (Burrows et al., 1998; Ripoll et al., 2003).
Plantibodies are the antibodies expressed in plants and also potential candidates for the development of nematode resistance. These are important because the establishment of a compatible plant–nematode interaction engages a series of processes against which plantibodies may be directed. RKNs and CNs depend on secretions of their pharyngeal glands to mimic re-differentiation of plant cells into specialized nematode feeding sites like giant cells or syncytia. Direction of plantibodies opposite to the active proteins from these secretions could be attenuated to suppress the parasitic ability of the nematode. There are only a few reports available in the literature regarding the use of plantibodies for nematode resistance (Fioretti et al., 2002; Sharon et al., 2002).
In addition to lectins and plantibodies, different variants of Bt toxins (Cry proteins) derived from Bacillus thuringiensis have shown promise to induce plant resistance against nematodes. These variants of Bt toxins are, however, more frequently used against phytophagous insects. Bt toxin was firstly used as an anti-nematode protein by Marroquin et al. (2000), when C. elegans was exposed to Cry5B and Cry6A which resulted in the reduction in nematode fertility and viability. The PPNs use feeding tube at the stylet orifice while feeding on the plants roots. The feeding tube operates as molecular sieve, allowing the uptake of certain molecules and excluding the others. The ultrastructure of feeding tubes from root-knot and cyst nematode differ which is based on the observation that root-knot nematodes are able to ingest larger proteins compared with cyst nematodes (Hussey and Mims, 1990; Böckenhoff and Grundler, 1994; Urwin et al., 1998; Sobczak et al., 1999; Li et al., 2007). Transgenic expression of 54 kDa Cry6A and Cry5B proteins in tomato hairy roots affected the reproduction of root-knot nematode M. incognita (Li et al., 2007, 2008). Western blotting technique showed that the 54 kDa Cry6A protein was shown to be ingested by M. incognita. On the other hand, this large protein could not be ingested by cyst nematodes (i.e., H. schachtii) due to small orifice of the feeding tube having the size limit up to approximately 23 kDa (Urwin et al., 1998). This limitation severely restricts the agronomic application of these toxins against PPNs.
Plant parasitic nematodes are highly dependent on their chemoreceptive neurons to sense distinct chemical stimuli for invasion into the plants. Nematodes use acetylcholinesterase (AChE) and/or nicotinic acetylcholine receptors for proper functioning of the nervous system. Chemodisruptive peptides are another important strategy to minimize the invasion of PPNs into the plant roots. Two peptides have been shown to bind with these receptors to inhibit their proper function (Winter et al., 2002). Both of these peptides disrupted nematode ability of chemoreception by blocking their reaction to chemical signal at very minute concentrations of up to 1 nm. Transgenic potato plants expressing a secreted peptide that inhibited nematode AChE leading to disorientation of invading nematode G. pallida, which resulted in a 52% decline in the number of female nematodes (Liu et al., 2005). The peptide is considered effective after its uptake from chemoreceptor sensillae through retrograde transport along nematode neurons to cholinergic synapses. Costa et al. (2009) has demonstrated that cyst nematode acetylcholinesterase gene (AChE) is expressed in chemo-and mechanosensory neurons of C. elegans, which further supports this hypothesis. Similarly, Wang et al. (2011) reported indirect evidence to support the mechanism by which such peptide disrupts chemosensory function in cyst nematodes. The peptide exhibits disulphide-constrained 7-mer with the amino acid sequence CTTMHPRLC that binds to nicotinic acetylcholine receptors. Incubation in the peptide solution or root-exudate from transgenic plants that secrete the peptide disrupted normal orientation of infective cyst nematodes to host root diffusate.
Moreover, chemosensory disruptive peptide that inhibits AChE has recently been expressed under the control of the constitutive CaMV35S promoter and the root tip-specific promoter in Arabidopsis and potato plants, where it confers resistance against H. schachtii and G. pallida (Lilley et al., 2011). This root tip-specific promoter from Arabidopsis gene (MDK4-20; At5g54370) directed expression of the nematode repellant peptide only at the sites of cyst nematode invasion and has shown strong level of resistance against PCN. This strategy has now been combined with the transgenic expression of a rice cystatin in potato to maintain high level of resistance against PCN without affecting soil quality (Green et al., 2012). By using the same technique, The International Institute of Tropical Agriculture (IITA), in partnership with the University of Leeds, UK, developed transgenic plantain for nematode resistance using maize digestive protease inhibitor cystatin and synthetic nematode repellent peptides (Roderick et al., 2012; Tripathi et al., 2013). Furthermore, pyramiding of cystatins and chemodisruptive peptide into different crop plants has shown high degree of nematode resistance and enhanced crop yields against root-knot nematodes (Chan et al., 2015; Tripathi et al., 2015, 2017).
RNA interference has emerged as a very useful tool for gene-silencing aimed at functional analysis of different genes by suppressing their expression in a wide variety of organisms including PPNs. In this strategy, the nematodes uptake double-stranded RNA (dsRNA) or short interfering RNAs (siRNAs) from the plants expressing these RNAs, which elicit a systemic RNAi response in nematodes. A schematic diagram elaborating the mechanism of in planta RNAi is shown in Figure 2. The transgenic expression of dsRNA targeting a specific nematode effector gene could be handful to suppress the expression of that effector gene, which is crucial for infection process. There are many review articles mushroomed out recently emphasizing the usefulness and application of RNAi technology to induce nematode resistance in plants by silencing the expression of nematode effectors mainly (Gheysen and Vanholme, 2007; Lilley et al., 2007; Fuller et al., 2008; Rosso et al., 2009; Maule et al., 2011; Tamilarasan and Rajam, 2012). In a recent review article most of the aspects of the RNAi application in nematode resistance are reviewed (Lilley et al., 2012). Lilley et al. (2012) have reviewed various features ranging from in vitro assays with C. elegans to delivering RNAi in planta as an important strategy for crop protection against cyst nematodes.
FIGURE 2. The mechanism of RNA interference (RNAi), the double-stranded RNA (dsRNA) is processed by the plant dicer enzyme (A) into (B). Once the dsRNA is uptaken by the nematode from the plant cell while feeding (C), the processing from dsRNA to short interfering RNA (siRNA) can be executed by the nematode dicer. Then, the siRNA is recognized by the RNA-induced silencing (RISC) complex of the nematode (D) and its unwinding into sense and antisense strands takes place. A proportion of the RISC complex loaded with the antisense strand interacts with the corresponding mRNA in the nematode (E) as a result the mRNA is cleaved by the RISC (F) and subsequently degraded (G). Moreover, the targeted mRNA can be made double-stranded after binding of the siRNA, and this dsRNA is then processed to produce additional siRNAs, intensifying the initial silencing signal (Gheysen and Vanholme, 2007).
Recently, Youssef et al. (2013a) have tested this approach by silencing the H. glycines gene HgALD, encoding fructose-1,6-diphosphate aldolase important in the conversion of glucose into energy and actin-based motility during parasite invasion into its host. Transgenic soybean roots expressing an RNAi construct targeted to silence HgALD revealed 58% reduction of females formed by H. glycines. Very recently, Tripathi et al. (2017) have reviewed the application of RNAi for the enhancement of nematode resistance by suppression of important effector proteins. Nevertheless, RNAi has become an established experimental tool for the enhancement of resistance against PPNs and also offers the prospect of being developed into a novel control strategy when delivered from transgenic plants.
The manipulation of expression of genes from the plants could also be useful for induction of resistance against cyst nematodes. Transgenic plants that overexpress genes correlated with resistance or have silenced genes which are important to syncytium development and nematode success are two obvious areas to explore (Klink and Matthews, 2009; Ali, 2012). This could be achieved by using precise delivery of transgene into the feeding sites as the constitutive overexpression or suppression of a particular gene could have detrimental effects on the growth and development of the plants (Ali et al., 2013b). For this purpose, promoters that are specifically expressed in the feeding sites of PPNs, i.e., Pdf2.1 or MIOX5, could be used (Siddique et al., 2009, 2011). Although constitutive promoters can deliver the gene of interest or suppress the genes which are important for compatible interaction (Ali and Abbas, 2016), however, it could be lethal for the plants to silence the genes which are also important for other physiological processes in addition to establishment of the nematodes. The promoters of the genes like Pdf2.1 or MIOX5 are specially and highly expressed in syncytia and several studies have shown the utility of these promoters to overexpress and silence the genes of interest specially into the feeding sites (Siddique et al., 2009, 2011; Ali et al., 2012, 2013b, 2014). In addition to syncytia specific promoters, root and root tip-specific promoters have also been used to drive site specific expression of proteinase inhibitors and nematode chemodistruptive peptides in several plant species (Lilley et al., 2011; Atkinson et al., 2012; Green et al., 2012).
The transcriptomes of various plant species infected with different nematode species demonstrated the upregulation of genes important for development of nematode feeding structures in the plant roots. The knockout mutants of two endo-1,4-β-glucanases, which were highly upregulated in syncytia, revealed less susceptibility in Arabidopsis in response to beet cyst nematode (Wieczorek et al., 2008). Likewise, an ATPase gene from Arabidopsis (At1g64110) was reported to be induced in syncytia caused by H. schachtii (Ali et al., 2013b). The knocking down of this gene using syncytia specific promoters (prom.AtPDF2.1 and prom.AtMIOX5) supported less number of nematodes.
Conversely, the nematodes are smart enough to suppress the defense mechanisms of the plants as most of the genes involved in defense related pathways were downregulated in the feeding sites revealed by plant transcriptomes in response to nematode infection (Szakasits et al., 2009; Barcala et al., 2010; Kyndt et al., 2012; Ali et al., 2015). One strongly downregulated group comprised peroxidase gene family, as out of top 100 differentially expressed genes with the strongest decrease in expression, 14 were peroxidases (Szakasits et al., 2009). Similarly, ethylene responsive transcription factor from Arabidopsis, AtRAP2.6, was one of the strongly downregulated transcripts in syncytia. This gene was driven through the constitutive promoter CaMV35S to overexpress in Arabidopsis at the global level, which resulted in reduced susceptibility in overexpression lines (Ali et al., 2013a). This also supports the debate of use of CaMV35S promoter to expression gene of interest in the syncytia induced by beet cyst nematodes in Arabidopsis roots (Ali and Abbas, 2016).
Another strategy is the expression of the genes involved in the defense pathways like camalexin and callose synthesis in plants (Hofmann et al., 2010; Millet et al., 2010; Mao et al., 2011; Birkenbihl et al., 2012). Recently, expression of AtPAD4 under the control of FMV-sgt promoter has resulted in enhanced resistance against soybean cyst and root-knot nematodes in soybean (Youssef et al., 2013b). Expression of AtPAD4 in soybean roots decreased the number of mature H. glycines females and M. incognita galls up to 68 and 77%, respectively. We have recently dissected the pathway of camalexin synthesis in plant–nematode interactions based on infection assays of AtWRKY33 and AtPAD3 mutants and overexpression lines (Ali et al., 2014). In this report, the syncytia specific overexpression of WRKY33 resulted in the suppression of susceptibility in Arabidopsis. Similarly, the overexpression of a soybean salicylic acid methyltransferase (GmSAMT1) gene is found to confer resistance to SCN (Lin et al., 2013).
As a consequence of enormous yield losses in crop plants imposed by the PPNs, the understanding of plant–nematode interaction is becoming of utmost importance. The nematode effectors include, for instance, cell wall degrading enzymes, the genes involved in molecular mimicry of plant genes for both compatible and incompatible plant–nematode interactions. Targeted silencing of known nematode effector proteins through in planta RNAi technology holds a great potential for plant resistance against various species of nematodes (Gheysen and Vanholme, 2007). A recently developed virus-induced gene silencing (VIGS) method provides a new tool to identify genes involved in soybean–nematode interactions (Kandoth et al., 2013). Similarly, the application of bioinformatics in the form of approaches like OrthoMCL could be very important for computational identification and analysis of effector proteins from PPNs (Williams et al., 1994). The development of population specific markers along with character compatibility method for diagnosis and phylogenetic inference of inter and intra specific populations of nematodes could also be done (Phiri et al., 2013). During the compatible plant–nematode interaction, nematodes are somehow able to suppress the defense related genes, the overexpression of which has led to enhanced resistance (Ali et al., 2013a). In addition to CaMV-35S promoter, several syncytia specific promoters could be used to overproduce the defense related genes in the feeding sites of the nematode to enhance resistance (Ali et al., 2013a, 2014; Ali and Abbas, 2016). This could be the interesting starting point for further studies to explicate how nematodes are able to suppress systemic plant defense mechanisms. It is concluded that the use of different transgenic strategies has shown good promise for nematode resistance. These have been helpful for reduction of nematode population on the plants on individual basis; however, by stacking all these molecular strategies together in the one plant will result in additive resistance, almost near to immunity against nematodes in crop plants.
MA conceived, designed and mainly develop the article; FA, AA, and FJ contributed toward the write up in main body of article; HL and AD reviewed and finalize the article.
This study is partially supported by Higher Education Commission of Pakistan.
The authors declare that the research was conducted in the absence of any commercial or financial relationships that could be construed as a potential conflict of interest.
The authors declare that all the appropriate permissions have been obtained from the copyright holders of any work that has been reproduced in the manuscript. We also thank Higher Education Commission of Pakistan for partial support.
Abad, P., Gouzy, J., Aury, J. M., Castagnone-Sereno, P., Danchin, E. G. J., Deleury, E., et al. (2008). Genome sequence of the metazoan plant-parasitic nematode Meloidogyne incognita. Nat. Biotechnol. 26, 909–915. doi: 10.1038/nbt.1482
Ali, M. A. (2012). Induction of Resistance Against Cyst Nematodes by Modifying Gene Expression in Syncytia. Ph.D. thesis, University of Natural Resources and Life Sciences, Vienna.
Ali, M. A., and Abbas, A. (2016). Analysis of reporter proteins GUS and DsRed driven under the control of CaMV35S promoter in syncytia induced by beet cyst nematode Heterodera schachtii in Arabidopsis roots.Adv. Life Sci. 3, 89–96.
Ali, M. A., Abbas, A., Azeem, F., Javed, N., and Bohlmann, H. (2015). Plant-nematode interactions: from genomics to metabolomics. Int. J. Agric. Biol. 17, 1071–1082. doi: 10.17957/IJAB/15.0037
Ali, M. A., Abbas, A., Kreil, D. P., and Bohlmann, H. (2013a). Overexpression of the transcription factor RAP2.6 leads to enhanced callose deposition in syncytia and enhanced resistance against the beet cyst nematode Heterodera schachtii in Arabidopsis roots. BMC Plant Biol. 13:47. doi: 10.1186/1471-2229-13-47
Ali, M. A., Plattner, S., Radakovic, Z., Wieczorek, K., Elashry, A., Grundler, F. M., et al. (2013b). An Arabidopsis ATPase gene involved in nematode-induced syncytium development and abiotic stress responses. Plant J. 74, 852–866. doi: 10.1111/tpj.12170
Ali, M. A., Shah, K. H., and Bohlmann, H. (2012). pMAA-Red: a new pPZP-derived vector for fast visual screening of transgenic Arabidopsis plants at the seed stage. BMC Biotechnol. 12, 37. doi: 10.1186/1472-6750-12-37
Ali, M. A., Wieczorek, K., Kreil, D. P., and Bohlmann, H. (2014). The beet cyst nematode Heterodera schachtii modulates the expression of WRKY transcription factors in syncytia to favour its development in Arabidopsis roots. PLoS ONE 9:e102360. doi: 10.1371/journal.pone.0102360
Atkinson, H. J., Grimwood, S., Johnston, K., and Green, J. (2004). Prototype demonstration of transgenic resistance to the nematode Radopholus similis conferred on banana by a cystatin. Transgenic Res. 13, 135–142. doi: 10.1023/B:TRAG.0000026070.15253.88
Atkinson, H. J., Lilley, C. J., and Urwin, P. E. (2012). Strategies for transgenic nematode control in developed and developing world crops. Curr. Opin. Biotechnol. 23, 251–256. doi: 10.1016/j.copbio.2011.09.004
Bakhetia, M., Charlton, W. L., Urwin, P. E., Mcpherson, M. J., and Atkinson, H. J. (2005). RNA interference and plant parasitic nematodes. Trends Plant Sci. 10, 362–367. doi: 10.1016/j.tplants.2005.06.007
Barcala, M., Garcia, A., Cabrera, J., Casson, S., Lindsey, K., Favery, B., et al. (2010). Early transcriptomic events in microdissected Arabidopsis nematode-induced giant cells. Plant J. 61, 698–712. doi: 10.1111/j.1365-313X.2009.04098.x
Bellafiore, S., Shen, Z. X., Rosso, M. N., Abad, P., Shih, P., and Briggs, S. P. (2008). Direct identification of the Meloidogyne incognita secretome reveals proteins with host cell reprogramming potential. PLoS Pathog. 4:e1000192. doi: 10.1371/journal.ppat.1000192
Birkenbihl, R. P., Diezel, C., and Somssich, I. E. (2012). Arabidopsis WRKY33 is a key transcriptional regulator of hormonal and metabolic responses toward Botrytis cinerea infection. Plant Physiol. 159, 266–285. doi: 10.1104/pp.111.192641
Böckenhoff, A., and Grundler, F. M. W. (1994). Studies on the nutrient-uptake by the beet cyst-nematode Heterodera schachtii by in situ microinjection of fluorescent probes into the feeding structures in Arabidopsis thaliana. Parasitology 109, 249–255. doi: 10.1017/S003118200007637X
Brodie, B. B., and Murphy, W. S. (1975). Population dynamics of plant nematodes as affected by combinations of fallow and cropping sequence. J. Nematol. 7, 91–92.
Burrows, P. R., Barker, A. D. P., Newell, C. A., and Hamilton, W. D. O. (1998). Plant-derived enzyme inhibitors and lectins for resistance against plant-parasitic nematodes in transgenic crops. Pestic. Sci. 52, 176–183. doi: 10.1002/(SICI)1096-9063(199802)52:2<176::AID-PS680>3.0.CO;2-T
Cai, D., Thurau, T., Tian, Y., Lange, T., Yeh, K. W., and Jung, C. (2003). Sporamin-mediated resistance to beet cyst nematodes (Heterodera schachtii Schm.) is dependent on trypsin inhibitory activity in sugar beet (Beta vulgaris L.) hairy roots. Plant Mol. Biol. 51, 839–849. doi: 10.1023/A:1023089017906
Cai, D. G., Kleine, M., Kifle, S., Harloff, H. J., Sandal, N. N., Marcker, K. A., et al. (1997). Positional cloning of a gene for nematode resistance in sugar beet. Science 275, 832–834. doi: 10.1126/science.275.5301.832
Caillaud, M. C., Dubreuil, G., Quentin, M., Perfus-Barbeoch, L., Lecomte, P., De Almeida Engler, J., et al. (2008). Root-knot nematodes manipulate plant cell functions during a compatible interaction. J. Plant Physiol. 165, 104–113. doi: 10.1016/j.jplph.2007.05.007
Chan, Y.-L., He, Y., Hsiao, T.-T., Wang, C.-J., Tian, Z., and Yeh, K.-W. (2015). Pyramiding taro cystatin and fungal chitinase genes driven by a synthetic promoter enhances resistance in tomato to root-knot nematode Meloidogyne incognita. Plant Sci. 231, 74–81. doi: 10.1016/j.plantsci.2014.11.010
Chan, Y.-L., Yang, A.-H., Chen, J.-T., Yeh, K.-W., and Chan, M.-T. (2010). Heterologous expression of taro cystatin protects transgenic tomato against Meloidogyne incognita infection by means of interfering sex determination and suppressing gall formation. Plant Cell Rep. 29, 231–238. doi: 10.1007/s00299-009-0815-y
Clayton, R., Storey, M., Parker, B., Ballingall, M., and Davies, K. (2008). Impact of Reduced Pesticide Availability on Control of Potato Cyst Nematodes and Weeds in Potato Crops. Oxford: Potato Council Ltd.
Costa, J. C., Lilley, C. J., Atkinson, H. J., and Urwin, P. E. (2009). Functional characterisation of a cyst nematode acetylcholinesterase gene using Caenorhabditis elegans as a heterologous system. Int. J. Parasitol. 39, 849–858. doi: 10.1016/j.ijpara.2008.12.007
Dababat, A. A., Ferney, G.-B. H., Erginbas-Orakci, G., Dreisigacker, S., Imren, M., Toktay, H., et al. (2016). Association analysis of resistance to cereal cyst nematodes (Heterodera avenae) and root lesion nematodes (Pratylenchus neglectus and P. thornei) in CIMMYT advanced spring wheat lines for semi-arid conditions. Breed. Sci. 66, 692–702. doi: 10.1270/jsbbs.15158
Dababat, A., Imren, M., Erginbas-Orakci, G., Ashrafi, S., Yavuzaslanoglu, E., Toktay, H., et al. (2015). The importance and management strategies of cereal cyst nematodes, Heterodera spp., in Turkey. Euphytica 202, 173–188. doi: 10.1007/s10681-014-1269-z
Dababat, A., and Sikora, R. A. (2007a). Importance of application time and inoculum density of Fusarium oxysporum 162 for biological control of Meloidogyne incognita on tomato. Nematropica 37, 267–275.
Dababat, A. A., and Sikora, R. A. (2007b). Induced resistance by the mutualistic endophyte, Fusarium oxysporum strain 162, toward Meloidogyne incognita on tomato. Biocontrol Sci. Technol. 17, 969–975. doi: 10.1080/09583150701582057
Decraemer, W., and Hunt, D. J. (2006). “Structure and classification,” in Plant Nematology, eds R. N. Perry and M. Moens (Wallingford: CABI Publishing).
Ernst, K., Kumar, A., Kriseleit, D., Kloos, D. U., Phillips, M. S., and Ganal, M. W. (2002). The broad-spectrum potato cyst nematode resistance gene (Hero) from tomato is the only member of a large gene family of NBS-LRR genes with an unusual amino acid repeat in the LRR region. Plant J. 31, 127–136. doi: 10.1046/j.1365-313X.2002.01341.x
Fioretti, L., Porter, A., Haydock, P. J., and Curtis, R. (2002). Monoclonal antibodies reactive with secreted-excreted products from the amphids and the cuticle surface of Globodera pallida affect nematode movement and delay invasion of potato roots. Int. J. Parasitol. 32, 1709–1718. doi: 10.1016/S0020-7519(02)00178-9
Fuller, V. L., Lilley, C. J., and Urwin, P. E. (2008). Nematode resistance. New Phytol. 180, 27–44. doi: 10.1111/j.1469-8137.2008.02508.x
Gheysen, G., Kyndt, T., Haegeman, A., Joseph, S., Remy, S., and Swennen, R. (2010). RNAi for research and applications in plant-nematode interactions. In Vitro Cell. Dev. Biol. Anim. 46, S28–S29.
Gheysen, G., and Vanholme, B. (2007). RNAi from plants to nematodes. Trends Biotechnol. 25, 89–92. doi: 10.1016/j.tibtech.2007.01.007
Green, J., Wang, D., Lilley, C. J., Urwin, P. E., and Atkinson, H. J. (2012). Transgenic potatoes for potato cyst nematode control can replace pesticide use without impact on soil quality. PLoS ONE 7:e30973. doi: 10.1371/journal.pone.0030973
Hofmann, J., Youssef-Banora, M., De Almeida-Engler, J., and Grundler, F. M. (2010). The role of callose deposition along plasmodesmata in nematode feeding sites. Mol. Plant Microbe Interact. 23, 549–557. doi: 10.1094/MPMI-23-5-0549
Huang, G. Z., Gao, B. L., Maier, T., Allen, R., Davis, E. L., Baum, T. J., et al. (2003). A profile of putative parasitism genes expressed in the esophageal gland cells of the root-knot nematode Meloidogyne incognita. Mol. Plant Microbe Interact. 16, 376–381. doi: 10.1094/MPMI.2003.16.5.376
Hussey, R. S., and Mims, C. W. (1990). Ultrastructure of esophageal glands and their secretory granules in the root-knot nematode Meloidogyne incognita. Protoplasma 156, 9–18. doi: 10.1007/BF01666501
Jones, M. G. K. (1981). Host cell responses to endoparasitic nematode attack: structure and function of giant cells and syncytia. Ann. Appl. Biol. 97, 353–372. doi: 10.1111/j.1744-7348.1981.tb05122.x
Jones, M. G. K., and Payne, H. L. (1978). Early stage of nematode-induced giant-cell formation in roots of Impatiens balsamina. J. Nematol. 10, 70–84.
Jung, C., Cai, D. G., and Kleine, M. (1998). Engineering nematode resistance in crop species. Trends Plant Sci. 3, 266–271. doi: 10.1016/S1360-1385(98)01247-3
Kandoth, P. K., Heinz, R., Yeckel, G., Gross, N. W., Juvale, P. S., Hill, J., et al. (2013). A virus-induced gene silencing method to study soybean cyst nematode parasitism in Glycine max. BMC Res. Notes 6:255. doi: 10.1186/1756-0500-6-255
Kandoth, P. K., Ithal, N., Recknor, J., Maier, T., Nettleton, D., Baum, T. J., et al. (2011). The soybean Rhg1 locus for resistance to the soybean cyst nematode Heterodera glycines regulates the expression of a large number of stress- and defense-related genes in degenerating feeding cells. Plant Physiol. 155,1960–1975. doi: 10.1104/pp.110.167536
Katan, J. (1981). Solar heating (solarization) of soil for control of soilborne pests. Annu. Rev. Phytopathol. 19, 211–236. doi: 10.1146/annurev.py.19.090181.001235
Kerry, B. (1988). Fungal parasites of cyst nematodes. Agric. Ecosyst. Environ. 24, 293–305. doi: 10.1016/0167-8809(88)90073-4
Kirkegaard, J. A., and Sarwar, M. (1998). Biofumigation potential of Brassicas. I. Variation in glucosinolate profiles of diverse field-grown Brassicas. Plant Soil 201, 71–89. doi: 10.1023/A:1004364713152
Klink, V. P., and Matthews, B. F. (2009). Emerging approaches to broaden resistance of soybean to soybean cyst nematode as supported by gene expression studies. Plant Physiol. 151, 1017–1022. doi: 10.1104/pp.109.144006
Kyndt, T., Nahar, K., Haegeman, A., De Vleesschauwer, D., Hofte, M., and Gheysen, G. (2012). Comparing systemic defence-related gene expression changes upon migratory and sedentary nematode attack in rice. Plant Biol. 14, 73–82. doi: 10.1111/j.1438-8677.2011.00524.x
Li, X.-Q., Tan, A., Voegtline, M., Bekele, S., Chen, C.-S., and Aroian, R. V. (2008). Expression of Cry5B protein from Bacillus thuringiensis in plant roots confers resistance to root-knot nematode. Biol. Control 47, 97–102. doi: 10.1016/j.biocontrol.2008.06.007
Li, X. Q., Wei, J. Z., Tan, A., and Aroian, R. V. (2007). Resistance to root-knot nematode in tomato roots expressing a nematicidal Bacillus thuringiensis crystal protein. Plant Biotechnol. J. 5, 455–464. doi: 10.1111/j.1467-7652.2007.00257.x
Lilley, C. J., Bakhetia, M., Charlton, W. L., and Urwin, P. E. (2007). Recent progress in the development of RNA interference for plant parasitic nematodes. Mol. Plant Pathol. 8, 701–711. doi: 10.1111/j.1364-3703.2007.00422.x
Lilley, C. J., Davies, L. J., and Urwin, P. E. (2012). RNA interference in plant parasitic nematodes: a summary of the current status. Parasitology 139, 630–640. doi: 10.1017/S0031182011002071
Lilley, C. J., Devlin, F., Urwin, P. E., and Atkinson, H. J. (1999). Parasitic nematodes, proteinases and transgenic plants. Parasitol. Today 15, 414–417. doi: 10.1016/S0169-4758(99)01513-6
Lilley, C. J., Urwin, P. E., Johnston, K. A., and Atkinson, H. J. (2004). Preferential expression of a plant cystatin at nematode feeding sites confers resistance to Meloidogyne incognita and Globodera pallida. Plant Biotechnol. J. 2, 3–12. doi: 10.1046/j.1467-7652.2003.00037.x
Lilley, C. J., Wang, D., Atkinson, H. J., and Urwin, P. E. (2011). Effective delivery of a nematode-repellent peptide using a root-cap-specific promoter. Plant Biotechnol. J. 9, 151–161. doi: 10.1111/j.1467-7652.2010.00542.x
Lin, J., Mazarei, M., Zhao, N., Zhu, J. J., Zhuang, X., Liu, W., et al. (2013). Overexpression of a soybean salicylic acid methyltransferase gene confers resistance to soybean cyst nematode. Plant Biotechnol. J. 11, 1135–1145. doi: 10.1111/pbi.12108
Liu, B., Hibbard, J. K., Urwin, P. E., and Atkinson, H. J. (2005). The production of synthetic chemodisruptive peptides in planta disrupts the establishment of cyst nematodes. Plant Biotechnol. J. 3, 487–496. doi: 10.1111/j.1467-7652.2005.00139.x
Liu, S., Kandoth, P. K., Warren, S. D., Yeckel, G., Heinz, R., Alden, J., et al. (2012). A soybean cyst nematode resistance gene points to a new mechanism of plant resistance to pathogens. Nature 492, 256–260. doi: 10.1038/nature11651
MacGuidwin, A. E. (1993). “Management of nematodes,” in Potato Health Management, ed. R. C. Rowe (St. Paul, MN: APS Press).
Mao, G., Meng, X., Liu, Y., Zheng, Z., Chen, Z., and Zhang, S. (2011). Phosphorylation of a WRKY transcription factor by two pathogen-responsive MAPKs drives phytoalexin biosynthesis in Arabidopsis. Plant Cell 23, 1639–1653. doi: 10.1105/tpc.111.084996
Marroquin, L. D., Elyassnia, D., Griffitts, J. S., Feitelson, J. S., and Aroian, R. V. (2000). Bacillus thuringiensis (Bt) toxin susceptibility and isolation of resistance mutants in the nematode Caenorhabditis elegans. Genetics 155, 1693–1699.
Martinuz, A., Schouten, A., and Sikora, R. A. (2012). Post-infection development of Meloidogyne incognita on tomato treated with the endophytes Fusarium oxysporum strain Fo162 and Rhizobium etli strain G12. Biocontrol 58, 95–104. doi: 10.1094/PHYTO-04-11-0120
Matthews, B. F., Beard, H., Macdonald, M. H., Kabir, S., Youssef, R. M., Hosseini, P., et al. (2013). Engineered resistance and hypersusceptibility through functional metabolic studies of 100 genes in soybean to its major pathogen, the soybean cyst nematode. Planta 237, 1337–1357. doi: 10.1007/s00425-013-1840-1
Maule, A. G., Mcveigh, P., Dalzell, J. J., Atkinson, L., Mousley, A., and Marks, N. J. (2011). An eye on RNAi in nematode parasites. Trends Parasitol. 27, 505–513. doi: 10.1016/j.pt.2011.07.004
Millet, Y. A., Danna, C. H., Clay, N. K., Songnuan, W., Simon, M. D., Werck-Reichhart, D., et al. (2010). Innate immune responses activated in Arabidopsis roots by microbe-associated molecular patterns. Plant Cell 22, 973–990. doi: 10.1105/tpc.109.069658
Milligan, S. B., Bodeau, J., Yaghoobi, J., Kaloshian, I., Zabel, P., and Williamson, V. M. (1998). The root knot nematode resistance gene Mi from tomato is a member of the leucine zipper, nucleotide binding, leucine-rich repeat family of plant genes. Plant Cell 10, 1307–1319. doi: 10.1105/tpc.10.8.1307
Moens, M., and Perry, R. N. (2009). Migratory plant endoparasitic nematodes: a group rich in contrasts and divergence. Annu. Rev. Phytopathol. 47, 313–332. doi: 10.1146/annurev-phyto-080508-081846
Opperman, C. H., Bird, D. M., Williamson, V. M., Rokhsar, D. S., Burke, M., Cohn, J., et al. (2008). Sequence and genetic map of Meloidogyne hapla: a compact nematode genome for plant parasitism. Proc. Natl. Acad. Sci. U.S.A. 105, 14802–14807. doi: 10.1073/pnas.0805946105
Paal, J., Henselewski, H., Muth, J., Meksem, K., Menendez, C. M., Salamini, F., et al. (2004). Molecular cloning of the potato Gro1-4 gene conferring resistance to pathotype Ro1 of the root cyst nematode Globodera rostochiensis, based on a candidate gene approach. Plant J. 38, 285–297. doi: 10.1111/j.1365-313X.2004.02047.x
Panella, L., and Lewellen, R. T. (2007). Broadening the genetic base of sugar beet: introgression from wild relatives. Euphytica 154, 383–400. doi: 10.1007/s10681-006-9209-1
Papolu, P. K., Dutta, T. K., Tyagi, N., Urwin, P. E., Lilley, C. J., and Rao, U. (2016). Expression of a cystatin transgene in eggplant provides resistance to root-knot nematode, Meloidogyne incognita. Front. Plant Sci. 7:1122. doi: 10.3389/fpls.2016.01122
Peumans, W. J., and van Damme, E. J. M. (1995). The role of lectins in plant defence. Histochem. J. 27, 253–271. doi: 10.1007/BF00398968
Phiri, A. M., De Pomerai, D., Buttle, D. J., and Behnke, J. M. B. (2013). Developing a rapid throughput screen for detection of nematicidal activity of plant cysteine proteinases: the role of Caenorhabditis elegans cystatins. Parasitology 141, 164–180. doi: 10.1017/S0031182013001364
Riley, I. T., Nicol, J. M., and Dababat, A. A. (2009). Cereal Cyst Nematodes: Status, Research and Outlook. Ankara: CIMMYT.
Ripoll, C., Favery, B., Lecomte, P., Van Damme, E., Peumans, W., Abad, P., et al. (2003). Evaluation of the ability of lectin from snowdrop (Galanthus nivalis) to protect plants against root-knot nematodes. Plant Sci. 164, 517–523. doi: 10.1016/S0168-9452(02)00448-X
Roderick, H., Tripathi, L., Babirye, A., Wang, D., Tripathi, J., Urwin, P. E., et al. (2012). Generation of transgenic plantain (Musa spp.) with resistance to plant pathogenic nematodes. Mol. Plant Pathol. 13, 842–851. doi: 10.1111/j.1364-3703.2012.00792.x
Rodriguez-Kabana, R., Morgan-Jones, G., and Chet, I. (1987). Biological control of nematodes: soil amendments and microbial antagonists. Plant Soil 100, 237–247. doi: 10.1007/BF02370944
Rosso, M. N., Jones, J. T., and Abad, P. (2009). RNAi and functional genomics in plant parasitic nematodes. Annu. Rev. Phytopathol. 47, 207–232. doi: 10.1146/annurev.phyto.112408.132605
Safari, E., Gororo, N. N., Eastwood, R. F., Lewis, J., Eagles, H. A., and Ogbonnaya, F. C. (2005). Impact of Cre1, Cre8 and Cre3 genes on cereal cyst nematode resistance in wheat. Theor. Appl. Genet. 110, 567–572. doi: 10.1007/s00122-004-1873-8
Sayre, R. M. (1986). Pathogens for biological control of nematodes. Crop Prot. 5, 268–276. doi: 10.1016/0261-2194(86)90062-1
Sayre, R. M., and Walter, D. E. (1991). Factors affecting the efficacy of natural enemies of nematodes. Annu. Rev. Phytopathol. 29, 149–166. doi: 10.3389/fpls.2016.00321
Sharon, E., Spiegel, Y., Salomon, R., and Curtis, R. H. (2002). Characterization of Meloidogyne javanica surface coat with antibodies and their effect on nematode behaviour. Parasitology 125, 177–185. doi: 10.1017/S0031182002001907
Siddique, S., Endres, S., Atkins, J. M., Szakasits, D., Wieczorek, K., Hofmann, J., et al. (2009). Myo-inositol oxygenase genes are involved in the development of syncytia induced by Heterodera schachtii in Arabidopsis roots. New Phytol. 184, 457–472. doi: 10.1111/j.1469-8137.2009.02981.x
Siddique, S., Wieczorek, K., Szakasits, D., Kreil, D. P., and Bohlmann, H. (2011). The promoter of a plant defensin gene directs specific expression in nematode-induced syncytia in Arabidopsis roots. Plant Physiol. Biochem. 49, 1100–1107. doi: 10.1016/j.plaphy.2011.07.005
Sikora, R. A. (1992). Management of the antagonistic potential in agricultural ecosystems for the biological control of plant parasitic nematodes. Annu. Rev. Phytopathol. 30, 245–270. doi: 10.1146/annurev.py.30.090192.001333
Simonetti, E., Alba, E., Montes, M. J., Delibes, A., and Lopez-Brana, I. (2010). Analysis of ascorbate peroxidase genes expressed in resistant and susceptible wheat lines infected by the cereal cyst nematode, Heterodera avenae. Plant Cell Rep. 29, 1169–1178. doi: 10.1007/s00299-010-0903-z
Singh, A. K., Sharma, A. K., and Shoran, J. (2009). “Heterodera avenae and its management on wheat in India,” in Cereal Cyst Nematodes: Status, Research and Outlook, eds I. T. Riley, J. M. Nicol, and A. Dababat (Ankara: CIMMYT).
Sobczak, M., Avrova, A., Jupowicz, J., Phillips, M. S., Ernst, K., and Kumar, A. (2005). Characterization of susceptibility and resistance responses to potato cyst nematode (Globodera spp.) infection of tomato lines in the absence and presence of the broad-spectrum nematode resistance Hero gene. Mol. Plant Microbe Interact. 18, 158–168. doi: 10.1094/MPMI-18-0158
Sobczak, M., Golinowski, W. A., and Grundler, F. M. W. (1999). Ultrastructure of feeding plugs and feeding tubes formed by Heterodera schachtii. Nematology 1, 363–374. doi: 10.1163/156854199508351
Sorribas, F., Ornat, C., Verdejo-Lucas, S., Galeano, M., and Valero, J. (2005). Effectiveness and profitability of the Mi-resistant tomatoes to control root-knot nematodes. Eur. J. Plant Pathol. 111, 29–38. doi: 10.1007/s10658-004-1982-x
Stirling, G. R. (1991). Biological Control of Plant-Parasitic Nematodes. Wallingford: CAB International.
Szakasits, D., Heinen, P., Wieczorek, K., Hofmann, J., Wagner, F., Kreil, D. P., et al. (2009). The transcriptome of syncytia induced by the cyst nematode Heterodera schachtii in Arabidopsis roots. Plant J. 57, 771–784. doi: 10.1111/j.1365-313X.2008.03727.x
Tamilarasan, S., and Rajam, M. V. (2012). Engineering crop plants for nematode resistance through host-derived RNA interference. Cell Dev. Biol. 2, 114. doi: 10.4161/bbug.2.4.16112
Tripathi, L., Atkinson, H., Roderick, H., Kubiriba, J., and Tripathi, J. N. (2017). Genetically engineered bananas resistant to Xanthomonas wilt disease and nematodes. Food and Energy Security doi: 10.1002/fes3.101
Tripathi, L., Babirye, A., Roderick, H., Tripathi, J. N., Changa, C., Urwin, P. E., et al. (2015). Field resistance of transgenic plantain to nematodes has potential for future African food security. Sci. Rep. 5:8127. doi: 10.1038/srep08127
Tripathi, L., Tripathi, J. N., Roderick, H., and Atkinson, H. J. (2013). Engineering nematode resistant plantains for sub-Saharan Africa. Acta Hortic. 974, 99–107. doi: 10.17660/ActaHortic.2013.974.11
Uehara, T., Sugiyama, S., Matsuura, H., Arie, T., and Masuta, C. (2010). Resistant and susceptible responses in tomato to cyst nematode are differentially regulated by salicylic acid. Plant Cell Physiol. 51, 1524–1536. doi: 10.1093/pcp/pcq109
Urwin, P. E., Atkinson, H. J., Waller, D. A., and Mcpherson, M. J. (1995). Engineered Oryzacystatin-I expressed in transgenic hairy roots confers resistance to Globodera pallida. Plant J. 8, 121–131. doi: 10.1046/j.1365-313X.1995.08010121.x
Urwin, P. E., Green, J., and Atkinson, H. J. (2003). Expression of a plant cystatin confers partial resistance to Globodera, full resistance is achieved by pyramiding a cystatin with natural resistance. Mol. Breed. 12, 263–269. doi: 10.1023/A:1026352620308
Urwin, P. E., Levesley, A., Mcpherson, M. J., and Atkinson, H. J. (2000). Transgenic resistance to the nematode Rotylenchulus reniformis conferred by Arabidopsis thaliana plants expressing proteinase inhibitors. Mol. Breed. 6, 257–264. doi: 10.1023/A:1009669325944
Urwin, P. E., Lilley, C. J., Mcpherson, M. J., and Atkinson, H. J. (1997). Resistance to both cyst and root-knot nematodes conferred by transgenic Arabidopsis expressing a modified plant cystatin. Plant J. 12, 455–461. doi: 10.1046/j.1365-313X.1997.12020455.x
Urwin, P. E., Mcpherson, M. J., and Atkinson, H. J. (1998). Enhanced transgenic plant resistance to nematodes by dual proteinase inhibitor constructs. Planta 204, 472–479. doi: 10.1007/s004250050281
Vain, P., Worland, B., Clarke, M. C., Richard, G., Beavis, M., Liu, H., et al. (1998). Expression of an engineered cysteine proteinase inhibitor (Oryzacystatin-IΔD86) for nematode resistance in transgenic rice plants. Theor. Appl. Genet. 96, 266–271. doi: 10.1007/s001220050735
van der Vossen, E. A., Van Der Voort, J. N., Kanyuka, K., Bendahmane, A., Sandbrink, H., Baulcombe, D. C., et al. (2000). Homologues of a single resistance-gene cluster in potato confer resistance to distinct pathogens: a virus and a nematode. Plant J. 23, 567–576. doi: 10.1046/j.1365-313x.2000.00814.x
Vasconcelos, I. M., and Oliveira, J. T. (2004). Antinutritional properties of plant lectins. Toxicon 44, 385–403. doi: 10.1016/j.toxicon.2004.05.005
Vieira, P., Wantoch, S., Lilley, C. J., Chitwood, D. J., Atkinson, H. J., and Kamo, K. (2015). Expression of a cystatin transgene can confer resistance to root lesion nematodes in Lilium longiflorum cv. ‘Nellie White’. Transgenic Res. 24, 421–432. doi: 10.1007/s11248-014-9848-2
Vishnudasan, D., Tripathi, M. N., Rao, U., and Khurana, P. (2005). Assessment of nematode resistance in wheat transgenic plants expressing potato proteinase inhibitor (PIN2) gene. Transgenic Res. 14, 665–675. doi: 10.1007/s11248-005-5696-4
Wang, D., Jones, L. M., Urwin, P. E., and Atkinson, H. J. (2011). A synthetic peptide shows retro- and anterograde neuronal transport before disrupting the chemosensation of plant-pathogenic nematodes. PLoS ONE 6:e17475. doi: 10.1371/journal.pone.0017475
Webster, J. M. (1995). “The host-parasite relationships of plant-parasitic nematodes,” in Advances in Parasitology, ed. B. Dawes (Cambridge, MA: Academic Press Inc).
Westphal, A. (2011). Sustainable approaches to the management of plant-parasitic nematodes and disease complexes. J. Nematol. 43, 122–125.
Wieczorek, K., Hofmann, J., Blochl, A., Szakasits, D., Bohlmann, H., and Grundler, F. M. W. (2008). Arabidopsis endo-1,4-beta-glucanases are involved in the formation of root syncytia induced by Heterodera schachtii. Plant J. 53, 336–351. doi: 10.1111/j.1365-313X.2007.03340.x
Williams, K. J., Fisher, J. M., and Langridge, P. (1994). Identification of RFLP markers linked to the cereal cyst nematode resistance gene (Cre) in wheat. Theor. Appl. Genet. 89, 927–930. doi: 10.1007/BF00224519
Williamson, V. M. (1998). Root-knot nematode resistance genes in tomato and their potential for future use. Annu. Rev. Phytopathol. 36, 277–293. doi: 10.1146/annurev.phyto.36.1.277
Williamson, V. M., and Kumar, A. (2006). Nematode resistance in plants: the battle underground. Trends Genet. 22, 396–403. doi: 10.1016/j.tig.2006.05.003
Winter, M. D., Mcpherson, M. J., and Atkinson, H. J. (2002). Neuronal uptake of pesticides disrupts chemosensory cells of nematodes. Parasitology 125, 561–565.
Youssef, R. M., Kim, K. H., Haroon, S. A., and Matthews, B. F. (2013a). Post-transcriptional gene silencing of the gene encoding aldolase from soybean cyst nematode by transformed soybean roots. Exp. Parasitol. 134, 266–274. doi: 10.1016/j.exppara.2013.03.009
Keywords: plant parasitic nematodes, R genes, protease inhibitors, RNAi, plant resistance
Citation: Ali MA, Azeem F, Abbas A, Joyia FA, Li H and Dababat AA (2017) Transgenic Strategies for Enhancement of Nematode Resistance in Plants. Front. Plant Sci. 8:750. doi: 10.3389/fpls.2017.00750
Received: 13 February 2017; Accepted: 21 April 2017;
Published: 09 May 2017.
Edited by:
Ian Douglas Godwin, The University of Queensland, AustraliaReviewed by:
Leena Tripathi, International Institute of Tropical Agriculture, KenyaCopyright © 2017 Ali, Azeem, Abbas, Joyia, Li and Dababat. This is an open-access article distributed under the terms of the Creative Commons Attribution License (CC BY). The use, distribution or reproduction in other forums is permitted, provided the original author(s) or licensor are credited and that the original publication in this journal is cited, in accordance with accepted academic practice. No use, distribution or reproduction is permitted which does not comply with these terms.
*Correspondence: Muhammad A. Ali, YW1qYWQuYWxpQHVhZi5lZHUucGs=
Disclaimer: All claims expressed in this article are solely those of the authors and do not necessarily represent those of their affiliated organizations, or those of the publisher, the editors and the reviewers. Any product that may be evaluated in this article or claim that may be made by its manufacturer is not guaranteed or endorsed by the publisher.
Research integrity at Frontiers
Learn more about the work of our research integrity team to safeguard the quality of each article we publish.