- 1Department of Neurology, Danish Headache Center, Rigshospitalet Glostrup, Faculty of Health and Medical Sciences, University of Copenhagen, Copenhagen, Denmark
- 2Department of Neurology, Nordsjaellands Hospital-Hilleroed, Hilleroed, Denmark
Objective: To systematically review the involvement of KATP channel activation in pain modulation in preclinical studies.
Background: KATP channels are expressed at several levels in the spinal and trigeminal pain pathways, where they seem to modulate nociceptive transmission.
Methods: PubMed and Embase databases were searched until 29 January 2024, using the following search string: [(pain) OR (nociception) OR (antinociception) AND (KATP channel) OR (ATP sensitive potassium channel)]. Non-English and unavailable records, as well as records with non-experimental methodology, were excluded. Inclusion criteria were preclinical studies measuring pain in vivo upon activation of the KATP channel by administering a stimulator or positive modulator. Records were screened based on title and abstract, and those that met the study inclusion criteria were reviewed based on study design, measurements, intervention, and outcomes.
Results: The search resulted in 569 records. In total, 126 duplicates were detected. Subsequently, 438 records were screened by title and abstract, resulting in the exclusion of 396. Based on inclusion criteria, 42 studies were included. The main findings of the present systematic review were that KATP channel openers can attenuate induced pain in various animal models and potentiate the effects of analgesics.
Conclusion: Local, systemic, spinal, and supraspinal activation of KATP channels can attenuate pain and potentiate the efficacy of analgesic drugs. One exception was levcromakalim, as the systemic levcromakalim administration, but not a local application, induced pain. This finding is consistent with those of recent human trials. Future studies should investigate the differences in KATP channel activation between rodents and humans, as well as the differences in activation sites between levcromakalim and other KATP channel openers.
1 Introduction
A large series of preclinical investigations have identified adenosine triphosphate (ATP)-sensitive potassium (KATP) channels as potential targets for novel drugs to treat several pain disorders (Al-Karagholi, 2023). KATP channels contribute to chemosensory transduction and generation/propagation of action potentials in peripheral nociceptive afferents. These channels are octamer proteins consisting of four pore-forming, inwardly rectifying subunits (Kir family) and four sulfonylurea subunits (SUR family) (Cui et al., 2001; Wu et al., 2011). The Kir subunit identified in KATP channels is the Kir6 subunit, which is expressed in two isoforms, Kir6.1 and Kir6.2 (Al-Karagholi, 2023). The SUR subunit is expressed in three isoforms: SUR1, SUR2A and SUR2B. The subtypes exist in tissues in different combinations with different properties. Kir6.2/SUR1 are expressed in pancreatic beta cells, trigeminal ganglion, trigeminal nucleus caudalis and central neurons. Kir6.2/SUR2A are found in cardiac and skeletal muscle. Kir6.1/SUR2B and Kir6.2/SUR2B exist in smooth muscle (Al-Karagholi, 2023). Distinct subunit expression has been detected in various cells, including neurons, vascular smooth muscle, and pancreatic beta cells (Babenko et al., 1998). Elevated intracellular ATP reportedly inhibits KATP channels, leading to reduced potassium efflux and depolarization (Cui et al., 2001; Yamashita et al., 1994). However, the opening of KATP channels results in hyperpolarization and, thus, a decrease in the neuronal firing frequency (Babenko et al., 1998) and dilation of vascular smooth muscle cells (Christensen et al., 2022). In the current study, we systematically reviewed the implications of KATP channels in pain transmission following in vivo central or peripheral administration of KATP channel openers in preclinical studies.
2 Methods
A systematic search was performed according to the Preferred Reporting Items for Systematic Reviews and Meta-Analyses (PRISMA) reporting guidelines. PubMed and Embase databases were searched until 29th January 2024, to identify studies that investigated the involvement of KATP channels in pain transmission using the following search string: [(pain) OR (nociception) OR (antinociception) AND (KATP channel) OR (ATP-sensitive potassium channel)]. Non-English records and those that could not be retrieved were excluded from the study. In addition, records using non-experimental methods (reviews, conference abstracts, case reports, and meta-analyses) were excluded. The full text was assessed if the title and abstract did not provide all the necessary information. We included in vivo preclinical studies exploring pain mechanisms upon the administration of a stimulator or a direct activating modulator of KATP channels using pain measurement techniques (Figure 1). Data regarding authors, year of publication, study design, measurements, intervention, and outcomes were collected. For the study design, an overview of the experimental protocol was obtained. Pain was measured, and interventions were performed using KATP channel openers. Data on experiments and outcomes beyond the scope of this review were not evaluated. Data were independently extracted by two investigators (SSB and MMA). Discrepancies were resolved through discussion between the two investigators.
3 Results
In total, 569 records were retrieved following the database search, of which 126 were identified as duplicates. Two non-English and three unavailable records were excluded. The remaining 438 articles were screened based on their titles and abstracts. Of these, 396 studies were excluded for the following reasons: non-experimental methodology, clinical trials, only in vitro experiments, only knockout animals used, no activating modulator employed, no pain measurements performed, or missing data on administration routes (Figure 1). The remaining 42 studies were included in the final analysis. Several KATP channel openers (KCO), such as pinacidil, diazoxide, cromakalim, levcromakalim, and NN414, have been used to examine the role of KATP channels in pain transmission (Table 1). The findings of the included studies are summarized in Tables 2–9.
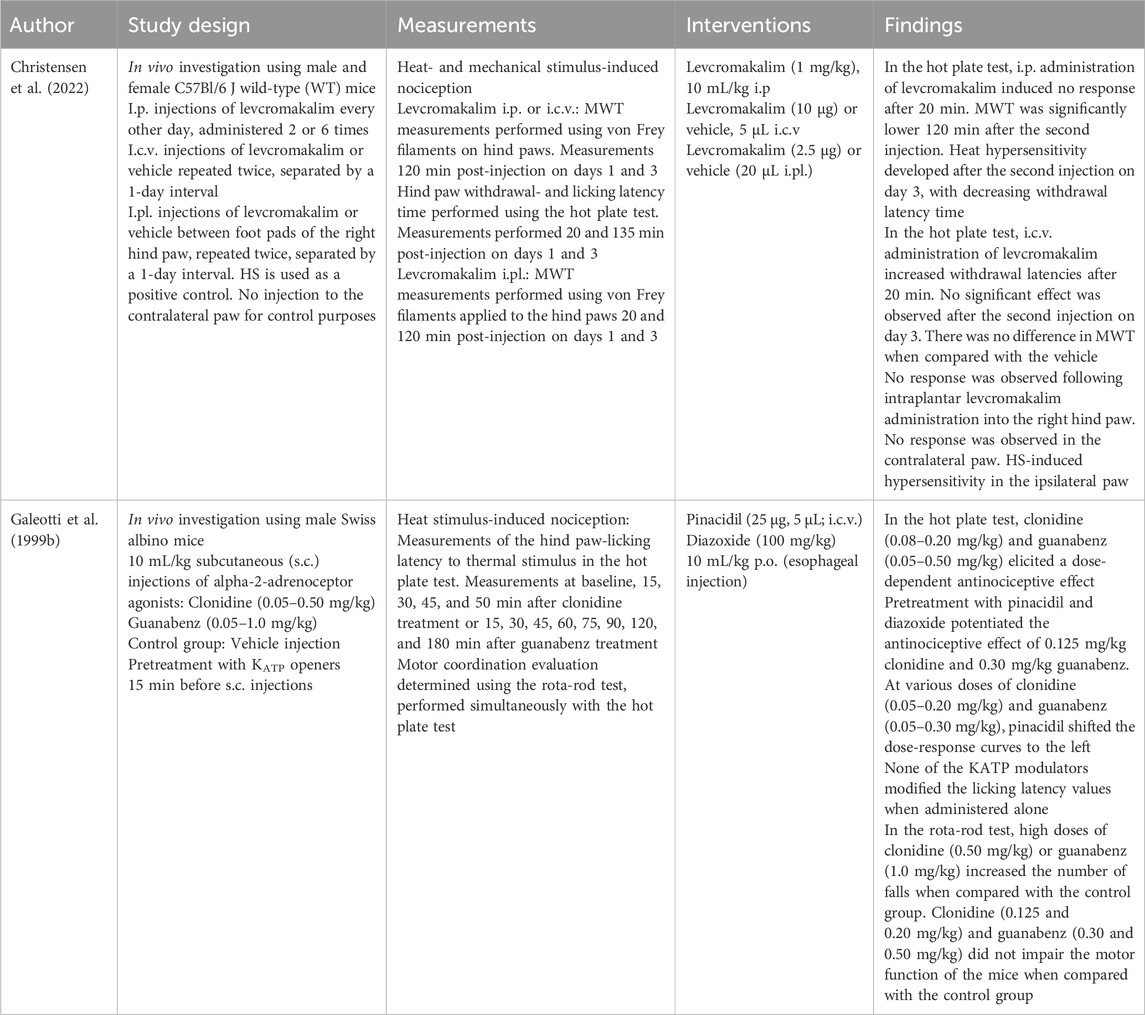
Table 8. Central intracerebroventricular, intraperitoneal, intraplantar, and peroral administration.
4 Discussion
The main findings of the present systematic review were that KCOs can attenuate induced pain in various animal models and potentiate the effects of analgesics. To present a clear overview of the results, we divided these findings based on the route of administration. Studies of KCOs administered to the central nervous system have been divided into central intrathecal (i.t.) and central intracerebroventricular (i.c.v.) administration.
4.1 Central i.t. administration
Pinacidil, diazoxide, cromakalim, levcromakalim, and NN414 have been tested against bone cancer pain (Xia et al., 2014), postoperative pain (Shen et al., 2015; Zhu et al., 2015; Qian et al., 2023; Cheng et al., 2006) neuropathic pain (Koh et al., 2016; Mixcoatl-Zecuatl et al., 2004; Mixcoatl-Zecuatl et al., 2006; Song et al., 2011; Luu et al., 2019) and peripheral nerve injury (Wu et al., 2011). In chronic pain models, KCO administration was found to attenuate pain sensation and increase nociceptive thresholds (Xia et al., 2014; Shen et al., 2015; Zhu et al., 2015; Qian et al., 2023; Cheng et al., 2006; Koh et al., 2016; Mixcoatl-Zecuatl et al., 2004; Mixcoatl-Zecuatl et al., 2006; Song et al., 2011; Luu et al., 2019). Pinacidil, diazoxide, and cromakalim did not modulate the baseline pain threshold in sham-operated or naïve animals (Wu et al., 2011; Xia et al., 2014). Induction of persistent pain decreased KATP channel activity and downregulated KATP channel expression in the spinal cord (Xia et al., 2014; Shen et al., 2015; Zhu et al., 2015). By acting on the remaining KATP channels, pinacidil attenuated the induced pain (Xia et al., 2014) and potentiated the analgesic effect of intraperitoneal (i.p.) administered nefopam (Mixcoatl-Zecuatl et al., 2006). Diazoxide reportedly potentiates the antiallodynic effects of N6-R-phenylisopropyladenosine (R-PIA) (Song et al., 2011). Interestingly, i.t. pinacidil administration elicited an antinociceptive effect in diabetic and non-diabetic mice (Zushida et al., 2002), while cromakalim was found to attenuate morphine tolerance (Cao et al., 2016a). These results indicate that the central activation of KATP channels exerts an antinociceptive effect. Conversely, Cheng et al. found that neither pinacidil nor diazoxide altered allodynia in a postoperative pain model (Cheng et al., 2006).
4.2 Central i.c.v. administration
Intracerebroventricular, administration of pinacidil potentiated the antinociceptive effect of several drugs administered subcutaneously (s.c.): tricyclic antidepressants (Galeotti et al., 2001), antihistamines (Galeotti et al., 1999a) and alpha-2-adrenoceptor agonists (Galeotti et al., 1999b). In the absence of any concurrently administered drugs, i.c.v. administration of pinacidil did not induce an antinociceptive effect.
Furthermore, i.c.v. administration of cromakalim potentiated the antinociceptive effect of s.c. administered mu-opioid receptor agonists morphine (Ocaña et al., 1995; Ocaña et al., 1996), buprenorphine, and methadone (Ocaña et al., 1996), but did not alter the antinociceptive effect of fentanyl or levorphanol (Ocaña et al., 1996). I.c.v. cromakalim also potentiated the subcutaneous effect of 5-HT1A receptor agonists (Robles et al., 1996), R-PIA (Ocaña and Baeyens, 1994) and clonidine (Ocaña et al., 1996). However, cromakalim did not alter the effects of s.c. administered U50,488H (a selective human kappa opioid receptor agonist) (Narita et al., 1993) and baclofen (Ocaña et al., 1996). Furthermore, cromakalim potentiated the effects of i.c.v. morphine, but not i.c.v. U50,488H (Narita et al., 1993). Collectively, these results suggest that KATP channel opening plays a role in mediating the analgesia of various types of drugs, with KATP channels functioning as downstream targets of receptor activation by these drugs. Considering cromakalim-induced analgesia when administered alone in naïve or control animals, the results were ambiguous. Some reported that cromakalim could induce an analgesic effect (Narita et al., 1993; Kamei et al., 1994; Sood et al., 2000), whereas others detected no such effect (Ocaña et al., 1995; Ocaña et al., 1996; Ocaña and Baeyens, 1994).
Three studies investigated the analgesic effect of i.c.v. pinacidil (Zushida et al., 2002) and i.c.v. cromakalim (Kamei et al., 1994; Sood et al., 2000) in diabetes models. Sood et al. (2000) reported that cromakalim induced substantial antinociception in diabetic mice, although to a lesser extent than that in non-diabetic mice. Moreover, cromakalim potentiated morphine-induced antinociception in the non-diabetic group but not in the diabetic group (Sood et al., 2000). This finding is in line with previous studies reporting that cromakalim can elicit a substantial antinociceptive effect in non-diabetic mice but not in diabetic mice, even after insulin treatment (Kamei et al., 1994). One potential explanation for this difference is the reduced activity or expression of supraspinal KATP channels in diabetes. Hyperglycemia and spleen-derived factors have been suggested to play a role in the reduced KATP channel activity (Kamei et al., 1994; Sood et al., 2000). The application of pinacidil provoked an antinociceptive effect in both diabetic and non-diabetic mice, with no marked differences in the potency ratio between the two groups (Zushida et al., 2002). I.c.v. administration of levcromakalim elicited an acute antinociceptive effect on heat-induced nociception without altering mechanical withdrawal thresholds (Christensen et al., 2022).
4.3 I.p. and peroral administration
Intraperitoneal administration of pinacidil could attenuate mechanical allodynia in postoperative pain models (Shen et al., 2015; Qian et al., 2016; Cao et al., 2015). I.p. diazoxide enhanced the antinociceptive effect of sildenafil against zymosan-induced nociception (zymosan is known to induce inflammatory pain) (Vale et al., 2007). Peroral (p.o.) administration of diazoxide potentiated the antinociceptive effect of s.c. administered alpha-2-adrenoceptor agonists clonidine and guanabenz but did not produce a notable effect when administered alone (Galeotti et al., 1999b). I.p. administration of levcromakalim (Christensen et al., 2022; Christensen et al., 2020) induced both thermal and mechanical hyperalgesia. These results differ from those of other KCOs and are further discussed below in relation to human trials.
4.4 Intramuscular (i.m.) and epidural administration
Intramuscular administration of pinacidil or diazoxide in the masseter muscle attenuated capsaicin-induced hypersensitivity (Niu et al., 2011). Pinacidil injection into the masseter muscle was shown to increase mechanical thresholds in trigeminal afferents (Cairns et al., 2008). These results suggest that KATP channels participate in the pain transmission of trigeminal afferent fibers and that the opening of these channels attenuates orofacial muscle pain.
Epidural co-administration of levcromakalim and morphine (Asano et al., 2000) potentiated the effect of morphine, although levcromakalim alone did not exert substantial antinociceptive effects (Asano et al., 2000). The observed potentiation may be due to the activation of mu-opioid receptors at the spinal cord level. Given that dissolving a higher dose of levcromakalim for epidural injection was not feasible, and with the dura mater functioning as a barrier, levcromakalim may fail to reach the spinal cord tissue (Asano et al., 2000). Notably, i.m. administration of the same dose of levcromakalim did not potentiate the effect of epidural morphine, indicating that the potentiation of morphine analgesia occurs at the spinal cord level (Asano et al., 2000).
4.5 Intraplantar administration
Pinacidil and diazoxide were co-applied to the paws with pain inducers, such as bradykinin (Du et al., 2011), formalin (Ghorbanzadeh et al., 2019; Ghorbanzadeh et al., 2018; Ortiz et al., 2002), λ-carrageenan, and prostaglandin E2 (PGE2) (Alves et al., 2004a; Alves et al., 2004b; Picolo et al., 2003; Sachs et al., 2004). Pinacidil and diazoxide were found to exert antinociceptive effects on pain induced by these agents and on heat- and mechanical-induced nociception. In formalin tests, pinacidil and diazoxide only influenced the late phases of the tests (Ghorbanzadeh et al., 2018; Ortiz et al., 2002). Notably, diazoxide potentiated the antinociceptive effect of carbamazepine against formalin-induced pain, with effects observed in both early and late phases (Ghorbanzadeh et al., 2019). Pinacidil applied to the dorsal root ganglion of the 5th lumbar nerve (L5) attenuated bradykinin-induced nociceptive behavior (Du et al., 2014). These results suggest that KATP channels are involved in peripheral antinociception of primary afferent neurons.
The effect of pinacidil on morphine tolerance was examined in a neuropathic pain model, revealing that pinacidil did not substantially impact morphine tolerance (Fisher et al., 2019). In contrast to pinacidil, diazoxide and NN414 attenuated morphine tolerance.
Diazoxide was examined in combination with analgesic drugs. In a model of PGE2-induced hyperalgesia, diazoxide reversed the antagonistic effect of NG-nitro L-arginine against diclofenac-induced antinociception (Alves et al., 2004a). Additionally, diazoxide potentiated the antinociceptive effect of fluoxetine against formalin-induced pain (Ghorbanzadeh et al., 2018). Combined with either dibutyrylguanosine cyclic monophosphate (db-cGMP) or sodium nitroprusside, diazoxide exerted marked antinociceptive effects against PGE2-induced pain (Alves et al., 2004b). Therefore, the analgesic properties of these drugs may be mediated via KATP channels. Injections of diclofenac, carbamazepine, or fluoxetine (Ghorbanzadeh et al., 2019; Ghorbanzadeh et al., 2018; Alves et al., 2004a), as well as KCOs (Du et al., 2011; Alves et al., 2004b), failed to elicit an antinociceptive effect when administered to contralateral paws in animals treated with pain inducers, indicating that the analgesic site of action is peripheral. Levcromakalim did not induce an analgesic effect (Christensen et al., 2022), which is discussed further below in relation to human trials.
4.6 Relation to human trials and future perspectives
Intraplantar administration of levcromakalim reportedly exerted no effect on mechanical withdrawal thresholds (Christensen et al., 2022), indicating that the hyperalgesic and allodynic effects of systemic administration (Christensen et al., 2022; Christensen et al., 2020) are not mediated via local activation of KATP channels in the paws. Hypersensitivity is likely to be mediated by vascular smooth muscle KATP channels (Christensen et al., 2022). These findings are consistent with trials conducted in healthy participants, where an intravenous infusion of levcromakalim reportedly induced headaches and dilated cephalic arteries (Al-Karagholi et al., 2019a); however, these effects were not observed when levcromakalim was administered intradermally or intramuscularly to the temporal muscle (Al-Karagholi et al., 2019b). Furthermore, all patients with migraine who received intravenous levcromakalim developed migraine attacks (Al-Karagholi et al., 2019c). These findings indicate that peripherally located KATP channels are unlikely to be sites of levcromakalim action. However, human data cannot be directly compared with rodent data because the measurement of pain is methodologically distinct, and interspecies differences in the molecular mechanisms underlying pain cannot be overlooked (Christensen et al., 2020). Data from human studies are inconsistent with the results of preclinical studies utilizing other KCOs; for example, systemic pinacidil was found to attenuate pain in a postoperative pain model in rodents (Shen et al., 2015; Qian et al., 2016; Cao et al., 2015), and diazoxide reportedly potentiates the antinociceptive effect of sildenafil (Vale et al., 2007) and alpha-2-adrenoceptor agonists (Galeotti et al., 1999b). Intraplantar (Du et al., 2011; Ghorbanzadeh et al., 2019; Ghorbanzadeh et al., 2018; Ortiz et al., 2002; Alves et al., 2004a; Alves et al., 2004b; Picolo et al., 2003; Sachs et al., 2004) or dorsal root ganglion (Du et al., 2014) administration of pinacidil and diazoxide attenuated locally induced pain in preclinical studies, in contrast to local administration of levcromakalim in humans (Al-Karagholi et al., 2019b) and animals (Christensen et al., 2022).
5 Conclusion
In preclinical studies, the opening of KATP channels attenuates induced pain and potentiates the effects of analgesic drugs at the spinal and supraspinal levels. The analgesic properties of cromakalim and the involvement of central KATP channel activity in pain modulation in diabetic populations warrant further investigation. Systemic and local administration of KCOs attenuates induced pain and potentiates the effects of analgesic drugs, except levcromakalim, which causes pain when administered systemically and has no effect on pain when administered locally. The effects of levcromakalim in preclinical investigations are consistent with the results observed in the human trials. Future studies should explore the differences in KATP channel activation between rodents and humans, as well as the differences in activation sites between levcromakalim and other KCOs.
Data availability statement
The original contributions presented in the study are included in the article/supplementary material, further inquiries can be directed to the corresponding author.
Author contributions
SB: Conceptualization, Data curation, Formal Analysis, Investigation, Methodology, Validation, Visualization, Writing–original draft, Writing–review and editing. LK: Validation, Writing–original draft, Writing–review and editing. MA-M A-K: Conceptualization, Formal Analysis, Investigation, Methodology, Project administration, Supervision, Validation, Writing–original draft, Writing–review and editing.
Funding
The author(s) declare that no financial support was received for the research, authorship, and/or publication of this article.
Conflict of interest
The authors declare that this study was conducted in the absence of any commercial or financial relationships that could be construed as potential conflicts of interest.
Publisher’s note
All claims expressed in this article are solely those of the authors and do not necessarily represent those of their affiliated organizations, or those of the publisher, the editors and the reviewers. Any product that may be evaluated in this article, or claim that may be made by its manufacturer, is not guaranteed or endorsed by the publisher.
Abbreviations
ATP, Adenosine triphosphate; BK, Bradykinin; CCI, Chronic constriction injury; DRG, Dorsal root ganglion; db-cGMP, Dibutyrylguanosine cyclic monophosphate; h, Hour; HS, Hypertonic saline; i.c.v., Intracerebroventricular; i.m., Intramuscular; i.p., Intraperitoneal; i.pl., Intraplantar; i.t., Intrathecal; i.v., Intravenous; KATP, Adenosine triphosphate-sensitive potassium channels; KCL, Potassium chloride; KCO, KATP channel openers; L5, Fifth lumbar nerve; Min, Minutes; MWT, Mechanical withdrawal threshold; NN414, Tifenazoxide; PGE2, Prostaglandin E2; p.o., Peroral; R-PIA, N6-R-phenylisopropyladenosine; s.c., Subcutaneous; SMIR, Skin/muscle incision and retraction; SNL, Spinal nerve ligation; STZ, Streptozotocin; WT, Wild type.
References
Al-Karagholi M. A. (2023). Involvement of potassium channel signalling in migraine pathophysiology. Pharm. (Basel) 16 (3), 438. doi:10.3390/ph16030438
Al-Karagholi M. A., Ghanizada H., Hansen J. M., Skovgaard L. T., Olesen J., Larsson H. B. W., et al. (2019a). Levcromakalim, an adenosine triphosphate-sensitive potassium channel opener, dilates extracerebral but not cerebral arteries. Headache 59 (9), 1468–1480. doi:10.1111/head.13634
Al-Karagholi M. A., Ghanizada H., Hansen J. M., Aghazadeh S., Skovgaard L. T., Olesen J., et al. (2019b). Extracranial activation of ATP-sensitive potassium channels induces vasodilation without nociceptive effects. Cephalalgia 39 (14), 1789–1797. doi:10.1177/0333102419888490
Al-Karagholi M. A., Hansen J. M., Guo S., Olesen J., Ashina M. (2019c). Opening of ATP-sensitive potassium channels causes migraine attacks: a new target for the treatment of migraine. Brain 142 (9), 2644–2654. doi:10.1093/brain/awz199
Alves D. P., Tatsuo M. A., Leite R., Duarte I. D. (2004a). Diclofenac-induced peripheral antinociception is associated with ATP-sensitive K+ channels activation. Life Sci. 74 (20), 2577–2591. doi:10.1016/j.lfs.2003.10.012
Alves D. P., Soares A. C., Francischi J. N., Castro M. S., Perez A. C., Duarte I. D. (2004b). Additive antinociceptive effect of the combination of diazoxide, an activator of ATP-sensitive K+ channels, and sodium nitroprusside and dibutyryl-cGMP. Eur. J. Pharmacol. 489 (1-2), 59–65. doi:10.1016/j.ejphar.2004.02.022
Asano T., Dohi S., Iida H. (2000). Antinociceptive action of epidural K+(ATP) channel openers via interaction with morphine and an alpha(2)- adrenergic agonist in rats. Anesth. Analg. 90 (5), 1146–1151. doi:10.1097/00000539-200005000-00027
Babenko A. P., Aguilar-Bryan L., Bryan J. (1998). A view of sur/kir6.x, katp channels. Annu. Rev. Physiology 60 (1), 667–687. doi:10.1146/annurev.physiol.60.1.667
Cairns B. E., Mann M. K., Mok E., Dong X. D., Svensson P. (2008). Diclofenac exerts local anesthetic-like actions on rat masseter muscle afferent fibers. Brain Res. 1194, 56–64. doi:10.1016/j.brainres.2007.11.060
Cao S., Liu Y., Wang H., Mao X., Chen J., Liu J., et al. (2016a). Ischemic postconditioning influences electron transport chain protein turnover in Langendorff-perfused rat hearts. PeerJ 4, e1706. doi:10.7717/peerj.1706
Cao Z., Dai W., Zhang R., Chen L., Yang X., Hu L., et al. (2016b). Opening of the adenosine triphosphate-sensitive potassium channel attenuates morphine tolerance by inhibiting JNK and astrocyte activation in the spinal cord. Clin. J. Pain 32 (7), 617–623. doi:10.1097/AJP.0000000000000299
Cao S., Qin Y., Chen J., Shen S. (2015). Effects of pinacidil on changes to the microenvironment around the incision site, of a skin/muscle incision and retraction, in a rat model of postoperative pain. Mol. Med. Rep. 12 (1), 829–836. doi:10.3892/mmr.2015.3465
Cheng J. K., Chen C. C., Yang J. R., Chiou L. C. (2006). The antiallodynic action target of intrathecal gabapentin: Ca2+ channels, KATP channels or N-methyl-d-aspartic acid receptors? Anesth. Analg. 102 (1), 182–187. doi:10.1213/01.ane.0000189550.97536.83
Christensen S. L., Munro G., Petersen S., Shabir A., Jansen-Olesen I., Kristensen D. M., et al. (2020). ATP sensitive potassium (K(ATP)) channel inhibition: a promising new drug target for migraine. Cephalalgia 40 (7), 650–664. doi:10.1177/0333102420925513
Christensen S. L., Rasmussen R. H., Cour S., Ernstsen C., Hansen T. F., Kogelman L. J., et al. (2022). Smooth muscle ATP-sensitive potassium channels mediate migraine-relevant hypersensitivity in mouse models. Cephalalgia 42 (2), 93–107. doi:10.1177/03331024211053570
Cui Y., Giblin J. P., Clapp L. H., Tinker A. (2001). A mechanism for ATP-sensitive potassium channel diversity: functional coassembly of two pore-forming subunits. Proc. Natl. Acad. Sci. U. S. A. 98 (2), 729–734. doi:10.1073/pnas.011370498
Du X., Hao H., Gigout S., Huang D., Yang Y., Li L., et al. (2014). Control of somatic membrane potential in nociceptive neurons and its implications for peripheral nociceptive transmission. Pain 155 (11), 2306–2322. doi:10.1016/j.pain.2014.08.025
Du X., Wang C., Zhang H. (2011). Activation of ATP-sensitive potassium channels antagonize nociceptive behavior and hyperexcitability of DRG neurons from rats. Mol. Pain 7, 35. doi:10.1186/1744-8069-7-35
Fisher C., Johnson K., Okerman T., Jurgenson T., Nickell A., Salo E., et al. (2019). Morphine efficacy, tolerance, and hypersensitivity are altered after modulation of SUR1 subtype K(ATP) channel activity in mice. Front. Neurosci. 13, 1122. doi:10.3389/fnins.2019.01122
Galeotti N., Ghelardini C., Bartolini A. (1999a). The role of potassium channels in antihistamine analgesia. Neuropharmacology 38 (12), 1893–1901. doi:10.1016/s0028-3908(99)00068-4
Galeotti N., Ghelardini C., Vinci M. C., Bartolini A. (1999b). Role of potassium channels in the antinociception induced by agonists of alpha2-adrenoceptors. Br. J. Pharmacol. 126 (5), 1214–1220. doi:10.1038/sj.bjp.0702395
Galeotti N., Ghelardini C., Bartolini A. (2001). Involvement of potassium channels in amitriptyline and clomipramine analgesia. Neuropharmacology 40 (1), 75–84. doi:10.1016/s0028-3908(00)00097-6
Ghorbanzadeh B., Kheirandish V., Mansouri M. T. (2019). Involvement of the L-arginine/nitric oxide/cyclic GMP/KATP channel pathway and PPARγ receptors in the peripheral antinociceptive effect of carbamazepine. Drug Res. (Stuttg). 69 (12), 650–657. doi:10.1055/a-0959-5896
Ghorbanzadeh B., Mansouri M. T., Naghizadeh B., Alboghobeish S. (2018). Local antinociceptive action of fluoxetine in the rat formalin assay: role of l-arginine/nitric oxide/cGMP/K(ATP) channel pathway. Can. J. Physiol. Pharmacol. 96 (2), 165–172. doi:10.1139/cjpp-2017-0003
Kamei J., Kawashima N., Narita M., Suzuki T., Misawa M., Kasuya Y. (1994). Reduction in ATP-sensitive potassium channel-mediated antinociception in diabetic mice. Psychopharmacol. Berl. 113 (3-4), 318–321. doi:10.1007/BF02245203
Koh W. U., Shin J. W., Bang J. Y., Kim S. G., Song J. G. (2016). The antiallodynic effects of nefopam are mediated by the adenosine triphosphate-sensitive potassium channel in a neuropathic pain model. Anesth. Analg. 123 (3), 762–770. doi:10.1213/ANE.0000000000001411
Luu W., Bjork J., Salo E., Entenmann N., Jurgenson T., Fisher C., et al. (2019). Modulation of SUR1 K(ATP) channel subunit activity in the peripheral nervous system reduces mechanical hyperalgesia after nerve injury in mice. Int. J. Mol. Sci. 20 (9), 2251. doi:10.3390/ijms20092251
Mannhold R. (2004). KATP channel openers: structure-activity relationships and therapeutic potential. Med. Res. Rev. 24 (2), 213–266. doi:10.1002/med.10060
Mixcoatl-Zecuatl T., Flores-Murrieta F. J., Granados-Soto V. (2006). The nitric oxide-cyclic GMP-protein kinase G-K+ channel pathway participates in the antiallodynic effect of spinal gabapentin. Eur. J. Pharmacol. 531 (1-3), 87–95. doi:10.1016/j.ejphar.2005.12.006
Mixcoatl-Zecuatl T., Medina-Santillán R., Reyes-García G., Vidal-Cantú G. C., Granados-Soto V. (2004). Effect of K+ channel modulators on the antiallodynic effect of gabapentin. Eur. J. Pharmacol. 484 (2-3), 201–208. doi:10.1016/j.ejphar.2003.11.022
Nakao K., Takahashi M., Kaneto H. (1996). Implication of ATP-sensitive K+ channels in various stress-induced analgesia (SIA) in mice. Jpn. J. Pharmacol. 71 (3), 269–272. doi:10.1254/jjp.71.269
Narita M., Takamori K., Kawashima N., Funada M., Kamei J., Suzuki T., et al. (1993). Activation of central ATP-sensitive potassium channels produces the antinociception and spinal noradrenaline turnover-enhancing effect in mice. Psychopharmacol. Berl. 113 (1), 11–14. doi:10.1007/BF02244326
Niu K., Saloman J. L., Zhang Y., Ro J. Y. (2011). Sex differences in the contribution of ATP-sensitive K+ channels in trigeminal ganglia under an acute muscle pain condition. Neuroscience 180, 344–352. doi:10.1016/j.neuroscience.2011.01.045
Ocaña M., Baeyens J. M. (1994). Role of ATP-sensitive K+ channels in antinociception induced by R-PIA, an adenosine A1 receptor agonist. Naunyn Schmiedeb. Arch. Pharmacol. 350 (1), 57–62. doi:10.1007/BF00180011
Ocaña M., Barrios M., Baeyens J. M. (1996). Cromakalim differentially enhances antinociception induced by agonists of alpha(2)adrenoceptors, gamma-aminobutyric acid(B), mu and kappa opioid receptors. J. Pharmacol. Exp. Ther. 276 (3), 1136–1142.
Ocaña M., Del Pozo E., Barrios M., Baeyens J. M. (1995). Subgroups among mu-opioid receptor agonists distinguished by ATP-sensitive K+ channel-acting drugs. Br. J. Pharmacol. 114 (6), 1296–1302. doi:10.1111/j.1476-5381.1995.tb13346.x
Ortiz M. I., Torres-López J. E., Castañeda-Hernández G., Rosas R., Vidal-Cantú G. C., Granados-Soto V. (2002). Pharmacological evidence for the activation of K(+) channels by diclofenac. Eur. J. Pharmacol. 438 (1-2), 85–91. doi:10.1016/s0014-2999(02)01288-8
Picolo G., Cassola A. C., Cury Y. (2003). Activation of peripheral ATP-sensitive K+ channels mediates the antinociceptive effect of Crotalus durissus terrificus snake venom. Eur. J. Pharmacol. 469 (1-3), 57–64. doi:10.1016/s0014-2999(03)01676-5
Qian C., Fan Y., Zong L., Miao C., Ji L. L., Wan L., et al. (2023). Opening K(ATP) channels induces inflammatory tolerance and prevents chronic pain. Brain Behav. Immun. 107, 76–86. doi:10.1016/j.bbi.2022.09.017
Qian L. P., Shen S. R., Chen J. J., Ji L. L., Cao S. (2016). Peripheral KATP activation inhibits pain sensitization induced by skin/muscle incision and retraction via the nuclear factor-κB/c-Jun N-terminal kinase signaling pathway. Mol. Med. Rep. 14 (3), 2632–2638. doi:10.3892/mmr.2016.5546
Robles L. I., Barrios M., Del Pozo E., Dordal A., Baeyens J. M. (1996). Effects of K+ channel blockers and openers on antinociception induced by agonists of 5-HT1A receptors. Eur. J. Pharmacol. 295 (2-3), 181–188. doi:10.1016/0014-2999(95)00643-5
Sachs D., Cunha F. Q., Ferreira S. H. (2004). Peripheral analgesic blockade of hypernociception: activation of arginine/NO/cGMP/protein kinase G/ATP-sensitive K+ channel pathway. Proc. Natl. Acad. Sci. U. S. A. 101 (10), 3680–3685. doi:10.1073/pnas.0308382101
Shen S., Cao S., Huang S., Chen J. (2015). Effect of adenosine triphosphate-sensitive potassium activation on peripheral and central pain sensitization. J. Surg. Res. 195 (2), 481–487. doi:10.1016/j.jss.2015.01.033
Song J. G., Hahm K. D., Kim Y. K., Leem J. G., Lee C., Jeong S. M., et al. (2011). Adenosine triphosphate-sensitive potassium channel blockers attenuate the antiallodynic effect of R-PIA in neuropathic rats. Anesth. Analg. 112 (6), 1494–1499. doi:10.1213/ANE.0b013e318212b833
Sood V., Sharma A., Singh M. (2000). Role of KATP channels in reduced antinociceptive effect of morphine in streptozotocin-induced diabetic mice. Indian J. Exp. Biol. 38 (5), 447–451.
Vale M. L., Rolim D. E., Cavalcante I. F., Ribeiro R. A., Souza M. H. (2007). Role of NO/cGMP/KATP pathway in antinociceptive effect of sildenafil in zymosan writhing response in mice. Inflamm. Res. 56 (2), 83–88. doi:10.1007/s00011-006-6109-8
Wu X. F., Liu W. T., Liu Y. P., Huang Z. J., Zhang Y. K., Song X. J. (2011). Reopening of ATP-sensitive potassium channels reduces neuropathic pain and regulates astroglial gap junctions in the rat spinal cord. Pain 152 (11), 2605–2615. doi:10.1016/j.pain.2011.08.003
Xia H., Zhang D., Yang S., Wang Y., Xu L., Wu J., et al. (2014). Role of ATP-sensitive potassium channels in modulating nociception in rat model of bone cancer pain. Brain Res. 1554, 29–35. doi:10.1016/j.brainres.2014.01.032
Yamashita S., Park J. B., Ryu P. D., Inukai H., Tanifuji M., Murase K. (1994). Possible presence of the ATP-sensitive K+ channel in isolated spinal dorsal horn neurons of the rat. Neurosci. Lett. 170 (2), 208–212. doi:10.1016/0304-3940(94)90320-4
Zhu X., Liu J., Gao Y., Cao S., Shen S. (2015). ATP-sensitive potassium channels alleviate postoperative pain through JNK-dependent MCP-1 expression in spinal cord. Int. J. Mol. Med. 35 (5), 1257–1265. doi:10.3892/ijmm.2015.2143
Keywords: nociception, antinociception, ATP sensitive potassium channel, potassium channel, headache
Citation: Beich SS, Kokoti L and Al-Karagholi MA-M (2025) Activation of KATP channels in pain modulation: a systematic review of preclinical studies. Front. Physiol. 16:1444270. doi: 10.3389/fphys.2025.1444270
Received: 05 June 2024; Accepted: 07 January 2025;
Published: 29 January 2025.
Edited by:
Sean Michael Wilson, Loma Linda University, United StatesReviewed by:
Isabella Salzer, Medical University of Vienna, AustriaShannon D. Shields, GenEdit Inc., United States
Copyright © 2025 Beich, Kokoti and Al-Karagholi. This is an open-access article distributed under the terms of the Creative Commons Attribution License (CC BY). The use, distribution or reproduction in other forums is permitted, provided the original author(s) and the copyright owner(s) are credited and that the original publication in this journal is cited, in accordance with accepted academic practice. No use, distribution or reproduction is permitted which does not comply with these terms.
*Correspondence: Mohammad Al-Mahdi Al-Karagholi, bWFoZGkuYWxrYXJhZ2hvbGlAZ21haWwuY29t