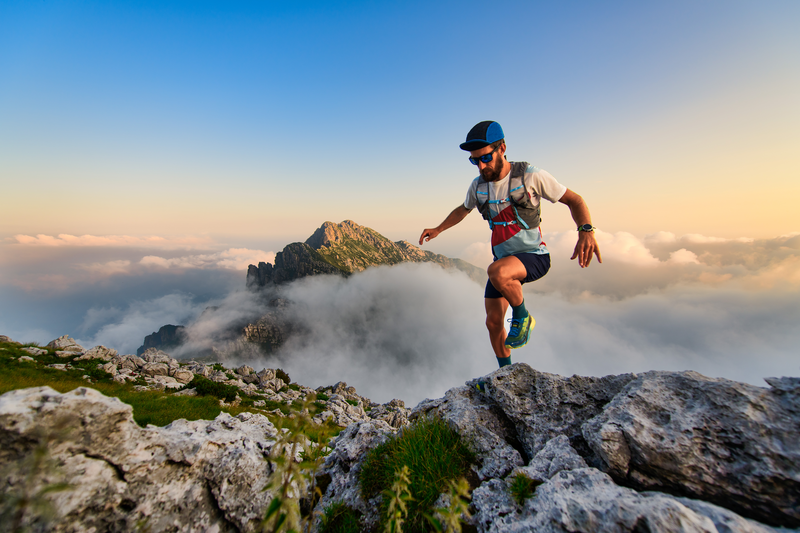
94% of researchers rate our articles as excellent or good
Learn more about the work of our research integrity team to safeguard the quality of each article we publish.
Find out more
ORIGINAL RESEARCH article
Front. Plant Sci. , 27 May 2016
Sec. Plant Nutrition
Volume 7 - 2016 | https://doi.org/10.3389/fpls.2016.00730
This article is part of the Research Topic Beneficial elements: novel players in plant biology for innovative crop production View all 11 articles
The low content of iodine (I) and selenium (Se) forms available to plants in soil is one of the main causes of their insufficient transfer in the soil-plant-consumer system. Their deficiency occurs in food in the majority of human and farm animal populations around the world. Both elements are classified as beneficial elements. However, plant response to simultaneous fertilization with I and Se has not been investigated in depth. The study (conducted in 2012–2014) included soil fertilization of carrot cv. “Kazan F1” in the following combinations: (1) Control; (2) KI; (3) KIO3; (4) Na2SeO4; (5) Na2SeO3; (6) KI+Na2SeO4; (7) KIO3+Na2SeO4; (8) KI+Na2SeO3; (9) KIO3+Na2SeO3. I and Se were applied twice: before sowing and as top-dressing in a total dose of 5 kg I⋅ha-1 and 1 kg Se⋅ha-1. No negative effects of I and Se fertilization were noted with respect to carrot yield. Higher accumulation and the uptake by leaves and storage roots of I and Se were obtained after the application of KI than KIO3, as well as of Na2SeO4 than Na2SeO3, respectively. Transfer factor values for leaves and roots were about a dozen times higher for Se than for I. Selenomethionine content in carrot was higher after fertilization with Na2SeO4 than with Na2SeO3. However, it was the application of Na2SeO3, KI+Na2SeO3 and KIO3+Na2SeO3 that resulted in greater evenness within the years and a higher share of Se from selenomethionine in total Se in carrot plants. Consumption of 100 g f.w. of carrots fertilized with KI+Na2SeO3 and KIO3+Na2SeO3 can supply approximately or slightly exceed 100% of the Recommended Daily Allowance for I and Se. Moreover, the molar ratio of I and Se content in carrot fertilized with KI+Na2SeO3 and KIO3+Na2SeO3 was the best among the research plots.
For the last few decades selenium (Se) has been considered as a beneficial element for plants – its indispensability for plants has not however been proved. Se can have a growth-promoting effect in many species of plants. Importantly, there is a strict relation between the uptake and metabolism of Se(IV) and P, as well as of Se(VI) and S (Kopsell and Kopsell, 2007; Zhang et al., 2013; Winkel et al., 2015).
The classification of iodine (I) into the group of beneficial elements for plants is not as unanimous as of selenium. There are known reports indicating its positive influence on higher plants (Lehr et al., 1958; Borst-Pauwels, 1961) as well as those underlining the lack of clear understanding and explanation of physiological role of I (Kabata-Pendias, 2011). Differences in iodine classification as a beneficial element result, among others, from the great difficulty in its determination – in our opinion, even greater than in the case of selenium. Another issue is diverse response of individual plant species to iodine application. Smoleń et al. (2016a) presented the hypothesis of genetic diversity with respect to plant reaction to iodine between the old traditional cultivars and those introduced as an effect of ‘green revolution.’ Separate problem are the differences in biomass productivity depending on iodine dose and chemical form: I- and IO3- (Blasco et al., 2008, 2012). There are, however, strong indications on positive influence of iodine on nitrogen use efficiency by plants (Blasco et al., 2012; Smoleń and Sady, 2012) or the improvement of tomato fruits (Kiferle et al., 2013; Smoleń et al., 2015).
What needs to be taken into account is the physiological role of I and Se for human and animals. The cause of insufficient transfer of I and Se in the soil-plant-consumer system is the low content of its available forms in the soil. It is also the reason for the limited supply of I and Se in food for several billion people and farm animals worldwide. It is estimated to affect nearly two-thirds of the global human population and is manifested by various diseases and health disorders (Hirschi, 2009; White and Broadley, 2009; Wu et al., 2015).
The relatively cheapest, while still effective way of counteracting the problem could be applying methods of agrotechnical plant biofortification (enrichment) with I and Se, and also with Zn and Fe, whose deficiency is highly widespread round the world as well (Cakmak, 2008; White and Broadley, 2009; Zhao and McGrath, 2009). In perspective, it also seems important to implement biotechnological methods of biofortification for developing new cultivars of plants with more efficient nutrient uptake from soils (White and Broadley, 2009). In comparison to Zn, Fe, and Se (White and Broadley, 2009; Singh et al., 2013; Wu et al., 2015), breeding programs aimed at developing genotypes with increased I content have not found common application (Lyons et al., 2004). Numerous studies, however, have been conducted on agrotechnical methods of plant biofortification/fertilization with individual application of I (Blasco et al., 2010; Kato et al., 2013; Lawson et al., 2015) or Se (Ríos et al., 2008, 2010; Hawrylak-Nowak et al., 2015; Hernández-Castro et al., 2015).
In many countries around the world, I prophylaxis is based on iodization of table salt. Due to the risk of developing various health problems associated with excessive salt consumption, the WHO has recently recommended searching for alternative ways of introducing I into food (WHO, 2004, 2014). In the WHO reports, however, the immense potential of plants with increased I content to support I deficiency prophylaxis was not recognized (WHO, 2014).
The implementation of agrotechnical methods of biofortification for combined fertilization with I and Se is difficult as neither element is a mineral nutrient for plants (Kopsell and Kopsell, 2007; Kabata-Pendias, 2011). In Finland and Malawi, nationwide agrotechnical programs for crop fertilization/biofortification with Se have been conducted (Eurola et al., 2003; Chilimba et al., 2012). On the other hand, enrichment with KIO3 of water used for watering fields in Xinjiang Province (China) is an isolated example of soil fertilization with I in agricultural practice (Ren et al., 2008).
In general, irrespective of the cultivation type (field, soilless, hydroponic), the iodide form (I-) is more rapidly taken up by roots, and at the same time is more toxic to plants than the iodate form (IO3-; Blasco et al., 2010; Kato et al., 2013). Some reports, however, have indicated better I absorption from soil after the application of IO3- than I- (Smoleń and Sady, 2012; Lawson et al., 2015). In part, this results from the varying preferences of individual plant species with respect to the uptake of I- or IO3- ions. Another factor, just as important, is the diverse physical and chemical properties of soils. They determine the sorption, speciation changes of I- and IO3- ions or their transformation into volatile I2 - I volatilization from soil (Yoshida et al., 1992; Schlegel et al., 2006; Pless et al., 2007). I uptake by roots is highly influenced by Cl concentration in the soil environment. There exists an antagonism between I and Cl, because chloride channels present in the cytoplasmic membrane of plant cells are easily permeable to iodides (Roberts, 2006).
In the case of Se, uptake preferences and plant toxicity of SeO42- and SeO32- also depend on the cultivation type – more precisely, on the environment in which the root system is developed. In the research of Ríos et al. (2008, 2010), the SeO42- form was characterized by better uptake and lower toxicity than SeO32- for lettuce plants cultivated in perlite. In hydroponic cultivation of cucumber the toxicity of the SeO32- form was higher than that of SeO42-, despite its lower accumulation in shoots and roots (Hawrylak-Nowak et al., 2015). In natural soils (in field and pot experiments) SeO42- ions are usually more easily taken up and at the same time more toxic to plants than SeO32- ions (Kopsell and Kopsell, 2007; Lavu et al., 2013; Li et al., 2015). This is caused by transformations they undergo in the soil environment, for example, the strong sorption of SeO32- ions with iron hydroxides [Fe(OH)3; Elrashidi et al., 1989; Kopsell and Kopsell, 2007]. The mobility and bioavailability of inorganic Se in the environment increase with pH, as well as with decreasing clay and iron oxide content in the soil (Winkel et al., 2015). The possibility of SeO32- and SeO42- uptake by plants from the soil is additionally regulated by plant availability of P and S. In plants, SeO32- ions are transported with the same transport proteins as phosphate, while SeO42- ions are transported with sulfate transporters (Winkel et al., 2015).
The aspects of I and Se interaction due to their simultaneous application have not yet been sufficiently investigated. It is still unknown whether or to what extent increased concentrations of both these elements in the soil environment affect plant growth, development and, finally, yield. Only a few studies in this area have been conducted so far, including hydroponic cultivation of spinach (Zhu et al., 2004) and lettuce (Smoleń et al., 2014). In field conditions, there was only a 1-year study conducted testing soil fertilization with Se+Zn+I in wheat, maize, soybean, potato, canola, and cabbage cultivation (Mao et al., 2014). There are, however, no results from field experiments carried out over more than 1 year documenting the effect of the simultaneous application of various chemical compounds of I and Se on plants.
The research hypothesis was that there is a possibility of increasing I and Se content in carrot plants by conducting simultaneous soil fertilization with mineral forms of these elements. Another one stated that simultaneous application of I and Se has a negative effect on the yield, as even small doses of both elements (not being plant nutrients) can be toxic to plants. It was also assumed that after plant fertilization with Se, a significant increase of organic forms of this element, including selenomethionine, would occur.
The research objective was to determine the effects of soil fertilization with different chemical forms of I (I- and IO3-) and Se (SeO32- and SeO42-) on crop yield, the efficiency of biofortification with these elements and selected chemical properties of carrot plants (Daucus carota L.).
In the years 2012–2014, a field study with carrot (Daucus carota L.) cv. “Kazan F1” cultivation was conducted in Marszowice near Kraków, Poland. Each year, carrot was cultivated on the same farm – on a different site within a single soil complex.
Carrot was cultivated on a heavy soil with a silty clay texture (Table 1). In 2012 and 2014 carrot was cultivated on the same field (with a total area of ~2 ha) but on its different parts characterized by various crop rotations within an area of 1 ha (coordinates: 50.1911481 N, 20.0902866 E, 306 masl). Each year, the preceding crop was wheat. Importantly, on the soil site on which carrot was grown in 2014, tobacco was cultivated in 2012, not carrot.
TABLE 1. Selected chemical properties of the 0–30 cm soil layer prior to the experiment in 2012–2014 (n = 4).
The value of soil pH was higher in 2013, as in that year carrot was cultivated on another field located about 500 m from the field used in 2012 and 2014 (coordinates: 50.1868649 N, 20.0925089 E, 306 masl). Despite the pH differences, soils from the fields under study were characterized by similar I and Se content in each year.
The study included soil fertilization with I and Se in the following combinations: (1) Control; (2) KI; (3) KIO3; (4) Na2SeO4; (5) Na2SeO3; (6) KI+Na2SeO4; (7) KIO3+Na2SeO4; (8) KI+Na2SeO3; (9) KIO3+Na2SeO3. I and Se were applied twice: before sowing (before ridge formation) and as a top-dressing – at canopy closure, each in a dose of 2.5 kg I⋅ha-1+ 0.5 kg Se⋅ha-1. The total amount of introduced I and Se was 5 kg I⋅ha-1 and 1 kg Se⋅ha-1, respectively. Pre-sowing fertilization with I and Se was conducted on April 12, 2012, April 18, 2013, and April 04, 2014, and top-dressing application on June 29, 2012, July 3, 2013 and July 4, 2014. I was applied as KI and KIO3 (puriss. p.a., Avantor Performance Materials, Gliwice, Poland), and Se as Na2SeO4 and Na2SeO3 (puriss. p.a., Sigma–Aldrich Co. LLC, St. Louis, MO, USA). The experiment was arranged in a split-plot design. Each treatment was randomized in four repetitions on 4 m × 6 m (24 m2) plots. The total area of the experiment was 864 m2.
One day prior to bed formation, based on the results of soil chemical analysis, pre-sowing fertilization with N, P, and K was conducted (along with I and Se application), in order to supplement the soil to the level optimal for carrot: N-100, P-80, and K-200 (in mgdm-3 of soil). N fertilization was applied as urea (Zakłady Azotowe “Puławy,” Puławy, Poland), P and N as ammonium phosphate (Grupa Azoty SA, Zakłady Chemiczne “Police,” Police, Poland) and K as 60% potassium salt (Zakład Obrotu Towarami Sp. z o. o., Dwikozy, Poland). Doses of these fertilizers in individual years, respectively, for 2012, 2013, and 2014, were as follows: urea 0.25, 0.10, and 0.25 t⋅ha-1, ammonium phosphate 0.80, 1.25, and 1.00 t⋅ha-1 as well as potassium salt 0.90, 1.10, and 1.00 t⋅ha-1.
Carrots were cultivated in one row on 40 cm wide and 30 cm high raised beds at a seeding rate of 37 seeds⋅m-1 (approximately 600,000 seeds per hectare). The seeds were sown on April 19, 2012, April 25, 2013, and April 05, 2014. The carrot roots were harvested on September 26, 2012, September 11, 2013, and September 9, 2014. During harvest, yield of carrot leaves and storage roots as well as plant density per hectare were determined. Marketable yield consisted of storage roots of cylindrical or close-to-cylindrical shape with a head diameter of ≥3 cm, undamaged by pests, not infected by fungi or bacteria, with no fractures and heads greened to a maximum of 0.5 cm. The length of a storage root was 15 cm minimum. At harvest, approximately 10 kg samples of carrot storage roots were chosen from each of the four plots (replications) for laboratory analysis. Only roots qualifying as marketable yield were taken for further chemical analysis.
During carrot harvest, soil samples from the layers 0–30 cm, 30–60 cm, and 60–90 cm were also collected separately for each of the research plots. Soil samples from each layer from each of the four plots (replications) were collected by soil drill (diameter 3 cm) for laboratory analysis. Additionally, before cultivation, eight individual samples from the whole area of the experimental fields were randomly collected (Table 1).
Samples of carrot leaves and storage roots were dried at 70°C in a laboratory dryer with forced air circulation and ground in a Pulverisette 14 Fritsch (Idar-Oberstein, Germany) variable speed rotor mill, using a 0.5 mm sieve. Samples thus prepared were subsequently analyzed with respect to the content of I and Se with an ICP-OES spectrometer (Prodigy, Leeman Labs, New Hampshire, MA, USA) and selenomethionine (SeMet) using a capillary electrophoresis (CE) analyzer PA 800 Plus CE system with DAD detection (Beckman Coulter, Indianapolis, IN, USA)
Iodine content in leaf and root samples was determined with the application of the cold vapor I2 generation (CVG) technique (Vtorushina et al., 2008, 2009). Air-dried plant samples (0.5 g) were digested in a mixture of super-pure 10 cm3 65% HNO3 (Merck, Whitehouse, Station, NJ, USA) and 0.8 cm3 70% HClO4 (Avantor Performance Materials, Gliwice, Poland) in a CEM MARS-5 Xpress (CEM World Headquarters, Matthews, NC, USA) microwave system using Teflon vessels. The process consisted of four steps with a gradual increase of temperature: 60, 80, and 100°C (each step involved 10 min of warming plus 5 min maintenance at the set temperature) and 130°C (10 min + 15 min). After digestion, solutions were transferred into a volume of 25 cm3 with redistilled water. Measurements using the CVG technique required the application of a gas/liquid separator using 30% hydrogen peroxide (Avantor Performance Materials, Gliwice, Poland) for isolating volatile I2 during measurement with an ICP-OES spectrometer (Vtorushina et al., 2008).
Selenium content was analyzed after sample digestion in nitric acid (Pałsawski and Migaszewski, 2006). Samples of air-dried carrot leaves and storage roots (0.5 g) were digested at 200°C (15 min of warming plus 15 min maintenance at the set temperature) in 10 cm3 65% super-pure HNO3 (Merck, Whitehouse, Station, NJ, USA) using a CEM MARS-5 Xpress microwave system. Samples were then transferred to the final volume of 25 cm3 using double-distilled water and analyzed with an ICP-OES spectrometer.
The SeMet content in leaf and root samples was determined using the following procedure. In 10 cm3 Falcon tubes, 5 cm3 of solution containing 40 mg protease and 20 mg lipase in demineralized water were added to 0.25 g of air-dried plant samples. The samples were incubated for 16 h at 20°C and centrifuged for 15 min at 4500 rpm (Zhao et al., 2011). The supernatants were filtered through 0.25 μm cellulose acetate membrane filters and analyzed using a CE analyzer with DAD detection, at 254 nm. A silica capillary tube with an i.d. of 75 μm, o.d. of 365 μm and a total length of 50 cm was used for the measurements. A negative power supply of -16 kV was applied. The running buffer solution was prepared as proposed by Zhao et al. (2011), containing 30 mmol NaH2PO4 (Avantor Performance Materials, Gliwice, Poland), 15 mmol Na2B4O7 (Sigma–Aldrich Co. LLC, St. Louis, MO, USA, puriss. p.a.) and 0.2 mmol CTAB (pH 8.80; Sigma–Aldrich Co. LLC, St. Louis, MO, USA, puriss. p.a.). Standards of L(+)-SeMet (Acros Organics, Geel, Belgium) were used for CE calibration.
Before carrot cultivation, soil samples were taken from the 0–30 cm layer, in order to characterize the physical and chemical properties of the soil site (Table 1). The results obtained are presented in Section “Plant Material and Treatments.” Prior to carrot cultivation, soil texture was analyzed using the Casagrande method modified by Pruszyński (Komornicki et al., 1991) as well as pH, total soil salinity (EC), redox potential (Eh), and the organic matter content. In soil samples mixed with water (1:2 v/v, soil:H2O), pH and Eh were measured potentiometrically and EC was analyzed using a conductivity meter. The organic matter in the soil was determined by the Tiurin method (Nowosielski, 1988; Komornicki et al., 1991).
Iodine and Se content in the soil before and after carrot cultivation was analyzed using the following procedure. Soil samples were dried at 70°C in a laboratory dryer with forced air circulation, ground in a mortar and sieved through a 1 mm sieve. Soil samples (2.5 g) were put into 30 cm3 Falcon tubes; 10 cm3 of double-distilled water and 1 cm3 of 25% TMAH (tetramethylammonium hydroxide - Sigma–Aldrich Co. LLC, St. Louis, MO, USA) were added. After mixing, the samples were incubated for 3 h at 90°C. After incubation, the samples were cooled to a temperature of approximately 20°C and filled to 30 cm3 with double-distilled water. After mixing, the samples were centrifuged for 15 min at 4500 rpm. The measurements, using an ICP-OES spectrometer (Prodigy, Leeman Labs, New Hampshire, MA, USA), were conducted in the supernatant (without decanting it). The method described above is our own modified procedure (Smoleń et al., 2016a) for the determination of total I (Yamada et al., 1996) and I and Se (McNally, 2011) content in soil using TMAH. The modification included the application of higher temperature (90°C, not 70°C) and omitting sample filtration after centrifugation. Filtration of samples leads to losses of analyzed elements. The temperature of 90°C is recommended for I determination after sample incubation with TMAH according to PN-EN 15111 (2008).
Analysis of the I and Se content in leaves and storage roots of carrot and soil was conducted using a ICP-OES Prodigy spectrometer (Prodigy, Leeman Labs, New Hampshire, MA, USA). Calibration of the instrument was performed by maintaining the same matrix as for the analyzed samples. For I determination in plant samples using the CVG technique, the most sensitive line of I: I-178.276 nm (with the detection limit of 0.5 μg I⋅dm-3) was chosen, as for this method no interference from P affects it (Vtorushina et al., 2009). After alkaline extraction of soil samples (using TMAH), the I-183.038 nm (with the detection limit for solution nebulization of app. 50 μg I⋅dm-3) line was used. For soil sample extraction with TMAH I-178.276 nm and I-206.163 spectral lines cannot be used due to the interferences from, respectively: P as well as Zn and Cr. The Se content in both the carrot (leaves and storage roots) and soil samples was analyzed using the Se-169.090 nm line.
Each year, carrot was grown from April to September. Meteorological data from dekadal (10 days) periods are presented including the mean daily air temperature, mean daily PAR value and total precipitation (Figure 1 – data from a HOBO Weather Station). From April to September, the total amount of rainfall was 293.4, 428.5, and 437.9 mm, whereas the mean daily air temperature was 16.1, 15.2, and 15.5°C in 2012, 2013, and 2014, respectively (Figure 1 – data from a HOBO Weather Station). The average daily PAR value in the period from carrot planting in field to the harvest was: 491.0, 465.3, and 569.6 μmo⋅lm-2⋅s-1 in 2012, 2013, and 2014, respectively.
For comparison, the mean air temperature for the period 1971–2000 from April to September was 14.3°C, and total precipitation was 435 mm (GUS, 2005). More precisely, in the years 1971–2000, the average monthly air temperature was 8.0°C in April, 13.4°C in May, 16.2°C in June, 17.8°C in July, 17.5°C in August, and 13.2°C in September. In the same period, the average monthly rainfall in the area under study was 50 mm in April, 74 mm in May, 94 mm in June, 81 mm in July, 76 mm in August, and 60 mm in September (GUS, 2005).
The most unfavorable weather conditions during carrot cultivation occurred in 2012 and mainly concerned the amount and distribution of rainfall (Figure 1). The total amount of rainfall from April to September 2012 was 141.6 mm lower, while in the years 2013–2014 it oscillated around the mean for the period of 1971–2000. A higher mean daily air temperature was also recorded from April to September 2012 when compared to the same period in 2013 and 2014 - it was 1.8°C higher than the mean from the years 1971–2000. That was caused by heat waves occurring in the summer months of 2012.
With respect to PAR, its highest total was noted in 2014, and at the same time it was the most evenly distributed throughout the entire period of carrot cultivation within all 3 years of the study (Figure 1). In the years 2012–2013, large fluctuations in PAR values in the subsequent dekadal periods from April to mid-August were recorded.
The I and Se transfer factor (TF) in the soil-plant (carrot leaves or storage roots) system was calculated using the following formula:
with C standing for I and Se content in plant/soil dry matter. Based on the results of crop yield measurement, I and Se determination (and dry weight) in carrot leaves and storage roots, values of I and Se uptake by leaves, storage roots and whole plants of carrot were calculated. Using the results of Se and SeMet content, the percentage ratio of Se present in SeMet in relation to the total Se content in the leaves and storage roots of carrot was calculated.
The percentage of Recommended Daily Allowance for I (RDA-I) and Se (RDA-Se) supplied from one serving of a 100 g portion of fresh carrot storage roots was calculated. In these calculations, results of I and Se determination in carrot as well as the recommended daily intake of these two elements: 150 μg I and 55 μg Se per day for adults were used (Food and Nutrition Board – Institute of Medicine, 2000; Andersson et al., 2007).
All data were subjected to analysis of variance using the ANOVA module of Statistica 10.0 PL. For determining the significance between the means, the Tukey test was used. The significance was declared at P < 0.05.
In the following years of the research, no statistically significant effect of I and Se fertilization was observed with respect to all measured yield parameters (Figures 2A–E). With the exception of the total yield of carrot storage roots, no significant interaction of treatments × year of study on tested yield parameters was demonstrated (Figure 2B). It was mostly affected by substantial differences in total yield of carrot storage roots in the subsequent years. In comparison to the control, the total yield of storage roots in 2012 decreased after the application of Na2SeO4 and Na2SeO3, while in other years remained at a level comparable to the control. Only in 2013, simultaneous soil application of I and Se compounds tended to lower the total yield of storage roots as compared to the control
FIGURE 2. Effect of iodine and selenium fertilization on yield: of leaves (A), total (B), and marketable yield (C) of storage roots as well as the biological yield of carrot (leaves and storage roots) (D), and the number of carrot plants per hectare (E) in the years 2012–2014. LSD, least significant difference; n.s., not significant, p < 0.05. Bars indicate standard error; (n = 4).
In 2012 and 2014, a lower planting density was observed than in 2013 (Figure 2E). However, only in 2012 the carrot leaves and root yield differed from the yield obtained in the years 2013–2014 (Figures 2A–D).
Fertilization with I and Se had a significant effect on the accumulation of I in carrot leaves and storage roots (Figures 3A,B), values of I TF for roots and leaves (Figures 3C,D) and I uptake (g I⋅ha-1) by leaves, storage roots and whole carrot plants (Figures 3E–G).
FIGURE 3. Iodine concentration in leaves (A) and storage roots (B), transfer factor (TF) of iodine to leaves (C) and storage roots (D) as well as iodine uptake by leaves (E), storage roots (F) and whole plants of carrot (G) in the years 2012–2014, depending on varying fertilization with iodine and selenium. LSD, least significant difference, p < 0.05. Bars indicate standard error; (n = 4).
Each year, the highest I content in carrot leaves and storage roots along with the highest I uptake was characterized by plants fertilized solely with KI (Figures 3A,B,E–G). In each year of the research, in combinations with KIO3 applied separately and together with Se, plants were fortified with I less effectively than when using KI (Figures 3A,B). This observation was further confirmed by lower I uptake by leaves and storage roots when using KIO3 rather than KI (Figures 3E,F). Simultaneous fertilization with KI/KIO3 and Se reduced I content and uptake by leaves and storage roots, in comparison to plants fertilized exclusively with I. To a greater extent, it applied to using the SeO32- form of Se.
Fertilization only with Se in the form of Na2SeO4 and Na2SeO3, as compared to the control, caused a substantial increase of I content in leaves and storage roots (Figures 3A,B) - however, much lower than after the application of KI and KIO3 separately and or with Se. After fertilization with Na2SeO4 and Na2SeO3, TF values for I uptake by leaves were at a level similar to or higher than after the application of KI and KIO3 separately and in combination with Se (Figure 3C). In storage roots, TF values for I in plants from Na2SeO4 and Na2SeO3 plots were lower than in plants treated with KI application (Figure 3D).
The highest accumulation, TF and I uptake by roots (Figures 3B,D,F), and the lowest content and TF of I by carrot leaves were noted in 2013, as compared to 2012 and 2014 (Figures 3A,C).
In comparison to the control and plots with the application of only I, in each year of the research, a significant increase of Se accumulation, TF values for Se, Se uptake and SeMet content in carrot leaves and storage roots were noted in plants from the plots with Se and Se+I (Figures 4A–G and 5A,B). In plants fertilized with Na2SeO4 separately or with KI/KIO3, the values of these parameters were up to several dozen times higher than in plants fertilized with Na2SeO3 in the respective combinations.
FIGURE 4. Selenium concentration in leaves (A) and storage roots (B), transfer factor (TF) of selenium to leaves (C) and storage roots (D) as well as selenium uptake by leaves (E), storage roots (F) and whole plants of carrot (G) in the years 2012–2014, depending on varying fertilization with iodine and selenium. LSD, least significant difference, p < 0.05. Bars indicate standard error; (n = 4).
FIGURE 5. Selenomethionine (SeMet) concentration in leaves (A) and storage roots (B) as well as the percentage of total selenium present in SeMet for carrot leaves (C) and storage roots (D) in the years 2012–2014, depending on varying fertilization with iodine and selenium. LSD, least significant difference, p < 0.05. Bars indicate standard error; (n = 4).
In relation to the control, fertilization with KI or KIO3 alone caused a considerable increase of Se content in storage roots – with the exception of KI application in 2014 (Figure 4B). In the case of leaves, a similar effect of soil fertilization with KI and KIO3 was noted only in 2014 (Figure 4A).
For each year of the research, diverse effects of relations between the application of Na2SeO4 alone or together with KI and KIO3 were noted with respect to Se content, Se TF values, Se uptake and SeMet content in carrot leaves and storage roots (Figures 4A–G and 5A,B). Conversely, fertilization with KI+Na2SeO3 and KIO3+Na2SeO3, compared to the application of Na2SeO3 alone, considerably lowered Se content, TF values and uptake - to a greater extent after the application of the I- form of I (Figures 4A–G). With respect to SeMet content, no significant interaction of KI and KIO3 with Na2SeO3 in relation to fertilization with Na2SeO3 alone was, however, observed (Figures 5A,B).
The percentage of total Se found in SeMet calculated for carrot leaves and storage roots (further expressed as “Se-SeMet in total Se”) was the parameter with the highest variability within the years (Figures 5C,D). Its values for leaves were significantly higher than for roots and varied from 18.4% (combination with Na2SeO4 in 2014) to 96.3% (KI+Na2SeO3 in 2014). In storage roots, selenium from selenomethionine accounted for between 16.2% (KIO3+Na2SeO4 in 2014) and 91.8% (KIO3+Na2SeO3 in 2014) of total Se. During the entire 3-year research period a substantial decrease in Se-SeMet in total Se for both leaves and roots was noted after fertilization with Na2SeO4 (and KI+Na2SeO4 and KIO3+Na2SeO4 in 2013 and 2014 – Figures 5C,D).
Soil fertilization with I and Se compounds substantially increased the percentage of the RDA-I and RDA-Se supplied by the intake of 100 g of fresh carrot roots (Figures 6A,B). The RDA-I and RDA-Se values for plants in each plot, and between them, were a highly varied characteristic throughout the research years.
FIGURE 6. Percentage of Recommended Daily Allowance (RDA) for iodine (A) and selenium (B) in 100 g portion of fresh carrot roots in the years 2012–2014, depending on varying fertilization with iodine and selenium. The dotted line represents a break (shortening) of the scale on the Y-axis between 170 and 470. LSD, least significant difference, p < 0.05. Bars indicate standard error; (n = 4).
In the case of I, a single portion of 100 g f.w. of carrot fertilized with KI in each research year (as well as KI+Na2SeO4 and KI+Na2SeO4 in 2013) would supply more I than recommended for adults (Figure 6A). In 2013, due to the application of KIO3 alone, a greater than 100% supply of RDA-I by a single portion of carrot was noted. It is significant that I content in a single portion of carrot fertilized with KIO3 together with Se (irrespective of its chemical form) every year would supply less than 100% of RDA-I.
In the case of Se, each year a single portion of 100 g f.w. of carrot fertilized with Na2SeO4, KI+Na2SeO4 and KIO3+Na2SeO4 would supply Se in a supraoptimal quantity - from 500 to 650% RDA-Se for adults (Figure 6B). The use of Na2SeO3 for biofortification purposes (applied separately and combined with I) affected RDA-Se to a much smaller extent than Na2SeO4 but its values still oscillated around or exceeded 100%.
It should be underlined that soil fertilization solely with KI or KIO3 also caused a significant increase of about 12% of daily consumer allowance of Se supplied with a single portion of fresh carrots when compared to the control – the relation was noted in 2012 and 2013 (Figure 6B).
In the years 2012–2014, a highly varied effect of I and Se fertilization on the content of these two elements in soil after carrot cultivation was noted (Figures 7A,B). The highest I and the lowest Se concentrations in soil (total in all layers) were determined in 2012, whereas in 2014 the opposite relations were observed.
FIGURE 7. Concentration of iodine (A) and selenium (B) in 0–30 cm, 30–60 cm, and 60–90 cm soil layers after carrot cultivation in the years 2012–2014, depending on varying fertilization with iodine and selenium compounds. LSD, least significant difference, p < 0.05. Bars indicate standard error; (n = 4).
In each research year, I content in soil from plots fertilized with this element exceeded that in the control and combinations treated with Se alone (Figure 7A). It was highest in the 0–30 cm layer and lowest in the 60–90 cm layer. Similarly in the case of Se, soil fertilized with this element accumulated more Se than from the control combination and those fertilized with KI and KIO3 (Figure 7B). The content of Se in each layer was in the following order: 0–30 cm > 30–60 cm > 60–90 cm (Figure 7B).
In the study conducted by Smoleń et al. (2016b) with lettuce cultivation according to the same experimental design, substantial symptoms of Na2SeO4 toxicity followed by lower lettuce yield was noted irrespective of additional application of iodine. Therefore it may be concluded that carrot is a species less sensitive, than lettuce, to the SeO42- form of Se. The cause of such an observation may be the lower Se accumulation in carrot plants (two to three times lower than in lettuce leaves – Smoleń et al., 2016b).
The lack of negative response of carrot plants (in terms of yield) to I fertilization may be the consequence of its tolerance to iodine. In a pot experiment carried out in a greenhouse, Dai et al. (2004) did not observe symptoms of toxicity nor reduction of carrot biomass in reaction to increasing doses of KIO3 fertilization (0, 1, and 5 mg I⋅kg-1 of soil) as opposed to spinach, pak choi and celery. The biochemical and physiological mechanisms responsible for such diverse plant responses to I are not, however, known.
In the present study, iodine uptake by carrot plants was substantially improved when this element was applied as I- than IO3-. Similar observations were noted for lettuce cultivated in perlite (Blasco et al., 2008). When comparing the absolute values of I TF for carrot with results from other studies, higher values of this parameter for IO3- were noted in the present study than shown by Dai et al. (2004) for carrot grown for 13 weeks in pots located in a greenhouse. This discrepancy may have been caused by the longer time of carrot cultivation in field, different type of soil and the choice of various cultivars.
Generally, the values of I and Se TF in carrot roots (and leaves) can vary to a large extent depending on the cultivation site (climatic zone) and physical and chemical properties of soils (Dai et al., 2004; Uchida et al., 2009; Jolly et al., 2013). An important problem with comparing values of I and Se TF is the diversity of analytical procedures for I and Se determination in soil (substrates in soilless cultures) applied by other authors (Dai et al., 2004; Blasco et al., 2008; Uchida et al., 2009; Jolly et al., 2013). Depending on the procedure (extraction, incubation, or digestion), I or Se forms of various solubility and availability to plants are determined (Yamada et al., 1996, 1999; McNally, 2011; Shetaya, 2011). The methodology of plant analysis is also of some importance, which in the end leads to obtaining different values of I and Se TF. The results of our research indicate that for both KI and KIO3, I TF values for leaves were lower than for roots. In the case of Se, this relation was noted only for Na2SeO4, whereas for Na2SeO3 the respective values of TF remained at a similar level.
The dose of Se used for fertilization (1 kg Se⋅ha-1) was five times lower than of I (5 kg I⋅ha-1). Still, irrespective of the research plot, the values of Se TF values for leaves and storage roots were higher than for I. This indicates considerably higher Se mobility, and therefore availability, to plants in the soil environment, since I undergoes very strong sorption in soil (Yoshida et al., 1992; Schlegel et al., 2006; Pless et al., 2007). The described relation is further confirmed by the values of Se and I uptake by leaves and roots. With a five times higher I than Se dose, the largest noted level of I uptake (leaves + roots) was 450 g I⋅ha-1 (for KI applied in 2013). In the case of Se, the total Se uptake value (leaves + roots) reached values from 400 g Se⋅ha-1 in 2012 to 700 g Se⋅ha-1 in 2014 (both for Na2SeO4). Therefore, the uptake of Se by carrot plants was much more effective than of I.
The transport of SeO32- ions in plants occurs using the same protein transporters as of phosphate ions, while for SeO42-, sulfate carriers are engaged (Winkel et al., 2015). Our studies revealed that in combinations fertilized with Na2SeO3 (alone or together with KI/KIO3) the correlation coefficient values for Se and P content in leaves and roots were -0.37* and 0.58*, respectively. This may suggest the inhibition of phosphate transport from roots to leaves under the influence of SeO32-. On the other hand, after the application of Na2SeO4 (alone or with KI/KIO3), Se and S content was negatively correlated, with correlation coefficient values of -0.53* and -0.86*, respectively, for leaves and roots. The negative impact of Na2SeO4 on sulfur content in plants did not, however, affect the biomass productivity, possibly due to the low requirements of this species toward S. The results of S and P determination as well as of other macro- and micro-nutrients in carrot plants will be the subject of other publications.
From our study we may assume that reduced I uptake in 2012 was probably a consequence of lower precipitation volume than in the years 2013–2014. In this context, however, the results of Se determination in soil after carrot cultivation are surprising. Its highest content was noted in 2014, while the lowest was in 2012, which was accompanied by the smallest Se uptake by carrot plants. These results indicate that in that latter year Se underwent the highest soil sorption, which reduced its uptake by plants.
Even more surprising were the extremely different concentrations of I and Se in 2012 and 2014, as in these years carrot was cultivated on various parts of the same field. Most probably, diversity with respect to pH, EC and the content of I, Se and organic matter along with diverse weather conditions in 2012–2014 affected these changes in I and Se uptake by plants. Another affecting factor could include speciation processes of I and Se that occur in soil, sometimes leading to its volatilization. We did not, however, study this problem. Additionally, overall soil diversity as well a varying history of cultivation of two separate parts of the field in 2012 and 2014 may have contributed to obtained differences in I and Se content in soil.
There is also no certainty which form of I and Se is analyzed in soil after sample incubation in TMAH. According to Yamada et al. (1996), this method allows determination of the total content of I in soil if the incubation is conducted for 3 h at 90°C. McNally (2011) stated that a similar method of sample incubation with TMAH also allows analysis of the total concentration of I in soil, but not of Se. Ponce de León et al. (2003) demonstrated high applicability of TMAH extraction for Se analysis in soil. Yamada et al. (1999) noted that sample incubation with TMAH conducted for 4 h at room temperature extracts I bound with humic acids. According to Shetaya (2011) sample extraction with TMAH allows analysis of partial or total content of I in soils. Additionally, this author noted that the conditions of incubation including changes of TMAH concentration, extraction time, temperature, or soil particle size did not affect the results of I determination. In our opinion the applied procedure of sample incubation with TMAH provides information of the total absorbed content of I and Se in soil rather than their direct availability to plants in mineral form.
Results of our study indicate that climatic conditions may have a substantial influence on obtaining diverse plant reaction to simultaneous application of I and Se. It cannot be clearly stated which of the monitored weather parameters (with its values varying in the years 2012–2014) contributed mostly to observed changes. Such effect can be determined only in studied conducted in strictly controlled conditions, e.g., in growth chambers
Both tested elements are expelled via leaves through methylation (Rhew et al., 2003; Winkel et al., 2015). We assume that due to the higher values of soil-to-plant TF of Se than I, metabolic pathways responsible for Se methylation were more intensively activated. An increase of I methylation after the application of KI or KIO3 alone was also probable. This translated into a diverse effect of fertilization with I or Se alone on the accumulation of Se and I, respectively, in carrot leaves and storage roots. Additionally, the rate of I and Se accumulation in plants was different in each year of the research. In the research by Smoleń et al. (2014) with hydroponic cultivation of lettuce, the introduction of IO3- or SeO42- into the nutrient medium had no impact on root uptake and further plant distribution of SeO42- and IO3-, respectively. Similar results were obtained in a field cultivation of lettuce (Smoleń et al., 2016b). Also, foliar spraying with IO3- and SeO42- did not affect root uptake of Se and I from the nutrient medium in carrot cultivation (Smoleń et al., 2014).
After uptake, SeO32- ions in roots are converted into organoselenium compounds (mainly Se-amino acids) and transported in that form to leaves. SeO42- ions, however, after root uptake, are firstly transported through the xylem to stems and then to leaves, in which they are reduced to SeO32-. The reduction process is followed by the synthesis of Se-amino acids, e.g., selenocysteine (SeCys) and SeMet. Biosynthesis of organoselenium compounds from SeO42- ions (after previous SeO32- reduction) does not occur in roots, or has a marginal effect, in comparison to Se-amino acid synthesis directly from SeO32- (Zhu et al., 2009; Longchamp et al., 2015; Winkel et al., 2015).
Kápolna et al. (2009), with the use of HPLC–ICP–MS, observed that, regardless of the selenium form (SeO32- or SeO42-) administered to plants, the dominant organoselenium compounds in the roots of carrot grown in pots were SeMet and γ-glutamyl-selenomethyl-selenocysteine, while the only one detected in carrot leaves was SeMet. They also demonstrated the presence of free SeO32- or SeO42- ions in carrot leaves and roots, respectively, for the form used in foliar feeding. Their concentration in leaves and roots (expressed as μg Se⋅g-1 d.m.) was from several to about a dozen times lower than that of SeMet, and higher (but only in roots) than of γ-glutamyl-selenomethyl-selenocysteine. In our research, we managed to determine only the content of SeMet in carrot leaves and storage roots. With our CE analyzer with DAD detection, we did not reveal the presence of any free SeO32- or SeO42- ions in carrot leaf and storage roots of plants from any combinations of the study (a detailed description of the methodology has been omitted in Section “Plant Analysis”). It can thus be stated that in carrot leaves and storage roots, Se was present in an organic form, with the dominant one being SeMet. An exception was the relatively lower, than in other plots, percentage of Se from SeMet in total Se in leaves and roots of plants fertilized with Na2SeO4 (alone and combined with KI and KIO3), as noted in 2013 and 2014. With no detection of free SeO42- ions, these results indicate that for more favorable weather conditions (occurring in the years 2013–2014 in relation to 2012), more SeO42- ions were transformed into organoselenium compounds other than SeMet, e.g., γ-glutamyl-selenomethyl-selenocysteine or products of the conversion of SeMet or SeCys into volatile selenocompounds: dimethyl selenide and dimethyl diselenide (Zhu et al., 2009; Winkel et al., 2015).
A basic parameter crucial for assessing the efficiency of simultaneous biofortification of plants with I and Se is RDA, which for these elements is 150 μg I and 55 μg Se for adults, and 200–300 μg I and 60–70 μg Se for pregnant and lactating women, respectively (Food and Nutrition Board – Institute of Medicine, 2000; Andersson et al., 2007). Taking these values into account, the optimum mass ratio of I:Se in food is therefore within 2.7–5.5:1. However, considering the molar mass of both elements, the optimum ratio of I:Se stays within 4.4–8.8:1. Molar ratio seems to be a more reliable indicator applicable in assessing the efficiency of biofortification regarding consumer demand for these nutrients.
In the 3-year research period, the molar ratio of I:Se in a single portion of 100 g f.w. of carrot from the respective research plots was within the following values: (1) Control (2.2–3.9:1), (2) KI (29.4–59.3:1), (3) KIO3 (8.4–42.1:1), (4) Na2SeO4 (0.06–0.009:1), (5) Na2SeO3 (0.32–0.65:1), (6) KI+Na2SeO4 (0.45–1.4:1), (7) KIO3+Na2SeO4 (0.31–0.61:1), (8) KI+Na2SeO3 (2.9–4.1:1), (9) KIO3+Na2SeO3 (1.6–4.4:1). According to this data, even in control plants the molar ratio of I:Se differs from the optimal for consumer need. It should be noted that for that combination the obtained RDA-I values ranged from 6.14% in 2014 to 7.6% in 2012, and of RDA-Se from 8.4% in 2012 to 41.2% in 2014. These results indicate a greater capacity of the control carrot to satisfy consumer demand for Se than I – increasing with the natural content of I and Se in soil.
The most promising results in terms of biofortification purposes were obtained for simultaneous fertilization of Na2SeO3 with KI or KIO3. Not only did the values of RDA-I and RDA-Se oscillate, or slightly exceeded 100%, but the molar ratio of I:Se content in carrot was the closest to optimal – as compared to other combinations. Also, in the above-mentioned research by Smoleń et al. (2016b) with field cultivation of lettuce after the application of KI+Na2SeO3 and KIO3+Na2SeO3, it was noted that a more optimal ratio of I:Se was obtained in plants fertilized simultaneously with Na2SeO3 and KI or KIO3 than after the application of Na2SeO4 with KI/KIO3.
Future research in this area needs to be expanded to establish the possibility of balancing daily human diets of I and Se by biofortified crop plants. Additionally, it is necessary to investigate the assimilability of these elements by consumers. Tonacchera et al. (2013) demonstrated that after the consumption of vegetables biofortified with I (potatoes, cherry tomatoes, carrots, and green salad), urinary I excretion, one of the major indicators of I status of the human population, increased. Kopeć et al. (2015) revealed higher I assimilability and improvement of this element’s metabolism in rats fed with I-enriched lettuce in relation to those fed with control lettuce and a diet containing mineral KI. In the research by Koronowicz et al. (2016), the inhibition of Caco-2 cancer cell proliferation was demonstrated after treatment with I-biofortified lettuce extract, but not KI as well as I-biofortified lettuce-mediated induction of mitochondrial apoptosis and/or cell differentiation. This study showed 1326 differently expressed Caco-2 transcripts after treatment of biofortified and non-fortified lettuce extract.
A common health problem is I and Se deficiency in the human and animal population. The increase of I and Se content in plants tissues through various strategies of plant biofortification can be included as one of the ways of innovative crop production. Simultaneous plant enrichment with I and Se stays within the scope of functional food production. Both tested elements are not essential for plants. The results of conducted studies widened the knowledge on its interaction in plants, at the same time proposing the implementation of simultaneous plant fertilization with I and Se into the agricultural practice, mostly in the areas with endemic deficiency of both beneficial elements.
Soil fertilization with KI, rather than KIO3, contributed to greater uptake and accumulation of I. For Se, it was the introduction of Na2SeO4 into the soil that improved Se uptake and increased its content, also in the organic form of SeMet, in carrot leaves and roots. Fertilization with Na2SeO3, however, stimulated the processes of Se conversion into an organic form, which was reflected by the increased percentage of total Se present in SeMet.
An interaction between I and Se with respect to the rate of accumulation of both beneficial elements was revealed. The clearest relation concerned the negative effect of Se (mainly Na2SeO3) on I content and uptake. The reverse interaction describing the limiting influence of I on Se uptake and accumulation was most distinctive when KI and Na2SeO3 were applied together. Interaction of Na2SeO4 with I with respect to Se uptake, TF values and the content of SeMet was far more diverse within the years of the study.
Our research has demonstrated the possibility of conducting simultaneous soil fertilization with both beneficial elements (I and Se) without the risk of reducing the carrot crop yield. The applied doses of I and Se increased I and Se enrichment of carrot to a level exceeding the possibility of balancing the diet with respect to RDA-I and RDA-Se. In further studies conducted on soils with low content of I and Se as well as in agricultural production, lower doses of both elements should be applied in order to avoid the excessive intake of I and Se with biofortified carrots by both humans and animals.
Combined soil fertilization with I+Se needs to be applied in areas with insufficient concentration of both beneficial elements in soils, and thus in plants/food. Because of higher TF values for Se than I, Se doses for fertilization should be significantly lower than those of I. Despite the lower uptake of Se from Na2SeO3, application of this compound (and not Na2SeO4) gave better results in terms of the percentage ratio of Se from SeMet in total Se content in carrot plants.
When cultivating plants on soils intrinsically rich in I and/or Se, additional introduction of I or Se can be conducted but in much lower doses, in order to ensure proper balancing of both beneficial elements content in plants and food. Particular attention should be paid to maintaining the optimal molar ratio of I:Se in plants within the values of: 4.4–8.8:1.
SS: Leader of the project, author of the method of carrot biofortification with iodine and selenium, coordinator of field experiments and laboratory analyses, conducted the analysis of results and prepared the manuscript; ŁS: conducted field experiments with carrot cultivation; conducted iodine and selenium analysis using ICP-OES; IL-S: co-founder of the project; involved in the preparation of the research project, author of the method of iodine biofortification of carrot, conducted statistical analysis of results; helped prepare the manuscript; RR: involved in conducting field experiment with carrot cultivation; conducted laboratory analyses using capillary electrophoresis; AKop: helped conducting carrot cultivation; prepared soil and plant samples. EP: helped conducting carrot cultivation; prepared soil and plant samples. RB-K: helped conducting carrot cultivation; prepared soil and plant samples. AKor: helped conducting carrot cultivation; prepared soil and plant samples. JK-D: helped conducting carrot cultivation; prepared soil and plant samples.
This work was financed by the Polish National Science Center – grant no. DEC-2011/03/D/NZ9/05560 “I and Se biofortification of selected vegetables, including the influence of these microelements on yield quality as well as evaluation of iodine absorption and selected biochemical parameters in rats fed with vegetables biofortified with iodine” planned for 2012–2015.
The authors declare that the research was conducted in the absence of any commercial or financial relationships that could be construed as a potential conflict of interest.
We would like to thank Mr. Mariusz Doniec for allowing us to conduct studies with carrot cultivation on his farm.
Andersson, M., de Benoist, B., Darnton-Hill, I., and Delange, F. (2007). Iodine Deficiency in Europe: A Continuing Public Health Problem. Geneva: World Health Organization.
Blasco, B., Rios, J. J., Cervilla, L. M., Sanchez-Rodriguez, E., Ruiz, J. M., and Romero, L. (2008). Iodine biofortification and antioxidant capacity of lettuce: potential benefits for cultivation and human health. Ann. Appl. Biol. 152, 289–299. doi: 10.1111/j.1744-7348.2008.00217.x
Blasco, B., Rios, J. J., Leyva, R., Cervilla, L. M., Sanchez-Rodriguez, E., Rubio-Wilhelmi, M. M., et al. (2010). Does iodine biofortification affect oxidative metabolism in lettuce plants? Biol. Trace Elem. Res. 142, 831–842. doi: 10.1007/s12011-010-8816-9
Blasco, B., Ríos, J. J., Sánchez-Rodríguez, E., Rubio-Wilhelmi, M. M., Leyva, R., Romero, L., et al. (2012). Study of the interactions between iodine and mineral nutrients in lettuce plants. J. Plant Nutr. 35, 1958–1969. doi: 10.1080/01904167.2012.716889
Borst-Pauwels, G. W. F. H. (1961). Iodine as a micronutrient for plants. Plant Soil 14, 377–392. doi: 10.1007/BF01666295
Cakmak, I. (2008). Enrichment of cereal grains with zinc: agronomic or genetic biofortification? Plant Soil 302, 1–17. doi: 10.1007/s11104-007-9466-3
Chilimba, A. D. C., Young, S. D., Black, C. R., Meacham, M. C., Lammel, J., and Broadley, M. R. (2012). Assessing residual availability of selenium applied to maize crops in Malawi. Field Crops Res. 134, 11–18. doi: 10.1016/j.fcr.2012.04.010
Dai, J. L., Zhu, Y. G., Zhang, M., and Huang, Y. Z. (2004). Selecting iodine-enriched vegetables and the residual effect of iodate application to soil. Biol. Trace Elem. Res. 101, 265–276. doi: 10.1385/BTER
Elrashidi, M. A., Adriano, D. C., and Lindsay, W. L. (1989). “Solubility, speciation, and transformation of selenium in soils,” in Selenium in Agriculture and the Environment, ed. L. W. Jacobs (Madison, WI: Soil Science Society of America and American Society of Agronomy), 51–64.
Eurola, M., Alfthan, G., Aro, A., Ekholm, P., Hietaniemi, V., Rainio, H., et al. (2003). Results of the Finnish Selenium Monitoring Program 2000–2001. Jokioinen: MTT Agrifood Research Finland.
Food and Nutrition Board – Institute of Medicine (2000). Dietary Reference Intakes for Vitamin C, Vitamin E, Selenium, Carotenoids. Washington, DC: National Academy Press.
GUS (2005). Environment Protection (2005). Information and Statistical Analysis. Warsaw: Central Statistical Office of Poland.
Hawrylak-Nowak, B., Matraszek, R., and Pogorzelec, M. (2015). The dual effects of two inorganic selenium forms on the growth, selected physiological parameters and macronutrients accumulation in cucumber plants. Acta Physiol. Plant. 37, 41. doi: 10.1007/s11738-015-1788-9
Hernández-Castro, E., Trejo-Téllez, L. I., Gómez-Merino, F. C., Rodríguez-Mendoza, M. N., Sánchez-García, P., and Robledo-Paz, A. (2015). Bioaccumulation of iron, selenium, nitrate, and proteins in chard shoots. J. Soil Sci. Plant Nutr. 15, 694–710. doi: 10.4067/S0718-95162015005000047
Hirschi, K. D. (2009). Nutrient biofortification of food crops. Annu. Rev. Nutr. 29, 401–421. doi: 10.1146/annurev-nutr-080508-141143
Jolly, Y. N., Islam, A., and Akbar, S. (2013). Transfer of metals from soil to vegetables and possible health risk assessment. Springerplus 2, 385. doi: 10.1186/2193-1801-2-385
Kápolna, E., Hillestrøm, P. R., Laursen, K. H., Husted, S., and Larsen, E. H. (2009). Effect of foliar application of selenium on its uptake and speciation in carrot. Food Chem. 115, 1357–1363. doi: 10.1016/j.foodchem.2009.01.054
Kato, S., Wachi, T., Yoshihira, K., Nakagawa, T., Ishikawa, A., Takagi, D., et al. (2013). Rice (Oryza sativa L.) roots have iodate reduction activity in response to iodine. Front. Plant Sci. 4:227. doi: 10.3389/fpls.2013.00227
Kiferle, C., Gonzali, S., Holwerda, H. T., Real Ibaceta, R., and Perata, P. M. (2013). Tomatofruits: a good target for iodine biofortification. Front. Plant Physiol. 4:205. doi: 10.3389/fpls.2013.00205
Komornicki, T., Oleksynowa, K., Tokaj, J., and Jakubiec, J. (1991). Guide for Soil Science and Geology Experiments. Part 2. Methods of Soil Analysis. Kraków: Agricultural University in Kracow.
Kopeć, A., Piątkowska, E., Bieżanowska-Kopeć, R., Pysz, M., Koronowicz, A., Kapusta-Duch, J., et al. (2015). Effect of lettuce biofortified with iodine by soil fertilization on iodine concentration in various tissues and selected biochemical parameters in serum of Wistar rats. J. Funct. Foods 14, 479–486. doi: 10.1016/j.jff.2015.02.027
Kopsell, D. A., and Kopsell, D. E. (2007). “Selenium,” in Handbook of Plant Nutrition, eds A. V. Barker and D. J. Pilbeam (Boca Raton, FL: CRC Press), 515549.
Koronowicz, A. A., Kopeć, A., Master, A., Smoleń, S., Piątkowska, E., Bieżanowska-Kopeć, et al. (2016). Transcriptome profiling of Caco-2 cancer cell line following treatment with extracts from iodine-biofortified lettuce (Lactuca sativa L.). PLoS ONE 11:e0147336. doi: 10.1371/journal.pone.0147336
Lavu, R. V. S., De Schepper, V., Steppe, K., Majeti, P. N., Tack, F., and Du Laing, G. (2013). Use of selenium fertilizers for production of Se-enriched Kenaf (Hibiscus cannabinus): effect on Se concentration and plant productivity. J. Plant Nutr. Soil Sci. 176, 634–639. doi: 10.1002/jpln.201200339
Lawson, P. G., Daum, D., Czauderna, R., Meuser, H., and Härtling, J. W. (2015). Soil versus foliar iodine fertilization as a biofortification strategy for field-grown vegetables. Front. Plant Sci. 6:450. doi: 10.3389/fpls.2015.00450
Lehr, J. J., Wybenga, J. M., and Rosanow, M. (1958). Iodine as a micronutrient for tomatoes. Plant Phys. 33, 421427. doi: 10.1104/pp.33.6.421
Li, J., Liang, D., Qin, S., Feng, P., and Wu, X. (2015). Effects of selenite and selenate application on growth and shoot selenium accumulation of pak choi (Brassica chinensis L.) during successive planting conditions. Environ. Sci. Pollut. Res. Int. 22, 11076–11086. doi: 10.1007/s11356-015-4344-7
Longchamp, M., Castrec-Rouelle, M., Biron, P., and Bariac, T. (2015). Variations in the accumulation, localization and rate of metabolization of selenium in mature Zea mays plants supplied with selenite or selenate. Food Chem. 182, 128–135. doi: 10.1016/j.foodchem.2015.02.137
Lyons, G. H., Stangoulis, J. C., and Graham, R. D. (2004). Exploiting micronutrient interaction to optimize biofortification programs: the case for inclusion of selenium and iodine in the HarvestPlus program. Nutr. Rev. 62, 247–252. doi: 10.1111/j.1753-4887.2004.tb00047.x
Mao, H., Wang, J., Wang, Z., Zan, Y., Lyons, G., and Zou, C. (2014). Using agronomic biofortification to boost zinc, selenium, and iodine concentrations of food crops grown on the loess plateau in China. J. Soil Sci. Plant Nutr. 14, 459–470. doi: 10.4067/s0718-95162014005000036
McNally, S. R. (2011). The Status of Iodine and Selenium in Waikato Soils. Master’s thesis, The University of Waikato, Hamilton.
Nowosielski, O. (1988). The Rules in Development of Fertilizing Strategies in Horticulture. Warsaw: PWRiL Publisher.
Pasławski, P., and Migaszewski, Z. M. (2006). The quality of element determinations in plant materials by instrumental methods. Pol. J. Environ. Stud. 15, 154–164.
Pless, J. D., Chwirka, J. B., and Krumhansl, J. L. (2007). Iodine sequestration using delafossites and layered hydroxides. Environ. Chem. Lett. 5, 85–89. doi: 10.1007/s10311-006-0084-8
PN-EN 15111 (2008). Foodstuffs – Determination of Trace Elements – Determination of Iodine by ICP-MS (Inductively Coupled Plasma Mass Spectrometry). Warsaw: Polish Committee of Standardization.
Ponce de León, C. A., DeNicola, K., Montes Bayón, M., and Caruso, J. A. (2003). Sequential extractions of selenium soils from Stewart Lake: total selenium and speciation measurements with ICP-MS detection. J. Environ. Monit. 25, 435–440. doi: 10.1039/B301108A
Ren, Q., Fan, F., Zhang, Z., Zheng, X., and DeLong, G. R. (2008). An environmental approach to correcting iodine deficiency: supplementing iodine in soil by iodination of irrigation water in remote areas. J. Trace Elem. Med. Biol. 22, 1–8. doi: 10.1016/j.jtemb.2007.09.003
Rhew, R. C., Østergaard, L., Saltzman, E. S., and Yanofsky, M. F. (2003). Genetic control of methyl halide production in Arabidopsis. Curr. Biol. 13, 1809–1813. doi: 10.1016/j.cub.2003.09.055
Ríos, J. J., Blasco, B., Cervilla, L. M., Rubio-Wilhelmi, M. M., Rosales, M. A., Sánchez-Rodríguez, E., et al. (2010). Nitrogen-use efficiency in relation to different forms and application rates of Se in lettuce plants. J. Plant Growth Regul. 29, 164–170. doi: 10.1007/s00344-009-9130-7
Ríos, J. J., Rosales, M. A., Blasco, B., Cervilla, L. M., Romero, L., and Ruiz, J. M. (2008). Biofortification of Se and induction of the antioxidant capacity in lettuce plants. Sci. Hortic. 116, 248–255. doi: 10.1016/j.scienta.2008.01.008
Roberts, S. K. (2006). Plasma membrane anion channels in higher plants and their putative functions in roots. New Phytol. 169, 647–666. doi: 10.1111/j.1469-8137.2006.01639.x
Schlegel, M. L., Reiller, P., Mercier-Bion, F., Barré, N., and Moulin, V. (2006). Molecular environment of iodine in naturally iodinated humic substances: insight from X-ray absorption spectroscopy. Geochim. Cosmochim. Acta 70, 5536–5551. doi: 10.1016/j.gca.2006.08.026
Shetaya, W. H. A. H. (2011). Iodine Dynamics in Soil. Ph.D. thesis, University of Nottingham, Nottingham.
Singh, R., Kumar, R., van Heusden, A. W., Yadav, R. C., and Visser, R. G. F. (2013). Genetic improvement of mungbean (Vigna radiata L.): necessity to increase the levels of the micronutrients iron and zinc: a review. J. Curr. Res. Sci. 1, 428–439.
Smoleń, S., Kowalska, I., and Sady, W. (2014). Assessment of biofortification with iodine and selenium of lettuce cultivated in the NFT hydroponic system. Sci. Hortic. 166, 9–16. doi: 10.1016/j.scienta.2013.11.011
Smoleń, S., Ledwożyw-Smoleń, I., and Sady, W. (2016a). The role of exogenous humic and fulvic acids in iodine biofortification in spinach (Spinacia oleracea L.). Plant Soil 402, 129–143. doi: 10.1007/s11104-015-2785-x
Smoleń, S., Skoczylas, Ł., Rakoczy, R., Ledwożyw-Smoleń, I., Kopeć, A., Piątkowska, E., et al. (2016b). Iodine and selenium biofortification of lettuce (Lactuca sativa L.) by soil fertilization with various compounds of these elements. Acta. Sci. Pol. Hort. Cult. 15, (in press).
Smoleń, S., and Sady, W. (2012). Influence of iodine form and application method on the effectiveness of iodine biofortification, nitrogen metabolism as well as the content of mineral nutrients and heavy metals in spinach plants (Spinacia oleracea L.). Sci. Hortic. 143, 176–183. doi: 10.1016/j.scienta.2012.06.006
Smoleń, S., Wierzbińska, J., Sady, W., Kołton, A., Wiszniewska, A., and Liszka-Skoczylas, M. (2015). Iodine biofortification with additional application of salicylic acid affects yield and selected parameters of chemical composition of tomato fruits (Solanum lycopersicum L.). Sci. Hortic. 188, 89–96. doi: 10.1016/j.scienta.2015.03.023
Tonacchera, M., Dimida, A., De Servi, M., Frigeri, M., Ferrarini, E., De Marco, G., et al. (2013). Iodine fortification of vegetables improves human iodine nutrition: in vivo evidence for a new model of iodine prophylaxis. J. Clin. Endocrinol. Metab. 98, E694–E697. doi: 10.1210/jc.2012-3509
Uchida, S., Tagami, K., and Ishikawa, N. (2009). “Concentration, soil-to-plant transfer factor and soil-soil solution distribution coefficient of selenium in the surface environment – 9106,” in Proceedings of the Symposium on Waste Management (WM ’09), Phoenix, AZ, USA, 1–5 March 2009 (Tucson, AZ: Arizona Board of Regents).
Vtorushina, E. A., Saprykin, A. I., and Knapp, G. (2008). Optimization of the conditions of oxidation vapor generation for determining chlorine, bromine, and iodine in aqueous solutions by inductively coupled plasma atomic-emission spectrometry. J. Anal. Chem. 63, 643–648. doi: 10.1134/S1061934808070071
Vtorushina, E. A., Saprykin, A. I., and Knapp, G. (2009). Use of oxidation and reduction vapor generation for lowering the detection limits of iodine in biological samples by inductively coupled plasma atomic emission spectrometry. J. Anal. Chem. 64, 129–135. doi: 10.1134/S1061934809020063
White, P. J., and Broadley, M. R. (2009). Biofortification of crops with seven mineral elements often lacking in human diets – iron, zinc, copper, calcium, magnesium, selenium and iodine. New Phytol. 182, 49–84. doi: 10.1111/j.1469-8137.2008.02738.x
WHO (2004). Global Strategy on Diet, Physical Activity and Heath. Geneva: World Health Organization.
WHO (2014). Salt Reduction and Iodine Fortification Strategies in Public Health. Report of a Joint Technical Meeting Convened by World Health Organization and The Global Health in Collaboration with the International Council for the Control of Iodine Deficiency Disorders Global Network. Australia, March 2013. Geneva: World Health Organization.
Winkel, L. H., Vriens, B., Jones, G. D., Schneider, L. S., Pilon-Smits, E., and Bañuelos, G. S. (2015). Selenium cycling across soil-plant-atmosphere interfaces: a critical review. Nutrients 7, 4199–4239. doi: 10.3390/nu7064199
Wu, Z., Bañuelos, G. S., Lin, Z. Q., Liu, Y., Yuan, L., Yin, X., et al. (2015). Biofortification and phytoremediation of selenium in China. Front. Plant Sci. 6:136. doi: 10.3389/fpls.2015.00136
Yamada, H., Kiriyama, T., Onagawa, Y., Hisamori, I., Miyazaki, C., and Yonebayashi, K. (1999). Speciation of iodine in soils. Soil Sci. Plant Nutr. 45, 563–568. doi: 10.1080/00380768.1999.10415819
Yamada, H., Kiriyama, T., and Yonebayashi, K. (1996). Determination of total iodine in soils by inductively coupled plasma mass spectrometry. Soil Sci. Plant Nutr. 42, 859–866. doi: 10.1080/00380768.1996.10416633
Yoshida, S., Muramatsu, Y., and Uchida, S. (1992). Studies on the sorption of I- (iodide) and. IO3- (iodate) onto andosols. Water Air Soil Pollut. 63, 321–329. doi: 10.1007/BF00475499
Zhang, K., Halitschke, R., Yin, C., Liu, C. J., and Gan, S. S. (2013). Salicylic acid 3-hydroxylase regulates Arabidopsis leaf longevity by mediating salicylic acid catabolism. Proc. Natl. Acad. Sci. U.S.A. 110, 14807–14812. doi: 10.1073/pnas.1302702110
Zhao, F. J., and McGrath, S. P. (2009). Biofortification and phytoremediation. Curr. Opin. Plant Biol. 12, 373–380. doi: 10.1016/j.pbi.2009.04.005
Zhao, Y. Q., Zheng, J. P., Yang, M. W., Yang, G. D., Wu, Y. N., and Fu, F. F. (2011). Speciation analysis of selenium in rice samples by using capillary electrophoresis-inductively coupled plasma mass spectrometry. Talanta 84, 983–988. doi: 10.1016/j.talanta.2011.03.004
Zhu, Y. G., Huang, Y., Hu, Y., Liu, Y., and Christie, P. (2004). Interactions between selenium and iodine uptake by spinach (Spinacia oleracea L.) in solution culture. Plant Soil 261, 99–105. doi: 10.1023/B:PLSO.0000035539.58054.e1
Keywords: selenomethionine, transfer factor, mineral nutrition, biofortification target, enrichment of plants, beneficial element
Citation: Smoleń S, Skoczylas Ł, Ledwożyw-Smoleń I, Rakoczy R, Kopeć A, Piątkowska E, Bieżanowska-Kopeć R, Koronowicz A and Kapusta-Duch J (2016) Biofortification of Carrot (Daucus carota L.) with Iodine and Selenium in a Field Experiment. Front. Plant Sci. 7:730. doi: 10.3389/fpls.2016.00730
Received: 22 December 2015; Accepted: 12 May 2016;
Published: 27 May 2016.
Edited by:
Fernando Carlos Gómez-Merino, Colegio de Postgraduados, MexicoReviewed by:
Elke Bloem, Julius Kühn-Institut, GermanyCopyright © 2016 Smoleń, Skoczylas, Ledwożyw-Smoleń, Rakoczy, Kopeć, Piątkowska, Bieżanowska-Kopeć, Koronowicz and Kapusta-Duch. This is an open-access article distributed under the terms of the Creative Commons Attribution License (CC BY). The use, distribution or reproduction in other forums is permitted, provided the original author(s) or licensor are credited and that the original publication in this journal is cited, in accordance with accepted academic practice. No use, distribution or reproduction is permitted which does not comply with these terms.
*Correspondence: Sylwester Smoleń, cy5zbW9sZW5Ab2dyLnVyLmtyYWtvdy5wbA==
Disclaimer: All claims expressed in this article are solely those of the authors and do not necessarily represent those of their affiliated organizations, or those of the publisher, the editors and the reviewers. Any product that may be evaluated in this article or claim that may be made by its manufacturer is not guaranteed or endorsed by the publisher.
Research integrity at Frontiers
Learn more about the work of our research integrity team to safeguard the quality of each article we publish.