- 1State Key Laboratory of Vegetation and Environmental Change, Institute of Botany, Chinese Academy of Science, Beijing, China
- 2University of Chinese Academy of Sciences, Beijing, China
- 3Chinese Academy of Meteorological Sciences, China Meteorological Administration, Beijing, China
In this study, the impact of future climate change on photosynthetic efficiency as well as energy partitioning in the Stipa bungeana was investigated by using chlorophyll fluorescence imaging (CFI) technique. Two thermal regimes (room temperature, T0: 23.0/17.0°C; High temperature, T6: 29.0/23.0°C) and three water conditions (Control, W0; Water deficit, W−30; excess precipitation, W+30) were set up in artificial control chambers. The results showed that excess precipitation had no significant effect on chlorophyll fluorescence parameters, while water deficit decreased the maximal quantum yield of photosystem II (PSII) photochemistry for the dark-adapted state (Fv/Fm) by 16.7%, with no large change in maximal quantum yield of PSII photochemistry for the light-adapted state (FV′/FM′) and coefficient of the photochemical quenching (qP) at T0 condition. Under T6 condition, high temperature offset the negative effect of water deficit on Fv/Fm and enhanced the positive effect of excess precipitation on Fv/Fm, Fv′/Fm′, and qP, the values of which all increased. This indicates that the temperature higher by 6°C will be beneficial to the photosynthetic performance of S. bungeana. Spatial changes of photosynthetic performance were monitored in three areas of interest (AOIs) located on the bottom, middle and upper position of leaf. Chlorophyll fluorescence images (Fv/Fm, actual quantum yield of PSII photochemistry for the light-adapted state (ΦPSII), quantum yield of non-regulated energy dissipation for the light-adapted state (ΦNO) at T0 condition, and ΦPSII at T6 condition) showed a large spatial variation, with greater value of ΦNO and lower values of Fv/Fm and ΦPSII in the upper position of leaves. Moreover, there was a closer relationship between ΦPSII and ΦNO, suggesting that the energy dissipation by non-regulated quenching mechanisms played a dominant role in the yield of PSII photochemistry. It was also found that, among all measured fluorescence parameters, the Fv/Fm ratio was most sensitive to precipitation change at T0, while ΦPSII was the most sensitive indicator at T6.
Introduction
High temperature and water stress as abiotic stress factors will limit plant growth and reduce crop productivity (Boyer, 1982; Wahid et al., 2007), and they always occur simultaneously in that high temperature increases both evaporation and potential evapotranspiration and exacerbates the negative influence of water deficit (Machado and Paulsen, 2001; Osório et al., 2011). Models of global climate change have predicted that the globally averaged surface temperature will be 1.5–4.0°C higher till 2100 and the extreme precipitation events will occur more frequently than before (IPCC, 2013). According to the study by Xu et al. (2009), temperature and precipitation change determine the physiological response of perennial grass to new environmental conditions to a large extent. Among all plant physiological functions, photosynthesis plays a pivotal role in plant carbon uptake, plant growth and biomass accumulation. It is commonly considered that stomatal limitation which influences the substomatal CO2 concentration is the main reason for the reduction of photosynthesis under moderate water deficit (Cornic, 2000). The limitation on CO2 assimilation may damage the balance between photochemical activity in photosystem II (PSII) and electron requirement for photosynthesis, resulting in the photodamage of PSII centers. Although plant photosynthetic apparatus appears to be highly resistant to water deficit (Giardi et al., 1996; Petsas and Grammatikopoulos, 2009; Zivcak et al., 2014), temperature rising can change the response of photosynthesis to water stress (Chaves et al., 2002). Among all plant physiological activities, photosynthesis has been proved to be most sensitive to high temperature and can be inhibited entirely by heat stress before other plant physiological symptoms occur (Berry and Bjorkman, 1980). High temperature damages several photosynthetic functions, such as Calvin cycle, photosystem I (PSI) and PSII. Many studies have reported that the cooperative effect of water stress and high temperature is more drastic than their single effect (Albert et al., 2011; Thomey et al., 2011; Bauweraerts et al., 2013). When water and heat stress occur simultaneously, water stress may impose a certain effect on the photosynthesis together with temperature through oxidative damage (Chaves et al., 2002). On this basis, the inhibitory effect and damage on photosynthesis can be studied when the two stresses coexist, even at a low light intensity.
For the quantitative detection of the changes in the photosynthetic apparatus and photosynthetic activity under various environmental stresses, chlorophyll fluorescence measurement has been demonstrated to be a fast, non-destructive, sensitive and reliable method (Berry and Bjorkman, 1980; Havaux, 1992; Martínez-Carrasco et al., 2002; Mielke et al., 2003; Xu et al., 2004; Xu and Zhou, 2006; Swoczyna et al., 2010; Tuba et al., 2010; Ogaya et al., 2011; Brestic et al., 2014; Kalaji et al., 2014; Lazár, 2015). However, the conventional chlorophyll fluorescence measurement approach is based on point measurements and cannot exhibit the physiological status of a whole plant (Lichtenthaler and Miehé, 1997; Ehlert and Hincha, 2008). Furthermore, habitual heterogeneity of photosynthetic activity over the leaf surface makes this approach highly error-prone (Ehlert and Hincha, 2008). To overcome these problems, a more advanced technique, chlorophyll fluorescence imaging (CFI), was developed to take a powerful role in identifying spatial heterogeneity of leaf photosynthetic performance (Omasa et al., 1987; Baker and Rosenqvist, 2004; Ivanov and Bernards, 2015). This provides new possibilities to understand the regulation mechanism of photosynthesis, and to assess the properties of the photosynthetic apparatus and the extent to which the plants are affected by different stresses (Gorbe and Calatayud, 2012; Shaw et al., 2014; Humplík et al., 2015; Ivanov and Bernards, 2015). One of the first works on experiments with CFI were carried out by Omasa et al. (1987). In their work, the analysis of CFI was proved to be a useful method in early warning diagnosis, functional analysis of disorders during environment stress and plant's ability to recover. CFI can also be used to study plant response to dynamic climate control as image information is the most intuitive, easily comprehensible, and provides useful information on plant status (Omasa, 1990; Calatayud et al., 2006; Gorbe and Calatayud, 2012). Because CFI detects fluorescence signal pixel-by-pixel, it also provides huge amount of data which can be used for a sophisticated statistical treatments which can lead to an early detection of plant stress (Lazár et al., 2006).
As a key vegetation type dominating the typical steppe in Loess Plateau, Stipa bungeana is a useful plant species which can control water loss and soil erosion and improve the ecological environment effectively for its developed root system. It is also a type of appetizing forage with high nutritive value for livestocks. Hence, the research concerning the photosynthetic physiological responses of S. bungeana to the major stresses becomes increasingly important in the context of the predicted future climatic changes. In the present study, it was confirmed that CFI is a useful and convenient method for detecting the physiological mechanism of response to higher temperature and precipitation change. Moreover, the spatial variations of chlorophyll a (Chl a) fluorescence parameters of S. bungeana under different environmental stresses were analyzed. This work aims to evaluate the impact of high temperature and precipitation change on the photosynthetic performance and the utilization of excess excitation energy in photosynthetic apparatus of S. bungeana. Specifically, the following questions were addressed: (1) Are there any negative or positive impact of high temperature and precipitation change on the photosynthetic apparatus of S. bungeana? (2) What are the mechanisms of PSII photoprotection for S. bungeana? (3) Which is the most sensitive fluorescence parameter in predicting the impact of future climate change on S. bungeana?
Materials
Plant Material and Growth Conditions
To understand the effects of high temperature and precipitation change on S. bungeana's photosynthesis characteristics, the changes of water and heat conditions were controlled for the seedlings germinated from seeds. The experiment was carried out at the Institute of Botany, Chinese Academy of Sciences. The seeds of S. bungeana were obtained from the grassland in Dongsheng (39°82′N, 110°00′E), Inner Mongolia. They were sterilized by soaking in 0.7% potassium permanganate solution for 8 min and rinsed. Then, these seeds were sown in plastic pots wrapped with plastic film. Each plastic pot was filled with 4.08 kg of dry soil and planted with four plants. In the chestnut soil, the organic carbon content was 12.3 g·kg−1 and the total nitrogen content was 1.45 g·kg−1. Polyethylene pots were used as the experimental containers, which were lined with plastic bags to prevent water leakage.
Different temperature and precipitation treatments were set according to the monthly average temperature and precipitation during S. bungeana 's blooming stage in the past 30 years (1978-2007). Considering the diurnal temperature variations, two temperature treatments 23.0/17.0°C (T0) and 29.0/23.0°C (T6) were selected for experiment. Three precipitation regimes were set: average monthly precipitation over 30 years (W0: 82.3 mm); the average increased by 30% (W+30); the average decreased by 30% (W−30). All the plants were grown in a naturally illuminated glasshouse (the CO2 concentration was maintained at 390 ppm with a photosynthetic photon flux density of 1000 μmol photons m−2·s−1) and the timing used for day/night regime was 16 h light/8 h dark.
Imaging of Chlorophyll Fluorescence Measurement
In order to investigate the spatial heterogeneity of Chl fluorescence parameters, Chl fluorescence imaging of leaves was performed by using an imaging-PAM fluorometer (Walz, Effeltrich, Germany). Chl a fluorescence parameter was measured in the healthy and fully expanded leaves of three plants from each treatment. To evaluate spatial heterogeneity, three areas of interest (AOI, AOI type: Rectangle) in the same leaf were selected, the first one in the bottom position of the leaf (AOI1), the second one in the middle position (AOI2) and the third one in the upper position (AOI3). Three replicates of each plant were used for AOI determination. All plants were placed in dark for 10 min before measurement. Images of maximum fluorescence in the dark-adapted state, Fm, was determined by applying a blue saturation pulse. The saturation pulse intensity was 8000 μmol photons m−2·s−1 for 0.8 s. Minimum Chl fluorescence yield F0 was determined using low frequency light pulses (0.5 μmol photons m−2·s−1). Then the images of maximal quantum yield of PSII photochemistry for the dark-adapted state Fv/Fm were captured, and Fv/Fm ratio were obtained as . To determine the maximum fluorescence yield in the light-adapted state (Fm′) and Chl fluorescence during actinic illumination (Fs), actinic illumination (336 μmol photons m−2·s−1) was switched on and saturating pulses were applied at 20 s intervals for 5 min. All the fluorescence levels for the light-adapted state of the sample were determined at the end of 5 min. The maximal quantum yield of PSII photochemistry for the light-adapted state was estimated by the Fv′/Fm′ and was calculated by measuring the above same parameters (F0′ and Fm′) on light-adapted leaves. In light-adapted state, the F0′ level was estimated using the approximation of Oxborough and Baker (1997): . The actual quantum yield of PSII photochemistry for the light-adapted state (ΦPSII) could be calculated by the formula: (Genty et al., 1989). The coefficient of the photochemical quenching (qP), which was used for the estimation of the fraction of open PSII centers, was calculated as: (Bilger and Schreiber, 1987). The quantum yields of PSII photochemical energy dissipation (ΦPSII), non-regulated (ΦNO), and regulated (ΦNPQ) thermal energy dissipation for the light-adapted state could be used to reflect the utilization of photons which are absorbed by the PSII antennae (Lazár, 2015). It has been proved that ΦPSII + ΦNPQ + ΦNO = 1 (Hendrickson et al., 2004; Kramer et al., 2004; Lazár, 2015). ΦNOin PSII was calculated by the equation and ΦNPQ was calculated by , separately. At last, it should be noted that all the chlorophyll fluorescence parameters were calculated by the Imaging Win v2.32 software.
Statistical Analysis
All statistical analysis was performed using SPSS 18.0 (SPSS, Chicago, Illinois, USA). The mean with standard deviation (±SD) of each treatment was shown. The parameters were analyzed by One-/Two-way analysis of variance (ANOVA) followed by Duncan's multiple range test (Duncan, 1955). The graphing were performed using Origin 9.0 software (Origin Lab, USA).
Results and Discussion
In this study, fluorescence imaging technique was used to provide real-time information of photosynthetic performance of S. bungeana under different heat and water conditions. The change of images revealed the spatial variation of photosynthetic efficiency in the leaves of S. bungeana under different climate environments.
Chlorophyll Fluorescence Parameters in Temperature Warming and Precipitation Change Leaves
Different chlorophyll fluorescence parameters were measured for the leaves of S. bungeana to determine the impact of high temperature and precipitation change on the photosynthesis. Maximal quantum yield of PSII photochemistry for the dark-adapted state (Fv/Fm) has been widely used as an indicator of environmental stress. It can reveal the potential electron transport of maximal PSII quantum yield in the dark-adapted state. The imaging of qP and Fv′/Fm′ facilitates the evaluation of their variations (Oxborough and Baker, 1997). The fraction of the open PSII can be quantified by the parameter qP (Lazár, 2015). The light-induced non-photochemical quenching is a process that regulates energy conversion in PSII to protect plants from photoinhibition. It represents the plant's ability to dissipate excess light energy that cannot be utilized in CO2 assimilation (Müller et al., 2001). Our results showed that under T0 condition, Fv/Fm in water-deficient (W−30) plants significantly decreased by 16.7% compared with the normally-watered (W0) plants. There is no significant difference between the over-watered (W+30) plants and W0 plants. Moreover, there is a great change in Fm but not F0 under W−30 condition, suggesting that the decrease in Fv/Fm was due to the decrease in Fm. Except for Fv/Fm, there were no significant changes in the other chlorophyll fluorescence parameters such as Fv′/Fm′ and qP in both W−30 and W+30 plants. The results indicate that (1) excess precipitation had no effect on S. bungeana at room temperature; (2) S. bungeana suffered from water deficit (decrease in Fv/Fm), and water stress inhibited plant's ability in thermal energy dissipation (Zivcak et al., 2014). This can be explained by the fact that an extreme decrease in trans-thylakoid pH gradient was not generated owing to cooperative consumption of light energy by CO2 fixation and photorespiration (Müller et al., 2001).
At the T6 condition, high temperature offset the negative effect of water deficit on Fv/Fm, and enhanced the positive effect of excess precipitation on Fv/Fm, Fv′/Fm′ and qP, leading to the increase in value. This indicates that the temperature higher by 6°C will be beneficial to the photosynthetic performance of S. bungeana. However, in the study by Xu and Zhou (2006), the combination of severe water stress and high temperature exhibited adverse effects on the PSII function of Leymus chinensis, which is similar to Petsas and Grammatikopoulos (2009)'s conclusion that PSII function of Phlomis fruticosa was progressively suppressed under long-term water deficit. This obvious difference may be explained by that S. bungeana can well adapt to stress environment for its well-developed root system (Cheng et al., 2012).
Furthermore, in the leaves of plants under optimum temperature and water condition, the mean value of Fv/Fm was 0.678 (Table 1), which was lower than the typical value of 0.83 for non-photoinhibited leaves (Björkman and Demmig, 1987). There are two possible reasons to explain the difference. One is the usage of a different intensity of illumination during plants growing (Brestic et al., 2014) and the timing used for day/night regime was also different when compared to natural conditions. The other one is S. bungeana grown in the plastic pots with a small size under weak illumination may limit S. bungeana's normal growth (Xu and Zhou, 2006). Therefore, the use of pots inside the greenhouse still requires further investigation.
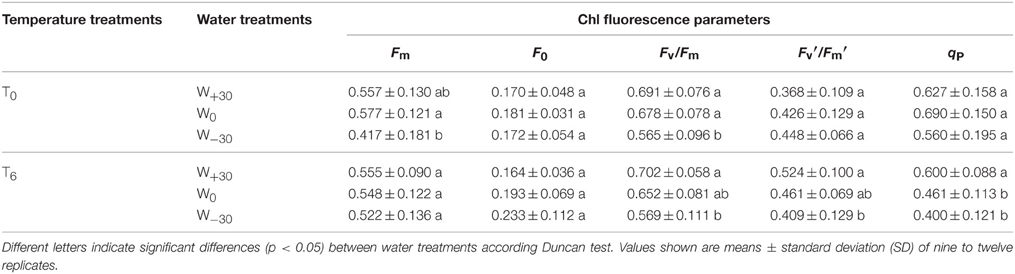
Table 1. Effects of precipitation treatments on maximum and minimum fluorescence yield in dark (Fm and F0, respectively), maximal quantum yield of PSII photochemistry for the dark-adapted state (Fv/Fm), coefficient of the photochemical quenching (qP) and maximal quantum yield of PSII photochemistry for the light-adapted state (Fv′/Fm′) of Stipa bungeana leaf under ambient temperature (T0) and high temperature (T6) conditions.
Utilization of Excess Excitation Energy Under High Temperature and Abnormal Water Conditions
Fv/Fm is known to be a sensitive indicator of plant photosynthetic performance (Björkman and Demmig, 1987). It reflects the maximum efficiency of photosynthetic apparatus converting the absorbed light energy into chemical energy, and has been widely used for the detection of photoinhibition (Dickmann et al., 1992; Herppich and Peckmann, 2000). The plants under stress have a lower value of Fv/Fm than those under normal environment (Papageorgiou and Govindjee, 2004; Tuba et al., 2010; Shaw et al., 2014). Calatayud et al. (2013) proposed several reasons for why the Fv/Fm ratio is preferable for the research of environmental stress. Firstly, it can be measured rapidly for the dark-adapted samples. Secondly, it is very useful for quick screening of stress-suffered plants in large quantities. Lastly, unlike the other parameters such as ΦPSII or qP, it does not need an extended period of illumination as a single saturation pulse is enough. However, the decrease of Fv/Fm can only reflect the degree of environmental stress, while the utilization of excess excitation energy is still unknown. To solve the problem, three fluorescence parameters which divides the allocation of absorbed light energy into three fractions: (1) utilized by PSII photochemistry (ΦPSII); (2) thermally dissipated via ΔpH and xanthophyll-dependent energy quenching (ΦNPQ); (3) non-regulated energy dissipation (ΦNO) (Demmig-Adams et al., 1996; Lazár, 2015). Among the latter three fluorescence parameters, ΦPSII reflects light-induced protective mechanism while ΦNO reflects a basal quenching which is not regulated by light. They shed a light on the study of plant's capacity to cope with excess excitation energy, and have been widely used to determine QA redox state and excitation energy fluxes in order to gain a better understanding of the stress response mechanisms (Calatayud et al., 2006; Osório et al., 2011).
As seen in Figure 1, the precipitation change had no significant effect (p > 0.05) on ΦPSII, ΦNPQ, and ΦNO at T0 condition, though ΦPSII decreased by 20.8 and 16.7% under W+30 and W−30 conditions and ΦNO increased by 21.0 and 23.9%, respectively. At the T6 condition, high temperature slightly decreased the value of ΦPSII at W0 and W−30 conditions. This suggests that heat dissipation of the excess light energy was activated to protect the photosynthetic apparatus from photoinhibitory damage (Ort and Baker, 2002; Omasa and Takayama, 2003). Whereas excess precipitation under T6 condition significantly increased ΦPSII, indicating that the increased precipitation can enhance the protective mechanism of PSII. In addition, ΦNPQ showed no obvious change under all environment conditions and the change of ΦNO was opposite to ΦPSII. This means that there was much overlap between ΦPSII and ΦNO, indicating that energy dissipation by non-regulated quenching mechanisms tends to dominate the yield of PSII photochemistry under drought and heat stress, with the xanthophyll cycle-mediated thermal dissipation playing possibly a less important role. Similar results were also reported by Osório et al. (2011).
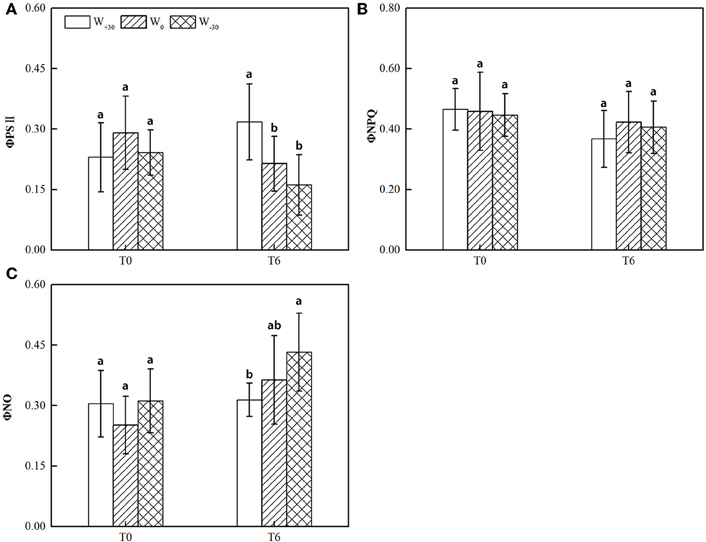
Figure 1. (A) Actual quantum yield of PSII photochemistry for the light-adapted state (ΦPSII), (B) quantum yield of regulated energy dissipation of PSII for the light-adapted state (ΦNPQ), (C) quantum yield of non-regulated energy dissipation of PSII for the light-adapted state (ΦNO) in the leaves of S. bungeana under two temperature treatments and three water treatments. Different letters indicate significant difference (p < 0.05) between water treatments in Duncan test. The values are expressed as mean ± standard deviation (SD), which was calculated with nine to twelve replicates.
Spatial Heterogeneity of Chlorophyll Fluorescence Parameters under Various Temperature and Water Conditions
In Table 2, the value of Chl a fluorescence was obtained from three leaves in each treatment. CFI reveals spatial changes in three areas of interest (AOIs) of the same leaves of S. bungeana, i.e., the bottom position of leaf, middle position of leaf and upper position of leaf as shown in Figure 2. For each AOI, the values of fluorescence parameter of all pixels within this area were averaged. In Figure 2, the images of a single leaf are used to show the heterogeneous distribution of light utilization (changes in ΦPSII, ΦNPQ, and ΦNO) and photosynthetic activity (change in Fv/Fm) over the surface of the whole leaf. The observation of changed image color is an intuitive process. Pixel-value images of Fv/Fm, ΦPSII, ΦNPQ, and ΦNO were displayed with the help of a false color code, ranging from black (0.000) to pink (ending 1.000).
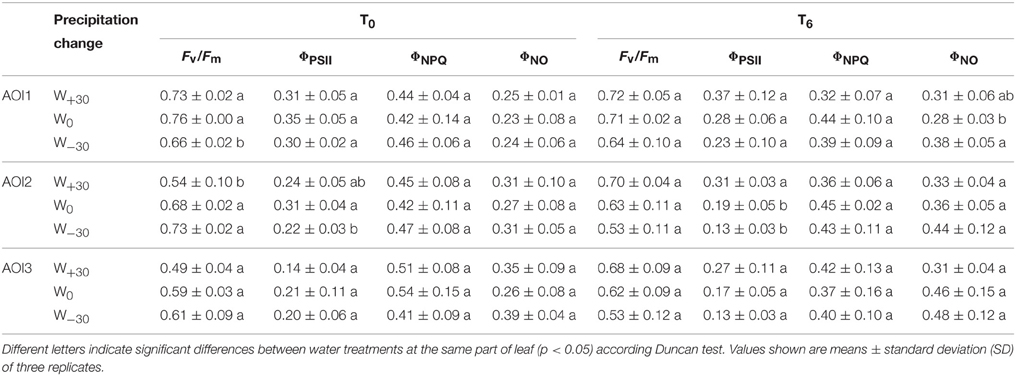
Table 2. Effect of high temperature and precipitation change on maximal quantum yield of PSII photochemistry for the dark-adapted state (Fv/Fm), actual quantum yield of PSII photochemistry for the light-adapted state (ΦPSII), quantum yield of regulated energy dissipation of PSII for the light-adapted state (ΦNPQ), and quantum yield of non-regulated energy dissipation of PSII for the light-adapted state (ΦNO) of Stipa bungeana in different AOIs.
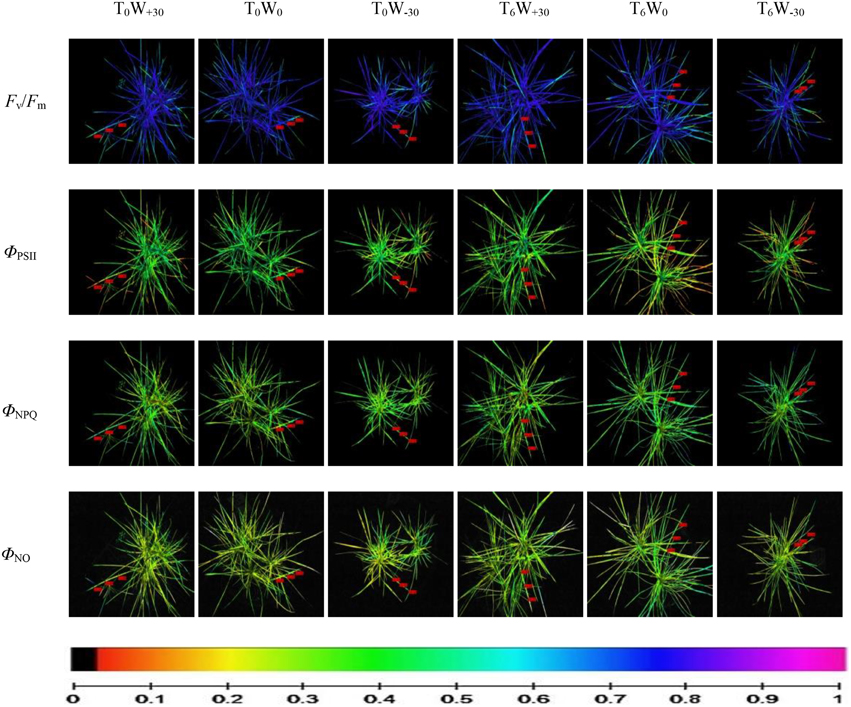
Figure 2. Use of chlorophyll fluorescence imaging of whole plant Stipa bungeana under different temperature and precipitation conditions. This figure illustrates several images of the same leaf of Stipa bungeana showing the spatial variation in the parameters ΦPSII, ΦNPQ, and ΦNO at steady state with actinic illumination of 336 μmol photons m−2·s−1, and in the parameter Fv/Fm after dark adaptation. The color scale showed at the bottom of the figure stands for values from 0 (black) to 1 (pink) based on Imaging Win v2.32 software. The three little red boxes in each image display the mean values of the selected fluorescence parameters within the AOI of one leaf.
According to Table 2, it was found that CFI exhibited the spatial changes in different AOIs of the leaf of S. bungeana. Three different AOI were considered for each leaf. Each datum in the table is the mean value of the corresponding AOI from all leaves. At T0 condition, excess precipitation did not alter the Fv/Fm in AOI1, but reduced the Fv/Fm by 13.0 and 9.2% in AOI2 and AOI3, respectively. Water deficit significantly decreased the Fv/Fm by 15.0% in AOI1, but there was no significant change in AOI2 and AOI3. At T6 condition, excess precipitation increased the Fv/Fm in AOI1, AOI2, and AOI3 by 2.8, 12.6, and 9.0%, respectively. This means that a 6°C higher temperature is beneficial for Fv/Fm under abundant water condition. In contrast, water deficit decreased the Fv/Fm in AOI1, AOI2, and AOI3 by 9.2, 14.9, and 16.8%, respectively. This suggests a reduction in light energy utilization by chloroplasts in the photosynthesis. According to the changes of Fv/Fm and the results of One-way ANOVA in Table 2, it can be concluded as follows. The middle position of leaf (AOI2) is most sensitive to excess precipitation under both T0 and T6 condition, while the bottom position (AOI1) and upper position (AOI3) are most sensitive to water deficit under both temperature conditions.
The contribution of different pathways to energy partitioning in PSII complexes is shown in Table 2. In AOI1 and AOI3, the actual quantum yield of PSII photochemistry for the light-adapted state (ΦPSII) which can indirectly reflect linear electron transport was not affected by precipitation change at both T0 and T6 treatment. This confirms that photoinhibition is not induced under these conditions. In AOI2, high temperature (T6) improved the effect of W+30 on Fv/Fm by 63.6%. This change of ΦPSII resulted from changes in the total non-photochemical quenching capacity (ΦNPQ+ ΦNO). The quantum yield of regulated energy dissipation (ΦNPQ) was quite similar in all AOIs under different environment conditions, indicating that no excess light energy was produced by precipitation change and high temperature. The values of quantum yield of non-regulated energy dissipation (ΦNO) were low and similar in all AOIs at T0. This means that there were sufficient photochemical conversion and protective regulatory mechanisms in the whole leaf. At T6 condition, water deficit increased ΦNO in whole leaf, indicating that high temperature exacerbated the negative effect of water deficit on energy dissipation. Both photochemical energy conversion and protective regulatory mechanism were not enough.
The Two-way ANOVA (Table 3) indicated that, Fv/Fm was significantly influenced by precipitation change at T0 condition and varied greatly at different AOIs (p < 0.01), exhibiting significant interaction of AOI and precipitation change (p < 0.01). The other chlorophyll fluorescence parameters such as ΦPSII and ΦNO exhibited significant difference across AOIs, but were not affected by precipitation change. Under condition, only ΦPSII was significantly affected by both AOI and precipitation change, but the interaction was not significant (p>0.05). To conclude, Fv/Fm is most sensitive to precipitation change at T0 condition, while ΦPSII is the most sensitive indicator at T6 condition. However, in the study by Lazár et al. (2006), even if there are no changes in the mean value of a fluorescence parameter, there can be the changes in shapes of statistical distributions of fluorescence parameter which is an early indication of a plant stress. Base on this, we should not only find the most sensitive parameter (Kalaji et al., 2014) and the most sensitive species (Swoczyna et al., 2010) but also find the best (statistical) method for detection of the stresses is more important. The use of fluorescence imaging and the detection of photosynthetic performance of Stipa bungeana response to climatic change still requires further investigation.
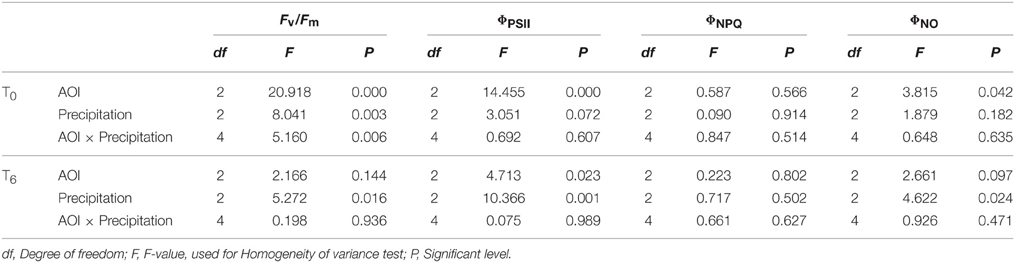
Table 3. Multiple range test among effects of areas of interest (AOI) and watering treatments on Stipa bungeana leaf Chl fluorescence parameters under ambient temperature (T0) and high temperature (T6) conditions based on the Two-way ANOVA.
Conclusion
Chlorophyll fluorescence imaging provided detailed intuitive information on the spatial heterogeneity of chlorophyll fluorescence parameters of S. bungeana and facilitated the investigation of plant photosynthetic performance under various temperature and water conditions. Our results showed that S. bungeana has strong ability in protecting photosynthetic apparatus against the photoinhibitory damage from drought, and a 6°C higher temperature could offset the negative effect of water deficit to a certain extent. On the other hand, excess precipitation had no significant effect on PSII at room temperature. But high temperature had a positive effect on PSII and significantly enhanced the photosynthesis of S. bungeana. We also found that it is energy dissipation by non-regulated quenching mechanisms rather than the xanthophyll cycle-mediated thermal dissipation that plays an important role in dominating the yield of PSII photochemistry under climate change. This study also found that Fv/Fm measured in AOIs was the most sensitive indicator to precipitation change under room temperature, while ΦPSII is more sensitive to precipitation change at higher temperature.
Author Contributions
GZ and YW conceived the experiment, YW, XL, and ZX conducted the experiment, XS analyzed the results and wrote the manuscript. All authors reviewed the manuscript. No conflict of interest exits in the submission of this manuscript, and the manuscript is approved by all authors for publication. We would like to declare that the work described was original research that has not been published previously, and not under consideration for publication elsewhere, in whole or in part.
Funding
This work was supported by the national Basic research Program of China [grant number 2010CB951301] and Chinese Academy of Sciences “Strategic Priority Research Program-Climate Change: Carbon Budget and Relevant Issues” [grant number XDA-05050408].
Conflict of Interest Statement
The authors declare that the research was conducted in the absence of any commercial or financial relationships that could be construed as a potential conflict of interest.
Acknowledgments
We thank Jun Chen, Liping Tan, Tao Liu, Bingrui Jia, Yanling Jiang, Feng Zhang, Jian Song, Zhixiang Yang, Hui Wang, Yaohui Shi, Yanhui Hou for their help during the experiment.
Abbreviations
AOI, areas of interest; Chl, chlorophyll; CFI, chlorophyll fluorescence imaging; PSII, photosystem II; Fv/Fm, maximal quantum yield of PSII photochemistry for the dark-adapted state; qP, coefficient of the photochemical quenching; ΦPSII, actual quantum yield of PSII photochemistry for the light-adapted state; ΦNPQ, quantum yield of regulated energy dissipation of PSII for the light-adapted state; ΦNO, quantum yield of non-regulated energy dissipation of PSII for the light-adapted state; Fv′/Fm′, maximal quantum yield of PSII photochemistry for the light-adapted state.
References
Albert, K. R., Ro-Poulsen, H., Mikkelsen, T. N., Michelsen, A., van der Linden, L., and Beier, C. (2011). Interactive effects of elevated CO2, warming, and drought on photosynthesis of Deschampsia flexuosa in a temperate heath ecosystem. J. Exp. Bot. 62, 4253–4266. doi: 10.1093/jxb/err133
Baker, N. R., and Rosenqvist, E. (2004). Applications of chlorophyll fluorescence can improve crop production strategies: an examination of future possibilities. J. Exp. Bot. 55, 1607–1621. doi: 10.1093/jxb/erh196
Bauweraerts, I., Wertin, T. M., Ameye, M., McGuire, M. A., Teskey, R. O., and Steppe, K. (2013). The effect of heat waves, elevated CO2 and low soil water availability on northern red oak (Quercus rubra L.) seedlings. Glob. Chang. Biol. 19, 517–528. doi: 10.1111/gcb.12044
Berry, J., and Bjorkman, O. (1980). Photosynthetic response and adaptation to temperature in higher plants. Annu. Rev. Plant Physiol. 31, 491–543. doi: 10.1146/annurev.pp.31.060180.002423
Bilger, W., and Schreiber, U. (1987). “Excitation energy and electron transfer,” in Photosynthesis, eds Govindjee, J. Barber, W. A. Cramer, J. H. C. Goedheer, J. Lavorel, R. Marcelle, and B. Zilinskas (Dordrecht: Springer), 157–162.
Björkman, O., and Demmig, B. (1987). Photon yield of O2 evolution and chlorophyll fluorescence characteristics at 77 K among vascular plants of diverse origins. Planta 170, 489–504. doi: 10.1007/BF00402983
Boyer, J. S. (1982). Plant productivity and environment. Science 218, 443–448. doi: 10.1126/science.218.4571.443
Brestic, M., Zivcak, M., Olsovska, K., Shao, H. B., Kalaji, H. M., and Allakhverdiev, S. I. (2014). Reduced glutamine synthetase activity plays a role in control of photosynthetic responses to high light in barley leaves. Plant Physiol. Biochem. 81, 74–83. doi: 10.1016/j.plaphy.2014.01.002
Calatayud, A., Roca, D., and Martínez, P. F. (2006). Spatial-temporal variations in rose leaves under water stress conditions studied by chlorophyll fluorescence imaging. Plant Physiol. Biochem. 44, 564–573. doi: 10.1016/j.plaphy.2006.09.015
Calatayud, Á., San Bautista, A., Pascual, B., Maroto, J. V., and López-Galarza, S. (2013). Use of chlorophyll fluorescence imaging as diagnostic technique to predict compatibility in melon graft. Sci. Hortic. 149, 13–18. doi: 10.1016/j.scienta.2012.04.019
Chaves, M. M., Pereira, J. S., Maroco, J., Rodrigues, M. L., Ricardo, C. P., Osorio, M. L., et al. (2002). How plants cope with water stress in the field? Photosynthesis and growth. Ann. Bot. 89, 907–916. doi: 10.1093/aob/mcf105
Cheng, J., Cheng, J., Hu, T., Shao, H., and Zhang, J. (2012). Dynamic changes of Stipa bungeana steppe species diversity as better indicators for soil quality and sustainable utilization mode in yunwu mountain nature reserve, Ningxia, China. CLEAN Soil Air Water 40, 127–133. doi: 10.1002/clen.201000438
Cornic, G. (2000). Drought stress inhibits photosynthesis by decreasing stomatal aperture – not by affecting ATP synthesis. Trends Plant Sci. 5, 187–188. doi: 10.1016/S1360-1385(00)01625-3
Demmig-Adams, B., et al. (1996). Using chlorophyll fluorescence to assess the fraction of absorbed light allocated to thermal dissipation of excess excitation. Physiol. Plant. 98, 253–264. doi: 10.1034/j.1399-3054.1996.980206.x
Dickmann, D. I., Liu, Z., Nguyen, P. V., and Pregitzer, K. S. (1992). Photosynthesis, water relations, and growth of two hybrid populus genotypes during a severe drought. Can. J. Forest Res. 22, 1094–1106. doi: 10.1139/x92-145
Duncan, D. B. (1955). Multiple range and multiple F tests. Biometrics 11, 1–42. doi: 10.2307/3001478
Ehlert, B., and Hincha, D. (2008). Chlorophyll fluorescence imaging accurately quantifies freezing damage and cold acclimation responses in Arabidopsis leaves. Plant Methods 4, 1–7. doi: 10.1186/1746-4811-4-12
Genty, B., Briantais, J.-M., and Baker, N. R. (1989). The relationship between the quantum yield of photosynthetic electron transport and quenching of chlorophyll fluorescence. Biochim. Biophys. Acta 990, 87–92. doi: 10.1016/S0304-4165(89)80016-9
Giardi, M. T., Cona, A., Geiken, B., Kučera, T., Masojídek, J., and Mattoo, A. K. (1996). Long-term drought stress induces structural and functional reorganization of photosystem II. Planta 199, 118–125. doi: 10.1007/BF00196888
Gorbe, E., and Calatayud, A. (2012). Applications of chlorophyll fluorescence imaging technique in horticultural research: a review. Sci. Hortic. 138, 24–35. doi: 10.1016/j.scienta.2012.02.002
Havaux, M. (1992). Stress Tolerance of Photosystem II in vivo: antagonistic effects of water, heat, and photoinhibition stresses. Plant Physiol. 100, 424–432. doi: 10.2307/4274644
Hendrickson, L., Furbank, R. T., and Chow, W. S. (2004). A simple alternative approach to assessing the fate of absorbed light energy using chlorophyll fluorescence. Photosynth. Res. 82, 73–81. doi: 10.1023/B:PRES.0000040446.87305.f4
Herppich, W. B., and Peckmann, K. (2000). Influence of drought on mitochondrial activity, photosynthesis, nocturnal acid accumulation and water relations in the CAM plants prenia sladeniana(ME-type) and crassula lycopodioides(PEPCK-type). Ann. Bot. 86, 611–620. doi: 10.1006/anbo.2000.1229
Humplík, J., Lazár, D., Husièková, A., and Spíchal, L. (2015). Automated phenotyping of plant shoots using imaging methods for analysis of plant stress responses a review. Plant Methods 11, 1–10. doi: 10.1186/s13007-015-0072-8
IPCC (2013). Climatic Change. The Physical Science Basis. Stockholm: Intergovernmental Panel on Climate Change.
Ivanov, D. A., and Bernards, M. A. (2015). Chlorophyll fluorescence imaging as a tool to monitor the progress of a root pathogen in a perennial plant. Planta 243, 263–279. doi: 10.1007/s00425-015-2427-9
Kalaji, H. M., Oukarroum, A., Alexandrov, V., Kouzmanova, M., Brestic, M., Zivcak, M., et al. (2014). Identification of nutrient deficiency in maize and tomato plants by in vivo chlorophyll a fluorescence measurements. Plant Physiol. Biochem. 81, 16–25. doi: 10.1016/j.plaphy.2014.03.029
Kramer, D., Johnson, G., Kiirats, O., and Edwards, G. (2004). New fluorescence parameters for the determination of QA redox state and excitation energy fluxes. Photosynth. Res. 79, 209–218. doi: 10.1023/B:PRES.0000015391.99477.0d
Lazár, D. (2015). Parameters of photosynthetic energy partitioning. J. Plant Physiol. 175, 131–147. doi: 10.1016/j.jplph.2014.10.021
Lazár, D., Sušila, P., and Nauš, J. (2006). Early detection of plant stress from changes in distributions of chlorophyll a fluorescence parameters measured with fluorescence imaging. J. Fluoresc. 16, 173–176. doi: 10.1007/s10895-005-0032-1
Lichtenthaler, H. K., and Miehé, J. A. (1997). Fluorescence imaging as a diagnostic tool for plant stress. Trends Plant Sci. 2, 316–320. doi: 10.1016/S1360-1385(97)89954-2
Machado, S., and Paulsen, G. (2001). Combined effects of drought and high temperature on water relations of wheat and sorghum. Plant Soil 233, 179–187. doi: 10.1023/A:1010346601643
Martínez-Carrasco, R., Sánchez-Rodriguez, J., and Pérez, P. (2002). Changes in chlorophyll fluorescence during the course of photoperiod and in response to drought in casuarina equisetifolia forst and Forst. Photosynthetica 40, 363–368. doi: 10.1023/A:1022618823538
Mielke, M. S., de Almeida, A.-A. F., Gomesa, F. P., Aguilarb, M. A. G., and Mangabeira, P. A. O. (2003). Leaf gas exchange, chlorophyll fluorescence and growth responses of Genipa americana seedlings to soil flooding. Environ. Exp. Bot. 50, 221–231. doi: 10.1016/S0098-8472(03)00036-4
Müller, P., Li, X.-P., and Niyogi, K. K. (2001). Non-Photochemical quenching. A response to excess light energy. Plant Physiol. 125, 1558–1566. doi: 10.2307/4279788
Ogaya, R., Peñuelas, J., Asensio, D., and Llusià, J. (2011). Chlorophyll fluorescence responses to temperature and water availability in two co-dominant Mediterranean shrub and tree species in a long-term field experiment simulating climate change. Environ. Exp. Bot. 73, 89–93. doi: 10.1016/j.envexpbot.2011.08.004
Omasa, K. (1990). “Physical methods,” in Plant Sciences, Vol. 11, Modern Methods of Plant Analysis, eds H.-F. Linskens and J. F. Jackson (Berlin; Heidelberg: Springer), Ch. 8, 203–243.
Omasa, K., and Takayama, K. (2003). Simultaneous measurement of stomatal conductance, non-photochemical quenching, and photochemical yield of photosystem ii in intact leaves by thermal and chlorophyll fluorescence imaging. Plant Cell Physiol. 44, 1290–1300. doi: 10.1093/pcp/pcg165
Omasa, K., Shimazaki, K.-I., Aiga, I., Larcher, W., and Onoe, M. (1987). Image analysis of chlorophyll fluorescence transients for diagnosing the photosynthetic system of attached leaves. Plant Physiol. 84, 748–752.
Ort, D. R., and Baker, N. R. (2002). A photoprotective role for O2 as an alternative electron sink in photosynthesis? Curr. Opin. Plant Biol. 5, 193–198. doi: 10.1016/S1369-5266(02)00259-5
Osório, M. L., Osório, J., Vieira, A. C., Gonçalves, S., and Romano, A. (2011). Influence of enhanced temperature on photosynthesis, photooxidative damage, and antioxidant strategies in Ceratonia siliqua L. seedlings subjected to water deficit and rewatering. Photosynthetica 49, 3–12. doi: 10.1007/s11099-011-0001-7
Oxborough, K., and Baker, N. (1997). Resolving chlorophyll a fluorescence images of photosynthetic efficiency into photochemical and non-photochemical components – calculation of qP and Fv-/Fm-; without measuring Fo. Photosyn. Res. 54, 135–142. doi: 10.1023/A:1005936823310
Papageorgiou, G., Govindjee (eds.). (2004). Chlorophyll Fluorescence: A Signature of Photosynthesis. Dordrecht: Kluwer Academic Publishers.
Petsas, A., and Grammatikopoulos, G. (2009). Drought resistance and recovery of photosystem II activity in a Mediterranean semi-deciduous shrub at the seedling stage. Photosynthetica 47, 284–292. doi: 10.1007/s11099-009-0044-1
Shaw, A. K., Ghosh, S., Kalaji, H. M., Bosa, K., Brestic, M., Zivcak, M., et al. (2014). Nano-CuO stress induced modulation of antioxidative defense and photosynthetic performance of Syrian barley (Hordeum vulgare L.). Environ. Exp. Bot. 102, 37–47. doi: 10.1016/j.envexpbot.2014.02.016
Swoczyna, T., Kalaji, H. M., Pietkiewicz, S., Borowski, J., and Zaraś-Januszkiewicz, E. (2010). Photosynthetic apparatus efficiency of eight tree taxa as an indicator of their tolerance to urban environments. Dendrobiology 63, 65–75.
Thomey, M. L., Collins, S. L., Vargas, R., Johnson, J. E., Brown, R. F., Natvig, D. O., et al. (2011). Effect of precipitation variability on net primary production and soil respiration in a Chihuahuan Desert grassland. Glob. Chang. Biol. 17, 1505–1515. doi: 10.1111/j.1365-2486.2010.02363.x
Tuba, T., Saxena, D. K., Srivastava, K., Singh, S., Czobel, S., and Kalaji, H. M. (2010). Chlorophyll a fluorescence measurements for validating the tolerant bryophytes for heavy metal (Pb) biomapping. Res. Commun. 98, 1501–1508.
Wahid, A., Gelani, S., Ashraf, M., and Foolad, M. R. (2007). Heat tolerance in plants: an overview. Environ. Exp. Bot. 61, 199–223. doi: 10.1016/j.envexpbot.2007.05.011
Xu, Z.-Z., Zhou, G.-S., and Li, H. (2004). Responses of chlorophyll fluorescence and nitrogen level of Leymus chinensis Seedling to changes of soil moisture and temperature. J. Environ. Sci. 16, 666–669. doi: 10.3321/j.issn:1001-0742.2004.04.029
Xu, Z. Z., and Zhou, G. S. (2006). Combined effects of water stress and high temperature on photosynthesis, nitrogen metabolism and lipid peroxidation of a perennial grass Leymus chinensis. Planta 224, 1080–1090. doi: 10.1007/s00425-006-0281-5
Xu, Z. Z., Zhou, G. S., and Shimizu, H. (2009). Effects of soil drought with nocturnal warming on leaf stomatal traits and mesophyll cell ultrastructure of a perennial grass. Crop Sci. 49, 1843–1851. doi: 10.2135/cropsci2008.12.0725
Keywords: Stipa bungeana, chlorophyll fluorescence imaging, photosynthetic efficiency, energy partitioning, high temperature, precipitation change
Citation: Song X, Zhou G, Xu Z, Lv X and Wang Y (2016) Detection of Photosynthetic Performance of Stipa bungeana Seedlings under Climatic Change using Chlorophyll Fluorescence Imaging. Front. Plant Sci. 6:1254. doi: 10.3389/fpls.2015.01254
Received: 17 August 2015; Accepted: 22 December 2015;
Published: 12 January 2016.
Edited by:
Boris Rewald, University of Natural Resources and Life Sciences, Vienna, AustriaReviewed by:
Hazem M. Kalaji, Warsaw University of Life Sciences, PolandDusan Lazar, Palacky University, Czech Republic
Copyright © 2016 Song, Zhou, Xu, Lv and Wang. This is an open-access article distributed under the terms of the Creative Commons Attribution License (CC BY). The use, distribution or reproduction in other forums is permitted, provided the original author(s) or licensor are credited and that the original publication in this journal is cited, in accordance with accepted academic practice. No use, distribution or reproduction is permitted which does not comply with these terms.
*Correspondence: Guangsheng Zhou, gszhou@ibcas.ac.cn;
Yuhui Wang, yuhuiwang@ibcas.ac.cn