- 1The Sainsbury Laboratory, Norwich, UK
- 2Shanghai Center for Plant Stress Biology, Shanghai Institutes for Biological Sciences, Chinese Academy of Sciences, Shanghai, China
- 3Department of Life Science, Chung-Ang University, Seoul, South Korea
In plants, activation of growth and activation of immunity are opposing processes that define a trade-off. In the past few years, the growth-promoting hormones brassinosteroids (BR) have emerged as negative regulators of pathogen-associated molecular pattern (PAMP)-triggered immunity (PTI), promoting growth at the expense of defense. The crosstalk between BR and PTI signaling was described as negative and unidirectional, since activation of PTI does not affect several analyzed steps in the BR signaling pathway. In this work, we describe that activation of PTI by the bacterial PAMP flg22 results in the reduced expression of BR biosynthetic genes. This effect does not require BR perception or signaling, and occurs within 15 min of flg22 treatment. Since the described PTI-induced repression of gene expression may result in a reduction in BR biosynthesis, the crosstalk between PTI and BR could actually be negative and bidirectional, a possibility that should be taken into account when considering the interaction between these two pathways.
Introduction
Plants need to integrate multiple environmental signals to finely regulate their growth and development in an adaptive manner. Activation of growth and activation of defense against potential pathogens are opposing processes, and the onset of one frequently results in inhibition of the other (Belkhadir et al., 2014; Huot et al., 2014). In the absence of pathogen challenge, growth is prioritized over defense; upon detection of a pathogen, defense responses are initiated, at the expense of growth. This trade-off between growth and defense is regulated at multiple levels, and its control has been shown to depend on the action of several plant hormones, including jasmonates, gibberellins, brassinosteroids (BR), and salicylic acid (Navarro et al., 2008; Albrecht et al., 2012; Belkhadir et al., 2012; Yang et al., 2012; Lozano-Durán et al., 2013; Chandran et al., 2014; Fan et al., 2014; Malinovsky et al., 2014).
The first layer of plant defense relies on the perception of conserved pathogen-associated molecular patterns (PAMPs) by pattern-recognition receptors (PRR) at the cell surface (Boller and Felix, 2009; Macho and Zipfel, 2014). Recognition of a PAMP by the cognate PRR initiates a signaling cascade that involves signal transduction from the plasma membrane to the nucleus, where transcription is heavily reprogrammed. PAMP-triggered signaling ultimately leads to the activation of the so-called PAMP-triggered immunity (PTI), which is sufficient to ward off most potential pathogens. Activation of PTI also results in a strong inhibition of growth, which can be easily detected when seedlings are treated with certain PAMPs (Gomez-Gomez and Boller, 2000; Kunze et al., 2004). Conversely, the growth-promoting hormones BR can inhibit PTI: activation of BR signaling, triggered by exogenous hormone treatments or by genetic overexpression or activation of components of the pathway, results in a suppression of several PTI responses in Arabidopsis (Albrecht et al., 2012; Belkhadir et al., 2012; Lozano-Durán et al., 2013; Fan et al., 2014; Malinovsky et al., 2014). Since activation of PTI was not found to affect the BR signaling pathway, the BR-PTI crosstalk was described as unidirectional and negative (Albrecht et al., 2012; Belkhadir et al., 2012).
In this work, we describe that activation of PTI by application of the bacterial PAMP flg22 results in the reduced expression of BR biosynthetic genes. This effect can be detected 15 min after treatment, and is sustained during a 24-h treatment. Moreover, this reduction of transcript levels does not require BR perception or signaling. Because the observed PTI-induced repression of gene expression may result in a decrease in BR biosynthesis, the crosstalk between PTI and BR could actually be indirect, negative, and bidirectional, a possibility that should be contemplated when considering the interaction between these two pathways.
Results
Flg22 Treatment Results in the Repression of BR Biosynthetic Genes
Activation of PTI induced by treatment with the bacterial PAMP flg22 leads to heavy transcriptional reprogramming in plants (Navarro et al., 2004; Zipfel et al., 2004). As part of these transcriptional changes, we observed that flg22 treatment consistently results in down-regulation of the BR marker gene CPD, which encodes a protein involved in BR biosynthesis (Szekeres et al., 1996; Table 1). An interrogation of publicly available microarray data (AtGenExpress collection; Schmid et al., 2005) revealed that flg22 treatment triggers a repression of several BR biosynthetic genes other than CPD, namely DWF4, BR6ox1, BR6ox2, CYP90C1, BAS1, SMT2, DWF1, and DWF7 (Table 1). Down-regulation of a subset of these genes can also be detected upon treatment with other PAMPs (Tables 1 and 2), although to a lesser extent. For further analyses, CPD and BR6ox2, which are repressed in response to both flg22 and elf18 (Tables 1 and 2), were selected as marker genes, and their repression following flg22 treatment could be confirmed by qPCR (Figure 1A). A time-course analysis, depicted in Figure 1B, revealed that down-regulation of CPD and DWF4 upon flg22 treatment can be detected 15 min after treatment, and is maintained over a 24-h treatment.
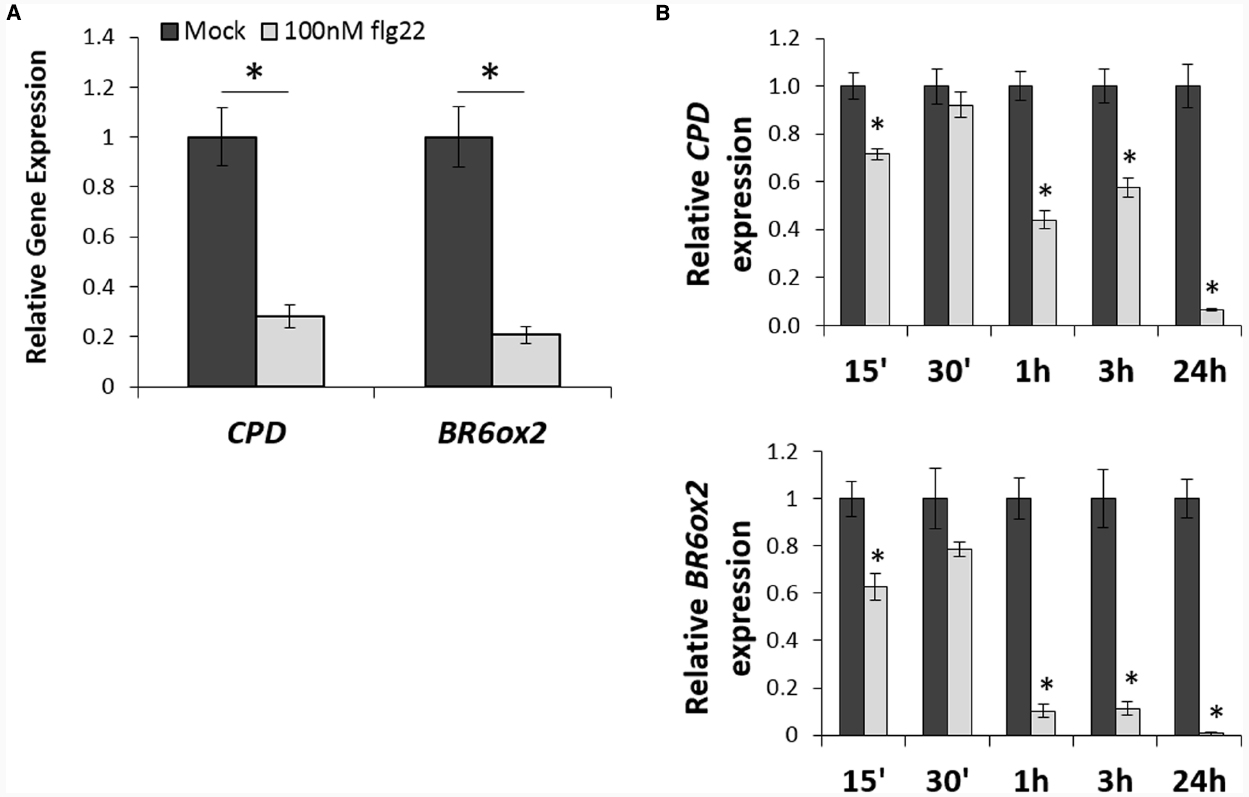
Figure 1. Flg22 treatment downregulates expression of BR biosynthetic genes. (A). Relative expression of the BR biosynthetic genes CPD and BR6ox2 following treatment with flg22, as measured by qPCR. Ten-day-old seedlings (Col-0 wild-type) were submerged in a 100 nM flg22 or mock solution for 1 h. Bars represent standard deviation, with n = 3. Asterisks indicate a statistically significant difference, according to a Student’s t-test, with p < 0.05. This experiment was repeated three times with similar results; values from one representative experiment are shown. (B) Relative expression of the BR biosynthetic genes CPD and BR6ox2 at different time points following treatment with flg22, as measured by qPCR. Ten-day-old seedlings (Col-0 wild-type) were submerged in a 100 nM flg22 or mock solution and samples were taken at 15 min, 30 min, 1 h, 3 h, and 24 h. Bars represent standard deviation, with n = 3. Asterisks indicate a statistically significant difference, according to a Student’s t-test, with p < 0.05. This experiment was repeated three times with similar results; values from one representative experiment are shown.
The flg22-mediated Repression of BR Biosynthetic Genes is Independent of BR Perception
Because expression of BR biosynthetic genes is subjected to a negative feedback loop, and therefore these genes are repressed upon activation of BR signaling (Bancos et al., 2002; He et al., 2005; Tanaka et al., 2005; Sun et al., 2010; Yu et al., 2011), we wondered whether the observed flg22-triggered repression of CPD and BR6ox2 required BR signaling. In order to determine this, we probed the expression changes of these two genes in the BR signaling mutants bri1-301, impaired in BR perception, and bin2-1, in which BR signaling is disrupted downstream of BR perception and upstream of BR-induced transcriptional changes (Peng et al., 2008; Xu et al., 2008). In both mutants, repression of CPD and BR6ox2 could be detected after 1- or 24-h flg22 treatments (Figures 2A,B), indicating that these expression changes do not require an intact BR signaling pathway.
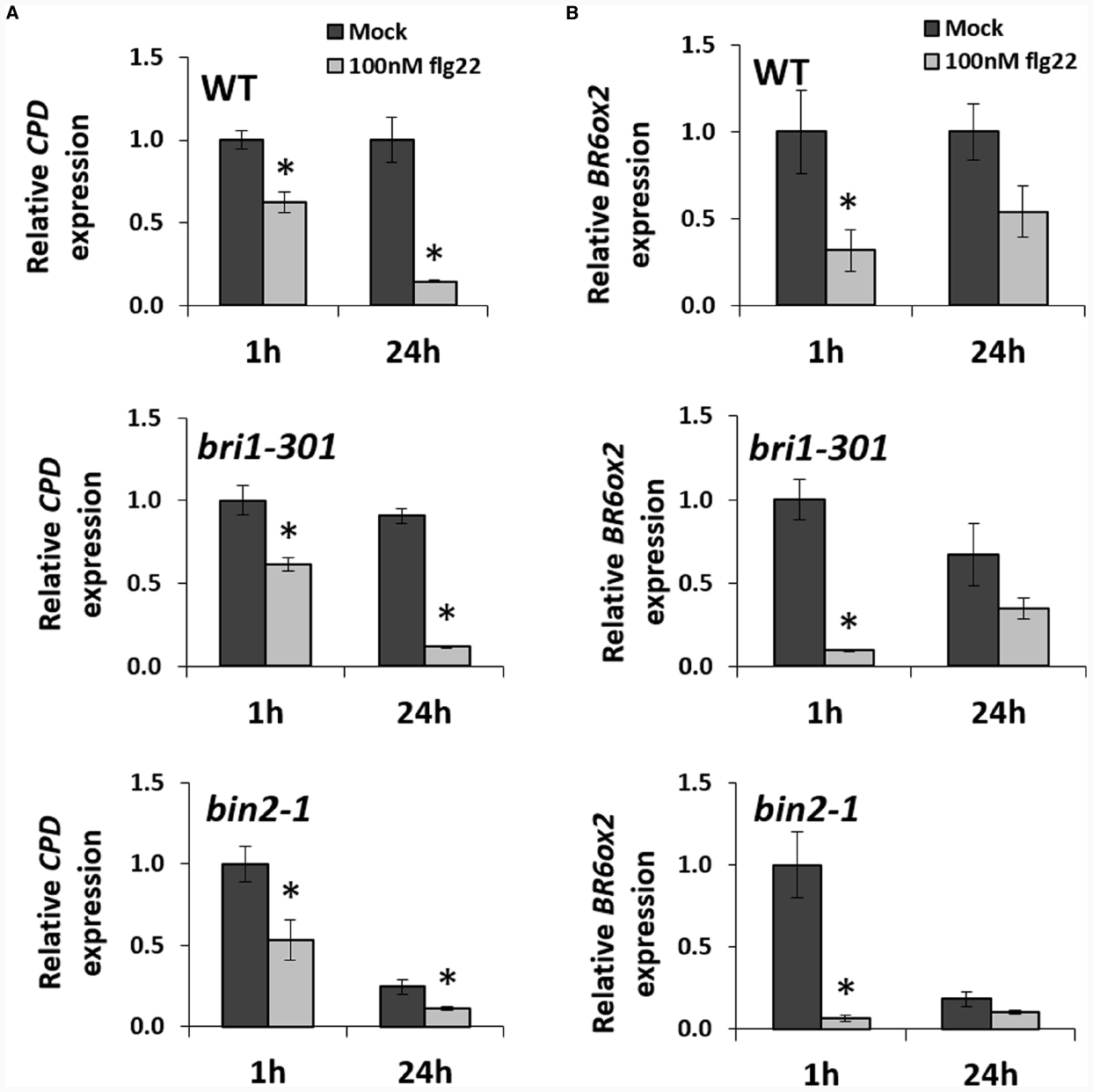
Figure 2. The flg22-triggered repression of BR biosynthetic genes does not require BR perception or signaling. Relative expression of the BR biosynthetic genes CPD (A) and BR6ox2 (B) in Arabidopsis Col-0 wild-type (WT) and the BR signaling mutants bri1-301 and bin2-1, following treatment with flg22, as measured by qPCR. Ten-day-old seedlings were submerged in a 100 nM flg22 or mock solution for 1 or 24 h. Bars represent standard deviation, with n = 3. Asterisks indicate a statistically significant difference, according to a Student’s t-test, with p < 0.05. This experiment was repeated three times with similar results; values from one representative experiment are shown.
Brassinosteroids signaling, as PTI signaling, leads to the transcriptional reprogramming of the cell (Nemhauser et al., 2006; Sun et al., 2010; Yu et al., 2011). The two major transcription factors mediating these changes are BZR1 and BES1 (Wang et al., 2002; Yin et al., 2002; Sun et al., 2010; Yu et al., 2011). Recently, BZR1 was described to mediate crosstalk between the BR and the PTI signaling pathways (Lozano-Durán et al., 2013); BES1 has also been proposed to interact with PTI responses (Kang et al., 2015). Both CPD and BR6ox2 are targets of BZR1, which down-regulates their expression when activated (He et al., 2005; Sun et al., 2010); while CPD has also been found to be repressed by BES1, BR6ox2 has not (Yu et al., 2011). The activation of BZR1 depends on its phospho-status, since only de-phosphorylated BZR1 is active (He et al., 2002; Zhao et al., 2002; Gampala et al., 2007; Ryu et al., 2007), as well as on the availability of interacting partners that act as transcriptional co-regulators (Luo et al., 2010; Bai et al., 2012; Gallego-Bartolome et al., 2012; Li et al., 2012; Oh et al., 2012). Upon activation of BR signaling following BR perception, BZR1 is rapidly de-phosphorylated (He et al., 2002; Tang et al., 2011). In order to determine if the flg22-triggered down-regulation of CPD and BR6ox2 expression depends on BZR1, we investigated the phospho-status of this transcription factor (BZR1-YFP; Gampala et al., 2007), as a proxy for its activation status, in response to flg22. As shown in Figure 3, de-phosphorylated BZR1 can be detected following treatment with the BR brassinolide (BL), but not flg22; co-treatment with flg22 does not affect the effect of BL. Taken together, these results indicate that the flg22-triggered repression of CPD and BR6ox2 is independent of BR signaling.
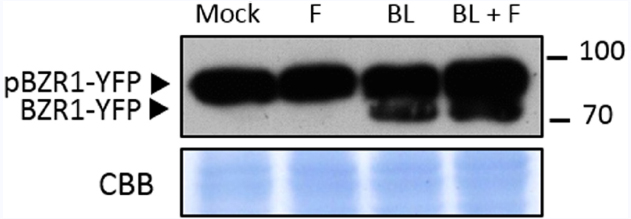
Figure 3. Treatment with flg22 does not affect phosphorylation of BZR1. Accumulation of BZR1-YFP in its phosphorylated (pBZR1-YFP) and de-phosphorylated (BZR1-YFP) forms upon treatment with flg22 (F), brassinolide (BL), flg22 and brassinolide (F+BL) or mock solution. Ten-day-old transgenic Arabidopsis seedlings expressing BZR1-YFP were submerged in a 100 nM flg22, 1 μM brassinolide, 100 nM flg22 + 1 μM brassinolide or mock solution for 1 h. Total proteins were separated in a 10% acrylamide gel and transferred to a PVDF membrane. The membrane was blotted with anti-GFP antibody. CBB: Coomassie brilliant blue. This experiment was repeated four times with similar results.
Discussion
A crosstalk between flg22-triggered and BR signaling had long been postulated, given that both signaling pathways (i) lead to opposing outcomes (i.e., onset of defense versus activation of growth), and (ii) share components involved in signal initiation or transduction (Belkhadir et al., 2014; Lozano-Durán and Zipfel, 2015). Such an interaction was later experimentally confirmed and described to be negative, unidirectional (since only activation of BR signaling negatively affects PTI signaling, and not vice-versa), and at least partially indirect (Vert and Chory, 2011; Albrecht et al., 2012; Belkhadir et al., 2012; Lozano-Durán et al., 2013; Fan et al., 2014; Malinovsky et al., 2014). In these studies, however, activation of PTI signaling was achieved by exogenous flg22 treatment, within a time scale of min to very few hours, and therefore any potential longer-term effect of this pathway could have gone unnoticed. Additionally, activation of PTI could affect BR accumulation rather than sensitivity of the signaling pathway, which would be masked by exogenous hormone treatments or overexpression of rate-limiting components (Albrecht et al., 2012; Belkhadir et al., 2012). Our results indicate that, although activation of PTI signaling by flg22 has been shown not to affect BR signaling (Albrecht et al., 2012; Belkhadir et al., 2012), it leads to a repression of BR biosynthetic genes. This effect can be detected already 15 min after flg22 treatment, and is sustained during a 24-h treatment. The flg22-triggered transcriptional repression of the BR biosynthetic marker genes CPD and BR6ox2 does not require BR perception or signaling, and therefore we hypothesize that it is a direct effect of the activation of flg22-induced PTI signaling. Since the promoters of CPD and BR6ox2 contain binding sites for WRKY and MYB transcription factors (Figure 4; Athena; O’Connor et al., 2005), which are known to mediate defense responses, one hypothesis would be that PTI-activated transcription factors, such as the ones belonging to these families, may directly mediate repression of BR biosynthetic genes. This potential PTI-mediated repression of BR biosynthesis could serve a double purpose: on one hand, it would work to alleviate the BR-mediated repression of PTI upon detection of an impending pathogen; on the other, it would inhibit BR-mediated growth, hence contributing to redirect resources towards immunity and away from growth.
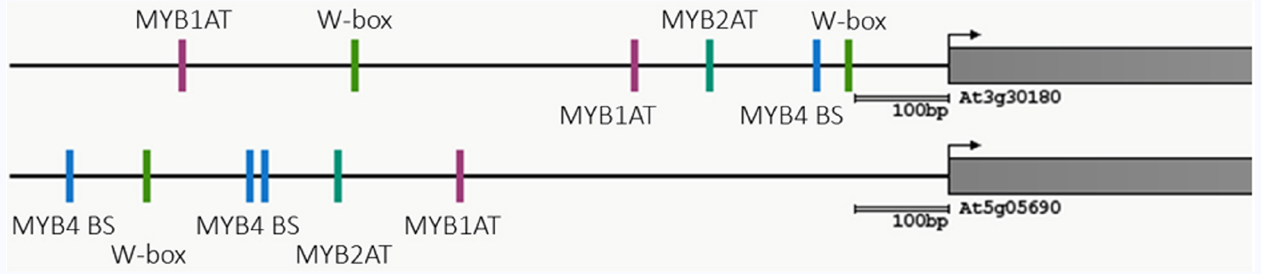
Figure 4. Common transcription binding sites present in the promoters of CPD (At5g05690) and BR6ox2 (At3g30180). The figure has been modified from the Athena output (http://www.bioinformatics2.wsu.edu/cgi-bin/Athena/cgi/home.pl).
Materials and Methods
Plant Materials and Growth Conditions
Arabidopsis thaliana Col-0 was used as genetic background for all experiments. Seedlings were grown as described in Lozano-Durán et al., 2013. The mutant lines bri1-301 and bin2-1 and the transgenic line BZR1-YFP have been previously characterized (Gampala et al., 2007; Peng et al., 2008; Xu et al., 2008).
RNA Extraction
RNA was extracted from 14-day-old seedlings as described in (Couto et al., 2015).
Quantitative Real-time PCR
First-strand cDNA synthesis was performed with the SuperScript III RNA transcriptase (Invitrogen) and oligo(dT) primer, according to the manufacturer’s instructions. For qPCR reactions, the reaction mixture consisted of cDNA first-strand template, primers (10 pmol each) and SYBR Green JumpStart Taq ReadyMix (Sigma). qPCR was performed in a BioRad CFX96 real-time system. UBQ10 was used as the internal control; expression in mock-treated Col-0 seedlings was used as the calibrator, with the expression level set to one. Relative expression was determined using the comparative Ct method (2-ΔΔCt). Each data point is the mean value of three biological replicates.
Protein Extraction and Immunoblotting
Protein extraction from 14-day-old seedlings and immunoblotting were performed as described in (Albrecht et al., 2012).
Conflict of Interest Statement
The authors declare that the research was conducted in the absence of any commercial or financial relationships that could be construed as a potential conflict of interest.
Acknowledgments
The authors would like to thank Jianming Li and Zhiyong Wang for sharing biological materials, and Alberto Macho for critical reading of the manuscript. Funding from the Gatsby Charitable Foundation for this work is acknowledged. Work in the Lozano-Durán laboratory is funded by the Shanghai Center for Plant Stress Biology of the Chinese Academy of Sciences. This work was partly supported by a grant from Next-Generation BioGreen 21 program (PJ011132 to S.-K. Kim), Rural Development Administration of Korea.
References
Albrecht, C., Boutrot, F., Segonzac, C., Schwessinger, B., Gimenez-Ibanez, S., Chinchilla, D., et al. (2012). Brassinosteroids inhibit pathogen-associated molecular pattern-triggered immune signaling independent of the receptor kinase BAK1. Proc. Natl. Acad. Sci. U.S.A. 109, 303–308. doi: 10.1073/pnas.1109921108
Bai, M. Y., Shang, J. X., Oh, E., Fan, M., Bai, Y., Zentella, R., et al. (2012). Brassinosteroid, gibberellin and phytochrome impinge on a common transcription module in Arabidopsis. Nat. Cell Biol. 14, 810–817. doi: 10.1038/ncb2546
Bancos, S., Nomura, T., Sato, T., Molnar, G., Bishop, G. J., Koncz, C., et al. (2002). Regulation of transcript levels of the Arabidopsis cytochrome p450 genes involved in brassinosteroid biosynthesis. Plant Physiol. 130, 504–513. doi: 10.1104/pp.005439
Belkhadir, Y., Jaillais, Y., Epple, P., Balsemao-Pires, E., Dangl, J. L., and Chory, J. (2012). Brassinosteroids modulate the efficiency of plant immune responses to microbe-associated molecular patterns. Proc. Natl. Acad. Sci. U.S.A. 109, 297–302. doi: 10.1073/pnas.1112840108
Belkhadir, Y., Yang, L., Hetzel, J., Dangl, J. L., and Chory, J. (2014). The growth-defense pivot: crisis management in plants mediated by LRR-RK surface receptors. Trends Biochem. Sci. 39, 447–456. doi: 10.1016/j.tibs.2014.06.006
Boller, T., and Felix, G. (2009). A renaissance of elicitors: perception of microbe-associated molecular patterns and danger signals by pattern-recognition receptors. Annu. Rev. Plant Biol. 60, 379–406. doi: 10.1146/annurev.arplant.57.032905.105346
Chandran, D., Rickert, J., Huang, Y., Steinwand, M. A., Marr, S. K., and Wildermuth, M. C. (2014). Atypical E2F transcriptional repressor DEL1 acts at the intersection of plant growth and immunity by controlling the hormone salicylic acid. Cell Host Microbe 15, 506–513. doi: 10.1016/j.chom.2014.03.007
Couto, D., Stransfeld, L., Arruabarrena, A., Zipfel, C., and Lozano-Duran, R. (2015). Broad application of a simple and affordable protocol for isolating plant RNA. BMC Res. Notes 8:154. doi: 10.1186/s13104-015-1119-7
Fan, M., Bai, M. Y., Kim, J. G., Wang, T., Oh, E., Chen, L., et al. (2014). The bHLH transcription factor HBI1 mediates the trade-off between growth and pathogen-associated molecular pattern-triggered immunity in Arabidopsis. Plant Cell 26, 828–841. doi: 10.1105/tpc.113.121111
Gallego-Bartolome, J., Minguet, E. G., Grau-Enguix, F., Abbas, M., Locascio, A., Thomas, S. G., et al. (2012). Molecular mechanism for the interaction between gibberellin and brassinosteroid signaling pathways in Arabidopsis. Proc. Natl. Acad. Sci. U.S.A. 109, 13446–13451. doi: 10.1073/pnas.1119992109
Gampala, S. S., Kim, T. W., He, J. X., Tang, W., Deng, Z., Bai, M. Y., et al. (2007). An essential role for 14-3-3 proteins in brassinosteroid signal transduction in Arabidopsis. Dev. Cell 13, 177–189. doi: 10.1016/j.devcel.2007.06.009
Gomez-Gomez, L., and Boller, T. (2000). FLS2: an LRR receptor-like kinase involved in the perception of the bacterial elicitor flagellin in Arabidopsis. Mol. Cell 5, 1003–1011. doi: 10.1016/S1097-2765(00)80265-8
He, J. X., Gendron, J. M., Sun, Y., Gampala, S. S., Gendron, N., Sun, C. Q., et al. (2005). BZR1 is a transcriptional repressor with dual roles in brassinosteroid homeostasis and growth responses. Science 307, 1634–1638. doi: 10.1126/science.1107580
He, J. X., Gendron, J. M., Yang, Y., Li, J., and Wang, Z. Y. (2002). The GSK3-like kinase BIN2 phosphorylates and destabilizes BZR1, a positive regulator of the brassinosteroid signaling pathway in Arabidopsis. Proc. Natl. Acad. Sci. U.S.A. 99, 10185–10190. doi: 10.1073/pnas.152342599
Huot, B., Yao, J., Montgomery, B. L., and He, S. Y. (2014). Growth-defense tradeoffs in plants: a balancing act to optimize fitness. Mol. Plant. 7, 1267–1287. doi: 10.1093/mp/ssu049
Kang, S., Yang, F., Li, L., Chen, H., Chen, S., and Zhang, J. (2015). The Arabidopsis transcription factor BRASSINOSTEROID INSENSITIVE1-ETHYL METHANESULFONATE-SUPPRESSOR1 is a direct substrate of MITOGEN-ACTIVATED PROTEIN KINASE6 and regulates immunity. Plant Physiol. 167, 1076–1086. doi: 10.1104/pp.114.250985
Kunze, G., Zipfel, C., Robatzek, S., Niehaus, K., Boller, T., and Felix, G. (2004). The N terminus of bacterial elongation factor Tu elicits innate immunity in Arabidopsis plants. Plant Cell 16, 3496–3507. doi: 10.1105/tpc.104.026765
Li, Q. F., Wang, C., Jiang, L., Li, S., Sun, S. S., and He, J. X. (2012). An interaction between BZR1 and DELLAs mediates direct signaling crosstalk between brassinosteroids and gibberellins in Arabidopsis. Sci. Signal. 5, ra72. doi: 10.1126/scisignal.2002908
Lozano-Durán, R., Macho, A. P., Boutrot, F., Segonzac, C., Somssich, I. E., and Zipfel, C. (2013). The transcriptional regulator BZR1 mediates trade-off between plant innate immunity and growth. eLife 2:e00983. doi: 10.7554/eLife.00983
Lozano-Durán, R., and Zipfel, C. (2015). Trade-off between growth and immunity: role of brassinosteroids. Trends Plant Sci. 20, 12–19. doi: 10.1016/j.tplants.2014.09.003
Luo, X. M., Lin, W. H., Zhu, S., Zhu, J. Y., Sun, Y., Fan, X. Y., et al. (2010). Integration of light- and brassinosteroid-signaling pathways by a GATA transcription factor in Arabidopsis. Dev. Cell 19, 872–883. doi: 10.1016/j.devcel.2010.10.023
Macho, A. P., and Zipfel, C. (2014). Plant PRRs and the activation of innate immune signaling. Mol. Cell 54, 263–272. doi: 10.1016/j.molcel.2014.03.028
Malinovsky, F. G., Batoux, M., Schwessinger, B., Youn, J. H., Stransfeld, L., Win, J., et al. (2014). Antagonistic regulation of growth and immunity by the Arabidopsis basic helix-loop-helix transcription factor homolog of brassinosteroid enhanced expression2 interacting with increased leaf inclination1 binding bHLH1. Plant Physiol. 164, 1443–1455. doi: 10.1104/pp.113.234625
Navarro, L., Bari, R., Achard, P., Lison, P., Nemri, A., Harberd, N. P., et al. (2008). DELLAs control plant immune responses by modulating the balance of jasmonic acid and salicylic acid signaling. Curr. Biol. 18, 650–655. doi: 10.1016/j.cub.2008.03.060
Navarro, L., Zipfel, C., Rowland, O., Keller, I., Robatzek, S., Boller, T., et al. (2004). The transcriptional innate immune response to flg22. Interplay and overlap with Avr gene-dependent defense responses and bacterial pathogenesis. Plant Physiol. 135, 1113–1128. doi: 10.1104/pp.103.036749
Nemhauser, J. L., Hong, F., and Chory, J. (2006). Different plant hormones regulate similar processes through largely nonoverlapping transcriptional responses. Cell 126, 467–475. doi: 10.1016/j.cell.2006.05.050
O’Connor, T. R., Dyreson, C., and Wyrick, J. J. (2005). Athena: a resource for rapid visualization and systematic analysis of Arabidopsis promoter sequences. Bioinformatics 21, 4411–4413. doi: 10.1093/bioinformatics/bti714
Oh, E., Zhu, J. Y., and Wang, Z. Y. (2012). Interaction between BZR1 and PIF4 integrates brassinosteroid and environmental responses. Nat. Cell Biol. 14, 802–809. doi: 10.1038/ncb2545
Peng, P., Yan, Z., Zhu, Y., and Li, J. (2008). Regulation of the Arabidopsis GSK3-like kinase BRASSINOSTEROID-INSENSITIVE 2 through proteasome-mediated protein degradation. Mol. Plant 1, 338–346. doi: 10.1093/mp/ssn001
Ryu, H., Kim, K., Cho, H., Park, J., Choe, S., and Hwang, I. (2007). Nucleocytoplasmic shuttling of BZR1 mediated by phosphorylation is essential in Arabidopsis brassinosteroid signaling. Plant Cell 19, 2749–2762. doi: 10.1105/tpc.107.053728
Schmid, M., Davison, T. S., Henz, S. R., Pape, U. J., Demar, M., Vingron, M., et al. (2005). A gene expression map of Arabidopsis thaliana development. Nat. Genet. 37, 501–506. doi: 10.1038/ng1543
Sun, Y., Fan, X. Y., Cao, D. M., Tang, W., He, K., Zhu, J. Y., et al. (2010). Integration of brassinosteroid signal transduction with the transcription network for plant growth regulation in Arabidopsis. Dev. Cell 19, 765–777. doi: 10.1016/j.devcel.2010.10.010
Szekeres, M., Nemeth, K., Koncz-Kalman, Z., Mathur, J., Kauschmann, A., Altmann, T., et al. (1996). Brassinosteroids rescue the deficiency of CYP90, a cytochrome P450, controlling cell elongation and de-etiolation in Arabidopsis. Cell 85, 171–182. doi: 10.1016/S0092-8674(00)81094-6
Tanaka, K., Asami, T., Yoshida, S., Nakamura, Y., Matsuo, T., and Okamoto, S. (2005). Brassinosteroid homeostasis in Arabidopsis is ensured by feedback expressions of multiple genes involved in its metabolism. Plant Physiol. 138, 1117–1125. doi: 10.1104/pp.104.058040
Tang, W., Yuan, M., Wang, R., Yang, Y., Wang, C., Oses-Prieto, J. A., et al. (2011). PP2A activates brassinosteroid-responsive gene expression and plant growth by dephosphorylating BZR1. Nat. Cell Biol. 13, 124–131. doi: 10.1038/ncb2151
Vert, G., and Chory, J. (2011). Crosstalk in cellular signaling: background noise or the real thing? Dev. Cell 21, 985–991. doi: 10.1016/j.devcel.2011.11.006
Wang, Z. Y., Nakano, T., Gendron, J., He, J., Chen, M., Vafeados, D., et al. (2002). Nuclear-localized BZR1 mediates brassinosteroid-induced growth and feedback suppression of brassinosteroid biosynthesis. Dev. Cell 2, 505–513. doi: 10.1016/S1534-5807(02)00153-3
Xu, W., Huang, J., Li, B., Li, J., and Wang, Y. (2008). Is kinase activity essential for biological functions of BRI1? Cell Res. 18, 472–478. doi: 10.1038/cr.2008.36
Yang, D. L., Yao, J., Mei, C. S., Tong, X. H., Zeng, L. J., Li, Q., et al. (2012). Plant hormone jasmonate prioritizes defense over growth by interfering with gibberellin signaling cascade. Proc. Natl. Acad. Sci. U.S.A. 109, E1192–E1200. doi: 10.1073/pnas.1201616109
Yin, Y., Wang, Z. Y., Mora-Garcia, S., Li, J., Yoshida, S., Asami, T., et al. (2002). BES1 accumulates in the nucleus in response to brassinosteroids to regulate gene expression and promote stem elongation. Cell 109, 181–191. doi: 10.1016/S0092-8674(02)00721-3
Yu, X., Li, L., Zola, J., Aluru, M., Ye, H., Foudree, A., et al. (2011). A brassinosteroid transcriptional network revealed by genome-wide identification of BESI target genes in Arabidopsis thaliana. Plant J. 65, 634–646. doi: 10.1111/j.1365-313X.2010.04449.x
Zhao, J., Peng, P., Schmitz, R. J., Decker, A. D., Tax, F. E., and Li, J. (2002). Two putative BIN2 substrates are nuclear components of brassinosteroid signaling. Plant Physiol. 130, 1221–1229. doi: 10.1104/pp.102.010918
Zipfel, C., Kunze, G., Chinchilla, D., Caniard, A., Jones, J. D., Boller, T., et al. (2006). Perception of the bacterial PAMP EF-Tu by the receptor EFR restricts Agrobacterium-mediated transformation. Cell 125, 749–760. doi: 10.1016/j.cell.2006.03.037
Keywords: immunity, brassinosteroids, crosstalk, transcriptional regulation, flagellin
Citation: Jiménez-Góngora T, Kim S-K, Lozano-Durán R and Zipfel C (2015) Flg22-Triggered Immunity Negatively Regulates Key BR Biosynthetic Genes. Front. Plant Sci. 6:981. doi: 10.3389/fpls.2015.00981
Received: 24 September 2015; Accepted: 26 October 2015;
Published: 09 November 2015.
Edited by:
Dingzhong Tang, Institute of Genetics and Developmental Biology, Chinese Academy of Sciences, ChinaReviewed by:
Kenichi Tsuda, Max Planck Institute for Plant Breeding Research, GermanyYoussef Belkhadir, Gregor Mendel Institute, Austria
Copyright © 2015 Jiménez-Góngora, Kim, Lozano-Durán and Zipfel. This is an open-access article distributed under the terms of the Creative Commons Attribution License (CC BY). The use, distribution or reproduction in other forums is permitted, provided the original author(s) or licensor are credited and that the original publication in this journal is cited, in accordance with accepted academic practice. No use, distribution or reproduction is permitted which does not comply with these terms.
*Correspondence: Cyril Zipfel, cyril.zipfel@tsl.ac.uk; Rosa Lozano-Durán, lozano-duran@sibs.ac.cn
†These authors have contributed equally to this work.