- 1Instituto de Biología Molecular y Celular de Plantas, Consejo Superior de Investigaciones Científicas, Polytechnic University of Valencia, Valencia, Spain
- 2Estación Experimental Agrícola Fabio Baudrit Moreno, Universidad de Costa Rica, Alajuela, Costa Rica
- 3Instituto de Hortofruticultura Subtropical y Mediterránea “La Mayora”, Consejo Superior de Investigaciones Científicas, University of Malaga, Algarrobo-Costa, Spain
- 4Departamento de Biología Aplicada, Escuela Politécnica Superior de Orihuela, Universidad Miguel Hernández, Orihuela, Spain
We have studied a genomic library of introgression lines from the Solanum pimpinellifolium accession TO-937 into the genetic background of the “Moneymaker” cultivar in order to evaluate the accession’s breeding potential. Overall, no deleterious phenotypes were observed, and the plants and fruits were phenotypically very similar to those of “Moneymaker,” which confirms the feasibility of translating the current results into elite breeding programs. We identified chromosomal regions associated with traits that were both vegetative (plant vigor, trichome density) and fruit-related (morphology, organoleptic quality, color). A trichome-density locus was mapped on chromosome 10 that had not previously been associated with insect resistance, which indicates that the increment of trichomes by itself does not confer resistance. A large number of quantitative trait loci (QTLs) have been identified for fruit weight. Interestingly, fruit weight QTLs on chromosomes 1 and 10 showed a magnitude effect similar to that of QTLs previously defined as important in domestication and diversification. Low variability was observed for fruit-shape-related traits. We were, however, able to identify a QTL for shoulder height, although the effects were quite low, thus demonstrating the suitability of the current population for QTL detection. Regarding organoleptic traits, consistent QTLs were detected for soluble solid content (SSC). Interestingly, QTLs on chromosomes 2 and 9 increased SSC but did not affect fruit weight, making them quite promising for introduction in modern cultivars. Three ILs with introgressions on chromosomes 1, 2, and 10 increased the internal fruit color, making them candidates for increasing the color of modern cultivars. Comparing the QTL detection between this IL population and a recombinant inbred line population from the same cross, we found that QTL stability across generations depended on the trait, as it was very high for fruit weight but low for organoleptic traits. This difference in QTL stability may be due to a predominant additive gene action for QTLs involved in fruit weight, whereas epistatic and genetic background interactions are most likely important for the other traits.
Introduction
Cultivated tomato, Solanum lycopersicum L., has undergone two domestication steps during its history (Blanca et al., 2012, 2015). The first domestication occurred early on in Ecuador and northern Peru, and was most likely carried out by ancient farmers on Solanum pimpinellifolium L. and/or S. lycopersicum var. cerasiforme. The second step most likely took place in Mesoamerica due to migrated pre-domesticated tomatoes. Spanish conquistadors brought the tomato from Mesoamerica to Europe, and from there it was spread all around the world. One consequence of this process was dramatic genetic erosion, caused especially by the bottleneck during the migration from the Andean regions to Mesoamerica. Early research (Williams and Clair, 1993) showed a much higher diversity in Andean S. pimpinellifolium and S. lycopersicum var. cerasiforme populations than among Mesoamerican cultivars, and this has been supported by recent high density single nucleotide polymorphism (SNP) variability analysis (Blanca et al., 2015). Therefore, accessions originating anywhere from the Andes to Mesoamerica will most certainly prove to be an important source of useful genetic diversity for tomato breeding.
After the introduction of tomato into Europe, intense breeding efforts were carried out in order to increase yield, adaptation, stability, and disease resistance (Bai and Lindhout, 2007). Despite these common objectives, the breeding goals changed over time due to the requirements of specific markets and uses. During the 1970 and 1980s, one of the most important breeding objectives, especially for fresh-market tomatoes, was to increase yield and shelf life. Both breeding objectives resulted in improved external quality, although at the expense of internal fruit quality. During the following decade, taste became the main breeding objective. Sugars, acids and more than 30 volatile compounds are known to influence tomato flavor (Tieman et al., 2012; Rambla et al., 2014). Organoleptic quality is a very complex trait as it depends on the evolving preferences of the market. Even so, significant improvement in tomato flavor seems possible by increasing the fruit sugar and acid contents and by modifying the balance between the two (Stevens et al., 1977). Wild species have mostly been used to introduce resistance genes, thus increasing the genetic diversity of modern cultivars compared to vintage cultivars (Sim et al., 2009, 2011, 2012).
The complex polygenic control of tomato fruit quality traits involves multiple quantitative trait loci (QTLs; Labate et al., 2007). Interestingly, favorable effects on fruit quality have been identified in wild species, such as S. pimpinellifolium, Solanum pennellii, Solanum cheesmaniae, and Solanum habrochaites (Eshed and Zamir, 1995; Bernacchi et al., 1998; Monforte et al., 2001; Fulton et al., 2002; Lippman et al., 2007), even though the fruits of these species are not usually consumed by humans. The exploitation of these QTLs in practical breeding has been very limited because of the inherent difficulties in implementing marker-assisted selection (MAS) for QTLs (Collard and Mackill, 2008) in addition to deleterious linkage drag. Nevertheless, a few successful studies have been reported (Gur and Zamir, 2004).
Both advanced backcross and introgression lines (ILs) may be used to facilitate the incorporation of genetic variability from wild species (Eshed and Zamir, 1995; Tanksley et al., 1996). ILs are developed by MAS and contain a unique chromosome fragment from a donor genotype (usually a wild species or unadapted germplasm) in a uniform elite genetic background. These collections are also called “genomic libraries of ILs” when the whole genome of the donor genotype is represented among the introgressions.
In tomato, ILs have been developed from S. pennellii LA0716 (Eshed and Zamir, 1994), S. habrochaites LA1777 (Monforte and Tanksley, 2000a), Solanum lycopersicoides LA 2951 (Chetelat and Meglic, 2000; Canady et al., 2005), S. habrochaites LA0407 (Francis et al., 2001) and S. habrochaites LYC4 (Finkers et al., 2007). In addition, a small number of ILs have been developed for the S. pimpinellifolium accessions LA1589 (Tanksley et al., 1996; Bernacchi et al., 1998) and LA2093 (Kinkade and Foolad, 2013). IL collections are extremely useful for identifying QTLs (Eshed and Zamir, 1995; Rousseaux et al., 2005), verifying QTL effects (Tanksley et al., 1996), studying QTL x environmental, QTL x genetic background and QTL x QTL interactions (Monforte et al., 2001), QTL fine mapping (Eshed and Zamir, 1996; Ku et al., 2000; Monforte and Tanksley, 2000b; Ashrafi et al., 2012) and introducing new genetic variability from wild species into elite germplasm (Tanksley and McCouch, 1997; Zamir, 2001; Gur and Zamir, 2004). IL analysis is also a powerful tool for genomics research, as it facilitates the study of the genetic basis of metabolome (Schauer et al., 2006), transcriptome and its correlation with metabolome (Lee et al., 2012), enzyme activity (Steinhauser et al., 2011) and QTL cloning (Frary et al., 2000; Fridman et al., 2000; Liu et al., 2002). The most widely used IL collection is the S. pennellii LA 0716 collection, which has facilitated the identification of more than 2,700 QTLs involved in agronomical important characters (Lippman et al., 2007).
The development of IL collections has traditionally required intense effort spanning several years (Eshed and Zamir, 1994; Eduardo et al., 2005). Barrantes et al. (2014) applied high-throughput genotyping in an IL breeding program, demonstrating that IL libraries can now be produced with far less effort at a lower cost. The IL population thus produced was derived from a cross between the cultivar Moneymaker (S. lycopersicum) and the S. pimpinellifolium accession TO-937. This accession is from Peru, i.e., the region where the tomato most likely underwent its first domestication step. It is therefore a quite suitable accession for introducing new genetic variability into the cultivated tomato genetic pool. Previous works have demonstrated that TO-937 harbors genetic variability that is of interest for breeding purposes, such as enhancing ascorbic acid (Lima-Silva et al., 2012), sugar, organic acid and carotenoid fruit content (Capel et al., 2015), as well as modifying aroma volatile compounds (Rambla et al., 2014) and resistance to pests (Fernández-Muñoz et al., 2000; Silva et al., 2014). Furthermore, populations derived from this accession were used to map the Uniform ripening (U) locus (Powell et al., 2012). In the current report, we present a thorough phenotypic characterization of this IL library, focusing mainly on fruit traits and the characterization of QTLs involved in fruit quality as the first step in introducing new genetic variability into the elite tomato gene pool.
Materials and Methods
Plant Material
A complete genomic library of 54 ILs derived from a cross between the wild S. pimpinellifolium (SP) accession TO-937 as a donor parent, obtained from the Instituto de Hortofruticultura Subtropical y Mediterránea “La Mayora” (IHSM-UMA-CSIC) germplasm bank, and the cultivar “Moneymaker” (S. lycopersicum) as recurrent parent (hereafter referred to as MM, Barrantes et al., 2014) were studied in the current report. In brief, each IL contains an average of 3.7% of the SP genome (range: 0.5–7.7%), altogether covering 98.8% of the donor parent genome, with an average introgression size of 25 Mb (ranging from 0.7 to 75 Mb). IL evaluation was performed during the spring-summer of 2013 at three locations in Spain: Alginet, Valencia (Agricultural Cooperative Alginet Coagri), School of Engineering of Orihuela, Alicante (Miguel Hernández University) and Algarrobo-Costa, Málaga (IHSM-UMA-CSIC). All three locations are on the Mediterranean coast of Spain, and, therefore, have similar weather conditions. ILs were grown in plastic greenhouses following a randomized complete block design with eight blocks, each containing one replicate per IL and six replicates of MM. In the first through seventh blocks, each replicate had a single plant, whereas in the eighth block each replicate consisted of three plants. The eighth block was used to better distinguish categorical traits between the ILs and MM.
Phenotypic Analysis
Traits were classified into two categories: descriptive and quantitative traits. Descriptive traits were only evaluated in block 8, as each replicate consisted of three plants, which made it easier to observe the differences between the ILs and MM categorically. This group of traits included: vigor (VIG), as the height of the plant at first fruit set expressed in cm; purple (PURP), as the presence or absence of anthocyanin coloration in branches and stems; trichomes (TRI), visually observed long trichome density on stems with a scale of: 0 = absence, 1 = low, 2 = medium, and 3 = high; and earliness (EAR), as days from transplanting to first ripe fruit and presence/absence of dark green shoulder (GS) on breaker fruit. Quantitative fruit traits were evaluated on four fruits harvested at light-red stage, selected from a large sample of fruits for being the most representative as regards homogeneity in maturity and size. A single sample per plant was obtained for blocks 1–7, whereas a pooled sample of three plants was obtained in block 8. Each fruit was weighted (FW in grams) and the external color (EC) was recorded at three points in the equatorial region of the tomato fruit using a Minolta Chroma Meter model CR-400 (Konica Minolta, Inc., Tokyo, Japan), applying the CIE Lab color space, where higher +a∗ indicates red and lower -a∗ indicates green, whereas higher b∗ indicates yellow and lower b∗ green. The color space is three-dimensional, where the third axis, L∗, represents black to white and the a∗-b∗ plane may be visualized as a color wheel that is lighter or darker depending on the level of L∗. Lower L∗ values represent a darker color. Chroma (C∗), a measure of color saturation, was calculated using the formula: (a∗2+b∗2)1/2. Hue-angle (H), in degrees, is the measurement of an object’s color in the a∗-b∗ plane and was calculated as (180/p)∗cos-1(a∗/C∗) for the positive values of b∗ obtained. Perception of hue angle differences depends on the chroma, with the differences being more detectable at higher chroma (Sacks and Francis, 2001). After longitudinal cutting, fruits were scanned at 300 dots per inch (dpi). The images were saved as jpeg files and imported into Tomato Analyzer 3.0 software for automated phenotypic analysis1. Fruit morphology descriptors were following Brewer et al. (2006): maximum diameter (FD, in cm), max length (FL, in cm) fruit shape: fruit shape index (FS), fruit shape circular (CIR), shoulder height (PSH, that is a measurement of the indentation of peduncle scar at the proximal end of the fruit). For internal color (IC), the Tomato Analyzer color module was calibrated with a scanned X-Rite Color Checker card. Images were previously processed with Photoshop CS5 v. 12. (Adobe Systems Incorporated, San Jose, CA, USA) in order to save an image of the same fragment of pericarp tissue in each fruit. Finally, CIELab color parameters were obtained using Tomato Analyzer (Darrigues et al., 2008). The organoleptic traits, such as soluble solid content (SSC), pH (PH), and titratable acidity (TA) were analyzed from tomato pericarp tissue from four fruits ground and stored at -20°C. Samples were thawed and centrifuged at 3500 rpm for 10 min, an aliquot of supernatant was used for measuring SSC (expressed in °Brix) using a digital refractometer (Atago CO LTD, Tokyo, Japan) and PH and TA (expressed in percentage of citric acid) were determined from 1 ml of the supernatant homogenized juice with the electronic analyzer PH-Matic23 (CRISON, Barcelona, Spain).
Statistical Analyses
For each trait, the genetic (G), location (L) and interaction (G-x-L) effects were estimated by two-way ANOVA. Heritability was estimated by one-way ANOVA in each locality (G-x-L was significant for nearly all traits, see below) as h2= Vg/Vt (where Vg represents genetic variance, estimated as the variance among genotypes, and Vt represents total variance). Pearson’s correlation coefficients among traits were calculated in each location. IL and control MM means were compared by a Dunnet’s test at p < 0.05. Only ILs that were significantly different from MM in at least two locations were considered for QTL assignment. QTLs were mapped in the chromosome regions that were covered by the TO-937 introgressions in the ILs that showed significant effects on the trait under study. In those cases where the means of two ILs with overlapping introgressions were significantly different from MM, a contrast test was performed between those ILs. When IL means were not different, the QTL was assumed to be located in the overlapping regions; when the means were different, two QTLs were assumed. All statistical analyses were performed with JMP v. 11 (SAS Institute, Cary, NC, USA).
Results
Recurrent Parent (MM)
The trait means and standard deviations of parental MM at the three locations are presented in Supplementary Table S1. No significant differences in FW were found between locations, with the average being 98.1 g, whereas other fruit dimension traits such as FL and FD showed significant differences (p ≤ 0.01), with higher values in Málaga. Shape-related traits showed statistically significant differences among locations (p ≤ 0.01), except for FS. Significant differences for organoleptic traits (SSC, PH, and TA) and color traits (IC and EC) were also found among locations.
Introgression Lines and QTL Mapping
In general, the phenotypes of both plant and fruit were very similar to the phenotypes of the recurrent MM. Only in very few cases did we observe extreme phenotypes. A thorough description of the phenotypes and the QTL mapping can be found below. In Supplementary Table S2, a summary of the comparison of each IL with MM across all locations is also shown.
Descriptive Traits
During the cultivation of the IL collection, several phenotypic characteristics that were consistent in at least two locations were clearly observed (Supplementary Figure S1). ILs SP_2-4 and SP_2-5 showed fast vegetative growth, defining a locus for plant vigor (vig2.1). SP_3-1, on the other hand, suffered such a drastic reduction of plant growth that it did not set fruits in two locations, which, in turn, permitted to define another locus (vig3.1). Furthermore, SP_5-2 and SP_5-3 displayed purple shoots, probably due to anthocyanin accumulation (purp5.1). SP_10-3, meanwhile, had denser trichomes (tri10.1) than MM. IL_2-5 set the first ripe fruit 8 days earlier than MM (ear2.1), whereas, IL_11-4 produced the first ripe fruit 8–13 days after MM (ear11.1). IL_10-1 and IL_10-2 had fruits with dark GSs at the breaker stage (gs10.1).
Fruit Size
The average FW of the whole IL collection across trials was 11.3% lower than MM (around 98 g per fruit, Table 1). The range was between 47.81 and 117.86 g (SP_3-3 and SP_12-5, respectively). FW was strongly correlated with its trait components: fruit length (FL) and fruit diameter (FD; r > 0.5, Supplementary Table S3). The effect of the genotype (G) was 35% of total variance; whereas the effects of the location (L) and the interaction (G-x-L) were lower than G effects, 19 and 5%, respectively (Table 1). FW was the trait with higher heritability (h2 range: 0.62–0.45, Supplementary Table S4). As a general rule, introgressions from SP had a negative effect on FW, except for SP_12-5, which increased FW 20% (Figure 1; Supplementary Figure S2). Three ILs (SP_1-2, SP_2-5, and SP_3-3) showed the most consistent effects among locations, and, on average, reduced FW by up to more than 40% in some locations. Fourteen additional ILs with consistent effects on FW allowed us to define a total of 12 QTLs: fw1.1, fw1.2, fw2.1, fw2.2, fw3.1, fw3.2, fw4.1, fw4.2, fw7.1, fw10.1, fw11.1, and fw12.1 (Table 2).
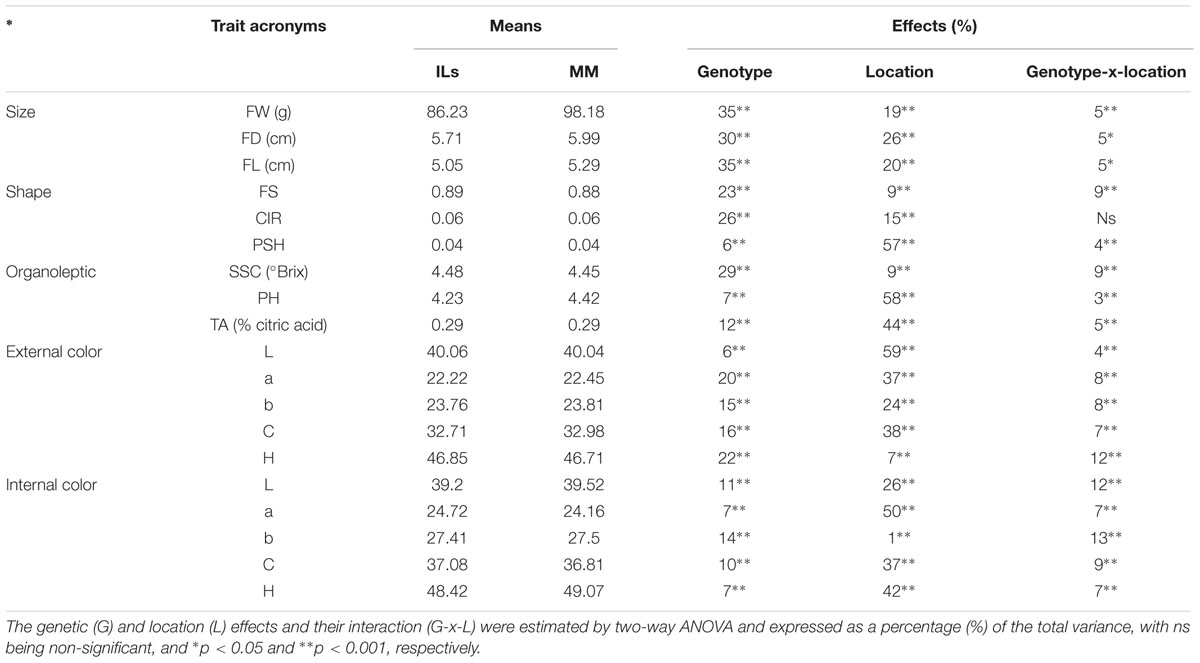
TABLE 1. Trait mean values [fruit weight (FW), diameter (FD), length (FL), shape (FS), circular shape (CIR), shoulder height (PSH), soluble solid content (SSC), pH (PH), titrable acidity (TA)] and the internal and external CIELab color system variables L∗, a∗, b∗, C∗ and H for the whole Introgression Line (IL) collection and the recurrent parent Moneymaker (MM).
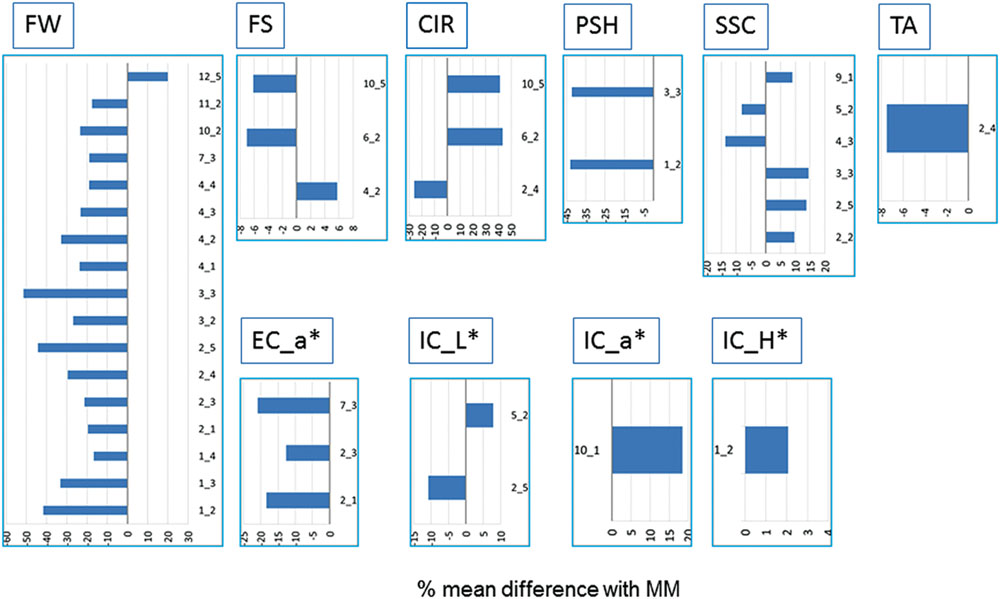
FIGURE 1. Differences between IL means and Moneymaker (MM) across the three locations expressed in percentage (%) from the MM mean. Only ILs showing significant mean differences with MM at p < 0.05 according to the Dunnet’s test in at least two locations are shown. Trait abbreviators are: fruit weight (FW), shape (FS), circular shape (CIR), shoulder height (PSH), soluble solid content (SSC), titrable acidity (TA) and internal and external color (EC; ICOL, ECOL) based on CIELab color system variables a∗, b∗, L∗, H, C∗.
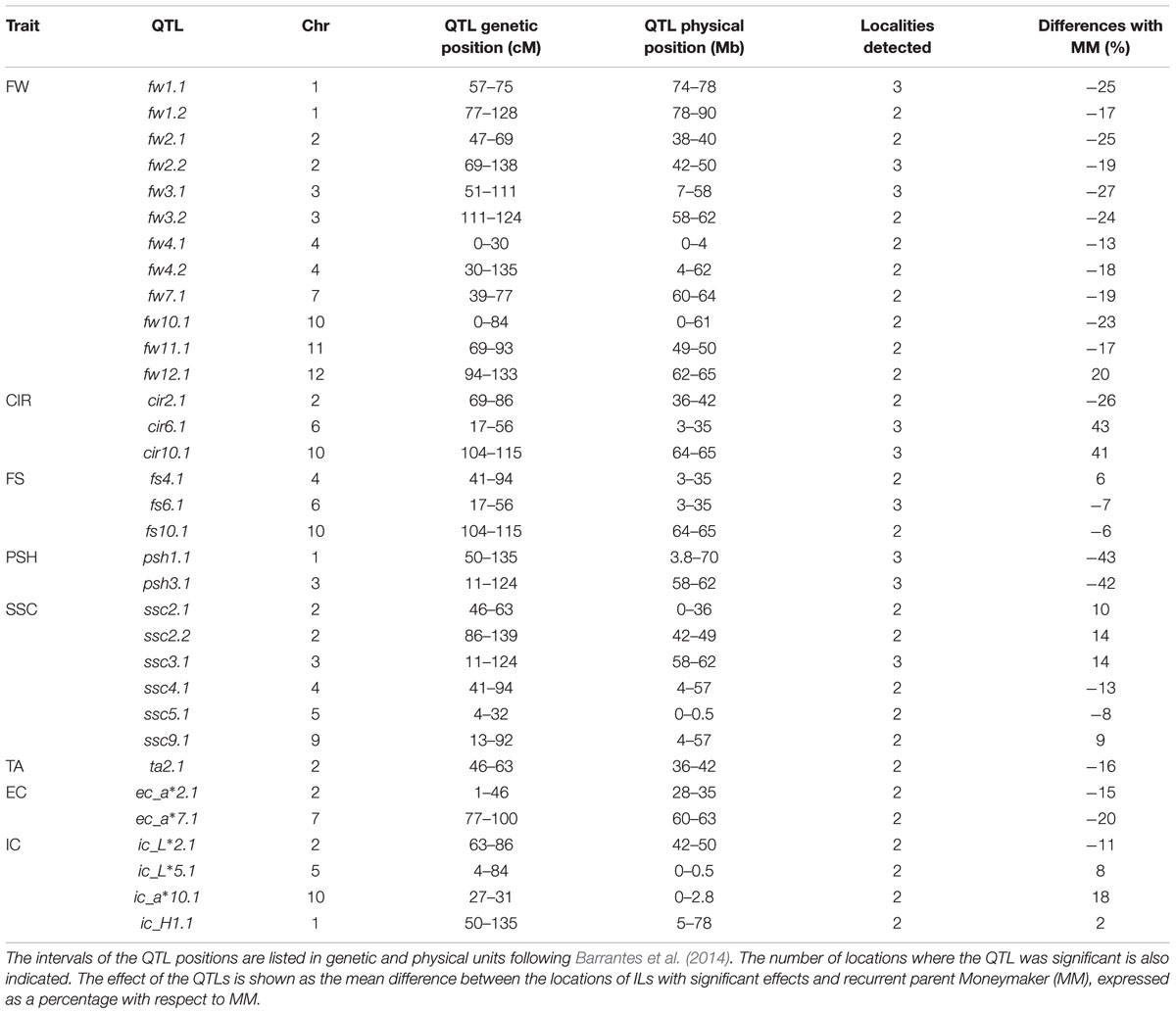
TABLE 2. Summary of the quantitative trait loci (QTLs) detected in the population of introgression lines for the analyzed traits [FW, FS, CIR, PSH, soluble solid content (SSC), TA, external (EC) and internal (IC) color the last two are based on CIELab color system variables L∗, a∗, b∗, C∗, H].
Fruit Shape-Related Traits
The fruit shape index (FS) showed a relatively modest variability in the IL collection, as the fruits were nearly round, just like MM (both the IL population and MM had the same mean value of FS = 0.89), ranging from 0.82 (SP_6-2) to 0.93 (SP_4-2), being the G effect 23% of total variance (Table 1). FS was negatively correlated with FD (r = -0.35, Supplementary Table S3) and positively with FL, but to a lesser extent (r = 0.27), indicating that FD is the most important determinant of the variation in FS in this population. Heritability ranged from 0.48 to 0.29 (Supplementary Table S4). SP_6-2, SP_4-2, and SP_10-5 showed consistent effects on FS. Three QTLs were defined with opposite effects: fs6.1 and fs10.1, which induced flattened fruits, and fs4.1, which induced more elongated fruits (Figure 2; Table 2).
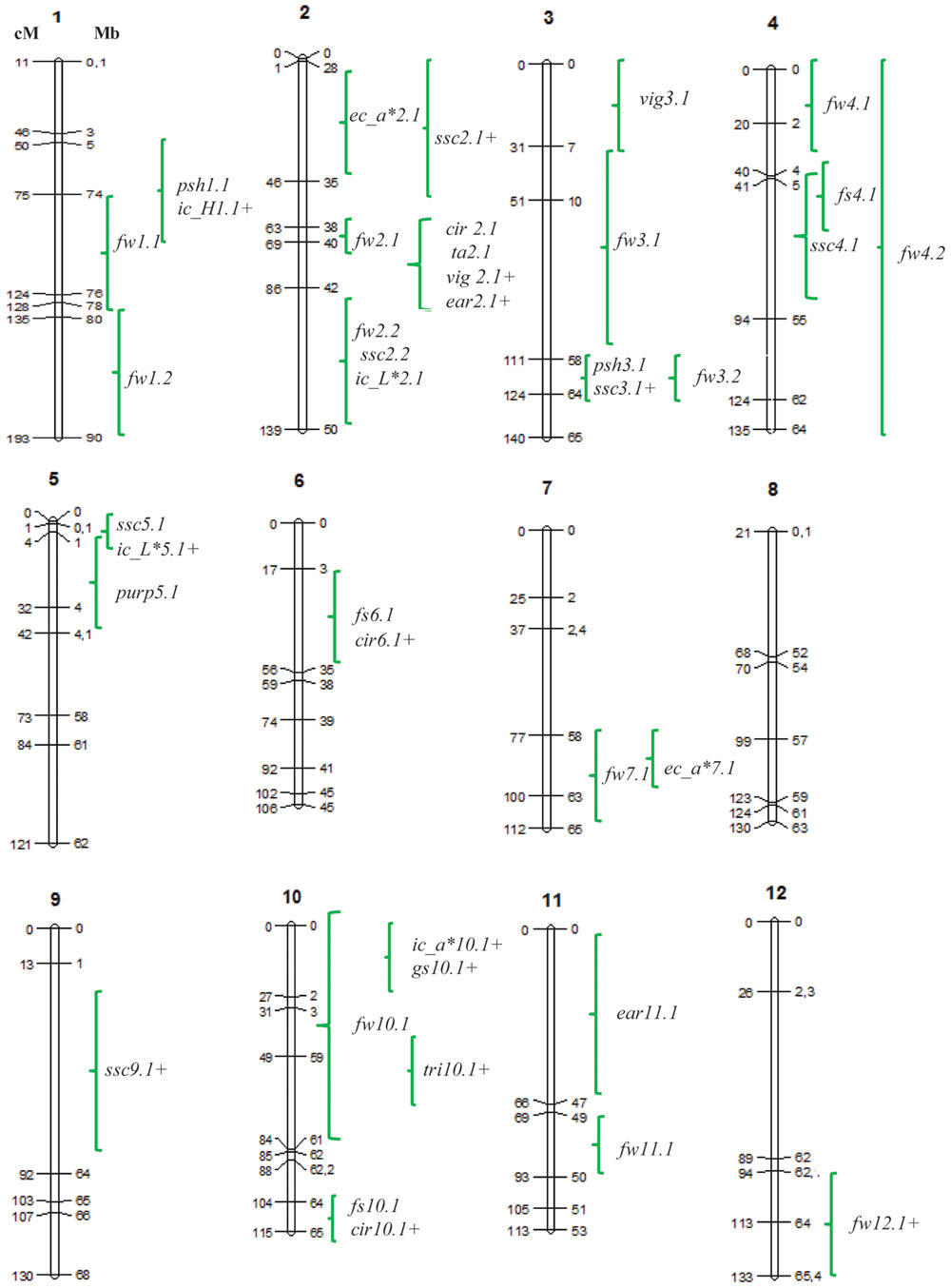
FIGURE 2. Quantitative trait loci (QTL) positions on the tomato map. Genetic distances (cM) are shown on the left of the chromosome drawings, and physical distances (Mb), according to the tomato genome version SL2.40, on the right. QTLs are named using an abbreviation of the trait: plant vigour (VIG), earliness (EA), presence or absence of anthocyanin coloration in branches and stems (PURP); trichome density (TRI), green shoulder on the fruit (GS), FW, FS, circular shape (CIR), shoulder height (PSH), soluble solid content (SSC), TA and internal and EC (ICOL, ECOL, respectively) based on CIELab color system variables a∗, b∗, L∗, H, C∗, followed by the chromosome on which they map and a digit indicating the number of the QTL within the chromosome. (∗) Indicates that the TO-937 wild allele increases the trait value.
The variability of circular fruit shape (CIR) was also low, being G effect 26% of total variance (Table 1), with an average value of 0.06 for the IL collection (the same value as MM, Table 1), ranging from 0.04 to 0.08 (SP_2-5 and SP_10-5, respectively). CIR clearly showed a high correlation (Supplementary Table S3) with FD (r = 0.48) but not with FL (r = -0.02), which supports the previous observation that FD is the principal determinant of FS variability in this population. Heritability was similar to FS, ranging from 0.47 to 0.35 (Supplementary Table S4). Additionally, SP_6-2 also increased CIR, making a total of three QTLs: cir2.1 induced up to 26% rounder fruit, while cir6.1 and cir10.1 induced 42% less round fruit on average (Figure 2; Table 2).
PSH averages were the same for both the ILs and MM (0.06), with a very low G effect and the L effect being the most important one, 6 and 27% of total variance, respectively (Table 1). Heritability was also a little bit lower than previous fruit shape traits, ranging from 0.30 to 0.35 (Supplementary Table S4). Despite the low G effect, ILs SP_1-2 and SP_3-3 showed statistically significant differences as compared to MM in all three locations, reducing PSH by as much as 42% (Table 2). Two stable QTLs were defined as a result: psh1.1 and psh3.1 (Figure 2).
Organoleptic Related Characters
The average SSC in the IL collection was similar to that of MM (4.48 ° Brix), although the range was wide, from 3.85° to 5.1 Brix° (SP_4-3 and SP_10-6, respectively). The G effect was 29% of total variance, whereas L effect and G-x-L interaction represented 9% of total variance both of them (Table 1). Interestingly, correlations between SSC and fruit size and other fruit morphology-related traits were very low and non-significant (r < 0.1, Supplementary Table S3). Heritability was near 0.5 (Supplementary Table S4). SP_3-3 increased SSC in all three trials, whereas four additional ILs increased it in two trials (Figure 1; Table 2). On the other hand, SP_4-3 and SP_5-2 decreased SSC by 13 and 8%, respectively. A total of six consistent QTLs were defined with opposite effects: ssc2.1, ssc2.2, ssc3.1, and ssc9.1 increased SSC by up to 13%, whereas ssc4.1 and ssc5.1 decreased SSC by up to 12% (Table 2; Figure 2).
The average TA and PH of the fruits of the IL collection were similar to those of MM (0.29 and 4.6, respectively). For both traits, the G effect was 12%, whereas L effect was the most important (44%, Table 1), indicating a low genetic variability for this trait in the current population. Correlations with other traits were generally low, except for SSC, which was relatively important (r = 0.44, Supplementary Table S3). Heritability ranged from 0.2 to 0.48 (Supplementary Table S4). Only SP_2-4 showed a significant TA decrease of 17% compared with MM in all three trials, defining the QTL ta2.1 on chromosome 2 (Figures 1 and 2; Table 2).
Fruit Color
External color average values were very similar between the ILs and MM. The G effect was low for L∗, b∗ and C ∗ (6–16%), moderate for a∗ and H (>20%, Table 1), and the L effect was important in most of them (>24% of total variance, p < 0.001). Meanwhile, interaction G-x-L was generally lower than 12% of total variance. EC positively correlated moderately with fruit size (r∼0.2, p = 0.004, Supplementary Table S3) and negatively with the organoleptic traits SSC and TA (r < 0.4, p = 0.004). Heritability ranged from 0.23 to 0.55 (Supplementary Table S4). Three ILs, SP_2-1, SP_2-3 and SP_7-3, showed significant differences in the a∗ color component, defining the QTLs ec_a∗2.1 and ec_a∗7.1 (Figure 1), associated in both cases with a reduction of the color red (Figure 2; Table 2).
Internal color average values were very similar between the ILs and MM, except for the components C∗ and H, which were slightly higher or slightly lower in the IL collection, respectively. The G effects represented between 7 and 14 of the total variance, being the L effect was higher in most cases (>26%), with the exception of b∗ (Table 1). The interaction G-x-L for IC components ranged from 7 to 13% of total variance (Table 1). No high correlation values between IC and the other traits were detected (Supplementary Table S3). ILs SP_1-2, SP_5-2, SP_10-2 increased the H, L∗ and a∗ components, respectively, whereas SP_2-5 decreased L∗ (Figure 1). Heritability ranged from 0.23 to 0.44 (Supplementary Table S4). A total of four QTLs were defined: ic_a∗2.1 and ic_a∗10.1, associated with an increased red-colored fruit, and ic_H1.1 and ic_L∗5.1, which reduced red coloration (Figure 2; Table 2).
In summary, a total of 33 QTLs with consistent effects in at least two locations were defined (Figure 2). These QTLs were mapped and covered different regions in all chromosomes, except chromosome 8, where no QTL was detected. The distribution of QTLs was not homogeneous among chromosomes, with the largest number of QTLs (8) being found in chromosome 2. Chromosomes 1, 3, 4, and 10 had four QTLs and the rest of the chromosomes showed fewer QTLs.
Discussion
QTLs Detected in the IL Population
To the best of our knowledge, the current work reports the first thoroughly evaluated IL collection with an S. pimpinellifolium accession as donor. SP is the phylogenetically closest wild species to the cultivated tomato, which could explain why, in general, the phenotypes of the ILs were so similar to MM, especially compared with other IL collections derived from more distant wild species (Eshed and Zamir, 1995; Bernacchi et al., 1998; Monforte et al., 2001). As a result, deleterious linkage drag effects are minimized in this collection, which facilitates the exploitation of this genetic resource in plant breeding. Furthermore, MM is a fresh-market variety, in contrast to the processing cultivars more widely used as genetic background (Eshed and Zamir, 1995; Monforte and Tanksley, 2000a).
A few extreme phenotypes were observed in the population, some of which affected general plant growth and architecture. SP_3-1 showed severe plant growth impairment, limiting fruit production drastically. This phenotype is very similar to the one caused by the pauper mutation, which maps on the short arm of chromosome 3 (tgrc.ucdavis.edu), so vig3.1 could represent an allele of the pau gene. As neither MM nor TO-937 showed any growth impairment, it is unlikely that a new mutation on pau would have occurred during IL development. We think that the effect can most likely be attributed to interactions between TO-937 alleles (pau or other genes) within the SP_3-1 introgression and other genes in MM genetic background. Another classical mutant, pro or procera (tgrc.ucdavis.edu), which exhibits a more rapid growth rate, produces tall, slender, weak plants and elongated internodes, and co-locates with the earliness QTL ear11.1 in IL SP_11-4.
SP_5-2 and SP_5-3 displayed the color purple on both primary and axillary shoots, indicating anthocyanin over-accumulation. The Af gene (anthocyanin free, tgrc.ucdavis.edu) is involved in the accumulation of anthocyanins as mutations (af) in this gene, and produces plants that do not accumulate anthocyanin in all tissues (Burdick, 1958). This gene is located in the short arm of chromosome 5 and encodes a chalcone isomerase enzyme (CHI) that catalyzes the synthesis of 2(S)-naringenin, a key intermediate in the flavonoid pathway, which is required for flavonoid production (Kang et al., 2014) This means that purp5.1 may be allelic to Af. As in the previous example, neither MM nor TO-937 displayed anthocyanin accumulation under normal non-stress conditions, suggesting that epistatic interactions between TO-937 alleles at CHI and with MM genetic background likely induced an expression of CHI, which would explain the resulting anthocyanin accumulation.
SP_10-3 displayed a much higher trichome density than MM, with long type-I trichomes (Luckwill, 1943) densely covering leaves and stems. Yang et al. (2011) isolated the Woolly gene (Wo), essential for trichome formation in all vegetative parts. This gene is located in the long arm of chromosome 2, meaning that SP_10-3 most likely contains a novel gene involved in trichome development. Trichomes have been associated with insect resistance (Guo et al., 1993; Blauth et al., 1998; Maluf et al., 2010). Salinas et al. (2013) mapped two QTLs in chromosome 2 from the wild tomato TO-937 that control resistance against the two-spotted spider mite (Tetrany chusurticae Koch) and which is based on short, glandular, type-IV trichomes that produce acylsucroses (Alba et al., 2009). However, no gene or QTL involved in insect resistance has been mapped in chromosome 10 so far, which suggests that the increase of trichome density induced by tric10.1 is not related to disease resistance. Since the h gene (hairs absent, tgrc.ucdavis.edu) produces absence of long trichomes (except on hypocotyl) and is located in the long arm of chromosome 10, it is likely that tric10.1 is allelic to h. No candidate gene is known for this gene.
Regarding fruit quality traits, in general no important deleterious phenotypes were observed. SP_10-1 and SP_10-2 yielded dark green-shouldered fruits as a consequence of the allelic replacement of the uniform ripening (u) gene that is located in the introgressions presented by the ILs and which corresponds to the reconstitution of the function of the GLK-2 gene involved in chloroplast development in fruit (Powell et al., 2012).
Fruit weight showed the highest genetic variability and heritability as well as the largest number of detected QTLs. The striking phenotypic differences between parental lines explain these results. The increase in FW is an important domestication and diversification trait. Major QTLs involved in this trait have been isolated in the last few years (Monforte et al., 2014), and some QTLs detected in the current report provide additional evidence for them: fw2.2 (Frary et al., 2000), fw3.2 (Chakrabarti et al., 2013) FAB, FIN (Xu et al., 2015), which are most likely responsible for the FW QTLs reported herein in chromosomes 2, 3, 4, and 11, respectively. These QTLs are involved in either domestication or improvement (Lin et al., 2014), together with other loci in chromosomes 5, 7, 9, and 12. The current population revealed additional FW QTLs on chromosomes 1 (SP_1-2, SP1_3, and SP_1-4) and 10 (SP_10-2), with effects of a comparable magnitude (Figure 1) to those previously described, even though they have not been previously associated to either domestication or improvement. These QTLs have not been found in other mapping populations derived from SP accessions, although they may be orthologous to FW QTLs from other wild tomato species, such as S. pennellii (Eshed and Zamir, 1995). Also worthy of note are the transgressive effects of IL_12-5 that increase FW. QTLs involved in FW have been mapped in this region, either increasing (Causse et al., 2004) or decreasing (Fulton et al., 2000; Prudent et al., 2009; Xu et al., 2013), indicating that there must be allelic variability for this QTL in the wild species germplasm that was probably not selected during domestication into the cultivated gene pool.
With respect to FS, the genetic variability observed in the current IL population was modest. At the same time, the number of detected QTLs was small and their effects were low. The QTL cir2.1, responsible for 26% of fruit round shape variability likely corresponds to QTLs detected in previous SP-derived populations (Grandillo and Tanksley, 1996; Tanksley et al., 1996; Bernacchi et al., 1998; van der Knaap and Tanksley, 2003). The physical position coincides with ovate, so cir2.1 could be a weak allele of the ovate gene (Liu et al., 2002). QTLs cir6.1 and cir 10.1, mapped in chromosomal regions previously associated with FS on chromosomes 6 (Bernacchi et al., 1998; van der Knaap and Tanksley, 2003) and 10 (Chen et al., 1999) in SP-derived populations, are most likely allelic QTLs.
Shoulder height QTLs were mapped for the first time by Brewer et al. (2007) in chromosomes 1, 2, and 7. In the current study, psh1.1 and psh3.1 were mapped, with psh1.1 located at the same position previously defined by Brewer et al. (2007) on chromosome 1. The appearance of shoulder height in tomato fruit is restricted to cultivated tomato, which is probably a consequence of domestication. It seems unlikely that ancient farmers would have selected for this trait intentionally. One possible explanation is a pleiotropic effect of fruit size increase; in fact, SP_1-2 and SP_3-3 decreased both FW and PSH, although other ILs that reduced FW did not show any effects on PSH. An alternative explanation is that the pleiotropic effect could be QTL-specific. SSC is one of the primary quality traits of tomato fruits. The amount of genetic variability in the current population was higher than it was for FS, with a total of five QTLs with opposite effects being detected (three increasing, two decreasing). No correlation was found between SSC and FW. SP_2-2 and SP_9-1 increased SSC but did not decrease FW, making those QTLs an appropriate choice for increasing SSC without negative effects on FW. QTLs on ILs SP_3-3, SP_4-3, and SP_5-1 have been detected in a very limited number of previous works (Tanksley et al., 1996; Chen et al., 1999), whereas QTLs on SP_2-2, SP_2-5, and SP_9-1 have been detected more frequently (Grandillo and Tanksley, 1996; Tanksley et al., 1996; Chen et al., 1999; Doganlar et al., 2002; Causse et al., 2004; Chaïb et al., 2006; Xu et al., 2013; Pereira da Costa et al., 2013). The QTL on SP_2-5 is likely a pleiotropic effect of fw2.2 (Frary et al., 2000), whereas the effect on SP_9-1 could be due to the apoplastic invertase Lin5 (Fridman et al., 2004). This difference in SSC QTL detection among different mapping populations most likely reflects a high genetic variability for this trait in both cultivated and wild germplasm.
Fruit color is also an important quality trait as it is associated with lycopene accumulation. Both EC and IC components showed a moderate genetic variance in this population, indicating low allelic diversity between MM and TO-937. Correlations between EC and IC components were low and non-significant, confirming a different genetic control for these traits (Monforte et al., 2001). All EC QTLs from TO-937 reduced red coloration, an unexpected result since TO-937 fruits are redder than MM fruits. On the other hand, SP_1-2, SP_2-5, and SP_10-1 displayed a more intense red IC, whereas SP_5-2 showed a diminished red IC, which makes those first ILs promising for the improvement of the nutritional quality of tomatoes. Phytoene synthase 2 (psy2) is located in the SP_2-5 introgression (Bartley and Scolnik, 1993), and is a strong candidate gene. QTLs for IC have been detected previously in the same genomic region, which expands the introgression of SP_1-2 (Grandillo and Tanksley, 1996; Bernacchi et al., 1998; Liu et al., 2003). Since the high pigment-2 (hp-2) locus maps in the same region (van Tuinen et al., 1997), it is likely that the current QTL is an allele of hp-2 with weaker effects. The loci on SP_5-2 and SP_10-1 are the most promising ones, although the introgressions still harbor a large number of genes that may turn out to be candidate genes.
Comparison of QTL Detection between RIL and IL Populations
A RIL population derived from the same parents as the current IL library was recently used to map QTLs involved in FW, SSC, TA and PH, among other traits (Capel et al., 2015), which gave us the opportunity to assess the effect of the genetic structure of the mapping population on QTL detection. In general, the fruits of the RIL population were smaller than those of the IL population, which was probably due to the accumulation of FW QTLs from SP in the RIL genomes. However, FW showed a similar range of phenotypic variation to the ILs in absolute values for FW (1.51–58.24 g among RILs, compared to 47.81–117.86 g among the ILs), while a higher range was observed for SSC, TA, and PH among RILs than the ILs. These differences in the range of variation suggest that additive gene action is common for FW, whereas, for the other traits, epistatic interactions among QTLs are significant contributors to the genetic variance.
Several FW QTLs were detected in both the RIL and IL populations on the same regions of chromosomes 1, 2, 7, and 11. Two additional QTLs were detected on other regions of chromosome 7 in the RILs, whereas FW QTLs on chromosomes 3, 4, 10, and 12 were only detected with the ILs. Moreover, QTLs on chromosomes 1 and 2 were separated as two linked QTLs with the ILs. Therefore, most of the QTLs detected in the RIL population were verified with the ILs, and further QTLs were also detected, which reflects a high consistency of FW QTLs across generations. This is compatible with the previous hypothesis on the additive effects of FW QTLs and the efficacy of ILs in detecting FW QTLs.
Of the seven SSC QTLs reported in the RIL population and the six SSC QTLs in the IL population, only two mapped in the same chromosomal region on chromosomes 2 and 3. Despite the lower consistence between generations found for TA, none of the TA QTLs detected in the RILs could be verified with the ILs, and the only QTL detected in the ILs (ta2.1) was not present in the RIL QTL map.
The stability of QTL effects over generations is a crucial issue when implementing MAS. The lack of this stability is one of the major factors that could explain the limited use of MAS for QTLs (Collard and Mackill, 2008). In tomato, what is probably the most thorough study on QTL effect stability over generations was carried out by Chaïb et al. (2006), where they found that, out of 10 QTLs detected in an RIL population, five were detected in BC3S1 and eight in BC3S3 populations, indicating a good stability.
In the current report, we have found that QTL stability depends on the trait in question, as it is high for FW, low for SSC and absent for TA. The discrepancy in QTL detection among generations can be attributed to experimental (environmental) and biological factors. Interaction with genetic background must have an important role; the fact that different traits show different levels of stability can be explained by differences in the importance of genetic background interactions in the expression of the QTLs, i.e., the prevalence of additive gene action versus epistasis. Of note is the fact that the comparison of trait distributions between the RILs and the ILs indicated that epistatic interactions most likely have an important role in SSC and TA traits, as all show a low QTL stability. Another factor could be the pleiotropic effects of fruit size on SSC and TA, as RIL fruits are much smaller than IL fruits.
The differences in QTL stability across generations observed in the current work reinforce the necessity of developing populations in the proper genetic background, depending on the objectives of the study. Transferring QTLs to different genetic backgrounds will always be a challenge, and it will probably always depend on each particular case. ILs are the populations of choice, especially for applied MAS, as the QTLs of interest can be evaluated in the final genetic background.
Author Contributions
WB: was involved in data acquisition, analysis, interpretation of the data, and drafted the manuscript; GL-C, SG-M, AA, and JR: was involved in data acquisition and critical review of the manuscript; RF-M: was involved in the design of the work, data acquisition, and critical review of the manuscript; AG: was involved in the design of the work, data acquisition and critical review of the manuscript; AM: was involved in the design of the work, data acquisition, analysis, and drafted the manuscript. All authors approve this version of the manuscript and agree to be accountable for all aspects of the work.
Conflict of Interest Statement
The authors declare that the research was conducted in the absence of any commercial or financial relationships that could be construed as a potential conflict of interest.
Acknowledgments
The authors want to thank Soledad Casal, Teresa León, Erika Moro, Teresa Caballero, Rafael Martínez, Silvia Presa, Adrián Grau, José Joaquín García, Javier Vives, Alberto Lara, Antonio Núñez, Luís Rodríguez, Rafael Gómez, Agricultural Cooperative Alginet Coagri and the Metabolomics Service of the IBMCP for their help in the field experiments and phenotyping, the funding from grant AGL2015-65246-R (Spanish MINECO, co-financed by European Union FEDER programme), and the EU Framework Program Horizon 2020 COST Action FA1106 Quality Fruit for networking activities. WB was supported by a fellowship granted by the Universidad de Costa Rica and CSIC-Spain by way of a collaboration agreement between CSIC/UCR. GL-C was supported by a JAE-Doc contract by CSIC co-funded by the European Social Fund (ESF).
Supplementary Material
The Supplementary Material for this article can be found online at: http://journal.frontiersin.org/article/10.3389/fpls.2016.01172
Footnotes
References
Alba, J. M., Montserrat, M., and Fernández-Muñoz, R. (2009). Resistance to the two-spotted spider mite (Tetranychus urticae) by acylsucroses of wild tomato (Solanum pimpinellifolium) trichomes studied in a recombinant inbred line population. Exp. Appl. Acarol. 47, 35–47. doi: 10.1007/s10493-008-9192-4
Ashrafi, H., Kinkade, M. P., Merk, H., and Foolad, M. R. (2012). Identification of novel QTLs for increased lycopene content and other fruit quality traits in a tomato RIL population. Mol. Breed. 30, 549–567. doi: 10.1007/s11032-011-9643-1
Bai, Y., and Lindhout, P. (2007). Domestication and breeding of tomatoes: what have we gained and what can we gain in the future? Ann. Bot. 100, 1085–1094. doi: 10.1093/aob/mcm150
Barrantes, W., Fernández-del-Carmen, A., López-Casado, G., González-Sánchez, M. A., Fernández-Muñoz, R., Granell, A., et al. (2014). Highly efficient genomics-assisted development of a library of introgression lines of Solanum pimpinellifolium. Mol. Breed. 34, 1817–1831. doi: 10.1007/s11032-014-0141-0
Bartley, G. E., and Scolnik, P. A. (1993). cDNA cloning, expression during development, and genome mapping of PSY2, a second tomato gene encoding phytoene synthase. J. Biol. Chem. 268, 25718–25721.
Bernacchi, D., Beck-Bunn, T., Emmatty, D., Eshed, Y., Inai, S., Lopez, J., et al. (1998). Advanced backcross QTL analysis of tomato. II Evaluation of near-isogenic lines carrying single-donor introgressions for desirable wild QTL-alleles derived from Lycopersicon hirsutum and Lycopersicon pimpinellifolium. Theor. Appl. Genet. 97, 170–180. doi: 10.1007/s001220051009
Blanca, J., Cañizares, J., Cordero, L., Pascual, L., Diez, M. J., and Nuez, F. (2012). Variation revealed by SNP genotyping and morphology provides insight into the origin of the tomato. PLoS ONE 7:e48198. doi: 10.1371/journal.pone.0048198
Blanca, J., Montero-Pau, J., Sauvage, C. H., Bauchet, G., Illa, E., Diez, M. J., et al. (2015). Genomic variation in tomato, from wild ancestors to contemporary breeding accessions. BMC. Genomics 16:257. doi: 10.1186/s12864-015-1444-1
Blauth, S. L., Churchill, G. A., and Mutschler, M. A. (1998). Identification of quantitative trait loci associated with acylsugar accumulation using intraspecific populations of the wild tomato, Lycopersicon pennellii. Theor. Appl. Genet. 96, 458–467. doi: 10.1007/s001220050762
Brewer, M. T., Lang, L., Fujimura, K., Dujmovic, N., Gray, S., and van der Knaap, E. (2006). Development of a controlled vocabulary and software application to analyze fruit shape variation in tomato and other plant species. Plant. Physiol. 141, 15–25. doi: 10.1104/pp.106.077867
Brewer, M. T., Moyseenko, J. B., Monforte, A. J., and van der Knaap, E. (2007). Morphological variation in tomato: a comprehensive study of Quantitative Trait Toci controlling fruit shape and development. J. Exp. Bot. 58, 1339–1349. doi: 10.1093/jxb/erl301
Canady, M. A., Meglic, V., and Chetelat, R. (2005). A library of Solanum lycopersicoides introgression lines in cultivates tomato. Genome 48, 685–697. doi: 10.1139/g05-032
Capel, C., Fernández del Carmen, A., Alba, J. M., Lima-Silva, V., Hernández-Gras, F., Salinas, et al. (2015). Wide-genome QTL mapping of fruit quality traits in a tomato RIL population derived from the wild-relative species Solanum pimpinellifolium L. Theor. Appl. Genet. 128, 2019–2035. doi: 10.1007/s00122-015-2563-4
Causse, M., Duffe, P., Gomez, M. C., Buret, M., Damidaux, R., Zamir, D., et al. (2004). A genetic map of candidate genes and QTLs involved in tomato fruit size and composition. J. Exp. Bot. 55, 1671–1685. doi: 10.1093/jxb/erh207
Chaïb, J., Lecomte, L., Buret, M., and Causse, M. (2006). Stability over genetic backgrounds, generations and years of quantitative trait locus (QTLs) for organoleptic quality in tomato. Theor. Appl. Genet. 112, 934–944. doi: 10.1007/s00122-005-0197-7
Chakrabarti, M., Zhang, N. A., Sauvage, C. H., Munos, S., Blanca, J., Cañizares, J., et al. (2013). A cytochrome P450 regulates a domestication trait in cultivated tomato. Proc. Natl. Acad. Sci. U.S.A. 110, 17125–17130. doi: 10.1073/pnas.1307313110
Chen, F. Q., Foolad, M. R., Hyman, J., St. Clair, D. A., and Beelaman, R. B. (1999). Mapping of QTLs for lycopene and other fruit traits in a Lycopersicon esculentum x L. pimpinellifolium cross and comparison of QTLs across tomato species. Mol. Breed. 5, 283–299. doi: 10.1023/A:1009656910457
Chetelat, R. T., and Meglic, V. (2000). Molecular mapping of chromosome segments introgressed from solanum lycopersicoides into cultivated tomato (Lycopersicum esculentum). Theor. Appl. Genet. 100, 232–241. doi: 10.1007/s001220050031
Collard, B. C., and Mackill, D. J. (2008). Marker-assisted selection: an approach for precision plant breeding in the twenty-firt century. Philos. Trans. R. Soc. B Biol. Sci. 363, 557–572. doi: 10.1098/rstb.2007.2170
Darrigues, A., Hall, J., van der Knaap, E., and Francis, D. M. J. (2008). Tomato Analyzer-Color Test: a new tool for efficient digital phenotyping. Am. Soc. Hortic. Sci. 133, 579–586.
Doganlar, S., Frary, A., Ku, H. M., and Tanksley, S. D. (2002). Mapping quantitative trait loci in inbred backcross lines of Lycopersicon pimpinellifolium (LA1589). Genome 45, 1189–1202. doi: 10.1139/g02-091
Eduardo, I., Arus, P., and Monforte, A. J. (2005). Development of a genomic library of near isogenic lines (NILs) in melon (Cucumis melo L.) from the exotic accession PI161375. Theor. Appl. Genet. 112, 139–148. doi: 10.1007/s00122-005-0116-y
Eshed, Y., and Zamir, D. (1994). Introgessions from Lycopersicon pennellii can improve the solute-solids yield of tomato hybrids. Theor. Appl. Genet. 88, 891–897. doi: 10.1007/BF01254002
Eshed, Y., and Zamir, D. (1995). An introgression line population of Lycopersicon pennellii in the cultivated tomato enables the identification and fine mapping of yield-associated QTL. Genetics 141, 1147–1162.
Eshed, Y., and Zamir, D. (1996). Less-than-additive epistatic interactions of quantitative trait loci in tomato. Genetics 143, 1807–1817.
Fernández-Muñoz, R., Dominguez, E., and Cuartero, J. (2000). A novel source of resistance to the two-spotted spider mite in Lycopersicon pimpinellifolium Jusl. Mill.: its genetics as affected by interplot interference. Euphytica 111, 169–173. doi: 10.1023/A:1003893432676
Finkers, R., Heusden, A. W., Dekens-Meijer, F., Kan, J. A., Maris, P., and Lindhout, P. (2007). The construction of a Solanum habrochaites LYC4 introgression line population and the identification of QTLs for resistance to Botrytis cinerea. Theor. Appl. Genet. 112, 1360–1373.
Francis, D. M., Kabelka, E., Bell, J., Franchino, B., and St. Clair, D. (2001). Resistance to bacterial canker in tomato (Lycopersicon hirsutum LA407) and its progeny derived from crosses to L. esculentum. Plant. Dis. 85, 1171–1176. doi: 10.1094/PDIS.2001.85.11.1171
Frary, A., Nesbitt, T. C., Grandillo, S., van der Knaap, E., Cong, B., Liu, J., et al. (2000). Cloning and transgenic expression of fw2.2: a quantitativetrait locus key to the evolution of tomato fruit. Science 289, 85–87. doi: 10.1126/science.289.5476.85
Fridman, E., Carrari, F., Liu, Y. S., and Zamir, D. (2004). Zooming in on a quantitative trait for tomato yield using interspecific introgressions. Science 305, 1786–1789. doi: 10.1126/science.1101666
Fridman, E., Pleban, T., and Zamir, D. (2000). A recombination hotspot delimits a wild-species quantitative trail locus for tomato sugar content to 484pb within an invertase gene. Proc. Natl. Acad. Sci. U.S.A. 97, 4718–4723. doi: 10.1073/pnas.97.9.4718
Fulton, T. M., Bucheli, P., Voirol, E., Lòpez, J., Pètiard, V., and Tanksley, S. D. (2002). Quantitative trait loci (QTL) affecting sugars, organics acids and other biochemical properties possibly contributing to flavor, identified in four advanced backcross populations of tomato. Euphytica 127, 163–177. doi: 10.1023/A:1020209930031
Fulton, T. M., Grandillo, S., Beck-Bun, T., Fridman, E., Frampton, A., Lopez, J., et al. (2000). Advanced backcross QTL analysis of a Lycopersicon esculentum x Lycopersicon parviflorum cross. Theor. Appl. Genet. 100, 1025–1042. doi: 10.1007/s001220051384
Grandillo, S., and Tanksley, S. D. (1996). QTL Analysis of horticultural traits differentiating the cultivated tomato from the closely related species Lycopersicon pimpinellifolium. Theor. Appl. Genet. 92:935. doi: 10.1007/BF00224033
Guo, Z., Weston, P. A., and Snyder, J. C. (1993). Repellency to two-spotted spider mite Tetranychus urticae Koch, as related to leaf surface chemistry of Lycopersicon hirsutum accessions. J. Chem. Ecol. 19, 2965–2979. doi: 10.1007/BF00980596
Gur, A., and Zamir, D. (2004). Unused natural variation can lift yield barriers in plant breeding. PLoS Biol. 2:e245. doi: 10.1371/journal.pbio.0020245
Kang, J. H., McRoberts, J., Shi, F., Moreno, J. E., Jones, A. D., and Howe, G. A. (2014). The flavonoid biosynthetic enzyme chalcone isomerase modulates terpenoid production in glandular trichomes of tomato. Plant. Phys. 164, 1161–1174. doi: 10.1104/pp.113.233395
Kinkade, M. P., and Foolad, M. R. (2013). Validation and fine mapping of lyc12.1, a QTL for increased tomato fruit lycopene content. Theor. Appl. Genet. 126, 2163–2175. doi: 10.1007/s00122-013-2126-5
Ku, H. M., Grandillo, S., and Tanksley, S. D. (2000). fs8.1, a major QTL, sets the pattern of tomato carpel shape well before anthesis. Theor. Appl. Genet. 101, 873–878. doi: 10.1007/s001220051555
Labate, J. A., Grandillo, S., Fulton, T., Muños, S., Caicedo, A. L., Peralta, I., et al. (2007). “Tomato,” in Genome Mapping and Molecular Breeding in Plants Vol. 5: Vegetables, ed. C. Kole (Berlin: Springer), 1–96.
Lee, J. M., Joung, J. G., McQuinn, R., Chung, M. Y., Fei, Z., Tieman, D., et al. (2012). Combined transcriptome genetic diversity and metabolite profiling in tomato fruit reveals that the ethylene response factor SIERF6 plays an important role in ripening and carotenoid accumulation. Plant. J. 70, 191–204. doi: 10.1111/j.1365-313X.2011.04863.x
Lima-Silva, V., Rosado, A., Amorin-Silva, V., Muñoz-Merida, A., Pons, C., Bombarely, A., et al. (2012). Genetic on genome-wide transcriptomic analyses identify co-regulation of oxidative response and hormone transcript abundance with vitamin C content in tomato fruit. BMC Genomics 13:187. doi: 10.1186/1471-2164-13-187
Lin, T., Zhu, G., Zhang, J., Xu, X., Yu, A., Zheng, Z., et al. (2014). Genomic analyses provide insights into the history of tomato breeding. Nat. Genet. 46, 1220–1226. doi: 10.1038/ng.3117
Lippman, Z. B., Semel, Y., and Zamir, D. (2007). An integrated view of quantitative trait variation using tomato interspecific introgression lines. Curr. Opin. Genet. Dev. 17, 545–552. doi: 10.1016/j.gde.2007.07.007
Liu, J. P., van Eck, J., Cong, B., and Tanksley, S. D. (2002). A new class of regulatory genes underling the cause of pear-shaped tomato fruit. Proc. Natl. Acad. Sci. U.S.A. 99, 813302–813306. doi: 10.1073/pnas.162485999
Liu, Y.-S., Gur, A., Ronen, G., Causse, M., Damidaux, R., Buret, M., et al. (2003). There is more to tomato fruit colour than candidate carotenoid genes. Plant Biotechnol. J. 1, 195–207. doi: 10.1046/j.1467-7652.2003.00018.x
Luckwill, L. C. (1943). The Genus Lycopersicon. An Historical, Biological, and Taxonomic Survey of the Wild and Cultivated Tomatoes. Aberdeen: Aberdeen Universtiy Press.
Maluf, W. R., Maciel, G. M., Gomes, L. A., Cardoso, M. D. G., and Goncalves, L. D. (2010). Broad-spectrum arthropod resistance in hybrids between high- and low-acylsugar tomato lines. Crop Sci. 50, 439–450. doi: 10.2135/cropsci2009.01.0045
Monforte, A. J., Díaz, A., Caño-Delgado, A., and van der Knaap, E. (2014). The genetic basic of fruit morphology in horticultural crops: lessons from tomato and melon. J. Exp. Bot. 65, 4625–4637. doi: 10.1093/jxb/eru017
Monforte, A. J., Friedman, E., Zamir, D., and Tanksley, S. D. (2001). Comparison of set of allelic QTL_NILs for chromosome 4 of tomato deductions about natural variation and implications for germoplasm utilization. Theor. Appl. Genet. 102, 572–590. doi: 10.1007/s001220051684
Monforte, A. J., and Tanksley, S. D. (2000a). Development of a set of near isogenic and backcross recombinant inbred lines containing most of the Lycopersicon hirsutum genome in a L. esculentum genetic background: a tool for gene mapping and gene discovery. Genome 43, 803–813. doi: 10.1139/g00-043
Monforte, A. J., and Tanksley, S. D. (2000b). Fine mapping of a quantitative trait locus (QTL) from Lycopersicon hirsutum chromosome 1 affecting fruit characteristics and agronomic traits: breaking linkage among QTLs affecting different traits and dissection of heterosis for yield. Theor. Appl. Genet. 100, 471–479. doi: 10.1007/s001220050061
Pereira da Costa, J. H., Rodriguez, R. G., Pratta, R. G., Picardi, A. L., and Zorzoli, R. (2013). QTL detection for fruit shelf life and quality traits across segregating populations of tomato. Sci. Hortic. 156, 47–53. doi: 10.1016/j.scienta.2013.03.015
Powell, A., Nguyen, C., Hill, T., Cheng, K. L., Figueroa, R., Aktos, H., et al. (2012). Uniform ripening encodes a golden 2-like transcription factor science regulating tomato fruit chloroplast development. Science 336, 1711–1715. doi: 10.1126/science.1222218
Prudent, M., Causse, M., Genard, M., Tripodi, P., Grandillo, S., and Bertin, N. (2009). Genetic and physiological analysis of tomato fruit weight and composition: influence of carbon availability on QTL detection. J. Exp. Bot. 60, 923–937. doi: 10.1093/jxb/ern338
Rambla, J. L., Tikunov, Y. M., Monforte, A. J., Bovy, A. G., and Granell, A. (2014). The expanded tomato fruit volatile landscape. J. Exp. Bot. 65, 4613–4623. doi: 10.1093/jxb/eru128
Rousseaux, M. C., Jones, C. M., Adams, D., Chetelat, R., Bennett, A., and Powell, A. (2005). QTL analysis of fruit antioxidants in tomato using Lycopersicon pennellii introgression lines. Theor. Appl. Genet. 111, 1396–1408. doi: 10.1007/s00122-005-0071-7
Sacks, E. J., and Francis, D. M. (2001). Genetic and environmental variation for flesh color of tomato fruit in a population of modern breeding lines. J. Am. Soc. Hortic. Sci. 126, 221–226.
Salinas, M., Capel, C., Alba, J. M., Mora, B., Cuartero, J., Fernández-Muñoz, R., et al. (2013). Genetic mapping of two QTL from the wild tomato Solanum pimpinellifolium L. controlling resistance against two-spotted spider mite (Tetranychus urticae Koch). Theor. Appl. Genet. 126, 83–92. doi: 10.1007/s00122-012-1961-0
Schauer, N., Semel, Y., Roessner, U., Gur, A., Balbo, I., Carrari, F., et al. (2006). Comprehensive metabolic profiling and phenotyping of interspecific introgression lines for tomato improvement. Nat. Biotechnol. 24, 447–454. doi: 10.1038/nbt1192
Silva, F., Michereff-Filho, M., Fonseca, E. N. M., Silva-Filho Texeira, C. A. A., Moita, W. A., Torres, B. J., et al. (2014). Resistance to Bemisia tabaci biotype B of Solanum pimpinellifolium is associated with higher densities of type IV glandular trichomes and acylasugar accumulation. Entomol. Exp. Appl. 151, 218–230. doi: 10.1111/eea.12189
Sim, S.-C., Durstewitz, G., Plieske, J., Wieseke, R., Ganal, M. W., and Van Deynze, A. (2012). Development of a large SNP genotyping array and generation of high-density genetic maps in tomato. PLoS ONE 7:e40563. doi: 10.1371/journal.pone.0040563
Sim, S.-C., Robbins, M. D., Chilcott, C., Zhu, T., and Francis, D. M. (2009). Oligonucleotide array discovery of polymorphisms in cultivated tomato (Solanum lycopersicum L) reveals patterns of SNP variation associated with breeding. BMC Genomics. 10:466. doi: 10.1186/1471-2164-10-466
Sim, S.-C., Robbins, M. D., Van Deynze, A., Michel, A. P., and Francis, D. M. (2011). Population structure and genetic differentiation associated with breeding history and selection in tomato (Solanum lycopersicum L.). Heredity 106, 927–935. doi: 10.1038/hdy.2010.139
Steinhauser, M. C., Steinhauser, D., Gibon, Y., Bolger, M., Arrivault, S., Usadel, B., et al. (2011). Identification of enzyme activity quantitative trait loci in a Solanum lycopersicum x Solanum pennellii introgression line population. Plant. Physiol. 157, 998–1014. doi: 10.1104/pp.111.181594
Stevens, M. A., Kader, A. A., Albright-Holton, M., and Alga, M. (1977). Genotypic variation on flavor and composition in fresh market tomatoes. J. Am. Soc. Hortic. Sci. 102:680.
Tanksley, S. D., Grandillo, S., Fulton, T. M., Zamir, D., Eshed, Y., Petiard, V., et al. (1996). Advanced backcross QTL analysis in a cross between an elite processing line of tomato and its wild relative L. pimpinellifolium. Theor. Appl. Genet. 92, 213–224. doi: 10.1007/BF00223378
Tanksley, S. D., and McCouch, S. R. (1997). Seed banks molecular maps: unlocking genetic from the wild. Science 277, 1063–1066. doi: 10.1126/science.277.5329.1063
Tieman, D., Bliss, P., Mclatyre, L. M., Blondon-ubeda, A., Bies, D., Odabasi, A. Z., et al. (2012). The chemical interactions underlying tomato flavor preferences. Curr. Biol. 22, 1035–1039. doi: 10.1016/j.cub.2012.04.016
van der Knaap, E., and Tanksley, S. D. (2003). The making of a bell pepper-shaped tomato fruit: identification of loci controlling fruit morphology in yellow stuffer tomato. Theor. Appl. Genet. 107, 139–147.
van Tuinen, A., Cordonnier-Pratt, M. M., Pratt, L. H., Verkerk, R., Zabel, P., and Koornneef, M. (1997). The mapping of phytochrome genes and photomorphogenic mutants of tomato. Theor. Appl. Genet. 94, 115–122. doi: 10.1007/s001220050389
Williams, C. E., and Clair, D. A. (1993). Phenetic relationships and levels of variability detected by restriction fragment length polymorphism and random amplified polymorphic DNA analysis of cultivated and wild accessions of Lycopersicon esculentum. Genome 36, 619–630. doi: 10.1139/g93-083
Xu, C., Liberatore, K. L., MacAlister, C. A., Huang, Z., Chu, Y.-H., Jiang, K., et al. (2015). A cascade of arabinosyltransferases controls shoot meristem size in tomato. Nat. Genet. 47, 784–792. doi: 10.1038/ng.3309
Xu, J., Ranc, N., Muños, E., Rolland, S., Bouchet, J. P., Desplat, N., et al. (2013). Phenotypic diversity and association mapping for fruit quality traits in cultivated tomato and related species. Theor. Appl. Genet. 126, 567–581. doi: 10.1007/s00122-012-2002-8
Yang, C. H., Li, H., Zhang, J., Wang, T., and Ye, Z. (2011). Fine-mapping of the woolly gene controlling multicellular trichome formation and embryonic development in tomato. Theor. Appl. Genet. 123, 625–633. doi: 10.1007/s00122-011-1612-x
Keywords: quantitative trait loci (QTL), germplasm, wild species, mapping, genotype by environment interaction
Citation: Barrantes W, López-Casado G, García-Martínez S, Alonso A, Rubio F, Ruiz JJ, Fernández-Muñoz R, Granell A and Monforte AJ (2016) Exploring New Alleles Involved in Tomato Fruit Quality in an Introgression Line Library of Solanum pimpinellifolium. Front. Plant Sci. 7:1172. doi: 10.3389/fpls.2016.01172
Received: 23 March 2016; Accepted: 21 July 2016;
Published: 17 August 2016.
Edited by:
Soren K. Rasmussen, University of Copenhagen, DenmarkReviewed by:
Andrea Mazzucato, Tuscia University, ItalyPaul Christiaan Struik, Wageningen University and Research Centre, Netherlands
Copyright © 2016 Barrantes, López-Casado, García-Martínez, Alonso, Rubio, Ruiz, Fernández-Muñoz, Granell and Monforte. This is an open-access article distributed under the terms of the Creative Commons Attribution License (CC BY). The use, distribution or reproduction in other forums is permitted, provided the original author(s) or licensor are credited and that the original publication in this journal is cited, in accordance with accepted academic practice. No use, distribution or reproduction is permitted which does not comply with these terms.
*Correspondence: Antonio J. Monforte, amonforte@ibmcp.upv.es