- 1AgResearch Ltd., Christchurch, New Zealand
- 2Bio-Protection Research Centre, Lincoln University, Lincoln, New Zealand
Field parasitism rates of the Argentine stem weevil Listronotus bonariensis (Kuschel; Coleoptera: Curculionidae) by Microctonus hyperodae Loan (Hymenoptera: Braconidae) are known to vary according to different host Lolium species that also differ in ploidy. To further investigate this, a laboratory study was conducted to examine parasitism rates on tetraploid Italian Lolium multiflorum, diploid Lolium perenne and diploid hybrid L. perenne ×L. multiflorum; none of which were infected by Epichloë endophyte. At the same time, the opportunity was taken to compare the results of this study with observations made during extensive laboratory-based research and parasitoid-rearing in the 1990s using the same host plant species. This made it possible to determine whether there has been any change in weevil susceptibility to the parasitoid over a 20 year period when in the presence of the tetraploid Italian, diploid perennial and hybrid host grasses that were commonly in use in the 1990’s. The incidence of parasitism in cages, in the presence of these three grasses mirrored what has recently been observed in the field. When caged, weevil parasitism rates in the presence of a tetraploid Italian ryegrass host were significantly higher (75%) than rates that occurred in the presence of either the diploid perennial (46%) or the diploid hybrid (52%) grass, which were not significantly different from each other. This is very different to laboratory parasitism rates in the 1990s when in the presence of both of the latter grasses high rates of parasitism (c. 75%) were recorded. These high rates are typical of those still found in weevils in the presence of both field and caged tetraploid Italian grasses. In contrast, the abrupt decline in weevil parasitism rates points to the possibility of evolved resistance by the weevil to the parasitoid in the diploid and hybrid grasses, but not so in the tetraploid. The orientation of plants in the laboratory cages had no significant effect on parasitism rates under any treatment conditions suggesting that plant architecture may not be contributing to the underlying mechanism resulting in different rates of parasitism. The evolutionary implications of what appears to be plant-mediated resistance of L. bonariensis to parasitism by M. hyperodae are discussed.
Introduction
Over the last 25 years there has been increasing confidence that the impact of the Argentine stem weevil (Listronotus bonariensis) on Lolium-based pasture grasses has declined (Goldson et al., 2014a,b, 2015). This has largely been based on the use of selected strains of Epichloë endophytes that confer pest resistance in ryegrass (Johnson et al., 2013) combined with the significant impact of the braconid parasitoid biological control agent, Microctonus hyperodae (e.g., Barker and Addison, 2006).
Recently, however, there has also been growing field evidence that M. hyperodae may be losing its efficacy as a biological control agent of L. bonariensis. This declining control has been based on reports of a notable reappearance of L. bonariensis damage to pasture (e.g., Popay et al., 2011). In response, and as part of an investigation into the loss of efficacy, research has been focused on comparing current weevil parasitism levels with those in the 1990s (e.g., Goldson et al., 2014a,b). Such data can be very variable due to fluctuations in weevil population dynamics and parasitoid oviposition activity. However, during L. bonariensis overwintering diapause and coinciding parasitoid diapause, parasitism rates remain constant due to the hiatus in the insects’ development (Goldson and Emberson, 1981; Goldson and McNeill, 1992). Such overwintering stability has therefore permitted meta-analyses of historical datasets, which have shown that parasitism rates have declined notably in Lolium-based pastures since the parasitoid’s initial establishment and equilibration in the first 6 years of its release (e.g., Goldson et al., 2014a,b).
It is tempting to attribute this downward trend in parasitism to weevil resistance arising from continuous and high parasitoid selection pressure over the last c. 20 years as has been discussed recently by Goldson et al. (2015). The prospect of resistance is supported by the fact that the parasitoid undergoes parthenogenic (thelytokous) reproduction while the weevil reproduces sexually. This situation is what is sometimes described as an ‘unequal evolutionary arms-race’. However, other mechanisms could also contribute to the decline, such as changed farming practice, climate change, and the use of novel endophytes. These possibilities have been variously investigated, thus far without identifying any clear causative reason for the parasitism decline (e.g., Goldson et al., 2015); thus the acquisition of rapidly evolved resistance remains a possibility.
Rapid evolution in insect biocontrol has been known to occur elsewhere. In a study of field crickets (Teleogryllus oceanicus) on the Hawaiian islands, Pascoal et al. (2014) showed that genetically based resistance in this species occurred twice and involved separate genetic changes on different islands within the archipelago. On both occasions the crickets stopped stridulating (after about 24 generations) because such activity attracted the parasitic fly (Ormia ochracea) and this species exerted negative selection pressure. Listronotus bonariensis may similarly have developed genetically based resistance as it has undergone c. 50 generations since the first releases of M. hyperodae.
It is possible that plant species used in pastures may play a part in the observed reduction in parasitism. Goldson et al. (2015) noted in the field that parasitism of L. bonariensis by M. hyperodae was significantly higher in tetraploid L. multiflorum (Italian) ryegrass paddocks than in diploid perennial (L. perenne) ryegrass paddocks. These perennial paddocks were exposed to the same L. bonariensis and M. hyperodae populations as the Italian paddocks. In order to see if such differences could also be detected in the laboratory, preliminary observations were made in 2014 and these suggested that weevils maintained on the Italian or on perennial plants had different parasitism rates (Goldson and Tomasetto, unpublished data).
Of the 23 different species of pasture plants now commercially available to farmers in New Zealand (Charlton and Belgrave, 1992; Charlton and Stewart, 1999), Lolium perenne, L. multiflorum, and the hybrid L. perenne × L. multiflorum (L. boucheanum syn. Hybridum) are the most common. A description of these three pasture plants can be found in Langer’s (1973) textbook.
This contribution describes a systematic laboratory study using grasses similar to those studied in the field by Goldson et al. (2015) to determine whether similar plant-associated differences in parasitism rates occurred in the controlled and very different environment of cages. At the same time, this also permitted direct comparison with those data obtained during similar and extensive laboratory-based parasitoid research and rearing throughout the 1990s (e.g., Goldson et al., 1993; McNeill et al., 1999, 2002). Through such comparison it was possible to determine whether, in the intervening years, there has been a reduction in laboratory weevil susceptibility to M. hyperodae similar to that which has been found in the recently collated extensive field parasitism data (e.g., Goldson et al., 2014a,b).
Materials and Methods
Grass Type and Parasitism Rate
The Lolium grasses used in this study were Italian tetraploid L. multiflorum (cv. Grasslands Tama), diploid L. perenne (cv. Grasslands Samson) and diploid hybrid L. perenne ×L. multiflorum (cv. Grasslands Manawa). For clarity and brevity these grasses are referred to as ‘Italian’ grass, ‘perennial’ grass and ‘hybrid’ grass, respectively; all were endophyte free. Endophytes were excluded because there is now a wide range of differently acting novel endophyte strains in use in New Zealand pasture grasses. Also endophytes may have subtle effects on parasitoid behavior although this has not shown up in a recent field study (e.g., Goldson et al., 2015). Finally endophytes often do not perform very well in the tetraploid L. multiflorum varieties, so in general it seemed prudent to exclude the endophyte variable from the experiment. The grass types that were chosen represent the typical pasture types used in New Zealand farming since the release of the parasitoid.
All experimental work was conducted at ambient laboratory temperatures (23 ± 2°C) and 16:8 L:D photoperiod. Weevil adults were collected from mid-Canterbury ryegrass pastures using a modified leaf blowing machine (Goldson et al., 2000) between January 11, 2016 and January 22, 2016. They were then purged of egg and larval parasitoids by storing them for a minimum of 40 days and a maximum of 55 days with the remaining unparasitised population used for the experiment. The M. hyperodae pupae that emerged from these weevils were reared to obtain adult parasitoids for this study. Overall as detailed below, the experiment comprised three main treatments (grass types) with two subtreatments (plant positioning). These were replicated four times. There we also four grass-free control cages making 28 cages in total.
The experiment was established on March 17, 2016 using 305 mm × 205 mm × 130 mm translucent plastic cages with gauze lids. The four grass main treatment replicates were the minimum required to deal with pseudoreplication (Johnson et al., 2016). All cages were stocked with 23 L. bonariensis and two M. hyperodae. Each cage contained one of the three grass species treatments in the form of two 150 mm long bouquets with their moistened roots and associated soil in tightly sealed small polythene bags at the base of the plants. This resulted in at least 40 tillers per box (Supplementary Figure S1). Each grass treatment comprised two subtreatments, in separate cages, whereby the bouquets were positioned either horizontally or vertically; thus the cages were, respectively, positioned either on one end, or lying flat (Supplementary Figure S1). This different positioning of the plants was specifically to gain an initial indication of whether departure from vertical plant architecture had an effect on weevil parasitism in any of the three grass types. Gerard (2000) noted that weevils tend to leave the upright foliage in the presence of the parasitoid and Phillips (2002) suggested that plant orientation in a cage may influence parasitoid efficacy. There was also a control treatment comprising four cages (two horizontal and two vertical) containing 23 L. bonariensis, two M. hyperodae and two water-soaked dental wicks to maintain humidity. All paired treatments were placed randomly in the laboratory (Supplementary Figure S1). Parasitoids were removed from the cages after 48 h. Thereafter the weevils were maintained in the same ambient conditions for another 3 days until March 22, 2016 when they were frozen at -20°C prior to being dissected to assess parasitism rates (i.e., number of parasitized weevils per total number of weevils dissected).
Comparative Rates of Parasitism between the 1990s and 2016
In this study, the opportunity was taken to use the same Lolium grass types as were used throughout the 1990s during general research into M. hyperodae including the parasitoid’s mass-rearing for release (Goldson et al., 1993; McNeill et al., 1999, 2002). This allowed us to directly compare the results obtained from this experiment with both published and unpublished work conducted in the 1990s. Notably, while the exposure periods of the weevils in some of the comparator experiments were sometimes longer than 48 h this was of minor importance as Phillips et al. (1996) have shown that parasitoid ovipositional effort declines rapidly after the first 48 h.
Statistical Analysis
To test for statistical significance between parasitism rates in the treatments and control, non-parametric complete random permutation tests (n cycles = 10000) were run for a one-way analysis of variance (ANOVA) via the package “lmPem” (Wheeler, 2010) and subsequently we tested the statistical significance via post hoc Tukey’s HSD pairwise permutation tests embedded in the package “stats” in R 3.2.1 (R Development Core Team, 2016). This approach implements the methods for permutation tests described by Kabacoff (2011).
Results
Grass Types and Parasitism Rates Within Foliage Positioning Subtreatments
The rate of parasitism of L. bonariensis by M. hyperodae in the presence of the experimental grass types and in the controls are presented in Supplementary Table S1 and the effects of grass type in Figure 1. In addition, Tables 1–3 show these results in the context of other studies yielding parasitism rates both in the 1990s and recently, in the presence of the Italian, diploid, and hybrid grasses, respectively. For purposes of comparison, all data have been normalized to be expressed as the effect of one parasitoid per population of weevils.
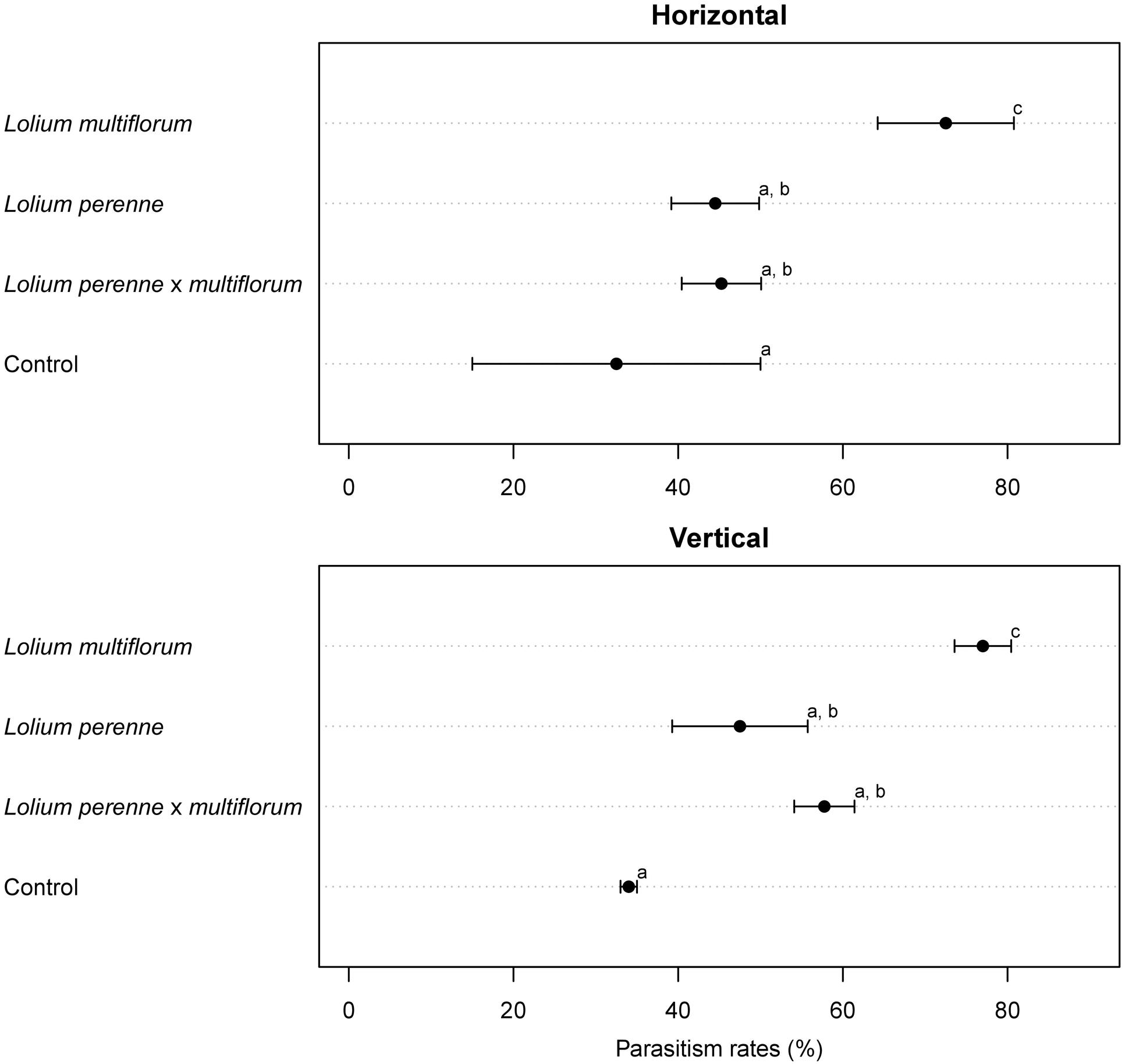
FIGURE 1. Cleveland dotplot for Microctonus hyperodae mean parasitism rates (%) as measured in Listronotus bonariensis in cages containing Italian tetraploid L. multiflorum (cv. Grasslands Tama), diploid L. perenne (cv. Grasslands Samson) and diploid hybrid L. perenne × L. multiflorum (cv. Grasslands Manawa) and in cages containing no Lolium spp (Control). The horizontal and vertical orientations (i.e., subtreatments) are shown here. Error bars represent SEM. Means with different letters were significantly different in pairwise comparisons.
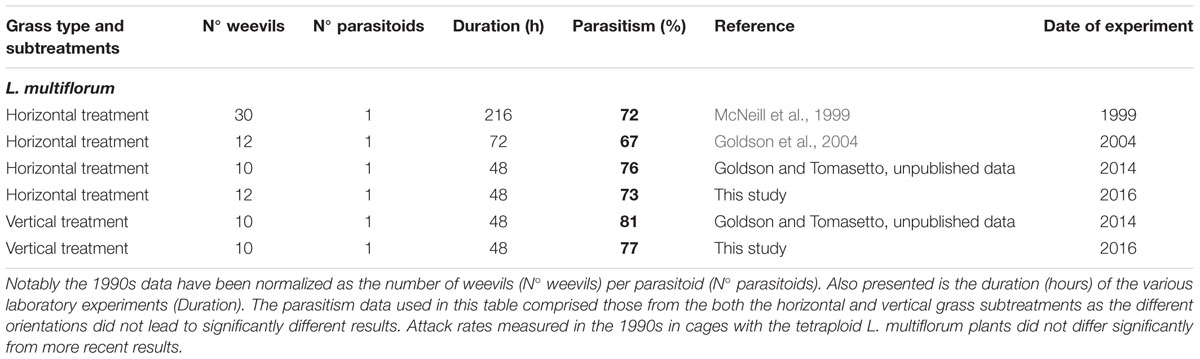
TABLE 1. Summary table presenting the results of this study and other published and unpublished laboratory work on Microctonus hyperodae parasitism rates (% shown in bold) in caged Listronotus bonariensis populations in the presence of tetraploid Lolium multiflorum (cv. Grasslands Tama) plants.
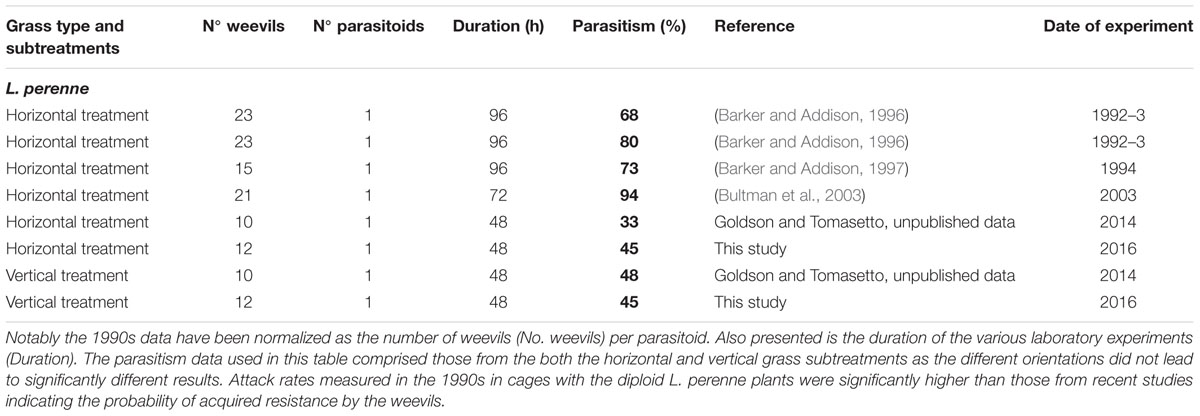
TABLE 2. Summary table presenting the results of this study and other published and unpublished laboratory work on M. hyperodae parasitism rates (% shown in bold) in caged L. bonariensis populations in the presence of diploid Lolium perenne (cv. Grasslands Samson) plants.
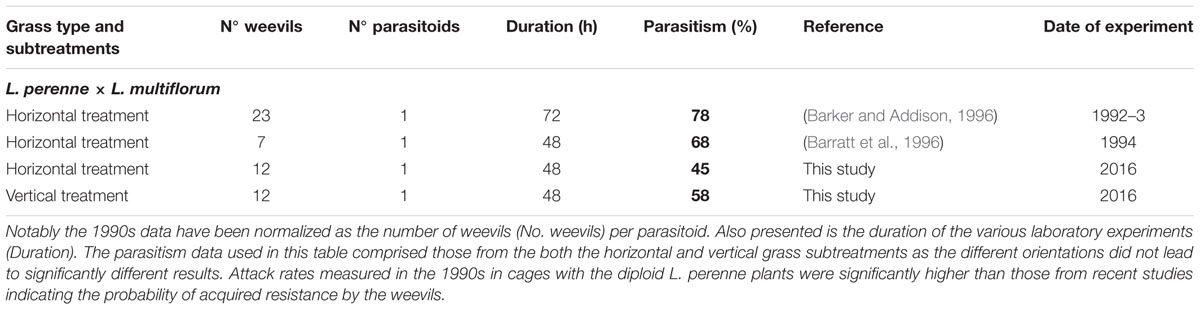
TABLE 3. Summary table presenting the results of this study and other published and unpublished laboratory work on M. hyperodae parasitism rates (% shown in bold) in caged L. bonariensis populations in the presence of diploid hybrid L. perenne × L. multiflorum (cv. Grasslands Manawa) plants.
Horizontal and Vertical Treatments Combined
The rate of parasitism in the presence of the Italian grass (75 ± 4%) was significantly higher than in either of the other grass treatments (P < 0.001). There was no significant difference in parasitism rates between cages containing perennial grass (46 ± 5%) and hybrid grass (52 ± 4%; P = 0.8). Parasitism rate in the control cages was 33 ± 7% and was significantly less than that found in cages containing grass (P < 0.01).
Horizontal Treatments
In the horizontal subtreatments, the rate of parasitism that occurred in the presence of Italian grass (73 ± 8%) was significantly higher (P < 0.001) than in either of the other horizontal subtreatments (Figure 1). There was no significant difference in parasitism rates between cages containing perennial grass (45 ± 5%) and hybrid grass (45 ± 5%, P = 0.08; Figure 1). Parasitism rate in the empty controls was 33 ± 18% which was not significantly different from the perennial and hybrid treatments but significantly less than in the Italian grass (P < 0.05; Figure 1).
Vertical Treatments
In the vertical subtreatments, the rate of parasitism that occurred in the presence of Italian grass (77 ± 3%) was significantly higher (P < 0.001) than in either of the other upright treatments (Figure 1). Again, there was no significant difference in parasitism rates in the cages containing the perennial grass (48 ± 8%) and the hybrid grass (58 ± 4%, P = 0.08; Figure 1). Parasitism in the empty control was 34 ± 1% which was not significantly different from the hybrid and perennial treatments (P = 0.5; Figure 1).
Horizontal versus Vertical Treatments
Horizontal versus vertical positioning of grass bouquets within the cages resulted in no significant differences in the rates of L. bonariensis parasitism by M. hyperodae across all of the grass types (Italian grass, P = 0.8; perennial grass P = 0.1, and hybrid grass = 0.8, respectively).
Comparative Parasitism Rates between the 1990s and 2016
In the 1990s experiments, only horizontal treatments were used, therefore only the data from the horizontal subtreatments in this study were used for direct comparisons.
Descriptive analysis of the horizontal data in Tables 1–3 show that in the 1990s, the mean parasitism rate in rearing cages containing perennial grass was 74 ± 4% as opposed to 39 ± 5% in the current study. Similarly the parasitism rate associated with the hybrid grass was 73 ± 6% in the 1990s compared with 45 ± 5% in this study. Conversely, the 73 ± 8% parasitism found in the presence of the Italian grass is very similar to that in the 1990s (70 ± 5%).
Discussion
An emphasis of this study was to determine whether there have been significant changes in parasitism rates of Argentine stem weevil by M. hyperodae on typical pasture grasses since the 1990s rather than it being a definitive study of grass type effect on parasitism levels. Such direct comparison with historical data was possible because the same tetraploid Italian and hybrid cultivars were used in this study as throughout the 1990s. Thus any varietal genetic uncertainty is controlled for. The diploid L. perenne does not have complex genetic origins thus the direct comparison of cv. Samson in this study to cv. Nui in the 1990s is legitimate as both were derived from old New Zealand perennial pasture.
Grass Types and Parasitism Rates
The observed 42% decline in M. hyperodae parasitism observed in this cage study in the diploid and hybrid grasses (Tables 2 and 3), compared to 1990s laboratory data, conforms to the findings of recent field-based studies that have indicated a similar c. 50% decline in parasitism rates in diploid grasses since the 1990s (Goldson et al., 2015). That such a reduction in parasitism did not occur in the presence of tetraploid Italian grass either in this study (Table 1) or in the field (Goldson et al., 2015) suggesting that whatever factor(s) reduced parasitism rates in the perennial and hybrid grasses (Tables 2 and 3) did not occur in the presence of Italian grass (Table 1). It is also significant that the laboratory parasitism rates in the Italian grasses in this study were typical of those previously occurring in both the perennial and hybrid grass types in the 1990s (Tables 2 and 3).
Insignificant Plant Orientation Effects
Horizontal versus vertical positioning of grass bouquets within the cages resulted in no significant differences in the rates of parasitism of L. bonariensis across all of the grass treatments (Table 1; Figure 1). Such result indicates that, at least in the absence of soil or detritus, the orientation of plant material does not affect parasitism rates. This is contrary to Phillips (2002) suggestion that plant orientation in a cage may influence parasitoid efficacy. Further, the lower attack rates in the diploid and hybrid grasses were unlikely to have been based on the avoidance of parasitism by the weevils abandoning the foliage in the presence of the parasitoid as discussed by Gerard (2000). In the horizontal treatment, the grass leaves were broadly spread across the floor of the cage obviating the ability of L. bonariensis to drop off. The results of this study also point to the probable incorrectness of the contention of Goldson et al. (2015) who suggested that the higher levels of parasitism in the tetraploid L. multiflorum could have resulted from a difference in the architecture of the tetraploid versus diploid and hybrid perennial plants. This architecture hypothesis would seem to have been possible when considering vertical plants. However, the horizontal plant placement was a gross departure from the natural growth habit, yet there were no differences in the levels of parasitism between plant positioning subtreatments and the upright plants. This suggests that plant architecture was unlikely to be the underlying cause of the observed differences in parasitism between the grass types.
Finally, all treatments in the cages comprised grass bouquets that were bundled at their stem bases where the roots entered the polythene bags thereby providing limited scope for the weevils to ‘hide’ from the parasitoids. This is clearly different from the growth habit of the plants in the field.
Ecological Implications
The lack of any notable difference in L. bonariensis parasitism rates in the cages containing diploid and endophyte-free hybrid grasses is significant ecologically. At the time of the first parasitoid releases, and in order to expedite its establishment by using areas with plentiful weevils, the work was conducted in either pure hybrid pastures or pastures comprising a mix of diploid and hybrid ryegrass (Goldson et al., 1998; Barker and Addison, 2006) as the hybrid is known to be preferred to the perennial as a host plant of the weevil (Goldson, 1982). As a consequence, some of the early parasitism field data were collected from these hybrid sites. This study has shown no differences in parasitism rates in the perennial and the hybrid grasses (Gaynor and Hunt, 1982; Goldson et al., 1998; Barker and Addison, 2006). This eliminates the prospect of any bias having occurred through possibly higher measured parasitism rates occurring in the limited and very early sampling in the hybrid grasses. This is consistent with the observation that high parasitism rates were typically found in the diploid pasture that surrounded the original release sites during investigation into the parasitoid’s lateral dispersal from the release sites (e.g., Barker and Addison, 1997; McNeill and Goldson, unpublished data).
Mechanisms for the Measured Differences in Attack Rates in the Laboratory and the Field
The attack rates measured in this caging study were very similar to those currently observed in the field (Goldson et al., 2015). This is surprising given the obvious environmental differences between the field and laboratory cages (e.g., no soil or detritus).
It can be hypothesized that the underlying mechanism for the observed general decline in parasitism rates since the 1990s (e.g., Goldson et al., 2014a,b) could have been based on the adoption of novel endophytes. However, none of the grasses in this laboratory study were infected with endophytes. Additionally, Goldson et al. (2015) in a 5-month summer field study, showed no significant field effects of endophyte on L. bonariensis parasitism rates in the mix of Lolium varieties and endophytes.
Contrary to the findings here, the data collected in the 1990s indicated no differences in parasitism rates, irrespective of grass type. At that time weevil parasitism rates in the hybrid and perennial grasses were comparable to those now only found in the tetraploid Italian plants (Tables 1–3).
Barker (1989) observed much higher rates of L. bonariensis feeding and oviposition in the leaves of tetraploid Italian grasses than in the perennial grasses. Related to this Phillips (2002) showed that weevil feeding, walking, grooming defecating, or mating predisposes it to higher levels of parasitoid attack and this therefore could be the reason for higher parasitism rates on the Italian grass. Conversely, Barker (1989) also showed that hybrid ryegrass (cv. Grasslands Manawa) is equally favored as a host by L. bonariensis as the Italian grass. In spite of this, the results here showed significantly less parasitism in the hybrid grasses than in the Italian grasses. This observation suggests that the intensity of weevil feeding and oviposition per se may not entirely be the reason for varied parasitism rates. Significantly, the growth habit of the hybrid grass is much closer to that of the diploids and neither of these ryegrass types support the same levels of leaf-feeding and oviposition as found in the Italian plants (Barker, 1989). It is also of interest that parasitism rates in grass-free control cages, while usually lower than in the cages with the grasses present, still showed substantial parasitism indicating that L. bonariensis remains susceptible to parasitism when not feeding or ovipositing.
The decline in parasitism in the hybrid and diploid grasses since that 1990s has not coincided with any sign of physiological resistance in the weevils. In spite of 1000s of weevils having been dissected by numerous workers since the introduction of the parasitoid, there has never been any observation of M. hyperodae early stages being encapsulated in L. bonariensis (e.g., Goldson et al., 2015).
Adaptive Implications
In general, the results in this study support the contention of Goldson et al. (2015) that if selection pressure has led to an enhancement of some kind of parasitoid-avoiding behaviors amongst L. bonariensis, then such evolution would most likely to have occurred in the country’s extensive diploid pastures rather than in the rare tetraploid Italian L. multiflorum pastures (B.R. Belgrave, Grasslanz Technology Ltd., pers. comm.).
Conclusion
It has been confirmed that different patterns of parasitism associated with different Lolium species and ploidy observed in the field also occurred in the laboratory experiments. At the same time, it has been demonstrated in the laboratory that diploid L. perenne and the diploid hybrid L. perenne ×L. multiflorum no longer support the levels of attack that were found in the 1990s. This is consistent with the contention that the weevil has evolved resistance to the parasitoid. The cause and mechanisms of this have yet to be determined; for example it is not known if there is a species or a ploidy effect, although field work has shown that parasitism levels in tetraploid L. perenne are no different from those in diploid L. perenne (Goldson et al., 2015). The possibility that resistance to a biological agent is dependent on plant type would seem to be unique in the literature.
There is now the prospect genetic and genomic analysis of both the weevils and parasitoid to explore further the underpinning of the observations in this contribution. By combining various approaches, the understanding of the reasons for success and failure in biological control must continue to develop (Mills and Kean, 2010).
Author Contributions
SG and FT conceived and designed the experiment. FT performed the analysis. SG and FT wrote the article with significant intellectual input from both authors. FT and SG conducted the experimental work described in the article with SG overseeing collection of further data used in Tables 1–3. Both authors contributed to the discussion and approved the final manuscript.
Conflict of Interest Statement
The authors declare that the research was conducted in the absence of any commercial or financial relationships that could be construed as a potential conflict of interest.
Acknowledgments
The authors gratefully acknowledge Shola Olaniyan for his participation in the establishment and running of this experiment. We received the expert technical and editorial advice from Alison Popay, Louise Winder, Scott Hardwick, Craig Phillips, Mark McNeill (all of AgResearch), and Steve Wratten (Lincoln University). Shaun Monk (Grasslanz) kindly provided seeds. Stephanie Hillis (student at Lincoln University) assisted with the field work. Morgan Shields (student at Lincoln University) contributed to the experiment establishment. The work was funded jointly by the Bio-Protection Research Center, Lincoln University and as part of an AGMARDT Postdoctoral Fellowship (Grant P15018 to F.T.). This paper is as part of a series of articles from the ninth Australasian Congress of Grassland Invertebrate Ecology (ACGIE) and received financial assistance for open access publication fees from ACGIE/Hawkesbury Institute for the Environment, Western Sydney University, Australia.
Supplementary Material
The Supplementary Material for this article can be found online at: http://journal.frontiersin.org/article/10.3389/fpls.2016.01259
References
Barker, G. M. (1989). Grass host preferences of Listronotus bonariensis (Coleoptera: Curculionidae). J. Econ. Entomol. 82, 1807–1816. doi: 10.1093/jee/82.6.1807
Barker, G. M., and Addison, P. J. (1996). Influence of clavicipitaceous endophyte infection in ryegrass on development of the parasitoid Microctonus hyperodae loan (Hymenoptera: Braconidae) in Listronotus bonariensis (Kuschel)(Coleoptera: Curculionidae). Biol. Control 7, 281–287. doi: 10.1006/bcon.1996.0095
Barker, G. M., and Addison, P. J. (1997). Clavicipitaceous endophytic infection in ryegrass influences attack rate of the parasitoid Microctonus hyperodae (Hymenoptera: Braconidae, Euphorinae) in Listronotus bonariensis (Coleoptera: Curculionidae). Environ. Entomol. 26, 416–420. doi: 10.1093/ee/26.2.416
Barker, G. M., and Addison, P. J. (2006). Early impact of endoparasitoid Microctonus hyperodae (Hymenoptera: Braconidae) after its establishment in Listronotus bonariensis (Coleoptera: Curculionidae) populations of northern New Zealand pastures. J. Econ. Entomol. 99, 273–287. doi: 10.1093/jee/99.2.273
Barratt, B. I. P., Evans, A. A., and Johnstone, P. D. (1996). Effect of the ratios of Listronotus bonariensis and Sitona discoideus (Coleoptera: Curculionidae) to their respective parasitoids Microctonus hyperodae and M. aethiopoides (Hymenoptera: Braconidae), on parasitism, host oviposition and feeding in the laboratory. Bull. Entomol. Res. 86, 101–108. doi: 10.1017/S0007485300052329
Bultman, T. L., McNeill, M. R., and Goldson, S. L. (2003). Isolate-dependent impacts of fungal endophytes in a multitrophic interaction. Oikos 102, 491–496. doi: 10.1034/j.1600-0706.2003.11477.x
Charlton, J. F. L., and Belgrave, B. R. (1992). “The range of pasture species in New Zealand and their use in different environments,” in Proceedings of the New Zealand Grassland Association, Gore, 99–104.
Charlton, J. F. L., and Stewart, A. V. (1999). “Pasture species and cultivars used in New Zealand-a list,” in Proceedings of the conference-New Zealand Grassland Association, Hawkes Bay, 147–166.
Gaynor, D. L., and Hunt, W. F. (1982). “The relationship between nitrogen supply, endophytic fungus, and Argentine stem weevil resistance in ryegrasses,” in Proceedings of the New Zealand Grassland Association, Blenheim, 267–263.
Gerard, P. J. (2000). “Ryegrass endophyte infection affects Argentine stem weevil adult behaviour and susceptibility to parasitism,” in Proceedings of the New Zealand Plant Protection Conference, ed. S. M. Zydenbos (Rotorua: New Zealand Plant Protection Society), 406–409.
Goldson, S. L. (1982). An examination of the relationship between Argentine stem weevil Listronotus bonariensis (Kuschel) and several of its host grasses. N. Z. J. Agric. Res. 25, 395–403. doi: 10.1080/00288233.1982.10417903
Goldson, S. L., and Emberson, R. M. (1981). Reproductive morphology of the Argentine stem weevil, Hyperodes bonariensis (Coleoptera: Curculionidae). N. Z. J. Zool. 8, 67–77. doi: 10.1080/03014223.1981.10427942
Goldson, S. L., and McNeill, M. R. (1992). “Variation in the critical photoperiod for diapause induction in Microctonus hyperodae, a parasitoid of Argentine stem weevil,” in Proceedings of the New Zealand Plant Protection Conference, ed. A. J. Popay (Wellington: New Zealand Plant Protection Society), 205–209.
Goldson, S. L., McNeill, M. R., Proffitt, J. R., Barker, G. M., Addison, P. J., Barratt, B. I. P., et al. (1993). Systematic mass rearing and release of Microctonus hyperodae (Hym.: Braconidae, Euphorinae), a parasitoid of the argentine stem weevil Listronotus bonariensis (Col.: Curculionidae) and records of its establishment in New Zealand. Entomophaga 38, 527–536. doi: 10.1007/BF02373087
Goldson, S. L., Proffitt, J. R., and Baird, D. B. (1998). Establishment and phenology of the parasitoid Microctonus hyperodae (Hymenoptera: Braconidae) in New Zealand. Environ. Entomol. 27, 1386–1392. doi: 10.1093/ee/27.6.1386
Goldson, S. L., Proffitt, J. R., Fletcher, L. R., and Baird, D. B. (2000). Multitrophic interaction between the ryegrass Lolium perenne, its endophyte Neotyphodium lolii, the weevil pest Listronotus bonariensis, and its parasitoid Microctonus hyperodae. N. Z. J. Agric. Res. 43, 227–233. doi: 10.1080/00288233.2000.9513423
Goldson, S. L., Proffitt, J. R., McNeill, M. R., Phillips, C. B., Barlow, N. D., and Baird, D. B. (2004). Unexpected Listronotus bonariensis (Coleoptera: Curculionidae) mortality in the presence of parasitoids. Bull. Entomol. Res. 94, 411–417. doi: 10.1079/BER2004314
Goldson, S. L., Tomasetto, F., and Popay, A. J. (2014a). Biological control against invasive species in simplified ecosystems: its triumphs and emerging threats. Curr. Opin. Insect Sci. 5, 50–56. doi: 10.1016/j.cois.2014.09.003
Goldson, S. L., Tomasetto, F., and Popay, A. J. (2015). Effect of Epichloë endophyte strains in Lolium spp. cultivars on Argentine stem weevil parasitism by Microctonus hyperodae. N. Z. Plant Prot. 68, 204–211.
Goldson, S. L., Wratten, S. D., Ferguson, C. M., Gerard, P. J., Barratt, B. I. P., Hardwick, S., et al. (2014b). If and when successful classical biological control fails. Biol. Control 72, 76–79. doi: 10.1016/j.biocontrol.2014.02.012
Johnson, L. J., de Bonth, A. C., Briggs, L. R., Caradus, J. R., Finch, S. C., Fleetwood, D. J., et al. (2013). The exploitation of epichloae endophytes for agricultural benefit. Fungal divers. 60, 171–188. doi: 10.1007/s13225-013-0239-4
Johnson, S. N., Gherlenda, A. N., Frew, A., and Ryalls, J. M. W. (2016). The importance of testing multiple environmental factors in legume-insect research: replication, reviewers and rebuttal. Front. Plant Sci. 7:489. doi: 10.3389/fpls.2016.00489
Kabacoff, R. (2011). R in Action: Data Analysis and Graphics with R. Greenwich, CT: Manning Publications Co.
McNeill, M. R., Goldson, S. L., Proffitt, J. R., Addison, P. J., and Phillips, C. B. (1999). “Selling parasitoids to the pastoral industry: a review of the commercial biological control programme targeting Listronotus bonariensis (Kuschel)(Coleoptera: Curculionidae),” in Proceedings of the 6th Australasian Applied Entomology Research Conference, ed. J. N. Matthiessen (Perth, WA: CSIRO Entomology, CSIRO Centre for Mediterranean Agricultural Research), 137–145.
McNeill, M. R., Goldson, S. L., Proffitt, J. R., Phillips, C. B., and Addison, P. J. (2002). A description of the commercial rearing and distribution of Microctonus hyperodae (Hymenoptera: Braconidae) for biological control of Listronotus bonariensis (Kuschel)(Coleoptera: Curculionidae). Biol. Control 24, 167–175. doi: 10.1016/S1049-9644(02)00018-X
Mills, N. J., and Kean, J. M. (2010). Behavioral studies, molecular approaches, and modeling: methodological contributions to biological control success. Biol. Control 52, 255–262. doi: 10.1016/j.biocontrol.2009.03.018
Pascoal, S., Cezard, T., Eik-Nes, A., Gharbi, K., Majewska, J., Payne, E., et al. (2014). Rapid convergent evolution in wild crickets. Curr. Biol. 24, 1369–1374. doi: 10.1016/j.cub.2014.04.053
Phillips, C. B. (2002). Observations of oviposition behavior of Microctonus hyperodae loan and M. aethiopoides Loan (Hymenoptera: Braconidae: Euphorinae). J. Hymenopt. Res. 11, 326–337.
Phillips, C. B., Barker, G. M., Roberts, R. L., McNeill, M. R., and Goldson, S. L. (1996). “Fecundity of wild and laboratory reared ecotypes of Microctonus hyperodae loan (Hymenoptera: Braconidae,” in Proceedings of the 49th New Zealand Plant Protection Conference, ed. M. O’Callaghan (Nelson: New Zealand Plant Protection Society), 285–290.
Popay, A. J., McNeill, M. R., Goldson, S. L., and Ferguson, C. M. (2011). The current status of Argentine stem weevil (Listronotus bonariensis) as a pest in the North Island of New Zealand. N. Z. Plant Prot. 64, 55–62.
R Development Core Team (2016). R: A Language and Environment for Statistical Computing. Vienna: R Foundation for Statistical Computing.
Wheeler, B. (2010). lmPerm: Permutation Tests for Linear Models. R Package Version 1.1-2. Available at: http://CRAN.R-project.org/package=lmPerm
Keywords: biological control of insect, decline, host plant effect, Lolium multiflorum, Lolium perenne, natural enemy, parasitism rate, pasture
Citation: Goldson SL and Tomasetto F (2016) Apparent Acquired Resistance by a Weevil to Its Parasitoid Is Influenced by Host Plant. Front. Plant Sci. 7:1259. doi: 10.3389/fpls.2016.01259
Received: 20 May 2016; Accepted: 08 August 2016;
Published: 23 August 2016.
Edited by:
Ivan Hiltpold, Western Sydney University, AustraliaReviewed by:
Carla Pinheiro, Faculdade de Ciências e Tecnologia da Universidade Nova de Lisboa, PortugalGary Michael Barker, Landcare Research, New Zealand
Copyright © 2016 Goldson and Tomasetto. This is an open-access article distributed under the terms of the Creative Commons Attribution License (CC BY). The use, distribution or reproduction in other forums is permitted, provided the original author(s) or licensor are credited and that the original publication in this journal is cited, in accordance with accepted academic practice. No use, distribution or reproduction is permitted which does not comply with these terms.
*Correspondence: Federico Tomasetto, ZmVkZXJpY290b21hc2V0dG9AZ21haWwuY29t