Corrigendum: Research Progress on the use of Plant Allelopathy in Agriculture and the Physiological and Ecological Mechanisms of Allelopathy
- College of Horticulture, Northwest A&F University, Yangling, China
Allelopathy is a common biological phenomenon by which one organism produces biochemicals that influence the growth, survival, development, and reproduction of other organisms. These biochemicals are known as allelochemicals and have beneficial or detrimental effects on target organisms. Plant allelopathy is one of the modes of interaction between receptor and donor plants and may exert either positive effects (e.g., for agricultural management, such as weed control, crop protection, or crop re-establishment) or negative effects (e.g., autotoxicity, soil sickness, or biological invasion). To ensure sustainable agricultural development, it is important to exploit cultivation systems that take advantage of the stimulatory/inhibitory influence of allelopathic plants to regulate plant growth and development and to avoid allelopathic autotoxicity. Allelochemicals can potentially be used as growth regulators, herbicides, insecticides, and antimicrobial crop protection products. Here, we reviewed the plant allelopathy management practices applied in agriculture and the underlying allelopathic mechanisms described in the literature. The major points addressed are as follows: (1) Description of management practices related to allelopathy and allelochemicals in agriculture. (2) Discussion of the progress regarding the mode of action of allelochemicals and the physiological mechanisms of allelopathy, consisting of the influence on cell micro- and ultra-structure, cell division and elongation, membrane permeability, oxidative and antioxidant systems, growth regulation systems, respiration, enzyme synthesis and metabolism, photosynthesis, mineral ion uptake, protein and nucleic acid synthesis. (3) Evaluation of the effect of ecological mechanisms exerted by allelopathy on microorganisms and the ecological environment. (4) Discussion of existing problems and proposal for future research directions in this field to provide a useful reference for future studies on plant allelopathy.
Introduction
Allelopathy is a sub-discipline of chemical ecology that is concerned with the effects of chemicals produced by plants or microorganisms on the growth, development and distribution of other plants and microorganisms in natural communities or agricultural systems (Einhellig, 1995). The study of allelopathy increased in the 1970s and has undergone rapid development since the mid-1990s, becoming a popular topic in botany, ecology, agronomy, soil science, horticulture, and other areas of inquiry in recent years. The allelopathic interaction can be one of the significant factors contributing to species distribution and abundance within plant communities and can be important in the success of invasive plants (Chou, 1999; Mallik, 2003; Field et al., 2006; Inderjit et al., 2006; Zheng et al., 2015), such as water hyacinth (Eichhornia crassipes Mart. Solms) (Jin et al., 2003; Gao and Li, 2004), spotted knapweed (Centaurea stoebe L. ssp. micranthos) (Broeckling and Vivanco, 2008) and garlic mustard (Alliaria petiolata M. Bieb) (Vaughn and Berhow, 1999). Allelopathy is also thought to be one of the indirect causes of continuous cropping obstacles in agriculture. As a result of the in-depth study of allelopathy, strategies for the management of agricultural production and ecological restoration involving the application of allelopathy and allelochemicals are improving. The main purposes of this review are to present conclusions regarding the application of allelopathy in agricultural production, to highlight the physiological and ecological mechanisms underlying plant allelopathy, to illustrate the effect of allelopathy on soil microorganisms and to discuss key points for further research.
Allelopathy and Allelochemicals
The definition of allelopathy was first used by Molish in 1937 to indicate all of the effects that directly and indirectly result from biochemical substances transferred from one plant to another (Molisch, 1937). Almost half a century later, the accepted targets of allelochemicals in the plant kingdom include algae, fungi and various microorganisms. The term was refined by Rice (1984) to define “any direct or indirect harmful or beneficial effect by one plant (including microorganisms) on another through production of chemical compounds that escape into the environment” (Rice, 1984). In 1996, the International Allelopathy Society broadened its definition of allelopathy to refer to any process involving secondary metabolites produced by plants, microorganisms, viruses and fungi that influence the growth and development of agricultural and biological systems. In addition, the allelopathic donor and receiver should include animals (Kong and Hu, 2001).
Allelochemicals, which are non-nutritive substances mainly produced as plant secondary metabolites or decomposition products of microbes, are the active media of allelopathy. Allelochemicals consist of various chemical families and are classified into the following 14 categories based on chemical similarity (Rice, 1974): water-soluble organic acids, straight-chain alcohols, aliphatic aldehydes, and ketones; simple unsaturated lactones; long-chain fatty acids and polyacetylenes; benzoquinone, anthraquinone and complex quinones; simple phenols, benzoic acid and its derivatives; cinnamic acid and its derivatives; coumarin; flavonoids; tannins; terpenoids and steroids; amino acids and peptides; alkaloids and cyanohydrins; sulfide and glucosinolates; and purines and nucleosides. Plant growth regulators, including salicylic acid, gibberellic acid and ethylene, are also considered to be allelochemicals. The rapid progress of analysis technology in recent years has made it possible to isolate and identify even minute amounts of allelochemicals and to perform sophisticated structural analyses of these molecules. The structures of some allelochemicals produced by plants are shown in Figure 1.
Management of Plant Allelopathy in Agriculture
Allelopathy is a natural ecological phenomenon. It has been known and used in agriculture since ancient times (Zeng, 2008, 2014). Allelochemicals can stimulate or inhibit plant germination and growth, and permit the development of crops with low phytotoxic residue amounts in water and soil, thus facilitating wastewater treatment and recycling (Macias et al., 2003; Zeng et al., 2008). They are a suitable substitute for synthetic herbicides because allelochemicals do not have residual or toxic effects, although the efficacy and specificity of many allelochemicals are limited (Bhadoria, 2011). The main purposes of research on allelopathy include the application of the observed allelopathic effects to agricultural production, reduction of the input of chemical pesticides and consequent environmental pollution, and provision of effective methods for the sustainable development of agricultural production and ecological systems (Macias et al., 2003; Li et al., 2010; Han et al., 2013; Jabran et al., 2015). The use of allelopathic crops in agriculture is currently being realized, e.g., as components of crop rotations, for intercropping, as cover crops or as green manure (Cheema and Khaliq, 2000; Singh et al., 2003; Cheema et al., 2004; Khanh et al., 2005; Reeves et al., 2005; Yildirim and Guvenc, 2005; Iqbal et al., 2007; Mahmood et al., 2013; Wortman et al., 2013; Farooq et al., 2014; Silva et al., 2014; Wezel et al., 2014; Haider et al., 2015). The applications of allelopathy in crop production in Pakistan are successful examples in recent years (Cheema et al., 2013). The suitable application of allelopathy toward the improvement of crop productivity and environmental protection through environmentally friendly control of weeds, insect pests, crop diseases, conservation of nitrogen in crop lands, and the synthesis of novel agrochemicals based on allelochemicals has attracted much attention from scientists engaged in allelopathic research.
Arrangement of Cropping Systems
Competition is one of the main modes of interaction between cultivated crops and their neighboring plants (Inderjit and Moral, 1997; Xiong et al., 2005; He et al., 2012b; An et al., 2013). Allelopathy is a chemical mechanism that provides plants with an advantage for competing for limited resources (Singh et al., 1999; He et al., 2012b; Gioria and Osborne, 2014). The ability of plants to suppress weeds is thus determined by crop allelopathy and competitiveness. Crop allelopathy can be effectively used to control weeds in the field, to alleviate allelopathic autotoxicity and reduce inhibitory influence among allelopathic crops (Iqbal et al., 2007; John et al., 2010; Farooq et al., 2013; Andrew et al., 2015), to improve the utilization rate of land and to increase the annual output of the soil by establishing reasonable crop rotation and intercropping systems. For example, Odeyemi et al. (2013) reported relative abundance and population suppression of plant parasitic nematodes under Chromolaena odorata (L.) (Asteraceae) fallow in a field study conducted over 2 years, and suggested that the use of bush fallow with C. odorata might become an integrated management practice in the management of nematode pests in crop production in south-western Nigeria. Intercropping is a common practice among farmers in developing countries for maximizing land resources and reducing the risks of single crop failure. Weed population density and biomass production can be markedly reduced using crop rotation and intercropping systems (Liebman and Dyck, 1993; Narwal, 2000; Nawaz et al., 2014; Jabran et al., 2015). Intercropping of sorghum (Sorghum bicolor L.), sesame (Sesamum indicum L.) and soybean (Glycine max L.) in a cotton (Gossypium hirsutum L.) field produced greater net benefits and a significant inhibition on purple nutsedge (Cyperus rotundus L.) in comparison with the cotton alone in a 2-year experiment (Iqbal et al., 2007). Recently, Wang et al. (2015) reported that eggplant/garlic relay intercropping is a beneficial cultivation practice to maintain stronger eggplant growth and higher yield. However, the allelopathy between different species may cause promontory or inhibitory effects. Farooq et al. (2014) reported that when grown in rotation with tobacco (Nicotiana tabacum L.), the stand establishment and growth of maize (Zea mays L.) were improved compared to mung bean (Vigna radiata L.), whereas mungbean stand establishment and growth were suppressed. Therefore, the allelopathic nature of crops must be considered in crop rotation, intercropping and stalk mulching (Xuan et al., 2005; Cheng et al., 2011; Cheng and Xu, 2013).
Straw Mulching
In conventional agriculture, weed control using herbicides is not only an expensive practice; it is also harmful to the environment. Allelopathic applications, such as straw mulching, provide sustainable weed management (Jabran et al., 2015), further reducing the negative impact of agriculture on the environment (Cheema and Khaliq, 2000; Cheema et al., 2004). Using allelopathic plants as ground cover species provides an environmental friendly option (Dhima et al., 2006; Moraes et al., 2009; Wang et al., 2013a). The allelochemicals from decomposed straw can suppress weed growth in farmlands, and reduce the incidence of pests and diseases. Moreover, straw mulch can improve the soil organic matter content and increase soil fertility. However, it may also have negative effects by increasing the C: N ratio of the soil. Research has shown that green wheat (Triticum aestivum L.) straw inhibits the growth of Ipomoea weeds in corn (Zea mays L.) and soybean fields, thereby reducing the need for herbicide application. Rye (Secale cereale L.) mulch significantly reduced the germination and growth of several problematic agronomic grass and broadleaf weeds (Figure 2; Schulz et al., 2013). The transformation reactions of rye allelochemicals, i.e., benzoxazinoids, in soil led primarily to the production of phenoxazinones, which can be degraded by several specialized fungi via the Fenton reaction. Because of their selectivity, specific activity, and presumably limited persistence in the soil, benzoxazinoids or rye residues are suitable means for weed control (Schulz et al., 2013). Furthermore, Tabaglio et al. (2008) found that the allelopathic inhibition effects on weeds differ between different cultivars of rye straw used for mulching. Xuan et al. (2005) concluded that the application of allelopathic plant materials at 1–2 tons ha–1 could reduce weed biomass by approximately 70%, and increase rice (Oryza sativa L.) yield by approximately 20% in paddy fields (1998–2003) compared with the respective controls. In the southeastern region of Brazil, coffee (Coffea arabica) fruit peels, which contain allelochemicals such as phenols, flavonoids and caffeine, are often used as an organic amendment in agricultural practice to control weeds (Silva et al., 2013). An et al. (2013) found that switchgrass (Panicum virgatum L.) plants and residues reduced the biomass and density of associated weeds, and their research provided weed management strategies in agroecosystems and important information for the introduction of switchgrass into new ecosystems. Water extracts of Conyza bonariensis (L.) Cronquist, Trianthema portulacastrum L., and Pulicaria undulata (L.) C. A. Mey. can be applied at a concentration of 10 g L–1 to manage the weed Brassica tournefortii Gouan by inhibiting germination and seedling growth (Abd El-Gawad, 2014). Moreover, some soybeans induce the germination of sunflower broomrape (Orobanche spp.), a noxious parasitic weed, which suggests that soybean has the potential to be used as a trap crop to reduce the seed bank of sunflower broomrape (Zhang et al., 2013b).
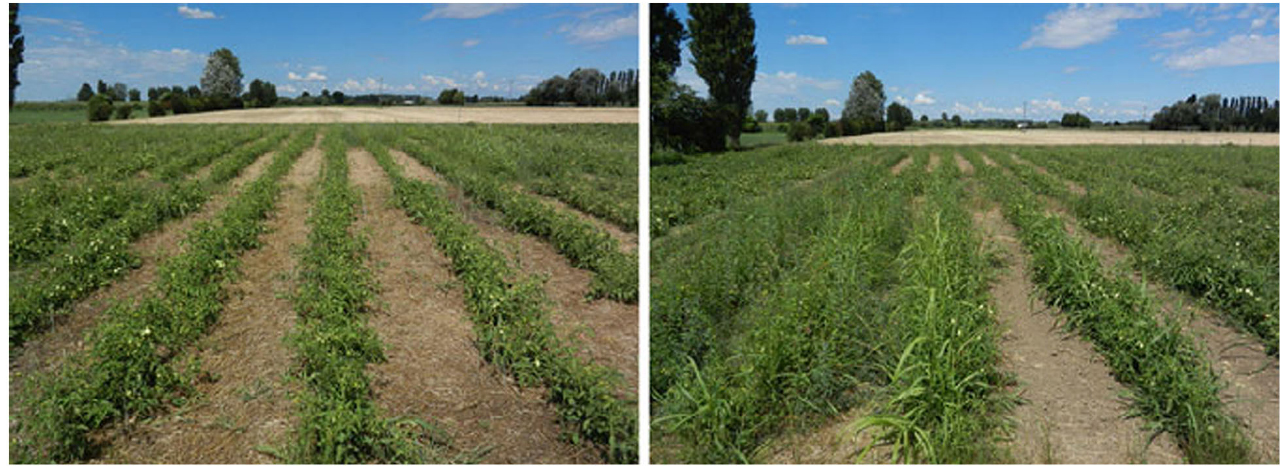
Figure 2. Field trial on rye mulch preceding a tomato crop in a biological farm (Schulz et al., 2013). Left, test plot with rye mulch left on the soil surface, showing the good weed suppression ability. Right, control plot without rye mulch, split into two treatments: left side, untreated sub-plot in which tomato plants are almost completely overgrown by weeds; right side, sub-plot with mechanical control by cultivation, in which tomato plants grow as well as those in the test plot.
Developing Environmentally Friendly Agrochemical and Microbial Pesticides
Allelochemicals with negative allelopathic effects are important components of plant defense mechanisms against weeds and herbivory. The technology that modifies allelochemicals for the production of environmentally friendly pesticides and plant growth regulators allows the effective management of agricultural production and confers few environmental problems in the soil due to the fairly high degradability of allelochemicals (Bhadoria, 2011; Ihsan et al., 2015). Uddin et al. (2014) revealed that sorgoleone, a hydrophobic compound found in Sorghum bicolor (L.) root exudates, was more effective in inhibiting weed growth after formulation as a wettable powder, while crop species were tolerant to it. Some microorganisms are capable of using sorgoleone as a carbon source. Sorgoleone can be mineralized via complete degradation to CO2 in soil, although the different chemical groups of the molecule were not mineralized equally (Gimsing et al., 2009). The strong weed-suppressive ability of formulated sorgoleone raised interest as an effective, natural, environmentally friendly approach for weed management. Plant growth-promoting rhizobacteria (PGPR) include a wide range of beneficial bacteria that confer positive effects on plants, such as eliciting induced systemic resistance (ISR), promoting plant growth and reducing susceptibility to diseases caused by plant pathogens (Kloepper et al., 1980, 2004). Allelopathic bacteria can achieve the same function in mixtures of bacteria that exhibit PGPR attributes and activity against allelopathic weeds, which reduces the inhibitory effect on susceptible plants caused by allelopathic weeds (Kremer, 2006; Mishra and Nautiyal, 2012). There are some organic herbicides or plant growth inhibitors that have been manufactured from allelopathic plant materials to inhibit weed growth in fields (Guillon, 2003; Ogata et al., 2008; Miyake, 2009). Ogata et al. (2008) manufactured a type of herbicide comprised of a mixture of components extracted from pine (Pinus L.), hinoki (Chamaecyparis obtusa Endl.), or Japanese cedar (Cryptomeria japonica D. Don) and bamboo (Bambusoideae; Poaceae) vinegar, which provided a practical method of utilizing plant allelopathy in paddy fields.
Reduction of Nitrogen Leaching and Environmental Pollution
Nitrogen leaching is a severe ecological problem due to water pollution. Mineralization of soil organic nitrogen, especially the nitrification of nitrogen fertilizer, is one of the main reasons for the enrichment of nitrogen in the soil. Biological nitrification inhibition (BNI) has gradually become the main target in investigating the effect of plants on soil nitrification. In recent years, studies have proven that nitrification-inhibiting substances (NIS) produced by plants are the first choice for soil nitrification management. For example, biological nitrification inhibition substances (BNIS) are allelochemicals that are able to inhibit soil nitrification. Wheat allelochemicals, such as ferulic acid, p-hydroxybenzoic acid and hydroxamic acid, can act on soil microbes to inhibit soil nitrification, reduce the emission of N2O, improve the utilization rate of nitrogen fertilizer and reduce pollution to the environment (Ma, 2005). Dietz et al. (2013) found that the allelopathic plantain (Plantago lanceolata L.) plant has inhibitory effects on soil nitrogen mineralization, suggesting that plantain could be utilized to reduce soil nitrogen leaching.
Breeding of Allelopathic Cultivars
Allelopathic cultivars, which have great potential to minimize the introduction of refractory chemicals and effectively control weeds in farmland ecosystems, represent the most promising application of allelopathy (Mahmoud and Croteau, 2002; Weston and Duke, 2003; Fragasso et al., 2013). Both conventional breeding methods and those developed using transgenic technology can be applied in the breeding of allelopathic cultivars. Successful cultivars must also combine a weed suppression ability with high yield potential, disease resistance, early maturity and quality traits (Gealy and Yan, 2012). Rondo, a rice cultivar that combines a high yield potential with rice blast resistance and weed suppression ability, has been grown in a commercial organic rice production operation in Texas and its weed-suppressive ability is superior to that of many commercial cultivars (Yan and McClung, 2010; Gealy and Yan, 2012). Huagan 3, a particularly promising F8 generation line derived from crosses between the local rice cultivars N9S and PI 312777, is considered to be the first commercially acceptable weed-suppressive cultivar in China (Kong et al., 2011). Bertholdsson (2010) bred spring wheat for improved allelopathic potential by conventional breeding. The material used originated from a cross between a Swedish cultivar with low allelopathic activity and a Tunisian cultivar with high allelopathic activity. The result from the field study was a 19% average reduction in weed biomass for the high allelopathic lines. However, a negative effect was that the grain yield was reduced by 9% in the high allelopathic lines. In this research, the high allelopathic lines showed a lower early biomass compared with the control. If the early biomass of the allelopathic wheat had also been improved, the weed biomass should have been much lower (Bertholdsson, 2004). Putative genes related to the weed competition ability of wheat have been found on chromosomes 1A, 2B, and 5D via quantitative trait locus (QTL) identification, which might be helpful for the breeding of allelopathic wheat (Zuo et al., 2012a). However, until now, a successful allelopathic wheat cultivar has not been obtained. To increase crop resistance to continuous cropping obstacles and autotoxicity and in the selection of crop successions, species’ detoxification potential should be considered as an important indicator of breeding.
Mechanisms Underlying Allelopathy
Allelopathy has been studied for quite some time, and many aspects of plant physiological and biochemical processes have been proved to be affected by allelochemicals (Zeng et al., 2001; Gniazdowska and Bogatek, 2005). A series of physiological and biochemical changes in plants induced by allelochemicals are detailed as follows.
Changes in the Micro- and Ultra-Structure of Cells
The shape and structure of plant cells are affected by allelochemicals. Volatile monoterpenes, eucalyptol and camphor can widen and shorten root cells, in addition to inducing nuclear abnormalities and increasing vacuole numbers (Bakkali et al., 2008; Pawlowski et al., 2012). Cruz Ortega et al. (1988) found that a corn pollen extract reduced mitotic activity by more than 50%, induced nuclear irregularities and pyknotic nuclei, and inhibited radicle and hypocotyl growth in watermelon (Citrullus lanatus var. lanatus). Upon exposure to hordenine and gramine, which are allelochemicals from barley (Hordeum vulgare) roots, the radicle tips of white mustard (Sinapis alba L.) exhibited damaged cell walls, increases in both the size and number of vacuoles, disorganization of organelles, and cell autophagy (Liu and Lovett, 1993). Likewise, cinnamic acid significantly deformed the ultrastructure of cucumber chloroplasts and mitochondria (Wu et al., 2004). After treatment with benzoic acid, mustard (Brassica juncea L.) roots displayed irregularly shaped cells arranged in a disorganized manner and disruption of cell organelles (Kaur et al., 2005). Allelochemicals from Convolvulus arvensis L. and catmint (Nepeta meyeri Benth.) can alter the random amplification of polymorphic DNA (RAPD) profiles of receiver plants (Kekec et al., 2013; Sunar et al., 2013). Citral is a volatile essential oil component of lemongrass (Cymbopogon citrates) and other aromatic plants that has been suggested to have allelopathic traits (Dudai et al., 1999). It was reported that citral can cause disruption of microtubules in wheat and Arabidopsis thaliana L. roots, where the mitotic microtubules were more strongly affected than the cortical microtubules (Chaimovitsh et al., 2010, 2012). Moreover, citral has a strong long-term disorganizing effect on the cell ultra-structure of A. thaliana seedlings, thickening the cell wall and reducing intercellular communication and the formation of root hairs (Grana et al., 2013).
Inhibition of Cell Division and Elongation
Allelochemical monoterpenoids (camphor, 1,8-cineole, beta-pinene, alpha-pinene, and camphene) affected cell proliferation and DNA synthesis in plant meristems (Nishida et al., 2005); 2(3H)-benzoxazolinone (BOA) inhibited the mitotic process, especially the G2-M checkpoint of lettuce (Sanchez-Moreiras et al., 2008); and sorgoleone reduced the number of cells in each cell division period, damaging tubulins and resulting in polyploid nuclei (Hallak et al., 1999). Burgos et al. (2004) argued that the rye allelochemicals BOA and 2, 4-dihydroxy-1,4(2H)-benzoxazin-3-one (DIBOA) significantly inhibited the regeneration of cucumber root cap cells and thus inhibited growth. Following the treatment of soybean with aqueous leaf extracts from Datura stramonium L., Cai and Mu (2012) found that higher concentrations of the extracts inhibited primary root elongation and lateral root development, decreased root hair length and density, inhibited cell division in root tips and increased the chromosomal aberration index and micronucleus index. Teerarak et al. (2012) suggested that the ethyl acetate fraction of Aglaia odorata Lour. leaves inhibited mitosis and induced mitotic abnormalities in Allium cepa roots by damaging chromatin organization and the mitotic spindle in roots exposed to the allelochemicals.
Imbalances in the Antioxidant System
The generation and clearing of reactive oxygen species (ROS) and the balance of the redox state in the cell play an important role in allelopathic effects. After exposure to allelochemicals, the recipient plants may rapidly produce ROS in the contact area (Bais et al., 2003; Ding et al., 2007), and alter the activity of antioxidant enzymes such as superoxide dismutase (SOD), peroxidase (POD; Zeng et al., 2001; Yu et al., 2003) and ascorbic acid peroxidase (APX; Zuo et al., 2012b) to resist oxidative stress. Batish et al. (2008) argued that caffeic acid induces significant changes in the activities of proteases, PODs, and polyphenol oxidases (PPOs) during root development and decreases the content of total endogenous phenolics in hypocotyl cuttings from mung bean (Phaseolus aureus). Shearer et al. (2012) found that allelopathic interactions led to changes in signal transduction and an imbalance between the production of reactive oxidant species and antioxidant capabilities within a coral holobiont. This oxidative imbalance resulted in rapid protein degradation and ultimately, apoptosis or necrosis of the coral Acropora millepora when compensatory transcriptional action by the coral holobiont insufficiently mitigated the damage caused by allelochemicals produced by Chlorodesmis fastigiata (Shearer et al., 2012).
Increases in Cell Membrane Permeability
Many studies have shown that allelochemicals significantly inhibit the activity of antioxidant enzymes and increase free radical levels, resulting in greater membrane lipid peroxidation and membrane potential alteration, which diminish the scavenging effect on activated oxygen and damage the whole membrane system of plants (Lin et al., 2000; Zeng et al., 2001; Lin, 2010; Harun et al., 2014; Sunmonu and Van Staden, 2014). The growth of Hordeum spontaneum, Avena ludoviciana, and wild mustard seedlings were found to be inhibited by an aqueous extract of barley aerial parts through increasing lipid peroxidation (Farhoudi et al., 2012; Farhoudi and Lee, 2013). Zuo et al. (2012b) argued that the combination of non-sterile shoots of wheat and Alopecurus aequalis weeds led to the accumulation of oxygen radical species, such as the superoxide radical O2– anion, H2O2 and malondialdehyde (MDA) in the leaves of transgenic (with Cu/ZnSOD and APX genes) and non-transgenic potato (Solanum tuberosum L.) seedlings, in addition to increasing membrane permeability and altering the activities of SOD and APX. Poonpaiboonpipat et al. (2013) found that lemongrass (Cymbopogon citratus) essential oil damages the membrane system of barnyard grass (Echinochloa crus-galli L.), causing lipid peroxidation and electrolyte leakage. Sun et al. (2014) investigated the generation of ROS induced by pyrogallic acid (PA) in Microcystis aeruginosa. They found O2– to be the precursor of H2O2 and showed that the hydroxyl radical OH·was generated at significant levels, demonstrating that PA caused oxidative stress in M. aeruginosa and that futile redox cycling of PA was the main source of excessive intracellular O2– and consequent H2O2 and OH·production.
Effect on the Plant Growth Regulator System
Allelochemicals can alter the contents of plant growth regulators or induce imbalances in various phytohormones, which inhibits the growth and development of plants, for example, with respect to seed germination and seedling growth. Most phenolic allelochemicals can stimulate IAA oxidase activity and inhibit the reaction of POD with IAA, bound GA or IAA to influence endogenous hormone levels (Yang et al., 2005).
Leslie and Romani (1988) found that salicylic acid inhibited the synthesis of ethylene in cell suspension cultures of pear (Pyrus communis). Through treatment of wheat seedlings with high concentrations of ferulic acid (2.50 mM), Liu and Hu (2001) found that the growth of wheat seedlings was inhibited by the accumulation of IAA, GA3, and CTK, with a simultaneous increase in ABA. An aqueous extract from rice was shown to significantly stimulate IAA oxidase activity in barnyard grass and reduce IAA levels, thereby damaging the growth regulation system and inhibiting seedling growth (Lin et al., 2001). Yang et al. (2008) investigated the mechanisms of two allelochemicals: DTD [4, 7-dimethyl-1-(propan-2-ylidene)-1, 4, 4a, 8a-tetrahydronaphthalene-2, 6(1H, 7H)-dione] and HHO [6-hydroxyl-5-isopropyl-3, 8-dimethyl-4a, 5, 6, 7, 8, 8a-hexahydronaphthalen-2(1H)-one], isolated from Ageratina adenophora Sprengel weeds. DTD at a higher concentration (1.5 mM), significantly increased the ABA content in the roots of rice seedlings, but this decreased sharply after 96 h of treatment. HHO also significantly enhanced the ABA content for 48 and 96 h. However, the application of DTD or HHO decreased the IAA and ZR contents in rice roots. The IAA/ABA and ZR/ABA ratios decreased quantitatively in response to higher concentrations of DTO or HHO. These results suggest that the endogenous hormones might have dependent as well as interactive effects on the responses of rice seedlings and their adaptability to DTD or HHO stress. Moreover, the results from another study indicated that cyanamide (1.2 mM) caused an imbalance of plant hormone (ethylene and auxin) homeostasis in tomato (Solanum lycopersicum L.) roots (Soltys et al., 2012).
Effect on the Functions and Activities of Various Enzymes
Allelochemicals exert different effects on the synthesis, functions, contents and activities of various enzymes. Previous studies have shown that the key enzyme λ-phosphorylase involved in seed germination might be inhibited by chlorogenic acid, caffeic acid and catechol (Rice, 1984; Einhellig, 1995). Additionally, POD, CAT, and cellulase can be suppressed by tannic acid, which can also reduce the synthesis of amylase and acid-phosphatase in the endosperm. Phenolic acids can increase the activity of phenylalanine ammonialyase (PAL) and β-glucosidase, while reducing the activity of phenol-β-glucose transferase, thus inhibiting root growth. In addition, protease, invertase and succinodehydrogenase (SDH) can be suppressed by allelochemicals.
Lin et al. (2001) argued that caffeic acid, gallic acid and phenols regulate phenylalanine metabolism by suppressing the activities of PAL and cinnamic acid-4-hydroxylase. An aquatic extract of the above-ground parts and rhizospheric soil of chrysanthemum (Chrysanthemum indicum L.) inhibited the activities of root dehydrogenase and nitrate reductase (NiR), reduced the contents of soluble sugar and soluble protein, and inhibited the root growth of stem cuttings of the same species (Zhou et al., 2010). Cheng (2012) investigated the effects of diethyl phthalate (DEP) on the enzyme activity and polypeptide accumulation of glutamine synthetase (GS) in greater duckweed (Spirodela polyrhiza L.) and found that DEP is toxic to this species due to the inhibition of GS isoenzymes in nitrogen assimilation and antioxidant enzymes.
Influence on Respiration
Allelochemicals affect plant growth by influencing different stages of respiration, such as electron transfer in the mitochondria, oxidative phosphorylation, CO2 generation and ATP enzyme activity. These chemicals can reduce oxygen intake, which prevents NADH oxidation, inhibits ATP synthesis enzyme activity, reduces ATP formation in mitochondria, disturbs plant oxidative phosphorylation and ultimately inhibits respiration; on the other hand, they can stimulate the release of CO2, which promotes respiration.
Cruz Ortega et al. (1988) found that an ethanol extract from corn pollen acted as an inhibitor of the electron pathway and decreased oxygen consumption; the specific inhibition site was most likely located upstream of cytochrome c. Rasmussen et al. (1992) found that sorgoleone interfered with the function of mitochondria isolated from etiolated soybean and corn seedlings by blocking electron transport at the b-c1 complex. Moreover, Hejl and Koster (2004b) observed that juglone could reach the mitochondria in the root cells of corn and soybean seedlings, thereby disrupting root oxygen uptake. Alpha-pinene, camphor, limonene and other monoterpenes significantly affect radicle and hypocotyl mitochondrial respiration in soybean and corn, but their targets are different. Alpha-pinene acts under at least two mechanisms: uncoupling of oxidative phosphorylation and inhibition of electron transfer. Alpha-pinene strongly inhibits mitochondrial ATP production, decreases the mitochondrial transmembrane potential and impairs mitochondrial energy metabolism. Camphor causes uncoupling of mitochondria. Limonene inhibits coupled respiration but does not affect basal respiration, and inhibits ATP synthetase and the activities of adenine nucleotide translocase complexes at concentrations of 1.0 and 5.0 mM (Abrahim et al., 2003a,b).
Effect on Plant Photosynthesis
The impacts of allelochemicals on plant photosynthesis mainly involve inhibition of or damage to the synthesis machinery and acceleration of the decomposition of photosynthetic pigments. Consequently, photosynthetic pigment contents are decreased, which blocks energy and electron transfer, reduces ATP synthesis enzyme activity, inhibits the synthesis of ATP, and affects stomatal conductance and transpiration, which inhibit the photosynthetic process (Meazza et al., 2002; Yu et al., 2003, 2006; Wu et al., 2004). Allelochemicals affect photosynthesis mainly by influencing the function of PS II (Weir et al., 2004; Wang et al., 2014). For example, sorgoleone inhibits the decay of variable fluorescence, blocks the oxidation of the PSII-reduced primary electron acceptor, Q–A, by the PSII secondary electron acceptor and that of QB by displacing QB from the D1 protein, thus inhibiting photochemical effects (Gonzalez et al., 1997). Similarly, Shao et al. (2009) demonstrated that the D1 protein is an important target in the damage caused to Microcystis by pyrogallol. Moreover, Uddin et al. (2012) found that sorgoleone reduced the Fv/Fm of weeds and inhibited weed growth. By studying the inhibitory effect of the dried macroalga Gracilaria tenuistipitata (Rhodophyta) on the microalga Phaeodactylum tricornutum, Ye et al. (2013) found a decrease in the number of active reaction centers and blockade of the electron transport chain. Poonpaiboonpipat et al. (2013) observed that a high concentration of essential oil from lemongrass (Cymbopogon citratus) leaves significantly decreased the chlorophyll a and b and carotenoid contents of barnyard grass and affected alpha-amylase activity in seeds, indicating that essential oil interferes with photosynthetic metabolism. However, aqueous extracts of leaves from Trema micrantha (Ulmaceae), an allelopathic plant, did not lead to inhibition of the synthesis of photosynthetic pigments in radish (Raphanus sativus L.) (Borella et al., 2014).
Influence on Water and Nutrient Uptake
Many allelochemicals affect nutrient absorption in plant roots or induce water stress through long-term inhibition of water utilization. Allelochemicals can inhibit the activities of Na+/K+-ATPase involved in the absorption and transport of ions at the cell plasma membrane, which suppresses the cellular absorption of K+, Na+, or other ions.
Bergmark et al. (1992) found that ferulic acid (250 μM) inhibited ammonium and NO3– uptake in corn seedlings, although ammonium uptake was less sensitive to this treatment than NO3–. Ferulic acid also inhibits Cl– uptake and increases the initial net K+ loss from roots exposed to a low K ammonium nitrate solution and delays recovery that results in a positive net uptake. Yuan et al. (1998) showed that the effects of allelochemicals, such as ferulic acid, benzaldehyde and 4-tert-butylbenzoic acid, on nitrogen absorption in wheat seedlings are negatively correlated, but the negative effects of NH4+-N on nitrogen absorption were stronger than those of NO3–-N. Yu and Matsui (1997) observed that cinnamic acid and the root exudates of cucumber inhibited the uptake of NO3–, SO42–, K+, Ca2+, Mg2+, and Fe2+ by cucumber seedlings. Through further study, Lv et al. (2002) found that cinnamic acid and p-hydroxybenzoic, the main allelochemicals found in cucumber root exudates, strongly inhibited the activities of root dehydrogenase, root-combined ATPase and nitrate reductase in cucumber, thus inhibiting the root uptake of K+, NO3–, and H2PO4–. Sorgoleone and juglone significantly inhibited H+-ATPase activity and the proton-pumping function across the root cell plasmalemma, which affected solute and water uptake in peas (Pisum sativum L.), soybeans and corn (Hejl and Koster, 2004a,b). Abenavoli et al. (2010) found that the allelochemicals trans-cinnamic, ferulic acid and p-coumaric acid inhibited net nitrate uptake and plasma membrane H+-ATPase activity in maize seedlings, while umbelliferone and caffeic acid had no effect on H+-ATPase activity. Sunflower (Helianthus annus L.) residues negatively affected plant development, the efficiency of translocation of assimilates and nutrient accumulation in radish plants (Barros de Morais et al., 2014).
The effects of allelochemicals on ion uptake are closely related to allelochemical concentrations and classifications. For example, a low concentration of dibutyl phthalate increases the absorption of N but decreases that of P and K. However, a high concentration of this chemical inhibits the absorption of N, P and K. Similarly, a low concentration of diphenylamine stimulates the absorption of N and K but inhibits the absorption of P by tomato roots (Geng et al., 2009).
Influence on Protein and Nucleic Acid Synthesis and Metabolism
Most alkaloids show allelopathic potential. Some can closely integrate with DNA and increase the temperature of DNA cleavage, while some can inhibit DNA polymerase I and prevent the transcription and translation of DNA, whereas others can inhibit protein biosynthesis (Wink and Latzbruning, 1995). Allelochemicals can also inhibit amino acid absorption, in addition to transport, thus interfering with protein synthesis, which affects cell growth (Abenavoli et al., 2003). All phenolic acids can affect the integrity of DNA and RNA. Ferulic acid and cinnamic acid as well as many phenols and alkaloids can also inhibit protein synthesis (Baziramakenga et al., 1997; Zeng et al., 2001; Li et al., 2010). This suggests that the observed allelopathic phenomenon is partly a result of the interaction of the allelochemicals with these basic targets, such as DNA, RNA, protein biosynthesis and related processes.
By analyzing the gene expression profile of A. thaliana after treatment with fagomine, gallic acid, and rutin, which are allelochemicals found in buckwheat (Fagopyrum esculentum Moench), Golisz et al. (2008) observed that genes that reacted to the allelochemicals mainly fell into several functional categories: interaction with the environment, subcellular localization, proteins with a binding function or cofactor requirement, cell rescue, defense and virulence, or metabolism. The plant response to allelochemicals was similar to the response to biotic or abiotic stress. This indicated that allelochemicals might have relevant functions in the cross-talk between biotic and abiotic stress signaling, as they generate ROS (Bais et al., 2003; Baerson et al., 2005; Golisz et al., 2008, 2011). Shao et al. (2009) found that the allelochemical pyrogallol affects the expression of psbA, mcyB, prx, and faab( in Microcystis aeruginosa, and indicated that membranes are the first target in the damage of Microcystis caused by pyrogallol. Guo et al. (2011) showed that HHO affected the expression of CHS, which is associated with the synthesis of various amino acids in Eupatorium adenophorum roots. Cyanamide alters the expression of the expansin genes, LeEXPA9 and LeEXPA18, which are responsible for cell wall remodeling after cytokinesis, thereby inhibiting the formation of tomato root (Soltys et al., 2012). In a recent study, Fang et al. (2015) found that the expression levels of miRNAs relevant to plant hormone signal transduction, p53 signaling pathways, nucleotide excision repair and the peroxisome proliferator-activated receptor were enhanced in barnyard grass co-cultured with allelopathic rice or treated with rice-produced phenolic acids. Kato-Noguchi et al. (2013) reported that the rice allelochemicals momilactone A and B might inhibit the germination of Arabidopsis seeds by inhibiting the degradation process of the storage proteins cruciferin and cruciferina.
Allelochemicals produced by donor plants act on receiver plants, while the receiver plants will react to the donor plants by inducing changes in gene expressions. The up-regulated expression of PAL, cinnamate-4-hydroxylase (C4H), ferulic acid 5-hydroxylase (F5H), and caffeic acid O-methyltransferases (COMT), which are involved in the biosynthesis of phenolic compounds in rice, is consistent with their inhibitory effects on barnyard grass, while barnyard grass induces the expression of genes related to the synthesis of phenolic compounds in allelopathic rice (He et al., 2012a).
Effects of Allelochemicals on Microorganisms and the Ecological Environment
Researchers have found that there are significant relationships between crop growth and soil microbes under the application of allelochemicals or in the presence of allelopathic plants (Figure 3; Barazani and Friedman, 1999; Bais et al., 2006; Mishra et al., 2013). Recent studies demonstrated that indirect effects of allelopathy as a mediator of plant–plant interactions were more important than the direct effects of an inhibitor (Zeng, 2014). Chemical-specific changes in soil microbes could generate negative feedbacks in soil sickness and plant growth (Stinson et al., 2006; Huang et al., 2013; Zhou et al., 2013; Li et al., 2014). Meanwhile, the rhizosphere soil microbes contribute to the allelopathic potential of plants through positive feedback (Inderjit et al., 2011; Zuo et al., 2014; Wu et al., 2015). Bacteria can help to increase inhibition by activating a non-toxic form of an allelochemical (Macias et al., 2003). For example, non-glycosylated compounds may be modified after release from plants and become more toxic (Tanrisever et al., 1987; Macias et al., 2005a). However, bacteria can also help susceptible plants to tolerate biotic stress associated with weeds, and to decrease the allelopathic inhibition of weeds by causing alterations in the expression patterns of some genes that might be responsible for different functions but ultimately lead to a self-defense process (Mishra and Nautiyal, 2012). In addition, the microbial degradation/transformation of allelochemicals in soil affects the effective dose of allelochemicals that can cause plant inhibition (Mishra et al., 2013; Li et al., 2015). Bacterial biofilms in rhizospheric regions can protect colonization sites from phytotoxic allelochemicals and can reduce the toxicity of these chemicals by degrading them (Mishra and Nautiyal, 2012; Mishra et al., 2012). Microorganisms have the ability to alter the components of allelochemicals released into an ecosystem, highlighting their key role in chemical plant–plant interactions and suggesting that allelopathy is likely to shape the vegetation composition and participate in the control of biodiversity in ecology (Fernandez et al., 2013). Some sesquiterpenoid lactones and sulfides are antimicrobial and can disrupt the cell walls of fungi and invasive bacteria, while others can protect plants from environmental stresses that would otherwise cause oxidative damage (Khan et al., 2011; Chadwick et al., 2013). Zhang et al. (2013a) found that antifungal volatiles released from Chinese chive (Allium Tuberosum Rottler) helped to control Panama disease (Fusarium wilt) in banana (Musa spp.) and showed that intercropping/rotation of banana with Chinese chive could control Panama disease and increase cropland biodiversity.
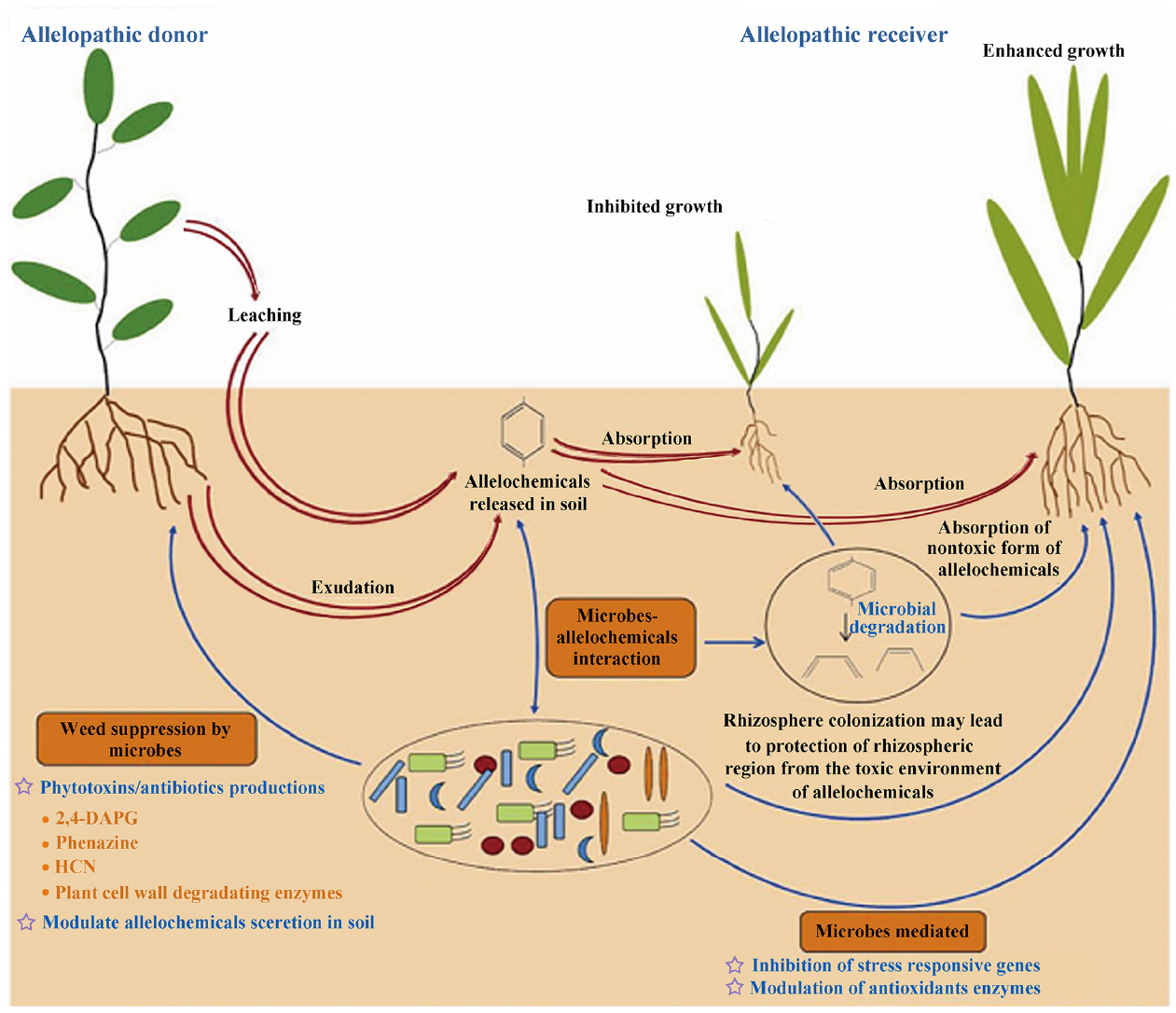
Figure 3. A schematic diagram showing the various roles of microbes in modulating the interaction of allelopathic donor-receiver species (Barazani and Friedman, 1999; Bais et al., 2006; Mishra et al., 2013). Red arrows with double lines indicate the phenomenon of allelopathy, and blue arrows with single lines indicate the involvement of various microbial processes in reducing/enhancing allelopathic inhibition by soil microorganisms. This figure explains that beneficial rhizobacteria can minimize the phytotoxicity of the allelopathic donor toward the allelopathic receiver by using various rhizospheric processes such as rhizosphere colonization, biofilm formation, and degradation/transformation of toxic allelochemicals or modulation of the defense mechanism in receiver species by inducing the expression of stress responsive genes or the activity of antioxidant enzymes. Furthermore, microbes also can play an important role in the activation of allelochemicals, e.g., through the release of non-toxic glycosides followed by microbial degradation to release the active allelochemical.
Wang et al. (2013b) indicated that the shift in the microbial community composition induced by barnyard grass infestation might generate a positive feedback in rice growth and reproduction in a given paddy system. The relative abundance and population of plant parasitic nematodes were significantly reduced in the presence of Chromolaena odorata (Asteraceae) fallow (Odeyemi et al., 2013). Pearse et al. (2014) found that radish soils had a net positive effect on Lupinus nanus biomass and explained that radish might alter the mutualistic/parasitic relationship between L. nanus and its rhizobial associates, with a net benefit to L. nanus. Fang et al. (2013) indicated that inhibiting the expression of the rice PAL gene reduced the allelopathic potential of rice and the diversity of the rhizosphere microflora. These findings suggested that PAL functions as a positive regulator of the rice allelopathic potential.
PGPR, such as root-colonizing Pseudomonas, Paenibacillus polymyxa, endophytes and Chryseobacterium balustinum Aur9, have been shown to alter plant gene expression and regulate plant allelochemical synthesis and signaling pathways to enhance disease resistance, adaptability and defense capabilities in response to biotic and abiotic stresses in plants (van Loon, 2007; Dardanelli et al., 2010; Mishra and Nautiyal, 2012).
Problems and Future Research Directions
Allelochemicals mainly consist of secondary metabolites that are released into the environment through natural pathways, such as volatilization, leaf leaching, residue decomposition, and/or root exudation. Therefore, it should first be noted how allelochemicals are released into the environment (Inderjit and Nilsen, 2003). The activity of allelochemicals varies with research techniques and operational processes (Peng et al., 2004). The natural state of allelochemicals may be changed somewhat during the process of extraction (Li et al., 2002). Therefore, researchers must be careful to determine whether a plant has allelopathic potential or separate and identify allelochemicals using organic solvents and aqueous extracts from plant tissues.
An allelochemical released into the environment is usually not a single substance, and the amounts of allelochemicals released under different conditions vary. Therefore, both the type and amount of allelochemicals released by plants should be considered when their allelopathic potential is investigated. Interactions such as synergy, antagonism and incremental effects between different allelochemicals should be evaluated because one allelochemical may not show allelopathic activity as a single component in a certain situation, but might increase allelopathy in association with other allelochemicals (Albuquerque et al., 2010).
The type and amount of allelochemicals released into the environment depend on the combined effects of the plant itself (plant factors) and environmental factors, as shown in Figure 4 (Albuquerque et al., 2010). The plant factors include the species, variety, growth stage and different tissues (Belz, 2007; Leao et al., 2012; Iannucci et al., 2013). Allelopathic effects vary between varieties or genotypes (Li and Shen, 2006; Zhou et al., 2011; Leao et al., 2012). Plants from the same environment or with close taxonomic proximity do not necessarily display similar production of secondary metabolites, and they may therefore not secrete the same quantity and quality of allelochemicals or have similar allelopathic effects (Chon and Nelson, 2010; Hagan et al., 2013; Imatomi et al., 2013). Lin et al. (2000) found that varietal differences in the allelopathic potential of rice were related to the genetic background. Environmental factors include both abiotic factors (e.g., irradiation, temperature, nutrient limitation, moisture, pH) and biotic factors (e.g., plant competition, diseases, insects, animal invasion, receptor feedback regulation; Anaya, 1999). In a recent study, endogenous levels of allelochemicals were used as indices of abiotic stress resistance. Meanwhile, the exogenous application of allelochemicals has been found to increase the endogenous level of the receivers, with a simultaneous increase in growth and resistance against abiotic stresses (Maqbool et al., 2013); consequently, appropriate environmental conditions are necessary for allelopathic studies. It has been noted that a stress environment can increase the release of allelochemicals from allelopathic plants (Albuquerque et al., 2010). Through studying the dynamic release of allelochemicals under different stress environments, we can clarify the release characteristics of allelochemicals and determine the conditions required for allelochemical release, thereby revealing the nature of allelochemicals.
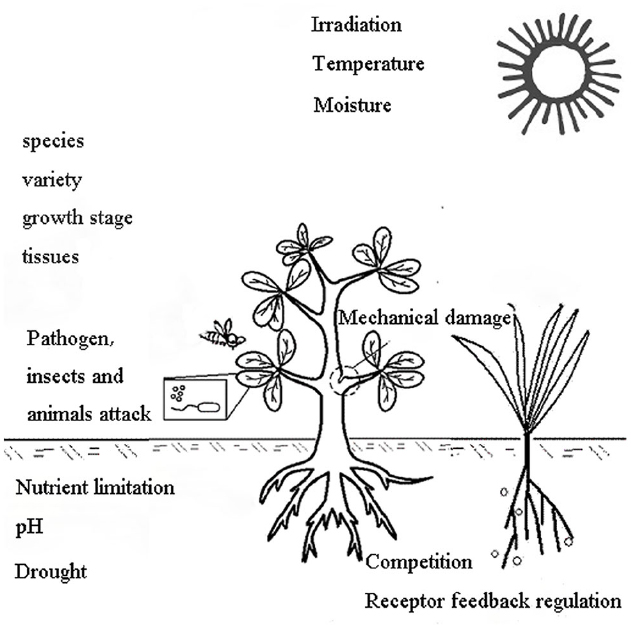
Figure 4. Induction of allelochemical production by the plant itself and environmental factors (Part of this figure was modified from Albuquerque; Albuquerque et al., 2010). The plant factors include species, variety, growth stage, tissue type, etc. Environmental factors include abiotic factors (irradiation, temperature, nutrient limitation, moisture, pH) and biotic factors (plant competition, diseases, insects, animal attack and receptor feedback regulation).
Allelochemicals can be degraded after they have been released into the soil; the half-life of allelochemicals varies from a few hours to a few months (Demuner et al., 2005; Macias et al., 2005b; Wang et al., 2007; Barto and Cipollini, 2009; Bertin et al., 2009), and this is mainly associated with the allelochemical concentration, soil type, soil enzymes, and soil microbial population and community structure (Macias et al., 2004; Understrup et al., 2005; Kong et al., 2008; Gu et al., 2009). Previous studies indicated that some allelochemicals had tremendous spatial and temporal heterogeneity (Weidenhamer, 2005; Dayan et al., 2009; Mohney et al., 2009; Weidenhamer et al., 2009, 2014), but these characteristics of most allelochemicals have not been confirmed. It was reported that polydimethylsiloxane (PDMS) microtubing (silicone tubing microextraction, or STME) could be used as a tool to provide a more finely resolved picture of allelochemical dynamics in the root zone (Weidenhamer, 2005; Mohney et al., 2009; Weidenhamer et al., 2009, 2014). Until now, much remains unknown about the fate or persistence of allelochemicals in the soil or their effects on soil chemistry or microflora (Belz, 2007).
Explaining how allelochemicals function is complicated due to the many classes of chemicals and different structures that have been identified as agents in allelopathy. There is no generic allelochemical, and we should certainly anticipate different mechanisms of action among allelopathic chemicals. Moreover, it should be investigated in future studies whether allelochemicals are absorbed through transport proteins or whether different allelochemicals have the same molecular targets in different species (Weston and Mathesius, 2013). The systematic study of allelochemical detoxification mechanisms in different species will help reveal the differences in detoxification mechanisms between plants and microbes.
Allelopathy is a complex process. Many allelochemicals have been identified to date. Due to the different sensitivities of different receptors to the same allelochemical and the various allelopathic activities of different allelochemicals, considerable further work is required in the field of allelochemical research. Very little is known about the transportation and biodegradation of allelochemicals in soil or the population genetics of allelopathic species, the establishment of practical ways of using allelochemicals in the field, the rapid adaptation of weeds to avoid them, the diversity of the soil microbial community that is maintained in their presence or the role of signal transduction in herbivore defense. These areas should be the focus of future investigations.
Considerable research has showed that allelopathy has good application potential in agricultural production. Until now, many allelopathic crops have been used in agricultural production, but the applications are limited to small-scale and regional areas. The structure and mode of action of many allelochemicals have been deeply revealed in recent years, and this has laid a good foundation for projects where allelochemicals are used to obtain the basic structures or templates for developing new synthetic herbicides.
The commonly used methods of weed control (herbicide application, mechanical weeding and hand weeding) are effective in agricultural production. However, there are many disadvantages associated with these methods, for example, the evolution of herbicide resistance in weeds, the negative impacts of herbicides on environmental, human and animal health, the expense of herbicides, the losses in soil structure and the enormous labor requirements. Many of the above problems can be allayed by creating diversity in weed control practices with the application of allelopathy. The combination of more than one weed control method has been proved to be effective in reducing the probability of herbicide resistance development in weeds. Moreover, the combined application of reduced synthetic herbicides dose and allelopathic extracts can provide control that is as effective as that obtained from the standard dose of herbicides (Farooq et al., 2011). Further, using diverse weed management practices in certain fields can ensure sustainable and effective weed control.
Conclusion
Allelopathy has been known and used in agriculture since ancient times; however, its recognition and use in modern agriculture are very limited. Allelopathy plays an important role in investigations of appropriate farming systems as well as in the control of weeds, diseases and insects, the alleviation of continuous cropping obstacles, and allelopathic cultivar breeding. Furthermore, allelochemicals can act as environmentally friendly herbicides, fungicides, insecticides and plant growth regulators, and can have great value in sustainable agriculture. Although allelochemicals used as environmentally friendly herbicides has been tried for decades, there are very few natural herbicides on the market that are derived from an allelochemical. However, there are a few research investigations testing natural-product herbicides. With increasing emphasis on organic agriculture and environmental protection, increasing attention has been paid to allelopathy research, and the physiological and ecological mechanisms of allelopathy are gradually being elucidated. Moreover, progress has been made in research on the associated molecular mechanisms. It is obvious that allelopathy requires further research for widespread application in agricultural production worldwide.
Conflict of Interest Statement
The authors declare that the research was conducted in the absence of any commercial or financial relationships that could be construed as a potential conflict of interest.
Acknowledgments
The authors acknowledge the editors for providing us with this opportunity to share our understanding of the practices of plant allelopathy in agriculture and the physiological and ecological mechanisms of allelopathy. This research and the writing of this review were supported by a project of the National Natural Science Foundation of China (No. 31471865).
Abbreviations
APX, ascorbic acid peroxidase; BNI, biological nitrification inhibition; BNIS, biological nitrification inhibition substances; BOA, 2(3H)-benzoxazolinone; C4H, cinnamate-4-hydroxylase; CAT, catalase; COMT, caffeic acid O-methyltransferases; DEP, diethyl phthalate; DIBOA, 4-dihydroxy-1,4(2H)-benzoxazin-3-one; DTD, [4, 7-dimethyl-1-(propan-2-ylidene)-1, 4, 4a, 8a-tetrahydronaphthalene-2, 6(1H, 7H)-dione]; F5H, ferulic acid 5-hydroxylase; GR, glutathione reductase; GS, glutamine synthetase; HHO, [6-hydroxyl-5-isopropyl-3, 8-dimethyl-4a, 5, 6, 7, 8, 8a-hexahydronaphthalen-2(1H)-one]; ISR, induced systemic resistance; MDA, malondialdehyde; NiR, nitrate reductase; NIS, nitrification-inhibiting substances; PA, pyrogallic acid; PAL, phenylalanine ammonialyase; PDMS, polydimethylsiloxane; PGPR, plant growth-promoting rhizobacteria; POD, peroxidase; PPO, polyphenol oxidase; QTL, quantitative trait locus; RAPD, random amplification of polymorphic DNA; ROS, reactive oxygen species; SDH, succinodehydrogenase; SOD, superoxide dismutase; STEM, silicone tubing microextraction.
References
Abd El-Gawad, A. M. (2014). Ecology and allelopathic control of Brassica tournefortii in reclaimed areas of the Nile Delta, Egypt. Turk. J. Bot. 38, 347–357. doi: 10.3906/bot-1302-29
Abenavoli, M. R., Lupini, A., Oliva, S., and Sorgona, A. (2010). Allelochemical effects on net nitrate uptake and plasma membrane H+-ATPase activity in maize seedlings. Biol. Plant. 54, 149–153. doi: 10.1007/s10535-010-0024-0
Abenavoli, M. R., Sorgona, A., Sidari, M., Badiani, M., and Fuggi, A. (2003). Coumarin inhibits the growth of carrot (Daucus carota L. cv. Saint Valery) cells in suspension culture. J. Plant Physiol. 160, 227–237. doi: 10.1078/0176-1617-00867
Abrahim, D., Francischini, A. C., Pergo, E. M., Kelmer-Bracht, A. M., and Ishii-Iwamoto, E. L. (2003a). Effects of alpha-pinene on the mitochondrial respiration of maize seedlings. Plant Physiol. Biochem. 41, 985–991. doi: 10.1016/j.plaphy.2003.07.003
Abrahim, D., Takahashi, L., Kelmer-Bracht, A. M., and Ishii-Iwamoto, E. L. (2003b). Effects of phenolic acids and monoterpenes on the mitochondrial respiration of soybean hypocotyl axes. Allelopathy J. 11, 21–30.
Albuquerque, M. B., Santos, R. C., Lima, L. M., Melo Filho, P. D. A., Nogueira, R. J. M. C., Câmara, C. A. G., et al. (2010). Allelopathy, an alternative tool to improve cropping systems. Rev. Agron Sust. Dev. 31, 379–395. doi: 10.1051/agro/2010031
Anaya, A. L. (1999). Allelopathy as a tool in the management of biotic resources in agroecosystems. Crit. Rev. Plant Sci. 18, 697–739. doi: 10.1080/07352689991309450
Andrew, I. K. S., Storkey, J., and Sparkes, D. L. (2015). A review of the potential for competitive cereal cultivars as a tool in integrated weed management. Weed Res. 55, 239–248. doi: 10.1111/wre.12137
An, Y., Ma, Y. Q., and Shui, J. F. (2013). Switchgrass (Panicum virgatum L.) plants and switchgrass residue reduce the biomass and density of associated weeds. Acta Agric. Scand. 63, 107–113. doi: 10.1080/09064710.2012.729605
Baerson, S. R., Sanchez-Moreiras, A., Pedrol-Bonjoch, N., Schulz, M., Kagan, I. A., Agarwal, A. K., et al. (2005). Detoxification and transcriptome response in Arabidopsis seedlings exposed to the allelochemical benzoxazolin-2(3H)-one. J. Biol. Chem. 280, 21867–21881. doi: 10.1074/jbc.M500694200
Bais, H. P., Vepachedu, R., Gilroy, S., Callaway, R. M., and Vivanco, J. M. (2003). Allelopathy and exotic plant invasion: from molecules and genes to species interactions. Science 301, 1377–1380. doi: 10.1126/science.1083245
Bais, H. P., Weir, T. L., Perry, L. G., Gilroy, S., and Vivanco, J. M. (2006). The role of root exudates in rhizosphere interactions with plants and other organisms. Annu. Rev. Plant Biol. 57, 233–266. doi: 10.1146/annurev.arplant.57.032905.105159
Bakkali, F., Averbeck, S., Averbeck, D., and Idaomar, M. (2008). Biological effects of essential oils—a review. Food Chem. Toxicol. 46, 446–475. doi: 10.1016/j.fct.2007.09.106
Barazani, O., and Friedman, J. (1999). Allelopathic bacteria and their impact on higher plants. Crit. Rev. Plant Sci. 18, 741–755. doi: 10.1080/07352689991309469
Barros de Morais, C. S., Silva Dos Santos, L. A., and Vieira Rossetto, C. A. (2014). Oil radish development agronomic affected by sunflower plants reduces. Biosci. J. 30, 117–128.
Barto, E. K., and Cipollini, D. (2009). Half-lives and field soil concentrations of Alliaria petiolata secondary metabolites. Chemosphere 76, 71–75. doi: 10.1016/j.chemosphere.2009.02.020
Batish, D. R., Singh, H. P., Kaur, S., Kohli, R. K., and Yadav, S. S. (2008). Caffeic acid affects early growth, and morphogenetic response of hypocotyl cuttings of mung bean (Phaseolus aureus). J. Plant Physiol. 165, 297–305. doi: 10.1016/j.jplph.2007.05.003
Baziramakenga, R., Leroux, G. D., Simard, R. R., and Nadeau, P. (1997). Allelopathic effects of phenolic acids on nucleic acid and protein levels in soybean seedlings. Can. J. Bot. 75, 445–450. doi: 10.1139/b97-047
Belz, R. G. (2007). Allelopathy in crop/weed interactions–an update. Pest. Manag. Sci. 63, 308–326. doi: 10.1002/ps.1320
Bergmark, C. L., Jackson, W. A., Volk, R. J., and Blum, U. (1992). Differential inhibition by ferulic acid of nitrate and ammonium uptake in Zea mays L. Plant Physiol. 98, 639–645. doi: 10.1104/pp.98.2.639
Bertholdsson, N. O. (2004). Variation in allelopathic activity over 100 years of barley selection and breeding. Weed Res. 44, 78–86. doi: 10.1111/j.1365-3180.2003.00375.x
Bertholdsson, N. O. (2010). Breeding spring wheat for improved allelopathic potential. Weed Res. 50, 49–57. doi: 10.1111/j.1365-3180.2009.00754.x
Bertin, C., Harmon, R., Akaogi, M., Weidenhamer, J. D., and Weston, L. A. (2009). Assessment of the phytotoxic potential of m-tyrosine in laboratory soil bioassays. J. Chem. Ecol. 35, 1288–1294. doi: 10.1007/s10886-009-9707-4
Bhadoria, P. (2011). Allelopathy: a natural way towards weed management. Am. J. Exp. Agric. 1, 7–20.
Borella, J., Martinazzo, E. G., Aumonde, T. Z., Do Amarante, L., De Moraes, D. M., and Villela, F. A. (2014). Performance of radish seeds and seedlings under action of aqueous extract of leaves of Trema Micrantha (Ulmaceae). Biosci. J. 30, 108–116.
Broeckling, C. D., and Vivanco, J. M. (2008). A selective, sensitive, and rapid in-field assay for soil catechin, an allelochemical of Centaurea maculosa. Soil Biol. Biochem. 40, 1189–1196. doi: 10.1016/j.soilbio.2007.12.013
Burgos, N. R., Talbert, R. E., Kim, K. S., and Kuk, Y. I. (2004). Growth inhibition and root ultrastructure of cucumber seedlings exposed to allelochemicals from rye (Secale cereale). J. Chem. Ecol. 30, 671–689. doi: 10.1023/B:JOEC.0000018637.94002.ba
Cai, S. L., and Mu, X. Q. (2012). Allelopathic potential of aqueous leaf extracts of Datura stramonium L. on seed germination, seedling growth and root anatomy of Glycine max (L.) Merrill. Allelopathy J. 30, 235–245.
Chadwick, M., Trewin, H., Gawthrop, F., and Wagstaff, C. (2013). Sesquiterpenoids lactones: benefits to plants and people. Int. J. Mol. Sci. 14, 12780–12805. doi: 10.3390/ijms140612780
Chaimovitsh, D., Abu-Abied, M., Belausov, E., Rubin, B., Dudai, N., and Sadot, E. (2010). Microtubules are an intracellular target of the plant terpene citral. Plant J. 61, 399–408. doi: 10.1111/j.1365-313X.2009.04063.x
Chaimovitsh, D., Rogovoy Stelmakh, O., Altshuler, O., Belausov, E., Abu-Abied, M., Rubin, B., et al. (2012). The relative effect of citral on mitotic microtubules in wheat roots and BY2 cells. Plant Biol. 14, 354–364. doi: 10.1111/j.1438-8677.2011.00511.x
Cheema, Z. A., and Khaliq, A. (2000). Use of sorghum allelopathic properties to control weeds in irrigated wheat in a semi arid region of Punjab. Agric. Ecosyst. Environ. 79, 105–112. doi: 10.1016/S0167-8809(99)00140-1
Cheema, Z. A., Khaliq, A., and Saeed, S. (2004). Weed control in maize (Zea mays L.) through sorghum allelopathy. J. Sustain. Agric. 23, 73–86. doi: 10.1300/J064v23n04_07
Cheema, Z., Farooq, M., and Khaliq, A. (2013). “Application of allelopathy in crop production: success story from Pakistan,” in Allelopathy, eds Z. A. Cheema, M. Farooq, and A. Wahid (Berlin Heidelberg: Springer-Verlag Press), 113–143.
Cheng, T. S. (2012). The toxic effects of diethyl phthalate on the activity of glutamine synthetase in greater duckweed (Spirodela polyrhiza L.). Aquat. Toxicol. 124–125, 171–178. doi: 10.1016/j.aquatox.2012.08.014
Cheng, Z. H., Wang, C. H., Xiao, X. M., and Khan, M. A. (2011). Allelopathic effects of decomposing garlic stalk on some vegetable crops. Afric. J. Biotechnol. 10, 15514–15520.
Cheng, Z. H., and Xu, P. (2013). Lily (Lilium spp.) root exudates exhibit different allelopathies on four vegetable crops. Acta Agric. Scand. 63, 169–175. doi: 10.1080/09064710.2012.734323
Chon, S. U., and Nelson, C. J. (2010). Allelopathy in compositae plants. A review. Agron. Sustain. Dev. 30, 349–358. doi: 10.1051/agro/2009027
Chou, C. H. (1999). Roles of allelopathy in plant biodiversity and sustainable agriculture. Crit. Rev. Plant Sci. 18, 609–636. doi: 10.1016/S0735-2689(99)00393-7
Cruz Ortega, R., Anaya, A. L., and Ramos, L. (1988). Effects of allelopathic compounds of corn pollen on respiration and cell division of watermelon. J. Chem. Ecol. 14, 71–86. doi: 10.1007/BF01022532
Dardanelli, M. S., Manyani, H., Gonzalez-Barroso, S., Rodriguez-Carvajal, M. A., Gil-Serrano, A. M., Espuny, M. R., et al. (2010). Effect of the presence of the plant growth promoting rhizobacterium (PGPR) Chryseobacterium balustinum Aur9 and salt stress in the pattern of flavonoids exuded by soybean roots. Plant Soil 328, 483–493. doi: 10.1007/s11104-009-0127-6
Dayan, F. E., Howell, J., and Weidenhamer, J. D. (2009). Dynamic root exudation of sorgoleone and its in planta mechanism of action. J. Exp. Bot. 60, 2107–2117. doi: 10.1093/jxb/erp082
Demuner, A. J., Barbosa, L. C. A., Chinelatto, L. S., Reis, C., and Silva, A. A. (2005). Sorption and persistence of sorgoleone in red-yellow latosol. Q. Nova 28, 451–455. doi: 10.1590/S0100-40422005000300016
Dhima, K. V., Vasilakoglou, I. B., Eleftherohorinos, I. G., and Lithourgidis, A. S. (2006). Allelopathic potential of winter cereals and their cover crop mulch effect on grass weed suppression and corn development. Crop. Sci. 46, 345–352. doi: 10.2135/cropsci2005-0186
Dietz, M., Machill, S., Hoffmann, H. C., and Schmidtke, K. (2013). Inhibitory effects of Plantago lanceolata L. on soil N mineralization. Plant Soil 368, 445–458. doi: 10.1007/s11104-012-1524-9
Ding, J., Sun, Y., Xiao, C. L., Shi, K., Zhou, Y. H., and Yu, J. Q. (2007). Physiological basis of different allelopathic reactions of cucumber and figleaf gourd plants to cinnamic acid. J. Exp. Bot. 58, 3765–3773. doi: 10.1093/jxb/erm227
Dudai, N., Poljakoff-Mayber, A., Mayer, A. M., Putievsky, E., and Lerner, H. R. (1999). Essential oils as allelochemicals and their potential use as bioherbicides. J. Chem. Ecol. 25, 1079–1089. doi: 10.1023/A:1020881825669
Einhellig, F. A. (1995). “Allelopathy-current status and future goals,” in Allelopathy: Organisms, Processes, and Applications, eds A. Inderjit, K. M. M. Dakshini, and F. A. Einhellig (Washington, DC: American Chemical Society Press), 1–24.
Fang, C., Li, Y., Li, C., Li, B., Ren, Y., Zheng, H., et al. (2015). Identification and comparative analysis of microRNAs in barnyardgrass (Echinochloa crus-galli) in response to rice allelopathy. Plant Cell Environ. 38, 1368–1381. doi: 10.1111/pce.12492
Fang, C., Zhuang, Y., Xu, T., Li, Y., Li, Y., and Lin, W. (2013). Changes in rice allelopathy and rhizosphere microflora by inhibiting rice phenylalanine ammonia-lyase gene expression. J. Chem. Ecol. 39, 204–212. doi: 10.1007/s10886-013-0249-4
Farhoudi, R., and Lee, D. J. (2013). Allelopathic effects of barley extract (Hordeum vulgare) on sucrose synthase activity, lipid peroxidation and antioxidant enzymatic activities of Hordeum spontoneum and Avena ludoviciana. Plant. Natl. Sci. Ind. B 83, 447–452. doi: 10.1007/s40011-012-0137-7
Farhoudi, R., Zangane, H. S., and Saeedipour, S. (2012). Allelopathical effect of barley [Hordeum vulgare (L.) cv. Karon] on germination and lipid peroxidation of wild mustard seedling. Res. Crop. 13, 467–471.
Farooq, M., Bajwa, A. A., Cheema, S. A., and Cheema, Z. A. (2013). Application of allelopathy in crop production. Int. J. Agric. Biol. 15, 1367–1378 doi: 10.1007/978-3-642-30595-5_6
Farooq, M., Hussain, T., Wakeel, A., and Cheema, Z. A. (2014). Differential response of maize and mungbean to tobacco allelopathy. Exp. Agric. 50, 611–624. doi: 10.1017/S0014479714000106
Farooq, M., Jabran, K., Cheema, Z. A., Wahid, A., and Siddique, K. H. (2011). The role of allelopathy in agricultural pest management. Pest. Manag. Sci. 67, 493–506. doi: 10.1002/ps.2091
Fernandez, C., Santonja, M., Gros, R., Monnier, Y., Chomel, M., Baldy, V., et al. (2013). Allelochemicals of Pinus halepensis as drivers of biodiversity in Mediterranean open mosaic habitats during the colonization stage of secondary succession. J. Chem. Ecol. 39, 298–311. doi: 10.1007/s10886-013-0239-6
Field, B., Jordan, F., and Osbourn, A. (2006). First encounters–deployment of defence-related natural products by plants. New Phytol. 172, 193–207. doi: 10.1111/j.1469-8137.2006.01863.x
Fragasso, M., Iannucci, A., and Papa, R. (2013). Durum wheat and allelopathy: toward wheat breeding for natural weed management. Front. Plant Sci. 4:375. doi: 10.3389/fpls.2013.00375
Gao, L., and Li, B. (2004). The study of a specious invasive plant, water hyacinth (Eichhornia crassipes): achievements and challenges. Chin. J. Plant Ecol. 28, 735–752.
Gealy, D. R., and Yan, W. (2012). Weed suppression potential of ‘Rondo’ and other Indica rice germplasm lines. Weed Technol. 26, 517–524. doi: 10.1614/WT-D-11-00141.1
Geng, G. D., Zhang, S. Q., and Cheng, Z. H. (2009). Effects of different allelochemicals on mineral elements absorption of tomato root. China Veget. 4, 48–51.
Gimsing, A. L., Baelum, J., Dayan, F. E., Locke, M. A., Sejero, L. H., and Jacobsen, C. S. (2009). Mineralization of the allelochemical sorgoleone in soil. Chemosphere 76, 1041–1047. doi: 10.1016/j.chemosphere.2009.04.048
Gioria, M., and Osborne, B. A. (2014). Resource competition in plant invasions: emerging patterns and research needs. Front. Plant Sci. 5:501. doi: 10.3389/fpls.2014.00501
Gniazdowska, A., and Bogatek, R. (2005). Allelopathic interactions between plants. Multi site action of allelochemicals. Acta Physiol. Plant 27, 395–407. doi: 10.1007/s11738-005-0017-3
Golisz, A., Sugano, M., and Fujii, Y. (2008). Microarray expression profiling of Arabidopsis thaliana L. in response to allelochemicals identified in buckwheat. J. Exp. Bot. 59, 3099–3109. doi: 10.1093/jxb/ern168
Golisz, A., Sugano, M., Hiradate, S., and Fujii, Y. (2011). Microarray analysis of Arabidopsis plants in response to allelochemical L-DOPA. Planta 233, 231–240. doi: 10.1007/s00425-010-1294-7
Gonzalez, V. M., Kazimir, J., Nimbal, C., Weston, L. A., and Cheniae, G. M. (1997). Inhibition of a photosystem II electron transfer reaction by the natural product sorgoleone. J. Agric. Food Chem. 45, 1415–1421. doi: 10.1021/jf960733w
Grana, E., Sotelo, T., Diaz-Tielas, C., Araniti, F., Krasuska, U., Bogatek, R., et al. (2013). Citral induces auxin and ethylene-mediated malformations and arrests cell division in Arabidopsis thaliana roots. J. Chem. Ecol. 39, 271–282. doi: 10.1007/s10886-013-0250-y
Guillon, M. (2003). Herbicidal Composition Comprising an Allelopathic Substance and Method of use Thereof. European patent No 1110456. Nogueres, France: European Patent Office.
Guo, H., Pei, X., Wan, F., and Cheng, H. (2011). Molecular cloning of allelopathy related genes and their relation to HHO in Eupatorium adenophorum. Mol. Biol. Rep. 38, 4651–4656. doi: 10.1007/s11033-010-0599-8
Gu, Y., Wang, P., and Kong, C. H. (2009). Urease, invertase, dehydrogenase and polyphenoloxidase activities in paddy soil influenced by allelopathic rice variety. Eur. J. Soil Biol. 45, 436–441. doi: 10.1016/j.ejsobi.2009.06.003
Hagan, D. L., Jose, S., and Lin, C. H. (2013). Allelopathic exudates of cogongrass (Imperata cylindrica): implications for the performance of native pine savanna plant species in the southeastern US. J. Chem. Ecol. 39, 312–322. doi: 10.1007/s10886-013-0241-z
Haider, G., Cheema, Z. A., Farooq, M., and Wahid, A. (2015). Performance and nitrogen use of wheat cultivars in response to application of allelopathic crop residues and 3, 4-dimethylpyrazole phosphate. Int. J. Agric. Biol. 17, 261–270.
Hallak, A. M. G., Davide, L. C., and Souza, I. F. (1999). Effects of sorghum (Sorghum bicolor L.) root exudates on the cell cycle of the bean plant (Phaseolus vulgaris L.) root. Genet. Mol. Biol. 22, 95–99. doi: 10.1590/S1415-47571999000100018
Han, X., Cheng, Z. H., Meng, H. W., Yang, X. L., and Ahmad, I. (2013). Allelopathic effect of decomposed garlic (Allium Sativum L.) stalk on lettuce (L. Sativa Var. Crispa L.). Pak. J. Bot. 45, 225–233.
Harun, M. A. Y. A., Robinson, R. W., Johnson, J., and Uddin, M. N. (2014). Allelopathic potential of Chrysanthemoides monilifera subsp. monilifera (boneseed): a novel weapon in the invasion processes. South Afric. J. Bot. 93, 157–166. doi: 10.1016/j.sajb.2014.04.008
He, H. B., Wang, H. B., Fang, C. X., Lin, Z. H., Yu, Z. M., and Lin, W. X. (2012b). Separation of allelopathy from resource competition using rice/barnyardgrass mixed-cultures. PLoS ONE 7:e37201. doi: 10.1371/journal.pone.0037201
He, H., Wang, H., Fang, C., Wu, H., Guo, X., Liu, C., et al. (2012a). Barnyard grass stress up regulates the biosynthesis of phenolic compounds in allelopathic rice. J. Plant Physiol. 169, 1747–1753. doi: 10.1016/j.jplph.2012.06.018
Hejl, A. M., and Koster, K. L. (2004a). The allelochemical sorgoleone inhibits root H+-ATPase and water uptake. J. Chem. Ecol. 30, 2181–2191. doi: 10.1023/B:JOEC.0000048782.87862.7f
Hejl, A. M., and Koster, K. L. (2004b). Juglone disrupts root plasma membrane H+-ATPase activity and impairs water uptake, root respiration, and growth in soybean (Glycine max) and corn (Zea mays). J. Chem. Ecol. 30, 453–471. doi: 10.1023/B:JOEC.0000017988.20530.d5
Huang, L. F., Song, L. X., Xia, X. J., Mao, W. H., Shi, K., Zhou, Y. H., et al. (2013). Plant-soil feedbacks and soil sickness: from mechanisms to application in agriculture. J. Chem. Ecol. 39, 232–242. doi: 10.1007/s10886-013-0244-9
Iannucci, A., Fragasso, M., Platani, C., and Papa, R. (2013). Plant growth and phenolic compounds in the rhizosphere soil of wild oat (Avena fatua L.). Front. Plant Sci. 4:509. doi: 10.3389/fpls.2013.00509
Ihsan, M. Z., Khaliq, A., Mahmood, A., Naeem, M., El-Nakhlawy, F., and Alghabari, F. (2015). Field evaluation of allelopathic plant extracts alongside herbicides on weed management indices and weed-crop regression analysis in maize. Weed Biol. Manag. 15, 78–86. doi: 10.1111/wbm.12070
Imatomi, M., Novaes, P., and Gualtieri, S. C. J. (2013). Interspecific variation in the allelopathic potential of the family Myrtaceae. Acta Bot. Bras 27, 54–61. doi: 10.1590/S0102-33062013000100008
Inderjit, Callaway, R. M., and Vivanco, J. M. (2006). Can plant biochemistry contribute to understanding of invasion ecology? Trends Plant Sci. 11, 574–580. doi: 10.1016/j.tplants.2006.10.004
Inderjit, and del Moral, R. (1997). Is separating resource competition from allelopathy realistic? Bot. Rev. 63, 221–230.
Inderjit, and Nilsen, E. T. (2003). Bioassays and field studies for allelopathy in terrestrial plants: progress and problems. Crit. Rev. Plant Sci. 22, 221–238. doi: 10.1080/713610857
Inderjit, Wardle, D. A., Karban, R., and Callaway, R. M. (2011). The ecosystem and evolutionary contexts of allelopathy. Trends Ecol. Evol. 26, 655–662. doi: 10.1016/j.tree.2011.08.003
Iqbal, J., Cheema, Z. A., and An, M. (2007). Intercropping of field crops in cotton for the management of purple nutsedge (Cyperus rotundus L.). Plant Soil 300, 163–171. doi: 10.1007/s11104-007-9400-8
Jabran, K., Mahajan, G., Sardana, V., and Chauhan, B. S. (2015). Allelopathy for weed control in agricultural systems. Crop. Prot. 72, 57–65. doi: 10.1016/j.cropro.2015.03.004
Jin, Z. H., Zhuang, Y. Y., Dai, S. G., and Li, T. L. (2003). Isolation and identification of extracts of Eichhornia crassipes and their allelopathic effects on algae. B. Environ. Contam. Toxicol. 71, 1048–1052. doi: 10.1007/s00128-003-0226-7
John, J., Shirmila, J., Sarada, S., and Anu, S. (2010). Role of Allelopathy in vegetables crops production. Allelopathy J. 25, 275–311.
Kato-Noguchi, H., Ota, K., Kujime, H., and Ogawa, M. (2013). Effects of momilactone on the protein expression in Arabidopsis germination. Weed Biol. Manag. 13, 19–23. doi: 10.1111/wbm.12005
Kaur, H., Inderjit, and Kaushik, S. (2005). Cellular evidence of allelopathic interference of benzoic acid to mustard (Brassica juncea L.) seedling growth. Plant Physiol. Biochem. 43, 77–81. doi: 10.1016/j.plaphy.2004.12.007
Kekec, G., Mutlu, S., Alpsoy, L., Sakcali, M. S., and Atici, O. (2013). Genotoxic effects of catmint (Nepeta meyeri Benth.) essential oils on some weed and crop plants. Toxicol. Ind. Health. 29, 504–513. doi: 10.1177/0748233712440135
Khanh, T. D., Chung, M. I., Xuan, T. D., and Tawata, S. (2005). The exploitation of crop allelopathy in sustainable agricultural production. J. Agron Crop. Sci. 191, 172–184. doi: 10.1111/j.1439-037x.2005.00172.x
Khan, M. A., Cheng, Z. H., Xiao, X. M., Khan, A. R., and Ahmed, S. S. (2011). Ultrastructural studies of the inhibition effect against Phytophthora capsici of root exudates collected from two garlic cultivars along with their qualitative analysis. Crop. Prot. 30, 1149–1155. doi: 10.1016/j.cropro.2011.04.013
Kloepper, J. W., Leong, J., Teintze, M., and Schroth, M. N. (1980). Enhanced plant growth by siderophores produced by plant growth-promoting rhizobacteria. Nature 286, 885–886. doi: 10.1038/286885a0
Kloepper, J. W., Ryu, C. M., and Zhang, S. (2004). Induced systemic resistance and promotion of plant growth by Bacillus spp. Phytopathology 94, 1259–1266. doi: 10.1094/PHYTO.2004.94.11.1259
Kong, C. H., Chen, X. H., Hu, F., and Zhang, S. Z. (2011). Breeding of commercially acceptable allelopathic rice cultivars in China. Pest. Manag. Sci. 67, 1100–1106. doi: 10.1002/ps.2154
Kong, C. H., and Hu, F. (2001). Allelopathy and its Application. Beijing: Chinese agricultural press
Kong, C. H., Wang, P., Gu, Y., Xu, X. H., and Wang, M. L. (2008). Fate and impact on microorganisms of rice allelochemicals in paddy soil. J. Agric. Food Chem. 56, 5043–5049. doi: 10.1021/jf8004096
Kremer, R. J. (2006). “The role of allelopathic bacteria in weed management,” in Allelochemicals: Biological Control of Plant Pathogens and Diseases, eds X. Inderjit and K. G. Mukerji (Dordrecht: Springer Netherlands Press), 143–155. doi: 10.1007/1-4020-4447-X_7
Leao, P. N., Engene, N., Antunes, A., Gerwick, W. H., and Vasconcelos, V. (2012). The chemical ecology of cyanobacteria. Nat. Prod. Rep. 29, 372–391. doi: 10.1039/c2np00075j
Leslie, C. A., and Romani, R. J. (1988). Inhibition of ethylene biosynthesis by salicylic acid. Plant Physiol. 88, 833–837. doi: 10.1104/pp.88.3.833
Li, S. T., Zhou, J. M., Wang, H. Y., and Chen, X. Q. (2002). Research surveys of allelopathy in plants. Chin. J. Eco. Agric. 10, 72–74.
Li, X. G., Ding, C. F., Hua, K., Zhang, T. L., Zhang, Y. N., Zhao, L., et al. (2014). Soil sickness of peanuts is attributable to modifications in soil microbes induced by peanut root exudates rather than to direct allelopathy. Soil Biol. Biochem. 78, 149–159. doi: 10.1016/j.soilbio.2014.07.019
Li, Y. P., Feng, Y. L., Chen, Y. J., and Tian, Y. H. (2015). Soil microbes alleviate allelopathy of invasive plants. Sci. Bull. 60, 1083–1091. doi: 10.1007/s11434-015-0819-7
Li, Z. H., and Shen, Y. X. (2006). Allelopathic effects of different varieties of Medicago sativa weed regrowth in winter. Acta Pratac Sin. 15, 36–42.
Li, Z. H., Wang, Q., Ruan, X., Pan, C. D., and Jiang, D. A. (2010). Phenolics and plant allelopathy. Molecules 15, 8933–8952. doi: 10.3390/molecules15128933
Liebman, M., and Dyck, E. (1993). Crop-rotation and intercropping strategies for weed management. Ecol. Appl. 3, 92–122. doi: 10.2307/1941795
Lin, W. X. (2010). Effect of self-allelopathy on AOS of Casuarina equisetif olia forst seedling. Fujian J. Agric. Sci. 25, 108–113.
Lin, W. X., He, H. Q., Guo, Y. C., Liang, Y. Y., and Chen, F. Y. (2001). Rice allelopathy and its physiobiochemical characteristics. Chin. J. Appl. Ecol. 12, 871–875.
Lin, W. X., Kim, K. U., and Shin, D. H. (2000). Rice allelopathic potential and its modes of action on Barnyardgrass (Echinochloa crus-galli). Allelopathy J. 7, 215–224.
Liu, D. L., and Lovett, J. V. (1993). Biologically active secondary metabolites of barley. II. Phytotoxicity of barley allelochemicals. J. Chem. Ecol. 19, 2231–2244. doi: 10.1007/BF00979660
Liu, X. F., and Hu, X. J. (2001). Effects of allelochemical ferulic acid on endogenous hormone level of wheat seedling. Chin. J. Ecol. Agric. 9, 96–98.
Lv, W. G., Zhang, C. L., Yuan, F., and Peng, Y. (2002). Mechanism of allelochemicals inhibiting continuous cropping cucumber growth. Sci. Agric. Sin. 35, 106–109.
Ma, Y. Q. (2005). Allelopathic studies of common wheat (Triticum aestivum L.). Weed Biol. Manag. 5, 93–104. doi: 10.1111/j.1445-6664.2005.00164.x
Macias, F. A., Marin, D., Oliveros-Bastidas, A., Castellano, D., Simonet, A. M., and Molinillo, J. M. (2005a). Structure-Activity Relationships (SAR) studies of benzoxazinones, their degradation products and analogues. phytotoxicity on standard target species (STS). J. Agric. Food Chem. 53, 538–548. doi: 10.1021/jf0484071
Macias, F. A., Oliveros-Bastidas, A., Marin, D., Castellano, D., Simonet, A. M., and Molinillo, J. M. (2005b). Degradation studies on benzoxazinoids. Soil degradation dynamics of (2R)-2-O-beta-D-glucopyranosyl-4-hydroxy-(2H)- 1,4-benzoxazin-3(4H)-one (DIBOA-Glc) and its degradation products, phytotoxic allelochemicals from Gramineae. J. Agric. Food Chem. 53, 554–561. doi: 10.1021/jf048702l
Macias, F. A., Marin, D., Oliveros-Bastidas, A., Varela, R. M., Simonet, A. M., Carrera, C., et al. (2003). Allelopathy as a new strategy for sustainable ecosystems development. Biol. Sci. Space 17, 18–23. doi: 10.2187/bss.17.18
Macias, F. A., Oliveros-Bastidas, A., Marin, D., Castellano, D., Simonet, A. M., and Molinillo, J. M. (2004). Degradation studies on benzoxazinoids. Soil degradation dynamics of 2,4-dihydroxy-7-methoxy-(2H)-1,4-benzoxazin-3(4H)-one (DIMBOA) and its degradation products, phytotoxic allelochemicals from gramineae. J. Agric. Food Chem. 52, 6402–6413. doi: 10.1021/jf0488514
Mahmood, A., Cheema, Z. A., Mushtaq, M. N., and Farooq, M. (2013). Maize-sorghum intercropping systems for purple nutsedge management. Arch. Agron Soil Sci. 59, 1279–1288. doi: 10.1080/03650340.2012.704547
Mahmoud, S. S., and Croteau, R. B. (2002). Strategies for transgenic manipulation of monoterpene biosynthesis in plants. Trends Plant Sci. 7, 366–373. doi: 10.1016/S1360-1385(02)02303-8
Mallik, A. U. (2003). Conifer regeneration problems in boreal and temperate forests with ericaceous understory: Role of disturbance, seedbed limitation, and keystone species change. Crit. Rev. Plant Sci. 22, 341–366. doi: 10.1080/713610860
Maqbool, N., Wahid, A., Farooq, M., Cheema, Z. A., and Siddique, K. H. M. (2013). “Allelopathy and abiotic stress interaction in crop plants,” in Allelopathy, eds Z. A. Cheema, M. Farooq, and A. Wahid (Berlin: Springer Berlin Heidelberg), 451–468. doi: 10.1007/978-3-642-30595-5_19
Meazza, G., Scheffler, B. E., Tellez, M. R., Rimando, A. M., Romagni, J. G., Duke, S. O., et al. (2002). The inhibitory activity of natural products on plant p-hydroxyphenylpyruvate dioxygenase. Phytochemistry 60, 281–288. doi: 10.1016/S0031-9422(02)00121-8
Mishra, S., Mishra, A., Chauhan, P. S., Mishra, S. K., Kumari, M., Niranjan, A., et al. (2012). Pseudomonas putida NBRIC19 dihydrolipoamide succinyltransferase (SucB) gene controls degradation of toxic allelochemicals produced by Parthenium hysterophorus. J. Appl. Microbiol. 112, 793–808. doi: 10.1111/j.1365-2672.2012.05256.x
Mishra, S., and Nautiyal, C. S. (2012). Reducing the allelopathic effect of Parthenium hysterophorus L. on wheat (Triticum aestivum L.) by Pseudomonas putida. Plant Growth Regul. 66, 155–165. doi: 10.1007/s10725-011-9639-1
Mishra, S., Upadhyay, R. S., and Nautiyal, C. S. (2013). Unravelling the beneficial role of microbial contributors in reducing the allelopathic effects of weeds. Appl. Microbiol. Biotechnol. 97, 5659–5668. doi: 10.1007/s00253-013-4885-y
Mohney, B. K., Matz, T., Lamoreaux, J., Wilcox, D. S., Gimsing, A. L., Mayer, P., et al. (2009). In situ silicone tube microextraction: a new method for undisturbed sampling of root-exuded thiophenes from marigold (Tagetes erecta L.) in soil. J. Chem. Ecol. 35, 1279–1287. doi: 10.1007/s10886-009-9711-8
Moraes, P. V. D., Agostinetto, D., Vignolo, G. K., Santos, L. S., and Panozzo, L. E. (2009). Cover crop management and weed control in corn. Planta Daninha 27, 289–296. doi: 10.1590/S0100-83582009000200011
Narwal, S. S. (2000). Weed management in rice: wheat rotation by allelopathy. Crit. Rev. Plant Sci. 19, 249–266. doi: 10.1016/S0735-2689(00)80004-0
Nawaz, A., Farooq, M., Cheema, S. A., and Cheema, Z. A. (2014). “Role of allelopathy in weed management,” in Recent Advances in Weed Management, eds B. S. Chauhan and G. Mahajan (New York: Springer-Verlag Press), 39–62.
Nishida, N., Tamotsu, S., Nagata, N., Saito, C., and Sakai, A. (2005). Allelopathic effects of volatile monoterpenoids produced by Salvia leucophylla: Inhibition of cell proliferation and DNA synthesis in the root apical meristem of Brassica campestris seedlings. J. Chem. Ecol. 31, 1187–1203. doi: 10.1007/s10886-005-4256-y
Odeyemi, I. S., Afolami, S. O., and Adigun, J. A. (2013). Plant parasitic nematode relative abundance and population suppression under Chromolaena odorata (Asteraceae) fallow. Int. J. Pest. Manage 59, 79–88. doi: 10.1080/09670874.2013.766776
Ogata, T., Hamachi, M., and Nishi, K. (2008). Organic Herbicide for Paddy Field. Japan patent No 2008050329. Tokyo: Japan Patent Office.
Pawlowski, A., Kaltchuk-Santos, E., Zini, C. A., Caramao, E. B., and Soares, G. L. G. (2012). Essential oils of Schinus terebinthifolius and S. molle (Anacardiaceae): Mitodepressive and aneugenic inducers in onion and lettuce root meristems. South Afric. J. Bot. 80, 96–103. doi: 10.1016/j.sajb.2012.03.003
Pearse, I. S., Bastow, J. L., and Tsang, A. (2014). Radish introduction affects soil biota and has a positive impact on the growth of a native plant. Oecologia 174, 471–478. doi: 10.1007/s00442-013-2779-4
Peng, S. L., Wen, J., and Guo, Q. F. (2004). Mechanism and active variety of allelochemicals. Acta Bot. Sin. 46, 757–766.
Poonpaiboonpipat, T., Pangnakorn, U., Suvunnamek, U., Teerarak, M., Charoenying, P., and Laosinwattana, C. (2013). Phytotoxic effects of essential oil from Cymbopogon citratus and its physiological mechanisms on barnyardgrass (Echinochloa crus-galli). Ind. Crop. Prod. 41, 403–407. doi: 10.1016/j.indcrop.2012.04.057
Rasmussen, J. A., Hejl, A. M., Einhellig, F. A., and Thomas, J. A. (1992). Sorgoleone from root exudate inhibits mitochondrial functions. J. Chem. Ecol. 18, 197–207. doi: 10.1007/BF00993753
Reeves, D. W., Price, A. J., and Patterson, M. G. (2005). Evaluation of three winter cereals for weed control in conservation-tillage nontransgenic cotton. Weed Technol. 19, 731–736. doi: 10.1614/WT-04-245R1.1
Sanchez-Moreiras, A. M., De La Pena, T. C., and Reigosa, M. J. (2008). The natural compound benzoxazolin-2(3H)-one selectively retards cell cycle in lettuce root meristems. Phytochemistry 69, 2172–2179. doi: 10.1016/j.phytochem.2008.05.014
Schulz, M., Marocco, A., Tabaglio, V., Macias, F. A., and Molinillo, J. M. (2013). Benzoxazinoids in rye allelopathy—from discovery to application in sustainable weed control and organic farming. J. Chem. Ecol. 39, 154–174. doi: 10.1007/s10886-013-0235-x
Shao, J., Wu, Z., Yu, G., Peng, X., and Li, R. (2009). Allelopathic mechanism of pyrogallol to Microcystis aeruginosa PCC7806 (Cyanobacteria): from views of gene expression and antioxidant system. Chemosphere 75, 924–928. doi: 10.1016/j.chemosphere.2009.01.021
Shearer, T., Rasher, D., Snell, T., and Hay, M. (2012). Gene expression patterns of the coral Acropora millepora in response to contact with macroalgae. Coral. Reefs. 31, 1177–1192. doi: 10.1007/s00338-012-0943-7
Silva, R. M. G., Brante, R. T., Santos, V. H. M., Mecina, G. F., and Silva, L. P. (2014). Phytotoxicity of ethanolic extract of turnip leaves (Raphanus Sativus L.). Biosci. J. 30, 891–902.
Silva, R. M. G., Brigatti, J. G. F., Santos, V. H. M., Mecina, G. F., and Silva, L. P. (2013). Allelopathic effect of the peel of coffee fruit. Sci. Hortic. Am. 158, 39–44. doi: 10.1016/j.scienta.2013.04.028
Singh, H. P., Batish, D. R., and Kohli, R. K. (1999). Autotoxicity: concept, organisms, and ecological significance. Crit. Rev. Plant Sci. 18, 757–772. doi: 10.1080/07352689991309478
Singh, H. P., Batish, D. R., and Kohli, R. K. (2003). Allelopathic interactions and allelochemicals: new possibilities for sustainable weed management. Crit. Rev. Plant Sci. 22, 239–311. doi: 10.1080/713610858
Soltys, D., Rudzinska-Langwald, A., Gniazdowska, A., Wisniewska, A., and Bogatek, R. (2012). Inhibition of tomato (Solanum lycopersicum L.) root growth by cyanamide is due to altered cell division, phytohormone balance and expansin gene expression. Planta 236, 1629–1638. doi: 10.1007/s00425-012-1722-y
Stinson, K. A., Campbell, S. A., Powell, J. R., Wolfe, B. E., Callaway, R. M., Thelen, G. C., et al. (2006). Invasive plant suppresses the growth of native tree seedlings by disrupting belowground mutualisms. PLoS Biol. 4:e140. doi: 10.1371/journal.pbio.0040140
Sun, X. M., Lu, Z. Y., Liu, B. Y., Zhou, Q. H., Zhang, Y. Y., and Wu, Z. B. (2014). Allelopathic effects of pyrogallic acid secreted by submerged macrophytes on Microcystis aeruginosa: role of ROS generation. Allelopathy J. 33, 121–129.
Sunar, S., Yildirim, N., Aksakal, O., and Agar, G. (2013). Determination of the genotoxic effects of Convolvulus arvensis extracts on corn (Zea mays L.) seeds. Toxicol. Ind. Health. 29, 449–459. doi: 10.1177/0748233712436644
Sunmonu, T. O., and Van Staden, J. (2014). Phytotoxicity evaluation of six fast-growing tree species in South Africa. South Afric. J. Bot. 90, 101–106. doi: 10.1016/j.sajb.2013.10.010
Tabaglio, V., Gavazzi, C., Schulz, M., and Marocco, A. (2008). Alternative weed control using the allelopathic effect of natural benzoxazinoids from rye mulch. Agron Sustain Dev. 28, 397–401. doi: 10.1051/agro:2008004
Tanrisever, N., Fronczek, F. R., Fischer, N. H., and Williamson, G. B. (1987). Ceratolin and other flavonoids from Ceratiola ericoides. Phytochemistry 26, 175–179.
Teerarak, M., Charoenying, P., and Laosinwattana, C. (2012). Physiological and cellular mechanisms of natural herbicide resource from Aglaia odorata Lour. on bioassay plants. Acta Physiol. Plant 34, 1277–1285. doi: 10.1007/s11738-011-0923-5
Uddin, M. R., Park, K. W., Han, S. M., Pyon, J. Y., and Park, S. U. (2012). Effects of sorgoleone allelochemical on chlorophyll fluorescence and growth inhibition in weeds. Allelopathy J. 30, 61–70.
Uddin, M. R., Park, S. U., Dayan, F. E., and Pyon, J. Y. (2014). Herbicidal activity of formulated sorgoleone, a natural product of sorghum root exudate. Pest. Manag. Sci. 70, 252–257. doi: 10.1002/ps.3550
Understrup, A. G., Ravnskov, S., Hansen, H. C., and Fomsgaard, I. S. (2005). Biotransformation of 2-benzoxazolinone to 2-amino-(3H)-phenoxazin-3-one and 2-acetylamino-(3H)-phenoxazin-3-one in soil. J. Chem. Ecol. 31, 1205–1222. doi: 10.1007/s10886-005-4257-x
van Loon, L. C. (2007). Plant responses to plant growth-promoting rhizobacteria. Eur. J. Plant Pathol. 119, 243–254. doi: 10.1007/s10658-007-9165-1
Vaughn, S. F., and Berhow, M. A. (1999). Allelochemicals isolated from tissues of the invasive weed garlic mustard (Alliaria petiolata). J. Chem. Ecol. 25, 2495–2504. doi: 10.1023/A:1020874124645
Wang, C. M., Chen, H. T., Li, T. C., Weng, J. H., Jhan, Y. L., Lin, S. X., et al. (2014). The role of pentacyclic triterpenoids in the allelopathic effects of Alstonia scholaris. J. Chem. Ecol. 40, 90–98. doi: 10.1007/s10886-013-0376-y
Wang, C. M., Jhan, Y. L., Yen, L. S., Su, Y. H., Chang, C. C., Wu, Y. Y., et al. (2013a). The allelochemicals of litchi leaf and its potential as natural herbicide in weed control. Allelopathy J. 32, 157–173.
Wang, M., Wu, C., Cheng, Z., and Meng, H. (2015). Growth and physiological changes in continuously cropped eggplant (Solanum melongena L.) upon relay intercropping with garlic (Allium sativum L.). Front. Plant Sci. 6:262. doi: 10.3389/fpls.2015.00262
Wang, P., Kong, C. H., Hu, F., and Xu, X. H. (2007). Allantoin involved in species interactions with rice and other organisms in paddy soil. Plant Soil 296, 43–51. doi: 10.1007/s11104-007-9288-3
Wang, P., Zhang, X. Y., and Kong, C. H. (2013b). The response of allelopathic rice growth and microbial feedback to barnyardgrass infestation in a paddy field experiment. Eur. J. Soil Biol. 56, 26–32. doi: 10.1016/j.ejsobi.2013.01.006
Weidenhamer, J. D. (2005). Biomimetic measurement of allelochemical dynamics in the rhizosphere. J. Chem. Ecol. 31, 221–236. doi: 10.1007/s10886-005-1337-x
Weidenhamer, J. D., Boes, P. D., and Wilcox, D. S. (2009). Solid-phase root zone extraction (SPRE): a new methodology for measurement of allelochemical dynamics in soil. Plant Soil 322, 177–186. doi: 10.1007/s11104-009-9905-4
Weidenhamer, J. D., Mohney, B. K., Shihada, N., and Rupasinghe, M. (2014). Spatial and temporal dynamics of root exudation: how important is heterogeneity in allelopathic interactions? J. Chem. Ecol. 40, 940–952. doi: 10.1007/s10886-014-0483-4
Weir, T. L., Park, S. W., and Vivanco, J. M. (2004). Biochemical and physiological mechanisms mediated by allelochemicals. Curr. Opin. Plant Biol. 7, 472–479. doi: 10.1016/j.pbi.2004.05.007
Weston, L. A., and Duke, S. O. (2003). Weed and crop allelopathy. Crit. Rev. Plant Sci. 22, 367–389. doi: 10.1080/713610861
Weston, L. A., and Mathesius, U. (2013). Flavonoids: their structure, biosynthesis and role in the rhizosphere, including allelopathy. J. Chem. Ecol. 39, 283–297. doi: 10.1007/s10886-013-0248-5
Wezel, A., Casagrande, M., Celette, F., Vian, J. F., Ferrer, A., and Peigne, J. (2014). Agroecological practices for sustainable agriculture. A review. Agron. Sustain. Dev. 34, 1–20. doi: 10.1007/s13593-013-0180-7
Wink, M., and Latzbruning, B. (1995). “Allelopathic properties of alkaloids and other natural-products-possible modes of action,” in Allelopathy: Organisms, Processes, and Applications, eds A. Inderjit, K. M. M. Dakshini, and F. A. Einhellig (Washington, DC: American Chemical Society Press), 117–126.
Wortman, S. E., Drijber, R. A., Francis, C. A., and Lindquist, J. L. (2013). Arable weeds, cover crops, and tillage drive soil microbial community composition in organic cropping systems. Appl. Soil Ecol. 72, 232–241. doi: 10.1016/j.apsoil.2013.07.014
Wu, F. Z., Pan, K., Ma, F. M., and Wang, X. D. (2004). Effects of ciunamic acid on photosynthesis and cell ultrastructure of cucumber seedlings. Acta Hortic Sin. 31, 183–188.
Wu, Z., Yang, L., Wang, R., Zhang, Y., Shang, Q., Wang, L., et al. (2015). In vitro study of the growth, development and pathogenicity responses of Fusarium oxysporum to phthalic acid, an autotoxin from Lanzhou lily. World J. Microbiol. Biotechnol. 31, 1227–1234. doi: 10.1007/s11274-015-1872-8
Xiong, J., Lin, W., Zhou, J., Wu, M., Chen, X., He, H., et al. (2005). Allelopathy and resources competition of rice under different nitrogen supplies. Chin. J. Appl. Ecol. 16, 885–889.
Xuan, T. D., Shinkichi, T., Khanh, T. D., and Min, C. I. (2005). Biological control of weeds and plant pathogens in paddy rice by exploiting plant allelopathy: an overview. Crop Prot 24, 197–206. doi: 10.1016/j.cropro.2004.08.004
Yan, W. G., and McClung, A. M. (2010). ‘Rondo,’ a long-grain indica rice with resistances to multiple diseases. J. Plant Regist. 4, 131–136. doi: 10.3198/jpr2009.07.0404crc
Yang, G. Q., Wan, F. H., Liu, W. X., and Guo, J. Y. (2008). Influence of two allelochemicals from Ageratina adenophora Sprengel on ABA, IAA, and ZR contents in roots of upland rice seedlings. Allelopathy J. 21, 253–262.
Yang, Q. H., Ye, W. H., Liao, F. L., and Yin, X. J. (2005). Effects of allelochemicals on seed germination. Chin. J. Ecol. 24, 1459–1465.
Ye, C., Zhang, M., and Yang, Y. (2013). “Photosynthetic inhibition on the microalga Phaeodactylum tricornutum by the dried macroalga Gracilaria tenuistipitata,” in Energy and Environment Materials, eds X. F. Tang, Y. Wu, Y. Yao, and Z. Z. Zhang (Beijin: China Academic Journal Electronic Publishing House), 725–731. doi: 10.4028/www.scientific.net/msf.743-744.725
Yildirim, E., and Guvenc, I. (2005). Intercropping based on cauliflower: more productive, profitable and highly sustainable. Eur. J. Agron. 22, 11–18. doi: 10.1016/j.eja.2003.11.003
Yu, J. H., Zhang, Y., Niu, C. X., and Li, J. J. (2006). Effects of two kinds of allelochemicals on photosynthesis and chlorophyll fluorescence parameters of Solanum melongena L. seedlings. Chin. J. Appl. Ecol. 17, 1629–1632.
Yu, J. Q., and Matsui, Y. (1997). Effects of root exudates of cucumber (Cucumis sativus) and allelochemicals on ion uptake by cucumber seedlings. J. Chem. Ecol. 23, 817–827. doi: 10.1023/B:JOEC.0000006413.98507.55
Yu, J. Q., Ye, S. F., Zhang, M. F., and Hu, W. H. (2003). Effects of root exudates and aqueous root extracts of cucumber (Cucumis sativus) and allelochemicals, on photosynthesis and antioxidant enzymes in cucumber. Biochem. Syst. Ecol. 31, 129–139. doi: 10.1016/S0305-1978(02)00150-3
Yuan, G. L., Ma, R. X., Liu, X. F., and Sun, S. S. (1998). Effect of allelochemicals on nitrogen absorption of wheat seeding. Chin. J. Eco. Agric. 39–41.
Zeng, R. (2008). “Allelopathy in Chinese ancient and modern agriculture,” in Allelopathy in Sustainable Agriculture and Forestry, eds R. Zeng, A. Mallik and S. Luo (New York: Springer New York Press), 39–59. doi: 10.1007/978-0-387-77337-7_3
Zeng, R. S. (2014). Allelopathy—the solution is indirect. J. Chem. Ecol. 40, 515–516. doi: 10.1007/s10886-014-0464-7
Zeng, R. S., Luo, S. M., Shi, Y. H., Shi, M. B., and Tu, C. Y. (2001). Physiological and biochemical mechanism of allelopathy of secalonic acid F on higher plants. Agron. J. 93, 72–79. doi: 10.2134/agronj2001.93172x
Zeng, R. S., Mallik, A. U., and Luo, S. M. (2008). Allelopathy in Sustainable Agriculture and Forestry. New York: Springer Press doi: 10.1007/978-0-387-77337-7
Zhang, H., Mallik, A., and Zeng, R. S. (2013a). Control of Panama disease of banana by rotating and intercropping with Chinese chive (Allium tuberosum Rottler): role of plant volatiles. J. Chem. Ecol. 39, 243–252. doi: 10.1007/s10886-013-0243-x
Zhang, W., Ma, Y., Wang, Z., Ye, X., and Shui, J. (2013b). Some soybean cultivars have ability to induce germination of sunflower broomrape. PLoS ONE 8:e59715. doi: 10.1371/journal.pone.0059715
Zheng, Y. L., Feng, Y. L., Zhang, L. K., Callaway, R. M., Valiente-Banuet, A., Luo, D. Q., et al. (2015). Integrating novel chemical weapons and evolutionarily increased competitive ability in success of a tropical invader. New Phytol. 205, 1350–1359. doi: 10.1111/nph.13135
Zhou, B., Kong, C. H., Li, Y. H., Wang, P., and Xu, X. H. (2013). Crabgrass (Digitaria sanguinalis) allelochemicals that interfere with crop growth and the soil microbial community. J. Agric. Food Chem. 61, 5310–5317. doi: 10.1021/jf401605g
Zhou, K., Wang, Z. F., Hao, F. G., and Guo, W. M. (2010). Effects of aquatic extracts from different parts and rhizospheric soil of chrysanthemum on the rooting of stem cuttings of the same species. Acta Bot. Bor. Occid. Sin. 762–768.
Zhou, Y. L., Wang, Y., Li, J. Y., and Xue, Y. J. (2011). Allelopathy of garlic root exudates. Chin. J. Appl. Ecol. 22, 1368–1372.
Zuo, S. P., Liu, G. B., and Li, M. (2012a). Genetic basis of allelopathic potential of winter wheat based on the perspective of quantitative trait locus. Field Crop. Res. 135, 67–73. doi: 10.1016/j.fcr.2012.07.005
Zuo, S. P., Ma, Y. Q., and Ye, L. T. (2012b). In vitro assessment of allelopathic effects of wheat on potato. Allelopathy J. 30, 1–10.
Keywords: allelochemical, allelopathy, agriculture practice, physiological mechanism, ecological mechanism, microorganism, agricultural sustainable development
Citation: Cheng F and Cheng Z (2015) Research Progress on the use of Plant Allelopathy in Agriculture and the Physiological and Ecological Mechanisms of Allelopathy. Front. Plant Sci. 6:1020. doi: 10.3389/fpls.2015.01020
Received: 14 July 2015; Accepted: 04 November 2015;
Published: 17 November 2015.
Edited by:
Richard Sayre, New Mexico Consortium at Los Alamos National Labs, USACopyright © 2015 Cheng and Cheng. This is an open-access article distributed under the terms of the Creative Commons Attribution License (CC BY). The use, distribution or reproduction in other forums is permitted, provided the original author(s) or licensor are credited and that the original publication in this journal is cited, in accordance with accepted academic practice. No use, distribution or reproduction is permitted which does not comply with these terms.
*Correspondence: Zhihui Cheng, Y2hlbmd6aEBud3N1YWYuZWR1LmNu