- 1BioCost Group, Department of Animal Biology, Plant Biology and Ecology, Faculty of Science, University of A Coruña, Spain
- 2Unit of Ecology, Faculty of Biology, University of Santiago de Compostela, Santiago de Compostela, Spain
- 3Centre for Invasion Biology, Department of Botany and Zoology, Stellenbosch University, Matieland, South Africa
- 4Invasive Species Programme, South African National Biodiversity Institute, Kirstenbosch Research Centre, Claremont, South Africa
Why some species become invasive while others do not is a central research request in biological invasions. Clonality has been suggested as an attribute that could contribute to plant invasiveness. Division of labor is an important advantage of clonal growth, and it seems reasonable to anticipate that clonal plants may intensify this clonal attribute in an invaded range because of positive selection on beneficial traits. To test this hypothesis, we collected clones of Carpobrotus edulis from native and invasive populations, grew pairs of connected and severed ramets in a common garden and under negative spatial covariance of nutrients and light to induce division of labor, and measured biomass allocation ratios, final biomass, and photochemical efficiency. Our results showed that both clones from the native and invaded range develop a division of labor at morphological and physiological level. However, the benefit from the division of labor was significantly higher in apical ramets from the invaded range than in ramets from the native area. This is a novel and outstanding result because it provides the first evidence that the benefit of a key clonal trait such as division of labor may have been subjected to evolutionary adaptation in the invaded range. The division of labor can therefore be considered an important trait in the invasiveness of C. edulis. An appropriate assessment of the influence of clonal traits in plant invasions seems key for understanding the underlying mechanisms behind biological invasions of new environments.
Introduction
The establishment of invasive alien species modifies the stability and functioning of populations, communities, and ecosystems, displaces native species and, as consequence, promotes a loss of biodiversity (Vitousek et al., 1996; Mack et al., 2000; Strayer, 2012). In a globalized world, biological invasions and their negative impacts increased dramatically during the last decades and represent one of the most important threats to the conservation of biodiversity worldwide (Vitousek et al., 1996; Mack et al., 2000). The study of biological invasions is a rapidly developing field in modern ecology, and a crucial request in this research area is to determine the traits underlying the invasion process. However, this issue remains unsolved (Roy, 1990; Groves and di Castri, 1991; Lodge, 1993; Rejmánek and Richardson, 1996; Alpert et al., 2000; Levine et al., 2003) and more effort needs to be devoted to identify the mechanisms that explain the success of invasive species (Alpert et al., 2000; Levine et al., 2003; Blackburn et al., 2011).
It seems reasonable to assume that some plant characteristics might better explain the success of invasive species than others. In particular, clonal propagation has been suggested as an attribute that could contribute to plant invasiveness (Pyšek, 1997; Liu et al., 2006; Wang et al., 2008; Song et al., 2013). In fact, many of the most aggressive invasive plant species show clonal growth, and a recent study has highlighted the importance of traits related to clonal propagation in successful invaders (Song et al., 2013). Key aspects such as physiological integration or the capacity for a division of labor make a significant contribution to the success of clonal plants in a wide range of habitats (Hartnett and Bazzaz, 1983; Slade and Hutchings, 1987; Alpert and Stuefer, 1997; Kliměs et al., 1997; Saitoh et al., 2002; Roiloa et al., 2007). Here, we aim to add new evidence that may help to elucidate the role of clonal traits in plant invasions.
A pivotal task in plant invasions is to clarify how exotic plants adapt to the new environments that they are invading. Three mechanisms could explain the adaptation to the invaded range: (i) ‘pre-adaptation’, when species show similar trait standards in native and invaded ranges, indicating that the trait is a successful strategy in either area (Fridley and Sax, 2014); (ii) ‘phenotypic plasticity’, when responses allow plants to adjust their morphological and physiological responses very precisely to the challenges presented by particular environmental conditions, promoting resource acquisition and success in a new habitat (Grime and Mackey, 2002; Valladares et al., 2007; Mommer et al., 2011); and (iii) ‘local adaptation’, whenever there is a rapid adaptive evolution due to new selection pressures, resulting in improved fitness in the introduced environment (Maron et al., 2004; Cano et al., 2008; Xu et al., 2010; Buswell et al., 2011). Common garden experiments comparing plants from native and invaded ranges are required to test whether trait shifts are due to phenotypic plasticity or local adaptation (Wolfe et al., 2004; Erfmeier and Bruelheide, 2005; Güsewell et al., 2006; Zou et al., 2007). Trait differences between populations from native and invaded ranges grown alongside in a common garden would be indicative of an episode of local adaptation to the introduced range. This result would support the evolution of invasiveness hypothesis where rapid genetic changes are driven by natural selection pressures in the invaded environment (Lee, 2002; Stockwell et al., 2003).
One of the most striking attributes of clonal growth is the capacity for a ‘division of labor’ (i.e., specialization to acquire locally abundant resources, thereby increasing clone’s overall performance). Stolon and rhizome internodes allow resource sharing between the connected ramets of a clonal system, which therefore are physiologically integrated. Resources are generally transferred from ramets growing under conditions of high resource supply to ramets located in areas where resource supply is low, following a source-sink system (Hartnett and Bazzaz, 1983; Roiloa and Retuerto, 2005). As result of this integration, supported ramets benefit in terms of growth and survival (e.g., Hartnett and Bazzaz, 1983; Slade and Hutchings, 1987; Saitoh et al., 2002; Roiloa and Retuerto, 2006). Heterogeneous distribution of essential resources, at spatial and temporal scale, is a characteristic of many natural environments (Chapin et al., 1987; Lechowicz and Bell, 1991; Caldwell and Pearcy, 1994). In this sense, natural habitats posses both favorable and unfavorable patches which are often negatively correlated in the space (Stuefer and Hutchings, 1994). This patchy distribution of resources commonly conducts to situations in which the resource-acquiring structures of clonal plant occupy sites that differ in quality (Hutchings and Wijesinghe, 1997). When the availabilities of two essential resources are negatively correlated in space, physiological integration can induce a plastic response in which one ramet specializes to acquire the resource that is locally abundant to it but scarce to other ramets (Friedman and Alpert, 1991; Birch and Hutchings, 1994; Stuefer et al., 1996; Alpert and Stuefer, 1997). Because the resource acquisition is expected to be more economical at high concentrations, the subsequent reciprocal transfer of resources between ramets should increase the overall performance of the clone (Stuefer et al., 1996; Alpert and Stuefer, 1997; Hutchings and Wijesinghe, 1997; Stuefer, 1998). This specialization to acquire locally abundant resources is termed ‘division of labor’ (Alpert and Stuefer, 1997; Hutchings and Wijesinghe, 1997; Stuefer, 1998), and appears to be a singular trait of clonal species. In comparison, non-clonal plants or disconnected ramets in a clonal system, typically respond to resources availability by following the optimal partitioning theory that predicts an increase in the relative allocation of biomass to structures specialized in acquiring the most limiting resource (Thornley, 1972; Bloom et al., 1985).
Plant invaders usually show faster growth rates in the introduced range than in the native area (Elton, 1958; Leger and Rice, 2003; Jakobs et al., 2004; Bossdorf et al., 2005). Division of labor could be accepted as an important advantage of clonal propagation, both at the native and invaded range. However, as the invasion process can be considered as a continuum with a number of filters that the successful invader must overcome (Richardson et al., 2000), it seems reasonable to anticipate that clonal plants may intensify this clonal attribute in the invaded range given a positive selection on beneficial traits, resulting in a rapid adaptive evolution for this trait (Roiloa et al., 2015). To test this hypothesis, we (i) collected clones of Carpobrotus edulis from native and invasive populations, (ii) grew pairs of connected and severed ramets in a common garden experiment, under negative spatial covariance of nutrients and light, in order to induce division of labor, and (iii) measured biomass allocation ratios, final biomass, and photochemical efficiency to quantify ramet specialization and performance at morphological and physiological level. Our specific hypothesis is that the capacity for division of labor, and consequent benefit, would be greater in populations from the invaded range (i.e., Portugal and Spain) than in those from the native range (i.e., South Africa). Thus, we predict that (i) connection would induce an increase in proportional root mass and a decrease in photochemical efficiency in ramets under low light and high nutrients, and that this specialization to acquire below-ground resources will be greater in ramets from the invaded range than in those from the native range; (ii) connection would induce a decrease in proportional root mass and an increase in photochemical efficiency in ramet under high light and low nutrients, and that this specialization to acquire above-ground resources will be greater in ramets from the invaded range than in ramets from the native range; and (iii) the subsequent reciprocal transfer of resources between connected ramets should increase overall performance of the clone, and this benefit would be greater in clones from the invaded range than in clones from the native range.
Many experiments have compared relative performance in invasive and native or exotic non-invasive species (Pyšek and Richardson, 2007; Garcia-Serrano et al., 2009). Nonetheless, relatively few studies have determined whether invaders change their functional strategies from the native to the introduced range (Zou et al., 2007; Leishman et al., 2014; Heberling et al., 2015). Our study appears to be the first in testing these differences for a key clonal trait such as division of labor in an aggressive invader. Assessing the influence of clonal traits in plant invasions is key for understanding the underlying mechanisms behind biological invasions and, therefore, for predicting future invasion scenarios as well as for designing efficient control strategies in invaded areas. Additionally, this information is likewise important for a better understanding of how plants respond and evolve in new environments.
Materials and Methods
Study Species
Carpobrotus edulis (L.) N.E. Br., is a mat-forming succulent clonal plant, native to the Cape Region (South Africa), and an aggressive invader in coastal ecosystems of all the Mediterranean climate regions around the world, including Australia, Europe and America (D’Antonio and Mahall, 1991; Traveset et al., 2008; Vilà et al., 2008). C. edulis forms dense mats and spreads horizontally by the production of numerous apical ramets that remain physiologically integrated by stolon connections (Wisura and Glen, 1993). Ramets produce roots after direct contact with the substrate and can survive if disconnection from the parent ramet occurs. This type of vegetative growth allows C. edulis a very effective colonization of the surrounding area, competing aggressively with local species and affecting negatively the diversity of the native flora (D’Antonio and Mahall, 1991; Traveset et al., 2008). Previous experiments have studied several aspects of the ecology of C. edulis as plant–pollinator networks, plant–soil feedbacks, or hybridization studies (Vilà and D’Antonio, 1998; Bartomeus et al., 2008; de la Peña et al., 2010). However, the importance of clonal traits in the expansion of this aggressive invader has been generally overlooked (but see Roiloa et al., 2010, 2013, 2014a,b).
Sampling Protocol
Four spatially separated populations of C. edulis were sampled in the native (Cape Region, South Africa) and four in the invaded range (Iberian Peninsula, South Europe) (see Figure 1). Plant material was collected in the native and in the invaded range from coastal sand dune systems where C. edulis typically inhabits. To have a more comprehensive illustration of the genetic variability, we selected 36 separated clumps in each of the eight populations sampled. Within a population, selected clumps were separated at least 25 m from the others. C. edulis forms compact clumps (Wisura and Glen, 1993) and it is reasonable to assume that each separated clump represents a different genotype. However, no genetic analyses were performed and the clumps might or might not differ in genotype. Four-member un-rooted clonal fragments were excised at the edge of each clump. Clonal fragments contained the first four units or modules (ramets sensu Harper, 1977) from the apices, and thus we ensured that all the experimental plant material had the same developmental stage. Plant material from the native range was collected at mid-January 2015 and maintained in the greenhouse during 3 months before the experiment began to minimize maternal environmental effects.
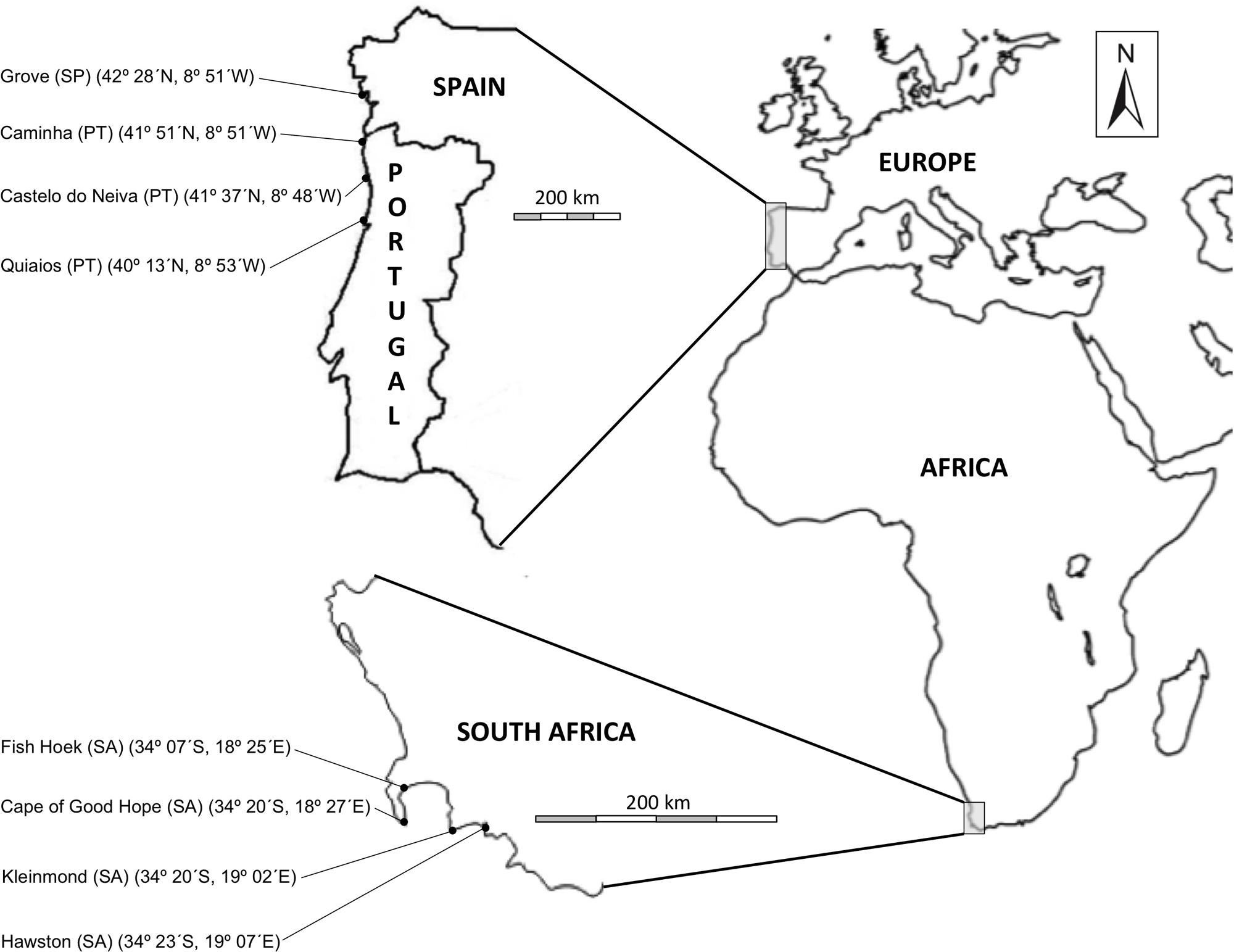
FIGURE 1. Site location (latitude and longitude) of Carpobrotus edulis populations sampled from the native (Cape Region in South Africa) and the invaded (Portugal and Spain in Europe) regions. (SA, South Africa; PT, Portugal; SP, Spain).
Experimental Design
In April 2015, 48 ramet pairs comprised by the third and fourth ramets from the apices were selected for size uniformity from the plant stock. Initial size of ramet pairs was estimated by fresh mass. Preliminary analysis showed that the initial sizes of ramet pairs from the native and the invaded range did not differ significantly (ANOVA F1,46 = 1.787, P = 0.188). The experimental design consisted of two crossed factors with region (native, invaded) and connection (connected, severed) as main factors. The region factor included ramet pairs from coastal sand dunes of the native (South Africa) and invaded range (Europe), as explained above. In the connection factor, ramets within each pair were either left connected (division of labor allowed) or severed (division of labor prevented). Ramets were severed by cutting the connecting stolon halfway between them. We did not observe any immediate negative side effect of cutting the stolon (e.g., sudden death or disease). From the 36 clumps sampled in each population, we randomly selected 6 clumps to obtain the 48 ramet pairs used in the experiment (8 populations × 6 clumps). Ramet pairs from each of the eight populations sampled in the field were equally represented and randomly assigned to each combination of region by connection treatments.
Each pair was subjected to a regime of resource availability known to induce division of labor via changes in allocation of mass between roots and shoots (Roiloa et al., 2014b). Older ramets (the “fourth” modules) were subjected to low light and high nutrient conditions, and younger ramets (the “third” modules) were exposed to high light and low nutrients conditions (see Figure 2). This regime of resource availability mimics the natural conditions of C. edulis growing at coastal sand dune habitats, where older ramets usually grow shaded by shrubs, which enrich the nutrient content of the soil, whereas developing younger ramets spread into the non-shaded sand with low nutrient content (S. R. Roiloa, personal observation). High nutrient conditions consisted of a 3:1 mixture of potting compost and sand. In the low nutrient conditions, ramets grew in sand. Low light conditions were created using a polypropylene shade cloth that reduces ambient light to 10%. Ramets in the high light treatment were left unshaded. Each pair of ramet was planted in a single 5L plastic pot hermetically divided into two equal compartments by plastic barriers to avoid root interactions (see Figure 2). None of the ramets had roots at the start of the experiment. Each treatment was replicated 12 times (n = 12). The common garden experiment was carried out in a open-end greenhouse at the University of Santiago de Compostela (Spain) (42° 52′ 26.65″ N, 8° 33′ 31.64″ W). In order to avoid confounding effect of position within the greenhouse, the four types of treatments were interspersed randomly. Plants were watered regularly with as much water as necessary to maintain soil moisture. Treatments began on 10 April 2015 and continued for 90 days.
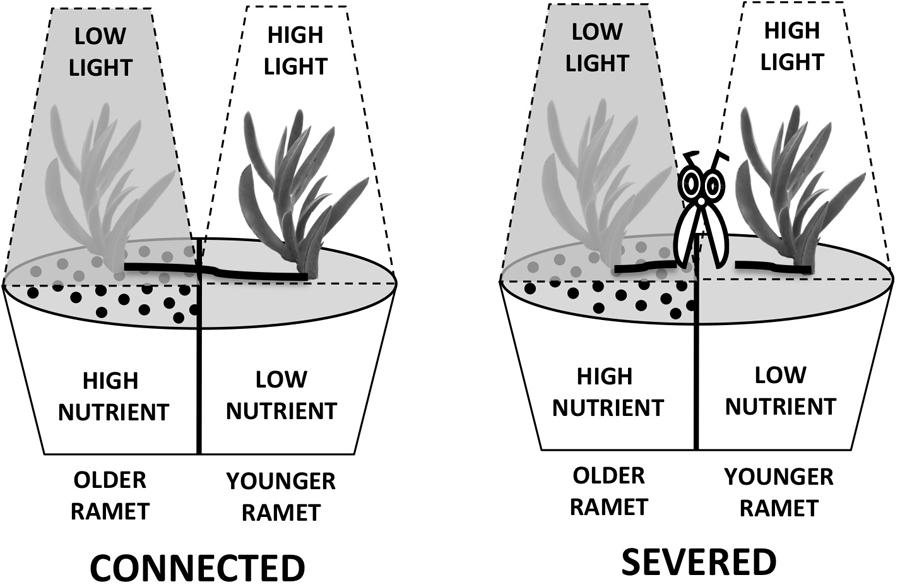
FIGURE 2. Scheme of the experiment, showing levels of light and nutrients given to pairs of connected or severed ramets. Older ramets were exposed to low light and high nutrient conditions. Younger ramets were exposed to high light and low nutrient conditions. See text for experimental design details.
Measurements
Spectral Reflectance
Leaf spectral reflectance parameters were measured 30, 60, and 90 days after treatment application using a portable spectrometer (UniSpec Spectral Analysis System, PP Systems, Haverhill, MA, USA). Specifically, we determined the photochemical reflectance index (PRI), that was calculated as (R539-R570)/(R539 + R570), where R539 and R570 are reflectances at 539 and 570 nm, respectively (Filella et al., 1996). This index correlates with both net CO2 uptake and photosynthetic radiation-use efficiency (mol CO2/mol photons) (Peñuelas et al., 1995; Filella et al., 1996; Gamon et al., 1997).
Chlorophyll Fluorescence
Immediately after reflectance measurements, chlorophyll fluorescence parameters were determined by the saturation pulse method (Schreiber et al., 1998) using a portable pulse-amplitude-modulate fluorometer (MINI-PAM photosynthesis yield analyser; Walz, Effeltrich, Germany). In particular, we measured the maximum quantum yield of photosystem II (PSII), Fv/Fm = (Fm – F0)/Fm, where Fm and F0 are the maximum and minimum fluorescence yield, respectively, of dark-adapted samples, after a saturation pulse (>5000 μmol photons m-2 s-1 of actinic white light) (see Bolhàr-Nordenkampf et al., 1989). The maximum PSII quantum yield (Fv/Fm) characterizes the photosynthetic process associated with electron transport (light reactions), and provides information on the efficiency of excitation energy capture by open PSII reaction centres (Butler and Kitajima, 1975). Fv/Fm correlates with the amount of carbon gained per unit of light absorbed (Bolhàr-Nordenkampf and Öquist, 1993). This variable was measured after a 30-min dark adaptation period, which allowed the PSII reaction centers of the leaf to be fully open.
Growth
At the end of the experiment, each ramet was separated into shoots (including leaves and stolons) and roots, dried at 80°C for 72 h, and weighed. The total dry mass (shoot dry mass + root dry mass) and the proportional biomass allocated to roots (root-shoot ratio, RSR = root dry mass/shoot dry mass) were calculated for older and younger ramets separately. Total dry mass at whole clone level (older + younger ramets) was also calculated.
Statistical Analysis
Prior to analyses, variables were transformed as necessary to meet the assumptions of parametric tests. Thus, the root/shoot (RSR) of older ramets were square root transformed. We analyzed differences in the total dry mass and the proportional biomass allocated to roots (RSR) by two-way analysis of variance (ANOVA) with region (native, invaded) and connection (connected or severed) as fixed effects. Separates analyses were conducted for older (with low light and high nutrients) and younger (with high light and low nutrients) ramets. Similarly, total dry mass at whole clone level (older + younger ramets) was compared by two-way ANOVA with region and connection as main factors. Changes in leaf spectral reflectance (PRI) and chlorophyll fluorescence (Fv/Fm) over time were analyzed with two-way analyses of variance with repeated measures (ANOVAR), using region and connection as between-subject effects. For these variables, we conduced separates analyses for older and younger ramets. Three ramets (one older and two younger) died during the experiment, and the corresponding pairs (older + younger) were excluded from the analyses. This reduced the number of replicates used in the different analyses, as indicated by the error degree of freedom. Significance level was set at P < 0.05. Statistical tests were performed with SPSS Statistics 19.0 (IBM, Armonk, New York, USA).
Results
Growth
The proportion of biomass allocated to roots by older and younger ramets, as determined by the root to shoot ratio (RSR), was significantly affected by the connection treatment (Table 1). Connection significantly increased the proportion of dry mass allocated to roots (RSR) in older ramets but it was decreased in younger ramets (Figure 3). The effect of region and the interaction between region and connection on root to shoot (RSR) was not significant neither in older nor in younger ramets (Table 1).
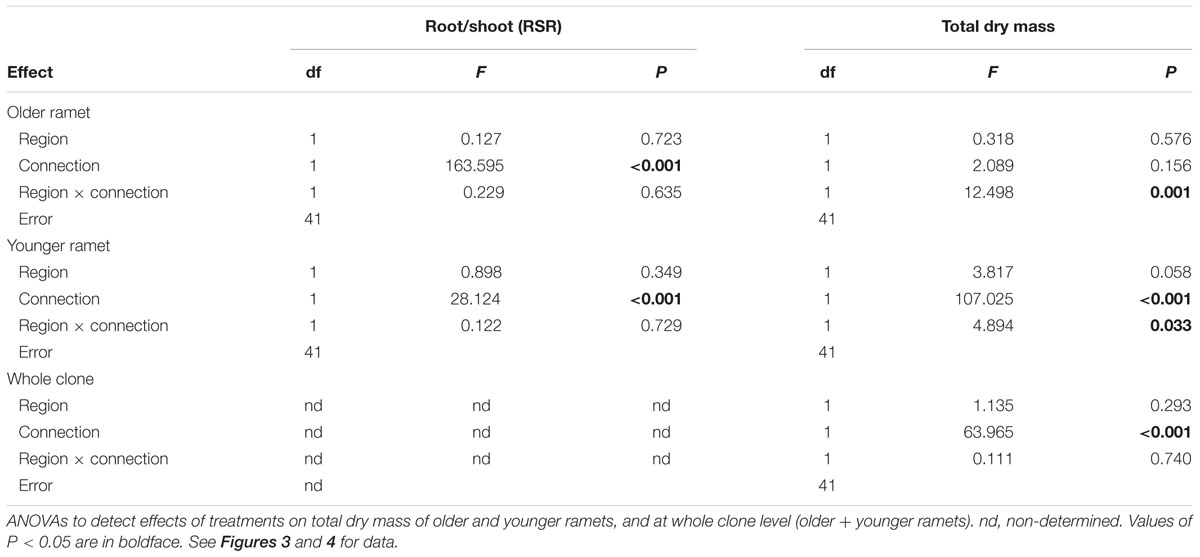
TABLE 1. Results of two-way analyses of variance (ANOVA) to examine the effects of region and connection on root to shoot ratio (RSR) of the older and younger ramets.
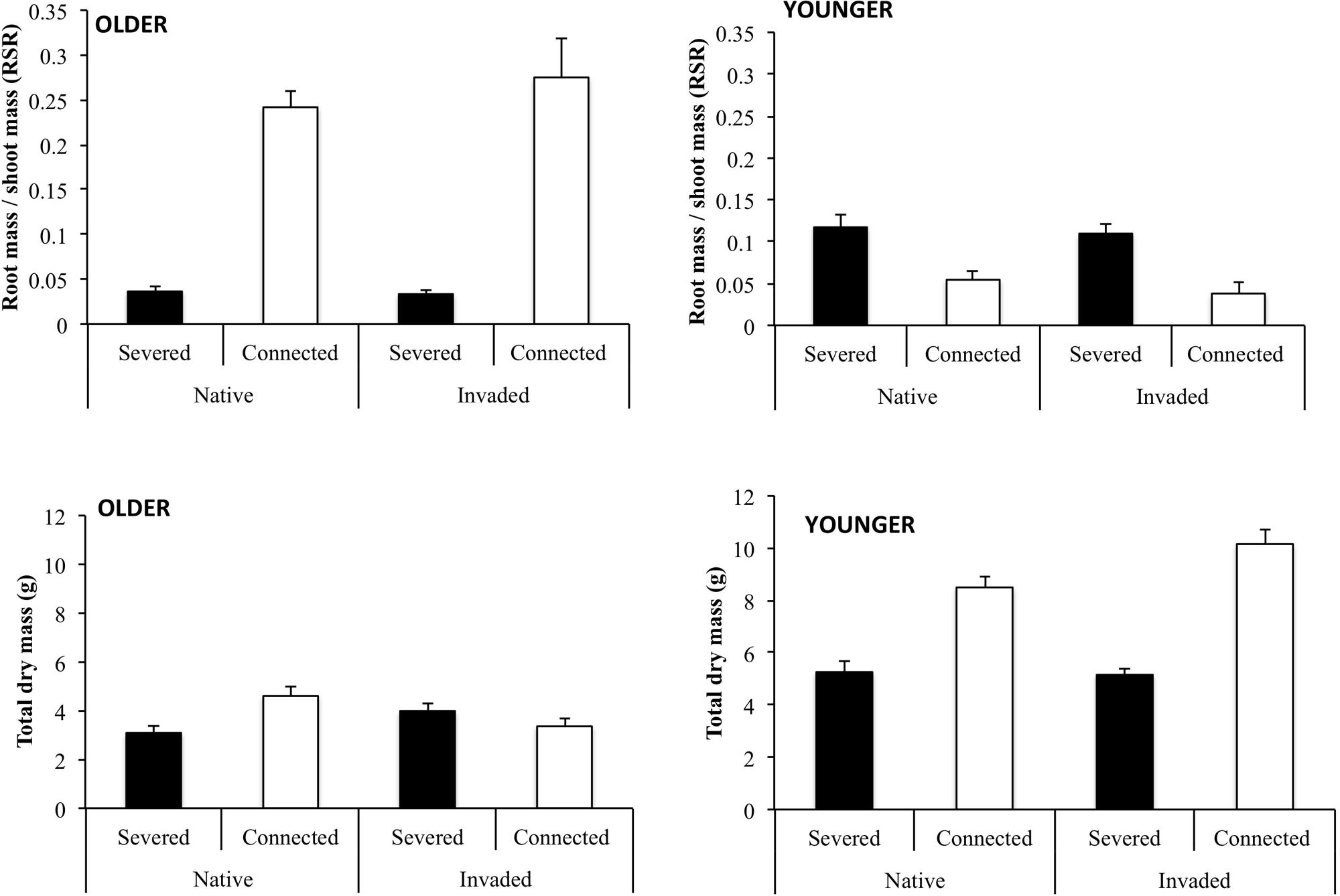
FIGURE 3. Final measurements (mean +SE) of the proportional dry mass allocated to roots (determined as the root to shoot ratio, RSR) and the total dry mass (g) of connected and severed older (left) and younger (right) ramets from native and invaded regions. See Table 1 for ANOVA results.
The final total dry mass of older ramets was significantly affected by the interaction between region and habitat (Table 1). Connection significantly increased the total dry mass in the genotypes from the native region. However, this effect was not maintained in the genotypes from the invaded region, where we detected a decrease of the total dry mass in the connected older ramets (Figure 3). On the other hand, connection significantly increased the final dry mass of younger ramets (Table 1; Figure 3). This positive effect of connection on the final dry mass of younger ramets was stronger in genotypes from the invaded region, as denoted by the significant effect of the interaction between factors region and connection (Table 1; Figure 3). This result indicates that the benefit of physiological integration was significantly higher in invasive populations than in native populations. At the whole clone level (older + younger ramets), our results showed a significant effect of connection, with an increase of the final total dry mass due to connection both in the genotypes from the native and the invaded region (Table 1; Figure 4). Neither the region nor the interaction between region and connection had a significant effect on total dry mass at the whole clone level (Table 1).
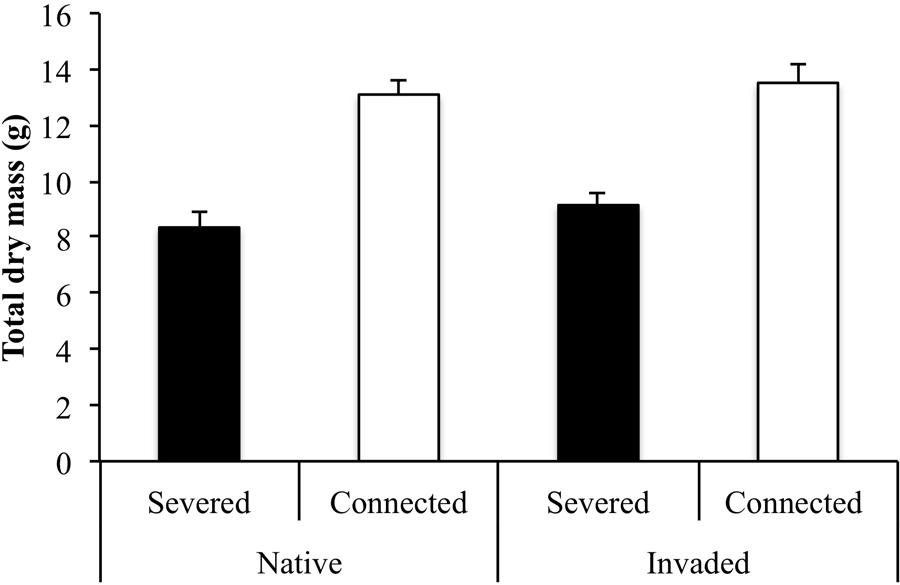
FIGURE 4. Final measurements (mean +SE) of the total dry mass (g) of the whole clones (older + younger ramets) from the native and invaded regions in the connected and severed treatments. See Table 1 for ANOVA results.
Leaf Spectral Reflectance and Chlorophyll Fluorescence
Older ramets from the invaded region showed a significantly higher PRI than older ramets from the native region (Table 2; Figure 5). Our results also showed a significant effect of the connection treatment on the PRI of older ramets (Table 2). Thus, there was a significant decrease in PRI of connected older ramets, regardless of whether they came from the native or the invaded region (Figure 5). We detected a significant effect of the interaction between region and connection in the maximum quantum yield of photosystem II (Fv/Fm) of older ramets (Table 2). Connection significantly increased Fv/Fm in older ramets of the genotypes from the native region, but decreased it in the older ramets of genotypes from the invaded region (Figure 5).
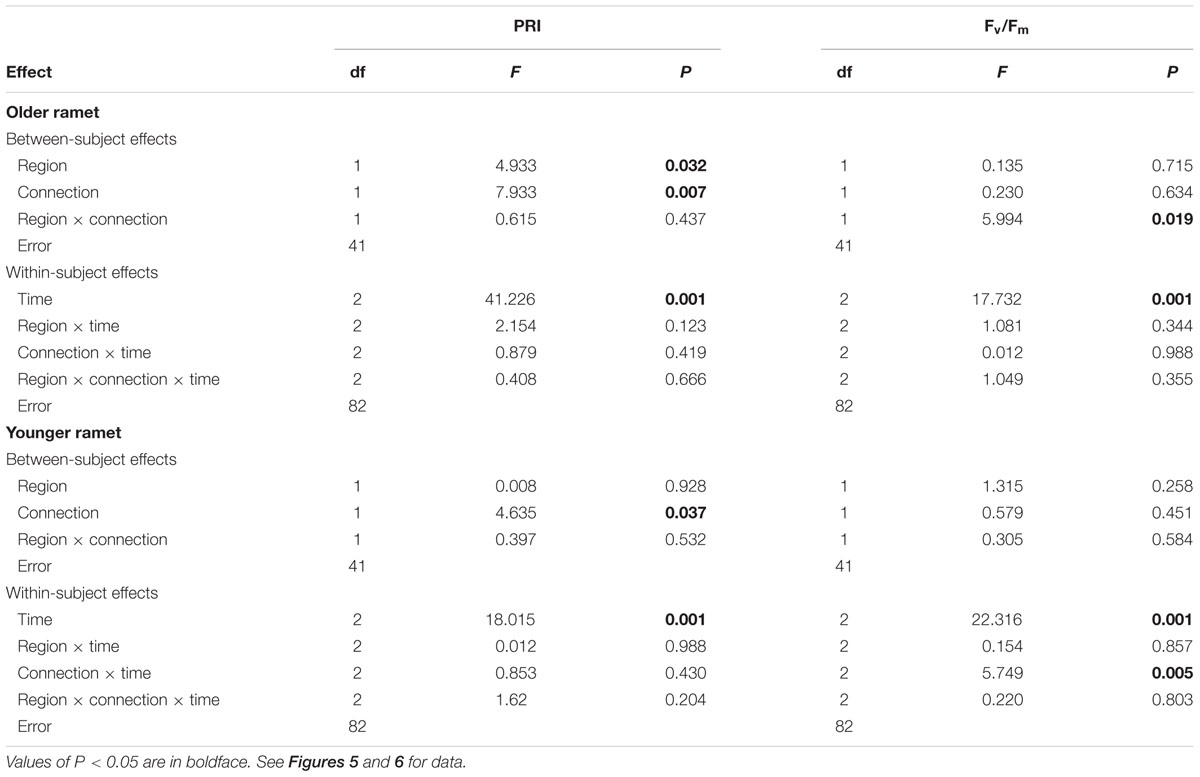
TABLE 2. Results of two-way repeated-measure analysis of variance (ANOVAR) with region and connection as between-subject effects, for differences in the photochemical reflectance index (PRI) and the maximum quantum yield of photosystem PSII (Fv/Fm) of older and younger ramets.
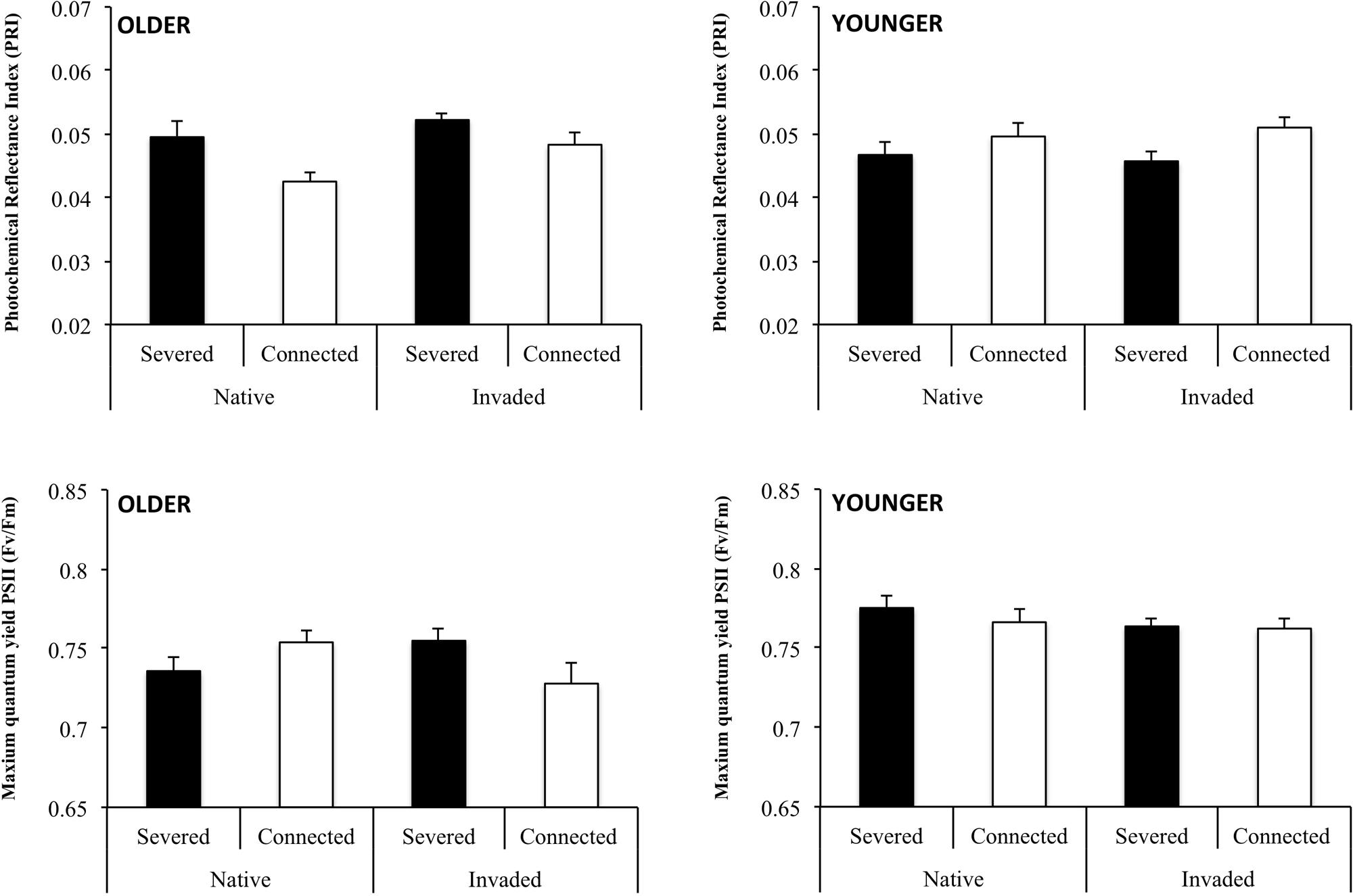
FIGURE 5. Mean values (+SE) of photochemical reflectance index (PRI) and the maximum quantum yield of photosystem II (Fv/Fm) for connected and severed older (left) and younger (right) ramets from the native and invaded regions. See Table 2 for ANOVA results.
Connection significantly affected the PRI of younger ramets (Table 2). PRI values were significantly higher in connected than in severed younger ramets both in genotypes from the native and the invaded region (Figure 5). Although we did not detect a significant between-subject effect of connection in younger ramets in terms of maximum quantum yield of photosystem II (Fv/Fm) (Table 2; Figure 5), this variable was significantly affected by the interaction of connection by time (within-subject effect) (Table 2). Thus, the Fv/Fm values of younger ramets changed with time, and this change was dependent on the connection treatment. Connected and severed younger ramets significantly inverted their Fv/Fm values during the experiment, regardless of their region of origin. At day 30 Fv/Fm values were higher in connected than in severed younger ramets, whereas severed younger ramets showed greater Fv/Fm values than connected ramets at days 60 and 90 (Figure 6).
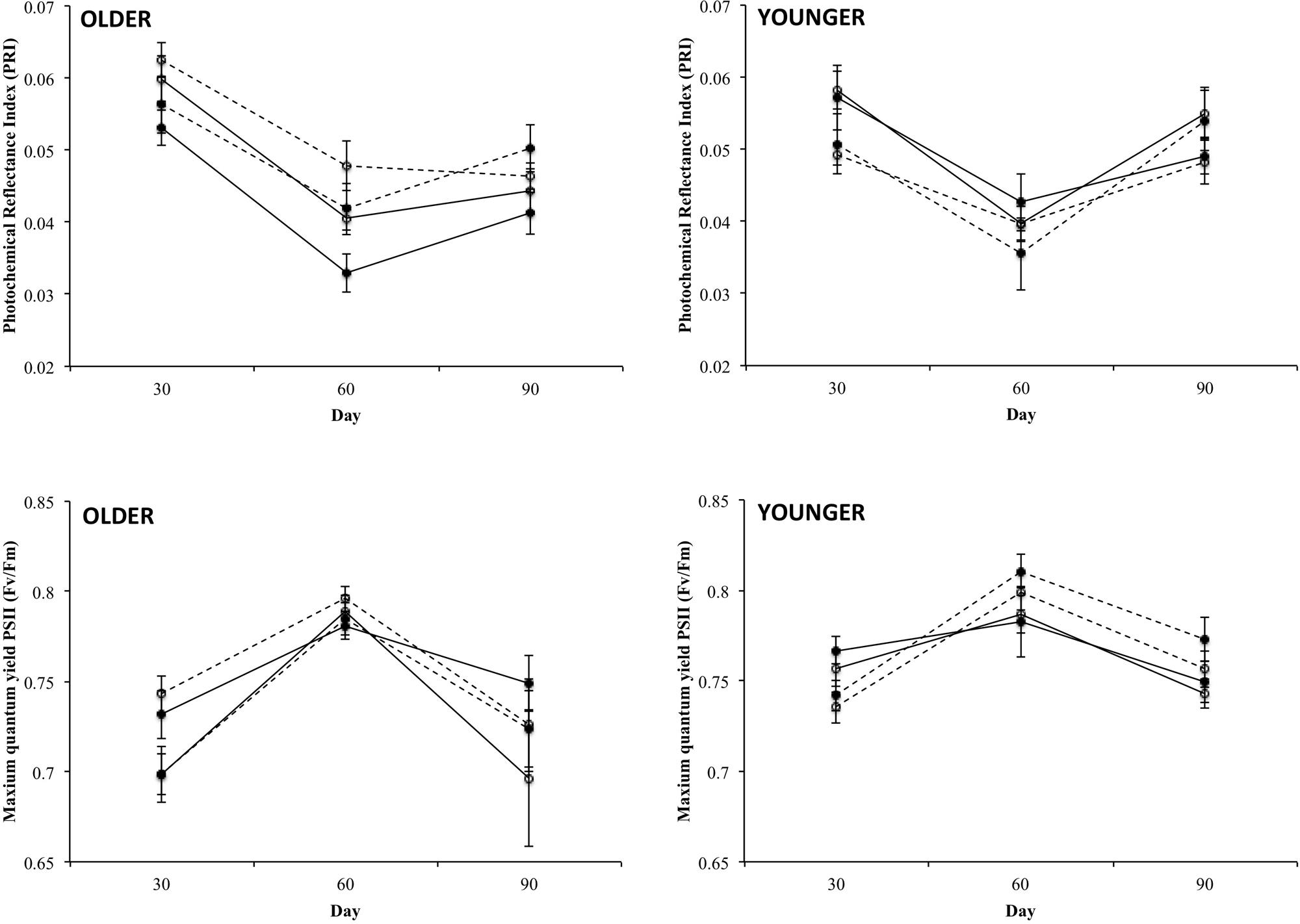
FIGURE 6. Time course of mean values (±SE) of photochemical reflectance index (PRI) and the maximum quantum yield of photosystem II (Fv/Fm) for connected (solid lines) and severed (dashed lines) treatments of older (left) and younger (right) ramets from the native (closed symbols) and invaded (open symbols) regions. See Table 2 for ANOVA results.
Discussion
Our results support the existence of division of labor, both at morphological and at physiological level, in native and invaded populations of C. edulis. As proposed in our hypotheses, we found that connection significantly increase the mass allocated to roots (RSR) in older ramets subjected to high nutrients and low light conditions, denoting a specialization to acquire the relatively abundant below-ground resource. On the other hand, connection significantly increased the photosynthetic radiation-use efficiency (as estimated by the PRI and reduced the proportional root mass (RSR) in younger ramets, which were subjected to high light and low nutrients conditions, indicating a specialization to acquire above-ground resources. In populations from both the native and the invaded range, resource sharing mediated by stolon connection significantly increased the final biomass at the whole clone level. These results provide strong evidence that ramets of clones of C. edulis have a capacity for division of labor, and that this specialization for acquiring the most abundant resource reports a benefit for the whole clone. However, our results do not support the prediction that clones from the invaded range may have a greater capacity for division of labor than those from the native range. Since resource acquisition is expected to be more economical where the resource is more abundant, the specialization to acquire the relatively rich resource and the subsequent reciprocal resource sharing between connected ramets would increase the overall performance of the clone (Stuefer et al., 1996; Alpert and Stuefer, 1997; Hutchings and Wijesinghe, 1997; Roiloa et al., 2014b). Similar results showing a capacity for division of labor at morphological and physiological level were recently reported by Roiloa et al. (2014b) in clones of C. edulis in the invaded range. Likewise, Roiloa et al. (2007) reported environmental-induced division of labor at morphological and physiological levels in clones of the stoloniferous Fragaria chiloensis. Both studies showed greater capacity for division of labor in clones from patchier habitats, where essential resources were negatively correlated. In these conditions, the division of labor between ramets would be specially beneficial, suggesting an adaptive division of labor induced by the environment (Roiloa et al., 2007, 2014b). Previous studies with other clonal plants also reported differences between genotypes for several clonal traits, including division of labor, resource sharing, or sexual/asexual shift, which suggest a potential for local adaptation (Lotscher and Hay, 1997; Alpert, 1999; Prati and Schmid, 2000; Alpert et al., 2003; Roiloa et al., 2007; Nilsson and D’Hertefeldt, 2008; D’Hertefeldt et al., 2014), and are in agreement with the evolutionary theory that predicts that populations evolve to generate traits to gain an advantage under their local conditions (Willians, 1966).
Although our results showed no differences in the capacity for division of labor between invasive and native populations, we found, however, significant differences in the benefits obtained by the division of labor among ramets. As reported, benefits were significantly higher in younger ramets from invasive populations than in those from native populations. This is a novel and outstanding result because it provides the first evidence that the benefit of a key clonal trait, as division of labor, may be subjected to evolutionary adaptation in the invaded range. Therefore, our results indicate that rapid genetic changes linked to selection pressures might contribute to the invasion of C. edulis in the introduced range. Rapid adaptive evolution of introduced populations could explain invasion success in a new environment (Maron et al., 2004; Sax et al., 2007). In this line, it seems reasonable to predict that positive selection of beneficial traits such as division of labor could be favoring the expansion of C. edulis in the introduced range and, therefore, promoting its invasiveness.
Interestingly, the benefit of division of labor detected in younger ramets at the invaded range seems to be obtained at the cost of the older ramets. This is, in native populations the reciprocal transport of resources between the connected older and younger ramets seems to be balanced and, consequently provided mutual benefits to both ramets. However, our results for the invasive populations showed a significant benefit of the connection for younger ramets, but a significant cost for older ramets, indicating an unbalanced share of resources. This result seemingly indicates that there was a unidirectional transport of resources from the older to the younger ramet in the population from the invaded range. As a result, growth increase was more pronounced in invasive younger ramets than in native ones, where resources transport appeared to be bidirectional, as described in previous works (e.g., Stuefer et al., 1996; Alpert and Stuefer, 1997; Hutchings and Wijesinghe, 1997). This trait shift in the introduced range could be promoting the expansion of younger ramets, contributing to the expansion of invasive plants. C. edulis spreads horizontally by the production of abundant younger ramets that remain integrated by stolon connections. Roiloa et al. (2010) found an association between the increase in total biomass and the horizontal expansion of apical ramets of C. edulis when colonizing a natural dune system in the invaded range. Thus, our finding of an intensification of the benefits derived from division of labor for apical ramets in populations from introduced range supports the idea that adaptation after introduction may favor the expansion of this aggressive invader.
Our pioneer results showing an unbalanced benefit for older and younger ramets from division of labor in populations from the invaded range could be explained within the evolution of increased competitive ability (EICA) hypothesis. The latter proposes that exotic plants, once established in the introduced range and liberated from their natural enemies, invest more in fast-growing and less in defence (Blossey and Nötzold, 1995; Mooney and Cleland, 2001; Hierro et al., 2005; Alba et al., 2012). Under this supposition, older ramets in the invasive range can reallocate resources from defensive traits to support connected younger ramets favoring the expansion of this aggressive invader. In any case, we recognize that our results are not robust enough to confirm this statement, and more experiments are necessary to elucidate if the unbalanced transport of resources described in our study could be explained by EICA hypothesis.
In addition, our results showed that photosynthetic efficiency (estimated by the PRI, which is correlated with net CO2 uptake and photosynthetic radiation-use efficiency, Peñuelas et al., 1995; Filella et al., 1996; Gamon et al., 1997) was significantly higher in older ramets from the invaded range than in older ramets from the native area. Previous studies have detected that invasive plants show greater growth rates in their introduced range than in the native range (Elton, 1958; Leger and Rice, 2003; Jakobs et al., 2004; Bossdorf et al., 2005). In another common garden experiment, Zou et al. (2007) also found that invasive populations of Sapium sebiferum showed significantly higher photosynthetic activity, in terms of net CO2 assimilation (determined directly by gas exchange measurements), than populations from their native range. Eco-physiological results also showed a significant increase in the PRI in connected younger ramets, both in the native and invaded range. This increases in photochemical efficiency was translated into a significant increase in the total mass, denoting a positive correlation between PRI and growth. However, and differing to the pattern obtained for total mass, we did not detect a more accentuate increase in photochemical activity in younger ramets from invaded range in comparison with values obtained in native populations. Probably, the significantly higher benefit in younger ramets from invasive populations was due to the support received from their connected older ramets. In this sense, the unidirectional transport of resources from the older to the younger ramet in the population from the invaded range increased more pronounced the growth in invasive younger ramets than in native ones, in spite of not showing differences in photochemical activity.
Conclusion
This is the first study reporting differences between native and invaded populations in the benefits derived from a key clonal trait such as division of labor. The results from this study seem consistent with a rapid adaptive evolution of the clonar invader C. edulis with a positive selection of the benefits from division of labor in the invaded range. The benefits from division of labor could therefore be considered an important trait in the invasiveness of C. edulis. However, whether this is the case for all clonal plant invaders or not remains to be clarified, and future research should include more clonal species. The contribution of clonal traits to the capacity to establish in new environments represents an exciting research field for understanding the mechanisms underlying plant invasions, and for a better knowledge of how plants respond and evolve in new habitat. In addition, experiments testing for differences between invasive and exotic non-invasive species are mandatory to understand the role of clonal traits in plant invasions. Understanding the influence of clonal life-history traits in plant invasions seems key for predicting future invasion scenarios and for devising efficient strategies of control and restoration of invaded areas.
Author Contributions
SR was involved in designing the experiment, field samples collection, set up the experiment, data collection, and analysis, manuscript preparation and submission. RR field samples collection, set up the experiment, data collection, and co-prepared manuscript. JC set up the experiment, data collection and co-prepared manuscript. AN and RB field samples collection and co-prepared manuscript.
Funding
Financial support for this study was provided by the Spanish Ministry of Economy and Competitiveness (projects Ref. CGL2013-44519-R, awarded to SRR and Ref. CGL2013-48885-C2-2-R, awarded to RR). European Regional Development Fund (ERDF) co-financed these projects. This is a contribution from the Alien Species Network (Ref. R2014/036 – Xunta de Galicia, Autonomous Government of Galicia).
Conflict of Interest Statement
The authors declare that the research was conducted in the absence of any commercial or financial relationships that could be construed as a potential conflict of interest.
Acknowledgments
We thank to L. Álvarez from the Spanish Ministry of Agriculture, Food, and the Environment for assistance in authorization procedure for the plant material importation from South Africa.
References
Alba, C., Bowers, M. D., and Hufbauer, R. (2012). Combining optimal defense theory and the evolutionary dilemma model to refine predictions regarding plant invasion. Ecology 93, 1912–1921. doi: 10.1890/11-1946.1
Alpert, P. (1999). Clonal integration in Fragaria chiloensis differs between populations: ramets from grassland are selfish. Oecologia 120, 69–76. doi: 10.1007/s004420050834
Alpert, P., Bone, E., and Holzapfel, C. (2000). Invasiveness, invasibility, and the role of environmental stress in preventing the spread of non-native plants. Perspect. Plant Ecol. Evol. Syst. 3, 52–66. doi: 10.1078/1433-8319-00004
Alpert, P., Holzapfel, C., and Slominski, C. (2003). Differences in performance between genotypes of Fragaria chiloensis with different degrees of resource sharing. J. Ecol. 91, 27–35. doi: 10.1046/j.1365-2745.2003.00737.x
Alpert, P., and Stuefer, J. F. (1997). “Division of labour in clonal plants,” in The Ecology and Evolution of Clonal Plants, eds H. de Kroon and J. van Groenendael (Leiden: Backhuys Publishers), 137–154.
Bartomeus, I., Vilà, M., and Santamaría, L. (2008). Contrasting effects of invasive plants in plant–pollination networks. Oecologia 155, 761–770. doi: 10.1007/s00442-007-0946-1
Birch, C. P. D., and Hutchings, M. J. (1994). Exploitation of patchily distributed soil resources by the clonal herb Glechoma hederacea. J. Ecol. 82, 653–664. doi: 10.2307/2261272
Blackburn, T. M., Pyšek, P., Bacher, S., Carlton, J. T., Duncan, R. P., Jaroší, V., et al. (2011). A proposed unified framework for biological invasions. Trends Ecol. Evol. 26, 333–339. doi: 10.1016/j.tree.2011.03.023
Bloom, A. J., Chapin, F. S. III, and Mooney, H. A. (1985). Resource limitation in plants—an economic analogy. Annu. Rev. Ecol. Syst. 16, 363–392. doi: 10.1146/annurev.es.16.110185.002051
Blossey, B., and Nötzold, R. (1995). Evolution of increased competitive ability in invasive non-indigenous plants: a hypothesis. J. Ecol. 83, 887–889. doi: 10.2307/2261425
Bolhàr-Nordenkampf, H. R., Long, S. P., Baker, N. R., Öquist, G., Scheiber, U., and Lechner, E. G. (1989). Chlorophyll fluorescence as a probe of the photosynthetic competence of leaves in the field: a review of current instrumentation. Funct. Ecol. 3, 497–514. doi: 10.2307/2389624
Bolhàr-Nordenkampf, H. R., and Öquist, G. (1993). “Chlorophyll fluorescence—a tool in photosynthesis research,” in Photosynthesis and Production in a Changing Environment, eds D. O. Hall, J. M. O. Scurlock, H. R. Bolhàr-Nordenkampf, R. C. Leegood, and S. P. Long (London: Chapman and Hall), 193–206.
Bossdorf, O., Auge, H., Lafuma, L., Rogers, W. E., Siemann, E., and Prati, D. (2005). Phenotypic and genetic differentiation in native versus introduced plant populations. Oecologia 144, 1–11. doi: 10.1007/s00442-005-0070-z
Buswell, J. M., Moles, A. T., and Hartley, S. (2011). Is rapid evolution common in introduced plant specie? J. Ecol. 99, 214–224. doi: 10.1111/j.1365-2745.2010.01759.x
Butler, W., and Kitajima, M. (1975). Fluorescence quenching in photosystem II of chloroplasts. Biochim. Biophys. Acta 376, 116–125. doi: 10.1016/0005-2728(75)90210-8
Caldwell, M. M., and Pearcy, R. W. (1994). Exploitation of Environmental Heterogeneity by Plants. Ecophysiological Processes Above and Belowground. San Diego: Academic Press.
Cano, L., Escarre, J., Fleck, I., Blanco-Moreno, J. M., and Sans, F. X. (2008). Increased fitness and plasticity of an invasive species in its introduced range: a study using Senecio pterophorus. J. Ecol. 96, 468–476. doi: 10.1111/j.1365-2745.2008.01363.x
Chapin, F. S. III, Bloom, A. J., Field, C. B., and Waring, R. H. (1987). Plant responses to multiple environmental factors. Bioscience 37, 49–57. doi: 10.2307/1310177
D’Antonio, C. M., and Mahall, B. E. (1991). Root profiles and competition between the invasive, exotic perennial, Carpobrotus edulis, and two native shrub species in California coastal scrub. Am. J. Bot. 78, 885–894. doi: 10.2307/2445167
de la Peña, E., de Clercq, N., Bonte, D., Roiloa, S. R., Rodriguez-Echeverria, S., and Freitas, H. (2010). Plant–soil feedback as a mechanism of invasion by Carpobrotus edulis. Biol. Inv. 12, 3637–3648. doi: 10.1007/s10530-010-9756-1
D’Hertefeldt, T., Eneström, J. M., and Pettersson, L. B. (2014). Geographic and habitat origin influence biomass production and storage translocation in the clonal plant Aegopodium podagraria. PLoS ONE 9:e85407. doi: 10.1371/journal.pone.0085407
Erfmeier, A., and Bruelheide, H. (2005). Invasive and native Rhododendron ponticum populations: is there evidence for genotypic differences in germination and growth? Ecography 28, 417–428. doi: 10.1111/j.0906-7590.2005.03967.x
Filella, I., Amaro, T., Araus, J. L., and Peñuelas, J. (1996). Relationship between photosynthetic radiation-use efficiency of barley canopies and the photochemical reflectance index (PRI). Physiol. Plant. 96, 211–216. doi: 10.1111/j.1399-3054.1996.tb00204.x
Fridley, J. D., and Sax, D. F. (2014). The imbalance of nature: revisiting a Darwinian framework for invasion biology. Global Ecol. Biogeogr. 23, 1157–1166. doi: 10.1111/geb.12221
Friedman, D., and Alpert, P. (1991). Reciprocal transport between ramets increases growth of Fragaria chiloensis when light and nitrogen occur in separate patches but only if patches are rich. Oecologia 86, 76–80. doi: 10.1007/BF00317392
Gamon, J. A., Serrano, I., and Surfus, J. S. (1997). The photochemical reflectance index: an optical indicator of photosynthetic radiation use efficiency across species, functional types, and nutrient levels. Oecologia 112, 492–501. doi: 10.1007/s004420050337
Garcia-Serrano, H., Caño, L., Escarré, J., Fleck, I., and Sans, F. X. (2009). Physiological comparison of alien Senecio inaequidens and S. pterophorus and native S. malacitanus: implications for invasion. Flora 204, 445–455. doi: 10.1007/s00442-008-1182-z
Grime, J. P., and Mackey, J. M. L. (2002). The role of plasticity in resource capture by plants. Evol. Ecol. 16, 299–307. doi: 10.1023/A:1019640813676
Groves, R. H., and di Castri, F. (1991). Biogeography of Mediterranean Invasions. Cambridge: Cambridge University Press.
Güsewell, S., Jakobs, G., and Weber, E. (2006). Native and introduced populations of Solidago gigantea differ in shoot production but not in leaf traits or litter decomposition. Funct. Ecol. 20, 575–584. doi: 10.1111/j.1365-2435.2006.01141.x
Hartnett, D. C., and Bazzaz, F. A. (1983). Physiological integration among intraclonal ramets in Solidago canadensis. Ecology 64, 779–788. doi: 10.2307/1937201
Heberling, J. M., Kichey, T., Decocq, G., and Fridley, J. D. (2015). Plant functional shifts in the invaded range: a test with reciprocal forest invaders of Europe and North America. Funct. Ecol. doi: 10.1111/1365-2435.12590
Hierro, J. L., Maron, J. L., and Callaway, R. M. (2005). A biogeographical approach to plant invasions: the importance of studying exotics in their introduced and native range. J. Ecol. 93, 5–15. doi: 10.1111/j.0022-0477.2004.00953.x
Hutchings, M. J., and Wijesinghe, D. K. (1997). Patchy habitats, division of labour and growth dividends in clonal plants. Trends Ecol. Evol. 12, 390–394. doi: 10.1016/S0169-5347(97)87382-X
Jakobs, G., Weber, E., and Edwards, P. J. (2004). Introduced plants of the invasive Solidago gigantea (Asteraceae) are larger and grow denser than conspecifics in the native range. Divers. Distrib. 10, 11–19. doi: 10.1111/j.1472-4642.2004.00052.x
Kliměs, L., Kliměsova, J., Hendriks, R., and van Groenendael, J. (1997). “Clonal plant architecture: a comparative analysis of form and function,” in The Ecology and Evolution of Clonal Plants, eds H. de Kroon and J. van Groenendael (Leiden: Backhuys Publishers), 1–29.
Lechowicz, M. J., and Bell, G. (1991). The ecology and genetics of fitness in forest plants. II. Microspatial heterogeneity of the edaphic environment. J. Ecol. 79, 687–696. doi: 10.2307/2260661
Lee, C. E. (2002). Evolutionary genetics of invasive species. Trends Ecol. Evol. 17, 386–391. doi: 10.1016/S0169-5347(02)02554-5
Leger, E. A., and Rice, K. J. (2003). Invasive California poppies (Eschscholzia californica Cham.) grow larger than native individuals under reduced competition. Ecol. Lett. 6, 257–264. doi: 10.1046/j.1461-0248.2003.00423.x
Leishman, M. R., Cooke, J., and Richardson, D. M. (2014). Evidence for shifts to faster growth strategies in the new ranges of invasive alien plants. J. Ecol. 102, 1451–1461. doi: 10.1111/1365-2745.12318
Levine, J. M., Vilà, M., D’Antonio, C. M., Dukes, J. S., Grigulis, K., and Lavorel, S. (2003). Mechanisms underlying the impacts of exotic plant invasions. Proc. R. Soc. B. 270, 775–781. doi: 10.1098/rspb.2003.2327
Liu, J., Dong, M., Miao, S., Li, Z., Song, M., and Wang, R. (2006). Invasive alien plants in China: role of clonality and geographical origin. Biol. Inv. 8, 1461–1470. doi: 10.1007/s10530-005-5838-x
Lodge, D. M. (1993). Biological invasions: lessons for ecology. Trends Ecol. Evol. 8, 133–137. doi: 10.1016/0169-5347(93)90025-K
Lotscher, M., and Hay, M. J. M. (1997). Genotypic differences in physiological integration, morphological plasticity and utilization of phosphorus induced by variation in phosphate supply in Trifolium repens. J. Ecol. 85, 341–350. doi: 10.2307/2960506
Mack, R. N., Simberloff, D., Lonsdale, W. M., Evans, H., Clout, M., and Bazzaz, F. A. (2000). Biotic invasions: causes, epidemiology, global consequences, and control. Ecol. Appl. 10, 689–710. doi: 10.1890/1051-0761(2000)010[0689:BICEGC]2.0.CO;2
Maron, J. L., Vila, M., Bommarco, R., Elmendorf, S., and Beardsley, P. (2004). Rapid evolution of an invasive plant. Ecol. Monogr. 74, 261–280. doi: 10.1890/03-4027
Mommer, L., Visser, E. J. W., van Ruijven, J., de Caluwe, H., Pierik, R., and de Kroon, H. (2011). Contrasting root behavior in two grass species: a test of functionality in dynamic heterogeneous conditions. Plant Soil 344, 347–360. doi: 10.1007/s11104-011-0752-8
Mooney, H. A., and Cleland, E. E. (2001). The evolutionary impact of invasive species. Proc. Natl. Acad. Sci. U.S.A. 98, 5446–5451. doi: 10.1073/pnas.091093398
Nilsson, J., and D’Hertefeldt, T. (2008). Origin matters for level of resource sharing in the clonal herb Aegopodium podagraria. Evol. Ecol. 22, 437–448. doi: 10.1007/s10682-007-9199-z
Peñuelas, J., Filella, I., and Gamon, J. A. (1995). Assessment of photosynthetic radiation-use efficiency with spectral reflectance. New Phytol. 131, 291–296. doi: 10.1111/j.1469-8137.1995.tb03064.x
Prati, D., and Schmid, B. (2000). Genetic differentiation of life-history traits within populations of the clonal plant Ranunculus reptans. Oikos 90, 442–456. doi: 10.1034/j.1600-0706.2000.900303.x
Pyšek, P. (1997). “Clonality and plant invasion: can a trait make a difference?,” in The Ecology and Evolution of Clonal Plants, eds H. de Kroon and J. van Groenendal (Leiden: Backhuys Publishers), 405–427.
Pyšek, P., and Richardson, D. M. (2007). “Traits associated with invasiveness in alien plants: where do we stand?,” in Biological Invasions, ed. W. Nentwig (Berlin: Springer), 97–126.
Rejmánek, M., and Richardson, D. M. (1996). What attributes make some plant species more invasive? Ecology 77, 1655–1661. doi: 10.2307/2265768
Richardson, D. M., Pyšek, P., Rejmánek, M., Barbour, M. G., Panetta, F. D., and West, C. J. (2000). Naturalization and invasion of alien plants: concepts and definitions. Divers. Distrib. 6, 93–107. doi: 10.1046/j.1472-4642.2000.00083.x
Roiloa, S. R., Alpert, P., Nishanth, T., Hancock, G., and Bhowmik, P. (2007). Greater capacity for division of labour in clones of Fragaria chiloensis from patchier habitats. J. Ecol. 95, 397–405. doi: 10.1111/j.1365-2745.2007.01216.x
Roiloa, S. R., Campoy, J. G., and Retuerto, R. (2015). Understanding the role of clonal integration in biological invasions. Ecosistemas 24, 76–83. doi: 10.7818/ECOS.2015.24-1.12
Roiloa, S. R., and Retuerto, R. (2005). Presence of developing ramets of Fragaria vesca increase photochemical efficiency in parent ramets. Int. J. Plant Sci. 166, 795–803. doi: 10.1086/431804
Roiloa, S. R., and Retuerto, R. (2006). Physiological integration ameliorates effects of serpentine soils in the clonal herb Fragaria vesca. Physiol. Plant. 128, 662–676. doi: 10.1111/j.1399-3054.2006.00790.x
Roiloa, S. R., Rodríguez-Echeverría, S., de la Peña, E., and Freitas, H. (2010). Physiological integration increases survival and growth of the clonal invader Capobrotus edulis. Biol. Inv. 12, 1815–1823. doi: 10.1007/s10530-009-9592-3
Roiloa, S. R., Rodríguez-Echeverría, S., and Freitas, H. (2014a). Effect of physiological integration in self/non-self genotype recognition on the clonal invader Carpobrotus edulis. J. Plant Ecol. 7, 413–418. doi: 10.1093/jpe/rtt045
Roiloa, S. R., Rodríguez-Echeverría, S., Freitas, H., and Retuerto, R. (2013). Developmentally-programmed division of labour in the clonal invader Carpobrotus edulis. Biol. Inv. 15, 1895–1905. doi: 10.3732/ajb.1400173
Roiloa, S. R., Rodríguez-Echeverría, S., López-Otero, A., Retuerto, R., and Freitas, H. (2014b). Adaptive plasticity to heterogeneous environments increases capacity for division of labor in the clonal invader Carpobrotus edulis (Aizoaceae). Am. J. Bot. 101, 1301–1308. doi: 10.3732/ajb.1400173
Roy, J. (1990). “In search of characteristics of plant invaders,” in Biological Invasions in Europe and the Mediterranean Basin, eds A. J. di Castri, A. J. Hansen, and M. Debushe (Dordrecht: Kluwer), 335–352.
Saitoh, T., Seiwa, K., and Nishiwaki, A. (2002). Importance of physiological integration of dwarf bamboo to persistence in forest understorey: a field experiment. J. Ecol. 90, 78–85. doi: 10.1046/j.0022-0477.2001.00631.x
Sax, D. F., Stachowicz, J. J., Brown, J. H., Bruno, J. F., Dawson, M. N., Gaines, S. D., et al. (2007). Ecological and evolutionary insights from species invasions. Trends Ecol. Evol. 22, 465–471. doi: 10.1016/j.tree.2007.06.009
Schreiber, U., Bilger, W., Hormann, H., and Neubauer, C. (1998). “Chlorophyll fluorescence as a diagnostic tool: basics and some aspects of practical relevance,” in Photosynthesis. A Comprehensive Treatise, ed. A. S. Raghavendra (Cambridge: Cambridge University Press), 320–336.
Slade, A. J., and Hutchings, M. J. (1987). An analysis of the costs and benefits of physiological integration between ramets in the clonal perennial herb Glechoma hederacea. Oecologia 73, 425–431. doi: 10.1007/BF00385260
Song, Y. B., Yu, F. H., Keser, L. H., Dawson, W., Fischer, M., Dong, M., et al. (2013). United we stand, divided we fall: a meta analysis of experiments on clonal integration and its relationship to invasiveness. Oecologia 171, 317–327. doi: 10.1007/s00442-012-2430-9
Stockwell, C. A., Hendry, A. P., and Kinnison, M. T. (2003). Contemporary evolution meets conservation biology. Trends Ecol. Evol. 18, 94–101. doi: 10.1016/S0169-5347(02)00044-7
Strayer, D. L. (2012). Eight questions about invasions and ecosystem functioning. Ecol. Lett. 15, 1199–1210. doi: 10.1111/j.1461-0248.2012.01817.x
Stuefer, J. (1998). Two types of division of labour in clonal plants: benefits, costs and constraints. Perspect. Plant Ecol. Evol. Syst. 1, 47–60. doi: 10.1078/1433-8319-00051
Stuefer, J. F., de Kroon, H., and During, H. J. (1996). Exploitation of environmental heterogeneity by spatial division of labour in a clonal plant. Funct. Ecol. 10, 328–334. doi: 10.2307/2390280
Stuefer, J. F., and Hutchings, M. J. (1994). Environmental heterogeneity and clonal growth: a study of the capacity for reciprocal translocation in Glechoma hederacea L. Oecologia 100, 302–308. doi: 10.1007/BF00316958
Thornley, J. M. (1972). A balanced quantitative model for root: shoot ratios in vegetative plants. Ann. Bot. 36, 431–441.
Traveset, A., Moragues, E., and Valladares, F. (2008). Spreading of the invasive Carpobrotus aff. acinaciformis in Mediterranean ecosystems: the advantage of performing in different light environments. Appl. Veg. Sci. 11, 45–54. doi: 10.1111/j.1654-109X.2008.tb00203.x
Valladares, F., Gianoli, E., and Gomez, J. M. (2007). Ecological limits to plant phenotypic plasticity. New Phytol. 176, 749–763. doi: 10.1111/j.1469-8137.2007.02275.x
Vilà, M., and D’Antonio, C. M. (1998). Hybrid vigor for clonal growth in Carpobrotus (Aizoaceae) in coastal California. Ecol. Appl. 8, 1196–1205. doi: 10.2307/2640972
Vilà, M., Siamantziouras, A. K. D., Brundu, G., Camarda, I., Lambdon, P., Médail, F., et al. (2008). Widespread resistance of Mediterranean island ecosystems to the establishment of three alien species. Divers. Distrib. 14, 839–851. doi: 10.1111/j.1472-4642.2008.00503.x
Vitousek, P. M., D’Antonio, C. M., Loope, L. L., and Westbrooks, R. (1996). Biological invasions as global environmental change. Am. Sci. 84, 468–478.
Wang, N., Yu, F. H., Li, P. X., He, W. M., Liu, F. H., Liu, J. M., et al. (2008). Clonal integration affects growth, photosynthetic efficiency and biomass allocation, but not the competitive ability, of the alien invasive Alternanthera philoxeroides under severe stress. Ann. Bot. 101, 671–678. doi: 10.1093/aob/mcn005
Willians, G. C. (1966). Adaptation and Natural Selection. Princeton, NJ: Princeton University Press.
Wisura, W., and Glen, H. F. (1993). The South African species of Carpobrotus (Mesembryanthema–Aizoaceae). Contrib. Bolus. Herb. 15, 76–107.
Wolfe, L. M., Elzinga, J. A., and Biere, A. (2004). Increased susceptibility to enemies following introduction in the invasive plant Silene latifolia. Ecol. Lett. 7, 813–820. doi: 10.1111/j.1461-0248.2004.00649.x
Xu, C. Y., Julien, M. H., Fatemi, M., Girod, C., van Klinken, R. D., Gross, C. L., et al. (2010). Phenotypic divergence during the invasion of Phyla canescens in Australia and France: evidence for selection-driven evolution. Ecol. Lett. 13, 32–44. doi: 10.1111/j.1461-0248.2009.01395.x
Keywords: biological invasions, biomass allocation, Carpobrotus edulis, chlorophyll fluorescence, clonal integration, division of labor, local adaptation, spectral reflectance
Citation: Roiloa SR, Retuerto R, Campoy JG, Novoa A and Barreiro R (2016) Division of Labor Brings Greater Benefits to Clones of Carpobrotus edulis in the Non-native Range: Evidence for Rapid Adaptive Evolution. Front. Plant Sci. 7:349. doi: 10.3389/fpls.2016.00349
Received: 08 January 2016; Accepted: 07 March 2016;
Published: 30 March 2016.
Edited by:
Boris Rewald, University of Natural Resources and Life Sciences, Vienna, AustriaReviewed by:
Yao-Bin Song, Hangzhou Normal University, ChinaBi-Cheng Dong, Beijing Forestry University, China
Copyright © 2016 Roiloa, Retuerto, Campoy, Novoa and Barreiro. This is an open-access article distributed under the terms of the Creative Commons Attribution License (CC BY). The use, distribution or reproduction in other forums is permitted, provided the original author(s) or licensor are credited and that the original publication in this journal is cited, in accordance with accepted academic practice. No use, distribution or reproduction is permitted which does not comply with these terms.
*Correspondence: Sergio R. Roiloa, sergio.roiloa@udc.es