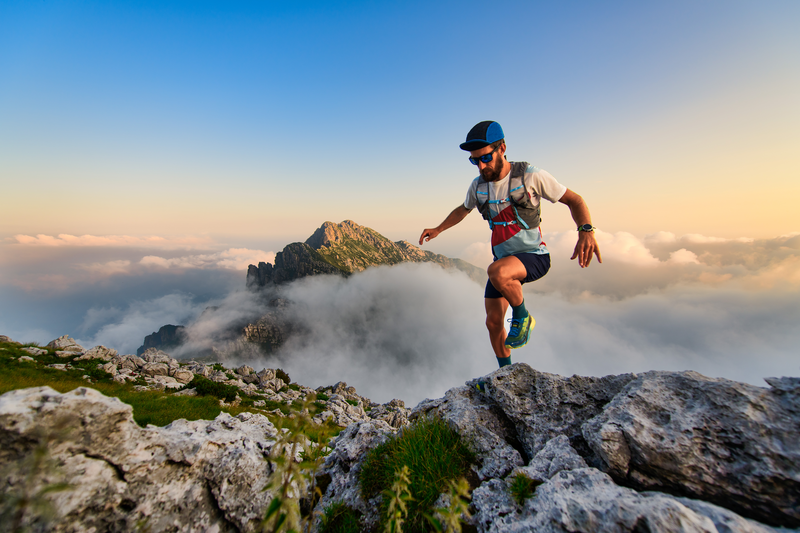
95% of researchers rate our articles as excellent or good
Learn more about the work of our research integrity team to safeguard the quality of each article we publish.
Find out more
ORIGINAL RESEARCH article
Front. Plant Sci. , 16 March 2016
Sec. Plant Genetics and Genomics
Volume 7 - 2016 | https://doi.org/10.3389/fpls.2016.00283
The American pokeweed plant, Phytolacca americana, is recognized for synthesizing pokeweed antiviral protein (PAP), a ribosome inactivating protein (RIP) that inhibits the replication of several plant and animal viruses. The plant is also a heavy metal accumulator with applications in soil remediation. However, little is known about pokeweed stress responses, as large-scale sequencing projects have not been performed for this species. Here, we sequenced the mRNA transcriptome of pokeweed in the presence and absence of jasmonic acid (JA), a hormone mediating plant defense. Trinity-based de novo assembly of mRNA from leaf tissue and BLASTx homology searches against public sequence databases resulted in the annotation of 59 096 transcripts. Differential expression analysis identified JA-responsive genes that may be involved in defense against pathogen infection and herbivory. We confirmed the existence of several PAP isoforms and cloned a potentially novel isoform of PAP. Expression analysis indicated that PAP isoforms are differentially responsive to JA, perhaps indicating specialized roles within the plant. Finally, we identified 52 305 natural antisense transcript pairs, four of which comprised PAP isoforms, suggesting a novel form of RIP gene regulation. This transcriptome-wide study of a Phytolaccaceae family member provides a source of new genes that may be involved in stress tolerance in this plant. The sequences generated in our study have been deposited in the SRA database under project # SRP069141.
The pokeweed plant, Phytolacca americana, is a member of the Phytolaccaceae family of flowering plants that includes 65 species of herbs, shrubs, and trees. Pokeweed is native to eastern North America and has become naturalized in Europe, the West Indies and Asia. This species is of interest because it synthesizes PAP, a RIP with RNA N-glycosidase activity. Several isoforms of PAP are reported to exist in pokeweed, exhibiting different temporal (PAP-I, PAP-II, PAP-III) and spatial (PAP-S, PAP-R, PAP-alpha) expression patterns (Irvin, 1975; Irvin et al., 1980; Barbieri et al., 1982; Bolognesi et al., 1990; Kataoka et al., 1992; Rajamohan et al., 1999). RIPs are present in less than 20% of angiosperm taxonomic orders and phylogenetic analysis indicates a complex evolutionary history (Di Maro et al., 2014). They are potent defense proteins effective against a range of viruses, fungi, and less commonly, insects (Stirpe, 2013).
Pokeweed has broad applications in agriculture and medicine. PAP inhibits the replication of several plant and animal viruses, either through ribosome inactivation which limits viral proliferation or by direct depurination of the viral genome (Lodge et al., 1993; Rajamohan et al., 1999; He et al., 2008; Karran and Hudak, 2008; Mansouri et al., 2009). Interestingly, recent work demonstrates that pokeweed accumulates high levels of heavy metals, especially cadmium and manganese, with promising applications in soil detoxification (Dou et al., 2009; Zhao et al., 2011, 2012). Nevertheless, little is known about pokeweed, as the genes involved in stress response have not been identified.
Here, we have sequenced the pokeweed mRNA transcriptome in the presence and absence of JA treatment. JA is a plant hormone that mediates defense against herbivores and necrotrophic pathogens. As herbivores are often viral vectors, the JA pathway also has important implications for virus resistance. We showed recently that PAP mRNA and protein levels increase in the presence of JA (Klenov et al., 2015). A link between jasmonate and other plant RIPs has previously been established. For example, PIP2 from Phytolacca insularis, ME1 from Mirabilis expansa and JIP60 from barley are induced by JA or its methyl jasmonate derivative (Dunaeva et al., 1999; Song et al., 2000; Vepachedu et al., 2003). Furthermore, expression of the insecticidal maize RIP2 is increased 100-fold at the RNA level upon caterpillar feeding, demonstrating the relevance of RIPs in anti-herbivory (Chuang et al., 2014). By sequencing the transcriptome of pokeweed treated with JA, we will gain novel information about the regulation of specific PAP isoforms and how these proteins are integrated within the larger network of pokeweed pathogen response. This work lays the important foundation to understand the resiliency of pokeweed to biotic and abiotic factors.
We report the de novo assembly and annotation of the pokeweed mRNA transcriptome from leaf tissue. Through a combination of differential expression and GO analysis, we identified JA-responsive genes and enriched GO terms involved in stress and defense. We confirmed the existence of several published PAP isoforms, reported their distinct responses to JA and cloned a potentially novel PAP isoform. Finally, we report the discovery of PAP NATs that are also JA-responsive, which may represent a novel form of RIP gene regulation.
Pokeweed seeds were treated with 37% sulfuric acid for 5 min and submerged in water for 4 days at room temperature. Seeds were germinated in soil (Promix BX) and maintained in a growth chamber (AC60, Biochambers, Winnipeg, MB, Canada) under fluorescent and incandescent lights at 180 μE m-2 s-1 with periods of 16 h day and 8 h night. Fertilizer was provided once every 2 weeks with N:P:K 20:20:20. For experimental treatment, plants were sprayed with 5 mL of 5 mM JA dissolved in 0.5% ethanol (to improve the solubility of JA). Negative control plants were sprayed with 0.5% ethanol. Following treatment, plants were returned to the chamber and leaf tissue was harvested 24 h later. All plants used in this study were at the 4-leaf stage of growth.
Total RNA was extracted from leaf tissue of pokeweed plants treated with 5 mM JA in 0.5% ethanol or 0.5% ethanol alone using the RNeasy Plant Mini Kit (Qiagen, Valencia, CA, USA). An equal amount of total RNA from three independent plants was pooled for each biological replicate. In total, six mRNA libraries were generated (n = 3 per treatment, from 18 total plants). Libraries were constructed with the TruSeq Stranded mRNA Library Preparation Kit (RS-122-2101, Illumina). Sequencing was performed on a single lane of an Illumina HiSeq 2500 machine by The Centre for Applied Genomics (The Hospital for Sick Children, Toronto, ON, Canada) to generate paired-end reads of 150 bases. Raw sequences are available at the SRA database under project # SRP069141.
Prior to assembly, adapters were clipped, low-quality bases were trimmed (Q < 30, averaged over four bases) and synchronicity of paired-end files was maintained using Trimmomatic v. 0.32.1 (Bolger et al., 2014) as follows: PE -phred33 ILLUMINACLIP:TruSeq3-PE.fa:2:30:10 SLIDINGWINDOW:4:30. The pokeweed transcriptome was assembled with Trinity v. r2014-04-13p1 (Grabherr et al., 2011) using the following command-line, which invoked paired-end, stranded information: –seqType fq –JM 48G –left reads-1.fq –right reads-2.fq –SS_lib_type RF –CPU 24.
Trinotate v. 2.0.1 (Haas et al., 2013) was used for transcriptome annotation. BLAST (Altschul et al., 1997) searches were conducted against both the SwissProt (Bairoch and Boeckmann, 1991) and UniRef90 (Suzek et al., 2007) databases (current as of January, 2015). Owing to strand-specific sequencing, only the plus strand of the transcriptome was queried with BLASTx. Transdecoder-predicted and translated ORFs were queried with BLASTp. The E-value threshold was set to 0.001 and only the top-scoring hit was retained. HMMER v. 3.1b2 (Finn et al., 2011) was used to search for conserved protein domains in predicted ORFs against the pfam-A (Finn et al., 2014) database (current as of January, 2015). BLAST homologies and Pfam domain entries were loaded into the pre-formatted Trinotate SQLite database which contained UniProt-associated annotation information.
The complete, Trinity-assembled transcriptome (Raw assembly) was filtered to retain only transcripts expressed at an abundance of 1 FPKM (Filtered assembly) or only BLASTx-annotated transcripts (BLASTx assembly). Assembly statistics were calculated and transcript coverage of each top-scoring unique hit was determined with custom scripts that came bundled with Trinity software. A local installation of the Galaxy platform (Giardine et al., 2005; Blankenberg et al., 2010; Goecks et al., 2010) was used for manipulation of large datasets.
Transcript-level rather than unigene-level expression was investigated in order to retain isoform-specific information. Reads from individual libraries were aligned to the reference transcriptome with bowtie v. 1.1.1. (Langmead et al., 2009) and quantified by RSEM v. 1.2.18 (Li and Dewey, 2011). A table of TMM-normalized FPKM expression values and a separate table of raw fragment counts were generated with custom scripts.
Differentially expressed transcripts were identified from raw counts with the Bioconductor package EdgeR v. 3.1 (Robinson et al., 2010) in the statistical program R (R Development Core Team, 2011). Three biological replicates for each condition were provided. A subset of differentially expressed transcripts (FDR < 0.001 and FC ≥ 4) was extracted and used to generate a heatmap of hierarchically clustered, log2-transformed and median-centered FPKM values. All scripts came bundled with Trinity software and default parameters were used, supplemented with the strand-specific parameter -SS_lib_type RF when applicable.
Blast2GO v. 3.0 (Conesa et al., 2005) was used to map GO terms to parent plant GOSlim terms in order to obtain a broad overview of the transcriptome. To identify enriched terms, a Fisher’s test was conducted in Blast2GO with FDR < 0.001. For NAT GO term enrichment, any NAT pair with at least one protein-coding transcript, as annotated by BLASTx, was included in the test set. For enrichment of JA-responsive NAT pairs, in addition to the above protein-coding requirement, only pairs with differentially expressed sense and antisense transcripts were included in the test set. The raw, Trinity-assembled pokeweed transcriptome served as the reference set for all Fisher’s tests conducted in this study.
Following transcriptome annotation, any transcript that matched a published PAP sequence as its top BLASTx hit and contained a predicted RIP protein domain was considered to be a PAP isoform. To identify NATs, a BLASTn search was conducted whereby the plus strand of the complete transcriptome was aligned to a local database containing the reverse complement of all transcripts. The BLASTn E-value threshold was set to 0.001.
All primer sequences used in this study are available in Supplementary Data Sheet 1. Reverse transcription was performed on 500 ng of total pokeweed RNA in a 20 μL reaction volume containing 5 mM DTT, 1 μM reverse primer, 1X First Strand Buffer (50 mM Tris-HCl pH 8.3, 75 mM KCl, 3 mM MgCl2), 0.5 mM dNTPs, 20 units Murine RNase Inhibitor (NEB) and 25 units Superscript III reverse transcriptase (Thermo Fisher). The reaction was incubated at 42°C for 1 h and heat inactivated at 70°C for 20 min.
Following cDNA synthesis, a PCR reaction was conducted, containing 1X Q5 buffer (NEB), 0.5 μM forward primer, 0.5 μM reverse primer, 200 mM dNTPs, 2 μL cDNA and 1 unit Q5 DNA polymerase (NEB) in a total volume of 50 μL. The PCR program included an initial denaturation of 95°C for 30 s, 30 cycles of 95°C for 30 s, 58°C for 30 s, 72°C for 120 s and finished with an extension at 72°C for 180 s. PCR products were separated on low-melt agarose and the correct size band excised and purified with EZ-10 Spin columns (Biobasic). The purified product was digested with BamHI and SalI, ligated into pBS-KSII and sequenced.
For qRT-PCR, the reverse transcription step was performed in the same manner as cloning except that 2 μg of total pokeweed RNA from either control or JA treated plants was used with reverse primers corresponding to a specific transcript or 28S rRNA as the internal control. The qPCR reaction contained 5 μL of cDNA, 0.7 μM forward primer, 0.7 μM reverse primer and 1X SYBR Green Mastermix (Clontech). Each reaction was split into three technical replicates and analyzed in a Qiagen Rotor-gene-Q real time PCR cycler. Ct-values were calculated with the ΔΔCt relative quantification method. Three biological replicates were conducted for each transcript. For statistical analysis, a one-tailed, unpaired Student’s t-test was conducted using GraphPad Prism v.5.01.
An overview of the entire study is provided in Supplementary Figure S1. A total of 406,995,054 high-quality reads from control and JA-treated pokeweed plants were combined and the mRNA transcriptome was assembled with Trinity software. Assembly statistics are provided in Table 1. The complete pokeweed transcriptome (Raw) contained 216,891 transcripts belonging to 177,709 unigenes. To identify contigs expressed at a reasonable threshold, the Raw assembly was filtered to retain only those having a minimum abundance of 1 FPKM; this reduced the number of transcripts and unigenes to 89,682 and 77,731, respectively (Filtered). The Raw assembly was also filtered on the basis of BLASTx annotation, which resulted in 59,096 and 38,291 annotated transcripts and unigenes, respectively (BLASTx). Furthermore, 16,245 unique proteins were represented by transcripts with at least 70% BLASTx alignment coverage, indicating a high-quality transcriptome assembly (Supplementary Data Sheet 2). The Trinotate report for BLASTx-annotated transcripts is available in Supplementary Data Sheet 3. Interestingly, only 38% of transcripts in the Filtered assembly were also BLASTx annotated, suggesting that the majority of pokeweed-expressed transcripts are not shared with those from available plants.
To assess the contiguity of our different assemblies, we also determined their N50 values (Table 1). The N50 statistic is a weighted median such that 50% of the assembly is contained in contigs equal to or larger than this value. N50 values for the Raw, Filtered, and BLASTx assemblies were 1,168, 1,617, and 2,102 bp, respectively. These values indicated that expressed and annotated transcripts tended to be longer in length, which was confirmed from the assembly length distributions (Figure 1). Notably, the Raw assembly had a majority of transcripts between 200 and 600 bp in length, while this size class accounted for a smaller proportion of the Filtered and BLASTx assemblies. These short transcripts could represent partial transcripts, microRNA precursors and/or assembly artifacts.
Functional annotation of the complete, assembled pokeweed transcriptome was carried out with the Trinotate pipeline and GO analysis was conducted using Blast2GO software. GO terms were mapped to corresponding plant GOSlim terms in order to obtain a summary of the transcriptome (Supplementary Figure S2). GOSlims are cut-down versions of the ontology with reduced detail of lower-level terms; they are useful for providing a general overview of the transcriptome. In total, 36,423 transcripts were annotated with 238,251 GO terms, distributed amongst the categories of Biological Process, Molecular Function, and Cellular Component. Within the Biological Process category, the most abundant terms included response to stress (5,471 transcripts; 15.0% of all transcripts), transport (5,365; 14.7%), and cellular protein modification process (5,200; 14.3%). Nucleotide binding (8,403; 23.1%), DNA binding (4,415; 12.1%), and kinase activity (3,219; 8.8%) comprised the majority of terms in the Molecular Function category. Finally, the Cellular Component distribution indicated that most annotated proteins localized to the plasma membrane (4,974; 13.7%), plastid (3,559; 9.8%), or cytosol (2,887; 7.9%). Taken together, GO analysis indicates that the majority of pokeweed transcripts can be grouped into a small number of broad yet distinct functional categories.
Following transcriptome annotation, our next goal was to identify JA-responsive genes through differential expression analysis. Briefly, reads from each library were individually aligned back to the complete reference transcriptome and the abundance of each transcript was determined; differential expression analysis was then conducted based on normalized read counts. The most abundant, BLASTx-annotated transcripts from control and JA-treated plants are summarized in Table 2 and abundances of all transcripts are available in Supplementary Data Sheet 4. Control plants had high expression of several genes encoding photosynthetic proteins, including RuBisCO and photosystem-associated components. Conversely, JA-treated plants had high abundance of transcripts encoding defense proteins, including two defensin-like proteins, two isoforms of PAP and a proteinase, in addition to constitutive plant metabolic proteins.
TABLE 2. Most abundant, BLASTx-annotated transcripts expressed in pokeweed under control (E) and JA treatments.
A total of 8,264 transcripts were differentially expressed between control and JA-treated plants (FDR < 0.05; Supplementary Figure S3). A subset of 2,770 (FDR < 0.001, FC ≥ 4) was defined for downstream analysis (Figure 2A). Of these, the majority of transcripts increased with JA treatment (2067; 75%). Furthermore, as shown in Figure 2B, most of the transcripts were expressed in both control and JA-treated plants (2192; 79%); interestingly, a considerable number was detected only in JA-treated plants (434; 16%). Of these JA treatment-specific transcripts, 165 encoded known proteins as annotated by BLASTx (Supplementary Data sheet 5). Many have well-established roles in defense, including a pathogenesis-related protein, and several chitinases, proteinases, peroxidases, and terpenoid biosynthesis enzymes. JA-induced transcription factor families such as ERF, MYB, and TIFY were identified, as well as two enzymes involved in JA biosynthesis, jasmonate O-methyltransferase and 4-coumarate-CoA ligase-like 5. Interestingly, among the most abundant, JA-specific transcripts was a putatively novel isoform of PAP, c115037_g1_i1.
FIGURE 2. Identification of JA-responsive genes in pokeweed. (A) Heat map of expression values (log2FPKM, median-centered) of the top differentially expressed transcripts (FDR < 0.001, FC ≥ 4). (B) Venn diagram depicting treatment-specific expression patterns of transcripts in (A).
The top JA-responsive, BLASTx-annotated transcripts are summarized in Table 3 and the complete list of differential expression results is provided in Supplementary Data Sheet 6. As expected, many of these transcripts encoded proteins involved in JA metabolism (Supplementary Figure S4). Additionally, several defense genes were among those most differentially expressed; these included intracellular ribonuclease LX, nerolidol synthases, antiviral protein alpha, and defensin-like protein. To obtain insight into the functional roles of JA-responsive transcripts in pokeweed, we conducted GO term enrichment analysis (Table 4). Up- and down-regulated transcripts were investigated separately in order to determine their independent contributions within the plant. Up-regulated transcripts were highly enriched in terms related to stress and defense responses, indicating marked transcriptional reprogramming in JA-treated plants. Down-regulated transcripts were not enriched in any well-defined stress responses and the enriched terms did not appear to represent any common themes.
To validate RNA-seq results, the expression of eight randomly selected transcripts and two selected transcripts (discussed below) from our defined subset was assessed by qRT-PCR from control and JA-treated plants (Figure 3). An R2 correlation value of 0.917 was obtained, indicating high correspondence between the two methods of transcript quantitation.
FIGURE 3. Validation of RNA-seq differential expression results. The correlation of JA-induced expression changes obtained from RNA-seq and qRT-PCR is shown for 10 transcripts, eight of which were randomly selected. Results for qRT-PCR are from three independent biological replicates for each transcript.
Following transcriptome annotation, we were able to identify assembled PAP isoforms on the basis of homology with published sequences. Six transcripts were annotated as RIPs through BLASTx and contained predicted RIP domains within their translated ORFs; their annotation information is summarized in Table 5. Two isoforms, PAP-I and PAP-II, had perfectly assembled ORFs. Partial transcripts of PAP-alpha and PAP-S were also identified, with 73 and 39% coverage and nearly 100% sequence identity with their respective hits. Interestingly, transcript c18776_g1_i1 had only 40% identity with PAP-alpha, its top BLASTx hit, and 86% coverage. Furthermore, the E-value of its identified RIP domain was more significant than that of PAP-II, which was correctly assembled. We cloned and sequenced the predicted ORF of c18776_g1_i1 from pokeweed total RNA, confirming expression of the transcript in the plant. Taken together, we hypothesize that transcript c18776_g1_i1 represents a novel PAP isoform. Transcript c115037_g1_i1, with 81% identity to PAP-I, may be another novel isoform; however, with only 38% coverage, we could not rule out the possibility that this transcript was an assembly artifact.
TABLE 5. Identification of PAP isoforms in the pokeweed mRNA transcriptome from BLASTx and Pfam annotations.
Differential expression results of the six identified PAP isoforms are provided in Table 6. With the exception of c18776_g1_i1, the hypothesized novel isoform, all other transcripts showed a significant increase in abundance upon JA treatment (FDR < 0.05). PAP-S had the most significant result, with a log2 FC of 12.07, a remarkable 4,300-fold increase. Transcript c115037_g1_i1, the other putative novel isoform, showed the highest log2 FC of 13.17. The expression patterns of c18776_g1_i1 and c115037_g1_i1 are distinct from PAP-alpha and PAP-I, their respective top hits, further supporting the hypothesis that they are novel isoforms. We validated the expression of c18776_g1_i1 by qRT-PCR. Although its reduction with JA treatment was not significant by RNA-seq (FDR < 0.05), qRT-PCR indicated a log2 FC of –1.04 (0.49 fold), which was significant (Student’s t-test, p < 0.05; Figure 3). The fully assembled PAP-I and PAP-II isoforms showed the lowest log2 FC of 3.83 and 3.11, respectively; interestingly, they were also the most abundant. Taken together, these results indicate that PAP isoforms respond differently to JA treatment, in terms of FC and abundance.
Owing to strand-specific sequencing of the pokeweed mRNA transcriptome, we were able to identify putative NATs. This involved performing a BLASTn search of the assembled transcriptome against its reverse complement to find transcript pairs having significant sequence complementarity (E < 0.001). In total, 52,305 NAT pairs were detected, although this number is an under-representation because only the best-matching partner for each transcript was retained in our analysis.
We conducted GO enrichment analysis to investigate the potential functional roles of NATs in pokeweed. As shown in Figure 4, NATs were enriched in 24 GO terms relative to all pokeweed transcripts from the raw assembly (FDR < 0.001). Of these, “chloroplast stroma” was most significant and three other chloroplast-related terms were also enriched: “chloroplast thylakoid membrane,” “chlorophyll biosynthetic process,” and “chloroplast thylakoid lumen.” From the 52,305 pairs of NATs, 2,502 transcripts were differentially expressed (FDR < 0.001, FC ≥ 4). Of the differentially expressed NATs, 88 pairs involved both partners, suggesting co-regulation in response to JA. The 88 pairs were enriched in three GO terms relative to all pokeweed transcripts: “nitrate assimilation,” “nitrate reductase (NADH) activity,” and “molybdopterin cofactor binding” (FDR < 0.001). The transcripts relating to these terms were annotated as NRT1/PTR FAMILY members and nitrate reductases. Therefore, it appears that in pokeweed, NATs in general are important in the chloroplast, while JA-responsive NATs may regulate nitrate metabolism.
FIGURE 4. Functional analysis of NATs in pokeweed. GO enrichment analysis was conducted on all NATs (Test Set) against the raw pokeweed transcriptome assembly (Reference Set). Enriched terms (FDR < 0.001) are listed in order of increasing significance (bottom to top).
As shown in Table 7, four PAP isoforms had significant NAT counterparts (PAP-I, PAP-II, and the two potentially novel isoforms). Each NAT showed perfect alignment identity and nearly full-length coverage with its respective PAP isoform. Interestingly, the expression of PAP isoforms and their corresponding NATs showed a strong positive correlation, with an R2 value of 0.909 (Figure 5). We cloned and sequenced the PAP-I NAT (c61645_g1_i1) from pokeweed total RNA to validate its expression. Furthermore, qRT-PCR of PAP-I NAT from JA-treated plants indicated a significant increase of 6.68 fold (log2FC of 2.74; Student’s t-test, p < 0.05; Figure 3). These results confirm the expression of a JA-responsive transcript that is antisense to the PAP-I sequence. In addition, they suggest that certain PAP isoforms may be regulated by NATs, which would constitute a novel form of PAP gene regulation.
FIGURE 5. Expression of PAP sense and antisense transcripts. The correlation of JA-induced expression changes obtained by RNA-seq is shown for each PAP isoform and its corresponding NAT.
Through transcriptome assembly, annotation and differential expression analysis, we identified genes that are significantly affected by JA and could mediate defense against pathogens and herbivores in P. americana. We validated the existence of several previously reported PAP isoforms and characterized their differential expression patterns. This work also led to the discovery of a potentially novel PAP isoform and identification of NATs that may regulate PAP gene expression.
We generated a robust pokeweed mRNA transcriptome by combining strand-specific, paired-end sequencing reads from biological replicates of JA-treated and control plants. Over 400 million processed reads were leveraged for assembly, which is considered very deep sequencing (Haas et al., 2013). Comparison of our assembly with sugar beet, a well-studied plant from the same taxonomic order as pokeweed, reveals important similarities to help validate our results. For example, de novo assembly of the sugar beet mRNA transcriptome yielded a total of 225,385 transcripts from 165,742 unique loci, providing an N50 value of 1,185 bp (Mutasa-Göttgens et al., 2012). These statistics are comparable to our pokeweed Raw assembly, which had 216,891 transcripts, 177,709 unigenes and an N50 value of 1,168 bp. Furthermore, others reported that approximately 80% of sugar beet unigenes are between 200 and 500 bp in length (Fugate et al., 2014), in agreement with the abundance of short transcripts we detected in pokeweed. The enrichment of short transcripts seems to be a general trend amongst de novo assembled transcriptomes, perhaps due to the assembly algorithm which relies on short overlapping sub-sequences known as k-mers (Ranjan et al., 2014). Through BLASTx searches against public sequence databases, we were able to annotate 59,096 transcripts. This is in line with other studies of non-model plants, with examples of approximately 40–50,000 annotated transcripts or unigenes (Farrell et al., 2014; Jin et al., 2014; Ranjan et al., 2014). Taken together, reports from other plants support the validity of our pokeweed assembly.
We were interested in identifying JA-responsive genes in pokeweed because this hormone mediates broad-spectrum defense. We reported 165 annotated transcripts specific to JA treatment. Many of these transcripts encoded factors involved in JA biosynthesis, JA signaling and JA-mediated defense, which have been identified in other transcriptome-wide studies involving transgenic plants deficient in JA biosynthesis or signaling (Abuqamar et al., 2006; Ku et al., 2011; Yan et al., 2014). Identification of these JA responses allows us to integrate PAP within broader defense pathways of pokeweed.
We also sought to identify genes other than PAP that may contribute to defense in this plant. One interesting candidate was intracellular ribonuclease LX, which showed a 14,664-fold increase with JA. Such changes are not uncommon in plants responding to biotic and abiotic stresses (Liu et al., 2013; Pierce and Rey, 2013; Li et al., 2014). This gene, characterized in tomato, is involved in programmed cell death responses including senescence and is thought to contribute to nutrient recycling by mediating RNA turnover (Lehmann et al., 2001; Lers et al., 2006). It is well-established that jasmonate is associated with localized cell death as part of the hypersensitive response in plants, providing a possible explanation for the increased expression of this gene in JA-treated plants. Future work will investigate if this enzyme has a role in pokeweed defense, as pathogen-responsive RNases have been reported in other plants (Galiana et al., 1997; Ayashi et al., 2003).
Another markedly up-regulated gene in our study was annotated as bark storage protein A and is involved in nitrogen storage in senescing leaves (Wetzel et al., 1989; Coleman et al., 1991). Storage proteins have previously been linked to jasmonate-mediated responses, such as VSP2 in Arabidopsis, an anti-insect acid phosphatase (Liu et al., 2005). A final candidate is defensin-like protein, which, in addition to being differentially expressed, was also the most abundant transcript in JA-treated plants. In Arabidopsis, the defensin PDF1.2 is a well-established marker of the ethylene and jasmonate signaling pathway that mediates resistance to pathogens (Manners et al., 1998; Penninckx et al., 1998). Identification of JA-responsive genes in pokeweed will allow a broader characterization of factors involved in defense in this plant. Furthermore, since only 38% of expressed pokeweed transcripts were annotated, this non-model plant transcriptome represents a source of new genes that could have agricultural benefits.
Through transcriptome annotation, we confirmed the presence of the following isoforms in pokeweed leaf tissue from 4-leaf plants: PAP-I, PAP-II, PAP-S, and PAP-alpha. Based on previous reports, PAP-I is expressed in spring leaves (Irvin, 1975), PAP-II in early summer leaves (Irvin et al., 1980), PAP-S in seeds (Barbieri et al., 1982) and PAP-alpha in various tissues (Kataoka et al., 1992). We also presented sequence and qRT-PCR evidence to support the existence of a novel PAP isoform. Our finding that PAP-I was the most abundant isoform in young plants, followed by PAP-II, agrees with their documented temporal profiles. Furthermore, the high abundance of the isoforms corresponds with the report that PAP comprises up to 0.5% of total soluble protein in leaves (Bonness et al., 1994). Interestingly, we only found one annotated PAP-S isoform. This disagrees with a previous finding that PAP-S is a mix of two seed isoforms, PAP-S1 and PAP-S2, having 85% nucleotide sequence identity (Honjo et al., 2002). Our result was not likely due to the assembly algorithm, since Trinity is able to differentiate between sequences that are up to 95% identical (Grabherr et al., 2011). This does not preclude the possibility that two PAP-S isoforms exist in seeds, as we only investigated leaf tissue. Furthermore, we did not identify PAP-R or PAP-III isoforms, which have been purified from root tissue and late summer leaves, respectively (Bolognesi et al., 1990; Rajamohan et al., 1999).
RNA-seq analysis allowed us to compare the expression patterns of multiple PAP isoforms simultaneously. This level of distinction in a single experiment has not yet been possible, likely due to difficulties related to high sequence identity amongst isoforms. With the exception of PAP-II, the nucleotide sequences of isoforms are >70% identical. In the current study, we found that all isoforms other than c18776_g1_i1 were significantly up-regulated with JA. PAP isoforms exhibited differences in terms of abundance and FC. Such differences could indicate that they have specialized roles in defense, or more generally within the plant. For example, analysis of two RIP isoforms in spinach revealed that one was highly expressed in embryos and not responsive to salicylic acid, while the other showed weak expression throughout the plant but was induced with salicylic acid (Kawade and Masuda, 2009). Authors suggested that the two RIPs may have different functions, one in embryogenesis and the other in defense. In pokeweed, PAP isoforms differ in their ability to depurinate eukaryotic and prokaryotic ribosomes in vitro (Honjo et al., 2002). A comprehensive RNA-seq study that includes different developmental stages and stresses would allow further insight into the possible roles of PAP isoforms.
Natural antisense transcripts are pairs of endogenous transcripts with high sequence complementarity, capable of forming double-stranded RNA and affecting gene expression in cis or trans. Cis-NATs are transcribed from the same genomic locus but opposite orientations and have perfect sequence complementarity, while trans-NATs are derived from different loci. Our sequence analysis identified over 52,000 NAT pairs in pokeweed. A recent study identified 37,238 NAT pairs in Arabidopsis and an astonishing 70% of annotated mRNAs were associated with antisense transcripts (Wang et al., 2014). Furthermore, 60% of NAT pairs were comprised of fully overlapping transcripts. In pokeweed, we found perfectly identical, fully overlapping antisense transcripts of PAP-I, PAP-II, c18776_g1_i1, and c115037_g1_i1. The fact that each NAT was perfectly complementary to its respective isoform suggests that they act in cis. We also found that PAP NATs and their corresponding sense transcripts had positively correlated responses to JA, indicating that NATs may regulate PAP expression. This agrees with studies in humans and other mammals which report significant positive correlations between sense and antisense transcription (Oeder et al., 2007; Ling et al., 2013). Fewer genome-wide studies of NAT transcription have been performed for plants. In Arabidopsis, a study of light-responsive long non-coding NATs identified 626 positively correlated and 766 negatively correlated pairs (Wang et al., 2014). NATs have been proposed to modulate gene expression at various levels, including transcription, post-transcriptional small RNA interference, mRNA splicing and RNA stability (Pelechano and Steinmetz, 2013; Zhang et al., 2013; Liu et al., 2015). Future work will investigate mechanisms by which NATs may regulate PAP expression.
Apart from PAP regulation, we conducted preliminary investigations into the broader roles of NATs in pokeweed. As a group, NATs were enriched in several GO terms relating to the chloroplast. Although the implications of this are unknown, it agrees with a previous report from Arabidopsis that the chloroplast encodes a diverse group of antisense RNAs mapping to protein-coding genes (Hotto et al., 2011). Approximately 2,500 NATs were JA-responsive and 88 pairs were considered biologically relevant because both sense and antisense strands were differentially expressed. These NAT pairs were enriched in GO terms relating to nitrate metabolism and the involved transcripts were annotated as NRT1/PTR FAMILY members and nitrate reductases. These enzymes have well-established roles in nitrate assimilation, which involves the reduction of nitrate to nitrite, followed by ammonium, which is ultimately incorporated into amino acids for plant growth (Dechorgnat et al., 2010). Importantly, the reduction of nitrite to ammonium occurs in chloroplasts, further supporting the connection between NATs and this specific organelle. Furthermore, NRT1/PTR FAMILY members capable of transporting jasmonoyl-isoleucine, the bioactive form of jasmonate, were recently identified (Chiba and Shimizu, 2015). Given that plant stress decouples nitrate assimilation and photosynthesis, mediated by the jasmonate/ethylene signaling pathway (Zhang et al., 2014), NATs in pokeweed may be involved in regulating the trade-off between stress response and plant growth.
Here, we have assembled and annotated the pokeweed leaf mRNA transcriptome under JA treatment. In addition to the identification of many differentially expressed transcripts, we also characterized the expression of multiple PAP isoforms through RNA-seq analysis, including a potentially novel isoform of PAP. Finally, we present the first report of NATs in the pokeweed plant and confirmed expression of a PAP-NAT, which may indicate a novel form of RIP gene regulation. The pokeweed transcriptome will enable further investigations into the robust defense strategies of this species and other Phytolaccaceae family members. Heterologous expression of PAP has already been applied successfully to produce virus resistant plants (Lodge et al., 1993; Zoubenko et al., 1997; Wang et al., 1998; Dai et al., 2003). Pokeweed accumulates high levels of metal from contaminated soils but little is known about the genes mediating this tolerance (Dou et al., 2009; Zhao et al., 2011, 2012). With a complete repository of pokeweed mRNA sequences, we anticipate the discovery of beneficial genes in this plant that could improve the resiliency of agricultural crops.
KN and KH conceived the design and coordination of the study. KN performed bioinformatic analyses, statistical testing and drafted the manuscript. AK carried out qRT-PCR validations and cloning. KH edited the manuscript. All authors read and approved the final manuscript.
This work was funded by a Discovery Grant to KH from the Natural Sciences and Engineering Research Council of Canada and CGS-M Scholarships to KN and AK.
The authors declare that the research was conducted in the absence of any commercial or financial relationships that could be construed as a potential conflict of interest.
The authors gratefully acknowledge permission to reproduce the KEGG pathway image #00592 from http://www.kegg.jp/kegg/kegg1.html used in Supplementary Figure S4.
The Supplementary Material for this article can be found online at: http://journal.frontiersin.org/article/10.3389/fpls.2016.00283
E, ethanol; FC, fold change; FDR, false discovery rate; FPKM, fragments per kilobase per transcript per million mapped reads; GO, gene ontology; JA, jasmonic acid; NAT, natural antisense transcript; ORF, open reading frame; PAP, pokeweed antiviral protein; RIP, ribosome inactivating protein; TMM, trimmed mean of m-values.
Abuqamar, S., Chen, X., Dhawan, R., Bluhm, B., Salmeron, J., Lam, S., et al. (2006). Expression profiling and mutant analysis reveals complex regulatory networks involved in Arabidopsis response to Botrytis infection. Plant J. 48, 28–44. doi: 10.1111/j.1365-313X.2006.02849.x
Altschul, S. F., Madden, T. L., Schäffer, A. A., Zhang, J., Zhang, Z., Miller, W., et al. (1997). Gapped BLAST and PSI-BLAST: a new generation of protein database search programs. Nucleic Acids Res. 25, 3389–3402. doi: 10.1093/nar/25.17.3389
Ayashi, T. H., Obayashi, D. K., Ariu, T. K., Ahara, M. T., Ada, K. H., Ouzuma, Y. K., et al. (2003). Genomic cloning of ribonucleases in Nicotiana glutinosa leaves, as induced in response to wounding or to TMV-infection, and characterization of their promoters. Biosci. Biotechnol. Biochem. 67, 2574–2583. doi: 10.1271/bbb.67.2574
Bairoch, A., and Boeckmann, B. (1991). The swiss-prot protein-sequence data-bank. Nucleic Acids Res. 22, 3093–3096.
Barbieri, L., Aron, G. M., Irvin, J. D., and Stirpe, F. (1982). Purification and partial characterization of another form of the antiviral protein from the seeds of Phytolacca americana L. (pokeweed). Biochem. J. 203, 55–59. doi: 10.1042/bj2030055
Blankenberg, D., Kuster, G., Von Coraor, N., Ananda, G., Lazarus, R., Mangan, M., et al. (2010). Galaxy: a web-based genome analysis tool for experimentalists. Curr. Protoc. Mol. Biol. 19, 19.10.1-21. doi: 10.1002/0471142727.mb1910s89
Bolger, A. M., Lohse, M., and Usadel, B. (2014). Trimmomatic: a flexible trimmer for Illumina sequence data. Bioinformatics 30, 2114–2120. doi: 10.1093/bioinformatics/btu170
Bolognesi, A., Barbieri, L., Abbondanza, A., Falasca, A. I., Carnicelli, D., Battelli, M. G., et al. (1990). Purification and properties of new ribosome-inactivating proteins with RNA N-glycosidase activity. Biochim. Biophys. Acta 1087, 293–302. doi: 10.1016/0167-4781(90)90002-J
Bonness, M. S., Ready, M. P., Irvin, J. D., and Mabry, T. J. (1994). Pokeweed antiviral protein inactivates pokeweed ribosomes; implications for the antiviral mechanism. Plant J. 5, 173–183. doi: 10.1046/j.1365-313X.1994.05020173.x
Chiba, Y., and Shimizu, T. (2015). Identification of Arabidopsis thaliana NRT1/PTR FAMILY (NPF) proteins capable of transporting plant hormones. J. Plant Res. 128, 679–686. doi: 10.1007/s10265-015-0710-2
Chuang, W.-P., Herde, M., Ray, S., Castano-Duque, L., Howe, G. A., and Luthe, D. S. (2014). Caterpillar attack triggers accumulation of the toxic maize protein RIP2. New Phytol. 201, 928–939. doi: 10.1111/nph.12581
Coleman, G. D., Chen, T. H., Ernst, S. G., and Fuchigami, L. (1991). Photoperiod control of poplar bark storage protein accumulation. Plant Physiol. 96, 686–692. doi: 10.1104/pp.96.3.686
Conesa, A., Götz, S., García-Gómez, J. M., Terol, J., Talón, M., and Robles, M. (2005). Blast2GO: a universal tool for annotation, visualization and analysis in functional genomics research. Bioinformatics 21, 3674–3676. doi: 10.1093/bioinformatics/bti610
Dai, W. D., Bonos, S., Guo, Z., Meyer, W. A., Day, P. R., and Belanger, F. C. (2003). Expression of pokeweed antiviral proteins in creeping bentgrass. Plant Cell Rep. 21, 497–502. doi: 10.1007/s00299-002-0534-0
Dechorgnat, J., Chardon, F., and Gaufichon, L. (2010). Nitrogen uptake, assimilation and remobilization in plants?: challenges for sustainable and productive agriculture. Ann. Bot. 105, 1141–1157. doi: 10.1093/aob/mcq028
Di Maro, A., Citores, L., Russo, R., Iglesias, R., and Ferreras, J. M. (2014). Sequence comparison and phylogenetic analysis by the maximum likelihood method of ribosome-inactivating proteins from angiosperms. Plant Mol. Biol. 85, 575–588. doi: 10.1007/s11103-014-0204-y
Dou, C. M., Fu, X. P., Chen, X. C., Shi, J. Y., and Chen, Y. X. (2009). Accumulation and detoxification of manganese in hyperaccumulator Phytolacca americana. Plant Biol. (Stuttg.) 11, 664–670. doi: 10.1111/j.1438-8677.2008.00163.x
Dunaeva, M., Goebel, C., Wasternack, C., Parthier, B., and Goerschen, E. (1999). The jasmonate-induced 60 kDa protein of barley exhibits N-glycosidase activity in vivo. FEBS Lett. 452, 263–266. doi: 10.1016/S0014-5793(99)00645-6
Farrell, J. D., Byrne, S., Paina, C., and Asp, T. (2014). De novo assembly of the perennial ryegrass transcriptome using an RNA-Seq strategy. PLoS ONE 9:e103567. doi: 10.1371/journal.pone.0103567
Finn, R. D., Bateman, A., Clements, J., Coggill, P., Eberhardt, R. Y., Eddy, S. R., et al. (2014). Pfam: the protein families database. Nucleic Acids Res. 42, D222–D230. doi: 10.1093/nar/gkt1223
Finn, R. D., Clements, J., and Eddy, S. R. (2011). HMMER web server: interactive sequence similarity searching. Nucleic Acids Res. 39, W29–W37. doi: 10.1093/nar/gkr367
Fugate, K. K., Fajardo, D., Schlautman, B., Ferrareze, J. P., Bolton, M. D., Campbell, L. G., et al. (2014). Generation and characterization of a sugarbeet transcriptome and transcript-based SSR markers. Plant Genome 7, 1–23. doi: 10.3835/plantgenome2013.11.0038
Galiana, E., Bonnet, P., Conrod, S., Keller, H., Panabières, F., Ponchet, M., et al. (1997). RNase activity prevents the growth of a fungal pathogen in tobacco leaves and increases upon induction of systemic acquired resistance with elicitin. Plant Physiol. 115, 1557–1567. doi: 10.1104/pp.115.4.1557
Giardine, B., Riemer, C., Hardison, R. C., Burhans, R., Elnitski, L., Shah, P., et al. (2005). Galaxy: a platform for interactive large-scale genome analysis. Genome Res. 15, 1451–1455. doi: 10.1101/gr.4086505
Goecks, J., Nekrutenko, A., and Taylor, J. (2010). Galaxy: a comprehensive approach for supporting accessible, reproducible, and transparent computational research in the life sciences. Genome Biol. 11:R86. doi: 10.1186/gb-2010-11-8-r86
Grabherr, M. G., Haas, B. J., Yassour, M., Levin, J. Z., Thompson, D. A., Amit, I., et al. (2011). Full-length transcriptome assembly from RNA-Seq data without a reference genome. Nat. Biotechnol. 29, 644–652. doi: 10.1038/nbt.1883
Haas, B. J., Papanicolaou, A., Yassour, M., Grabherr, M., Blood, P. D., Bowden, J., et al. (2013). De novo transcript sequence reconstruction from RNA-seq using the trinity platform for reference generation and analysis. Nat. Protoc. 8, 1494–1512. doi: 10.1038/nprot.2013.084
He, Y.-W., Guo, C.-X., Pan, Y.-F., Peng, C., and Weng, Z.-H. (2008). Inhibition of hepatitis B virus replication by pokeweed antiviral protein in vitro. World J. Gastroenterol. 14, 1592–1597. doi: 10.3748/wjg.14.1592
Honjo, E., Dong, D., Motoshima, H., and Watanabe, K. (2002). Genomic clones encoding two isoforms of pokeweed antiviral protein in seeds (PAP-S1 and S2) and the N-glycosidase activities of their recombinant proteins on ribosomes and DNA in comparison with other isoforms. J. Biochem. 131, 225–231. doi: 10.1093/oxfordjournals.jbchem.a003092
Hotto, A. M., Schmitz, R. J., Fei, Z., Ecker, J. R., and Stern, D. B. (2011). Unexpected diversity of chloroplast noncoding RNAs as revealed by deep sequencing of the Arabidopsis transcriptome. G3 (Bethesda) 1, 559–570. doi: 10.1534/g3.111.000752
Irvin, J. (1975). Purification and partial characterization of the antiviral protein from Phytolacca americana which inhibits eukaryotic protein synthesis. Arch. Biochem. Biophys. 169, 522–528. doi: 10.1016/0003-9861(75)90195-2
Irvin, J., Kelly, T., and Robertus, J. (1980). Purification and properties of a second antiviral protein from Phytolacca americana which inactivates eukaryotic ribosomes. Arch. Biochem. Biophys. 200, 418–425. doi: 10.1016/0003-9861(80)90372-0
Jin, J., Panicker, D., Wang, Q., Kim, M. J., Liu, J., Yin, J.-L., et al. (2014). Next generation sequencing unravels the biosynthetic ability of Spearmint (Mentha spicata) peltate glandular trichomes through comparative transcriptomics. BMC Plant Biol. 14:292. doi: 10.1186/s12870-014-0292-5
Karran, R. A., and Hudak, K. A. (2008). Depurination within the intergenic region of Brome mosaic virus RNA3 inhibits viral replication in vitro and in vivo. Nucleic Acids Res. 36, 7230–7239. doi: 10.1093/nar/gkn896
Kataoka, J., Habuka, N., Masuta, C., Miyano, M., and Koiwai, A. (1992). Isolation and analysis of a genomic clone encoding a pokeweed antiviral protein. Plant Mol. Biol. 20, 879–886. doi: 10.1007/BF00027159
Kawade, K., and Masuda, K. (2009). Transcriptional control of two ribosome-inactivating protein genes expressed in spinach (Spinacia oleracea) embryos. Plant Physiol. Biochem. 47, 327–334. doi: 10.1016/j.plaphy.2008.12.020
Klenov, A., Neller, K. C. M., Burns, L. A., Krivdova, G., and Hudak, K. A. (2015). A small RNA targets pokeweed antiviral protein transcript. Physiol. Plant. 156, 241–251. doi: 10.1111/ppl.12393
Ku, A., Tran, D. H. T., Winge, P., Jørstad, T. S., Reese, J. C., and Troczy, J. (2011). Testing the importance of jasmonate signalling in induction of plant defences upon cabbage aphid (Brevicoryne brassicae) attack. BMC Genomics 12:423. doi: 10.1186/1471-2164-12-423
Langmead, B., Trapnell, C., Pop, M., and Salzberg, S. L. (2009). Ultrafast and memory-efficient alignment of short DNA sequences to the human genome. Genome Biol. 10:R25. doi: 10.1186/gb-2009-10-3-r25
Lehmann, K., Hause, B., Altmann, D., and Köck, M. (2001). Tomato ribonuclease LX with the functional endoplasmic reticulum retention motif HDEF is expressed during programmed cell death processes, including xylem differentiation, germination, and senescence. Plant Physiol. 127, 436–449. doi: 10.1104/pp.010362
Lers, A., Sonego, L., Green, P. J., and Burd, S. (2006). Suppression of LX ribonuclease in tomato results in a delay of leaf senescence and abscission. Plant Physiol. 142, 710–721. doi: 10.1104/pp.106.080135
Li, B., and Dewey, C. N. (2011). RSEM: accurate transcript quantification from RNA-Seq data with or without a reference genome. BMC Bioinformatics 12:323. doi: 10.1186/1471-2105-12-323
Li, X., Lu, J., Liu, S., Liu, X., Lin, Y., and Li, L. (2014). Identification of rapidly induced genes in the response of peanut (Arachis hypogaea) to water deficit and abscisic acid. BMC Biotechnol. 14:58. doi: 10.1186/1472-6750-14-58
Ling, M. H. T., Ban, Y., Wen, H., Wang, S. M., and Ge, S. X. (2013). Conserved expression of natural antisense transcripts in mammals. BMC Genomics 14:243. doi: 10.1186/1471-2164-14-243
Liu, J.-J., Sturrock, R. N., and Benton, R. (2013). Transcriptome analysis of Pinus monticola primary needles by RNA-seq provides novel insight into host resistance to Cronartium ribicola. BMC Genomics 14:884. doi: 10.1186/1471-2164-14-884
Liu, X., Hao, L., Li, D., Zhu, L., and Hu, S. (2015). Long Non-coding RNAs and their biological roles in plants. Genomics Proteomics Bioinformatics 13, 137–147. doi: 10.1016/j.gpb.2015.02.003
Liu, Y., Ahn, J.-E., Datta, S., Salzman, R. A., Moon, J., Huyghues-Despointes, B., et al. (2005). Arabidopsis vegetative storage protein is an anti-insect acid phosphatase. Plant Physiol. 139, 1545–1556. doi: 10.1104/pp.105.066837
Lodge, J. K., Kaniewski, W. K., and Tumer, N. E. (1993). Broad-spectrum virus resistance in transgenic plants expressing pokeweed antiviral protein. Proc. Natl. Acad. Sci. U.S.A. 90, 7089–7093. doi: 10.1073/pnas.90.15.7089
Manners, J. M., Penninckx, I. A. M. A., Vermaere, K., Kazan, K., Brown, R. L., Morgan, A., et al. (1998). The promoter of the plant defensin gene PDF1.2 from Arabidopsis is systemically activated by fungal pathogens and responds to methyl jasmonate but not to salicylic acid. Plant Mol. Biol. 38, 1071–1080. doi: 10.1023/A:1006070413843
Mansouri, S., Choudhary, G., Sarzala, P. M., Ratner, L., and Hudak, K. A. (2009). Suppression of human T-cell leukemia virus I gene expression by pokeweed antiviral protein. J. Biol. Chem. 284, 31453–31462. doi: 10.1074/jbc.M109.046235
Mutasa-Göttgens, E. S., Joshi, A., Holmes, H. F., Hedden, P., and Göttgens, B. (2012). A new RNASeq-based reference transcriptome for sugar beet and its application in transcriptome-scale analysis of vernalization and gibberellin responses. BMC Genomics 13:99. doi: 10.1186/1471-2164-13-99
Oeder, S., Mages, J., Flicek, P., and Lang, R. (2007). Uncovering information on expression of natural antisense transcripts in Affymetrix MOE430 datasets. BMC Genomics 8:200. doi: 10.1186/1471-2164-8-200
Pelechano, V., and Steinmetz, L. M. (2013). Gene regulation by antisense transcription. Nat. Rev. Genet. 14, 880–893. doi: 10.1038/nrg3594
Penninckx, I. A., Thomma, B. P., Buchala, A., Métraux, J. P., and Broekaert, W. F. (1998). Concomitant activation of jasmonate and ethylene response pathways is required for induction of a plant defensin gene in Arabidopsis. Plant Cell 10, 2103–2113. doi: 10.1105/tpc.10.12.2103
Pierce, E. J., and Rey, M. E. C. (2013). Assessing global transcriptome changes in response to south african cassava mosaic virus [ZA-99] infection in susceptible Arabidopsis thaliana. PLoS ONE 8:e67534. doi: 10.1371/journal.pone.0067534
R Development Core Team (2011). R: A Language and Environment for Statistical Computing. Vienna: R Foundation Statistical Computing. doi: 10.1007/978-3-540-74686-7 1, 409.
Rajamohan, F., Venkatachalam, T. K., Irvin, J. D., and Uckun, F. M. (1999). Pokeweed antiviral protein isoforms PAP-I, PAP-II, and PAP-III depurinate RNA of human immunodeficiency virus (HIV)-1. Biochem. Biophys. Res. Commun. 260, 453–458. doi: 10.1006/bbrc.1999.0922
Ranjan, A., Ichihashi, Y., Farhi, M., Zumstein, K., Townsley, B., David-Schwartz, R., et al. (2014). De novo assembly and characterization of the transcriptome of the parasitic weed dodder identifies genes associated with plant parasitism. Plant Physiol. 166, 1186–1199. doi: 10.1104/pp.113.234864
Robinson, M. D., McCarthy, D. J., and Smyth, G. K. (2010). edgeR: a Bioconductor package for differential expression analysis of digital gene expression data. Bioinformatics 26, 139–140. doi: 10.1093/bioinformatics/btp616
Song, S. K., Choi, Y., Moon, Y. H., Kim, S. G., Choi, Y. D., and Lee, J. S. (2000). Systemic induction of a Phytolacca insularis antiviral protein gene by mechanical wounding, jasmonic acid, and abscisic acid. Plant Mol. Biol. 43, 439–450. doi: 10.1023/A:1006444322626
Stirpe, F. (2013). Ribosome-inactivating proteins: from toxins to useful proteins. Toxicon 67, 12–16. doi: 10.1016/j.toxicon.2013.02.005
Suzek, B. E., Huang, H., McGarvey, P., Mazumder, R., and Wu, C. H. (2007). UniRef: comprehensive and non-redundant UniProt reference clusters. Bioinformatics 23, 1282–1288. doi: 10.1093/bioinformatics/btm098
Vepachedu, R., Bais, H. P., and Vivanco, J. M. (2003). Molecular characterization and post-transcriptional regulation of ME1, a type-I ribosome-inactivating protein from Mirabilis expansa. Planta 217, 498–506. doi: 10.1007/s00425-003-1014-7
Wang, H., Chung, P. J., Liu, J., Jang, I. C., Kean, M. J., Xu, J., et al. (2014). Genome-wide identification of long noncoding natural antisense transcripts and their responses to light in Arabidopsis. Genome Res. 24, 444–453. doi: 10.1101/gr.165555.113
Wang, P., Zoubenko, O., and Tumer, N. E. (1998). Reduced toxicity and broad spectrum resistance to viral and fungal infection in transgenic plants expressing pokeweed antiviral protein II. Plant Mol. Biol. 38, 957–964. doi: 10.1023/A:1006033126011
Wetzel, S., Demmers, C., and Greenwood, J. S. (1989). Seasonally fluctuating bark proteins are a potential form of nitrogen storage in three temperate hardwoods. Planta 178, 275–281. doi: 10.1007/BF00391854
Yan, H., Yoo, M., Koh, J., Liu, L., Chen, Y., and Acikgoz, D. (2014). Molecular reprogramming of Arabidopsis in response to perturbation of jasmonate signaling. J. Proteome Res. 13, 5751–5766. doi: 10.1021/pr500739v
Zhang, G., Yi, H., and Gong, J. (2014). The Arabidopsis ethylene/jasmonic Acid-NRT signaling module coordinates nitrate reallocation and the trade-off between growth and environmental adaptation. Plant Cell 26, 3984–3998. doi: 10.1105/tpc.114.129296
Zhang, X., Lii, Y., Wu, Z., Polishko, A., Zhang, H., Chinnusamy, V., et al. (2013). Mechanisms of small RNA generation from Cis-NATs in response to environmental and developmental cues. Mol. Plant 6, 704–715. doi: 10.1093/mp/sst051
Zhao, H., Wu, L., Chai, T., Zhang, Y., Tan, J., and Ma, S. (2012). The effects of copper, manganese and zinc on plant growth and elemental accumulation in the manganese-hyperaccumulator Phytolacca americana. J. Plant Physiol. 169, 1243–1252. doi: 10.1016/j.jplph.2012.04.016
Zhao, L., Sun, Y., Le Cui, S. X., Chen, M., Yang, H. M., Liu, H. M., et al. (2011). Cd-induced changes in leaf proteome of the hyperaccumulator plant Phytolacca americana. Chemosphere 85, 56–66. doi: 10.1016/j.chemosphere.2011.06.029
Keywords: Phytolacca americana, pokeweed, RNA-seq, transcriptome, jasmonic acid, ribosome inactivating protein, pokeweed antiviral protein, natural antisense transcript
Citation: Neller KCM, Klenov A and Hudak KA (2016) The Pokeweed Leaf mRNA Transcriptome and Its Regulation by Jasmonic Acid. Front. Plant Sci. 7:283. doi: 10.3389/fpls.2016.00283
Received: 14 December 2015; Accepted: 22 February 2016;
Published: 16 March 2016.
Edited by:
Paula Casati, Centro de Estudios Fotosinteticos – Consejo Nacional de Investigaciones Científicas y Técnicas, ArgentinaReviewed by:
Elina Welchen, Universidad Nacional del Litoral, ArgentinaCopyright © 2016 Neller, Klenov and Hudak. This is an open-access article distributed under the terms of the Creative Commons Attribution License (CC BY). The use, distribution or reproduction in other forums is permitted, provided the original author(s) or licensor are credited and that the original publication in this journal is cited, in accordance with accepted academic practice. No use, distribution or reproduction is permitted which does not comply with these terms.
*Correspondence: Katalin A. Hudak, aHVkYWtAeW9ya3UuY2E=
Disclaimer: All claims expressed in this article are solely those of the authors and do not necessarily represent those of their affiliated organizations, or those of the publisher, the editors and the reviewers. Any product that may be evaluated in this article or claim that may be made by its manufacturer is not guaranteed or endorsed by the publisher.
Research integrity at Frontiers
Learn more about the work of our research integrity team to safeguard the quality of each article we publish.