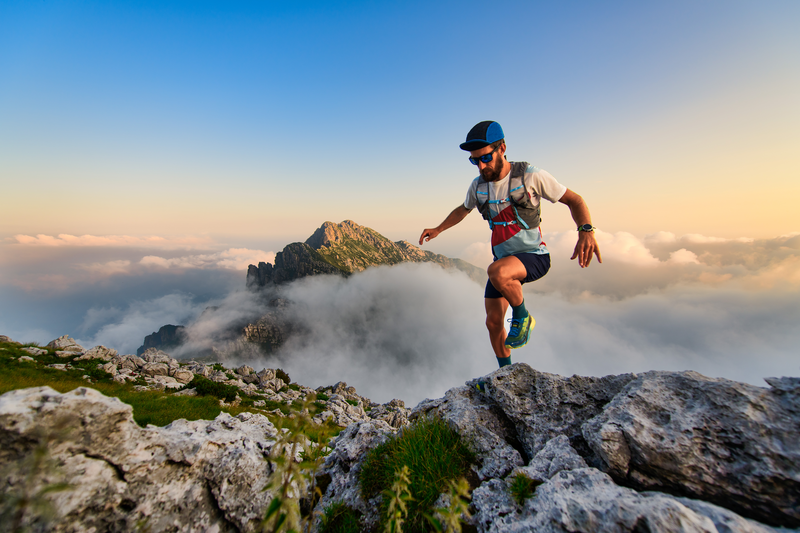
94% of researchers rate our articles as excellent or good
Learn more about the work of our research integrity team to safeguard the quality of each article we publish.
Find out more
ORIGINAL RESEARCH article
Front. Plant Sci. , 28 July 2016
Sec. Plant Breeding
Volume 7 - 2016 | https://doi.org/10.3389/fpls.2016.01090
This article is part of the Research Topic Genomic Approaches for Improvement of Understudied Grasses. View all 12 articles
Scarcity of irrigation water and increasing soil salinization has threatened the sustainability of forage production in arid and semi-arid region around the globe. Introduction of salt-tolerant perennial species is a promising alternative to overcome forage deficit to meet future livestock needs in salt-affected areas. This study presents the results of a salinity tolerance screening trial which was carried out in plastic pots buried in the open field for 160 buffelgrass (Cenchrus ciliaris L.) accessions for three consecutive years (2003–2005). The plastic pots were filled with sand, organic, and peat moss mix and were irrigated with four different quality water (EC 0, 10, 15, and 20 dS m−1). The results indicate that the average annual dry weights (DW) were in the range from 122.5 to 148.9 g/pot in control; 96.4–133.8 g/pot at 10 dS m−1; 65.6–80.4 g/pot at 15 dS m−1, and 55.4–65.6 g/pot at 20 dS m−1. The highest DW (148.9 g/pot) was found with accession 49 and the lowest with accession 23. Principle component analysis shows that PC-1 contributed 81.8% of the total variability, while PC-2 depicted 11.7% of the total variation among C. ciliaris accessions for DW. Hierarchical cluster analysis revealed that a number of accessions collected from diverse regions could be grouped into a single cluster. Accessions 3, 133, 159, 30, 23, 142, 141, 95, 49, 129, 124, and 127 were stable, salt tolerant, and produced good dry biomass yield. These accessions demonstrate sufficient salinity tolerance potential for promotion in marginal lands to enhance farm productivity and reduce rural poverty.
Salt-affected soils and millions of hectares of marginal lands have limited the scope for crop production (Wang et al., 2012). According to the Food and Agriculture Organization (FAO), 34 million hectares (11% of the total irrigated area of the world) are affected by different levels of salinization (Food Agriculture Organization of the United Nations, 2012). Moreover, the world loses 0.25–0.5 M ha of agricultural land annually because of salt buildup, which mainly results from irrigation, especially in arid and semiarid areas (Peng et al., 2008; Qadir et al., 2014). Soil salinity reduces the productivity of most crops, although to a varying extent depending on species (Roy et al., 2014; Hussain et al., 2016). Besides improving water management practices to reduce salt accumulation in the root zone, there is a need to improve salinity tolerance of strategically important crops. The use of low quality saline water for plant production is an option to conserve limited freshwater resources, particularly for the water-scarce regions of Arabian and African peninsula.
The cultivation of forages, biomass crops, and perennial grasses as feedstock for energy, biomaterials, and livestock rearing have been promoted as an opportunity to improve sustainability of forage supply, energy security, and contributing to the rural development (Ahmad, 2010). Therefore, demand for sustainable biomass production for livestock and energy use has raised the interest in perennial crops like Cenchrus ciliaris L. Buffelgrass (C. ciliaris L.) is a perennial (C4) forage grass (family poaceae), sometimes produces rhizomes and is native to the Arabian Peninsula. The C. ciliaris is dominant in natural grazing zones of Ethiopia (Angassa and Baars, 2000), Australia (Buldgen and Francois, 1998), and North Africa (Mseddi et al., 2004). Buffelgrass has proved useful for pasture and soil retention in a wide range of environments due to its drought tolerance, high biomass, deep roots, rapid response to summer rains, and resistance to overgrazing. With extensive belowground systems, cultivation of perennial grasses present high efficiencies in the use of nutrient and water resources and control of soil erosion, carbon sequestration with the restoration of soil properties (fertility, structure, organic matter). Compared with annual systems, herbaceous perennial crops have the advantages of erodibility, and crop management options, such as pesticides and fertilizers inputs (Zhang et al., 2011; Fernando et al., 2012). The salt tolerance of different C. ciliaris genotypes need to be evaluated to test their suitability for marginal environments to offer a more practical solution for effective utilization of salt affected soils. Among buffelgrass, accession from North America, “Texas 4464” has been reported as drought tolerant (Ayerza, 1981), and “Biloela” as salt-tolerant (Graham and Humphreys, 1970). Therefore, strategies for mitigating salinity problems in crop production include both development of management options (Shannon, 1997) and genetic improvement of current cultivars (Krishnamurthy et al., 2007).
Germplasm of a specific crop collected from the diverse sources offers greater genetic diversity and may furnish useful traits to widen the genetic base of crop species. The collection, screening and description of the existing variability among the forage crops are the first step in the performance evaluation and selection process (Ponsens et al., 2010). Knowledge about germ- plasm diversity and salinity tolerance evaluation will be an excellent tool to screen and select high yielding accessions for further evaluation under field conditions. Screening large numbers of genotypes for salinity tolerance in the field is notoriously difficult because of the variability of salinity within fields (Daniells et al., 2001). Moreover, it would be difficult to determine the critical parameters under field conditions since any environmental change could result in dramatic change in the plant's response to salinity (Shannon, 1997). Although C. ciliaris response to salinity stress has been a topic of many researchers (Arshad et al., 2000; Hacker and Waite, 2001; Jorge et al., 2008; Ksiksi and El-Shaigy, 2012); to best of our knowledge, no study has evaluated and characterized the C. ciliaris genotypes in terms of agro-morphological attributes and dry matter yield responses so far. This study evaluates the morphological and biomass yield responses of C. ciliaris genotypes to water salinity in pot culture trial.
In a study of salt stress on buffelgrass and its effects on productivity decline; Lanza Castelli et al. (2010) has found that C. ciliaris accession, Texas 4464, is susceptible to salt stress at 300 mM NaCl concentrations at the seedling stage, while Americana showed tolerance against salinity. The fresh weight, root length, and plant height of these accessions were least affected by salinity. However, screening and selection of large numbers of buffelgrass genotypes for salinity tolerance is lacking. Therefore, the current research was undertaken with the aim to identify superior genotypes for forage production under hot arid conditions of UAE using naturally available low quality saline water. The results of this research are expected to provide useful information that can be used to group accessions for relative comparison and evaluation of biomass yield in areas where problems of soil and water salinity are increasing.
The field trials were conducted for three growing seasons during 2003–2005 on the experimental farm of the International center for Biosaline Agriculture (ICBA), located on the eastern side of Dubai between 25°5′ N and 55°23′ E with an elevation of 30 m above mean sea level. The soil of the experimental field is Carbonatic, Hyperthermic Typic Torripsamment having a negligible level of inherent soil salinity (0.2 dS m−1). The study area is characterized by very hot, dry days in summer (April–October), when temperatures can reach 45°C, while the winter days (December–February) are mainly cooler and dry with low average night-time temperatures (10°C). Because of the aridity, and relatively cloudless skies, there are great extremes of temperature variation, but there are also wide variations between the seasons. Air temperature follows a regular seasonal trend, with a minimum in January and a maximum in July. According to the ICBA weather station data the annual average temperature of the study area was 10°C during the month of January while average maximum temperature during summer, July, was 45°C. The average annual rainfall is < 80 mm and falls in short, torrential bursts during the summer months.
In total, 160 accessions of buffelgrass (C. ciliaris L.) were used in this experiment as shown in Table 1. The seeds of C. ciliaris L. were received from United States Department of Agriculture (USDA); originated from Asia, Africa, Australia, India, USA and some local landraces and commercial cultivars were also included in the study. Seeds of C. ciliaris genotypes (three per pot) were sown in poly vinyl chloride pots (30 × 30 cm) on 2nd March 2003. The pots were buried in the open field (Figure 1), near the net house at experimental research station (ICBA, Dubai, UAE) for three consecutive years (2003–2005). After uniformity of emergence, seedlings were thinned to one plant per pot to maintain good stand establishment. Experimental soil achieved field capacity and permanent wilting point at 0.1 bar and 15 bars, respectively. All pots were irrigated to upper limit of field capacity before planting.
Table 1. Buffel grass (Cenchrus ciliaris L.) germplasm collection with genebank entry numbers and country of origin.
Figure 1. Screening Cenchrus ciliaris for salt tolerance in pots buried in open field at Research Station, ICBA, Dubai, UAE. (A) Germination, (B) growth and tillering, (C) heading stage, and (D) harvesting.
The soil was collected from an open area of ICBA that had not been previously irrigated with saline water. Textural analysis showed that the resulting soil had 98% sand, 0.5% silt, and 1% clay, which would be classified as sandy soils. The plastic pots were filled with soil mix (20 kg/pot) containing 50% sand, 25% organic fertilizer, and 25% peat moss. The fertilizer NPK was mixed periodically at the start of the trial and after each harvest. The weeds were eradicated by hand weeding.
The C. ciliaris accessions were arranged in the main plot while saline water treatment (0, 10, 15, and 20 dS m−1) was randomized in RCBD split plot design. Initially, the pots were irrigated with fresh water up till 1 month to facilitate the germination and seedling establishment. One pot was assigned to each accession per replication and four replications per treatment were maintained throughout the trial. The saline water treatments were applied 1 month after sowing and each salinity treatment was made by diluting ground water (EC: 25 dS m−1) with fresh water (1.5 dS m−1) in a separate tank to achieve the target salinities and then delivered to the pots via drip irrigation system. Irrigations were applied on daily basis at rates equivalent to ET0 plus 20% for leaching requirements. The four salinity levels were maintained constantly throughout the growth period during all the years.
The field experiment was equipped with a drip system (pressure compensating (PC), micro flapper with 4 L hr−1 flow rate) and 0.5 m distance between rows and 0.25 m between drippers. Each pot has one dripper; the pot area was 0.12 m2 which means that for each 4 liters the depth would be 33 mm hr−1. Irrigation monitoring, scheduling and salinity management were achieved using Decagon®; sensors. During the summer season (9 months from March–November) the pots were irrigated 30 min per day which means an irrigation depth of 16 mm per pot with the leaching fraction, but during mild winter season (3 months from December–February) the irrigation duration was 20 min which means an irrigation depth of 11 mm per day with the leaching fraction. The drippers were tested biweekly to check the distribution uniformity and coefficient using the method of the low quarter average divided by the average of the whole readings, the data (not shown) that the distribution uniformity was not < 85% with a distribution coefficient of 80%.
The plants were harvested at heading stage and weighed with an analytical balance. Table 2 demonstrates the harvesting dates and number of harvests (cuts) obtained during each year. For each harvest, the total fresh weight of the collected biomass samples was weighed in g/pot. The samples were dried in a forced air oven at 60°C for 72 h and dry matter yield (DW) was determined.
Statistical analyses were performed on fresh biomass (FW) and dry weight (DW) in two steps:
(1) The experimental data for each cut (fresh weight, dry weight) of 160 genotypes were analyzed using a general linear model with salinity treatment and genotypes as fixed factor and year as a random factor using SPSS for Windows version 17.0 (SPSS Inc., Chicago, IL, USA). Difference between treatments means were compared using Tukey's HSD test.
(2) Principal component analysis (PCA) was performed on genotypic trait means (FW, DW), to partition the performance of accessions under salt stress conditions. The variate stands for any response variable and “individual” stands for any entry in this analysis. PCA helps the selection of entries, at 25% intensity of selection, based on entries loadings on the first and the second components at each salinity level. The accessions were selected and ability of each entry was determined using its loadings on two components of PCA.
(3) Hierarchical cluster analysis of 160 buffelgrass accessions using JMP 12.0 software was carried out taking fresh biomass yield and dry weight taking into account means of all years at overall salinity. The between-groups linkage method of clustering was adopted using Euclidean distances.
The salinity treatments significantly influenced the growth and biomass yield of various C. ciliaris accessions. The average biomass yield (fresh and dry weight) were calculated by taking the average of 3 years' data for each salinity level (Figures 2, 3). Fresh weight (FW) ranged between 50 and 450 g/pot in control, 50–350 g/pot at low salinity (10 dS m−1), 50–300 g/pot at medium high salinity (15 dS m−1) and 50–200 g/pot at high salinity (20 dS m−1) (Figures 2A–D). The curve was bell shaped in control pots and right skewed distribution when the values clustered more toward lower production groups at high salinity (Figure 2D). Accession 127 proved to be stable and higher yielder that produced the maximum FW (400.8 g/pot) in control and 348.9 g/pot fresh biomass at 10 dS m−1. However, at medium and higher salinity (15, 20 dS m−1), its biomass yield decreased significantly and produced 180.1 and 122.3 g/pot, respectively. Accession 49 proved to be stable genotype that produced the 2nd highest biomass yield (351.1 g/pot) in the control pots while 292.4 g/pot at 10 dS m−1, 219.3 g/pot at 15 dS m−1, and 181.7 g/pot at 20 dS m−1, respectively. At medium and higher salinity (15 and 20 dS m−1), accession 30 produced 233.8 g and 154.2 g/pot FW and demonstrated as medium salt tolerant genotypes.
Figure 2. Frequency distribution and yield range (g/pot) of 160 buffelgrass accessions (Means of fresh weight at A–D at heading stage). Whereas, A = 0, B = 10, C = 15, and D = 20 dS m−1 salinity level.
Figure 3. Frequency distribution and yield range (g/pot) of 160 buffelgrass accessions (Mean of dry weight at A–D at heading stage). Whereas, A = 0, B = 10, C = 15, and D = 20 dS m−1 salinity level.
The dry weight range was in the range of 20–160 g/pot in control, 20–140 g/pot at 10 dS m−1, 100–20 g/pot at 15 dS m−1, and 20–80 g/pot at 20 dS m−1 respectively (Figures 3A–D). The curve distribution was bell shaped in control pots and right skewed at high salinity (20 dS m−1) (Figure 3D). The range of variation reduced with an increase in salinity levels and ranged between 20 and 160 g/pot when salinity varies from 0 to 20 dS m−1. The reduction in dry biomass was 87.5, 85.7, 80, and 75% at 10, 15, and 20 dS m−1, respectively (Figures 3A–D). The average mean DW was 148.9 g/pot recorded for accession 49 in control while accession 127 produced the highest dry biomass (141.4 g/pot), in control pots, respectively (data not shown). The accession 124 produced the maximum DW (80.3 g/pot) at 20 dS m−1 salinity and proved to be stable and higher yield genotype. At highest salinity (20 dS m−1), accession 49 produced the maximum dry biomass (65.6 g/pot) (data not shown).
Fresh biomass (total annual yield) ranged from 301.6 to 400.8 g/pot in control, 256.2–348.9 g/pot at 10 dSm−1, 192.5–233.8 g/pot at 15 dSm−1, and 164.8–185.0 g/pot at 20 dS m−1) among the top 10 accessions of C. ciliaris (Figure 4). The results illustrated that FW in 112, 37, 30, 23, and 46 accessions were more affected by the salinity at 10, 15, and 20 dS m−1, respectively. The accessions 49, 159, 129, 30, 38, were the least affected by increasing the salinity level from 0 to 20 dS m−1. However, accession 129 produced good fresh biomass yield in control, 10 dS m−1, and 15 dS m−1 salinity while it was not a stable genotype at higher salinity (20 dS m−1) that produced less FW as compared to other top 10 genotypes (Figure 4). The salinity tolerance values in DW from all buffelgrass accessions show a high degree of variability under the various salinity levels. Average annual DW yield ranged from 122.5 to 148.9 g/pot in control; 96.4–133.7 g/pot at 10 dS m−1; from 65.6 to 80.3 g/pot at 15 dS m−1 and 55.4–65.6 g/pot at higher salinity (20 dS m−1). The highest DW (148.9 g/pot) was produced by accession 49 in control and the lowest one (55.4 g/pot) was recorded in accession 23 following treatment at the highest salinity level (20 dS m−1) (Figure 5).
Figure 4. Effect of different irrigation water salinity levels on fresh biomass yield (total annual yield) of TOP 10 Cenchrus ciliaris genotypes. Error bars represent standard deviations of the mean of four replicates. Whereas, (A) = 0, (B) = 10, (C) = 15, and (D) = 20 dS m−1 salinity level.
Figure 5. Effect of different irrigation water salinity levels on dry weight (total annual dry biomass) of TOP 10 Cenchrus ciliaris genotypes. Error bars represent standard deviations of the mean of four replicates. Whereas, (A) = 0, (B) = 10, (C) = 15, and (D) = 20 dS m™1 salinity level.
The results of principal components analysis show that out of four components, only 1 had extracted Eigen values over 1. This is based on Chatfield and Collin (1980) assumption which stated that components with an Eigen value of < 1 should be eliminated. The extracted component was subsequently rotated according to Varimax rotation in order to make interpretation easier and fundamental significance of extracted component to the irrigation water salinity. Principle component PC 1 was extracted having Eigen value >1 and contributed 83.5% of the total variability among the C. ciliaris genotypes assessed for fresh biomass (Figure 6). However, the PC 2 depicted 10.7% of the total variation for the same parameter. Furthermore, PC1 contributed 81.8% of the total variability among the 160 genotypes and PC2 only depicted 11.7% of the total variation for dry weight (DW). Salinity applies a gradual selection pressure on the entries that was higher for dry biomass than for fresh biomass. PCA of C. ciliaris genotypes revealed diverse grouping pattern. The separation on the basis of PC1 and PC2 revealed that the genotypes were scattered in all the quarters, which show the high level of genotypic variation among the accessions (Figure 6). The first principal component correlated with five of the original variables (DWS2, FW2, FWS3, DWS1, and FWS4) and more strongly with the DWS2. In fact, we could state that based on this correlation, principal component is primarily a measure of the DWS2 (data not shown). In the first principal components, accessions 49, 22, 16, 42, 8, 149, showed more genotypic variation based on FW and are located close to axis line. In the second principal components, accessions 49, 133, 23, 3, 30, 6, showed more genotypic variation based on dry weight (DW) trait and are also located close to axis line.
Figure 6. Principal component analysis of the variables: Fresh weight (FW) and dry weight (DW) at four water salinity levels (0, 10, 15, 20 dS m−1). Two dimensional scatter plot of genotypic relationship among 160 Cenchrus ciliaris accessions as revealed by first two principal components.
Germplasm evaluation for quantitative traits may help to work out the relative importance of various traits within each cluster. Hierarchical cluster analysis based on agronomical traits (fresh and dry biomass) divided the 160 accessions into 6 main clusters. Maximum number of accessions (42) were present in group I, followed group II (27), group III (26), group IV (19), group V (17), group VI (17), and group VII (12) (Figure 7). Hierarchical cluster analysis showed that some of accessions originated from various geographical areas were grouped into the same cluster, while many others fell into different clusters. Group VII consisted of highly salt tolerant genotypes (3, 133, 159, 30, 23, 142, 141, 95, 49, 129, 124, and 127) that acquired maximum biomass yield at all target salinities and also among the top 10 accessions. Grouping pattern indicated that the clusters were heterogeneous with regard to the geographical origin and some entries from different geographic regions were pooled in the same cluster (Figure 7).
Figure 7. Dendrogram showing the relationship among 160 accessions of Cenchrus ciliaris based on plant biomass in a Hierarchical Clustering analysis.
The observations of present study were largely consistent with the available descriptions of commercial cultivars. The genotype 159 (Biloela) was salt tolerant, productive and produced higher biomass than other genotypes at different water salinities (0, 10, 15, 20 dS m−1). Other commercial genotypes 158 (Gayndah) and 160 did not show the good performance in terms of fresh and dry biomass and were not stable at medium and high salinities (data not shown).
Yield assessment conducted in field trials provides the most reliable information on salt tolerance; however, spatial and temporal variability in soil salinity (Richards, 1983) make it difficult to obtain reproducible information. The advantages of using pots buried in open field are that the saline conditions can be controlled and constantly monitored. Salt affects the growth of plants by limiting the absorption of water and essential nutrients through roots. Salt stress has an immediate effect on cell growth and enlargement, and high concentrations of salts can be extremely toxic (Munns and Tester, 2008; Hussain et al., 2016). In the present study, there was wide variation among C. ciliaris genotypes grown under different salinity levels regarding their biomass yield. The fresh and dry weight of all genotypes was decreased with increase in salt stress. However, salt-sensitive genotypes showed more reduction in their biomass as compared to tolerant genotypes. Lanza Castelli et al. (2010) demonstrated a significant reduction (50–70%) in height and shoot fresh weight at 30 dS m−1 in Texas and Biloela accessions. In the present study, the accessions 49, 127, 129, 30, 38, were the least affected by increasing salinity. The accession 129 produced good biomass at low salinity; however, it was not a stable genotype at higher salinity. Therefore, it is quite suitable for low and medium saline areas where water salinity falls in the range 5–15 dS m−1, respectively. Our results demonstrate that accessions from Africa were more stable, salt tolerant and attained high biomass yield as compared to accessions from other regions. Previously, it was reported that, C. ciliaris is a dominant grass along the sandy beaches of Eritrea on soils with salt content of 0.006–0.251% (Hemming, 1961). Skerman and Riveros (1990), demonstrated that it grows well in saline soils; although it is less salt tolerant than Chloris gayana, Cynodon spp., and Panicum antidotale.
The DW from all C. ciliaris accessions shows a high variability under the various salinity levels. The highest DW was produced by accession 49 and the lowest by accession 23. Similar studies were conducted on other grass species such as Bermuda grass (Rodríguez and Miller, 2000), Catharanthus roseus (Jaleel et al., 2008) and C. ciliaris (Lanza Castelli et al., 2010) and showed that the plant growth, tiller, number of leaves and dry weight decreased under salt stress. Ions of sodium (Na+) and chlorine (Cl−) (>40 mmol/L) can be toxic to plants because they can create imbalance in plant nutrition due to decreased nutrient uptake and translocation to new shoots (Munns et al., 2000; Tester and Davenport, 2003; Munns and Tester, 2008). Nawazish et al. (2006) found that shoot dry weight was severely affected in the C. ciliaris ecotype from Faisalabad, where it decreased from 29.83 to 8.02 g/plant. High salinity modifies plant metabolisms, which results in altered plant morphology; cultivar type, duration, and intensity of stress determine the extent of morphological modification. These results indicate that dry biomass yield can be used as a screening and selection criteria for evaluating the salt tolerance behavior among a large collection of plant accessions. Based on their relative performance (DW) there is a chance of selecting the genotypes within each salinity level. Several accessions from the top 10 selection with high biomass yield potential are of particular interest as forage resource in salt-affected agro-ecosystems for livestock and are highly suitable for arid and semi-arid hot dry regions.
From present results, we found that, PCA grouped accessions together with more morphological similarities; the clusters did not necessarily include all the accessions from the same or nearby sites. Diversity of populations within a geographical origin and similarity of populations beyond geographical limits have also been reported in C. ciliaris genotypes (Jorge et al., 2008). Among the good performed accessions, genotypes 127, 129, 49, 23, 27, 16, and 42 attained higher DW. It was also found that variables (genotypes) close to an axis correlate more with that principal component; one may consider that axis is a combination of its neighboring variables. Divergence studies of morphological and agronomical traits using principal component and cluster analysis have also been reported by other researchers (Warwick et al., 2006; Dawood et al., 2009) which were in support of present investigation that both cluster and PCA disclosed complex relationship among the accessions in a more understandable way. The variability among the accessions from diverse origin could be related primarily to their morphological differences and secondly to agronomic use. Accessions evaluated in this work exhibited a reasonable level of diversity for some of the studied traits (FW, DW) of economic significance providing a resource for future crop improvement. For the improvement of C. ciliaris, it would be desirable to use diverse accessions with more variability for use in future breeding programs. For use in natural resource management and for soil stabilization, C. ciliaris accessions which are shorter, more prostrate and more rhizomatous should be selected, because they would provide better ground cover (IBPGR, 1984).
A cluster analysis was used in this study to facilitate the evaluation of salt tolerance among different genotypes. The major advantage of the utilization of a multivariate analysis in the evaluation for salt tolerance is the allowance of a simultaneous analysis on multiple parameters and increase in the accuracy in the rankings of genotypes. Another advantage is the convenience to rank genotypes when plants are evaluated at different salt levels, e.g., moderate and high salt levels. Cluster analysis proved their validity to establish genotypic diversity within accessions. These findings are supported by Iqbal et al. (2010) who concluded that quantitative characters revealed more reliability than biochemical markers that are specific for particular conditions. Germplasm evaluation for quantitative traits may help to work out the relative importance of various traits within each cluster. Accessions from different geographical regions were pooled in the same cluster. Our results coincide with the classification of 68 populations of C. ciliaris where most of the populations were grouped mainly due to their agronomical attributes and not related to their geographic origin (Jorge et al., 2008). Group VII consists of highly salt tolerant genotypes that acquired maximum biomass yield at all target salinities and were also among the top 10 accessions. Grouping was not associated with the geographic distribution; instead accessions were mainly grouped based on their morphological traits (FW, DW). Thus, it cannot be generalized that all the accessions having same origin would always have low diversity among them. For example, in Australia, where C. ciliaris is grown extensively, rainfall can occur during the cooler months and most cultivars of C. ciliaris respond poorly to these rains owing to the cold temperatures. Accessions originating from cool, dry environments could be promising for these areas through improved performance in spring, owing to a better response to rains during the cooler seasons (Hacker and Waite, 2001). Divergence studies of morphological and agronomical attributes using principal component and cluster analysis have been made by different researchers (Dawood et al., 2009).
A good starting point in selecting suitable accessions for dry areas is to test accessions with good agro-morphological attributes originated from areas with very low rainfall. Several accessions of potential interest were collected from arid areas of South Africa, Zimbabwe, Tanzania and hot arid environments of UAE and Kenya. Salinity tolerance is generally an important parameter when choosing accessions to evaluate, especially within a country, because of the strong correlation between genotype and environment (G × E). A total of 100 accessions, originating from South Africa are found in four of the five clusters, showing that there is not a strong link between environment and morphology, confirming the findings in a study on dry matter and phenology of C. ciliaris in Tunisia (Mseddi et al., 2004). Some other studies also classified American and Gayndah in the same group (Pengelly et al., 1992).
The present study was largely consistent with the available descriptions of commercial cultivars. One main reason for discrepancies among experimental findings could be a strong influence of the environment. Within-cultivar high variation in C. ciliaris is a well-established fact (Jorge et al., 2008) that may be one of the reasons why commercial cultivars are not consistent in their performance. They further concluded that accessions belonging to identical agro-morphological groups were found from a wide range of environments in sub-Saharan Africa. Inherent genetic variability present in perennial grasses collected from different habitats of Cholistan desert were evaluated and high amount of genotypic variations were recorded among the accessions (Arshad et al., 2000). A genotypic characterization of the collection could provide further evidence to confirm the classification of this study.
We found significant variation for salt tolerance among a large collection of buffelgrass genotypes representing global diversity, USDA collection, commercial varieties and local landraces. We identified several accessions that were stable and productive at high salinity and other accessions whom were stable but low yielder. Accession 3, 133, 159, 30, 23, 142, 141, 95, 49, 129, 124, and 127 were stable, salt tolerant and produced good dry biomass yield at target salinities during all years. These accessions can be exploited to widen the genetic base of existing C. ciliaris accessions against salt tolerance. These accessions hold an appropriate salinity tolerance potential and can be grown to enhance farm productivity in arid and semi-arid areas of the globe. Furthermore; introduction of salt-tolerant perennial species is a promising alternatives to overcome salinity problems in the arid regions. The conservation of fresh water resources and its use for high value purposes, while using low quality saline water can provide both ecological and economic benefits, essential for sustainable agriculture in dry lands.
MH conducted the experiment, collected data, and drafted the manuscript. AA designed the experiment, followed upon data collection, provided support through the PCA statistical analysis and edited the manuscript.
The authors declare that the research was conducted in the absence of any commercial or financial relationships that could be construed as a potential conflict of interest.
The authors gratefully acknowledge the International Fund for Agricultural Development (IFAD), Arab Fund for Economic and Social Development (AFESD), and the Islamic Development Bank (IDB) for their financial support through several regional projects. We are highly grateful to Dr. Susan Robertson (ICBA, Dubai, UAE) for help in English language and grammar correction. We are thankful to Dr. Asad Qureshi (ICBA, Dubai, UAE) for help in critical revision of the article. We are extremely thankful to anonymous reviewers for the critical and valuable comments that have greatly improved the article.
Ahmad, F. (2010). Leptochloa Fusca cultivation for utilization of salt-affected soil and water resources in Cholistan desert. Rev. Soc. Nat. 22, 141–149. doi: 10.1590/S1982-45132010000100010
Angassa, A., and Baars, R. M. T. (2000). Ecological condition of encroached and non-encroached rangelands in Borana, Ethiopia. Afr. J. Ecol. 38, 321–328. doi: 10.1046/j.1365-2028.2000.00250.x
Arshad, M., Rao, A., Akbar, G., and Akhtar, M. (2000). Eco-physiological adaptability of Sporobolus iocladus, from Cholistan, Pakistan. Pak. J. Biol. Sci 2, 1604–1607. doi: 10.3923/pjbs.1999.1604.1607
Ayerza, R. (1981). Buffelgrass: Utilisation and Productivity of a Promising Grass. Buenos Aires: Hemisferio Sur.
Buldgen, A., and Francois, J. (1998). Physiological reactions to imposed water deficit by Andropogon gayanus cv. Bisquamulatus and Cenchrus ciliaris cv. Biloela in a mixed fodder crop. J. Agric. Sci. 131, 31–38. doi: 10.1017/S0021859698005462
Chatfield, C., and Collin, A. J. (1980). Introduction to Multivariate Analysis. New York, NY: Chapman and Hall in Association with Methuen.
Daniells, I. G., Holland, J. F., Young, R. R., Alston, C. L., and Bernardi, A. L. (2001). Relationship between yield of grain sorghum (Sorghum bicolor) and soil salinity under field conditions. Aust. J. Exp. Agric. 41, 211–217. doi: 10.1071/EA00084
Dawood, A., Kikuchi, A., Jatoi, S. A., Kiuchi, F., and Watanabe, K. N. (2009). Genetic variation in the chloroplast DNA (cpDNA) of Zingiberaceae taxa from Myanmar envisaged by the PCR-RFLP analysis. Ann. Appl. Biol. 155, 91–101. doi: 10.1111/j.1744-7348.2009.00322.x
Food Agriculture Organization of the United Nations (2012). FAO Statistical Yearbook 2012: World Food and Agriculture. Available online at: http://reliefweb.int/sites/reliefweb.int/files/resources/i2490e.pdf (Accessed March 12, 2014).
Fernando, A. L., Boléo, S., Barbosa, B., Costa, J., Sidella, S., Nocentini, A., et al. (2012). “Perennial grasses: environmental benefits and constraints of its cultivation in Europe,” in 20th European Biomass Conference and Exhibition, 18–22 June 2012 (Milano), 2092–2094.
Graham, T. W., and Humphreys, L. R. (1970). Salinity responses of cultivars of Buffelgrass (Cenchrus ciliaris L.). Aust. J. Exp. Agric. Anim. Husb. 10, 725–728.
Hacker, J. B., and Waite, R. B. (2001). Selecting buffelgrass (Cenchrus ciliaris) with improved spring yield in subtropical Australia. Trop. Grassl. 35, 205–210.
Hemming, D. F. (1961). The ecology of the coastal area of northern Eritrea. J. Ecol. 49, 55–78. doi: 10.2307/2257423
Hussain, M. I., Lyra, D. A., Farooq, M., Nikoloudakis, N., and Khalid, N. (2016). Salt and drought stresses in Safflower: a review. Agron. Sustain. Dev. 36, 4. doi: 10.1007/s13593-015-0344-8
IBPGR (1984). Forage and Browse Plants for Arid and Semiarid Africa. Kew: IBPGR/Royal Botanic Gardens.
Iqbal, M. S., Ghafoor, A., and Qureshi, A. S. (2010). Evaluation of Nigella sativa L. for genetic variation and ex-situ conservation. Pak. J. Bot. 42, 2489–2495.
Jaleel, C. A., Beemarao, S., Ramalingam, S., and Rajaram, P. (2008). Soil salinity alters growth, chlorophyll content and secondary metabolite accumulation in Catharanthus roseus. Turk. J. Biol. 32, 79–83.
Jorge, M. A. B., Van DE Wouw, M., Hanson, J., and Mohammed, J. (2008). Characterization of a collection of buffelgrass (Cenchrus ciliaris). Trop. Grassl. 42, 27–39.
Krishnamurthy, L., Serraj, R., Hash, C. T., Al-Dakheel, A. J., and Reddy, B. V. S. (2007). Screening sorghum genotypes for salinity tolerant biomass production. Euphytica 156, 15–24. doi: 10.1007/s10681-006-9343-9
Ksiksi, T. S., and El-Shaigy, N. O. (2012). Growth responses of nutrient-stressed Cenchrus ciliaris under carbon dioxide enrichment. J. Earth Sci. Clim. Change 3:127. doi: 10.4172/2157-7617.1000127
Lanza Castelli, S., Grunberg, K., Munoz, N., Griffa, S., Colomba, E. L., Ribotta, A., et al. (2010). Oxidative damage and antioxidants defenses as potential indicators of salt-tolerant Cenchrus ciliaris L. genotypes. Flora 205, 622–626. doi: 10.1016/j.flora.2010.04.004
Mseddi, K., Mnif, L., Chaied, M., Neffati, M., and Roux, M. (2004). Aboveground phytomass productivity and morphological variability of Tunisian accessions of Cenchrus ciliaris L. Afr. J. Range Forage Sci. 21, 49–55. doi: 10.2989/10220110409485833
Munns, R., Guo, J., Passioura, J. B., and Cramer, G. R. (2000). Leaf water status controls daytime but not daily rates of leaf expansion in salt-treated barley. Aust. J. Plant Physiol. 27, 949–957. doi: 10.1071/PP99193
Munns, R., and Tester, M. (2008). Mechanisms of salinity tolerance. Ann. Rev. Plant Biol. 59, 651–681. doi: 10.1146/annurev.arplant.59.032607.092911
Nawazish, S., Hameedm, M., and Naurin, S. (2006). Leaf anatomical adaptations of Cenchrus ciliaris L. from the Salt Range, Pakistan against drought stress. Pak. J. Bot. 38, 1723–1730.
Peng, Y. L., Gao, Z. W., Gao, Y., Liu, G. F., Sheng, L. X., and Wang, D. L. (2008). Ecophysiological characteristics of alfalfa seedlings in response to various mixed salt alkaline stresses. J. Integ. Plant Biol. 50, 29–39. doi: 10.1111/j.1744-7909.2007.00607.x
Pengelly, B. C., Hacker, J. B., and Eagles, D. A. (1992). The classification of a collection of buffelgrasses and related species. Trop. Grassl. 26, 1–6.
Ponsens, J., Hanson, J., Schellberg, J., and Moeseler, B. M. (2010). Characterization of phenotypic diversity, yield and response to drought stress in a collection of Rhodes grass (Chloris gayana Kunth) accessions. Field Crops Res. 118, 57–72. doi: 10.1016/j.fcr.2010.04.008
Qadir, M., Quillerou, E., Nangia, V., Murtaza, G., Singh, M., Thomas, R. J., et al. (2014). Economics of salt-induced land degradation and restoration. Nat. Res. Forum 38, 282–295. doi: 10.1111/1477-8947.12054
Richards, R. (1983). Should selection for yield in saline regions be made on saline or non-saline soils? Euphytica 32, 431–438. doi: 10.1007/BF00021452
Rodríguez, I. R., and Miller, G. L. (2000). Using a chlorophyll meter to determine the chlorophyll concentration, nitrogen concentration and visual quality of St. Augustine grass. Hortscience 35, 751–754.
Roy, S. J., Negrao, S., and Tester, M. (2014). Salt resistant crop plants. Curr. Opin. Biotechol. 26, 115–124. doi: 10.1016/j.copbio.2013.12.004
Shannon, M. C. (1997). Adaptation of plants to salinity. Adv. Agron. 60, 75–120. doi: 10.1016/S0065-2113(08)60601-X
Skerman, P. J., and Riveros, F. (1990). Tropical Grasses. FAO Plant Production and Protection Series No. 23. Rome: FAO.
Tester, M., and Davenport, R. J. (2003). Na+ transport and Na+ tolerance in higher plants. Ann. Bot. 91, 503–527. doi: 10.1093/aob/mcg058
Wang, Q., Wu, C., Xie, B., Liu, Y., Cui, J., Chen, G., et al. (2012). Model analysing the antioxidant responses of leaves and roots of switchgrass to NaCl-salinity stress. Plant Physiol. Biochem. 58, 288–296. doi: 10.1016/j.plaphy.2012.06.021
Warwick, S. I., Gugel, R. K., McDonald, T., and Falk, K. C. (2006). Genetic variation of Ethiopian mustard (Brassica carinata) germplasm in western Canada. Genet. Res. Crop Evol. 53, 297–312. doi: 10.1007/s10722-004-6108-y
Keywords: buffelgrass, biomass yield, multivariate analysis, salt tolerance, genotypic diversity
Citation: Al-Dakheel AJ and Hussain MI (2016) Genotypic Variation for Salinity Tolerance in Cenchrus ciliaris L. Front. Plant Sci. 7:1090. doi: 10.3389/fpls.2016.01090
Received: 02 April 2016; Accepted: 11 July 2016;
Published: 28 July 2016.
Edited by:
Teresa Donze, University of Nebraska–Lincoln, USACopyright © 2016 Al-Dakheel and Hussain. This is an open-access article distributed under the terms of the Creative Commons Attribution License (CC BY). The use, distribution or reproduction in other forums is permitted, provided the original author(s) or licensor are credited and that the original publication in this journal is cited, in accordance with accepted academic practice. No use, distribution or reproduction is permitted which does not comply with these terms.
*Correspondence: M. Iftikhar Hussain, bS5pZnRpa2hhckBiaW9zYWxpbmUub3JnLmFl; bWloNzg2QGdtYWlsLmNvbQ==
Disclaimer: All claims expressed in this article are solely those of the authors and do not necessarily represent those of their affiliated organizations, or those of the publisher, the editors and the reviewers. Any product that may be evaluated in this article or claim that may be made by its manufacturer is not guaranteed or endorsed by the publisher.
Research integrity at Frontiers
Learn more about the work of our research integrity team to safeguard the quality of each article we publish.