- Key Laboratory of Crop Physiology, Ecology and Production Management, Ministry of Agriculture, Nanjing Agricultural University, Nanjing, China
Insect-proof net cultivation (IPN), rice-duck farming (RD), and organic matter return (OM) are important methods to realize sustainable development of rice production. A split-plot field experiment was performed to study the effects of IPN, RD, and OM on the rice yield, dry matter accumulation and N utilization. Results showed that compared to inorganic N fertilizer (IN), wheat straw return, and biogas residue return increased the rice yield by 2.11–4.28 and 4.78–7.67%, respectively, and also improved dry matter and N accumulation after the elongation stage (EG), dry matter and N translocation, and N recovery efficiency (NRE). These results attributed to an increase in leaf SPAD values and net photosynthetic rate (Pn) after the EG. Compared to conventional rice farming (CR), RD promoted the rice yield by 1.52–3.74%, and contributed to higher the leaf photosynthesis, dry matter and N accumulation, dry matter and N translocation, and NRE. IPN decreased the intensity of sun radiation in the nets due to the coverage of the insect-proof nets, which declined the leaf Pn, dry matter accumulation and translocation, N absorption and translocation, and NRE compared to open field cultivation (OFC). The rice yield of IPN were 2.48–4.98% lower than that of OFC. Compared to the interaction between CR and IN, the interaction between RD and OM improved the rice yield by 5.26–9.33%, and increased dry matter and N accumulation after the EG, dry matter and N translocation, and NRE. These results indicated that OM, RD and the interaction between RD and OM could promote dry matter accumulation and N utilization, which was beneficial to improve the rice yield.
Introduction
Rice is one of the main food crops worldwide and plays an important role in global food production and consumption. Over the past 60 years, food production has been greatly improved through the use of high-yield varieties and modern fertilizers, irrigation and pesticides (Zeng et al., 2012). However, with the continuous increasing world population, food security has become an increasingly important concern. Improvement in rice production is essential for ensuring global food security (Hu et al., 2013). It is estimated that, to satisfy the rapid growth of population in rice consuming countries by 2030, rice production should be increased by 40% (Khush, 2005). Furthermore, along with the decrease of agricultural land area and continuing of environmental deterioration (Kant et al., 2012), China and other developing countries are facing the dual challenge of increasing rice yield while at the same time reducing environmental threats (Chen et al., 2011). Rice yield is comprehensively influenced by cultivation environment, soil nutrients, and field management. Field management is easily controlled by human factors. Thus, the improvement of field management plays an important role in increasing rice yield.
Rice diseases and insect pests are the major limiting barriers of yield. Chemical pesticides and fungicides are commonly used to prevent diseases and insect pests to avoid yield loss. However, pesticides and fungicides can be retained in the surface water or soil, which may diminish the effectiveness and cause serious harm to the environment. Insect-proof nets provide an ecological and effective approach for controlling the infection and transfer of plant diseases and insect pests (Guo et al., 2015). RD is a mode in which a certain number of ducks are raised in a rice field to eat weeds, insects, and small aquatic animals (Xu et al., 2017). Additionally, the ducks wander while feeding and excreting, which is helpful for intertilling, weeding, building soil fertility, and stimulating rice growth (Suh, 2014). RD, which is highly praised by rice growers, rice consumers, and government, has been known as the best ecological method for developing sustainable agriculture.
Rice-wheat rotation is the dominant farming system in the Yangtze River region of China, which can produce large amounts of straw residue (Wang X.H. et al., 2015). However, due to the transfer of rural labor, some farmers directly burn straw to save time. But burning causes severe environment pollution and soil degradation and thus it is forbidden by law in China (Zhang et al., 2014). Returning straw into the soil may be an effective agricultural practice (Seufert et al., 2012). This method not only solves environmental problems but also promotes the nutrient recycling and sustainable environmental development. Previous studies have indicated that straw return was an effective means to improve soil quality and rice yield (Liu et al., 2014). BR is the solid residue that remains after the anaerobic fermentation of organic wastes, including crop straw and human and animal excreta, and contains N, P, K, calcium, magnesium, humic acid, organic acid, and cellulose (Liu et al., 2010). Thus, BR is a high-quality organic fertilizer.
Currently, through a series of subsidy policies, the government advocates wheat straw and organic fertilizer return. In Jiangsu, China, more than 70% of the total rice-wheat growing region practices straw return. As the key technology in rice ecological control, insect-proof net mulching has attracted significant attention and has been applied by agricultural workers. However, few studies on the effects of insect-proof net mulching, RD, and OM on rice yield and population quality have been conducted. The objectives of this study were to investigate the effects of IPN, RD, and OM on rice dry matter accumulation and N utilization, and to further explore the relationships between dry matter accumulation, N utilization and rice yield in rice production.
Materials and Methods
Site Descriptions
The field experiments were conducted at the Baiwei Farm of Nanjing Agricultural University (32°34′ N, 120°24′ E) from 2014 to 2015. The experimental region is characterized by a subtropical monsoon climate. The annual mean temperature at Baiwei farm is 14.5°C; the mean temperature during the rice growing season is 22.5°C; the annual mean precipitation is 1025 mm; the annual total solar radiation is 4.99 × 109 J m-2; and the annual total solar radiation during the rice growing season is 3.01 × 109 J m-2. The fore-rotating crop was wheat, and the soil was clay, with soil properties as follows: organic matter 24.6 g kg-1, total N 1.26 g kg-1, available N 97.2 mg kg-1, available P 24.3 mg kg-1, and available K 95.7 mg kg-1.
Experimental Design
Using a split-plot design, the experiments took cultivation environment as main plot, and OFC and IPN as two treatments. IPN used a rigid frame and a flat roof covered with white nets on the outside for insect proofing. Using cultural practice as subplot, the experimental design included two treatments, i.e., CR and RD. Fertilizer management was used as sub-subplot, including IN and OM, and OM refers to WS and BR.
The experiment was performed with equal amounts of nutrients. The amount of WS to the soil was 6000 kg ha-1, and the amount of BR after fermentation of wheat straw was 10,500 kg ha-1. Both wheat straw and biogas residue were used as base fertilizers. All treatments received the same amount of nutrient in rice season, including 300 kg N ha-1, 150 kg P2O5 ha-1, and 150 kg K2O ha-1, and deficient nutrients were supplemented using inorganic fertilizer. N was applied as follows: 15% as base fertilizer, 45% as tiller fertilizer, and 40% as panicle fertilizer. Tiller fertilizer was used in an equal amount and applied on the 7th day and 14th day after transplanting. P2O5 was used entirely as base fertilizer, and K2O was used as base fertilizer and panicle fertilizer at equal amounts. To calculate the N utilization efficiency in each treatment, an additional treatment was established in which N was not applied but P2O5 and K2O were added.
The experimental variety was Nanjing9108, which was sown on May 24th, and seedlings by substrate nursing were mechanically transplanted on June 15th with a hill spacing of 13.3 cm × 30 cm and four seedlings per hole. The experiment was performed in three replicates with the plot area of 200 m2 (16 m × 12.5 m); the plots were separated by ridges using plastic film, and the irrigation and drainage in each plot were performed separately. Ducklings were introduced into the RD area with a density of 225 ducks ha-1 on the 17th day after transplanting. The RD fields were surrounded by nylon nets (1 m in height) to prevent the ducks from escaping, and a shed for the ducks was also built in the corner of each RD plot. The ducks were retrieved at the HD. A standing water of about 5–8 cm was maintained in the field during the period of raising ducks.
Parameter Measurements
Climatic Conditions
The wind speed and CO2 concentration during the rice growing period from May to October were provided by the local Meteorological Station. From the booting stage to the grain filling stage, three weather types were chosen, i.e., sunny days, cloudy days, and overcast days to measure light intensity by an illuminometer (TES1339, Lexian Electronic Technology Company, China). For 3 days, the light intensity was tested simultaneously on each day at 20 cm above the rice canopy inside and outside the nets in the morning (9:00–10:00), at noon (12:00–13:00), and in the afternoon (15:00–16:00), and the light intensity was tested five times at 10-min intervals.
Chlorophyll Content
A SPAD-502 chlorophyll meter was used to estimate the SPAD values of the top leaf (all of the expanding leaves on the top) at the main growth stages, i.e., the TP, ETC, EG, HD and 30 days after transplanting (30 DAH). Thirty leaves in each treatment were chosen to determine the chlorophyll contents at the upper, middle and lower positions, and the mean values were used.
Net Photosynthetic Rate and Transpiration Rate
On sunny days between 10:00 and 11:00, 10 plants in each treatment were chosen to determine the net Pn, Tr, Gs, and Ci in the top leaf (all of the expanding leaves on the top) by a gas exchange analyser (Li-6400, Li-COR, Inc., Lincolin, NE, USA) at the main growth stages, i.e., the ETC, EG, HD, and 30 DAH. For environmental factors with a relatively large influence on gas exchange parameters, before determining the leaf gas exchange parameter, the environmental conditions were controlled as follows: the flow rate was 500 μmol s-1, the CO2 concentration was 380 μmol mol-1, the temperature of leaf chamber was within ± 6°C of atmosphere temperature, and the photosynthetic active radiation intensity was 1200 μmol m-2 s-1.
Dry Matter Accumulation and N Content
Five holes of representative plants were chosen in each plot at the ETC, EG, HD, and MT. After the stems, leaves, and panicles (HD and MT) were separated, fresh samples were killed out at 105°C for 30 min and then oven-dried at 80°C until a constant weight was reached to determine the dry matter weight. Then, the samples were milled and sieved to determine their total N content by using the Kjeldahl method.
Yield Determination
In each plot, 65 m2 of rice was chosen to determine the actual yield and yield components, which mainly refer to the effective panicle number, number of grains per panicle, seed-setting rate and grain weight at the mature stage.
Analysis Methods
The dry matter or N accumulation rate (kg⋅ha-1⋅d-1) = the D-value of dry matter or N accumulation in the two aboveground samples/the interval time between the two samples.
The amounts of apparent dry matter or N translocation from vegetative organs after the heading stage (DT or NT, respectively, kg⋅ha-1) = the amounts of dry matter or N accumulation in the aboveground vegetation at the heading stage – the amounts of dry matter or N accumulation in the aboveground vegetation at the mature stage.
The apparent dry matter or N translocation efficiency from vegetative organs after the heading stage (DTE or NTE, respectively, %) = the amounts of apparent dry matter or N translocation from vegetative organs after the heading stage/the amounts of dry matter or N accumulation in the aboveground vegetation at the heading stage.
The contribution rates of the transferred dry matter or N from the vegetative organs to grain after the heading stage (DCR or NCR, respectively, %) = the amounts of apparent dry matter or N translocation from the vegetative organs after the heading stage/the amounts of dry matter or N accumulation in the grains at the mature stage.
The N recovery efficiency (NRE, %) = (the total N uptake in the N application area – the total N uptake in the area without N application)/the amount of N application × 100.
The N agronomic efficiency (NAE, %) = (the rice yield in the N application area – the rice yield in the area without N application)/the amount of applied N × 100.
The N physiological efficiency (NPE, kg⋅kg-1) = (the rice yield in the N application area – the rice yield in the area without N application)/(the total N uptake in the N application area – the total N uptake in the area without N application).
The N grain production efficiency (NGPE, kg⋅kg-1) = the rice yield/the total N uptake.
The N dry matter production efficiency (NDMPE, kg⋅kg-1) = the accumulation of aboveground dry matter at the mature stage/the total N uptake.
The N uptake per 100 kg of grains (NUG, 100 kg kg-1) = the total N uptake/the rice yield.
Data Analysis
SPSS and Office 2007 were used to process and analyze the data, and the results were expressed as the mean values of three replicates. Least significant difference (LSD) tests were used to compare the means for each treatment in the same year. Origin 8.1 was used to visualize the data, and the standard errors of the means were calculated and presented in the graphs as error bars. Analyses of variance (F-value) of rice leaf photosynthesis, dry matter accumulation, N absorption, and N utilization efficiency were performed. Then, linear relationships between the rice yield and the dry matter accumulation, N absorption and N utilization efficiency, and the significance probability levels of the results were given at ∗P < 0.05 and ∗∗P < 0.01, respectively. Based on the data analysis summarized in Table 1, the results for 2014 and 2015 showed a similar trend; accordingly, except for the rice yield and yield components, the subsequent analyses described in the text focused on the 2014 data.
Ethics Statement
This study was carried out in accordance with the Guidelines for Experimental Animals established by Ministry of science and technology of the People’s Republic of China. All experimental protocols were approved by Animal Ethics committee of Nanjing Agricultural University (Nanjing, China).
Results
Wind Speed, CO2 Concentration, and Light Intensity
Insect-proof net cultivation had significant effect on wind speed, light intensity and CO2 concentration. The wind speed of IPN was 0.01–0.73 m s-1 lower than that of OFC, and CO2 concentration of IPN was decreased by 3.62–9.52% compared to OFC (Figure 1). Regardless of what the weather type was, IPN significantly decreased the light intensity in the nets compared to OFC (Table 2).
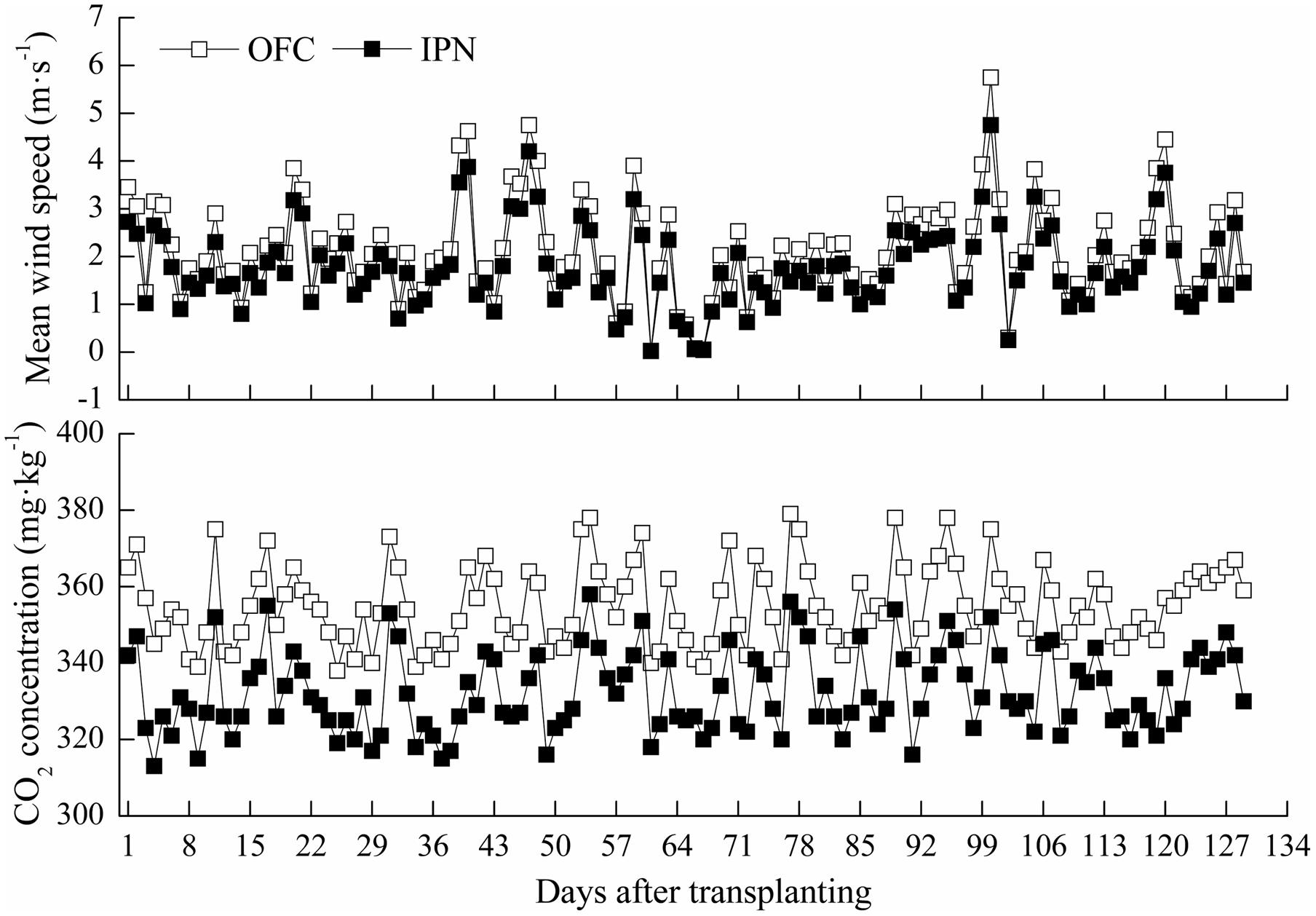
FIGURE 1. The effects of IPN on the wind speed and CO2 concentration of the rice fields. OFC, open field cultivation; IPN, insect-proof net cultivation.
Rice Yield
There were significant differences in rice yields between IN and OM (Figure 2). The rice yields of WS and BR were 2.11–4.28 and 4.78–7.67% higher than that of IN, respectively. The higher rice yield of WS was mainly attributed to more grains per panicle, and the greater rice yield of BR was attributed to the more effective panicle number or grain number per panicle (Table 3). The rice yields showed significant differences between CR and RD. The rice yield of RD was 1.52–3.74% higher than that of CR, mainly because of the more effective panicle number, grain number per panicle, seed-setting rate and grain weight.
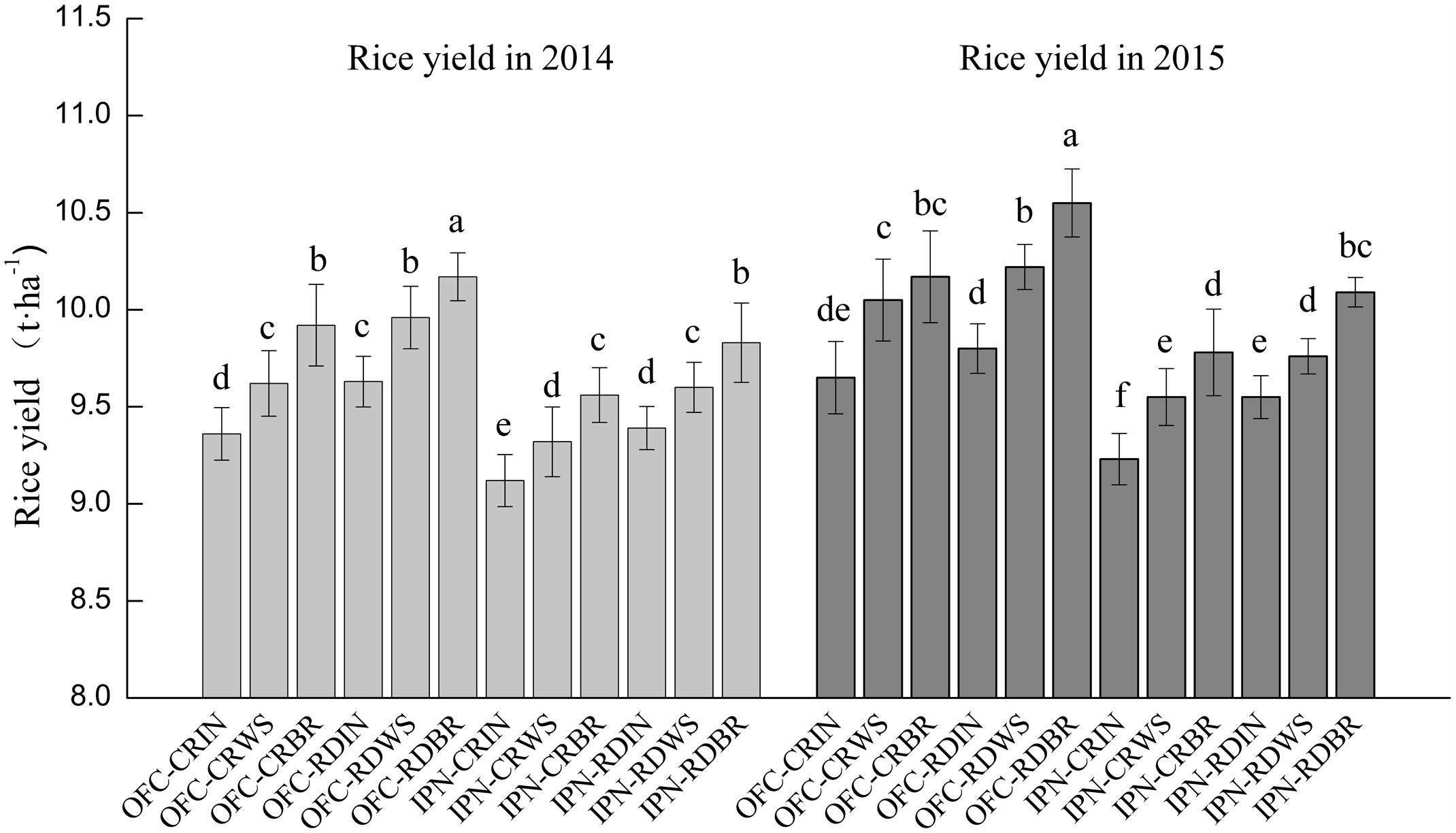
FIGURE 2. The effects of IPN, RD, and OM on the rice yield. IN, inorganic N fertilizer; WS, wheat straw return; BR, biogas residue return; CR, conventional rice farming; RD, rice-duck farming; OFC, open field cultivation; IPN, insect-proof net cultivation. Different letters above the column indicate significant differences at P < 0.05. Vertical bars represent the standard errors of means.
Compared to OFC, IPN significantly decreased the rice yield by 2.48–4.98% due to a lower effective panicle number (Figure 2; Table 3), which suggested that insect-proof net mulching was not beneficial for rice yield. The rice yield of the interaction between RD and OM was increased by 5.26–9.33% compared to the interaction between CR and IN due to the higher grain number per panicle of WS and the greater effective panicle number, grain number per panicle and grain weight of BR (Table 3). The rice yields of 2014 were 1.19–4.49% lower than those of 2015. The lower rice yields in 2015 mainly resulted from the temperature during the later stages of growth in 2014, which was not beneficial for rice growth.
Photosynthesis in Leaves
During rice growth, the SPAD values increased gradually from the TP to HD and peaked at the HD before decreasing (Figure 3). No significant difference was found between IN and OM at the TP. The leaf SPAD values of WS and BR were lower than those of IN at the ETC and EG. However, at the HD and 30 DAH, WS increased the leaf SPAD values by 4.01–5.13 and 2.99–5.86%, respectively, and BR increased them by 5.71–7.47 and 8.38–8.64%, respectively, compared to IN. The leaf SPAD values of RD were higher than those of CR. There were significant differences in the leaf SPAD values between CR and RD at the ETC and EG, and RD increased those by 5.54–7.19 and 4.50–5.98%, respectively. Compared to OFC, the leaf SPAD values were increased by IPN, indicating IPN could promote the leaf chlorophyll content. At the ETC and EG, the interaction between RD and WS decreased the leaf SPAD values while the interaction between RD and BR showed the opposite trend. However, at the HD and 30 DAH, the interaction between RD and OM increased the leaf SPAD values compared to the interaction between CR an IN.
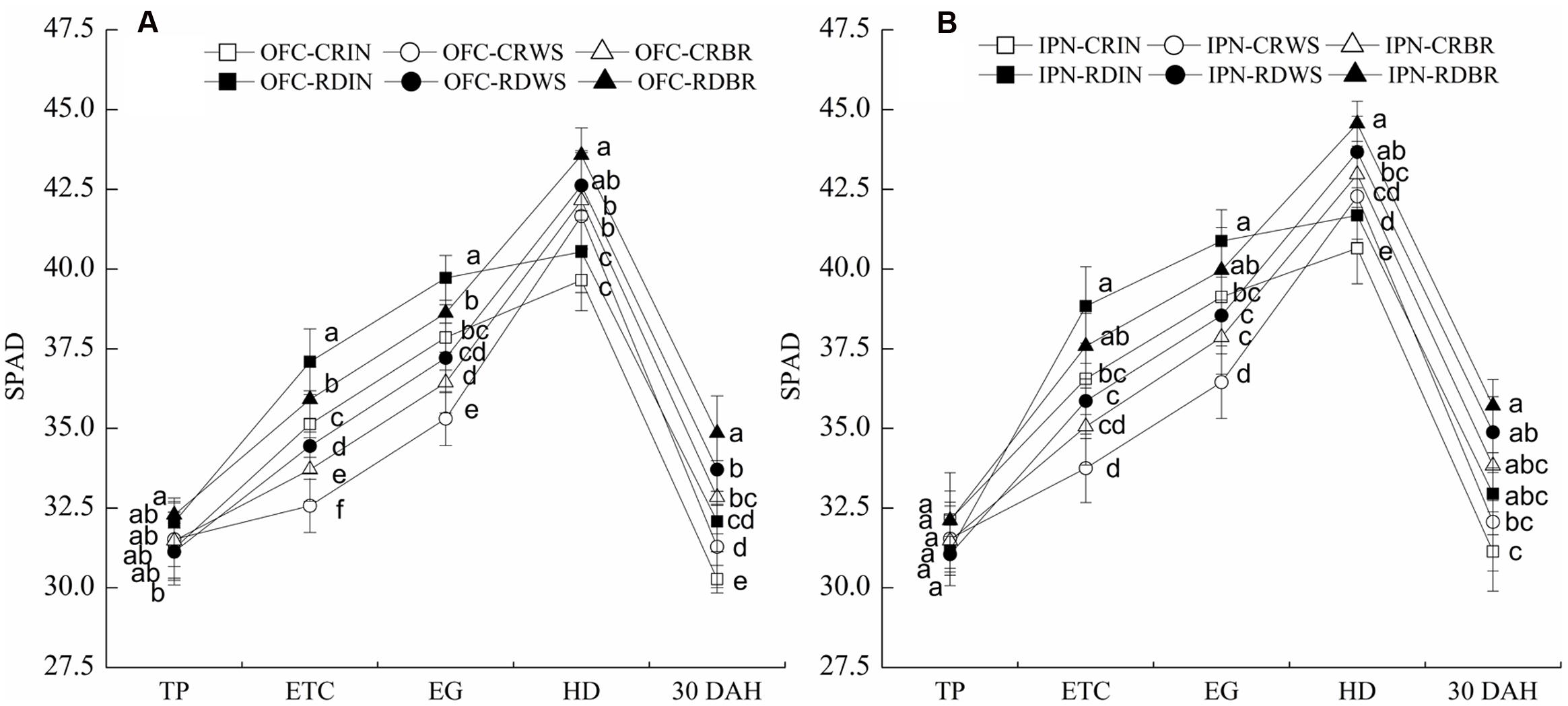
FIGURE 3. The effects of IPN, RD, and OM on the leaf SPAD values of rice. (A: open field cultivation) and (B: insect-proof net cultivation). TP, transplanting stage; ETC, effective tiller critical leaf stage; EG, elongation stage; HD, heading stage; 30 DAH, 30 days after heading; IN, inorganic N fertilizer; WS, wheat straw return; BR, biogas residue return; CR, conventional rice farming; RD, rice-duck farming; OFC, open field cultivation; IPN, insect-proof net cultivation. Different letters in the figure indicate significant differences at P < 0.05. The vertical bars represent the standard errors of means.
In the rice growth process, the leaf Pn, Tr, and Gs initially increased, peaked at the HD and then decreased, while Ci exhibited the opposite pattern (Table 4). In contrast with IN, the Pn, Tr, Gs of WS and BR were lower at the ETC and EG. However, at the HD and 30 DAH, both WS and BR contributed to higher leaf Pn, Tr, and Gs than those of IN, while the trend of Ci was the opposite. At the ETC, EG, HD and 30 DAH, the Pn, Tr, and Gs of RD were higher than those of CR, and Ci exhibited the opposite pattern. For the coverage with insect-proof nets, the leaf Pn, Tr, and Gs of IPN were decreased but the Ci was increased compared to OFC. Regarding the interaction between RD and OM, there was no significant influence on leaf photosynthetic characteristics. The Pn, Tr, and Gs of the interaction between RD and OM were all higher than those of the interaction between CR and IN, while Ci showed the opposite trend.
Dry Matter Accumulation and Translocation
During rice growth, the amount and ratio of dry matter accumulation increased, and the dry matter accumulation rate gradually increased and reached its peak from the EG to HD, then decreased (Table 5). Compared to IN, WS, and BR significantly increased the dry matter accumulation at the MT by 7.05–7.50 and 12.17–14.79%, respectively. The differences in the DT, DTE, and DCR were significant between IN and OM. WS and BR increased the DT by 12.72–15.32 and 23.90–27.46%, respectively, and improved the DTE and DCR. WS and BR had lower dry matter accumulation, accumulation ratio and rate than IN from the TP to EG but higher values than IN from the EG to MT.
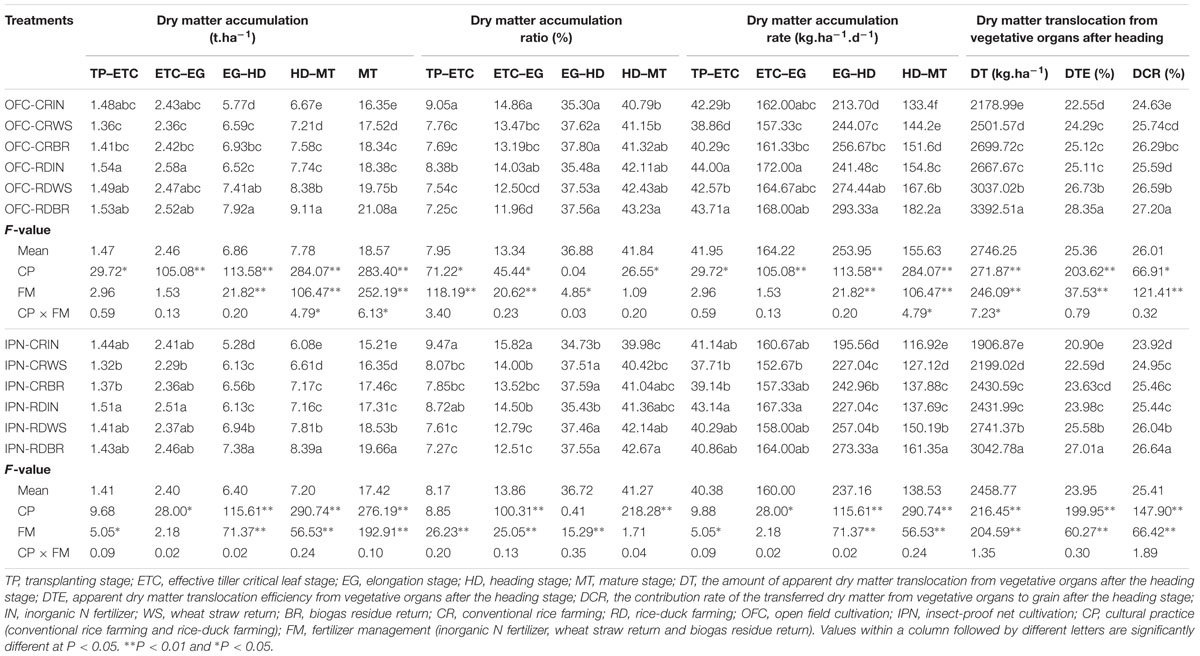
TABLE 5. The effects of IPN, RD, and OM on the dry matter accumulation and translocation characteristics of rice.
There were significant differences in the DT, DTE, DCR and the dry matter accumulation at the MT (Table 5). Compared to CR, RD increased the DT and the dry matter accumulation at the MT by 21.40–27.54 and 12.42–14.94%, respectively. RD had higher DTE, DCR, dry matter accumulation and accumulation rate from the TP to MT, while the dry matter accumulation ratio was lower than that of CR from the TP to EG but higher than that of CR from the EG to MT. However, IPN decreased the DT and the dry matter accumulation at the MT by 8.84–12.49 and 4.80–6.97%, respectively, and it also declined the DTE, DCR and dry matter accumulation compared to OFC. The interaction between RD and OM had no significant influence on the dry matter accumulation and translocation. However, compared to the interaction between CR and IN, the interaction between RD and OM increased the DT and the dry matter accumulation at the MT by 39.38–59.57 and 20.80–29.26%, respectively, and improved the DTE and DCR.
The correlation analysis indicated that the dry matter accumulations from the TP to EG, from the HD to MT and during the MT were positively correlated with the rice yields under IN, WS and BR, respectively (Table 6). There were significantly positive correlations between the dry matter accumulation from the EG to MT, during the MT and the rice yields under CR, RD, OFC, and IPN, respectively. The positive correlations between the DT, the DTE, the dry matter accumulation and the rice yield were found. These results suggested that the high dry matter accumulation and translocation were beneficial to enhance the rice yield.
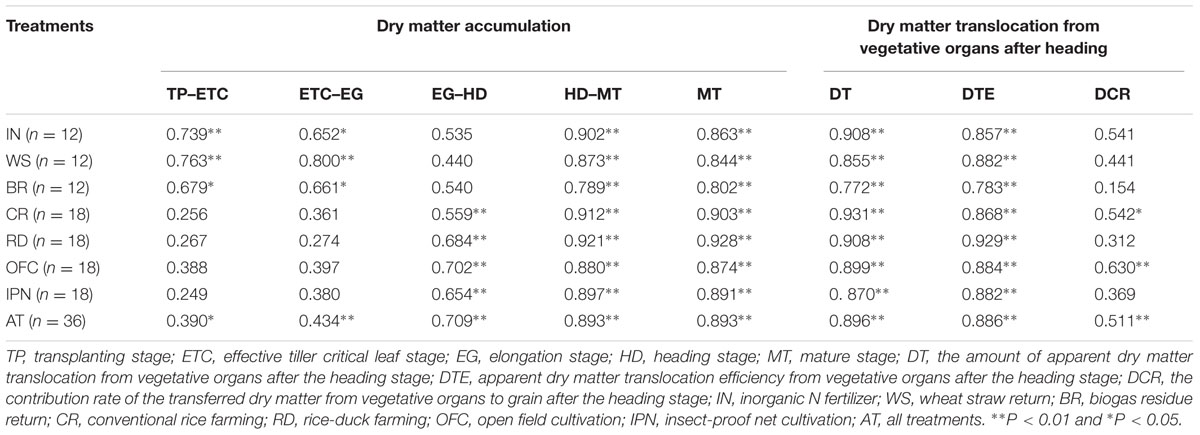
TABLE 6. The effects of IPN, RD, and OM on the correlations between rice yield and the dry matter accumulation and translocation.
Nitrogen Accumulation and Translocation
There were significant differences in the N accumulation and translocation between IN and OM. Compared to IN, WS increased the NT and the N accumulation at the MT by 8.72–12.64 and 5.39–7.62%, respectively, and BR increased those by 16.86–23.57 and 10.35–15.07%, respectively (Table 7). WS and BR had higher NTE and NCR than IN. However, the N accumulation, uptake ratio and rate were lower than those of IN from the TP to EG but higher than those of IN from the EG to MT.
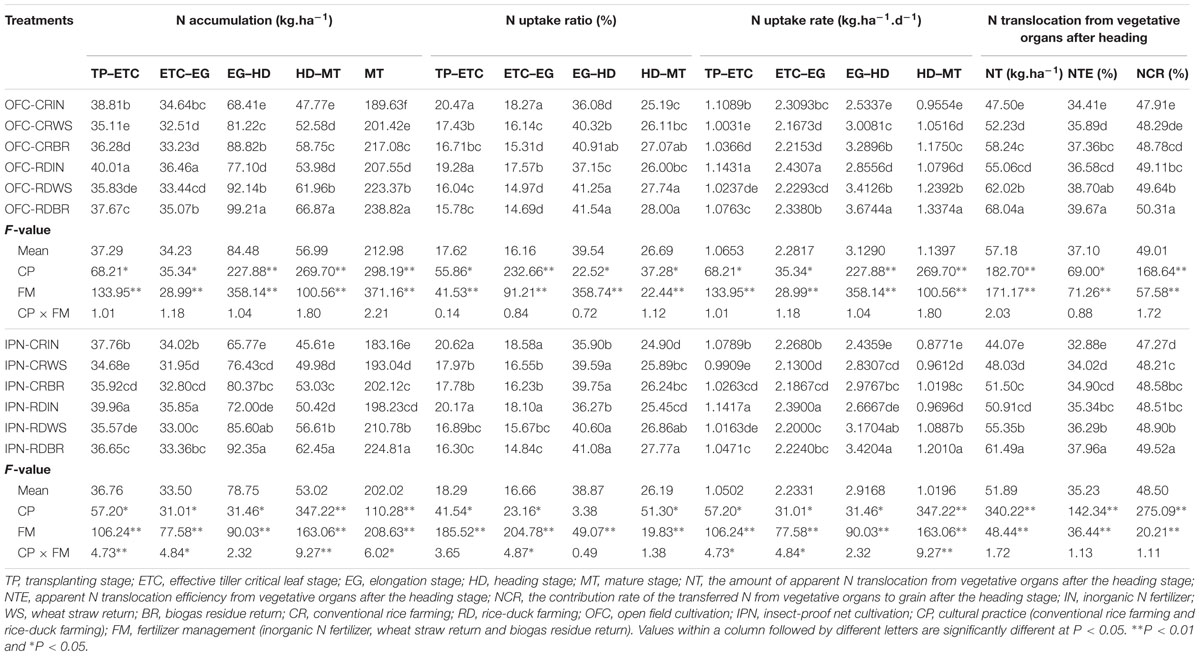
TABLE 7. The effects of IPN, RD, and OM on the N accumulation and translocation characteristics of rice.
Significant differences were found in the N accumulation and translocation between CR and RD. Compared to CR, RD increased the NT and the N accumulation at the MT by 15.24–19.40 and 8.23–11.23%, respectively (Table 7). The NTE, NCR, N accumulation and uptake rate from the TP to MT of RD were higher than those of CR; the N uptake ratio of RD was lower than that of CR from the TP to EG but higher than that of CR from the EG to MT. Compared to OFC, the NT and the N accumulation at the MT of IPN were decreased by 7.22–11.57 and 3.41–6.89%, respectively. Meanwhile, IPN had a lower NTE, NCR, and N accumulation than OFC. Regarding the interaction between RD and OM, the NT, NTE, NCR, and N accumulation at the MT were higher than those of the interaction between CR and IN (Table 7). The interaction between RD and OM increased the NT and the N accumulation at the MT by 25.60–43.24 and 15.08–25.94%, respectively, which were higher than the single OM or RD.
Correlation analysis showed that the N accumulations from the TP to MT and during the MT were positively correlated with the rice yields of IN, WS, and BR, respectively (Table 8). The N accumulations from the EG to MT and during the MT were positively correlated with the rice yields under CR, RD, OFC and IPN, respectively. The positive correlations were found between the NT, the NTE, the N accumulation from the EG to MT, during the MT and the rice yield. These results suggested that the relatively strong N accumulation after the EG was important for achieving high yield.
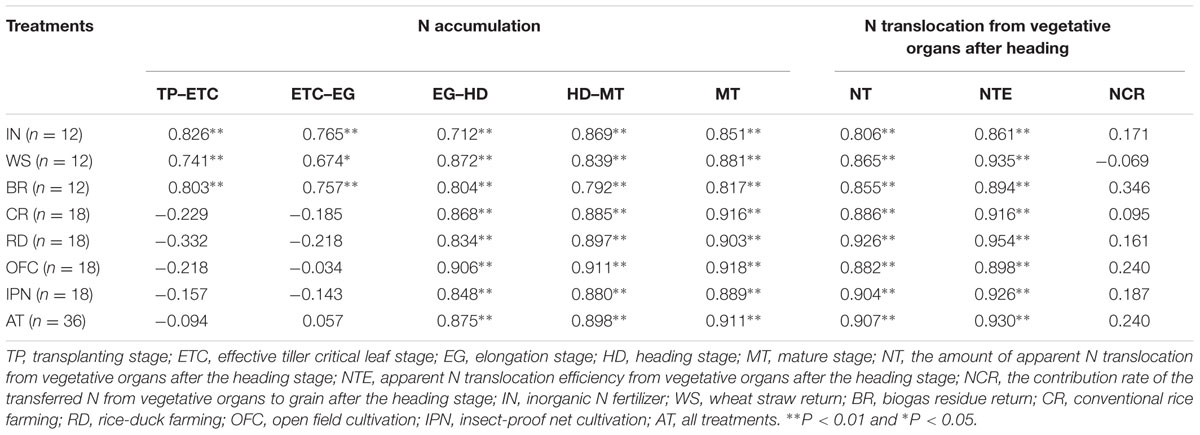
TABLE 8. The effects of IPN, RD, and OM on the correlations between the yield and the N accumulation and translocation of rice.
Nitrogen Utilization Efficiency
There were noticeable differences in NRE between IN and OM. Compared to IN, WS, and BR increased NRE by 12.06–14.88 and 23.11–31.01%, respectively (Table 9). WS and BR improved the NAE and NUG, while decreased the NPE and NGPE. Under OFC, the NDMPE of WS and BR was lower than that of IN (except for the treatment OFC-CRWS), while IPN showed an opposite pattern. These results indicated that the decomposition of organic matter could be affected by insect-proof net mulching.
Compared to CR, RD significantly increased the NRE by 18.39–22.48% (Table 9). RD also increased the NAE, NDMPE, and NUG but decreased the NPE and NGPE. However, the NRE of IPN was 7.32–12.91% lower than that of OFC. Compared to OFC, IPN decreased the NAE and NUG but increased the NPE and NGPE. The NDMPE of IPN was lower than that of OFC (except for the treatment IPN-CRBR). The interaction between RD and OM increased the NRE by 33.67–55.57% (Table 9). Meanwhile, the interaction between RD and OM had higher NAE, NDMPE, and NUG but lower NPE and NGPE compared to the interaction between CR and IN.
Correlation analysis indicated that there were positive correlations between the NRE, the NAE and the rice yield under WS and BR (Table 10). The rice yields under IN, CR, RD, OFC, and IPN were positively correlated with the NRE, NAE, and NDMPE but were negatively correlated with the NPE and NGPE. The rice yield had positive correlations with NRE, NAE, NDMPE, and NUG but had negative correlations with the NPE and NGPE, which suggested that increasing the NRE and NAE were beneficial to improve the rice yield.
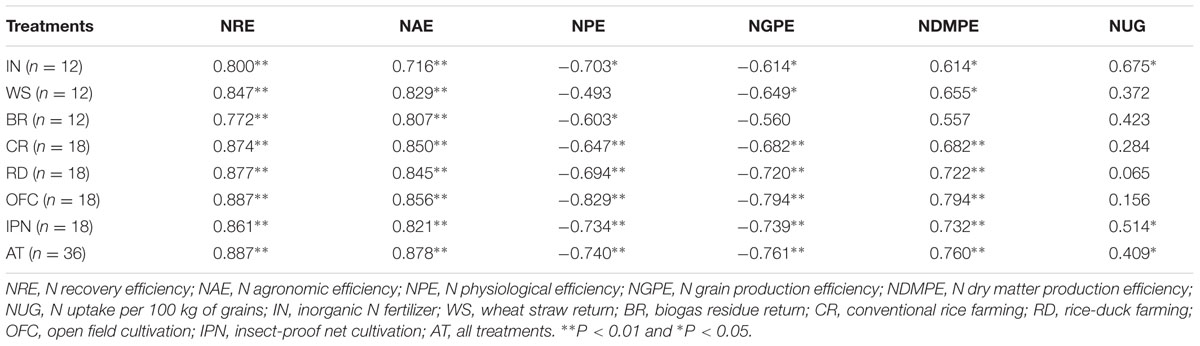
TABLE 10. The effects of IPN, RD, and OM on the correlations between the rice yield and the N utilization efficiency.
Discussion
Dry Matter Accumulation and Translocation Characteristics, and Their Relationships with Rice Yield
Dry matter accumulation and translocation could limit rice yield, as shown by the dry matter accumulation and dry matter translocation ratio to grains (San-oh et al., 2004). In the present study, WS and BR decreased the dry matter accumulation from the TP to EG but increased the dry matter accumulation from the EG to MT (Table 5). This was primarily because the microorganisms increased rapidly and consumed a portion of the mineral N after returning wheat straw into the soil (Chen et al., 2014). On the other hand, wheat straw decomposition produced reducing harmful substances at an earlier stage, which influenced rice root growth (Bradford and Peterson, 2000). However, at later stage, the degradation of soil microorganisms produced large amounts of organic matter and physiological activators, which improved soil fertility (Hao et al., 2010). OM didn’t benefit rice population development at an earlier stage but was beneficial for the development at middle and later stages. The results were similar to the findings of Ye et al. (2008) that the application of wheat straw could lengthen photosynthetic time, improve photosynthetic efficiency and promote the translocation of photosynthetic products to grains. However, the researches by Rao and Mikkelsen (1976) showed that straw return had adverse effects on rice growth and nutrition, which might be due to the different planting methods.
Rice-duck farming improved the leaf area index and effective leaf area ratio in the middle and lower parts of rice and enhanced the leaf photosynthetic ability, which provided a foundation for high yield (Liu et al., 2015). In this study, RD promoted the dry matter accumulation and translocation, and increased the rice yield (Table 5; Figure 2), which might be due to the fact that the feeding habits and activities of the ducks stimulated rice growth; on the other hand, the intertillage and manure fertilizer promoted the formation of a strong source and efficient flow and resulted in great sink activity. However, these results were different from the reports of Zhang et al. (2010) who showed that the organic rice yield of RD was lower than that of CR and was not beneficial to improve the rice yield, and the differences were mainly due to the different planting density. Facing a large area of crop lodging due to excessive fertilization in rice production, RD provide a new farming mode in which ducks play a role in controlling weeds, fertilizing rice plants, enhancing lodging resistance of the rice stalks and easing yield loss (Wang et al., 2008).
The major effect of shading is the reduction of light intensity (Chan and Mackenzie, 1972). Shading resulted in a decrease in rice yield (Moula, 2009). In the study, IPN increased the leaf SPAD values of rice in a netting house (Figure 3), while decreased the Pn, dry matter accumulation and translocation, and rice yield (Tables 4 and 5; Figure 2), possibly because IPN reduced the wind speed, air motion, CO2 content and light intensity (Figure 1; Table 2), which inhibited photosynthesis and dry matter accumulation, and was not conducive to the rice production. These results were similar to the reports of Wang L. et al. (2015) who showed that shading increased the flag leaf chorophyll content but decreased the net photosynthetic rate and grain yield. The interaction between RD and OM promoted the dry matter accumulation from the EG to MT, the dry matter translocation and rice yield (Table 5; Figure 2), which might lie in the activities of the ducks in the field, which potentially accelerated the decomposition of wheat straw and biogas residue and promoted the release of nutrients.
The relatively strong light absorption, translocation, and utilization ability of flag leaves promoted dry matter accumulation, and the relatively high dry matter accumulation at a late stage was the basis for grain filling (Zhang et al., 2003). In the study, the dry matter accumulation and translocation were positively correlated with the rice yield (Table 6). The results were in conformity with the findings of Deng et al. (2015) that the rice yield was positively correlated with the dry matter accumulation after panicle initiation stage and the post-anthesis transfer of accumulated dry matter into grain. Therefore, an increase in dry matter accumulation was helpful for the improvement of rice production.
Nitrogen Accumulation and Translocation, Nitrogen Utilization Efficiency, and their Relationships with Rice Yield
N is an indispensable nutrient for rice growth, and the supply of N strongly regulates rice yield (Yousaf et al., 2016), N absorption and translocation. The chlorophyll content of rice leaves is an active component of N utilization and is closely related to leaf photosynthetic ability (Shiratsuchi et al., 2006; Ata-Ul-Karim et al., 2016). In this study, WS and BR decreased the leaf SPAD values, Pn, N accumulation before the EG and N translocation but increased the N accumulation from the EG to MT and N translocation (Figure 3; Tables 4 and 7). The results might be that the high C/N ratio of wheat straw promoted the mass propagation of microorganisms, which competed with rice for N after being returned to the field. This led to a decrease in the amount of soil N taken up by the plants at earlier stage (Xiong et al., 2015). Afterward, organic matter gradually decomposed and released the nutrient substances, which was beneficial for the N absorption of rice. OM promoted the cycling of organic matter and relieved environmental problems resulting from the use of large quantities of chemical N fertilizer.
Rice-duck farming played an important regulatory role in alleviating nutrient shortages. In this study, RD increased the amount of N accumulation and translocation (Table 7), which might be that the return of duck manure to the soil and the activities of the ducks increased the amount of N taken up by the plants. The results were similar to the findings of Yu et al. (2009) and Zhang (2012). In this study, because of the insect-proof net mulching, IPN decreased the N accumulation and translocation. However, the interaction between RD and OM increased the N accumulation from the EG to MT and the N translocation, which were higher than single RD or OM (Table 7), and this might be due to the dual influences of RD and OM. Sun et al. (2012) found that the rice yield was significantly and positively correlated with N accumulation and translocation. In this study, the N accumulation from the EG to MT and the N translocation were positively correlated with the rice yield (Table 8). The results showed that the N accumulation from the EG to MT played a vital role in N accumulation and the greater N accumulation between the EG and the MT corresponded with the higher rice yield.
The N utilization efficiency of rice involves carbohydrate metabolism, nutrient signal transmission, and protein synthesis and degradation within plants as well as regulatory feedback via bioactivators (Chen et al., 2003). Therefore, it is important to study the N utilization efficiency of plants with respect to rice growth and yield (Massel et al., 2016). In the study, OM increased NRE, NAE and NUG but decreased NPE and NGPE (Table 9), which was similar to the reports of Yan et al. (2015) that rice N accumulation, NRE and NAE were significantly increased under WS. In addition, the present study showed that IPN decreased NRE and NAE and was associated with a risk of reducing nutrient utilization. However, RD and the interaction between RD and OM had the higher NRE, NAE, and NUG (Table 9). The results indicated that OM, RD and the interaction between RD and OM provided the methods for accumulating the high N content.
In this study, NRE, NAE, NDMPE, and NUG were positively correlated with rice yield but negatively correlated with NPE and NGPE (Table 10), which were consistent with the reports of Ntanos and Koutroubas (2002) and Li et al. (2014). However, Peng et al. (2006) noted that the internal N utilization efficiency (NGPE) was supplementary to the NAE. The results were different from the present study, the differences might be due to the fact that the greater increment in the rice N accumulation than in the rice yield observed in the present study. Higher NRE, NAE, NDMPE, and NUG and lower NPE and NGPE corresponded with higher rice yield. Accordingly, it is possible to increase dry matter and N accumulation while achieving high yield and high N utilization efficiency.
Conclusion
Organic matter return increased the dry matter accumulation, N absorption and utilization after EG and also improved the NRE and rice yield due to the high photosynthesis. The magnitude of the increase in rice yield was greater for BR than for WS. RD had the greater rice leaf photosynthesis, dry matter and N accumulation, dry matter and N translocation, and NRE, which finally resulted in the higher rice yield. However, insect-proof nets decreased the intensity of the radiation reaching the plants and therefore were not beneficial for the dry matter accumulation and translocation, N accumulation and utilization, NRE and rice yield. The interaction between RD and OM promoted the leaf Pn, dry matter accumulation, N absorption, NRE and rice yield of rice, and the effect of the interaction between RD and OM was better than that of single RD or OM. In addition, the dry matter accumulation, the N accumulation from the EG to MT, dry matter and N translocation were positively correlated with the rice yield. Therefore, OM, RD and the interaction between RD and OM contribute to increasing the rice yield, which can relieve the pressure of global food.
Author Contributions
QW and XL conceived and designed the research. XL, GX, and YH carried out the experiments. QW, XL, and GX analyzed experimental data. XL and QW wrote the main manuscript text. All authors reviewed the manuscript.
Funding
This study was financially supported by the central finance for agricultural innovative technology extension [TG (15)030, TG (15)107, TG (16)006], the key technology R&D Program of Jiangsu province (BE2013355), Agrotechnical Innovation Projects of Jiangsu Province (SXGC [2015]112, SXGC [2015]259, SXGC [2016]309), Key R&D projects of huaian city (HAC2015017).
Conflict of Interest Statement
The authors declare that the research was conducted in the absence of any commercial or financial relationships that could be construed as a potential conflict of interest.
Abbreviations
BR, biogas residue return; Ci, intercellular CO2 concentration; CR, conventional rice farming; DCR or NCR, the contribution rate of the transferred dry matter or N, respectively, from vegetative organs to grain after the heading stage; DT or NT, the amount of apparent dry matter or N translocation, respectively, from vegetative organs after the heading stage; DTE or NTE, apparent dry matter or N translocation efficiency, respectively, from vegetative organs after the heading stage; 30 DAH, 30 days after heading; EG, elongation stage; ETC, effective tiller critical leaf stage; Gs, stomatal conductance; HD, heading stage; IN, inorganic N fertilizer; IPN, insect-proof net cultivation; MT, mature stage; NAE, N agronomic efficiency; NDMPE, N dry matter production efficiency; NGPE, N grain production efficiency; NPE, N physiological efficiency; NRE, N recovery efficiency; NUG, N uptake per 100 kg of grains; OFC, open field cultivation; OM, organic matter return; Pn, net photosynthetic rate; RD, rice-duck farming; TP, transplanting stage; Tr, transpiration rate; WS, wheat straw return.
References
Ata-Ul-Karim, S. T., Cao, Q., Zhu, Y., Tang, L., Rehmani, M. I., and Cao, W. X. (2016). Non-destructive assessment of plant nitrogen parameters using leaf chlorophyll measurements in rice. Front. Plant Sci. 7:1829. doi: 10.3389/fpls.2016.01829
Bradford, J. M., and Peterson, G. A. (2000). “Conservation tillage,” in Handbook of Soil Science, ed. M. E. Summer (Boca Raton, FL: CRC Press), G247–G269.
Chan, W., and Mackenzie, A. F. (1972). Effects of shading and nitrogen on growth of corn (Zea mays L.) under field conditions. Plant Soil 36, 59–70. doi: 10.1007/BF01373457
Chen, L., Zhang, J. B., Zhao, B. Z., Yan, P., Zhou, G. X., and Xin, X. L. (2014). Effects of straw amendment and moisture on microbial communities in Chinese fluvo-aquic soil. J. Soils Sediments 14, 1829–1840. doi: 10.1007/s11368-014-0924-2
Chen, Q. S., Yi, K. K., Huang, G., Wang, X. B., Liu, F. Y., Wu, Y. R., et al. (2003). Cloning and expression pattern analysis of N-starvation-induced genes in rice. Acta Bot. Sin. 45, 974–980.
Chen, X., Cui, Z., Vitousek, P. M., Cassman, K. G., Matson, P. A., Bai, J., et al. (2011). Integrated soil-crop system management for food security. Proc. Natl. Acad. Sci. U.S.A. 108, 6399–6404. doi: 10.1073/pnas.1101419108
Deng, F., Wang, L., Ren, W. J., Mei, X. F., and Li, S. X. (2015). Optimized nitrogen managements and polyaspartic acid urea improved dry matter production and yield of indica hybrid rice. Soil Till. Res. 145, 1–9. doi: 10.1016/j.still.2014.08.004
Guo, Z., Liu, H. J., Yuan, H. Y., Yang, G. Y., Zheng, J. C., and Chen, L. G. (2015). Insect-proof nets affect paddy field microclimate parameters and grain quality of different japonica rice varieties. J. Crop Sci. Biotechnol. 18, 73–81. doi: 10.1007/s12892-014-0018-0
Hao, J. H., Ding, Y. F., Wang, Q. S., Liu, Z. H., Li, G. H., Qiao, J., et al. (2010). Effect of wheat crop straw application on the quality of rice population and soil properties. J. Nanjing Agric. Univ. 33, 13–18.
Hu, L. L., Ren, W., Tang, J., Li, N., Zhang, J., and Chen, X. (2013). The productivity of traditional rice-fish co-culture can be increased without increasing nitrogen loss to the environment. Agric. Ecosyst. Environ. 177, 28–34. doi: 10.1016/j.agee.2013.05.023
Kant, S., Swnwweera, S., Rodin, J., Materne, M., Burch, D., Rothstein, S. J., et al. (2012). Improving yield potential in crops under elevated CO2: integrating the photosynthetic and nitrogen utilization efficiencies. Front. Plant Sci. 3:162. doi: 10.3389/fpls.2012.00162
Khush, G. S. (2005). What it will take to feed 5.0 billion rice consumers in 2030. Plant Mol. Biol. 59, 1–6. doi: 10.1007/s11103-005-2159-5
Li, M., Zhang, H. C., Yang, X., Ge, M. J., Ma, Q., Wei, H. Y., et al. (2014). Accumulation and utilization of N, phosphorus and potassium of irrigated rice cultivars with high productivities and high N utilization efficiencies. Field Crops Res. 161, 55–63. doi: 10.1016/j.fcr.2014.02.007
Liu, C., Lu, M., Cui, J., Li, B., and Fang, C. M. (2014). Effects of straw carbon input on carbon dynamics in agricultural soils: a meta-analysis. Glob. Change Biol. 20, 1366–1381. doi: 10.1111/gcb.12517
Liu, L. X., Chen, H. T., and Han, Y. J. (2010). Determination and analysis of physical characteristics and fiber chemical composition of biogas residue. Trans. Chin. Soc. Agric. Eng. 26, 277–280.
Liu, X., Wang, Q. S., Xu, G. C., and Feng, J. X. (2015). Ecological effect and technology mode of rice-duck farming system. Chin. Agric. Sci. Bull. 31, 90–96.
Massel, K., Campbell, B. C., Mace, E. S., Tai, S. S., Tao, Y. F., Worland, B. G., et al. (2016). Whole genome sequencing reveals potential new targets for improving nitrogen uptake and utilization in Sorghum bicolor. Front. Plant Sci. 7:1544. doi: 10.3389/fpls.2016.01544
Ntanos, D. A., and Koutroubas, S. D. (2002). Dry matter and N accumulation and translocation for indica and japonica rice under Mediterranean conditions. Field Crops Res. 74, 93–101. doi: 10.1016/S0378-4290(01)00203-9
Peng, S. B., Buresh, R. J., Huang, J. L., Yang, J. C., Zhou, Y. B., Zhong, X. H., et al. (2006). Strategies for overcoming low agronomic N utilization efficiency in irrigated rice systems in China. Field Crops Res. 96, 37–47. doi: 10.1016/j.fcr.2005.05.004
Rao, D. N., and Mikkelsen, D. S. (1976). Effect of rice straw incorporation on rice plant growth and nutrition. Agron. J. 68, 752–755. doi: 10.2134/agronj1976.00021962006800050017x
San-oh, Y., Mano, Y., Ookawa, T., and Hirasawa, T. (2004). Comparison of dry matter production and associated characteristics between direct-sown and transplanted rice plants in a submerged paddy field and relationships to planting patterns. Field Crops Res. 87, 43–58. doi: 10.1016/j.fcr.2003.09.004
Seufert, V., Ramankutty, N., and Foley, J. A. (2012). Comparing the yields of organic and conventional agriculture. Nature 485, 229–232. doi: 10.1038/nature11069
Shiratsuchi, H., Yamagishi, T., and Ishii, R. (2006). Leaf N distribution to maximize the canopy photosynthesis in rice. Field Crops Res. 95, 291–304. doi: 10.3732/ajb.0800019
Suh, J. (2014). Theory and reality of integrated rice-duck farming in Asian developing countries: a systematic review and SWOT analysis. Agric. Syst. 125, 74–81. doi: 10.1016/j.agsy.2013.11.003
Sun, Y. J., Ma, J., Sun, Y. Y., Xu, H., Yang, Z. Y., Liu, S. J., et al. (2012). The effects of different water and N managements on yield and N utilization efficiency in hybrid rice of China. Field Crops Res. 127, 85–98. doi: 10.1016/j.fcr.2011.11.015
Wang, L., Deng, F., and Ren, W. J. (2015). Shading tolerance in rice is related to better light harvesting and use efficiency and grain filling rate during grain filling period. Field Crops Res. 180, 54–62. doi: 10.1016/j.fcr.2015.05.010
Wang, Q. S., Zhen, R. H., Ding, Y. F., and Wang, S. H. (2008). Strong stem effect and physiological characteristics of rice plant under rice-duck farming. Chin. J. Appl. Ecol. 19, 2661–2665.
Wang, X. H., Yang, H. S., Liu, J., Wu, J. S., Chen, W. P., Wu, J., et al. (2015). Effects of ditch-buried straw return on soil organic carbon and rice yields in a rice-wheat rotation system. Catena 127, 56–63. doi: 10.1002/jsfa.7196
Xiong, R. H., Hang, Y. H., Wang, Q. S., Xu, G. C., Liu, X., and Wu, H. (2015). Wheat straw returned combined with N as base fertilizers and topdressing at tiller stage improving the tiller emergency, earbearing traits and yield for machine-transplanted super japonica rice. Trans. Chin. Soc. Agric. Eng. 31, 136–146.
Xu, G. C., Liu, X., Wang, Q. S., Yu, X. C., and Hang, Y. H. (2017). Integrated rice-duck farming mitigates the global warming potential in rice season. Sci. Total Environ. 575, 58–66. doi: 10.1016/j.scitotenv.2016.09.233
Yan, F. J., Sun, Y. J., Ma, J., Xu, H., Li, Y., Dai, Z., et al. (2015). Effects of wheat straw mulching and N management on grain yield, rice quality and N utilization in hybrid rice under different soil fertility conditions. Chin. J. Rice Sci. 29, 56–64.
Ye, W. P., Xie, X. L., Wang, K. R., and Li, Z. G. (2008). Effects of rice straw manuring in different periods on growth and yield of rice. Chin. J. Rice Sci. 22, 65–70.
Yousaf, M., Li, X. K., Zhang, Z., Ren, T., Cong, R. H., Tahir, S., et al. (2016). Nitrogen fertilizer management for enhancing crop productivity and nitrogen use efficiency in a rice-oilseed rape rotation system in China. Front. Plant Sci. 7:1496. doi: 10.3389/fpls.2016.01496
Yu, X., Wang, Q. S., Wang, X. W., Wang, S. H., Jiang, Z. M., Tang, L. C., et al. (2009). Effects of the amount of basic and tillering N on population quality and N utilization of machine-transplanted rice in rice-duck farming system. Plant Nutr. Fertil. Sci. 15, 529–536.
Zeng, X. M., Han, B. J., Xu, F. S., Huang, J. L., Cai, H. M., and Shi, L. (2012). Effects of modified fertilization technology on the grain yield and nitrogen use efficiency of midseason rice. Field Crops Res. 137, 203–212. doi: 10.1016/j.fcr.2012.08.012
Zhang, F. (2012). Characteristics of nutrient return and uptake in rice-duck mutualism ecosystem of double rice cropping season. Chin. J. Eco Agric. 20, 265–269. doi: 10.3724/SP.J.1011.2012.00265
Zhang, M. M., Zong, L. G., and Xie, T. Z. (2010). Effect of integrated organic duck-rice farming on the dynamics of soil nutrient and associated economic benefits. Chin. J. Eco Agric. 18, 256–260. doi: 10.3724/SP.J.1011.2010.00256
Zhang, Y., Zang, G. Q., Tang, Z. H., Chen, X. H., and Yu, Y. S. (2014). Burning straw, air pollution, and respiratory infections in China. Am. J. Infect. Control. 42:815. doi: 10.1016/j.ajic.2014.03.015
Keywords: rice-duck farming, insect-proof net cultivation, organic matter, dry matter accumulation, N utilization
Citation: Liu X, Xu G, Wang Q and Hang Y (2017) Effects of Insect-Proof Net Cultivation, Rice-Duck Farming, and Organic Matter Return on Rice Dry Matter Accumulation and Nitrogen Utilization. Front. Plant Sci. 8:47. doi: 10.3389/fpls.2017.00047
Received: 17 September 2016; Accepted: 09 January 2017;
Published: 24 January 2017.
Edited by:
Karabi Datta, University of Calcutta, IndiaReviewed by:
Stefano Cesco, Free University of Bozen-Bolzano, ItalyZhixing Zhang, Fujian Agriculture and Forestry University, China
Quanzhi Zhao, Henan Agricultural University, China
Copyright © 2017 Liu, Xu, Wang and Hang. This is an open-access article distributed under the terms of the Creative Commons Attribution License (CC BY). The use, distribution or reproduction in other forums is permitted, provided the original author(s) or licensor are credited and that the original publication in this journal is cited, in accordance with accepted academic practice. No use, distribution or reproduction is permitted which does not comply with these terms.
*Correspondence: Qiangsheng Wang, YWVmc2xhYkAxNjMuY29t; cXN3YW5nQG5qYXUuZWR1LmNu