- 1College of Horticulture, China Agricultural University, Beijing, China
- 2Institute of Germplasm Resources, Xinjiang Academy of Agricultural Science, Urumqi, China
- 3Graduate School of Horticulture, Chiba University, Matsudo, Japan
Light insufficient stress caused by canopy interception and mutual shading is a major factor limiting plant growth and development in intensive crop cultivation. Supplemental lighting can be used to give light to the lower canopy leaves and is considered to be an effective method to cope with low irradiation stress. Leaf photosynthesis, stomatal regulation, and plant growth and development of young tomato plants were examined to estimate the effects of supplemental lighting with various composite spectra and different light orientations. Light-emitting diodes (LEDs) of polychromatic light quality, red + blue (R/B), white + red + blue (W/R/B), white + red + far-red (W/R/FR), and white + blue (W/B) were assembled from the underneath canopy or from the inner canopy as supplemental lighting resources. The results showed that the use of supplemental lighting significantly increased the photosynthetic efficiency, and reduced stomatal closure while promoting plant growth. Among all supplemental lighting treatments, the W/R/B and W/B from the underneath canopy had best performance. The different photosynthetic performances among the supplemental lighting treatments are resulted from variations in CO2 utilization. The enhanced blue light fraction in the W/R/B and W/B could better stimulate stomatal opening and promote photosynthetic electron transport activity, thus better improving photosynthetic rate. Compared with the inner canopy treatment, the supplemental lighting from the underneath canopy could better enhance the carbon dioxide assimilation efficiency and excessive energy dissipation, leading to an improved photosynthetic performance. Stomatal morphology was highly correlated to leaf photosynthesis and plant development, and should thus be an important determinant for the photosynthesis and the growth of greenhouse tomatoes.
Introduction
Although controlled by genetic characteristics, the morphology, and development of plants are sensitive to fluctuations in environmental factors. Light is one of the most important variables regulating biological reactions and material accumulation in plants (Kopsell and Kopsell, 2008). Currently, intensive cultivation schedules have been adopted in greenhouse crop production to achieve high yield. However, this can result in insufficient light reaching the lower canopies and altered plant morphogenesis and photosynthesis (Hogewoning et al., 2010a; Terfa et al., 2013). In greenhouse tomato cultivation, the light interception of each canopy layer decreases sharply down the plant profile, and mutual shading also occurs (Acock et al., 1978; Xu et al., 1997; Lu et al., 2012a; Tewolde et al., 2016). No more than 35% of the total intercepted solar light reaches the leaves under the tomato fruit trusses (Cockshull et al., 1992; Lu et al., 2012a), and such a shortage of light triggers an extremely low net photosynthetic rate and premature leaf senescence (Acock et al., 1978; Xu et al., 1997).
Supplemental lighting, using artificial light resources and employed in lower canopies, is considered to be an efficient method for relieving low-light stress on plants. Numerous studies the effects of application of supplemental lighting have been conducted on various species via aspects of the canopy layer (Hovi et al., 2004; Hovi and Tahvonen, 2008; Pettersen et al., 2010), light source (Lu et al., 2012a,b; Piringer and Cathey, 1960), light intensity (Demers et al., 1998; Dorais, 2003), and light period (Piringer and Cathey, 1960; Tewolde et al., 2016). Among those, the selection of optimized light wavelength is more complex and is often reported with mixed results (Okamoto et al., 1996; Li and Kubota, 2009; Ni et al., 2009; Lu et al., 2012b). For example, blue light suppresses hypocotyl elongation in wheat (Goins et al., 1997) and tomato (Massa et al., 2008), but improves the dry matter production and the photosynthetic capacity in pepper (Brown et al., 1995), wheat (Goins et al., 1997), and spinach (Matsuda et al., 2007). In contrast, red light seems to be most effective in the biomass assimilation of lettuce (Yanagi et al., 1996; Kim et al., 2006), but not spinach and radish (Okamoto et al., 1996; Yorio et al., 2001). Similarly, different red/far-red ratios show contrary results in phytochemical content (Alokam et al., 2002) and plant photomorphogenesis (Kirdmanee et al., 1993; Brown et al., 1995; Runkle and Heins, 2001). These results have shown the viability of optimizing the light quality in promoting plant morphology and productivity to eventually improve the greenhouse economic benefits. However, previous studies have mainly examined only a few selected sole light qualities or the compound spectrum with only the combination of red/blue or red/far-red at one time, and there are no reports examining the effects of polychromatic light quality (the combination of white, blue, red, and far-red) affecting plant growth and development. Therefore, it is necessary to investigate the polychromatic light quality effects when provided as supplemental lighting resource applied for horticultural crop production.
Photosynthesis is sensitive to light condition and essential for plant growth. Improved leaf photosynthesis would enhance plant development (Hovi et al., 2004; Hovi and Tahvonen, 2008; Pettersen et al., 2010). The investigation of leaf structure–function relationships in photosynthesis shows that internal maximum photosynthesis rates were not close to the leaf surface of the upper epidermis, where light intensity was highest, but instead occurred in the middle and lower palisade layers (Nishio et al., 1993; Evans, 1995; Sun et al., 1998; Sun and Nishio, 2001; Evans and Vogelmann, 2003). These deeper layers have higher electron transport activities and greater amounts of photosynthetic proteins (Terashima and Inoue, 1985; Terashima and Evans, 1988; Sun and Nishio, 2001). This indicated that the supplemental lighting from the underneath canopy (with light orientation to the abaxial epidermis) might function better in improving leaf and plant development than using the supplemental lighting from the inner canopy (with light orientation to the adaxial epidermis).
Stomatal regulation, which is highly correlated with leaf photosynthesis, governs the overall CO2 assimilation, and water loss from plants (Casson and Hetherington, 2010; Araújo et al., 2011). Stomatal behavior can be affected by the light wavelength through energy conversion (Shimazaki et al., 2007; Chen et al., 2012), membrane ion transport (Fan et al., 2004; Araújo et al., 2011), and metabolic activity in guard cells (Talbott and Zeiger, 1993; Mott et al., 2008; O'Carrigan et al., 2014). Tomato (Lycopersicon esculentum Mill.) is regarded as one of the most important horticultural crops in the world and previous researches have demonstrated the distinct influence of supplemental lighting quality on plant development (Demers et al., 1998; Massa et al., 2008), which consequently affects fruit developing speed (Lu et al., 2012b), yield (Lu et al., 2012b; Gómez et al., 2013), and quality (Dorais, 2003; Lu et al., 2012b; Olle and Viršile, 2013), and eventually determined the economic applicability of the application of this technique. In addition, the stomata are mostly distributed on the abaxial epidermis of tomato leaves. We thus hypothesized that supplemental lighting from the underneath canopy have more obvious improvement on the stomatal regulation and photosynthesis capacity, which might consequently better stimulate plant growth and development, compared with supplemental lighting from the inner canopy. White light has a higher penetration rate through the tomato canopy than other colors (Lu et al., 2012b), blue light could contribute a larger stomata size (Sharkey and Raschke, 1981; Loreto et al., 2009) in leaves and a higher health index of tomato plants (Chang et al., 2010; Chen et al., 2014), and red/blue and red/far-red light are the most commonly used in horticulture cultivation. Thus, we added white light to the spectrum in this study to investigate the effects of polychromatic supplemental lighting. Plants undergo the most vegetative growth before anthesis and the fluctuation of environment factors would cause largely morphological changes at this stage (Poorter and Garnier, 1996; Matsuda et al., 2014). Therefore, in this study, to understand how plants respond to the interaction of light quality and light orientation, we treated young tomato plants with/without supplemental lighting with different polychromatic light quality levels orientated from underneath or inner canopy for 4 weeks, and investigated the resulting fluctuations in leaf photosynthesis and stomatal behavior, as well as the consequent response of plant morphologic development.
Materials and Methods
Plant Material and Growth Conditions
The experiment was conducted in a glass greenhouse (Venlo-type, with double spans and a north–south orientation) in Urumqi, China (43°46′12″N, 87°40′48″E) from September, 2015 to April, 2016. Tomato (“NS3389,” Agricultural Science and Technology Co. Ltd., Guangzhou, China) seeds were sown into trays with commercial substrate (Peilei No. 2, Peile Organic Fertilizer Co., Zhenjiang, China) and germinated in an environmentally controlled box (RTOP-1000D, Top Yun Co. Ltd., Hanzhou, China) for 24 days. Other environment factors were fixed, including the photosynthetic photon flux density (PPFD), photocycle, day/night temperatures, and CO2 concentration of 350 μmol·m−2·s−1, 16 h, 23/18°C, and 800 μmol·mol−1, according to Matsuda et al. (2011, 2014). The trays were sub-irrigated every other day, with a commercial nutrient solution at an electrical conductivity of 1.5 dS·m−1.
At 24 days after sowing, each seedling was transplanted into the greenhouse at a plant density of 16.6 plant·m−2 with an automatically irrigated nutrient solution (Nakano et al., 2010). The greenhouse environment was maintained, with daytime mean air temperature of 27 ± 2°C, a night-time mean air temperature of 20 ± 2°C, and a daily mean relative humidity above 60%. Although the CO2 concentration was not measured, it was assumed to be close to the outside level, based on measurements in the same season in another year (data not shown).
Supplemental Lighting Treatment
Light-emitting diodes (LEDs; Philips Co. Ltd., Netherlands) were used as the light source. Four polychromatic light, red + blue (R/B, R:B = 3:1), white + red + blue (W/R/B, W: R:B = 3:2:1), white + red + far-red (W/R/FR, W:R:FR = 3:2:1), and white + blue (W/B, W:B = 2:1), were applied from two light orientations: supplemental lighting from the underneath and inner canopy (Figure 1). LEDs were fixed to movable girders that ensured the lighting distance from the adaxial epidermis of inner canopy leaves or the abaxial epidermis of the lowest leaf truss was maintained at 10 cm. The measured PPFD was 200 μmol·m−2·s−1 at 10 cm from the LED module. Plants without supplemental lighting were considered to be the control plants. Each treatment consisted of three bench rows of plants, with each row containing 20 plants, with a 16 h photo cycle each day (during 8:00–24:00 at GMT +8, which is 6:00–22:00 local time).
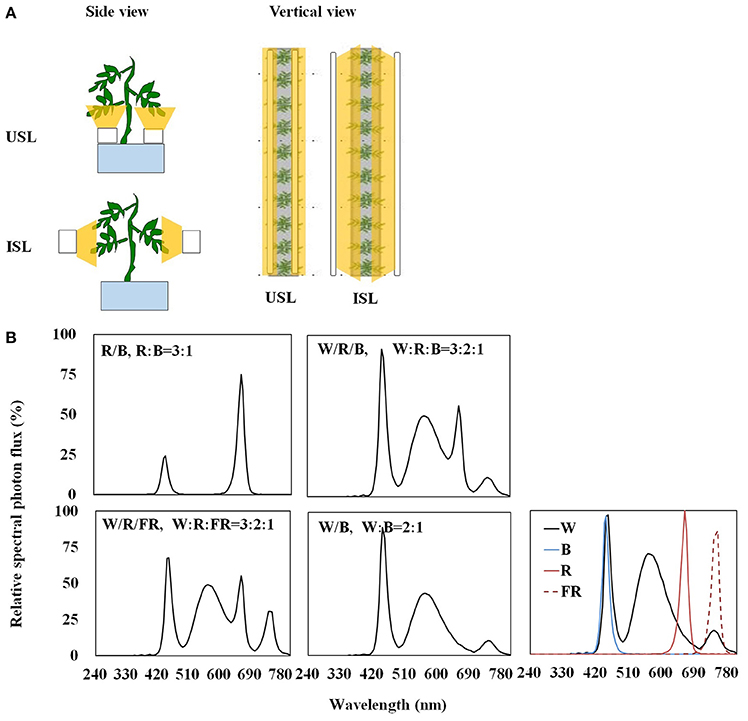
Figure 1. Schematic diagram (A) and relative spectral photon flux of polychromatic LEDs (B) of the supplemental lighting treatment in this experiment. Supplemental lighting from the underneath canopy (USL) or from the inner canopy (ISL) was applied to plants from the time of transplantation while a no supplemental lighting condition was considered to be the control. Each supplemental lighting module was kept fixed at a 10 cm distance to the abaxial or adaxial epidermis of the leaf, respectively, with a PPFD of 200 μmol·m−2 ·s−1. The light quality contains red + blue (R/B, R:B = 3:1), white + red + blue (W/R/B, W: R:B = 3:2:1), white + red + far-red (W/R/FR, W:R:FR = 3:2:1) and white + blue (W/B, W:B = 2:1). The spectral property of each LED module used for polychromatic LEDs combination also shown in (B). The wavelengths of the light sources were recorded at 240–800 nm with a spectrometer (SR9910-v7, Irradiant Ltd., Tranent, UK). A digital timer, dimmer, and transformer were used to maintain the light period (16 h, GMT +8 8:00–24:00) and light intensity.
Gas-Exchange Parameter Measurements
Gas-exchange measurements were conducted on the second terminal leaflets of leaves on the fifth youngest node (Matsuda et al., 2014) with a portable photosynthesis system (Li-6400XT; Li-Cor Inc., Lincoln, NE, USA) during 11:00–16:00, GMT +8 (9:00–14:00, local time) on the 28th day after transplanting. The net photosynthetic rate (PN), stomatal conductance (Gs), transpiration rate (Tr), and intercellular CO2 concentration (Ci) were measured. Measurements were conducted with PPFD, leaf temperature, CO2 concentration, and relative humidity at 800 ± 5 μmol·m−2·s−1, 28 ± 1°C, 400 ± 2 μmol·m−2, and 63 ± 2%, respectively.
The light and CO2 response curve measurement was conducted to calculate the light-saturated maximum photosynthetic rate (PNmax), apparent quantum yield (AQY), CO2-saturated maximum photosynthetic rate (Amax), and carboxylation efficiency (CE). The leaf temperature was set at 25°C, and the PPFD and CO2 concentrations ranged from 1600 to 0 μmol·m−2·s−1and 1200 to 0 μmol·mol−1, respectively. The PN–PPFD and PN–Ci curves were plotted using a non-linear curving-fitting routine with the PN data and the corresponding light intensity or intercellular CO2 concentration, respectively. Indexes were identified as PNmax and Amax, the maximum net photosynthetic rate at the saturation light intensity and CO2 concentration, respectively (Bassman and Zwier, 1991). AQY were the initial slope of the PN−PPFD (Lambers et al., 2008; Skillman, 2008), and CE also known as Vcmax, the maximum velocity of ribulose 1,5-bisphosphate carboxylase/oxygenase (Rubisco) for carboxylation, which can be calculated from PN−Ci curve curves according to equation from FvCB model (Farquhar et al., 1980; Sharkey et al., 2007):
where Vcmax is the maximum velocity of Rubisco for carboxylation, Cc is the CO2 partial pressure at Rubisco, Kc is the Michaelis constant of Rubisco for carbon dioxide, O is the partial pressure of oxygen at Rubisco, and Ko is the inhibition constant (usually taken to be the Michaelis constant) of Rubisco for oxygen. The symbol Γ* is the photorespiratory compensation point and Rd is day respiration. This equation lends itself to a linear regression approach to estimating Vcmax as the slope and −Rd as the intercept.
Chlorophyll Fluorescence Parameter Measurements
Chlorophyll fluorescence parameters were measured to evaluate the light absorption, electron transfer, thermal dissipation, and excitation distribution in the photosystem of tomato plants treated with or without supplemental lighting from the underneath or inner canopy with polychromatic LEDs after the adaption of the leaves to stable light or dark states. Leaf chlorophyll fluorescences levels were measured simultaneously using a portable photosynthesis system (Li-6400XT, Li-Cor Inc.) with an integrated fluorescence fluorometer (Li 6400-40 leaf chamber fluorometer, Li-Cor Inc.). The gas supply (ambient CO2 concentrations and 21% O2), actinic light (LED with 90% red light, 630 nm; 10% blue light, 470 nm) and measurement light (630 nm, 1 μmol·m−2·s−1) were all setting in accordance with Sun et al. (2016). The steady state chlorophyll fluorescence level (Fs), minimum chlorophyll fluorescence at the open PSII center (Fo, dark treated; F′o, light adapted), maximum chlorophyll fluorescence at the closed PSII center (Fm, dark treated; F′m, light adapted) were all determined in accordance with the work of Kramer et al. (2004). The maximum quantum yield of the PSII primary photochemistry [Fv/Fm; (Fm−Fo)/Fm], efficiency of excitation energy capture by open PSII reaction centers [F′v/F′m = (F′m − F′o)/F′m], quantum yield of the PSII electron transport [ΦPSII; (F′m − Fs)/ = F′m], and non-photochemical quenching [NPQ = (Fm − F′m)/ F′m] were calculated from the measured parameters (Maxwell and Johnson, 2000).
Stomatal Assays
Stomatal assays were carried out essentially as described in work of O'Carrigan et al. (2014) and conducted on abaxial epidermal strips of the leaves at the same position of photosynthesis measurement on the 29th day after transplanting during 11:00–16:00, GMT +8 (9:00–14:00, local time). The samples were peeled, immersed in a transparent nail polish buffer, and mounted on glass slides before micro-imaging. Images of each epidermal strip were taken under a Leica microscope (Leica Microsystems AG, Solms, Germany) fitted with a Nikon NIS-F1 CCD camera and a Nikon DS-U3 controller (Nikon, Tokyo, Japan), and analyzed with a Nikon NIS Element software. Stomatal density was defined as the number of stomata per mm2 and stomatal index was calculated as ([number of stomata]/[number of epidermal cells + number of stomata]) × 100 (Kubínová, 1994). The stomatal aperture width and length was defined in Figure 5, and stomatal pore area was calculated by assuming an oval pore shape according to Chen et al. (2010, 2012).
Plant Growth Analyses
On the 30th day after transplantation, plants were destructively harvested for the determination of the dry weights of the shoots and roots, the height and diameter of stems, health index, leaf areas, specific leaf area (leaf area per unit leaf mass), leaf chlorophyll contents, flower number, and carbohydrate determination. Plants were washed with distilled water and weighed after wiping the water off. The leaf area per plant was measured using a leaf area meter (LI-3000C; Li-Cor Inc.). The leaf chlorophyll content was determined using a chlorophyll meter (SPAD-502; Minolta, Osaka, Japan). Samples were oven dried at 80°C until a constant weight was attained, and the dry weight subsequently recorded. The health index, widely used as general evaluation of the young plant growth quality (Fujii, 1952, 1953; Lu et al., 1984; Ge, 1987; Zhang et al., 1992; Song, 1999; Yang and Zhou, 2010; Huang et al., 2012; Chen et al., 2014), was calculated as (stem diameter/stem height) × total dry weight, according to Fan et al. (2013). The carbohydrates, including the soluble-sugar and starch, were measured in samples of the milled leaf material. Soluble sugars were extracted with 80% (v/v) ethanol at 80°C and their contents were determined enzymatically, and starch in the 80% ethanol-insoluble fraction was extracted and digested, and the resultant glucose content was assayed by Nelson–Somogyi's method (Matsuda et al., 2014).
Statistical Analyses
Duncan's multiple range test was performed at P < 0.05 for among all treatments and two-way analysis of variance (ANOVA) was performed at P < 0.05 with supplemental light quality and light orientation as sources of variation. SPSS 11.0 software (SPSS Inc., Chicago, IL, USA) was used for the statistical analyses.
Results
Gas-Exchange Parameter
Compared with the control, regardless of the light quality, the supplemental lighting from the inner canopy significantly increased the PN, Gs, Ci, and Tr (Figures 2A–D). In contrast, while the supplemental lighting from the underneath canopy significantly increased the PN, Gs, and Tr, it had no significant effects on Ci. Among the supplemental lighting treatments, as regard of light quality, data in the W/R/B and W/B was significantly higher than those in the R/B and W/R/FR. The leaf photosynthesis capacity was significantly promoted by supplemental lighting. Compared with the control, supplemental lighting significantly increased the PNmax, Amax, AQY, and CE (Figure 3). Among the supplemental lighting treatments, the data in the supplemental lighting from the underneath canopy were generally higher than those in the supplemental lighting from the inner canopy and data in the W/R/B and W/B were significantly higher than those in the R/B and W/R/FR. The PNmax, Amax, and AQY were highest in the W/R/B from treatments of supplemental lighting from the underneath canopy and increased by 86.5, 70.0, 53.6%, respectively, compared with the control (Figures 3C–E). The CE was highest in the W/B from treatment of supplemental lighting from the underneath canopy and increased 57.5% compared with the control (Figure 3F).
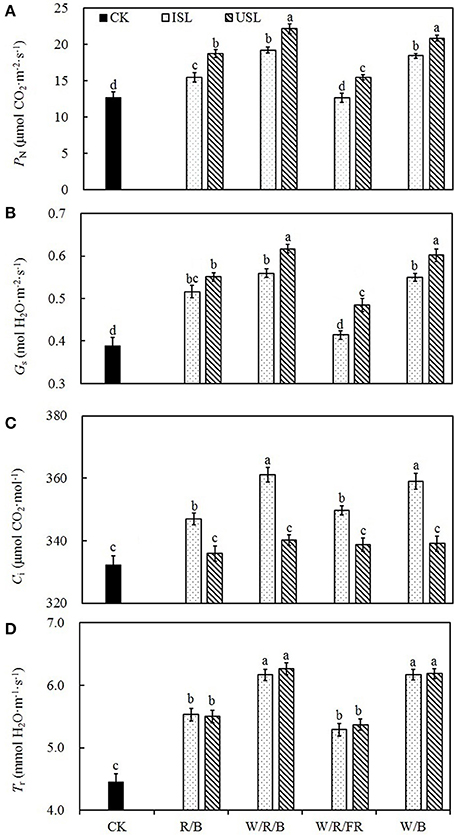
Figure 2. The effects of different treatments on the net photosynthetic rate (PN; A), stomatal conductance (Gs; B), intercellular CO2 concentration (Ci; C), and transpiration rate (Tr; D) in the leaves of tomato plants. Supplemental lighting from the underneath canopy (USL) or from the inner canopy (ISL) was applied to plants from the time of transplantation while a no supplemental lighting condition was considered to be the control (CK). Parameters were measured on the second terminal leaflets of leaves on the fifth youngest node for each treatment. Measurements were conducted with PPFD, leaf temperature, CO2 concentration, and relative humidity at 800 ± 5 μmol·m−2·s−1, 28 ± 1°C, 400 ± 2 μmol·m−2, and 63 ± 2%, respectively. Means ± SE (n = 8) different letters indicate significant differences at P < 0.05 according to Duncan's multiple range test.
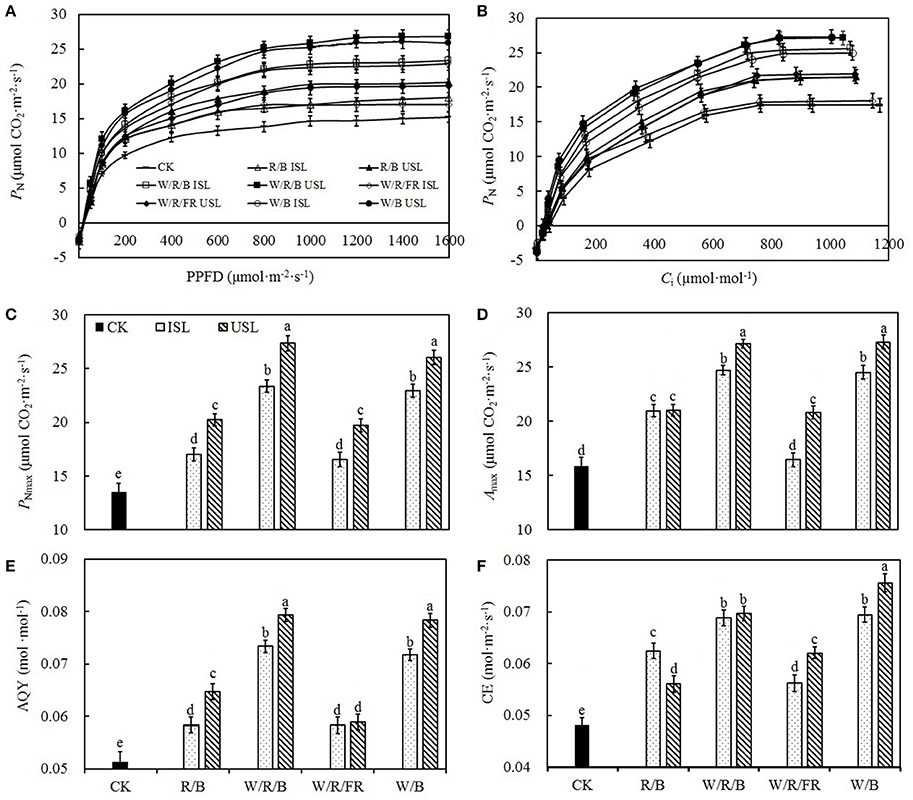
Figure 3. The effects of different treatments on the light response curve (A), CO2 response curve (B), light-saturated maximum photosynthetic rate (PNmax; C), CO2-saturated maximum photosynthetic rate (Amax; D), apparent quantum yield (AQY; E), and carboxylic efficiency (CE; F) in the leaves of tomato plants. Supplemental lighting from the underneath canopy (USL) or from the inner canopy (ISL) was applied to plants from the time of transplantation while a no supplemental lighting condition was considered to be the control (CK). Parameters were measured on the second terminal leaflets of leaves from the fifth youngest node for each treatment. Means ± SE (n = 8) different letters indicate significant differences at P < 0.05 according to Duncan's multiple range test.
Chlorophyll Fluorescence Parameter
Compared with the control, the supplemental lighting from the underneath canopy significantly increased the efficiency of the excitation energy captured by the open PSII reaction centers (F′v/F′m; Figure 4B), the quantum yield of the PSII electron transport (ΦPSII; Figure 4C), and the non-photochemical quenching (NPQ; Figure 4D), but had no effect on the maximum quantum yield of the PSII primary photochemistry (Fv/Fm; Figure 4A). Under treatment of supplemental lighting from the inner canopy, the W/R/B and W/B significantly increased ΦPSII and NPQ but had no effect on Fv/Fm, F′v/F′m, while R/B and W/R/FR had no effect on any of the indexes.
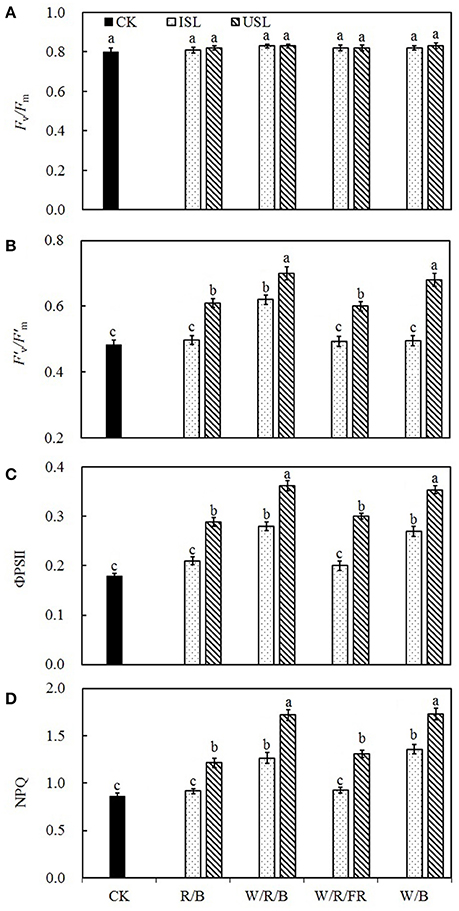
Figure 4. The effects of different treatments on maximum quantum yield of the PSII primary photochemistry (Fv/Fm; A), the efficiency of excitation energy capture by PSII (F′v/F′m; B), the quantum yield of PSII electron transport (ΦPSII; C), and non-photochemical quenching (NPQ; D) in leaves of tomato plants. Supplemental lighting from the underneath canopy (USL) or from the inner canopy (ISL) was applied to plants from the time of transplantation while a no supplemental lighting condition was considered to be the control (CK). Parameters were measured on the second terminal leaflets of leaves on the fifth youngest node for each treatment. Means ± SE (n = 8) different letters indicate significant differences at P < 0.05 according to Duncan's multiple range test.
Stomatal Characteristics
Compared with the control, the supplemental lighting significantly increased stomatal density but not influenced stomatal index (Figure 6). The data on the stomatal density in the treatments of supplemental lighting from the underneath canopy were significantly higher than those in the treatments of supplemental lighting from the inner canopy, while no significant difference among the light quality was observed. The stomatal aperture size was significantly affected by the supplemental lighting (Figures 5, 7). The aperture length was significantly decreased, while the aperture width was significantly increased when the leaf was exposed to supplemental lighting (Figures 7A,B), resulting in a significantly higher width/length ratio and a larger stomatal pore area (Figures 7C,D). The data in the W/R/B and W/B were generally higher than those in the R/B and W/R/FR, and the data in the treatments of supplemental lighting from the underneath canopy were generally higher than those in the treatments of supplemental lighting from the inner canopy.
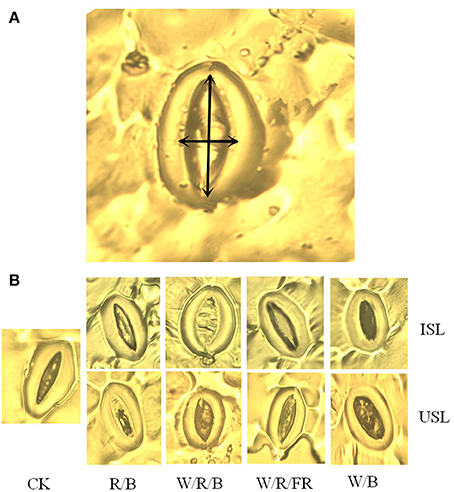
Figure 5. Representative stomatal images for the measurements of stomatal parameters. Supplemental lighting from the underneath canopy (USL) or from the inner canopy (ISL) was applied to plants from the time of transplantation while a no supplemental lighting condition was considered to be the control (CK). (A) A light micrograph of stomata aperture width (horizontal arrow) and aperture length (vertical arrow) are indicated by double arrows. (B) Typical stomata aperture closure responses to different treatments in this experiment are shown.
Plant Growth and Carbohydrate Accumulation
Supplemental lighting had positive effects on the plant growth and carbohydrate accumulation (Table 1). With the exception of the stem height, the data in supplemental lighting were obviously higher than the data in the control. Generally, the data in the W/R/B and W/B were higher than those in other light quality and the data in the treatments of supplemental lighting from the underneath canopy were higher than the inner canopy treatments. With the excepted of the leaf area, the light quality, light orientation, or quality × orientation had a significant influence on the measured index.
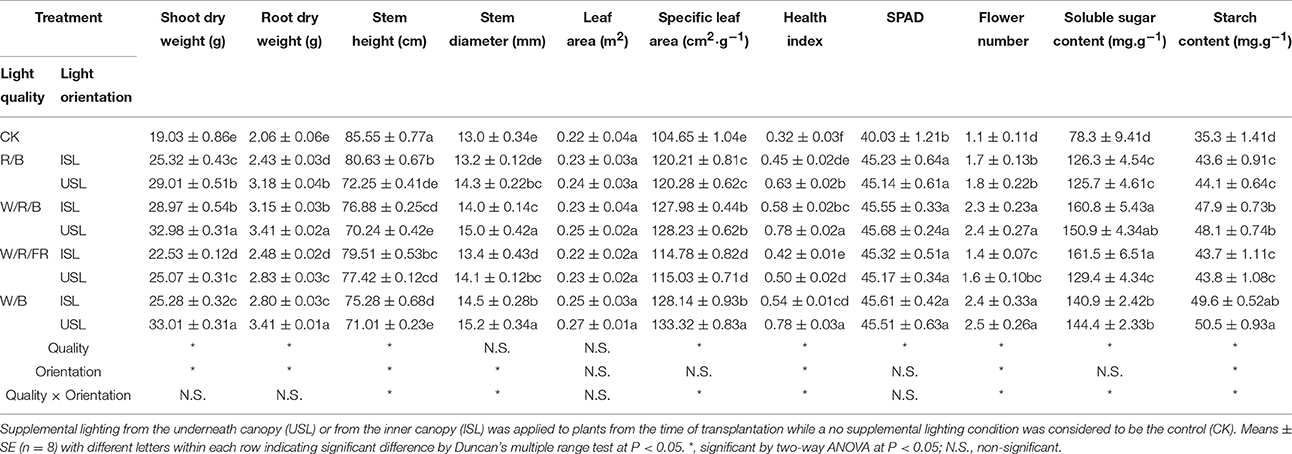
Table 1. Effects of polychromatic supplemental lighting quality and light orientation on tomato plant morphological characteristics and carbohydrate accumulation.
Correlation Analysis of Growth, Photosynthesis and Stomatal Parameters
The aperture width/length, stomatal pore area and stomatal index, Fv/Fm, ΦPSII, starch content, stem dry weight, specific leaf area, flower number, and health index were highly significantly correlated to photosynthetic performance and growth development of tomato plants (P < 0.05; Table 2). Furthermore, two-way ANOVA analysis showed that there were highly significant effects of light quality, light orientation, and interaction factors between the quality × orientation on the growth, stomatal and gas exchange parameters (Tables 1, 3). However, there were no significant quality effects on the stem diameter, leaf area (Table 1), F′v/, stomatal density, and aperture length (Table 3). The orientation had no effects on the leaf area, specific leaf area, chlorophyll content, soluble sugar content (Table 1), Fv/Fm, stomatal index, aperture length, aperture width/aperture length (Table 3). There was also no significant quality × orientation effects on the shoot dry weight, root dry weight, leaf area (Table 1), Fv/Fm, stomatal index, and aperture length (Table 3).
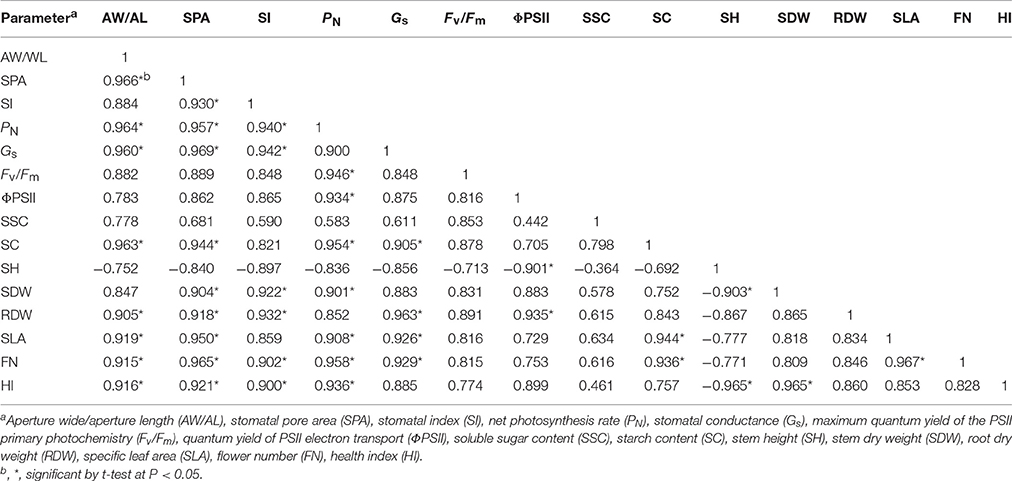
Table 2. Correlation analysis of selected stomatal parameters, photosynthetic characteristics and plant development of tomato plants under different treatments.
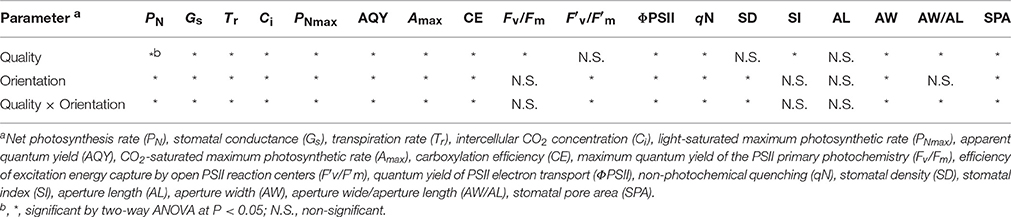
Table 3. Two-way ANOVA analysis of the effects of supplemental light quality, light orientation, and quality × orientation interaction on photosynthetic characteristics and stomatal parameters of tomato plants under different treatment conditions.
Discussion
Plant morphogenesis and development are controlled by genetics as well as environmental factors such as light quality, intensity, and orientation (Pearcy, 1988, 1990; Aldesuquy et al., 2000; Naumburg and Ellsworth, 2002; Hogewoning et al., 2010a,b; Wahidin et al., 2013). Low-light conditions in intensive greenhouse crop cultivation will reduce the plant growth and restrict the productive capacity (Demers et al., 1998; Frantz et al., 2000; Demers and Gosselin, 2002; Steinger et al., 2003; Shimazaki et al., 2007). Our results demonstrated that supplemental lighting treatment significantly improved the photosynthetic performance and stomatal regulations, thus improving the biomass production of tomato plants.
Plant photosynthesis is extremely sensitive to supra-optimal light conditions. Low light stress can damage the photosynthetic apparatus (Naumburg and Ellsworth, 2002), degrade the photosynthetic pigments (Aldesuquy et al., 2000), and suppress the carbon assimilation (Nawrocki et al., 2015). In our study, supplemental lighting treatment was found to improve the photosynthesis ability of leaves (Figures 2–4), which was in accordance with the previous work done to other species (Hovi et al., 2004; Hovi and Tahvonen, 2008; Massa et al., 2008). Chlorophyll captures light and soaks up the energy from it. The chlorophyll content closely related to the photosynthesis ability of leaf (Tewolde et al., 2016), and lack of the light-harvesting complex will affect chloroplasts structure and decrease chlorophyll content (Kovács et al., 2006). Powles (1984) had found that when plant suffered from photoinhibition induced by visible light, the photosynthetic apparatus was injured and chlorophyll content decreased dramatically, while Sokawa and Hase (1967) declared that in low light condition, enhanced light illumination would trigger chlorophyll formation and accompanied with increased light harvesting and modified photoreceptor. In this study, the chlorophyll content was enhanced in plants treated with supplemental lighting (SPAD, Table 1), which indicated the improvement of photosynthetic apparatus integrity and light harvesting efficiency. However, there was no significant difference in the chlorophyll content among the supplemental lighting treatments, indicating that the variation in the increased PN among the supplemental lighting treatments was probably due to variations in the CO2 supply (the quantity that entered leaf through stomatal aperture, not the ambient CO2 concentration) and/or assimilation efficiency. We observed significantly higher PN and Gs (Figures 2A,B) in the W/R/B and W/B treatment conditions, indicating that plants treated with these types of polychromatic supplemental lighting had performed better CO2 utilization efficiency. Considering the relative spectral distribution, there are larger proportions of blue light in W/R/B and W/B. Tough pure blue light has negative effects on photosynthesis, especially on tree species (McCree, 1972; Sarala et al., 2009; Pallozzi et al., 2013), adding blue light to the other spectrum could stimulate photosynthesis in wheat (Goins et al., 1997) and tomato (Arena et al., 2016). Sharkey and Raschke (1981) found that blue light could induce stomatal opening, thus increasing the stomatal pore area (Figure 7D) allowing for a higher availability of CO2 in the mesophyll. The data of PNmax, Amax, AQY, and CE (Figures 3C,F) are also significantly higher in W/R/B and W/B. PNmax and Amax are related to the activities of photosynthetic electron transport and phosphorylation. AQY represents CO2 assimilation or oxygen release when one photon is absorbed by the plant, and CE represents the carboxylation efficiency (Farquhar et al., 1980; Reng et al., 2003). These improved photosynthetic parameters confirmed the hypothesis that the enhanced blue light fraction in polychromatic illumination could promote photosynthetic electron transport activity and enhance the CO2 assimilation efficiency. This result was in consistent with the findings of Hogewoning et al. (2010b), who determined that the photosynthetic capacity of cucumber leaves increased as the blue light fraction increased. Chlorophyll fluorescence parameter variations provided the further explanation of optimized photosynthetic regulation under SL treatment. We observed significantly higher ΦPSII (Figure 4C) and NPQ (Figure 4D) under the W/R/B and W/B treatments. ΦPSII represents the electron supply for photosynthesis, highly correlated to PN (Table 2), while NPQ suggests excessive energy dissipation ability, a most common form of photo protector against stress (Maxwell and Johnson, 2000). Thus, W/R/B and W/B improved the actual quantum yield of PSII electron transport and relieved light insufficiency stress in tomato leaves.
On the other side, compared with the control, plants under the treatments of supplemental lighting from the inner canopy generally presented with increased PN, Gs, Ci, and Tr (Figures 2A–D), which indicated that, in addition to the enhancement of chlorophyll content (Table 1), the increase in PN was mostly caused by improved stomatal conductance, which provide sufficient CO2 for photosynthesis (Farquhar and Sharkey, 1982). This result was in accordance with the research on cucumber (Hao and Papadopoulos, 1999), which demonstrates that after treatment of supplemental lighting, the PN was increased with Gs and expanded stomatal aperture. However, in the treatments of supplemental lighting from the underneath canopy, accompanied with an increase in the PN, Gs, and Tr of tomato plants, Ci was unaffected compared with the control. Combining the increased in PNmax, Amax, AQY, and CE (Figures 3C–F), these results suggest that in addition to the influence from chlorophyll content, the increase in the PN by supplemental lighting from the underneath canopy was mostly related to the highly improved CO2 assimilation efficiency, rather than to the simply enhanced CO2 supply. Studies on the effects of abaxial lighting treatment on plant photosynthesis in Paspalum dilatatum (Soares et al., 2008) and Helianthus annuus (Wang et al., 2008) also showed photosynthesis improvements closely related to CE. Given that Tr is similar to that in treatments of supplemental lighting from the inner canopy, the CO2 assimilation efficiency should be the determining factor of PN variation between the treatments of supplemental lighting from the underneath and inner canopy conditions. Fv/Fm (Figure 4A) was not statistically changed, reconfirming that the variation of increased PN among supplemental lighting treatments was due to variation in CO2 utilization and independent of light-harvesting. However, the increased F′v/, ΦPSII, and NPQ (Figures 4B–D) suggested that supplemental lighting from the underneath canopy improved the quantum yield of PSII electron transport and the excessive energy dissipation ability of tomato leaves. The increased ΦPSII meant that the majority of the photons absorbed by PSII and used in photochemistry were promoted to increase the level of the photorespiration rate. Therefore, the supplemental lighting from the underneath canopy could promote quantum yields of both PSII electron transport and carboxylation rates of tomato plants, leading to an increase in the photosynthetic efficiency, which is in accordance with the observed photosynthesis improvements by the application of abaxial lighting treatment on sunflower plants (Wang et al., 2008). In this study, the supplemental lighting from the inner canopy did not affect F′v/ (Figure 4B), and only partly increased ΦPSII and NPQ (in W/R/B and W/B, Figures 4C,D). Data of the above parameters were significantly lower than those in the treatments of supplemental lighting from the underneath canopy. This results reconfirmed the lower carboxylation efficiency in plants treated with supplemental lighting from inner canopy, and this induced a relatively lower PN, compared with the other kind of light orientation treatment (Figure 2).
Stomatal morphogenesis and behavior are controlled by genetic as well as environmental factors, such as in light (Meckel et al., 2007; Mott et al., 2008; O'Carrigan et al., 2014). In this study, stomatal density was not affected by the light quality of supplemental lighting, and the stomatal index was not affected by the supplemental lighting orientation and quality × orientation (Figure 6; Table 3). However, the stomatal form and aperture size was significantly affected by the supplemental lighting conditions (Figures 5, 7). The aperture width was significantly increased in supplemental lighting treatment, accompanied by an increased aperture width/length ratio and stomatal pore area (Figure 7), suggesting that supplemental lighting could remit stomatal closure to promote enter-cell CO2 supply other than enhancing the stomatal number, which was in accordance with previous research on the cowpea (Schoch et al., 1980) and other tomato species (Gay and Hurd, 1975; Lee et al., 2007). The stomatal closure, usually induced by environmental stress, prevents CO2 from entering the mesophyll cells (Mott et al., 2008; Araújo et al., 2011) and decreases the internal CO2 concentration (Lake et al., 2001). Additionally, the stomatal morphology and density are correlated with leaf photosynthesis and plant development (Table 2). The aperture width/length and stomatal pore area are highly positively correlated to the PN, Gs, specific leaf area, flower number, and health index, but are negatively linked to the stem height (Table 2). This was in accordance with the work of O'Carrigan et al. (2014), who found that a decrease in the aperture area could reduce the PN of tomato leave and induce excessive plant growth and a decrease in the flower number. These results indicated that the enlargement of the aperture could increase the CO2 supply and that stomatal morphology should be an important determinant of photosynthesis and growth of greenhouse cultivated tomato.
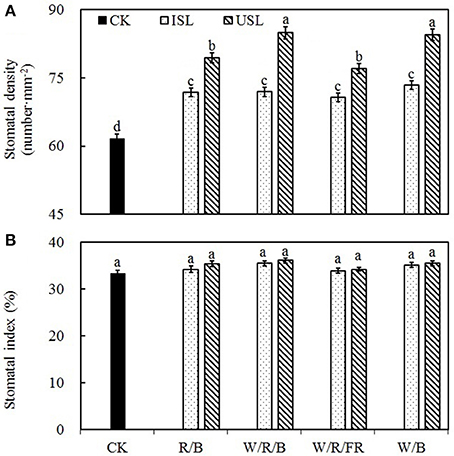
Figure 6. The effects of different treatments on the stomatal density (A) and stomatal index (B) in the leaves of tomato plants. Supplemental lighting from the underneath canopy (USL) or from the inner canopy (ISL) was applied to plants from the time of transplantation while a no supplemental lighting condition was considered to be the control (CK). Parameters were measured on the second terminal leaflets of leaves on the fifth youngest node for each treatment. Means ± SE (n = 16) different letters indicate significant differences at P < 0.05 according to Duncan's multiple range test.
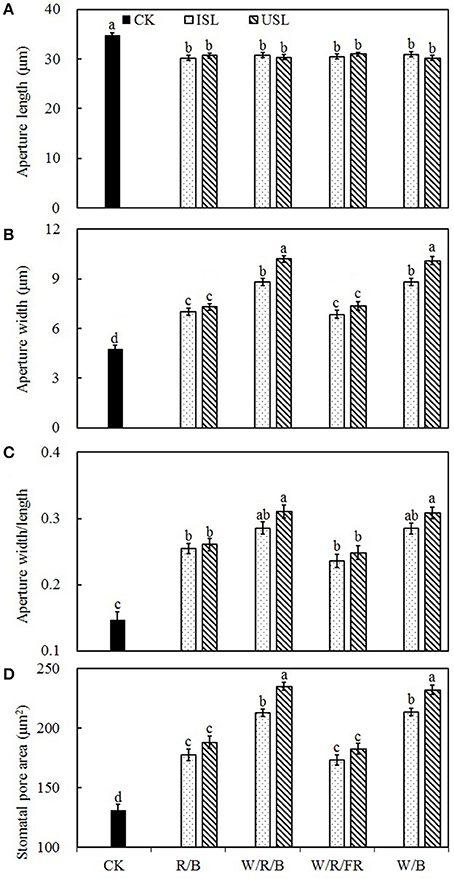
Figure 7. The effects of different treatments on the aperture length (A), aperture width (B), aperture width/length (C), and stomatal pore area (D) in the leaves of tomato plants. Supplemental lighting from the underneath canopy (USL) or from the inner canopy (ISL) was applied to plants from the time of transplantation while a no supplemental lighting condition was considered to be the control (CK). Parameters were measured on the second terminal leaflets of leaves on the fifth youngest node for each treatment. Means ± SE (n = 16) different letters indicate significant differences at P < 0.05 according to Duncan's multiple range test.
Enhanced leaf photosynthesis capacity and optimized stomatal regulation can enhance plant development (Hovi et al., 2004; Hovi and Tahvonen, 2008; Pettersen et al., 2010; O'Carrigan et al., 2014). In this study, tomato morphological features were notably influenced by the application of supplemental lighting (Table 1), reconfirming that plant morphology could be improved by increasing the light intensity (Seibert et al., 1975; Marschner and Cakmak, 1989) and also demonstrating the feasibility of cultivating tomato intensively through the application of supplemental lighting to the lower canopy. The remarkably improved plant profile (dry weight of both stems and roots, specific leaf area, health index) and reproductive speed (flower number) in W/R/B and W/B (Table 1), indicating a decreased unit cultivation period and an increased annual production time, which potentially improves the benefit return of this technique. These results reconfirmed that the enlarging blue light fraction in polychromatic illumination has better performance. Meanwhile, the indoleacetic acid (IAA) oxidase activity can be promoted by enhancing blue light proportion in illumination, which decreases the IAA level, consequently preventing excessive growth and guaranteeing reproductive development in various species, such as broad bean (Assmann et al., 1985), pepper (Brown et al., 1995), and lettuce (Li and Kubota, 2009). Additionally, under red light conditions, adding blue light irradiation could trigger epidermal cell elongation of the abaxial side and inhibit leaf epinasty in the geranium (Fukuda et al., 2008), results in more direct irradiation interception. Although R/B consisted of blue light, the green light spectrum was added to the W/R/B and W/B, and the addition of green light could enhance the photochemical content (Li and Kubota, 2009) and drive leaf photosynthesis more efficiently than red light (Terashima et al., 2009). A large proportional increase in the far-red light could significantly limit the biomass accumulation (Wang et al., 2009), which explained the inhibition of tomato plant growth in W/R/FR condition compared with other supplemental lighting treatment conditions.
Conclusion
Supplemental lighting with polychromatic light applied from either inner canopy or underneath canopy effectively increased tomato photosynthetic efficiency, reduced stomatal closure and improved plant development. W/R/B and W/B from underneath canopy promoted plants with higher health index and faster development, and presented better performance. CO2 utilization efficiency determined the variation of photosynthetic performance among the supplemental lighting treatments. An enhanced blue light fraction in W/R/B and W/B could better stimulate stomatal opening and promote photosynthetic electron transport activity, thus better improving photosynthetic rate. The mechanisms of photosynthesis improvement differed for the two light orientation treatments. The supplemental lighting from the inner canopy improved the photosynthesis of tomato plants by increasing the stomatal conductance to enhance the CO2 supply for leaf, thereby promoting photosynthetic electron transport activity. The supplemental lighting from the underneath canopy improved photosynthesis by enhancing the CO2 supply as well as increasing the CO2 assimilation efficiency and excessive energy dissipation, of which the enhancement contributed to a higher photosynthetic rate compared with the treatment of supplemental lighting from the inner canopy. Stomatal morphology was highly positively associated with leaf photosynthesis and plant development, and is therefore believed to be an important determinant for photosynthesis and growth of greenhouse cultivated tomato.
Author Contributions
YS and CJ contribute equally to this manuscript. YS, CJ, and LG conceived and designed the experiments. YS performed the experiments. YS and CJ analyzed the data and prepared the manuscript.
Funding
This work was supported by Xinjiang Major Project: Research and Demonstration of Critical Technology for Efficient and Sustainable Development of Xinjiang Agricultural Industrial Facilities (No. 201130104).
Conflict of Interest Statement
The authors declare that the research was conducted in the absence of any commercial or financial relationships that could be construed as a potential conflict of interest.
References
Acock, B., Charles-Edwards, D. A., Fitter, D. J., Hand, D. W., Ludwig, L. J., and Warren Wilson, J. (1978). The contribution of leaves from different levels within a tomato crop to canopy net photosynthesis: an experimental examination of two canopy models. J. Exp. Bot. 111, 815–827. doi: 10.1093/jxb/29.4.815
Aldesuquy, H. S., Abdel-Fattah, G. M., and Baka, Z. A. (2000). Changes in chlorophyll, polyamines and chloroplast ultrastructure of puccinia striiformis induced ‘greenislands’ on detached leaves of Triticum aestivum. Plant Physiol. Biochem. 38, 613–620. doi: 10.1016/S0981-9428(00)00783-X
Alokam, S., Chinnappa, C. C., and Reid, D. M. (2002). Red/far-red light mediated stem elongation and anthocyanin accumulation in Stellaria longipes: differential response of alpine and prairie ecotypes. Can. J. Bot. 80, 72–81. doi: 10.1139/b01-137
Araújo, W. L., Fernie, A. R., and Nunes-Nesi, A. (2011). Control of stomatal aperture. A renaissance of the old guard. Plant Signal. Behav. 6, 1305–1311. doi: 10.4161/psb.6.9.16425
Arena, C., Tsonev, T., Donevac, D., De Micco, V., Michelozzi, M., Brunetti, C., et al. (2016). The effect of light quality on growth, photosynthesis, leaf anatomy and volatile isoprenoids of a monoterpene-emitting herbaceous species (Solanum lycopersicum L.) and an isoprene-emitting tree (Platanus orientalis L.). Environ. Exp. Bot. 130, 122–132. doi: 10.1016/j.envexpbot.2016.05.014
Assmann, S. M., Simoncini, L., and Schroeder, J. I. (1985). Blue light activates electrogenic ion pumping in guard cell protoplasts of Vicia faba. Nature 318, 285–287. doi: 10.1038/318285a0
Bassman, J. B., and Zwier, J. G. (1991). Gas exchange characteristics of Populus trichocarpa, Populus deltoides and Populus trichocarpa × P. deltoides clones. Tree Physiol. 11, 145–149. doi: 10.1093/treephys/8.2.145
Brown, C. S., Schuerger, A. C., and Sager, J. C. (1995). Growth and photomorphogenesis of pepper plants under red light-emitting diodes with supplemental blue or far-red lighting. J. Am. Soc. Horticul. Sci. 120, 808–813.
Casson, S. A., and Hetherington, A. M. (2010). Environmental regulation of stomatal development. Curr. Opin. Plant Biol. 13, 90–95. doi: 10.1016/j.pbi.2009.08.005
Chang, T., Liu, X., Xu, Z., and Yang, Y. (2010). Effect of light spectra energy distribution on growth and development of tomato seedlings. Sci. Agric. Sin. 43, 1748–1756 (in Chinese with English abstract). doi: 10.3864/j.issn.0578-1752.2010.08.025
Chen, C., Huang, M., Kuan-Hung Lin, K., Wong, S., Huang, W., and Yang, D. (2014). Effects of light quality on the growth, development and metabolism of rice seedlings (Oryza sativa L.). Res. J. Biotechnol. 9, 15–24. Available online at: https://www.researchgate.net/publication/261988437
Chen, Z. H., Hills, A., Bätz, U., Amtmann, A., Lew, V. L., and Blatt, M. R. (2012). Systems dynamic modeling of the stomatal guard cell predicts emergent behaviors in transport, signaling, and volume control. Plant Physiol. 159, 1235–1251. doi: 10.1104/pp.112.197350
Chen, Z. H., Hills, A., Lim, C. K., and Blatt, M. R. (2010). Dynamic regulation of guard cell anion channels by cytosolic free Ca2+ concentration and protein phosphorylation. Plant J. 61, 816–825. doi: 10.1111/j.1365-313X.2009.04108.x
Cockshull, K. E., Graves, C. J., and Cave, C. R. J. (1992). The influence of shading on yield of greenhouse tomatoes. J. Horticul. Sci. Biotechnol. 67, 11–24. doi: 10.1080/00221589.1992.11516215
Demers, D. A., Dorais, M., Wien, C. H., and Gosselin, A. (1998). Effects of supplemental light duration on greenhouse tomato (Lycopersicon esculentum Mill.) plants and fruityields. Sci. Hortic. 74, 295–306. doi: 10.1016/S0304-4238(98)00097-1
Demers, D. A., and Gosselin, A. (2002). Growing greenhouse tomato and sweet pepper under supplemental lighting: optimal photoperiod, negative effects of long photoperiod and their causes. Acta Hortic. 580, 83–88. doi: 10.17660/ActaHortic.2002.580.9
Dorais, M. (2003). “The use of supplemental lighting for vegetable crop production: light intensity, crop response, nutrition, crop management, cultural practices,” in Canadian Greenhouse Conference (Toronto), 1–8.
Evans, J. R. (1995). Carbon fixation profiles do reflect light absorption profiles in leaves. Aust. J. Plant Physiol. 22, 865–873. doi: 10.1071/PP9950865
Evans, J. R., and Vogelmann, T. C. (2003). Profiles of 14C fixation through spinach leaves in relation to light absorption and photosynthetic capacity. Plant Cell Environ. 26, 547–560. doi: 10.1046/j.1365-3040.2003.00985.x
Fan, L. M., Zhao, Z., and Assmann, S. M. (2004). Guard cells: a dynamic signaling model. Curr. Opin. Plant Biol. 7, 537–546. doi: 10.1016/j.pbi.2004.07.009
Fan, X. X., Xu, Z. G., Liu, X. Y., Tang, C. M., Wang, L. W., and Han, X. (2013). Effects of light intensity on the growth and leaf development of young tomato plants grown under a combination of red and blue light. Sci. Hortic. 153, 50–55. doi: 10.1016/j.scienta.2013.01.017
Farquhar, G. D., and Sharkey, T. D. (1982). Stomatal conductance and photosynthesis. Ann. Rev. Plant Physiol. 33, 317–345. doi: 10.1146/annurev.pp.33.060182.001533
Farquhar, G. D., von Caemmerer, S. V., and Berry, J. A. (1980). A biochemical model of photosynthetic CO2 assimilation in leaves of C3 species. Planta 149, 78–90. doi: 10.1007/BF00386231
Frantz, J. M., Joly, R. J., and Mitchell, C. A. (2000). Intracanopy lighting influences radiation capture, productivity, and leaf senescence in cowpea canopies. J. Am. Soc. Hortic. Sci. 125, 694–701.
Fujii, T. (1952). Cultivation Techniques of Tomato. Kyoto: Takii Shubyo Shuppanbu Press (in Japanese).
Fukuda, N., Fujita, M., Ohta, Y., Sase, S., Nishimura, S., and Ezura, H. (2008). Directional blue light irradiation triggers epidermal cell elongation of abaxial side resulting in inhibition of leaf epinasty in geranium under red light condition. Sci. Hortic. 115, 176–182.
Gay, A. P., and Hurd, R. G. (1975). The influence of light on stomatal density in the tomato. New Phytol. 75, 37–46.
Ge, X. (1987). Overview of the study of health index of Solanaceous vegetables. China Vegetables 1, 32–34 (in Chinese).
Goins, G. D., Yorio, N. C., Sanwo, M. M., and Brown, C. S. (1997). Photo morphogenesis, photosynthesis, and seed yield of wheat plants grown under red light-emitting diodes (LEDs) with and without supplemental blue lighting. J. Exp. Bot. 48, 1407–1413. doi: 10.1093/jxb/48.7.1407
Gómez, C., Morrow, R. C., Bourget, C. M., Massa, G. D., and Mitchell, C. A. (2013). Comparison of intracanopy light-emitting diode towers and overhead high-pressure sodium lamps for supplemental lighting of greenhouse-grown tomatoes. Hort. Technol. 23, 93–98. Available online at: http://horttech.ashspublications.org/content/23/1/93.full.pdf+html
Hao, X., and Papadopoulos, A. P. (1999). Effects of supplemental lighting and cover materials on growth, photosynthesis, biomass partitioning, early yield and quality of greenhouse cucumber. Sci. Hortic. 80, 1–18.
Hogewoning, S. W., Douwstra, P., Trouwborst, G., van Ieperen, W., and Harbinson, J. (2010a). An artificial solar spectrum substantially alters plant development compared with usual climate room irradiance spectra. J. Exp. Bot. 61, 1267–1276. doi: 10.1093/jxb/erq005
Hogewoning, S. W., Trouwborst, G., Maljaars, H., Poorter, H., van Ieperen, W., and Harbinson, J. (2010b). Blue light dose–responses of leaf photosynthesis, morphology, and chemical composition of Cucumis sativus grown under different combinations of red and blue light. J. Exp. Bot. 61, 3107–3117. doi: 10.1093/jxb/erq132
Hovi, T., Nakkila, J., and Tahvonen, R. (2004). Intra-canopy lighting improves production of year-round cucumber. Sci. Hortic. 102, 283–294. doi: 10.1016/j.scienta.2004.04.003
Hovi, T., and Tahvonen, R. (2008). Effect of inter-lighting on yield and external fruit quality in year-round cultivated cucumber. Sci. Hortic. 116, 152–161. doi: 10.1016/j.scienta.2007.11.010
Huang, S., Xu, F., Wang, W., Du, J., Ru, M., Wang, J., et al. (2012). Seedling index of Salvia miltiorrhiza and its simulation model. Chinese J. Appl. Ecol. 23, 2779–2785 (in Chinese with English abstract). Available online at: http://www.cjae.net/CN/Y2012/V23/I10/2779
Kim, H. H., Wheeler, R. M., Sager, J. C., Goins, G. D., and Norikane, J. H. (2006). Evaluation of lettuce growth using supplemental green light with red and blue light-emitting diodes in a controlled environment-a review of research at Kennedy Space Center. Acta Hortic. 711, 111–119. doi: 10.17660/ActaHortic.2006.711.11
Kirdmanee, C., Kitaya, Y., and Kozai, T. (1993). “Effect of supplemental far-red lighting and photosynthetic photon flux density on stem elongation and dry weight increase of Eucalyptus camaldulensis in vitro plantlets,” in XVth International Botanical Congress (Yokohama).
Kopsell, D. A., and Kopsell, D. E. (2008). Genetic and environmental factors affecting plant lutein/zeaxanthin. Agro Food Ind. Hi Tech. 19, 44–46. Available online at: https://www.researchgate.net/publication/242179178
Kovács, L., Damkjaer, J., Kereïche, S., Ilioaia, C., Ruban, A. V., Boekema, E. J., et al. (2006). Lack of the light-harvesting complex CP24 affects the structure and function of the grana membranes of higher plant chloroplasts. Plant Cell 18, 3106–3120. doi: 10.1105/tpc.106.045641
Kramer, D. M., Johnson, G., Kiirats, O., and Edwards, G. E. (2004). New fluorescence parameters for the determination of QA redox state and excitation energy fluxes. Photosyn. Res. 79, 209–218. doi: 10.1023/B:PRES.0000015391.99477.0d
Kubínová, L. (1994). Recent stereological methods for measuring leaf anatomical characteristics: estimation of the number and sizes of stomata and mesophyll cells. J. Exp. Bot. 45, 119–127. doi: 10.1093/jxb/45.1.119
Lake, J. A., Quick, W. P., Beerling, D. J., and Woodward, F. I. (2001). Plant development: signals from mature to new leaves. Nature 411:154. doi: 10.1038/35075660
Lambers, H., Chapin, F. S. III., and Pons, T. L. (2008). Plant Physiological Ecology. New York, NY: Springer Science Business Media.
Lee, S. H., Tewari, R. K., Hahn, E. J., and Park, K. Y. (2007). Photon flux density and light quality induce changes in growth, stomatal development, photosynthesis and transpiration of Withania somnifera L. Dunal. plantlets. Plant Cell Tissue Organ Culture 90, 141–151. doi: 10.1007/s11240-006-9191-2
Li, Q., and Kubota, C. (2009). Effects of supplemental light quality on growth and phytochemicals of baby leaf lettuce. Environ. Exp. Bot. 67, 59–64. doi: 10.1016/j.envexpbot.2009.06.011
Loreto, F., Tsonev, T., and Centritto, M. (2009). The impact of blue light on leaf mesophyll conductance. J. Exp. Bot. 60, 2283–2290. doi: 10.1093/jxb/erp112
Lu, G., Zhang, H., and Zhou, C. (1984). A preliminary study of tomato health index. China Vegetables 1, 13–17 (in Chinese).
Lu, N., Maruo, T., Johkan, M., Hohjo, M., Tsukagoshi, S., Ito, Y., et al. (2012a). Effects of supplemental lighting within the canopy at different developing stages on tomato yield and quality of single-truss tomato plants grown at high density. Environ. Control Biol. 500, 1–11. doi: 10.2525/ecb.50.1
Lu, N., Maruo, T., Johkan, M., Hohjo, M., Tsukagoshi, S., Ito, Y., et al. (2012b). Effects of supplemental lighting with light-emitting diodes (LEDs) on tomato yield and quality of single-truss tomato plants grown at high planting density. Environ. Control Biol. 50, 63–74. doi: 10.2525/ecb.50.63
Marschner, H., and Cakmak, I. (1989). High light intensity enhances chlorosis and necrosis in leaves of zinc, potassium, and magnesium deficient bean (Phaseolus vulgaris) plants. J. Plant Physiol. 134, 308–315. doi: 10.1016/S0176-1617(89)80248-2
Massa, G. D., Kim, H. H., Wheeler, R. M., and Mitchell, C. A. (2008). Plant productivity in response to LED lighting. Hort. Sci. 43, 1951–1956. Available online at: http://hortsci.ashspublications.org/content/43/7/1951.full.pdf+html
Matsuda, R., Ohashi-Kaneko, K., Fujiwara, K., and Kurata, K. (2007). Analysis of the relationship between blue-light photon flux density and the photosynthetic properties of spinach (Spinaciaoleracea, L.) leaves with regard to the acclimation of photosynthesis to growth irradiance. Soil Sci. Plant Nutr. 53, 459–465. doi: 10.1111/j.1747-0765.2007.00150.x
Matsuda, R., Ozawa, N., and Fujiwara, K. (2014). Leaf photosynthesis, plant growth, and carbohydrate accumulation of tomato under different photoperiods and diurnal temperature differences. Sci. Hortic. 170, 150–158. doi: 10.1016/j.scienta.2014.03.014
Matsuda, R., Suzuki, K., Nakano, A., Higashide, T., and Takaichi, M. (2011). Responses of leaf photosynthesis and plant growth to altered source-sink balance in a Japanese and a Dutch tomato cultivar. Sci. Hortic. 127, 520–527. doi: 10.1016/j.scienta.2010.12.008
Maxwell, K., and Johnson, G. N. (2000). Chlorophyll fluorescence – apractical guide. J. Exp. Bot. 51, 659–668. doi: 10.1093/jexbot/51.345.659
McCree, K. J. (1972). The action spectrum, absorptance and quantum yield of photo-synthesis in crop plants. Agric. Meteorol. 9, 191–216. doi: 10.1016/0002-1571(71)90022-7
Meckel, T., Gall, L., Semrau, S., Homann, U., and Thiel, G. (2007). Guard cells elongate: relationship of volume and surface area during stomatal movement. Biophys. J. 92, 1072–1080. doi: 10.1529/biophysj.106.092734
Mott, K. A., Sibbernsen, E. D., and Shope, J. C. (2008). The role of the mesophyll in stomatal responses to light and CO2. Plant Cell Environ. 31, 1299–1306. doi: 10.1111/j.1365-3040.2008.01845.x
Nakano, Y., Sasaki, H., Nakano, A., Suzuki, K., and Takaichi, M. (2010). Growth and yield of tomato plants as influenced by nutrient application rates with quantitative control in closed rock wool cultivation. J. Jpn. Soc. Hortic. Sci. 79, 47–55.
Naumburg, E., and Ellsworth, D. S. (2002). Short-term light and leaf photosynthetic dynamics affect estimates of daily understory photosynthesis in four tree species. Tree Physiol. 22, 393–401. doi: 10.1093/treephys/22.6.393
Nawrocki, W. J., Tourasse, N. J., Taly, A., Rappaport, F., and Wollman, F. A. (2015). The plastid terminal oxidase: its elusive function points to multiple contributions to plastid physiology. Ann. Rev. Plant Biol. 66, 49–74. doi: 10.1146/annurev-arplant-043014-114744
Ni, J., Chen, X., Chen, C., and Xu, Q. (2009). Effects of supplemental different light qualities on growth, photosynthesis, biomass partition and early yield of greenhouse cucumber. Sci. Agric. Sin. 42, 2615–2623. doi: 10.1016/S0304-4238(98)00217-9
Nishio, J. N., Sun, J., and Vogelmann, T. C. (1993). Carbon fixation gradients across spinach leaves do not follow internal light gradients. Plant Cell. 5, 953–961. doi: 10.1105/tpc.5.8.953
O'Carrigan, A., Hinde, E., Lu, N., Xu, X., Duanc, H., Huang, G., et al. (2014). Effects of light irradiance on stomatal regulation and growth of tomato. Environ. Exp. Bot. 98, 65–73. doi: 10.1016/j.envexpbot.2013.10.007
Okamoto, K., Yanagi, T., Takita, S., Tanaka, M., Higuchi, T., and Ushida, Y. (1996). Development of plant growth apparatus using blue and red LED as artificial light source. Acta Hortic. 440, 111–116. doi: 10.17660/ActaHortic.1996.440.20
Olle, M., and Viršile, A. (2013). The effects of light-emitting diode lighting on greenhouse plant growth and quality. Agric. Food Sci. 22, 223–234. Available online at: http://ojs.tsv.fi/index.php/AFS/article/view/7897/6303
Pallozzi, E., Tsonev, T., Marino, G., Copolovici, L., Niinemets, Ü., Loreto, F., et al. (2013). Isoprenoid emissions, photosynthesis and mesophyll diffusion conductance in response to blue light. Environ. Exp. Bot. 95, 50–58. doi: 10.1016/j.envexpbot.2013.06.001
Pearcy, R. W. (1988). Photosynthetic utilization of light flecks by understory plants. Aust. J. Plant Physiol. 15, 223–238. doi: 10.1071/PP9880223
Pearcy, R. W. (1990). Sunflecks and photosynthesis in plant canopies. Annu. Rev. Plant Physiol. Plant Mol. Biol. 41, 421–453. doi: 10.1146/annurev.pp.41.060190.002225
Pettersen, R. I., Torre, S., and Gislerod, H. R. (2010). Effects of intera-canopy lighting on photosynthesis characteristics in cucumber. Sci. Hortic. 125, 77–81. doi: 10.1016/j.scienta.2010.02.006
Piringer, A. A., and Cathey, H. M. (1960). Effect of photoperiod, kind of supplemental light and temperature on the growth and flowering of petunia plants. Proc. Am. Soc. Horticul. Sci. 76, 649–660.
Poorter, H., and Garnier, E. (1996). Plant growth analysis: an evaluation of experimental design and computational methods. J. Exp. Bot. 47, 1343–1351. doi: 10.1093/jxb/47.9.1343
Powles, S. B. (1984). Photoinhibition of photosynthesis induced by visible light. Annu. Rev. Plant Physiol. 35, 15–44. doi: 10.1146/annurev.pp.35.060184.000311
Reng, X., Li, X., Zhang, X., Ye, W., Andrea, F., and Michael, R. (2003). The studies about the photosynthetic response of the four desert plants. Acta Ecol. Sin. 23, 598–605. doi: 10.1111/j.1399-3054.2012.01679.x
Runkle, E. S., and Heins, R. D. (2001). Specific functions of red, far red, and blue light in flowering and stem extension of long-day plants. J. Am. Soc. Horticul. Sci. 126, 275–282. Available online at: http://journal.ashspublications.org/content/126/3/275.full.pdf+html
Sarala, M., Taulavuori, E., Karhu, J., Savonen, E.-M., Laine, K., Kubin, E., et al. (2009). Improved elongation of Scots pine seedlings under blue light depletion isnot dependent on resource acquisition. Funct. Plant Biol. 36, 742–751. doi: 10.1071/FP09012
Schoch, P. G., Zinsou, C., and Sibi, M. (1980). Dependence of the stomatal index on environmental factors during stomatal differentiation in leaves of Vigna sinensis L.:1. Effect of light intensity. J. Exp. Bot. 31, 1211–1216. doi: 10.1093/jxb/31.5.1211
Seibert, M., Wetherbee, P. J., and Job, D. D. (1975). The effects of light intensity and spectral quality on growth and shoot initiation in tobacco callus. Am. Soc. Plant Biol. 56, 130–139. doi: 10.1104/pp.56.1.130
Sharkey, T. D., Bernacchi, C. J., Farquhar, G. D., and Singsaas, E. L. (2007). Fitting photosynthetic carbon dioxide response curves for C3 leaves. Plant Cell Environ. 30, 1035–1040. doi: 10.1111/j.1365-3040.2007.01710.x
Sharkey, T. D., and Raschke, K. (1981). Effect of light quality on stomatal opening in leaves of Xanthium strumarium L. Plant Physiol. 68, 1170–1174. doi: 10.1104/pp.68.5.1170
Shimazaki, K., Doi, M., Assmann, S. M., and Kinoshita, T. (2007). Light regulation of stomatal movement. Annu. Rev. Plant Biol. 58, 219–247. doi: 10.1146/annurev.arplant.57.032905.105434
Skillman, J. B. (2008). Quantum yield variation across the three pathways of photosynthesis: not yet out of the dark. J. Exp. Bot. 59, 1647–1661. doi: 10.1093/jxb/ern029
Soares, A. S., Driscoll, S. P., Olmos, E., Harbinson, J., Arrabaça, M. C., and Foyer, C. H. (2008). Adaxial/abaxial specification in the regulation of photosynthesis and stomatal opening with respect to light orientation and growth with CO2 enrichment in the C4 species Paspalum dilatatum. New Phytol. 177, 186–198. doi: 10.1111/j.1469-8137.2007.02218.x
Sokawa, Y., and Hase, E. (1967). Effect of light on the chlorophyll formation in the “glucose-bleached” cells of Chlorella protothecoides. Plant Cell Physiol. 8, 495–508.
Song, S. (1999). Study on the index of the healthy seedling of cucumber. J. Hebei Vocat. Tech. Teach. College 13, 58–63 (in Chinese with English abstract).
Steinger, T., Roy, B. A., and Stanton, M. L. (2003). Evolution in stressful environments II: adaptive value and costs of plasticity in response to low light in Sinapis arvensis. J. Evol. Biol. 16, 313–323. doi: 10.1046/j.1420-9101.2003.00518.x
Sun, J., Lu, N., Xu, H., Maruo, T., and Guo, S. (2016). Root zone cooling and exogenous spermidine root-pretreatment promoting Lactuca sativa L. growth and photosynthesis in the high-temperature season. Front. Plant Sci. 7:368. doi: 10.3389/fpls.2016.00368
Sun, J., and Nishio, J. N. (2001). Why abaxial illumination limits photosynthetic carbon fixation in spinach leaves? Plant Cell Physiol. 42, 1–8. doi: 10.1093/pcp/pce001
Sun, J., Nishio, J. N., and Vogelmann, T. C. (1998). Green light drives CO2 fixation deep within leaves. Plant Cell Physiol. 39, 1020–1026. doi: 10.1093/oxfordjournals.pcp.a029298
Talbott, L. D., and Zeiger, E. (1993). Sugar and organic acid accumulation in guard cells of Vicia faba in response to red and blue light. Plant Physiol. 102, 1163–1169. doi: 10.1104/pp.102.4.1163
Terashima, I., and Evans, J. R. (1988). Effects of light and nitrogen nutrition on the organization of the photosynthetic apparatus in spinach. Plant Cell Physiol. 29, 143–155.
Terashima, I., Fujita, T., Inoue, T., Chow, W. S., and Oguchi, R. (2009). Green light drives leaf photosynthesis more efficiently than red light in strong white light: revisiting the enigmatic question of why leaves are green. Plant Cell Physiol. 50, 684–697. doi: 10.1093/pcp/pcp034
Terashima, I., and Inoue, Y. (1985). Palisade tissue chloroplasts and spongy tissue chloroplasts in spinach: biochemical and ultrastructural differences. Plant Cell Physiol. 26, 63–75.
Terfa, M. T., Solhaug, K. A., Gislerød, H. R., Olsen, J. E., and Torre, S. (2013). A high proportion of blue light increases the photosynthesis capacity and leaf formation rate of Rosa hybrida but does not affect time to flower opening. Physiol. Plant 148, 146–159. doi: 10.1111/j.1399-3054.2012.01698.x
Tewolde, F. T., Lu, N., Shiina, K., Maruo, T., Takagaki, M., Kozai, T., et al. (2016). Nighttime supplemental LED inter-lighting improves growth and yield of single-truss tomatoes by enhancing photosynthesis in both winter and summer. Front. Plant Sci. 7:448. doi: 10.3389/fpls.2016.00448
Wahidin, S., Idris, A., and Shaleh, S. R. (2013). The influence of light intensity and photoperiod on the growth and lipid content of microalgae Nannochloropsis sp. Bioresour. Technol. 129, 7–11. doi: 10.1016/j.biortech.2012.11.032
Wang, H., Gu, M., Cui, J., Shi, K., Zhou, Y., and Yu, J. (2009). Effects of light quality on CO2 assimilation, chlorophyll-fluorescence quenching, expression of Calvin cycle genes and carbohydrate accumulation in Cucumis sativus. J. Photochem. Photobiol. B 96, 30–37. doi: 10.1016/j.jphotobiol.2009.03.010
Wang, Y., Noguchi, K., and Terashima, I. (2008). Distinct light responses of the adaxial and abaxial stomata in intact leaves of Helianthus annuus L. Plant Cell Environ. 31, 1307–1316. doi: 10.1111/j.1365-3040.2008.01843.x
Xu, H. L., Gauthier, L., Desjardins, Y., and Gosselin, A. (1997). Photosynthesis in leaves, fruits, stem and petioles of greenhouse-grown tomato plants. Photosynthetica 33, 113–112. doi: 10.1023/A:1022135507700
Yanagi, T., Okamoto, K., and Takita, S. (1996). Effects of blue, red, and blue/red lights of two different PPF levels on growth and morphogenesis of lettuce plants. Acta Hortic. 440, 117–122. doi: 10.17660/ActaHortic.1996.440.21
Yang, P., and Zhou, X. (2010). Effect of different fertilizer application standard on pumpkin root shoot ratio and healthy index. Southwest China. J. Agric. Sci. 23, 115–118 (in Chinese with English abstract). doi: 10.3969/j.issn.1001-4829.2010.01.025
Yorio, N. C., Goins, G. D., Kagie, H. R., Wheeler, R. M., and Sager, J. C. (2001). Improving spinach, radish, and lettuce growth under red light-emitting diodes (LEDs) with blue light supplementation. Hort. Sci. 36, 380–383. Available online at: http://hortsci.ashspublications.org/content/36/2/380.full.pdf
Keywords: light insufficiency, supplemental lighting, composite spectrums, underneath and inner canopy, leaf photosynthesis, stomatal regulation, plant growth
Citation: Song Y, Jiang C and Gao L (2016) Polychromatic Supplemental Lighting from underneath Canopy Is More Effective to Enhance Tomato Plant Development by Improving Leaf Photosynthesis and Stomatal Regulation. Front. Plant Sci. 7:1832. doi: 10.3389/fpls.2016.01832
Received: 30 August 2016; Accepted: 21 November 2016;
Published: 09 December 2016.
Edited by:
Iker Aranjuelo, Agribiotechnology Institute (IdAB)-CSIC-UPNA, SpainReviewed by:
Margherita Irene Beruto, Tuscia University, ItalyRosario Muleo, University of Tuscia, Italy
Copyright © 2016 Song, Jiang and Gao. This is an open-access article distributed under the terms of the Creative Commons Attribution License (CC BY). The use, distribution or reproduction in other forums is permitted, provided the original author(s) or licensor are credited and that the original publication in this journal is cited, in accordance with accepted academic practice. No use, distribution or reproduction is permitted which does not comply with these terms.
*Correspondence: Lihong Gao, Z2FvbGhAY2F1LmVkdS5jbg==
†These authors have contributed equally to this work.