- Department of Plant Sciences, School of Life Sciences, University of Hyderabad, Hyderabad, India
Dynamic regulation of stomatal aperture is essential for plants to optimize water use and CO2 uptake. Stomatal opening or closure is accompanied by the modulation of guard cell turgor. Among the events leading to stomatal closure by plant hormones or microbial elicitors, three signaling components stand out as the major converging points. These are reactive oxygen species (ROS), cytosolic free Ca2+, and ion channels. Once formed, the ROS and free Ca2+ of guard cells regulate both downstream and upstream events. A major influence of ROS is to increase the levels of NO and cytosolic free Ca2+ in guard cells. Although the rise in NO is an important event during stomatal closure, the available evidences do not support the description of NO as the point of convergence. The rise in ROS and NO would cause an increase of free Ca2+ and modulate ion channels, through a network of events, in such a way that the guard cells lose K+/Cl−/anions. The efflux of these ions decreases the turgor of guard cells and leads to stomatal closure. Thus, ROS, NO, and cytosolic free Ca2+ act as points of divergence. The other guard cell components, which are modulated during stomatal closure are G-proteins, cytosolic pH, phospholipids, and sphingolipids. However, the current information on the role of these components is not convincing so as to assign them as the points of convergence or divergence. The interrelationships and interactions of ROS, NO, cytosolic pH, and free Ca2+ are quite complex and need further detailed examination. Our review is an attempt to critically assess the current status of information on guard cells, while emphasizing the convergence and divergence of signaling components during stomatal closure. The existing gaps in our knowledge are identified to stimulate further research.
Introduction
Stomata are tiny pores found on the leaf surface of higher plants, which facilitate the evaporation of H2O via transpiration and intake of CO2 for photosynthetic carbon assimilation (Acharya and Assmann, 2009). Stomata are also major points of entry for pathogens into the plants (Melotto et al., 2006, 2008). Therefore, the regulation of stomatal aperture is essential for limiting the loss of H2O as well as restricting pathogen entry. The guard cells are quite sensitive to several internal and external stimuli, including abiotic (drought, light, temperature, high CO2, humidity) or biotic factors (pathogens and elicitors). Plant hormones (such as abscisic acid, ABA, methyl jasmonate, MJ) and polyamines (PAs) induce stomatal closure. Elicitors such as salicylic acid (SA), chitosan, and Flg22 also cause stomatal closure (Alcázar et al., 2010; Jing et al., 2012; Gayatri et al., 2013; Ye et al., 2013; Agurla et al., 2014). Stomata open when guard cells are turgid and close when the guard cells are flaccid (Blatt, 2000). During stomatal opening, guard cells accumulate osmotically active components, such as potassium ions, anions, malate and sucrose, leading a decrease in water potential, influx of water, and increase in turgor. In contrast, the reversal of these events leads to flaccidity in guard cells and stomatal closure (Vavasseur and Raghavendra, 2005; Bright et al., 2006; Roelfsema et al., 2012).
Among several effectors, the effects of ABA (a phytohormone) on stomatal movements have been studied in detail. ABA induced stomatal closure is mediated by many signaling components like cytoplasmic pH, reactive oxygen species (ROS), reactive nitrogen species (nitric oxide, NO), cytosolic free Ca2+, G-proteins, protein kinases, protein phosphatases, phospholipids, phospholipases, and sphingolipids (Wang and Song, 2008; Raghavendra et al., 2010; Umezawa et al., 2010; García-Mata and Lamattina, 2013; Song et al., 2014). The diverse spectrum of signaling components during stomatal closure have been reviewed frequently (Kim et al., 2010; Joshi-Saha et al., 2011; Gayatri et al., 2013; Agurla et al., 2014; Kollist et al., 2014; Song et al., 2014; Murata et al., 2015; Lee et al., 2016).
There are yet questions about the sequence of the signaling events during stomatal closure. For e.g., cytosolic free Ca2+ may act at either downstream or upstream of ROS/NO. The changes in cytosolic pH of guard cells may be important at either downstream or upstream of ROS or NO. The production of NO precedes that of ROS, but NO can act as antioxidant as well. Despite these ambiguities, it is clear that a rise in ROS or NO triggers a rise in free Ca2+ of guard cells, modulate the ion channels and cause an efflux of K+/Cl−/malate, leading to loss in turgor of guard cells. We emphasize that the signaling events during stomatal closure converge at ROS, cytosolic Ca2+, and ion channels. Similarly, ROS, NO, and Ca2+ form the points of divergence.
Points of Convergence: ROS, Cytosolic Free Ca2+, and Ion Channels
When guard cells are exposed to signals originating from abiotic or biotic factors the process of signal transduction is initiated. During this process, three points can be recognized as those of convergence: ROS, cytosolic free Ca2+, and anion channels. For e.g., plant hormones (such as ABA or MJ) and microbial elicitors invariably cause an increase in the levels of ROS or NO in guard cells, leading to rise in free Ca2+ within the guard cells (Table 1). There are excellent reviews, emphasizing the role of ROS (Kollist et al., 2014; Song et al., 2014; Murata et al., 2015), NO (Hancock et al., 2011; García-Mata and Lamattina, 2013; Gayatri et al., 2013; Agurla et al., 2014), and cytosolic free Ca2+ in guard cells (Kim et al., 2010; Roelfsema and Hedrich, 2010). Hormones and elicitors interact with different receptor entities, but the subsequent steps converge to activate NADPH oxidase, increase ROS, NO, and Ca2+ in guard cells (Figure 1). Although NO in guard cells is a key signaling component, there is no sufficient evidence to describe it as point of convergence. While it is clear that ROS can cause an increase in NO of guard cells, no other components that can raise NO levels has been described.
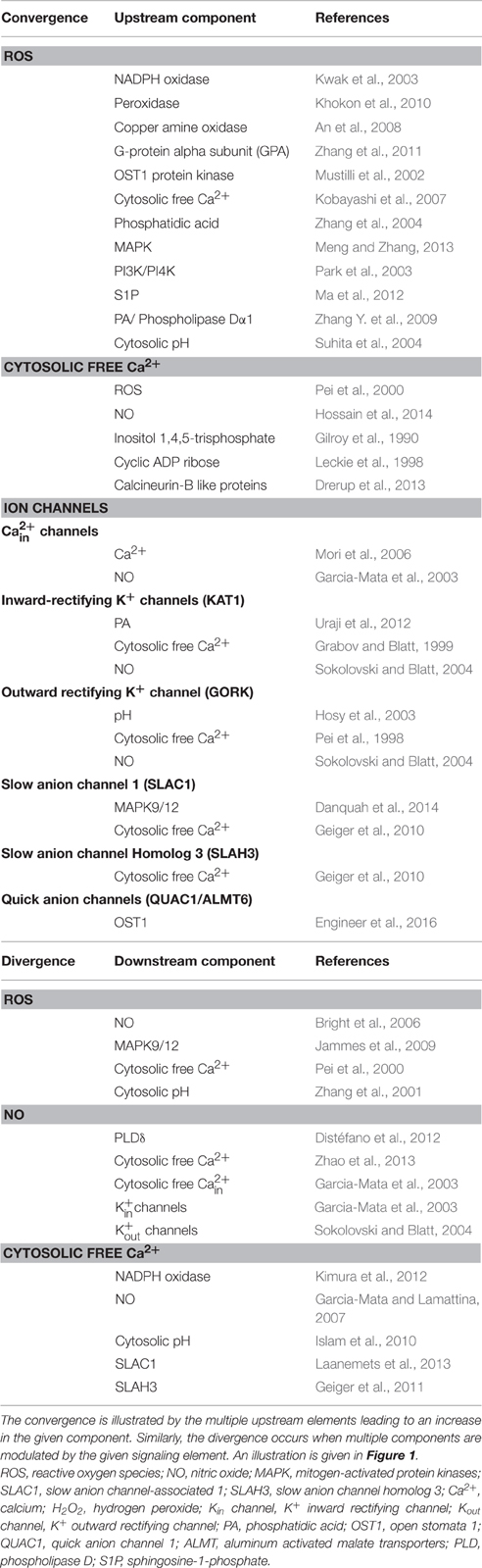
Table 1. Major points of convergence as well as divergence during signal transduction leading to stomatal closure by hormones or elicitors.
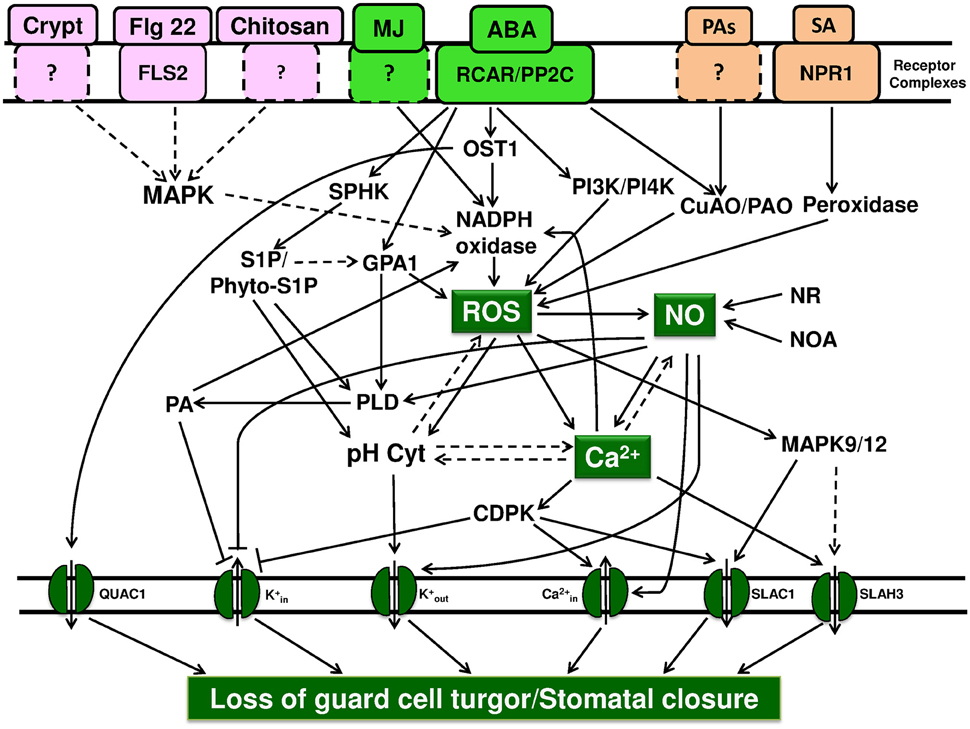
Figure 1. Key points of convergence and divergence during stomatal closure in response to plant hormones and elicitors. Stomatal closure is the result of ion efflux out of guard cells, loss of their turgor, and forms the ultimate step during signal transduction. We suggest that ROS, cytosolic free Ca2+, and ion channels form points of convergence during stomatal closure by a variety of abiotic/abiotic factors. Similarly, ROS, NO, and cytosolic Ca2+ are identified as points of divergence. The activation of NADPH oxidase and ROS production are among the earliest events. Similarly, the modulation of ion channels, influx of free Ca2+ along with efflux of K+ and anions, are the final steps, leading to the loss of ions/turgor of guard cells. The binding of ABA to RCAR/PYR or Flg22 to FLS2 or SA to S-receptor are well established, while receptors of cryptogein, chitosan, and PAs are yet to be characterized. ROS: When ABA binds to the receptor (RCAR/PYR/PYL), PP2C becomes non-functional, leading to phosphorylation, and activation of OST1 protein kinase. The elevated kinase activity along with Ca2+, activates NADPH oxidase, and subsequently elevates ROS production. Besides NADPH oxidase, CuAO/PAO are also involved in the increase of ROS in guard cells. The levels of ROS can be elevated by also peroxidase, for e.g., upon salicylic acid binding to its receptor. Further, G-protein alpha subunit induces the ROS production through the activation of NADPH oxidase. Modulation of ROS levels by NO, cytosolic Ca2+, cytosolic pH can occur by direct or indirect mechanisms but these reactions need to be established. Cytosolic free Ca2+: the rise in the levels of ROS and NO, can increase the levels of cytosolic free Ca2+, by either release of Ca2+from internal stores or influx of external Ca2+ through plasma membrane channels. Ca2+ also activates SLAH3 and SLAC1 ion channels, while inhibiting ion channels. Ion channels: the modulation of cation/anion channels results in the net efflux of K+/Cl−/ malate and influx of Ca2+, making guard cells to lose turgor and causing stomatal closure. NO: NR, nitrate reductase; NOA, nitric oxide associated 1 are the sources of NO. Although there are suggestions that ROS, cytosolic Ca2+ or cytosolic pH can elevate NO levels, the mechanism is not known. The rise in NO leads to divergent actions, namely the rise in cytosolic Ca2+, activation of PLD, and subsequently NADPH oxidase. Further, NO activates ion channels, inhibits K+ channels, and activates ion channels. Other components: The role of cytosolic pH is not completely understood. The available evidence suggests that the cytosolic pH may act parallely with the events involving ROS/NO/cytosolic free Ca2+. Similarly, G-proteins, phospholipids, phospholipases, phosphatidyl inositol kinases, sphingolipids, and MAP kinases also act in such a way to cause the loss of turgor in guard cells and stomatal closure. Solid arrows represent the events which are documented, while broken arrows represent the possible effects/suggestions.
Reactive Oxygen Species (ROS)
A marked rise in ROS of guard cells is a consistent feature of stomatal closure induced by ABA, MJ, and even microbial elicitors (Zhang H. et al., 2009; Song et al., 2014). While the effect of ABA on NADPH oxidase is mediated by ABA-receptors-protein phosphatase interactions (Raghavendra et al., 2010), the mechanism of NADPH oxidase stimulation by elicitors is ambiguous. Certain MAP kinases activated by elicitors could in turn activate NADPH oxidase (Zhang H. et al., 2009).
There has been overwhelming evidence that NADPH oxidase is the major ROS source in ABA, MJ, or SA induced stomatal closure. However, the source of ROS may not always be NADPH oxidase, as ROS production in response to elicitors, such as SA, yeast elicitor, and chitosan can occur through a salicylhydroxamic acid (SHAM) sensitive peroxidase or amine oxidases (e.g., copper amine oxidase or polyamine oxidase) (Khokon et al., 2011; Gao et al., 2013; Murata et al., 2015). During stomatal closure induced by methylglyoxal (MG), isothiocyanates or thiocyanates, the rise in ROS of Arabidopsis guard cells was mediated by a SHAM sensitive peroxidase (Hoque et al., 2012; Hossain et al., 2013). Activation of NADPH oxidase can occur also by phosphatidic acid (PA) (Zhang H. et al., 2009). Thus, the ROS of guard cells is a major point of convergence. The ROS production by different systems, involving NADPH oxidase or peroxidase has been reviewed recently by Murata et al. (2015).
Cytosolic Free Calcium
Calcium (Ca2+) is an important secondary messenger during stomatal closure (McAinsh et al., 1990; Hubbard et al., 2012). The role of Ca2+ is confirmed by monitoring of Ca2+ in guard cells by fluorescent probes, the Ca2+ chelators, and Ca2+ channel blockers (Pei et al., 2000; Kim et al., 2010). The rise in Ca2+, due to influx or release from internal sources like endoplasmic reticulum, further activates anion channels and inhibits the channels, all leading to stomatal closure. There are suggestions that Ca2+ may act also upstream of ROS and NO (Garcia-Mata et al., 2003). In contrast, Zhang et al. (2011) observed that calcium channels functioned downstream of H2O2 in G-protein α-subunit (gpa1) mutants. In gpa1 mutants, ABA-induced ROS production was disrupted, but Ca2+ channels were activated by exogenous H2O2 application.
Ion Channels
The ion channels represent the last step of signal transduction, leading to stomatal closure. The ionic status driven by the activity of cation/anion channels determines the turgor state of guard cells. Rise in free Ca2+ of guard cells causes the efflux of K+/Cl−/other ions. The detailed descriptions of ion channels, their intracellular location, encoding genes, along with mutants are made in a few reviews (Hedrich, 2012; Roelfsema et al., 2012; Kollist et al., 2014). Plants have several types of K+ channels, which can allow either inward or outward movement of K+. The channels open up, when the membrane potential becomes hyperpolarized. In contrast, outward-rectifying K+channels () open when the membrane potential is depolarized.
Guard cell Ca2+-permeable cation channels are stimulated by H2O2 and NO, whose levels are raised by ABA or MJ during stomatal closure (Mori et al., 2006; Rienmüller et al., 2010). Elevated free Ca2+ in guard cells can be due to the activation of Ca2+ channels in not only plasma membrane but also vacuolar or internal membrane network. The activation of ion channels would promote efflux of malate and other anions make the guard cells lose turgor and cause stomatal closure. But, there is considerable ambiguity on the relative dominance and specificity of different ion channels. Guard cells are known to contain slow anion channel-associated 1 (SLAC), quick anion channel 1 (QUAC), slow anion channel homolog 3 (SLAH), and even aluminum activated malate transporters (ALMT) (Roelfsema et al., 2012). Further work is required to elucidate the role of each of these different types of anion channels and their interactions.
Points of Divergence: ROS, NO, and Cytosolic Free Ca2+
The rise in levels of ROS, NO, or cytosolic free Ca2+ in guard cells trigger multiple events downstream (Table 1). The ability to induce diverse effects makes these three signaling components qualified to be the points of divergence (Figure 1). The rise in ROS of guard cells initiates several downstream events: NO production, elevation of cytosolic free Ca2+, and rise in cytosolic pH (Wang and Song, 2008; Song et al., 2014). Kinetic studies indicated that ROS production was prior to the NO production (Gonugunta et al., 2008). The positioning of the ROS was further confirmed by using Arabidopsis mutants and hydrogen-rich water (HRW) (Xie et al., 2014). The impaired NO synthesis and stomatal closure in response by HRW and rescue of closure by exogenous application of NO in rbohF mutant indicated that ROS functioned as an upstream signaling component. The importance of ROS in NO production was also demonstrated in mutants deficient in G-proteins and nitrate reductase (Bright et al., 2006; He et al., 2013).
Nitric oxide (NO) is a small, gaseous molecule involved in growth, development and even disease resistance of plants (Domingos et al., 2015). Studies using modulators (scavengers/inhibitors/donors) of NO production emphasized the importance of NO during stomatal closure (Gayatri et al., 2013; Agurla et al., 2014). NO production in guard cells of Arabidopsis and Vicia faba is essential for stomatal closure by SA and yeast elicitor (Sun et al., 2010; Khokon et al., 2011). Real time monitoring studies suggested that NO acted as a downstream signaling component to the ROS as well as pH (Gonugunta et al., 2008; Srivastava et al., 2009). Nitric oxide synthase (NOS) is the source of NO in animal cells, but the presence/operation of NOS in plant cells is quite uncertain. Both nitrate reductase (NR) and NOA1 (nitric oxide associated) are shown to be the sources of NO in guard cells of V. faba and Arabidopsis (Hao et al., 2010; Gao et al., 2013).
The interaction of NO with the other signaling components is quite crucial (Gayatri et al., 2013). In guard cells, NO can cause multiple effects, namely rise in internal Ca2+, cytosolic alkalization, and activation of channels (Gonugunta et al., 2008; Jing et al., 2010). NO is also essential for the elevation of the signaling components, like PLDα1 and PLDδ, during PA induced stomatal closure (Distéfano et al., 2008, 2010; Uraji et al., 2012).
The components of downstream signaling by Ca2+ in guard cells are quite intriguing. The changes in Ca2+ are sensed and mediated by the different types of intracellular calcium binding proteins like calmodulins, calcium dependent protein kinases (CDPKs, particularly, CPK3, and CPK6) and calcium sensing receptors (CAS) (Mori et al., 2006). Ca2+-dependent CPK6, CPK21, and CPK23 activate SLAC1 in oocytes (Geiger et al., 2010; Brandt et al., 2012). In contrast, Ca2+-independent protein kinases like OST1 are involved in ABA activation of intracellular calcium channels (Murata et al., 2015). Ca2+-independent SnRK2 protein kinases such as OST1, have been shown to activate SLAC1 in Xenopus leavis oocytes (Geiger et al., 2009; Lee et al., 2009; Brandt et al., 2012). Such Ca2+ activation of S-type anion currents is an early and essential step during stomatal closure (Siegel et al., 2009; Chen et al., 2010).
Other Components
Cytosolic pH
Cytoplasmic pH is a signaling component in developmental processes, such as root growth (Scott and Allen, 1999). A marked rise in cytoplasmic pH is a common feature during stomatal closure by ABA, MJ, elicitors, and even S1P (Suhita et al., 2004; Gonugunta et al., 2008). Cytosolic alkalization and production of NO in the guard cells and stomatal closure were observed on exposure to ethephon (source of ethylene) and pyrabactin (Jing et al., 2010; Puli and Raghavendra, 2012). Similarly, darkness or ultraviolet B (UV-B) exogenous Ca2+ induced stomatal closure was also accompanied by the increase in cytoplasmic pH and ROS (Ma et al., 2013; Zhu et al., 2014). In a reverse of the situation, fusicoccin (a fungal phytotoxin, produced by Fusicoccum amygdale) induced stomatal opening, by causing cytoplasmic acidification, and lowering of NO levels, even in presence of ABA (Huang et al., 2013).
Among the upstream components leading to the alkalization of cytoplasm in guard cells are the elevated ROS, PA/PLD, NO, and S1P/phytoS1P. However, the exact trigger of guard cell alkalization on exposure to ABA or MJ or elicitors and the downstream events of cytoplasmic pH change are not clear. A possibility is that on cytoplasmic alkalization, the channels are activated, triggering K+ efflux and collapse of turgor in guard cells (Blatt and Armstrong, 1993). Cytosolic alkalization needs to coordinate with the increase in cytosolic free Ca2+ during ABA or MJ induced stomatal closure (Islam et al., 2010). Unlike the role of ROS, NO, and cytosolic Ca2+as points of convergence and divergence, the action of cytoplasmic pH seems to be parallel. Further experiments are needed to make cytoplasmic pH qualified to be called as a point of convergence.
G-Proteins
Although the modulation of heterotrimeric G proteins is known to be an important component leading to stomatal closure, the exact mode of G-protein action is ambiguous. Ge et al. (2015) suggested that ethylene induced stomatal closure was mediated through Gα induced ROS production in Arabidopsis thaliana. In similar case, Arabidopsis gpa1 mutants, deficient in G-protein α subunit, are impaired in Ca2+-channel activation, and ROS production, in response to ABA (Zhang et al., 2011). G-proteins were essential for the production of ROS as well as NO during the effects of UV-B irradiation or external Ca2+ (Li et al., 2009; Zhang et al., 2012; He et al., 2013). Most of these evidences suggest that G-proteins induce an increase in the levels of ROS in guard cells. It is not clear if ROS production is due to or independent of NADPH oxidase.
Phospho- and Sphingolipids
Phosphatidic acid (PA), the product of phospholipase C/D (PLC/PLD) induced stomatal closure by inhibiting channel in the guard cells, besides interacting with ABI1 and activating NADPH oxidase (Jacob et al., 1999; Zhang et al., 2004). NO induced stomatal closure was restricted by PLC/PLD inhibitors (Distéfano et al., 2008), suggesting that PA acts downstream of the NO during stomatal closure in V. faba. Furthermore, ABA-induced NO production was impaired in pldα1 mutant guard cells (Distéfano et al., 2008). Phosphoshingolipids such as sphingosine-1-phosphate (S1P) and phytosphingosine-1-phosphate (phytoS1P) regulate multiple functions in plants besides stomatal closure (Ng et al., 2001; Coursol et al., 2005; Puli et al., 2016). ABA activates sphingosine kinases (SHPKs), leading to the production of S1P. However, our knowledge of downstream signaling components of S1P is limited (Coursol et al., 2003).
Interactions among Signaling Components and with Environmental Factors
Signaling components, particularly ROS and NO, play an important role in not only stomatal closure but also in integrating stimuli from abiotic or biotic stress (Song et al., 2014; Saxena et al., 2016). The marked interactions between ROS, NO, Ca2+, and pH are pointed out (Zhang et al., 2001; Gonugunta et al., 2009; Song et al., 2014). ROS and NO interact with each other and can increase cytosolic Ca2+ and modulate ion channels. However, the feedback relationship between NO and ROS is obscure. Similarly, cytoplasmic pH may act directly on ion channels, particularly or indirectly by modulating ROS and/or NO, yet the mechanism of such action is not completely clear. Further, Ca2+ also can interact with NO and pH (Wang et al., 2011; Gayatri et al., 2013). It is likely that ABA plays a key role in these interactions. Endogenous ABA is involved during MJ-induced stomatal closure (Munemasa et al., 2007, 2011; Ye et al., 2013). Both the Ca2+-dependent and Ca2+-independent signaling pathways are considered to function during stomatal closure (Kim et al., 2010; Roelfsema et al., 2012). However, the interrelationships of such Ca2+-dependent and independent pathways during guard cell signal transduction are yet to be elucidated.
Interactions of guard cell signaling components with environmental factors are not only interesting but are essential for adaptation. Drought raises the levels of ROS and ABA levels in plant tissues, with both these phenomena leading to stomatal closure (Saxena et al., 2016). The effects of CO2 induced stomatal closure can also be mediated by ABA (Chater et al., 2015). Further experiments are needed to identify the exact link between CO2 and ABA. An increase in ROS due to elevated CO2 in guard cells (Kolla et al., 2007) could raise the endogenous ABA levels and amplify the signaling events leading to stomatal closure. Similar involvement and interactions of ROS, NO, and pH are reported during UV-B induced stomatal closure (He et al., 2013; Zhu et al., 2014).
Concluding Remarks
The patterns and action sequence of signaling components during stomatal closure have been worked out using different triggers, such as ABA, MJ, and chitosan (Gonugunta et al., 2009). Both plant hormones or microbial elicitors cause an increase in ROS, NO, pH, and free Ca2+ of guard cells, modulate ion channels, and cause an efflux of K+/Cl−/malate from guard cells, leading to stomatal closure. We emphasize that ROS, cytosolic Ca2+, and ion channels are the points of convergence (Figure 1). The cytosolic pH, G-proteins, and phospho-/sphingolipids are also important components during stomatal closure but they may be acting in parallel. Further work required to elucidate the perception of signals, such as methyl jasmonate or elicitors and how they activate NADPH oxidase leading to ROS production. Several of the unresolved questions make the stomatal guard cells an ideal system for studying signal transduction mechanism in plant cells.
Author Contributions
AR proposed the topic. AR and AS collected the literature, critically assessed the information, and wrote the manuscript together.
Conflict of Interest Statement
The authors declare that the research was conducted in the absence of any commercial or financial relationships that could be construed as a potential conflict of interest.
Acknowledgments
Our work on stomatal guard cells is supported by grants to AR of a JC Bose National Fellowship (No. SR/S2/JCB-06/2006) from the Department of Science and Technology and another from Department of Biotechnology (No. BT/PR9227/PBD/16/748/2007), both in New Delhi. SA is supported by a Senior Research Fellowship of University Grants Commission. We also thank DBT-CREBB, DST-FIST, and UGC-SAP for support of infrastructure in Department/School.
References
Acharya, B. R., and Assmann, S. M. (2009). Hormone interactions in stomatal function. Plant Mol. Biol. 69, 451–462. doi: 10.1007/s11103-008-9427-0
Agurla, S., Gayatri, G., and Raghavendra, A. S. (2014). Nitric oxide as a secondary messenger during stomatal closure as a part of plant immunity response against pathogens. Nitric Oxide 43, 89–96. doi: 10.1016/j.niox.2014.07.004
Alcázar, R., Altabella, T., Marco, F., Bortolotti, C., Reymond, M., Koncz, C., et al. (2010). Polyamines: molecules with regulatory functions in plant abiotic stress tolerance. Planta 231, 1237–1249. doi: 10.1007/s00425-010-1130-0
An, Z., Jing, W., Liu, Y., and Zhang, W. (2008). Hydrogen peroxide generated by copper amine oxidase is involved in abscisic acid-induced stomatal closure in Vicia faba. J. Exp. Bot. 59, 815–825. doi: 10.1093/jxb/erm370
Blatt, M. R. (2000). Cellular signaling and volume control in stomatal movements in plants. Annu. Rev. Cell Dev. Biol. 16, 221–241. doi: 10.1146/annurev.cellbio.16.1.221
Blatt, M. R., and Armstrong, F. (1993). K+ channels of stomatal guard cells: abscisic-acid evoked control of the outward rectifier mediated by cytoplasmic pH. Planta 191, 330–341. doi: 10.1007/BF00195690
Brandt, B., Brodsky, D. E., Xue, S., Negi, J., Iba, K., Kangasjärvi, J., et al. (2012). Reconstitution of abscisic acid activation of SLAC1 anion channel by CPK6 and OST1 kinases and branched ABI1 PP2C phosphatase action. Proc. Natl. Acad. Sci. U.S.A. 109, 10593–10598. doi: 10.1073/pnas.1116590109
Bright, J., Desikan, R., Hancock, J. T., Weir, I. S., and Neill, S. J. (2006). ABA-induced NO generation and stomatal closure in Arabidopsis are dependent on H2O2 synthesis. Plant J. 45, 113–122. doi: 10.1111/j.1365-313X.2005.02615.x
Chater, C., Peng, K., Movahedi, M., Dunn, J. A., Walker, H. J., Liang, Y. K., et al. (2015). Elevated CO2-induced responses in stomata require ABA and ABA signaling. Curr. Biol. 25, 2709–2716. doi: 10.1016/j.cub.2015.09.013
Chen, Z. H., Hills, A., Lim, C. K., and Blatt, M. R. (2010). Dynamic regulation of guard cell anion channels by cytosolic free Ca2+ concentration and protein phosphorylation. Plant J. 61, 816–825. doi: 10.1111/j.1365-313X.2009.04108.x
Coursol, S., Fan, L. M., Stunff, H. L., Spiegel, S., Gilroy, S., Assmann, S. M., et al. (2003). Sphingolipid signalling in Arabidopsis guard cells involves heterotrimeric G proteins. Nature 423, 651–654. doi: 10.1038/nature01643
Coursol, S., Stunff, H., Lynch, D. V., Gilroy, S., Assmann, S. M., Spiegel, S., et al. (2005). Arabidopsis sphingosine kinase and the effects of phytosphingosine-1-phosphate on stomatal aperture. Plant Physiol. 137, 724–737. doi: 10.1104/pp.104.055806
Danquah, A., de Zelicourt, A., Colcombet, J., and Hirt, H. (2014). The role of ABA and MAPK signaling pathways in plant abiotic stress responses. Biotechnol. Adv. 32, 40–52. doi: 10.1016/j.biotechadv.2013.09.006
Distéfano, A. M., García-Mata, C., Lamattina, L., and Laxalt, A. M. (2008). Nitric oxide-induced phosphatidic acid accumulation: a role for phospholipases C and D in stomatal closure. Plant Cell Environ. 31, 187–194. doi: 10.1111/j.1365-3040.2007.01756.x
Distéfano, A. M., Lanteri, M. L., ten Have, A., García-Mata, C., Lamattina, L., and Laxalt, A. M. (2010). Nitric oxide and phosphatidic acid signaling in plants. Plant Cell Monogr. 16, 223–242. doi: 10.1007/978-3-642-03873-0_15
Distéfano, A. M., Scuffi, D., García-Mata, C., Lamattina, L., and Laxalt, A. M. (2012). Phospholipase Dδ is involved in nitric oxide-induced stomatal closure. Planta 236, 1899–1907. doi: 10.1007/s00425-012-1745-4
Domingos, P., Prado, A. M., Wong, A., Gehring, C., and Feijo, J. A. (2015). Nitric oxide: a multitasked signaling gas in plants. Mol. Plant. 8, 506–520. doi: 10.1016/j.molp.2014.12.010
Drerup, M. M., Schlücking, K., Hashimoto, K., Manishankar, P., Steinhorst, L., Kuchitsu, K., et al. (2013). The calcineurin B-like calcium sensors CBL1 and CBL9 together with their interacting protein kinase CIPK26 regulate the Arabidopsis NADPH oxidase RBOHF. Mol. Plant. 6, 559–569. doi: 10.1093/mp/sst009
Engineer, C. B., Hashimoto-Sugimoto, M., Negi, J., Israelsson-Nordström, M., Azoulay-Shemer, T., Rappel, W. J., et al. (2016). CO2 sensing and CO2 regulation of stomatal conductance: advances and open questions. Trends Plant Sci. 21, 16–30. doi: 10.1016/j.tplants.2015.08.014
Gao, J., Wang, N., and Wang, G. X. (2013). Saccharomyces cerevisiae induced stomatal closure mainly mediated by salicylhydroxamic acid-sensitive peroxidases in Vicia faba. Plant Physiol. Biochem. 65, 27–31. doi: 10.1016/j.plaphy.2013.01.008
Garcia-Mata, C., Gay, R., Sokolovski, S., Hills, A., Lamattina, L., and Blatt, M. R. (2003). Nitric oxide regulates K+ and Cl−channels in guard cells through a subset of abscisic acid-evoked signaling pathways. Proc. Natl. Acad. Sci. U.S.A. 100, 11116–11121. doi: 10.1073/pnas.1434381100
Garcia-Mata, C., and Lamattina, L. (2007). Abscisic acid (ABA) inhibits light-induced stomatal opening through calcium-and nitric oxide-mediated signaling pathways. Nitric Oxide 17, 143–151. doi: 10.1016/j.niox.2007.08.001
García-Mata, C., and Lamattina, L. (2013). Gasotransmitters are emerging as new guard cell signaling molecules and regulators of leaf gas exchange. Plant Sci. 201–202, 66–73. doi: 10.1016/j.plantsci.2012.11.007
Gayatri, G., Agurla, S., and Raghavendra, A. S. (2013). Nitric oxide in guard cell as an important second messenger during stomatal closure. Front. Plant Sci. 4:425. doi: 10.3389/fpls.2013.00425
Ge, X.-M., Cai, H.-L., Lei, X., Zhou, X., Yue, M., He, J.-M., et al. (2015). Heterotrimeric G protein mediates ethylene-induced stomatal closure via hydrogen peroxide synthesis in Arabidopsis. Plant J. 82, 138–150. doi: 10.1111/tpj.12799
Geiger, D., Maierhofer, T., Al-Rasheid, K. A., Scherzer, S., Mumm, P., Liese, A., et al. (2011). Stomatal closure by fast abscisic acid signaling is mediated by the guard cell anion channel SLAH3 and the receptor RCAR1. Sci. Signal. 4, ra32. doi: 10.1126/scisignal.2001346
Geiger, D., Scherzer, S., Mumm, P., Marten, I., Ache, P., Matschi, S., et al. (2010). Guard cell anion channel SLAC1 is regulated by CDPK protein kinases with distinct Ca2+ affinities. Proc. Natl. Acad. Sci. U.S.A. 107, 8023–8028. doi: 10.1073/pnas.0912030107
Geiger, D., Scherzer, S., Mumm, P., Stange, A., Marten, I., Bauer, H., et al. (2009). Activity of guard cell anion channel SLAC1 is controlled by drought-stress signaling kinase phosphatase pair. Proc. Natl. Acad. Sci. U.S.A. 106, 21425–21430. doi: 10.1073/pnas.0912021106
Gilroy, S., Read, N. D., and Trewavas, A. J. (1990). Elevation of cytoplasmic calcium by caged calcium or caged inositol trisphosphate initiates stomatal closure. Nature 346, 769–771. doi: 10.1038/346769a0
Gonugunta, V. K., Srivastava, N., Puli, M. R., and Raghavendra, A. S. (2008). Nitric oxide production occurs after cytosolic alkalinization during stomatal closure induced by abscisic acid. Plant Cell Environ. 31, 1717–1724. doi: 10.1111/j.1365-3040.2008.01872.x
Gonugunta, V. K., Srivastava, N., and Raghavendra, A. S. (2009). Cytosolic alkalinization is a common and early messenger preceding the production of ROS and NO during stomatal closure by variable signals, including abscisic acid, methyl jasmonate and chitosan. Plant Signal. Behav. 4, 561–564. doi: 10.4161/psb.4.6.8847
Grabov, A., and Blatt, M. R. (1999). A steep dependence of inward-rectifying potassium channels on cytosolic free calcium concentration increase evoked by hyperpolarization in guard cells. Plant Physiol. 119, 277–288. doi: 10.1104/pp.119.1.277
Hancock, J. T., Neill, S. J., and Wilson, I. D. (2011). Nitric oxide and ABA in the control of plant function. Plant Sci. 181, 555–559. doi: 10.1016/j.plantsci.2011.03.017
Hao, F., Zhao, S., Dong, H., Zhang, H., Sun, L., and Miao, C. (2010). Nia1 and Nia2 are involved in exogenous salicylic acid-induced nitric oxide generation and stomatal closure in Arabidopsis. J. Integr. Plant Biol. 52, 298–307. doi: 10.1111/j.1744-7909.2010.00920.x
He, J.-M., Ma, X.-G., Zhang, Y., Sun, T.-F., Xu, F.-F., Chen, Y.-P., et al. (2013). Role and inter- relationship of Gα protein, hydrogen peroxide, and nitric oxide in ultraviolet B-induced stomatal closure in Arabidopsis leaves. Plant Physiol. 161, 1570–1583. doi: 10.1104/pp.112.211623
Hedrich, R. (2012). Ion channels in plants. Physiol. Rev. 92, 1777–1811. doi: 10.1152/physrev.00038.2011
Hoque, T. S., Uraji, M., Ye, W., Hossain, M. A., Nakamura, Y., and Murata, Y. (2012). Methylglyoxal-induced stomatal closure accompanied by peroxidase-mediated ROS production in Arabidopsis. J. Plant Physiol. 169, 979–986. doi: 10.1016/j.jplph.2012.02.007
Hossain, M. A., Ye, W., Munemasa, S., Nakamura, Y., Mori, I. C., and Murata, Y. (2014). Cyclic adenosine 5′-diphosphoribose (cADPR) cyclic guanosine 3′,5′-monophosphate positively function in Ca2+ elevation in methyl jasmonate-induced stomatal closure, cADPR is required for methyl jasmonate-induced ROS accumulation NO production in guard cells. Plant Biol. 16, 1140–1144. doi: 10.1111/plb.12175
Hossain, M. S., Ye, W., Hossain, M. A., Okuma, E., Uraji, M., Nakamura, Y., et al. (2013). Glucosinolate degradation products, isothiocyanates, nitriles, and thiocyanates, induce stomatal closure accompanied by peroxidase-mediated reactive oxygen species production in Arabidopsis thaliana. Biosci. Biotechnol. Biochem. 77, 977–983. doi: 10.1271/bbb.120928
Hosy, E., Vavasseur, A., Mouline, K., Dreyer, I., Gaymard, F., Porée, F., et al. (2003). The Arabidopsis outward K+ channel GORK is involved in regulation of stomatal movements and plant transpiration. Proc. Natl. Acad. Sci. U.S.A. 100, 5549–5554. doi: 10.1073/pnas.0733970100
Huang, A. X., She, X. P., Zhang, Y. Y., and Zhao, J. L. (2013). Cytosolic acidification precedes nitric oxide removal during inhibition of ABA induced stomatal closure by fusicoccin. Russ. J. Plant Physiol. 60, 60–68. doi: 10.1134/S1021443712060076
Hubbard, K. E., Siegelm, R. S., Valerio, G., Brandt, B., and Schroeder, J. I. (2012). Abscisic acid and CO2 signaling via calcium sensitivity priming in guard cells, new CDPK mutant phenotypes and a method for improved resolution of stomatal stimulus-response analyses. Ann. Bot. 109, 5–17. doi: 10.1093/aob/mcr252
Islam, M. M., Hossain, M. A., Jannat, R., Munemasa, S., Nakamura, Y., Mori, I. C., et al. (2010). Cytosolic alkalization and cytosolic calcium oscillation in Arabidopsis guard cells response to ABA and MeJA. Plant Cell Physiol. 51, 1721–1730. doi: 10.1093/pcp/pcq131
Jacob, T., Ritchie, S., Assmann, S. M., and Gilroy, S. (1999). Abscisic acid signal transduction in guard cells is mediated by phospholipase D activity. Proc. Natl. Acad. Sci. U.S.A. 96, 12192–12197. doi: 10.1073/pnas.96.21.12192
Jammes, F., Song, C., Shin, D., Munemasa, S., Takeda, K., Gu, D., et al. (2009). MAP kinases MPK9 and MPK12 are preferentially expressed in guard cells and positively regulate ROS mediated ABA signaling. Proc. Natl. Acad. Sci. U.S.A. 106, 20520–20525. doi: 10.1073/pnas.0907205106
Jing, L., Hua, L. G., Xia, H. L., and Xin, L. (2010). Ethylene-induced nitric oxide production and stomatal closure in Arabidopsis thaliana depending on changes in cytosolic pH. Chin. Sci. Bull. 55, 2403–2409. doi: 10.1007/s11434-010-4033-3
Jing, L., Zhi-hui, H., Guo-hua, L., Li-xia, H., and Xin, L. (2012). Hydrogen sulphide may function downstream of nitric oxide in ethylene induced stomatal closure in Vicia faba L. J. Integr. Agric. 11, 1644–1653. doi: 10.1016/S2095-3119(12)60167-1
Joshi-Saha, A., Valon, C., and Leung, J. (2011). Brand new START: abscisic acid perception and transduction in the guard cell. Sci. Signal. 4, re4. doi: 10.1126/scisignal.2002164
Khokon, M. A. R., Hossain, M. A., Munemasa, S., Uraji, M., Nakamura, Y., Mori, I. C., et al. (2010). Yeast elicitor-induced stomatal closure and peroxidase-mediated ROS production in Arabidopsis. Plant Cell Physiol. 51, 1915–1921. doi: 10.1093/pcp/pcq145
Khokon, M. A. R., Okuma, E., Hossain, M. A., Munemasa, S., Uraji, M., Nakamura, Y., et al. (2011). Involvement of extracellular oxidative burst in salicylic acid-induced stomatal closure in Arabidopsis. Plant Cell Environ. 34, 434–443. doi: 10.1111/j.1365-3040.2010.02253.x
Kim, T. H., Böhmer, M., Hu, H., Nishimura, N., and Schroeder, J. I. (2010). Guard cell signal transduction network: advances in understanding abscisic acid, CO2, and Ca2+ signaling. Annu. Rev. Plant Biol. 61, 561–591. doi: 10.1146/annurev-arplant-042809-112226
Kimura, S., Kaya, H., Kawarazaki, T., Hiraoka, G., Senzaki, E., Michikawa, M., et al. (2012). Protein phosphorylation is a prerequisite for the Ca2+-dependent activation of Arabidopsis NADPH oxidases and may function as a trigger for the positive feedback regulation of Ca2+ and reactive oxygen species. Biochim. Biophys. 1823, 398–405. doi: 10.1016/j.bbamcr.2011.09.011
Kobayashi, M., Ohura, I., Kawakita, K., Yokota, N., Fujiwara, M., Shimamoto, K., et al. (2007). Calcium-dependent protein kinases regulate the production of reactive oxygen species by potato NADPH oxidase. Plant Cell 19, 1065–1080. doi: 10.1105/tpc.106.048884
Kolla, V. A., Vavasseur, A., and Raghavendra, A. S. (2007). Hydrogen peroxide production is an early event during bicarbonate induced stomatal closure in abaxial epidermis of Arabidopsis. Planta 225, 1421–1429. doi: 10.1007/s00425-006-0450-6
Kollist, H., Nuhkat, M., and Roelfsema, M. R. (2014). Closing gaps: linking elements that control stomatal movement. New Phytol. 203, 44–62. doi: 10.1111/nph.12832
Kwak, J. M., Mori, I. C., Pei, Z. M., Leonhardt, N., Torres, M. A., Dangl, J. L., et al. (2003). NADPH oxidase AtrbohD and AtrbohF genes function in ROS-dependent ABA signalling in Arabidopsis. EMBO J. 22, 2623–2633. doi: 10.1093/emboj/cdg277
Laanemets, K., Wang, Y. F., Lindgren, O., Wu, J., Nishimura, N., Lee, S., et al. (2013). Mutations in the SLAC1 anion channel slow stomatal opening and severely reduce K+ uptake channel activity via enhanced cytosolic Ca2+ and increased Ca2+ sensitivity of K+ uptake channels. New Phytol. 197, 88–98. doi: 10.1111/nph.12008
Leckie, C. P., McAinsh, M. R., Allen, G. J., Sanders, D., and Hetherington, A. M. (1998). Abscisic acid-induced stomatal closure mediated by cyclic ADP-ribose. Proc. Natl. Acad. Sci. U.S.A. 95, 15837–15842. doi: 10.1073/pnas.95.26.15837
Lee, S. C., Lan, W., Buchanan, B. B., and Luan, S. (2009). A protein kinase-phosphatase pair interacts with an ion channel to regulate ABA signaling in plant guard cells. Proc. Natl. Acad. Sci. U.S.A. 106, 21419–21424. doi: 10.1073/pnas.0910601106
Lee, Y., Kim, Y. J., Kim, M. H., and Kwak, J. M. (2016). MAPK cascades in guard cell signal transduction. Front. Plant Sci. 7:80. doi: 10.3389/fpls.2016.00080
Li, J.-H., Liu, Y.-Q., Lü, P., Lin, H.-F., Bai, Y., Wang, X.-C., et al. (2009). A signaling pathway linking nitric oxide production to heterotrimeric G protein and hydrogen peroxide regulates extra cellular calmodulin induction of stomatal closure in Arabidopsis. Plant Physiol. 150, 114–124. doi: 10.1104/pp.109.137067
Ma, Y., She, X., and Yang, S. (2012). Sphingosine-1-phosphate (S1P) mediates darkness-induced stomatal closure through raising cytosol pH and hydrogen peroxide (H2O2) levels in guard cells in Vicia faba. Sci. China Life. Sci. 55, 974–983. doi: 10.1007/s11427-012-4386-8
Ma, Y., She, X., and Yang, S. (2013). Cytosolic alkalization-mediated H2O2 and NO production are involved in darkness induced stomatal closure in Vicia faba. Can. J. Plant Sci. 93, 119–130. doi: 10.4141/cjps2012-040
McAinsh, M. R., Brownlee, C., and Hetherington, A. M. (1990). Abscisic acid-induced elevation of guard cell cytosolic Ca2+ precedes stomatal closure. Nature 343, 186–188. doi: 10.1038/343186a0
Melotto, M., Underwood, W., and He, S. Y. (2008). Role of stomata in plant innate immunity and foliar bacterial diseases. Annu. Rev. Phytopathol. 46, 101–122. doi: 10.1146/annurev.phyto.121107.104959
Melotto, M., Underwood, W., Koczan, J., Nomura, K., and He, S. Y. (2006). Plant stomata function in innate immunity against bacterial invasion. Cell 126, 969–980. doi: 10.1016/j.cell.2006.06.054
Meng, X., and Zhang, S. (2013). MAPK cascades in plant disease resistance signaling. Annu. Rev. Phytopathol. 51, 245–266. doi: 10.1146/annurev-phyto-082712-102314
Mori, I. C., Murata, Y., Yang, Y., Munemasa, S., Wang, Y. F., Andreoli, S., et al. (2006). CDPKs CPK6 and CPK3 function in ABA regulation of guard cell S-type anion channels and Ca2+ permeable channels and stomatal closure. PLoS Biol. 4, 1749–1762. doi: 10.1371/journal.pbio.0040327
Munemasa, S., Mori, I. C., and Murata, Y. (2011). Methyl jasmonate signaling and signal crosstalk between methyl jasmonate and abscisic acid in guard cells. Plant Signal. Behav. 6, 939–941. doi: 10.4161/psb.6.7.15439
Munemasa, S., Oda, K., Watanabe-Sugimoto, M., Nakamura, Y., Shimoishi, Y., and Murata, Y. (2007). The coronatine-insensitive 1 mutation reveals the hormonal signaling interaction between abscisic acid and methyl jasmonate in Arabidopsis guard cells. Specific impairment of ion channel activation and second messenger production. Plant Physiol. 143, 1398–1407. doi: 10.1104/pp.106.091298
Murata, Y., Mori, I. C., and Munemasa, S. (2015). Diverse stomatal signaling and the signal integration mechanism. Annu. Rev. Plant Biol. 66, 21.1–21.24. doi: 10.1146/annurev-arplant-043014-114707
Mustilli, A. C., Merlot, S., Vavasseur, A., Fenzi, F., and Giraudat, J. (2002). Arabidopsis OST1 protein kinase mediates the regulation of stomatal aperture by abscisic acid and acts upstream of reactive oxygen species production. Plant Cell 14, 3089–3099. doi: 10.1105/tpc.007906
Ng, C. K. Y., Carr, K., McAinsh, M. R., Powell, B., and Hetherington, A. M. (2001). Drought-induced guard cell signal transduction involves sphingosine-1-phosphate. Nature 410, 596–599. doi: 10.1038/35069092
Park, K. Y., Jung, J. Y., Park, J., Hwang, J. U., Kim, Y. W., Hwang, I., et al. (2003). A role for phosphatidylinositol 3-phosphate in abscisic acid-induced reactive oxygen species generation in guard cells. Plant Physiol. 132, 92–98. doi: 10.1104/pp.102.016964
Pei, Z. M., Baizabal-Aguirre, V. M., Allen, G. J., and Schroeder, J. I. (1998). A transient outward-rectifying K+ channel current down-regulated by cytosolic Ca2+ in Arabidopsis thaliana guard cells. Proc. Natl. Acad. Sci. U.S.A. 95, 6548–6553. doi: 10.1073/pnas.95.11.6548
Pei, Z. M., Murata, Y., Benning, G., Thomine, S., Klusener, B., Allen, G. J., et al. (2000). Calcium channels activated by hydrogen peroxide mediate abscisic acid signalling in guard cells. Nature 406, 731–734. doi: 10.1038/35021067
Puli, M. R., and Raghavendra, A. S. (2012). Pyrabactin, an ABA agonist, induced stomatal closure and changes in signaling components of guard cells in abaxial epidermis of Pisum sativum. J. Exp. Bot. 63, 1349–1356. doi: 10.1093/jxb/err364
Puli, M. R., Rajsheel, P., Aswani, V., Agurla, S., Kuchitsu, K., and Raghavendra, A. S. (2016). Stomatal closure induced by phytosphingosine-1-phosphate and sphingosine-1-phosphate depends on nitric oxide and pH of guard cells in Pisum sativum. Planta. doi: 10.1007/s00425-016-2545-z. [Epub ahead of print].
Raghavendra, A. S., Gonugunta, V. K., Christmann, A., and Grill, E. (2010). ABA perception and signaling. Trends Plant Sci. 15, 395–401. doi: 10.1016/j.tplants.2010.04.006
Rienmüller, F., Beyhl, D., Lautner, S., Fromm, J., Al-Rasheid, K. A. S., Ache, P., et al. (2010). Guard cell-specific calcium sensitivity of high density and activity SV/TPC1 channels. Plant Cell Physiol. 51, 1548–1554. doi: 10.1093/pcp/pcq102
Roelfsema, M., and Hedrich, R. (2010). Making sense out of Ca2+ signals: their role in regulating stomatal movements. Plant Cell Environ. 33, 305–321. doi: 10.1111/j.1365-3040.2009.02075.x
Roelfsema, M. R., Hedrich, R., and Geiger, D. (2012). Anion channels: master switches of stress responses. Trends Plant Sci. 17, 221–229. doi: 10.1016/j.tplants.2012.01.009
Saxena, I., Srikanth, S., and Chen, Z. (2016). Cross talk between H2O2 and interacting signal molecules under plant stress response. Front. Plant Sci. 7:570. doi: 10.3389/fpls.2016.00570
Scott, A. C., and Allen, N. S. (1999). Changes in cytosolic pH within Arabidopsis root Columella cells play a key role in the early signaling pathway for root gravitropism. Plant Physiol. 121, 1291–1298. doi: 10.1104/pp.121.4.1291
Siegel, R. S., Xue, S., Murata, Y., Yang, Y., Nishimura, N., Wang, A., et al. (2009). Calcium elevation-dependent and attenuated resting calcium-dependent abscisic acid induction of stomatal closure and abscisic acid-induced enhancement of calcium sensitivities of S-type anion and inward-rectifying K+ channels in Arabidopsis guard cells. Plant J. 59, 207–220. doi: 10.1111/j.1365-313X.2009.03872.x
Sokolovski, S., and Blatt, M. R. (2004). Nitric oxide block of outward-rectifying K+ channels indicates direct control by protein nitrosylation in guard cells. Plant Physiol. 136, 4275–4284. doi: 10.1104/pp.104.050344
Song, Y., Miao, Y., and Song, C. P. (2014). Behind the scenes: the roles of reactive oxygen species in guard cells. New Phytol. 201, 1121–1140. doi: 10.1111/nph.12565
Srivastava, N., Gonugunta, V. K., Puli, M. R., and Raghavendra, A. S. (2009). Nitric oxide production occurs downstream of reactive oxygen species in guard cells during stomatal closure induced by chitosan in abaxial epidermis of Pisum sativum. Planta 229, 757–765. doi: 10.1007/s00425-008-0855-5
Suhita, D., Raghavendra, A. S., Kwak, J. M., and Vavasseur, A. (2004). Cytosolic alkalinization precedes reactive oxygen species production during methyl jasmonate and abscisic acid-induced stomatal closure. Plant Physiol. 134, 1536–1545. doi: 10.1104/pp.103.032250
Sun, L. R., Hao, F. S., Lu, B. S., and Ma, L. Y. (2010). AtNOA1 modulates nitric oxide accumulation and stomatal closure induced by salicylic acid in Arabidopsis. Plant Signal. Behav. 5, 1022–1024. doi: 10.4161/psb.5.8.12293
Umezawa, T., Nakashima, K., Miyakawa, T., Kuromori, T., Tanokura, M., Shinozaki, K., et al. (2010). Molecular basis of the core regulatory network in ABA responses: sensing, signaling and transport. Plant Cell Physiol. 51, 1821–1839. doi: 10.1093/pcp/pcq156
Uraji, M., Katagiri, T., Okuma, E., Ye, W., Hossain, M. A., Masuda, C., et al. (2012). Cooperative function of PLDδ and PLDα1 in abscisic acid induced stomatal closure in Arabidopsis. Plant Physiol. 159, 450–460. doi: 10.1104/pp.112.195578
Vavasseur, A., and Raghavendra, A. S. (2005). Guard cell metabolism and CO2 sensing. New Phytol. 165, 665–682. doi: 10.1111/j.1469-8137.2004.01276.x
Wang, P., and Song, C. P. (2008). Guard-cell signalling for hydrogen peroxide and abscisic acid. New Phytol. 178, 703–718. doi: 10.1111/j.1469-8137.2008.02431.x
Wang, W. H., Yi, X. Q., Han, A. D., Liu, T. W., Chen, J., Wu, F. H., et al. (2011). Calcium-sensing receptor regulates stomatal closure through hydrogen peroxide and nitric oxide in response to extracellular calcium in Arabidopsis. J. Exp. Bot. 63, 177–190. doi: 10.1093/jxb/err259
Xie, Y., Mao, Y., Zhang, W., Lai, D., Wang, Q., and Shen, W. (2014). Reactive oxygen species-dependent nitric oxide production contributes to hydrogen-promoted stomatal closure in Arabidopsis. Plant Physiol. 165, 759–773. doi: 10.1104/pp.114.237925
Ye, W., Hossain, M. A., Munemasa, S., Nakamura, Y., Mori, I. C., and Murata, Y. (2013). Endogenous abscisic acid is involved in methyl jasmonate-induced reactive oxygen species and nitric oxide production but not in cytosolic alkalization in Arabidopsis guard cells. J. Plant Physiol. 170, 1212–1215. doi: 10.1016/j.jplph.2013.03.011
Zhang, H., Fang, Q., Zhang, Z., Wang, Y., and Zheng, X. (2009). The role of respiratory burst oxidase homologues in elicitor-induced stomatal closure and hypersensitive response in Nicotiana benthamiana. J. Exp. Bot. 60, 3109–3122. doi: 10.1093/jxb/erp146
Zhang, H., Wang, M., Wang, W., Li, D., Huang, Q., Wang, Y., et al. (2012). Silencing of G proteins uncovers diversified plant responses when challenged by three elicitors in Nicotiana benthamiana. Plant Cell Environ. 35, 72–85. doi: 10.1111/j.1365-3040.2011.02417.x
Zhang, W., Jeon, B. W., and Assmann, S. M. (2011). Heterotrimeric G-protein regulation of ROS signaling and calcium currents in Arabidopsis guard cells. J. Exp. Bot. 62, 2371–2379. doi: 10.1093/jxb/erq424
Zhang, W., Qin, C., Zhao, J., and Wang, X. (2004). Phospholipase D1-derived phosphatidic acid interacts with ABI1 phosphatase 2C and regulates abscisic acid signaling. Proc. Natl. Acad. Sci. U.S.A. 101, 9508–9513. doi: 10.1073/pnas.0402112101
Zhang, X., Dong, F. C., Gao, J. F., and Song, C. P. (2001). Hydrogen peroxide-induced changes in intracellular pH of guard cells precede stomatal closure. Cell Res. 11, 37–43. doi: 10.1038/sj.cr.7290064
Zhang, Y., Zhu, H., Zhang, Q., Li, M., Yan, M., Wang, R., et al. (2009). Phospholipase Dα1 and phosphatidic acid regulate NADPH oxidase activity and production of reactive oxygen species in ABA-mediated stomatal closure in Arabidopsis. Plant Cell 21, 2357–2377. doi: 10.1105/tpc.108.062992
Zhao, X., Li, Y. Y., Xiao, H. L., Xu, C. S., and Zhang, X. (2013). Nitric oxide blocks blue light-induced K+ influx by elevating the cytosolic Ca2+ concentration in Vicia faba guard cells. J. Integr. Plant Biol. 55, 527–536. doi: 10.1111/jipb.12038
Keywords: ABA, cytosolic free Ca2+, cytosolic pH, ROS, guard cells, ion channels, nitric oxide, secondary messengers
Citation: Agurla S and Raghavendra AS (2016) Convergence and Divergence of Signaling Events in Guard Cells during Stomatal Closure by Plant Hormones or Microbial Elicitors. Front. Plant Sci. 7:1332. doi: 10.3389/fpls.2016.01332
Received: 24 April 2016; Accepted: 05 August 2016;
Published: 24 August 2016.
Edited by:
Girdhar Kumar Pandey, University of Delhi, IndiaReviewed by:
Chun-Peng Song, Henan University, ChinaGaurav Zinta, Shanghai Center for Plant Stress Biology (PSC), China
Francesca Sparla, University of Bologna, Italy
Copyright © 2016 Agurla and Raghavendra. This is an open-access article distributed under the terms of the Creative Commons Attribution License (CC BY). The use, distribution or reproduction in other forums is permitted, provided the original author(s) or licensor are credited and that the original publication in this journal is cited, in accordance with accepted academic practice. No use, distribution or reproduction is permitted which does not comply with these terms.
*Correspondence: Agepati S. Raghavendra, as_raghavendra@yahoo.com; asrsls@gmail.com