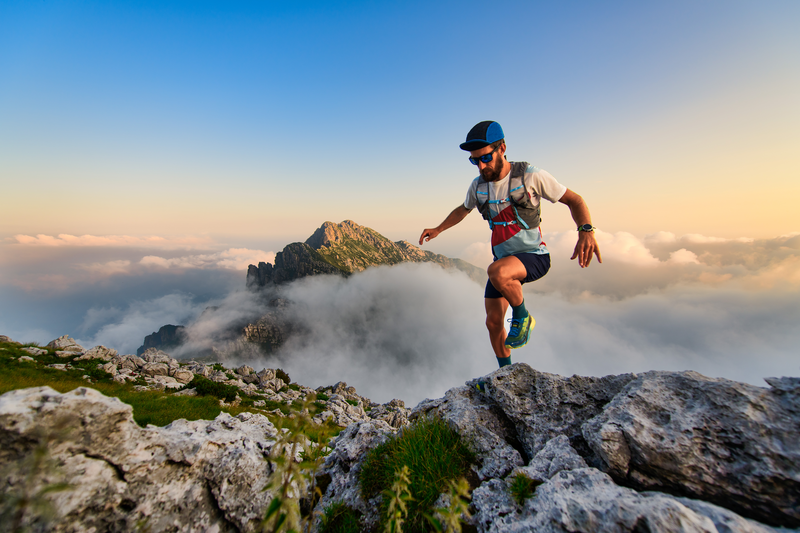
95% of researchers rate our articles as excellent or good
Learn more about the work of our research integrity team to safeguard the quality of each article we publish.
Find out more
PERSPECTIVE article
Front. Physiol. , 19 February 2025
Sec. Mitochondrial Research
Volume 16 - 2025 | https://doi.org/10.3389/fphys.2025.1509159
Flow Mediated Skin Fluorescence (FMSF) is a new non-invasive diagnostic method for assessing vascular circulation and/or metabolic regulation. The method measures stimulation of the circulation in response to post-occlusive reactive hyperemia (PORH). It analyzes the dynamical changes in NADH fluorescence emitted from skin tissue, providing information on mitochondrial metabolic status and intracellular oxygen delivery through the circulatory system. Assessment of the vascular state using the FMSF technique is based on three parameters: flowmotion (FM) under normoxia conditions, hypoxia sensitivity (HS), and hyperemic response (HRmax). The functioning of mitochondria can be assessed by analyzing the ischemic response (IRmax), hypoxia sensitivity (HS), and the basal level of NADH fluorescence. There is a close relationship between the functioning of mitochondria and the vascular system. Despite these interactions, mitochondrial and vascular regulatory function can be monitored separately as well as simultaneously by the FMSF technique. Uniquely, this approach delivers information on both mitochondrial and vascular function based on a single measurement.
Flow Mediated Skin Fluorescence (FMSF) is a novel non-invasive diagnostic technique based on the measurement of nicotinamide adenine dinucleotide (NADH) fluorescence from skin tissue cells. The diagnostic potential of the method is based primarily on stimulation of the circulation in response to post-occlusive reactive hyperemia (PORH). This is the most popular test used to assess vascular reactivity in both macro- and microcirculation. Occlusion of the brachial artery induces reactive hyperemia. Several important mediators are involved, with nitric oxide (NO) acting as a potent vasodilator of muscle-type arteries (Roustit and Cracowski, 2013). The progress and treatment of various diseases associated with vascular dysfunction can be monitored.
NADH and its oxidized form (NAD+) play a crucial role in biological systems as redox coenzymes (Gebicki et al., 2004). Under normal aerobic conditions, NADH is oxidized to NAD+ in mitochondria. During hypoxia, insufficient oxidation leads to excessive NADH accumulation. During hyperemia accelerated oxidation leads to excessive NADH depletion. These processes lead to different balances of NADH/NAD+. Therefore, the NADH/NAD+ couple can be treated as a sensitive marker of changes in both mitochondrial function and vascular perturbations responsible for the transport of oxygen and other nutrients to cells. Thus, measuring NADH fluorescence should enable assessment of both vascular circulation and metabolic regulation.
As the name of the method itself suggests, the FMSF technique measures NADH fluorescence from the skin, mainly on the forearm. The fluorescence of NADH is the strongest component in the overall fluorescence emitted from human skin and the skin is the most easily accessible measurement site. The AngioExpert, a device constructed by Angionica Ltd., measures NADH fluorescence (460 nm) excited by a 340 nm ultraviolet light at a sampling frequency of 25 Hz (Katarzynska et al., 2019b). The methodological aspects of the method (the source of the signal - NADH, reproducibility of measurements) were investigated (Bugaj et al., 2019; Hellmann et al., 2017; Tarnawska et al., 2018). The penetration of exciting light in skin tissue is low (about 0.3–0.5 mm) and a substantial fraction is absorbed by the epidermis and papillary dermis (Cicchi et al., 2013). The NADH signal is not disturbed by the presence of blood vessels (Hou et al., 2022).
Mayevsky’s pioneering work first drew attention to the potential of using NADH fluorescence to determine mitochondrial function in vivo (Mayevsky and Barbiro-Michaely, 2009; Mayevsky and Rogatsky, 2007). NADH fluorescence and mitochondrial dynamics have also been used to study the metabolism of keratinocytes in human skin (Balu et al., 2013). Although the keratinocytes have lower mitochondrial density than other cells, the mechanisms of NADH accumulation during ischemia and of NADH oxidation during hyperemia may reflect the general mechanism of mitochondria functioning in the cells of other organs (Pawlak-Chomicka et al., 2023; Ronquist et al., 2003; Waddell et al., 2023). Numerous other studies have shown that the skin microcirculation reflects the systemic microcirculation, its dysfunction, and pathologies (Cracowski and Roustit, 2020). This makes skin cells a sensitive marker of early disorders of vascular circulation, as it is possible to test microvascular blood circulation via changes in skin biochemistry–especially the mitochondrial NADH redox state (as an internal marker) of epidermal cells, which depends on blood circulation and is sensitive to its changes. This approach should allow the monitoring vascular dysfunction, assuming proper mitochondrial functioning. It should also enable monitoring of mitochondrial dysfunction, assuming proper functioning of the circulatory system. Obviously, there may be some overlap in the perturbations related to these factors.
To date, most studies on the FMSF technique have concentrated on the assessment of vascular circulation (Gebicki et al., 2021; Katarzynska et al., 2022; Marcinek et al., 2024a; Marcinek et al., 2024b; Mikosiński et al., 2023). The FMSF method was compared with other microcirculation assessment techniques using PORH, like, for example laser Doppler flowmetry (LDF), laser speckle contrast imaging (LSCI), flow mediated dilation (FMD), reactive hyperemia peripheral arterial tonometry (RH-PAT) (Liu et al., 2024; Szczepanek et al., 2024).
In this paper we focus on the application of the FMSF technique in assessing mitochondrial function. We provide examples illustrating vascular and mitochondrial dysfunctions in the distinct phases of FMSF measurement, including baseline collection, ischemia, and hyperemia with reperfusion.
The analysis involved two patient groups: one with vascular diseases (CVD) and the other with type 2 diabetes (DM2), as described in previous publications (Katarzynska et al., 2020; Mikosiński et al., 2023). Brief clinical characteristics of the analyzed groups are presented in the Supplementary Material.
During the initial stage of FMSF measurements, the level of NADH fluorescence is determined.
The level of basal fluorescence (FLbase) is expected to be related to the overall NADH/NAD+ redox balance, providing at minimum insight into whether this balance in specific diseases is shifted towards reduction (increase in NADH fluorescence) or oxidation (decrease in NADH fluorescence). The validity of this approach can be confirmed by comparing different patient groups. For example, in the group of patients with DM2, the basal fluorescence was shifted towards reduction (increase of NADH fluorescence) compared to the group of patients with CVD (Table 1) (see also: Wu et al., 2016; Yan, 2021). In both patient groups, women showed higher basal fluorescence, although the difference was not always statistically significant (Supplementary Table S1). Significantly higher levels of NADH fluorescence have been observed for patients with mitochondrial disease and m.3243A>G mutation confirmed in genetic testing (van Kraaij et al., 2023). Other examples also seem to confirm this differentiation–e.g. in the case of patients with psoriasis the NADH fluorescence level in psoriatic lesions was significantly reduced (decreased fluorescence) (Gebicki et al., 2023a). In contrast, it has been shown in the case of competitive athletes strenuous physical exertion (exertion to exhaustion) can significantly shift the NADH level towards reduction (increased fluorescence) (Bugaj et al., 2019; Bugaj et al., 2020).
Table 1. Analysis of the FMSF parameters for CVD and DM2 groups. Results are shown as mean ± standard deviation (SD) with corresponding p-values indicating only statistically significant differences (p < 0.05). Reproduced from Mikosiński et al. (2023), Katarzynska et al. (2020).
Unfortunately, the level of baseline fluorescence varies widely among homogeneous groups of patients, depending on skin pigmentation, suntan, and skin lesions. This introduces a significant error when the NADH/NAD+ balance and mitochondrial function are interpreted based solely on NADH fluorescence. Additional calibration methods are therefore required, both for the measurement method and for the patient’s skin type and condition.
The FMSF signal is collected for 3 min and normalized with respect to the mean fluorescence value. Normalization of the signal makes the result independent of measurement conditions related to the individual characteristics of the patient’s skin and/or different technical factors, as only relative changes are analyzed.
The normalized fluorescence signal at the baseline, which characterizes the resting flow, is collected using the FMSF method over a period of 3 min. There are no strict time constraints, other than the patient’s comfort during the measurement, which requires immobility. The signal typically shows slight intensity changes, resulting from microcirculation oscillations–also called flowmotion (Aalkjær et al., 2011; Bernjak et al., 2008; Gebicki et al., 2020; Marcinek et al., 2023; Nilsson and Aalkjaer, 2003). The parameter FM (flowmotion), which can be a simple measure of these oscillations, is based on assessment of oscillations in terms of the mean square deviations of the experimental signal (at a sampling frequency of 25 Hz) from the baseline. Whereas the FM parameter varies within quite a broad range, log (FM) remains normally distributed.
The strength of oscillations contained in the FMSF signal can be determined using the Fast Fourier Transform (FFT) algorithm. The oscillations are grouped into three frequency intervals, corresponding to endothelial, neurogenic, and myogenic activities, respectively: ≤0.021 Hz, (0.021–0.052 Hz), and (0.052–0.15 Hz) (Bernjak et al., 2008; Gebicki et al., 2020; Marcinek et al., 2023). Comparison of FM parameters allows for the assessment of microcirculation functioning in different patients (Gebicki et al., 2020). A significant reduction in the FM parameter indicates microvascular dysfunction. Analysis of the components of the oscillation and their interrelationships allows for the diagnosis of conditions including extreme fatigue in athletes and post-COVID fatigue (with statistically significant short-term or long-term reductions in endothelial oscillations, respectively) (Chudzik et al., 2022). Relative reductions or increases of myogenic oscillations can be used to assess stress of various origins (Gebicki et al., 2023b; Marcinek et al., 2023).
In the second stage of measurements, known as the ischemic response (IR), a change in NADH fluorescence is observed due to occlusion of the brachial artery by a cuff inflated to 60 mmHg above the systolic blood pressure of the patient.
Since blood supply is completely blocked at this stage, the fluorescence signal does not have the characteristics of blood flow (the signal remains completely smooth, there are no visible microcirculation oscillations, including in the cardiac component). The ischemic response can be entirely attributed to a change in cellular oxygen metabolism from an aerobic process to anaerobic processes (including glycolysis). Any disturbances in the ischemic part can be attributed to mitochondrial dysfunction. The use of FMSF-PORH method to assess mitochondrial functioning seems to be a perspective approach, due to the fact that most methods using PORH remain “blind” during the occlusion period.
The typical kinetics of ischemic changes, which are particularly characteristic for volunteers with no diagnosed health problems, is presented in Figure 1A. Such signal waveforms are also observed for some patients with diagnosed diseases, including diseases associated with mitochondrial dysfunction. After approximately 3 min or less, the signal stabilizes. The IRmax parameter, which is a measure of the ischemic response, corresponds to the maximum change in NADH fluorescence occurring at this stage (usually after 3 min of occlusion). This value typically reaches several percent relative to the baseline fluorescence, although in some cases it can reach over 20 percent. In patients suffering from various diseases, it typically reaches much lower values (Table 1).
Figure 1. Exemplary FMSF traces showing different levels of mitochondrial function: (A) adequate mitochondrial function (female, 64 y.); (B) slightly impaired mitochondrial function (female, 77 y., diabetes, hypertension, cardiovascular disease); (C) impaired mitochondrial function (female, 69 y. diabetes, hypertension, cardiovascular disease); (D) Significantly impaired mitochondrial function (female, 65 y. hypercholesterolemia, hypertension, cardiovascular disease, heart failure).
However, deviations from a typical adequate ischemic response are often observed in this range. In some cases, the change in NADH fluorescence is weaker (Figure 1B), or it slightly deviates from the baseline before occlusion indicating impaired ischemic response (Figure 1C). However, sometimes the fluorescence signal, and therefore the concentration of the reduced NADH form, drops by several percent, almost as a mirror image of the typical signal course. In such cases, we can speak of a negative, abnormal response to occlusion (significantly impaired ischemic response) (see Figure 1D). Additionally, changes in the shape of the signal course are often observed, as well as some irregularities. The course remains characteristic for a given patient (Mikosiński et al., 2023; Pawlak-Chomicka et al., 2023).
Although the mechanisms of atypical ischemic responses–such as unexplained changes in the transition from oxidative phosphorylation to anaerobic glycolysis, changes in the share of mitochondrial and cytoplasmic NADH in fluorescence, and/or changes in the mitochondrial lactate metabolism (Glancy et al., 2021) –remain unclear, atypical FMSF signal patterns may be the result of impaired metabolic processes associated with the loss of or altered mitochondrial functionality (Pawlak-Chomicka et al., 2023). These changes cannot be assigned to changes in blood flow, including microvascular circulation, as there is no flow at all.
In the analyzed patient groups, atypical courses were quite numerous (about 50%). At the same time, no changes were observed in the FM parameter, which confirms the lack of significant differences in vascular microcirculation between the groups with typical and atypical ischemic responses (Table 1). Atypical courses have also been reported to occur in large numbers among patients with Systemic Lupus Erythematosus (Bogaczewicz et al., 2019), as indicated by the range of observed changes in IRmax, as well as among patients with diabetic foot ulcers with low prognosis of healing (Los-Stegienta et al., 2021).
It is worth emphasizing that the same factors which in the short term reduce the level of ischemic response, such as strenuous physical exertion, also cause increases in the level of basal fluorescence (Bugaj et al., 2019), indicating a decrease in mitochondrial efficiency as a result of strenuous exertion. On the other hand, intake of inorganic NO3− or NO3−-rich products, such as beetroot juice, through the increase of NO in vivo, transiently elevates the level of ischemic response IRmax (approx. 30%), while decreasing the level of basal fluorescence (Jurga et al., 2024). This allows us to assign both these effects to the mitochondrial reaction. It has recently been demonstrated that dietary nitrate significantly improves mitochondrial efficiency by improving oxidative phosphorylation efficiency, which reduces oxygen cost during exercise and maximal ATP production (Larsen et al., 2011).
The ischemic response parameter IRmax can therefore provide a direct measure of mitochondrial efficiency or dysfunction.
After occlusion, the cuff pressure is released and the NADH fluorescence falls below the baseline, reaching a minimum followed by a return to the baseline. This third measurement stage, called the hyperemic response (HR), consists of a very rapid decrease in NADH fluorescence due to hyperemia (20–30 s), followed by a slow return of NADH fluorescence to the baseline due to reperfusion (approximately 3 min).
The most important parameter characterizing this stage is HRmax, which is a measure of the maximum decrease in NADH fluorescence after the release of flow in the brachial artery. This decrease occurs as oxygen flows rapidly into the epidermal cells, relative to the resting flow, due to the release of NO and the dilation of the brachial artery. This process can be correlated with the results obtained from FMD or LSCI and mainly concerns the macrocirculation (Gori et al., 2008; Liu et al., 2024; Thijssen et al., 2011). The diagnostic potential of the HRmax parameter includes assessment of dysfunction of the macrocirculation, which facilitates assessment of the risk of developing cardiovascular diseases often comorbid with other diseases, such as diabetes mellitus (Hellmann et al., 2017; Katarzynska et al., 2020; Katarzynska et al., 2019a; Tarnawska et al., 2018).
In the case of atypical signal courses with a negative ischemic response (IRmax < 0), the HRmax parameter cannot be so easily interpreted, because the very rapid hyperemic decrease in NADH fluorescence after the release of arterial flow is perturbed by the changes caused in the NADH/NAD+ redox balance by the negative ischemic response. Nevertheless, the slow return of the NADH fluorescence signal to the pre-occlusion baseline level with almost identical kinetics to typical FMSF signals allows for the interpretation of HRmax to assess the risk of developing cardiovascular diseases.
A characteristic feature of FMSF patterns, which appears in most cases at this stage, are clearly visible, strong oscillations of the microcirculation on the reperfusion line. The altered strength and frequency of oscillations (in particular myogenic oscillations (0.052–0.15 Hz)) reflects the reaction of the vascular microcirculation to transient ischemia. The intensity of these myogenic oscillations is called hypoxia sensitivity (HS), because hypoxia is responsible for the increased activity of the vessels after post-occlusive reactive hyperemia. Whereas the HS parameter varies within a very broad range, log (HS) remains normally distributed. The HS parameter, similarly to efficient stabilization of HIF-1α in microvascular smooth muscle cells during transient hypoxia, reflects the microcirculatory response to hypoxia. It allows for assessment of microcirculatory dysfunction in diabetes, cardiovascular disease, peripheral arterial disease, and hypertension, as well as assessment of exercise tolerance (Marcinek et al., 2023).
In patients with impaired ischemic response, a decrease in HS may additionally confirm mitochondrial dysfunction. Growing evidence from recent studies indicates a close connection between hypoxia inducible factor (HIF-1α) stabilization and mitochondria. HIF-1α regulates mitochondrial respiration and oxidative stress. On the other hand, there is evidence supporting mitochondria-triggered HIF-1α activation under various conditions, including hypoxia (Fuhrmann and Brüne, 2017; Huang et al., 2023; Jewell et al., 2001; Kierans and Taylor, 2021; Li et al., 2019; Mialet-Perez and Belaidi, 2024).
It is therefore unsurprising that severe redox imbalance, which is a significant problem occurring in diabetes and its complications, leads to impaired ischemic response (IRmax) and a lower response to hypoxia, as measured by the HS parameter. The statistically significant reduction in the HS parameter observed in the case of patients with diabetic kidney disease may accompany mitochondrial dysfunction (Los-Stegienta et al., 2022). Low values for the HS parameter were also associated with poor prognosis for healing in difficult-to-heal wounds (including diabetic foot ulcers) (Los-Stegienta et al., 2021). In long COVID patients treated for 3 months with angiotensin-converting enzyme inhibitors and beta-adrenolytics, a statistically significant increase in the HS parameter was observed, with a simultaneous and also statistically significant improvement in the ischemic response IRmax (Romanowska-Kocejko et al., 2025).
The correlation between the HS and IRmax parameters is more complicated, however. In healthy individuals, a short-term decrease in the IRmax (intense exertion) or an increase in the IRmax (a single dietary nitrate uptake) is associated with increases or decreases in the intensity of myogenic oscillations, respectively. The microcirculation compensates for changes in the NADH/NAD+ balance associated with hypoxia or hyperoxygenation of epidermal cells. Finally, in the case of serious microcirculation disorders, the HS parameter remains very low and does not respond at all to hypoxic conditions causing NADH/NAD+ imbalance.
In conclusion, by interpreting the parameters and the dynamics of the NADH fluorescence signal emitted from skin cells in response to ischemia and subsequent hyperemia, it is possible to identify vascular and mitochondrial dysfunctions that accompany or can lead to the development of chronic diseases. In the FMSF method, decreasing values of flowmotion (FM), hyperemia response (HRmax), and hypoxia sensitivity (HS) parameters enable the identification of vascular circulation disorders. Decreasing values of ischemia response (IRmax) and hypoxia sensitivity (HS) parameters, with simultaneous increases in baseline fluorescence (FLbase), indicate mitochondrial impairment. All the above parameters are weakly negatively correlated with age (Supplementary Table S2), except for baseline fluorescence FLbase which increases with age. Differences are also observed between women and men. FLbase is higher in women, IRmax is lower, and HRmax is also higher. The higher FLbase and lower IRmax values indicate greater susceptibility to mitochondrial dysfunction, while the higher HRmax indicates better macrocirculation in women.
Monitoring mitochondrial impairment based on NADH/NAD+ balance cannot be performed without simultaneous monitoring of vascular circulation. Dysfunction of one of these factors affects the other. The Flow Mediated Skin Fluorescence method, which uses various parameters to assess vascular circulation and mitochondrial function, allows us to study the feedback between mitochondrial and vascular regulation.
The original contributions presented in the study are included in the article/Supplementary Material, further inquiries can be directed to the corresponding authors.
The presented perspective is based on previously published research, cited in our work, conducted in accordance with ethical requirements. The studies were conducted in accordance with the local legislation and institutional requirements.
AM: Conceptualization, Writing–original draft, Writing–review and editing. JK: Methodology, Writing–review and editing. JG: Supervision, Writing–review and editing.
The author(s) declare that financial support was received for the research, authorship, and/or publication of this article. This work was supported by the European Union from the resources of the European Regional Development Fund under the Smart Growth Operational Program, Grant No. POIR 01.01.01-00-0540/15-00.
JG and AM are inventors of the patents protecting the use of FMSF technology. AM, JK and JG are employed by Angionica Ltd.
The author(s) declare that no Generative AI was used in the creation of this manuscript.
All claims expressed in this article are solely those of the authors and do not necessarily represent those of their affiliated organizations, or those of the publisher, the editors and the reviewers. Any product that may be evaluated in this article, or claim that may be made by its manufacturer, is not guaranteed or endorsed by the publisher.
The Supplementary Material for this article can be found online at: https://www.frontiersin.org/articles/10.3389/fphys.2025.1509159/full#supplementary-material
Aalkjær C., Boedtkjer D., Matchkov V. (2011). Vasomotion – what is currently thought? Acta Physiol. (Oxf). 202, 253–269. doi:10.1111/j.1748-1716.2011.02320.x
Balu M., Mazhar A., Hayakawa C. K., Mittal R., Krasieva T. B., König K., et al. (2013). In vivo multiphoton NADH fluorescence reveals depth-dependent keratinocyte metabolism in human skin. Biophys. J. 104, 258–267. doi:10.1016/j.bpj.2012.11.3809
Bernjak A., Clarkson P. B. M., McClintock P. V. E., Stefanovska A. (2008). Low-frequency blood flow oscillations in congestive heart failure and after beta1-blockade treatment. Microvasc. Res. 76, 224–232. doi:10.1016/j.mvr.2008.07.006
Bogaczewicz J., Tokarska K., Wozniacka A. (2019). Changes of NADH fluorescence from the skin of patients with systemic Lupus Erythematosus. Biomed. Res. Int. 2019, 5897487. doi:10.1155/2019/5897487
Bugaj O., Kusy K., Kantanista A., Korman P., Wieliński D., Zieliński J. (2020). The effect of a 7-week training period on changes in skin NADH fluorescence in highly trained athletes. Appl. Sci. 10, 5133. doi:10.3390/app10155133
Bugaj O., Zieliński J., Kusy K., Kantanista A., Wieliński D., Guzik P. (2019). The effect of exercise on the skin content of the reduced form of NAD and its response to transient ischemia and reperfusion in highly trained athletes. Front. Physiol. 10, 600. doi:10.3389/fphys.2019.00600
Chudzik M., Cender A., Mordaka R., Zieliński J., Katarzynska J., Marcinek A., et al. (2022). Chronic fatigue associated with post-COVID syndrome versus transient fatigue caused by high-intensity exercise: are they comparable in terms of vascular effects? Vasc. Health Risk Manag. 18, 711–719. doi:10.2147/VHRM.S371468
Cicchi R., Vogler N., Kapsokalyvas D., Dietzek B., Popp J., Pavone F. S. (2013). From molecular structure to tissue architecture: collagen organization probed by SHG microscopy. J. Biophot. 6, 129–142. doi:10.1002/jbio.201200092
Cracowski J.-L., Roustit M. (2020). “Human skin microcirculation,” in Comprehensive Physiology. Editor Y. S. Prakash, 1105–1154. doi:10.1002/cphy.c190008
Fuhrmann D. C., Brüne B. (2017). Mitochondrial composition and function under the control of hypoxia. Redox Biol. 12, 208–215. doi:10.1016/j.redox.2017.02.012
Gebicki J., Filipiak T., Marcinek A., Wozniacka A. (2023a). Assessment of NADH/NAD+ redox imbalance in psoriatic lesions using the FMSF technique: therapeutic aspects. Sensors 23, 8718. doi:10.3390/s23218718
Gebicki J., Katarzynska J., Cholewinski T., Sieron L., Marcinek A. (2020). Flowmotion monitored by flow mediated skin fluorescence (FMSF): a tool for characterization of microcirculatory status. Front. Physiol. 11, 702. doi:10.3389/FPHYS.2020.00702
Gebicki J., Katarzynska J., Marcinek A. (2023b). Effect of psychological stress on microcirculation oscillations: diagnostic aspects. Vasc. Health Risk Manag. 19, 79–82. doi:10.2147/VHRM.S399082
Gebicki J., Marcinek A., Zielinski J. (2021). Assessment of microcirculatory status based on stimulation of myogenic oscillations by transient ischemia: from health to disease. Vasc. Health Risk Manag. 17, 33–36. doi:10.2147/VHRM.S292087
Gebicki J., Marcinek A., Zielonka J. (2004). Transient species in the stepwise interconversion of NADH and NAD+. Acc. Chem. Res. 37, 379–386. doi:10.1021/ar030171j
Glancy B., Kane D. A., Kavazis A. N., Goodwin M. L., Willis W. T., Gladden B. G. (2021). Mitochondrial lactate metabolism: history and implications for exercise and disease. J. Physiol. 599, 863–888. doi:10.1113/JP278930
Gori T., Dragoni S., Lisi M., Di Stolfo G., Sonnati S., Fineschi M., et al. (2008). Conduit artery constriction mediated by low flow. A novel noninvasive method for the assessment of vascular function. J. Am. Coll. Cardiol. 51, 1953–1958. doi:10.1016/j.jacc.2008.01.049
Hellmann M., Tarnawska M., Dudziak M., Dorniak K., Roustit M., Cracowski J.-L. (2017). Reproducibility of flow mediated skin fluorescence to assess microvascular function. Microvasc. Res. 113, 60–64. doi:10.1016/j.mvr.2017.05.004
Hou H., Du G., Wang Y., Su C., Guo L., Chen X. (2022). Noninvasive in vivo study of NADH fluorescence and its real-time intrinsic dynamical changes: experiments and seven-layered skin model Monte Carlo simulations. J. Innov. Opt. Health Sci. 15, 2230006. doi:10.1142/S1793545822300063
Huang X., Zhao L., Peng R. (2023). Hypoxia-inducible factor 1 and mitochondria: an intimate connection. Biomolecules 13, 50. doi:10.3390/biom13010050
Jewell U. R., Kvietikova I., Scheid A., Bauer C., Wenger R. H., Gassmann M. (2001). Induction of HIF–1α in response to hypoxia is instantaneous. FASEB J. 15, 1312–1314. doi:10.1096/fj.00-0732fje
Jurga J., Samborowska E., Zielinski J., Olek R. A. (2024). Effects of acute beetroot juice and sodium nitrate on selected blood metabolites and response to transient ischemia: a crossover randomized clinical trial. J. Nutr. 154, 491–497. doi:10.1016/j.tjnut.2023.12.018
Katarzynska J., Borkowska A., Czajkowski P., Los A., Szczerbiński L., Milewska-Kranc A., et al. (2019a). Flow Mediated Skin Fluorescence technique reveals remarkable effect of age on microcirculation and metabolic regulation in type 1 diabetes. Microvasc. Res. 124, 19–24. doi:10.1016/j.mvr.2019.02.005
Katarzynska J., Borkowska A., Los A., Marcinek A., Cypryk K., Gebicki J. (2020). Flow-mediated skin fluorescence (FMSF) technique for studying vascular complications in type 2 diabetes. J. Diabetes Sci. Technol. 14, 693–694. doi:10.1177/1932296819895544
Katarzynska J., Lipinski Z., Cholewinski T., Piotrowski L., Dworzynski W., Urbaniak M., et al. (2019b). Non-invasive evaluation of microcirculation and metabolic regulation using flow mediated skin fluorescence (FMSF): technical aspects and methodology. Rev. Sci. Instrum. 90, 104104. doi:10.1063/1.5092218
Katarzynska J., Zielinski J., Marcinek A., Gebicki J. (2022). New approach to non-invasive assessment of vascular circulation based on the response to transient ischemia. Vasc. Health Risk Manag. 18, 113–116. doi:10.2147/VHRM.S358983
Kierans S. J., Taylor C. T. (2021). Regulation of glycolysis by the hypoxia-inducible factor (HIF): implications for cellular physiology. J. Physiol. 599, 23–37. doi:10.1113/JP280572
Larsen F. J., Schiffer T. A., Borniquel S., Sahlin K., Ekblom B., Lundberg J. O., et al. (2011). Dietary inorganic nitrate improves mitochondrial efficiency in humans. Cell Metab. 13, 149–159. doi:10.1016/j.cmet.2011.01.004
Li H.-S., Zhou Y.-N., Li L., Li S.-F., Long D., Chen X.-L., et al. (2019). HIF-1α protects against oxidative stress by directly targeting mitochondria. Redox Biol. 25, 101109. doi:10.1016/j.redox.2019.101109
Liu P., Bi T., Du G., Yan L., Hou H. (2024). Dynamic non-invasive detection of NADH based on blood flow-mediated skin fluorescence (FMSF) method. Open J. Appl. Sci. 14, 1437–1453. doi:10.4236/ojapps.2024.146095
Los-Stegienta A., Borkowska A., Cypryk K. (2022). Assessment of microvascular function using a novel technique Flow Mediated Skin Fluorescence (FMSF) in patients with diabetic kidney disease: a preliminary study. Microvasc. Res. 144, 104417. doi:10.1016/j.mvr.2022.104417
Los-Stegienta A., Katarzynska J., Borkowska A., Marcinek A., Cypryk K., Gebicki J. (2021). Differentiation of diabetic foot ulcers based on stimulation of myogenic oscillations by transient ischemia. Vasc. Health Risk Manag. 17, 145–152. doi:10.2147/VHRM.S307366
Marcinek A., Katarzynska J., Cypryk K., Los-Stegienta A., Slowikowska-Hilczer J., Walczak-Jedrzejowska R., et al. (2024b). Assessment of microvascular function based on flowmotion monitored by the flow-mediated skin fluorescence technique. Biosensors 14, 459. doi:10.3390/bios14100459
Marcinek A., Katarzynska J., Gebicki J. (2024a). A new approach to vascular screening: identification of impaired vascular function using the FMSF technique. Sensors 24, 1721. doi:10.3390/s24061721
Marcinek A., Katarzynska J., Sieron L., Skokowski R., Zieliński J., Gebicki J. (2023). Non-invasive assessment of vascular circulation based on flow mediated skin fluorescence (FMSF). Biology 12, 385–1271. doi:10.3390/biology12030385
Mayevsky A., Barbiro-Michaely E. (2009). Use of NADH fluorescence to determine mitochondrial function in vivo. Int. J. Biochem. Cell Biol. 41, 1977–1988. doi:10.1016/j.biocel.2009.03.012
Mayevsky A., Rogatsky G. G. (2007). Mitochondrial function in vivo evaluated by NADH fluorescence: from animal models to human studies. Am. J. Physiol. Cell Physiol 292, C615–C640. doi:10.1152/ajpcell.00249.2006
Mialet-Perez J., Belaidi E. (2024). Interplay between hypoxia inducible Factor-1 and mitochondria in cardiac diseases. Free Radic. Biol. Med. 221, 13–22. doi:10.1016/j.freeradbiomed.2024.04.239
Mikosiński J., Mikosiński P., Kwapisz A., Katarzynska J., Gebicki J. (2023). Conclusions from an observational study of patients with vascular diseases using the FMSF technique. Vasc. Health Risk Manag. 19, 755–764. doi:10.2147/VHRM.S442344
Nilsson H., Aalkjaer C. (2003). Vasomotion: mechanisms and physiological importance. Mol. Interv. 3, 79–89. doi:10.1124/mi.3.2.79
Pawlak-Chomicka R., Uruski P., Krauze T., Piskorski J., Tykarski A., Guzik P. (2023). Arterial blood pressure features of hypertensive patients with typical and atypical 460 nm skin fluorescence response to transient ischaemia. J. Clin. Med. 12, 5886. doi:10.3390/jcm12185886
Romanowska-Kocejko M., Braczko A., Jędrzejewska A., Zarczynska-Buchowiecka M., Kocejko T., Kutryb-Zajac B., et al. (2025). Follow-up assessment of the microvascular function in patients with long COVID. Microvasc. Res. 157, 104748. doi:10.1016/j.mvr.2024.104748
Ronquist G., Andersson A., Bendsoe N., Falck B. (2003). Human epidermal energy metabolism is functionally anaerobic. Exp. Dermatol. 12, 572–579. doi:10.1034/j.1600-0625.2003.00018.x
Roustit M., Cracowski J.-L. (2013). Assessment of endothelial and neurovascular function in human skin microcirculation. Trends Pharmacol. Sci. 34, 373–384. doi:10.1016/j.tips.2013.05.007
Szczepanek E., Marczyk B., Chukwu O., Chłopicki S., Sacha T. (2024). Endothelial function in patients with chronic myeloid leukemia treated with tyrosine kinase inhibitors is not related to cardiovascular risk assessed by the Systematic Coronary Risk Estimation 2 algorithm. Pol. Arch. Intern. Med. 134, 16719–9. doi:10.20452/pamw.16719
Tarnawska M., Dorniak K., Kaszubowski M., Dudziak M., Hellmann M. (2018). A pilot study with flow mediated skin fluorescence: a novel device to assess microvascular endothelial function in coronary artery disease. Cardiol. J. 25, 120–127. doi:10.5603/CJ.a2017.0096
Thijssen D. H. J., Black M. A., Pyke K. E., Padilla J., Atkinson G., Harris R. A., et al. (2011). Assessment of flow-mediated dilation in humans: a methodological and physiological guideline. Am. J. Physiol. Heart Circ. Physiol. 300, H2–H12. doi:10.1152/ajpheart.00471.2010
van Kraaij S. J. W., Pereira D. R., Smal B., Summo L., Konkel A., Lossie J., et al. (2023). Identification of peripheral vascular function measures and circulating biomarkers of mitochondrial function in patients with mitochondrial disease. Clin. Transl. Sci. 16, 1258–1271. doi:10.1111/cts.13530
Waddell J., Khatoon R., Kristian T. (2023). Cellular and mitochondrial NAD homeostasis in health and disease. Cells 12, 1329. doi:10.3390/cells12091329
Wu J., Jin Z., Zheng H., Yan L.-J. (2016). Sources and implications of NADH/NAD+ redox imbalance in diabetes and its complications. Diabetes Metab. Syndr. Obes. 9, 145–153. doi:10.2147/DMSO.S106087
Keywords: FMSF technique, NADH fluorescence, vascular circulation, mitochondrial dysfunction, microcirculatory oscillations
Citation: Marcinek A, Katarzynska J and Gebicki J (2025) Simultaneous assessment of mitochondrial and vascular function using the Flow Mediated Skin Fluorescence technique. Front. Physiol. 16:1509159. doi: 10.3389/fphys.2025.1509159
Received: 10 October 2024; Accepted: 03 February 2025;
Published: 19 February 2025.
Edited by:
Marc Germain, Université du Québec à Trois-Rivières, CanadaReviewed by:
Magdalena Agnieszka Zabielska-Kaczorowska, Medical Univeristy of Gdansk, PolandCopyright © 2025 Marcinek, Katarzynska and Gebicki. This is an open-access article distributed under the terms of the Creative Commons Attribution License (CC BY). The use, distribution or reproduction in other forums is permitted, provided the original author(s) and the copyright owner(s) are credited and that the original publication in this journal is cited, in accordance with accepted academic practice. No use, distribution or reproduction is permitted which does not comply with these terms.
*Correspondence: Andrzej Marcinek, YW5kcnplai5tYXJjaW5la0BwLmxvZHoucGw=, YW5kcnplai5tYXJjaW5la0Bhbmdpb25pY2EuY29tLnBs; Jerzy Gebicki, amVyenkuZ2ViaWNraUBwLmxvZHoucGw=, amVyenkuZ2ViaWNraUBhbmdpb25pY2EuY29tLnBs
Disclaimer: All claims expressed in this article are solely those of the authors and do not necessarily represent those of their affiliated organizations, or those of the publisher, the editors and the reviewers. Any product that may be evaluated in this article or claim that may be made by its manufacturer is not guaranteed or endorsed by the publisher.
Research integrity at Frontiers
Learn more about the work of our research integrity team to safeguard the quality of each article we publish.