- 1Texas A&M AgriLife Research and Extension Center, Texas A&M University System, Weslaco, TX, USA
- 2Department of Plant Biology, Ecology, and Evolution, Oklahoma State University, Stillwater, OK, USA
- 3Department of Plant Pathology and Microbiology, Texas A&M University, College Station, TX, USA
It is increasingly evident that new environmentally sustainable agricultural practices will be necessary to support an estimated human population of 9 billion by 2050. Considering the predicted climate change-associated events such as flooding, drought, increased temperatures, and increased soil salinity, it is critical to broaden our study and use of cereals and small grains. Millets offer significant potential toward meeting these goals. The genus Setaria comprises more than one hundred species distributed in a wide range of habitats in Asia, Africa, South America, and North America. Millets, including foxtail millet (Setaria italica), pearl millet (Pennisetum glaucum) and proso millet (Panicum milaceum), are consumed by millions of humans across the world, as well as used as forage and bird feed. Recently, foxtail millet and its wild-progenitor, green foxtail millet (S. viridis), have emerged as model plant systems for studying C4 grass biology and other agronomic traits (Doust et al., 2009; Li and Brutnell, 2011; Lata et al., 2013; Mandadi et al., 2014; Xianmin et al., 2014; Brutnell, 2015; Brutnell et al., 2015). Here, we comment on the significance of S. italica and S. viridis as model systems for agronomic and translational research related to improvement of nutritional quality, biotic and abiotic stress responses, photosynthetic efficiency and biomass potential of C4 grasses.
Foxtail millet is in the Paniceae tribe (sub-family Panicoideae of the Poaceae), which also contains pearl millet and proso millet. Foxtail millet was domesticated from green foxtail in northern China ca. 8000 years ago (Barton et al., 2009), and has been widely cultivated in arid and semi-arid regions of Asia, Africa and the Americas (Lata et al., 2013). It is one of the most resilient cereal crops, with good yields in dry and marginal land with minimal agricultural inputs. Foxtail millet also adapts well to adverse weather conditions such as low and unpredictable precipitation (Lata et al., 2013; Muthamilarasan and Prasad, 2015). Furthermore, it appears to be resilient to multiple abiotic and biotic stresses such as drought (Zhang et al., 2005; Meng et al., 2009; Lata et al., 2011), salinity (Ardie et al., 2015) and fungal diseases (Xu et al., 2011). Because global climate change will have significant adverse effects on the production of other major cereals, foxtail-, pearl- and proso-millets are increasingly attractive alternatives for small grain production (Tadele, 2016).
Green foxtail millet has also come into the spotlight in recent years as an attractive alternative to more established grass model systems such as rice and maize (Figure 1). S. viridis offers several research advantages, including short stature (10–30 cm), rapid life cycle (6–9 weeks), prolific seed production (~13,000 seeds per plant), self-compatibility, small genome size (~395 Mb), diploid genetics (2n = 18), ability to be grown in controlled environments under relatively low light levels, and amenability to transformation (Devos et al., 1998; Doust et al., 2009; Brutnell et al., 2010; Li and Brutnell, 2011; Wang et al., 2011; Xianmin et al., 2014; Saha and Blumwald, 2016). Like maize, green and foxtail millets are C4 plants, but they have smaller genomes and are true diploids. In combination with sorghum (Sorghum bicolor), S. italica and S. viridis are proving valuable in studying C4 photosynthesis, with the long-term goal of engineering C4 traits into important C3 crops such as rice and wheat.
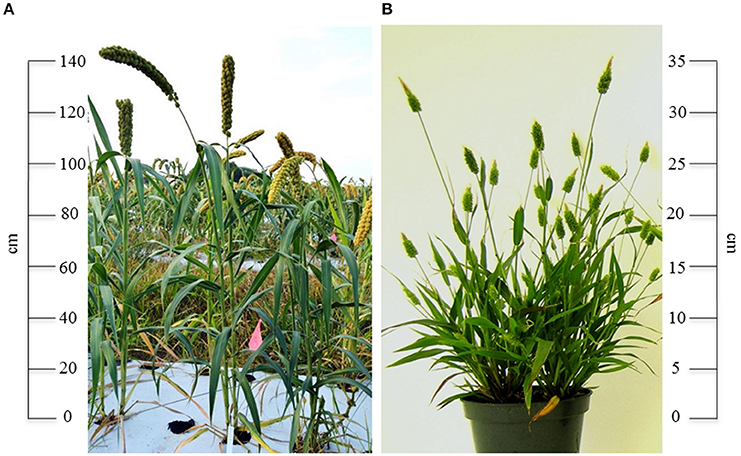
Figure 1. (A) Setaria italica and (B) S. viridis are emerging model species for millet and C4 grass biology. Photo credits: John Hodge and Jesse D. Pyle.
The S. italica and S. viridis system offer many genetic resources, including sequenced draft genomes (Bennetzen et al., 2012; Zhang et al., 2012) (Setaria viridis v1.1, DOE-JGI, http://phytozome.jgi.doe.gov) and a high-density haplotype map of genome variation (Jia et al., 2013). Comparisons of the arrangement of the nine chromosomes of S. italica and S. viridis with the corresponding chromosomes of rice and sorghum reveal relatively few rearrangements, and the genome sequence has been used to guide the assembly of the polyploid genome of its close relative, Panicum virgatum (switchgrass), a promising bioenergy feedstock (Daverdin et al., 2015). The high-quality genome sequence of foxtail millet has also allowed in-depth analyses of transposon family dynamics and locations that reveal hitherto unsuspected variation between transposon families in insertion site preference and turnover (Bennetzen et al., 2016). Mutant populations have been characterized in both foxtail millet and green foxtail millet, and the identity of candidate genes was revealed by novel high-throughput sequencing approaches (Li et al., 2016; Liu et al., 2016; Martins et al., 2016; Xue et al., 2016). S. italica and S. viridis have been used in the characterization of important agronomic traits, including yield-related architectural traits such as height, branching, biomass, flowering time and domestication-related traits such as shattering (Qian et al., 2012; Jia et al., 2013; Mauro-Herrera et al., 2013; Wang et al., 2013; Doust et al., 2014; Gupta et al., 2014; Layton and Kellogg, 2014; Qie et al., 2014; Fahlgren et al., 2015; Fang et al., 2016; Hodge and Kellogg, 2016; Liu et al., 2016; Mauro-Herrera and Doust, 2016).
In plants, salicylic acid (SA), jasmonic acid (JA) and ethylene (ET) are key defense hormones that mediate signaling during plant-microbe interaction. Prevailing evidence, primarily based on studies of dicot plants, suggest that SA and JA/ET pathways are antagonistic to each other during plant-microbe interactions. Recently, in conjunction with Brachypodium distachyon (a C3 grass), S. viridis was used to characterize the dynamics of C3 vs. C4 plant defense signaling responses against a diverse group of grass-infecting viruses (Scholthof, 1999; Mandadi and Scholthof, 2012; Mandadi et al., 2014). This study showed that SA and JA/ET crosstalk does exist in S. viridis during grass-virus interactions; however, there were also unique C4 host-dependent signaling responses. S. italica was also used to dissect grass defense signaling pathways modulated during an incompatible interaction with a fungal pathogen, Uromyces setariae-italicae (Li et al., 2015). Together, these studies demonstrate the utility of S. italica and S. viridis for fundamental research pertaining to plant-microbe interactions.
S. italica and S. viridis are also very useful for translational research related to bioenergy traits. In addition to plant-derived sugars it has been shown that cell-wall derived cellulose, hemicellulose and lignin are sustainable sources for bioenergy production. Owing to their superior carbon-assimilation and photosynthetic pathways, several C4 grasses such as switchgrass, Miscanthus, and energycane have emerged as feedstocks for plant ligno-cellulosic biomass (Brutnell et al., 2010). Efforts are underway to engineer C4 photosynthetic pathways into C3 crops (e.g., rice) to improve photosynthetic capacity and yield (von Caemmerer et al., 2012; Karki et al., 2013). However, the genomes and genetics of the leading bioenergy grasses are complex, and are a hindrance in deciphering the mechanisms of C4 photosynthesis, and ultimately to engineer them into C3 grasses. Both S. italica and S. viridis are C4 grasses and their genomes are highly syntenic to other Panicoid grasses (Kumari et al., 2013) and, in this context, these model plants offer powerful tools to understand the genetics of biomass production of panicoid bioenergy grasses, as well as to limn the evolution of C4 vs. C3 traits (Doust et al., 2009; Li and Brutnell, 2011; Lata et al., 2013; Mandadi et al., 2014; Brutnell et al., 2015; Muthamilarasan and Prasad, 2015; Martin et al., 2016).
In addition to enabling research into agronomic traits, S. italica and S. viridis are valuable models in the study of phytonutrient pathways pertaining to small grain millets. For example, S. italica is a good source of calories and essential micronutrients, and has higher nutritive value than major cereal grains like rice, wheat and sorghum. S. italica also contains high levels of proteins, dietary fibers, vitamins, anti-oxidants and non-starchy polysaccharides with low glycemic index, when compared to rice, wheat and sorghum (Taylor et al., 2006; Suma and Urooj, 2012; Amadou et al., 2013; Muthamilarasan and Prasad, 2015; Muthamilarasan et al., 2015). Moreover, over fifty phenolic compounds belonging to classes such as hydroxybenzoic acids, hydroxycinnamic acids and flavonoids were identified from S. italica (Chandrasekara and Shahidi, 2011b). In in vitro studies, phenolic compounds derived from S. italica, exhibited health promoting properties including antioxidant and free radical quenching, which helps in boosting immunity and inhibiting cancer cell proliferation (Dykes and Rooney, 2006; Chandrasekara and Shahidi, 2010, 2011a,b, 2012; Muthamilarasan and Prasad, 2015). Notwithstanding these reported nutritional qualities, certain anti-nutritional constraints were also reported for S. italica. These include the presence of toxic substances, some of which might be harmful to human health. For instance, some foxtail millet grains contain goitrogens that suppress thyroid activity which may have a role in goiter—an enlargement of the thyroid gland (Gaitan et al., 1989; Gluchoff-Fiasson et al., 1989; Elnour et al., 2000). The major goitrogenic and antithyroid compounds isolated were C-glycosylflavones (Gaitan et al., 1989; Gluchoff-Fiasson et al., 1989). Furthermore, in vivo experiments with vitexin (one of the three major C-glycosylflavones) in rats revealed evidence of antithyroid activity (Gaitan et al., 1995). In this context, further research is needed to carefully delineate the nutritional and anti-nutritional properties of phytochemicals present in S. italica and perhaps other small grain millets. The availability of S. italica and S. viridis genomic and genetic resources should enable research into identifying and inactivating such anti-nutritional compounds using novel genome-editing technologies (e.g., CRISPR-CAS9) to enhance S. italica nutritional traits.
Given the importance of S. italica as a food crop and in the study of grass biology, we suggest that S. italica, and its wild-progenitor, S. viridis, are promising translational research models to study nutritional pathways, abiotic and biotic stress resistance pathways, as well as to advance C4 photosynthesis and bioenergy research.
Author Contributions
SP, SI, AD, KS, and KM contributed to the design, preparation, and editing of the manuscript.
Funding
This manuscript was supported by funds from USDA-NIFA-AFRI (2016-67013-24738) to KS and KM, Texas A&M AgriLife Research Bioenergy/Bioproducts Grant (124738-96210) to KM, and NSF Plant Genome (IOS-1339332) to AD.
Conflict of Interest Statement
The authors declare that the research was conducted in the absence of any commercial or financial relationships that could be construed as a potential conflict of interest.
References
Amadou, I., Le, G. W., Amza, T., Sun, J., and Shi, Y. H. (2013). Purification and characterization of foxtail millet-derived peptides with antioxidant and antimicrobial activities. Food Res. Int. 51, 422–428. doi: 10.1016/j.foodres.2012.12.045
Ardie, S. W., Khumaida, N., Nur, A., and Fauziah, N. (2015). Early identification of salt tolerant foxtail millet (Setaria italica L. Beauv). Procedia Food Sci. 3, 303–312. doi: 10.1016/j.profoo.2015.01.033
Barton, L., Newsome, S. D., Chen, F. H., Wang, H., Guilderson, T. P., and Bettinger, R. L. (2009). Agricultural origins and the isotopic identity of domestication in northern China. Proc. Natl. Acad. Sci. U.S.A. 106, 5523–5528. doi: 10.1073/pnas.0809960106
Bennetzen, J. L., Schmutz, J., Wang, H., Percifield, R., Hawkins, J., Pontaroli, A. C., et al. (2012). Reference genome sequence of the model plant Setaria. Nat. Biotechnol. 30, 555–561. doi: 10.1038/nbt.2196
Bennetzen, J., Park, M., Wang, H., and Zhou, H. (2016). “LTR retrotransposon dynamics and specificity in Setaria italica,” in Genetics and Genomics of Setaria, eds A. Doust and X. Diao (Cham: Springer International Publishing). Available online at: http://www.springer.com/us/book/9783319451039#aboutBook
Brutnell, T. P. (2015). Model grasses hold key to crop improvement. Nat. Plants 1, 15062. doi: 10.1038/NPLANTS.2015.62
Brutnell, T. P., Bennetzen, J. L., and Vogel, J. P. (2015). Brachypodium distachyon and Setaria viridis: model genetic systems for the grasses. Annu. Rev. Plant Biol. 66, 465–485. doi: 10.1146/annurev-arplant-042811-105528
Brutnell, T. P., Wang, L., Swartwood, K., Goldschmidt, A., Jackson, D., Zhu, X.-G., et al. (2010). Setaria viridis: a model for C4 photosynthesis. Plant Cell 22, 2537–2544. doi: 10.1105/tpc.110.075309
Chandrasekara, A., and Shahidi, F. (2010). Content of insoluble bound phenolics in millets and their contribution to antioxidant capacity. J. Agric. Food Chem. 58, 6706–6714. doi: 10.1021/jf100868b
Chandrasekara, A., and Shahidi, F. (2011a). Antiproliferative potential and DNA scission inhibitory activity of phenolics from whole millet grains. J. Funct. Foods 3, 159–170. doi: 10.1016/j.jff.2011.03.008
Chandrasekara, A., and Shahidi, F. (2011b). Determination of antioxidant activity in free and hydrolyzed fractions of millet grains and characterization of their phenolic profiles by HPLC-DAD-ESI-MSn. J. Funct. Foods 3, 144–158. doi: 10.1016/j.jff.2011.03.007
Chandrasekara, A., and Shahidi, F. (2012). Bioaccessibility and antioxidant potential of millet grain phenolics as affected by simulated in vitro digestion and microbial fermentation. J. Funct. Foods 4, 226–237. doi: 10.1016/j.jff.2011.11.001
Daverdin, G., Bahri, B. A., Wu, X., Serba, D. D., Tobias, C., Saha, M. C., et al. (2015). Comparative relationships and chromosome evolution in switchgrass (Panicum virgatum) and its genomic model, foxtail millet (Setaria italica). Bioenergy Res. 8, 137–151. doi: 10.1007/s12155-014-9508-7
Devos, K. M., Wang, Z. M., Beales, J., Gale, M. D., and Sasaki, T. (1998). Comparative genetic maps of foxtail millet (Setaria italica) and rice (Oryza sativa). Theor. Appl. Genet. 96, 63–68. doi: 10.1007/s001220050709
Doust, A. N., Kellogg, E. A., Devos, K. M., and Bennetzen, J. L. (2009). Foxtail millet: a sequence-driven grass model system. Plant Physiol. 149, 2003–2005. doi: 10.1104/pp.108.129627
Doust, A. N., Lukens, L., Olsen, K. M., Mauro-Herrera, M., Meyer, A., and Rogers, K. (2014). Beyond the single gene: how epistasis and gene-by-environment effects influence crop domestication. Proc. Natl. Acad. Sci. U.S.A. 111, 6178–6183. doi: 10.1073/pnas.1308940110
Dykes, L., and Rooney, L. W. (2006). Sorghum and millet phenols and antioxidants. J. Cereal Sci. 44, 236–251. doi: 10.1016/j.jcs.2006.06.007
Elnour, A., Hambraeus, L., Eltom, M., Dramaix, M., and Bourdoux, P. (2000). Endemic goiter with iodine sufficiency: a possible role for the consumption of pearl millet in the etiology of endemic goiter. Am. J. Clin. Nutr. 71, 59–66.
Fahlgren, N., Feldman, M., Gehan, M. A., Wilson, M. S., Shyu, C., Bryant, D. W., et al. (2015). A versatile phenotyping system and analytics platform reveals diverse temporal responses to water availability in Setaria. Mol. Plant 8, 1520–1535. doi: 10.1016/j.molp.2015.06.005
Fang, X., Dong, K., Wang, X., Liu, T., He, J., Ren, R., et al. (2016). A high density genetic map and QTL for agronomic and yield traits in foxtail millet [Setaria italica (L.) P. Beauv.]. BMC Genomics 17:336. doi: 10.1186/s12864-016-2628-z
Gaitan, E., Cooksey, R. C., Legan, J., and Lindsay, R. H. (1995). Antithyroid effects in vivo and in vitro of vitexin: a C-glucosylflavone in millet. J. Clin. Endocrinol. Metab. 80, 1144–1147. doi: 10.1210/jcem.80.4.7714083
Gaitan, E., Lindsay, R., Reichert, R., Ingbar, S., Cooksey, R., Legan, J., et al. (1989). Antithyroid and goitrogenic effects of millet: role of C-glycosylflavones. J. Clin. Endocrinol. Metab. 68, 707–714. doi: 10.1210/jcem-68-4-707
Gluchoff-Fiasson, K., Jay, M., and Viricel, M. R. (1989). Flavone O- and C-glycosides from Setaria italica. Phytochemistry 28, 2471–2475. doi: 10.1016/S0031-9422(00)98008-7
Gupta, S., Kumari, K., Muthamilarasan, M., Parida, S. K., and Prasad, M. (2014). Population structure and association mapping of yield contributing agronomic traits in foxtail millet. Plant Cell Rep. 33, 881–893. doi: 10.1007/s00299-014-1564-0
Hodge, J. G., and Kellogg, E. A. (2016). Abscission zone development in Setaria viridis and its domesticated relative, Setaria italica. Am. J. Bot. 103, 998–1005. doi: 10.3732/ajb.1500499
Jia, G., Huang, X., Zhi, H., Zhao, Y., Zhao, Q., Diao, X., et al. (2013). A haplotype map of genomic variations and genome-wide association studies of agronomic traits in foxtail millet (Setaria italica). Nat. Genet. 45, 957–961. doi: 10.1038/ng.2673
Karki, S., Rizal, G., and Quick, W. P. (2013). Improvement of photosynthesis in rice (Oryza sativa L.) by inserting the C4 pathway. Rice 6:28. doi: 10.1186/1939-8433-6-28
Kumari, K., Muthamilarasan, M., Misra, G., Gupta, S., Subramanian, A., Parida, S. K., et al. (2013). Development of eSSR-markers in Setaria italica and their applicability in studying genetic diversity, cross-transferability and comparative mapping in millet and non-millet species. PLoS ONE 8:e67742. doi: 10.1371/journal.pone.0067742
Lata, C., Bhutty, S., Bahadur, R. P., Majee, M., and Prasad, M. (2011). Association of an SNP in a novel DREB2-like gene SiDREB2 with stress tolerance in foxtail millet [Setaria italica (L.)]. J. Exp. Bot. 62, 3387–3401. doi: 10.1093/jxb/err016
Lata, C., Gupta, S., and Prasad, M. (2013). Foxtail millet: a model crop for genetic and genomic studies in bioenergy grasses. Crit. Rev. Biotechnol. 33, 328–343. doi: 10.3109/07388551.2012.716809
Layton, D. J., and Kellogg, E. A. (2014). Morphological, phylogenetic, and ecological diversity of the new model species Setaria viridis (Poaceae: Paniceae) and its close relatives. Am. J. Bot. 101, 539–557. doi: 10.3732/ajb.1300428
Li, P., and Brutnell, T. P. (2011). Setaria viridis and Setaria italica, model genetic systems for the Panicoid grasses. J. Exp. Bot. 62, 3031–3037. doi: 10.1093/jxb/err096
Li, W., Tang, S., Zhang, S., Shan, J., Tang, C., Chen, Q., et al. (2016). Gene mapping and functional analysis of the novel leaf color gene SiYGL1 in foxtail millet Setaria italica (L.) P. Beauv. Physiol. Plant. 157, 24–27. doi: 10.1111/ppl.12405
Li, Z. Y., Wang, N., Dong, L., Bai, H., Quan, J. Z., Liu, L., et al. (2015). Differential gene expression in foxtail millet during incompatible interaction with Uromyces setariae-italicae. PLoS ONE 10:e0123825. doi: 10.1371/journal.pone.0123825
Liu, X., Tang, S., Jia, G., Schnable, J. C., Su, H., Tang, C., et al. (2016). The C-terminal motif of SiAGO1b is required for the regulation of growth, development and stress responses in foxtail millet (Setaria italica (L.) P. Beauv). J. Exp. Bot. 67:erw135. doi: 10.1093/jxb/erw135
Mandadi, K. K., Pyle, J. D., and Scholthof, K.-B. G. (2014). Comparative analysis of antiviral responses in Brachypodium distachyon and Setaria viridis reveal conserved and unique outcomes among C3 and C4 plant defenses. Mol. Plant Microbe Interact. 27, 1277–1290. doi: 10.1094/MPMI-05-14-0152-R
Mandadi, K. K., and Scholthof, K.-B. G. (2012). Characterization of a viral synergism in the monocot Brachypodium distachyon reveals distinctly altered host molecular processes associated with disease. Plant Physiol. 160, 1432–1452. doi: 10.1104/pp.112.204362
Martin, A. P., Palmer, W. M., Brown, C., Abel, C., Lunn, J. E., Furbank, R. T., et al. (2016). A developing Setaria viridis internode: an experimental system for the study of biomass generation in a C4 model species. Biotechnol. Biofuels 9, 45. doi: 10.1186/s13068-016-0457-6
Martins, P. K., Mafra, V., de Souza, W. R., Ribeiro, A. P., Vinecky, F., Basso, M. F., et al. (2016). Selection of reliable reference genes for RT-qPCR analysis during developmental stages and abiotic stress in Setaria viridis. Sci. Rep. 6:28348. doi: 10.1038/srep28348
Mauro-Herrera, M., and Doust, A. N. (2016). Development and genetic control of plant architecture and biomass in the Panicoid grass, Setaria. PLoS ONE 11:e0151346. doi: 10.1371/journal.pone.0151346
Mauro-Herrera, M., Wang, X., Barbier, H., Brutnell, T. P., Devos, K. M., and Doust, A. N. (2013). Genetic control and comparative genomic analysis of flowering time in Setaria (Poaceae). G3 3, 283–295. doi: 10.1534/g3.112.005207
Meng, Q., Guan, Z., Feng, B., Chai, Y., and Hu, Y. (2009). Principal component analysis and fuzzy clustering on drought-tolerance related traits of foxtail millet (Setaria italica). Sci. Agric. Sin. 42, 2667–2675. doi: 10.3864/j.issn.0578-1752.2009.08.005
Muthamilarasan, M., Dhaka, A., Yadav, R., and Prasad, M. (2015). Exploration of millet models for developing nutrient rich graminaceous crops. Plant Sci. 242, 89–97. doi: 10.1016/j.plantsci.2015.08.023
Muthamilarasan, M., and Prasad, M. (2015). Advances in Setaria genomics for genetic improvement of cereals and bioenergy grasses. Theor. Appl. Genet. 128, 1–14. doi: 10.1007/s00122-014-2399-3
Qian, J., Jia, G., Zhi, H., Li, W., Wang, Y., Li, H., et al. (2012). Sensitivity to gibberellin of dwarf foxtail millet varieties. Crop Sci. 52, 1068–1075. doi: 10.2135/cropsci2011.04.0192
Qie, L., Jia, G., Zhang, W., Schnable, J., Shang, Z., Li, W., et al. (2014). Mapping of quantitative trait locus (QTLs) that contribute to germination and early seedling drought tolerance in the interspecific cross Setaria italica x Setaria viridis. PLoS ONE 9:e101868. doi: 10.1371/journal.pone.0101868
Saha, P., and Blumwald, B. (2016). Spike-dip transformation of Setaria viridis. Plant J. 86, 89–101. doi: 10.1111/tpj.13148
Scholthof, K.-B. G. (1999). A synergism induced by satellite panicum mosaic virus. Mol. Plant. Microbe Interact. 12, 163–166. doi: 10.1094/MPMI.1999.12.2.163
Suma, P. F., and Urooj, A. (2012). Antioxidant activity of extracts from foxtail millet (Setaria italica). J. Food Sci. Technol. 49, 500–504. doi: 10.1007/s13197-011-0300-9
Tadele, Z. (2016). “Drought adaptation in millets,” in Abiotic and Biotic Stress in Plants - Recent Advances and Future Perspectives, eds A. K. Shanker and C. Shanker (Rijeka: InTech), 639–662. Available online at: http://www.intechopen.com/books/abiotic-and-biotic-stress-in-plants-recent-advances-and-future-perspectives
Taylor, J. R. N., Schober, T. J., and Bean, S. R. (2006). Novel food and non-food uses for sorghum and millets. J. Cereal Sci. 44, 252–271. doi: 10.1016/j.jcs.2006.06.009
von Caemmerer, S., Quick, W. P., and Furbank, R. T. (2012). The development of C4 rice: current progress and future challenges. Science 336, 1671–1672. doi: 10.1126/science.1220177
Wang, J., Wang, Z., Yang, H., Yuan, F., Guo, E., Tian, G., et al. (2013). Genetic analysis and preliminary mapping of a highly male-sterile gene in foxtail millet (Setaria italica L. Beauv.) using SSR markers. J. Integr. Agric. 12, 2143–2148. doi: 10.1016/S2095-3119(13)60392-5
Wang, M.-Z., Pan, Y.-L., Li, C., Liu, C., Zhao, Q., Ao, G.-M., et al. (2011). Culturing of immature inflorescences and Agrobacterium-mediated transformation of foxtail millet (Setaria italica). African J. Biotechnol. 10, 16466–16479. doi: 10.5897/AJB10.2330
Xianmin, D., James, S., Bennetzen, J. L., and Jiayang, L. (2014). Initiation of Setaria as a model plant. Front. Agric. Sci. Eng. 1:16. doi: 10.15302/J-FASE-2014011
Xu, W., Wei, L., Qu, W., Liang, Z., Wang, J., Peng, X., et al. (2011). A novel antifungal peptide from foxtail millet seeds. J. Sci. Food Agric. 91, 1630–1637. doi: 10.1002/jsfa.4359
Xue, C. X., Zhi, H., Fang, X., Liu, X., Tang, S., Chai, Y., et al. (2016). Characterization and fine mapping of SiDWARF2 (D2) in foxtail millet. Crop Sci. 56, 95–103. doi: 10.2135/cropsci2015.05.0331
Zhang, G., Liu, X., Quan, Z., Cheng, S., Xu, X., Pan, S., et al. (2012). Genome sequence of foxtail millet (Setaria italica) provides insights into grass evolution and biofuel potential. Nat. Biotechnol. 30, 549–554. doi: 10.1038/nbt.2195
Keywords: Setaria, foxtail millet, green foxtail, C4 grasses, nutrition, genomics, biotic and abiotic stress, model systems
Citation: Pant SR, Irigoyen S, Doust AN, Scholthof K-BG and Mandadi KK (2016) Setaria: A Food Crop and Translational Research Model for C4 Grasses. Front. Plant Sci. 7:1885. doi: 10.3389/fpls.2016.01885
Received: 15 September 2016; Accepted: 29 November 2016;
Published: 15 December 2016.
Edited by:
Manoj Prasad, National Institute of Plant Genome Research, IndiaReviewed by:
Swati Puranik, Aberystwyth University, UKStefania Grando, International Crops Research Institute for the Semi-Arid Tropics, India
Copyright © 2016 Pant, Irigoyen, Doust, Scholthof and Mandadi. This is an open-access article distributed under the terms of the Creative Commons Attribution License (CC BY). The use, distribution or reproduction in other forums is permitted, provided the original author(s) or licensor are credited and that the original publication in this journal is cited, in accordance with accepted academic practice. No use, distribution or reproduction is permitted which does not comply with these terms.
*Correspondence: Kranthi K. Mandadi, a2ttYW5kYWRpQHRhbXUuZWR1