- 1Avian Immunobiology Laboratory, Department of Poultry Science, University of Georgia, Athens, GA, United States
- 2Department of Animal Nutrition and Nutritional Diseases, Faculty of Veterinary Medicine, Ankara University, Ankara, Türkiye
Impaired intestinal integrity in broilers reduces performance and health, highlighting the importance of accurately measuring intestinal permeability (IP) to maintain gut health. The objective of this study was to evaluate the efficiency of iohexol as an IP marker in broilers challenged with Eimeria maxima, Clostridium perfringens, or both during both peak challenge (day [d] 21) and recovery (d 28) periods. One-day-old male Ross 708 birds (n = 56) were distributed into 4 treatment groups: NC (no-challenge control); EM (challenged with 5,000 E. maxima sporulated oocysts/bird on d 15); CP (challenged with 1.0 × 108 CFUs/bird of C. perfringens on d 19 and d 20); and EM + CP (challenged by co-infection of E. maxima and C. perfringens as described). On d 21 and d 28, each bird received an iohexol dose of 64.7 mg/kg body weight via oral gavage. One hour later, blood samples were collected from 14 birds (12 in EM) per group on d 21 and from 7 birds (6 in EM) on d 28. For lesion scoring and ileum collection, 7 birds per group (6 birds in EM) were sampled on each d 21 and d 28. Birds in the EM and EM + CP groups had lower body weight gain (BWG) compared to the NC and CP groups on d 19–21 (P ≤ 0.05). These birds also exhibited significantly greater lesion scores and markedly higher serum iohexol levels on d 21 (P ≤ 0.05). However, no significant differences in serum iohexol levels were observed among treatment groups following recovery on d 28. Moreover, significant differentials were observed in the mRNA abundance of key tight junction proteins (CLDN1, CLDN2, and ZO3), pro-inflammatory cytokines (IL-1β, IFNγ, and IL-22), and gut health markers (GLP2, OLFM4, and MUC2) in the EM and EM + CP groups compared to the NC and CP groups on d 21. In conclusion, this study demonstrates that iohexol is an effective marker for assessing IP in broilers under different enteric challenge conditions.
1 Introduction
The gastrointestinal tract (GIT) serves as the primary defense line and is the largest immune organ in poultry. An intact and functional intestinal barrier prevents the translocation of harmful substances, such as toxins, antigens, and pathogens, to the underlying tissue, while selectively allowing the absorption of nutrients, electrolytes, and water into the bloodstream (Schoultz and Keita, 2020). The mucin layer covering the surface of the mucosal membranes and tight junction (TJ) proteins, which seal the paracellular space between adjacent epithelial cells, are essential components of intestinal integrity (Emami et al., 2019). Disruption or dysfunction of intestinal integrity leads to increased intestinal permeability (IP), allowing the translocation of pathogens and undesired substances, which further triggers inflammation and a systemic inflammatory response (Vanuytsel et al., 2021). Pathogens, environmental stress, poor quality diet, mycotoxins, or microbial imbalance in the GIT could increase IP and compromise the barrier function in broilers. Considering the importance of gut health in broilers, especially as the industry is moving towards removing subtherapeutic antibiotics from the diets, increased attention to the concept of “leaky gut” led researchers to emphasize the need for reliable markers to assess IP.
Several markers including 51chromium-labeled ethylenediamine tetra-acetic acid (51Cr-EDTA), polyethylene glycols, 14Carbon (C)-mannitol, and 14C-inulin, lactulose-L-rhamnose, lucifer yellow, and horseradish peroxidase have been used for the assessment of IP (Schoultz and Keita, 2020). Even though these tests are considered as accurate, they are time consuming, lack standardization, and cannot be performed retrospectively (Camilleri, 2019; Seethaler et al., 2021). Additionally, the radioactivity of 51Cr-EDTA and the bacterial fermentation of sugar-based markers in the intestines have necessitated the investigation of new potential markers. Fluorescein isothiocyanate-dextran (FITC-d) is a fluorescently labeled sugar molecule with a molecular weight of 4 kDa. FITC-d is a well-documented paracellular probe and widely used in experiments conducted with broilers under various experimental challenge conditions in recent years (Hernandez-Patlan et al., 2019; Osho et al., 2023; Souza et al., 2024). However, a variety of factors influence the serum concentration of FITC-d including gavage dose, fasting period, and quantification protocol. Additionally, its light sensitivity can reduce the fluorescence signal and potentially affect measurement accuracy.
Iohexol is a water-soluble, non-ionic, non-radioactive radiological imaging contrast agent, which has long been recognized as an excellent marker for glomerular filtration rate in humans (Gaspari et al., 2018) and animals (Von Hendy-Willson and Pressler, 2011). Since it does not bind to serum proteins or metabolized, it is considered a safe, inert, and highly stable agent in biological samples (Gerova et al., 2011; Carrier et al., 2022). Due to its moderately high molecular weight (821 Da) and stability in the intestinal environment, it is also considered a potential IP marker for the assessment of intestinal integrity under various challenging conditions. Its potential use as an alternative to 51Cr-EDTA first time investigated by Andersen and Laerum (1995). Further studies, especially in humans with inflammatory bowel disease condition suggested that iohexol is a suitable marker for assessing intestinal integrity and barrier function (Gerova et al., 2011). Also, it has been successfully used for the assessment of IP in various animals including dogs (Frias et al., 2012), horses (McGilloway et al., 2023), and rats (Forsgård et al., 2016). In recent years, evaluation of intestinal barrier function using iohexol is also proven in clinically healthy birds (Wilhelm et al., 2020) and in birds challenged with coccidiosis (Rysman et al., 2023).
This study evaluated the efficiency of iohexol as an IP marker in broilers challenged with Eimeria maxima, Clostridium perfringens, or both during both peak challenge and recovery periods. Overall, the presented findings provide new insight into the usefulness of iohexol in monitoring intestinal permeability under various enteric challenge conditions.
2 Materials and methods
2.1 Birds, management, and challenge protocol
A total of 56 one-day-old male Ross 708 birds were individually weighed and wing tagged at initial placement and were raised on a standard starter diet for 15 days (d) with ad libitum access to feed and water. Subsequently, birds were distributed into 4 treatment groups each consisting of 14 birds. Three different challenge models along with a no-challenge control were used between d 15–28. The negative control (NC) served as a no-challenge control group. Birds in E. maxima (EM) group received an oral gavage of 5,000 E. maxima sporulated oocysts/bird on d 15. C. perfringens (CP) group were challenged with 1.0
2.2 Iohexol inoculation and sampling procedure
On d 21, individual bird BW was recorded and used to determine the exact dose of iohexol for each bird based on the rate of 64.7 mg/kg BW. Iohexol (Thermo Scientific Waltham, MA, United States) was diluted in sterile distilled water and given via oral gavage. The concentration of iohexol was determined based on previously published data to ensure accurate dosing for the assessment of IP (Rysman et al., 2023). This dosage was also used in broilers as a marker of renal function (Dhondt et al., 2019; Stroobant et al., 2020). After 1 h, blood samples (14 birds/group for NC, CP, and EM + CP and 12 birds/group for EM on d 21) were collected from the wing vein by direct venipuncture and stored in serum tubes. Samples were kept at room temperature for about 30 min and then centrifuged (1,500
2.3 Lesion scoring
Lesions in the jejunum and ileum were scored on d 21. Coccidiosis lesion scoring was performed according to the method of Johnson and Reid (1970) based on scores ranging from 0 (no gross lesions) to 4 (most severe lesions). NE lesion scoring was based on a 0–3 score, with 0 being normal and 3 being the most severe as previously described by Hofacre et al. (1998).
2.4 Sample preparation and serum iohexol determination
Serum samples were prepared by diluting 100 μL of serum with 100 μL of Milli-Q water. Each sample was then spiked with 25 μL of the internal standard iohexol-d5 (Cayman Chemicals, Ann Arbor, MI, United States) at a 100 μg/mL concentration. To precipitate proteins, 15 μL of 100% trifluoroacetic acid were added to each sample, followed by vortexing for 10 s. The samples were then centrifuged at 13,000
Serum iohexol levels were analyzed by liquid chromatography-mass spectrometry (LC-MS) using an ACQUITY UPLC HSS T3 Column (2.1 mm × 50 mm, 1.8 µm, Waters Co., MA, United States). Mobile phase A consisted of 5% acetonitrile (ACN) and 95% water with 0.1% formic acid, and mobile phase B consisted of 95% ACN and 5% water with 0.1% formic acid. The flow rate was set at 0.4 mL/min and the injection volume was 5 µL. The gradient profile was set as follows: 0.0 min at 100% A, 0.5 min at 100% A, 6.0 min at 50% A, 6.1 min at 0% A, 8.0 min at 0% A, 8.1 min at 100% A, and 10.0 min at 100% A. Mass spectrometric detection was carried out on a Waters SYNAPT G2-Si High-Definition MS system with an electrospray ionization source operating in positive mode. The capillary voltage was set at 3.0 kV, the cone voltage at 25 V, and the source temperature at 120°C.
2.5 Total RNA extraction and reverse transcription
A 20–30 mg aliquot of ileum tissue was weighed into a 2-mL microcentrifuge tube and homogenized in 800 μL TRI Reagent (Zymo Research, Irvine, CA, United States) by a Bead Mill Homogenizer (VWR International, Radnor, PA, United States). Total RNA was extracted from the homogenate using the Direct-zol RNA Kits (Zymo Research) according to the manufacturer’s recommendations. Total RNA concentration was determined at optical density (OD) of 260 (NanoDrop OneC Spectrophotometer, Thermo Fisher Scientific, Waltham, MA, United States), and RNA purity was verified by evaluating the 260/280 OD ratios. RNA samples with a ratio in the range of 1.8–2.0 were considered high quality and were used to synthesize cDNA. RNA integrity was evaluated by gel electrophoresis on 1.5% agarose gel in 0.5 × TAE buffer. After extraction, 2 µg total RNA were used to synthesize first-strand cDNA using the qScript cDNA SuperMix synthesis kit (Quantabio, Beverly, MA, United States) according to the manufacturer’s recommendation and the cDNA was stored at −20°C.
2.6 Quantitative real-time PCR
The mRNA abundance of tight junction proteins [claudin (CLDN)1, CLDN2, CLDN3, zona occludens (ZO)1, ZO2, ZO3, occludin (OCLN), E-cadherin, junctional adhesion molecule (JAM)-A], pro-inflammatory cytokines [interleukin (IL)-1β, IL-22, and interferon gamma (IFNγ)], and intestinal health and function [glucagon-like peptide 2 (GLP2), olfactomedin 4 (OLFM4) and mucin-2 (MUC2)] related genes were determined by QuantStudio™ 3 Real-Time PCR System (Applied Biosystems, Waltham, MA, United States) using PowerTrack™ Fast SYBR Green Master Mix (Applied Biosystems). Details of primer sets are provided in Table 1. cDNA was diluted, and 2 μL of the diluted cDNA were added to each well of a 96-well plate. Subsequently, 8 μL of the real-time PCR master mix comprising 5 μL of PowerTrack™ Fast SYBR Green Master Mix, 0.5 μL of forward primer, 0.5 μL of reverse primer, and 2 μL of sterile nuclease-free water, were added to each well resulting in a final reaction volume of 10 μL. For the PCR reaction, samples were subjected to initial enzyme activation at 95°C for 2 min followed by 40 cycles of denaturation at 95°C for 5 s, annealing and extension at 60°C for 30 s. Product specificity was confirmed by analysis of the melting curves. The mRNA levels were analyzed using 18 S ribosomal subunit (18 S) gene as an endogenous control. Average mRNA abundance relative to 18 S for each sample was calculated using the 2−ΔΔCt method (Livak and Schmittgen, 2001). The calibrator for each gene was the average ΔCt value from the NC group on a particular sampling day.
2.7 Statistical analysis
Data were subjected to a one-way analysis of variance (ANOVA) using the JMP Pro 17. When significant differences were noted, Tukey’s test was used to compare separated means. The influence of different challenge models on lesion scores were performed with the non-parametric Mann-Whitney U test. Statistical differences were considered significant at p ≤ 0.05. All figures were generated using GraphPad Prism 8 software (San Diego, CA, United States).
3 Results
3.1 Growth performance
Growth performance of broilers after the challenge period is shown in Table 2. Following E. maxima challenge until C. perfringens inoculation, no significant differences were observed among treatments in terms of BWG, FI, and FCR during d 15–19. Birds in EM and EM + CP treatments had lower BWG compared to NC and CP groups between d 19 and 21 (p < 0.001). Moreover, birds in EM and EM + CP groups exhibited statistically compromised FCR compared to NC and CP groups, with the EM + CP birds having the worst FCR, followed by EM during d 19–21 (p < 0.001). Birds in the CP group showed similar growth performance to NC birds during the challenge period.
3.2 Lesion scores
Eimeria maxima and NE related lesion scores are shown in Figure 1. As expected, no lesions were observed in the NC group. Additionally, no visible lesions were seen in the CP group. Due to the different lesion scoring criteria used for the EM and EM + CP groups, their lesion scores were evaluated separately, revealing that both the EM (p = 0.014) and EM + CP (p ≤ 0.001) groups exhibited significantly greater lesion scores compared to the NC group on d 21.
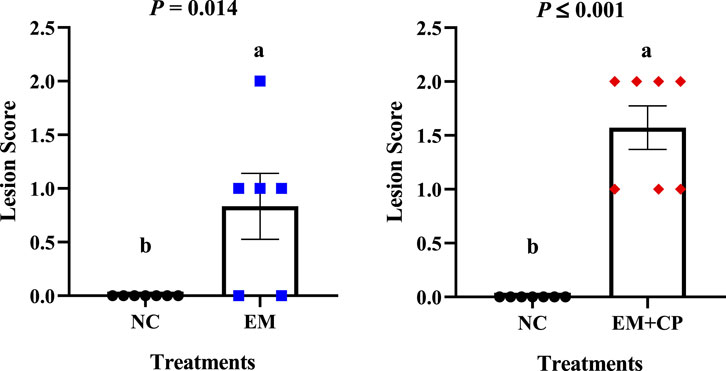
Figure 1. Lesion scores in the jejunum and ileum on d 21. Differences were determined by Mann-Whitney U test at 5% probability. NC: no-challenge control; EM: E. maxima; EM + CP: E. maxima + C. perfringens. (a,b)Bars with different letters letters are significantly different (p ≤ 0.05).
3.3 Serum iohexol level
Serum iohexol level of broilers during the peak challenge (d 21) and recovery (d 28) periods are shown in Figure 2. On d 21, a significant difference in serum iohexol levels were observed among the treatment groups (p ≤ 0.001). The EM (5.73 μg/mL) and EM + CP (7.60 μg/mL) groups exhibited markedly greater serum iohexol levels compared to NC (0.32 μg/mL) and CP (0.32 μg/mL) groups. Although there were no significant differences among the treatment groups in terms of serum iohexol level on d 28, the EM (0.37 μg/mL) and EM + CP (0.37 μg/mL) groups tended to have higher (p = 0.070) levels compared to NC (0.27 μg/mL) and CP (0.28 μg/mL) groups.
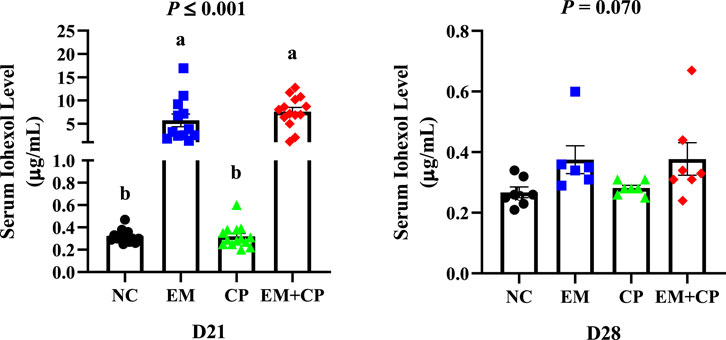
Figure 2. Serum iohexol levels of NC, EM, CP, and EM + CP treatments on d 21 and d 28. Data are presented as mean ± SEM with individual variables. NC: no-challenge control; EM: E. maxima; CP: C. perfringens; EM + CP: E. maxima + C. perfringens. Data are presented as mean ± SEM (n = 14 birds/group for NC, CP, and EM + CP while n = 12 birds/group for EM on d 21 and n = 7 birds/group for NC, CP, and EM + CP while n = 6 birds/group for EM on d 28). (a,b)Bars with different letters are significantly different (p ≤ 0.05).
3.4 mRNA abundance of gut integrity and immune response-related genes in the ileum on d 21
Effects of EM, CP, or EM + CP challenge on mRNA abundance of TJ protein-related genes in the ileum of birds are shown in Figure 3. CLDN1 (p ≤ 0.001) and CLDN2 (p = 0.039) mRNA abundance were significantly higher in EM and EM + CP groups compared to NC and CP groups. mRNA abundance of ZO2 showed a significant difference among treatment groups (p = 0.037) where the EM + CP treatment resulted in the highest fold change compared to NC group. mRNA abundance of ZO3 was significantly lower in EM and EM + CP groups compared to NC group but not significantly different from the CP group (p = 0.019). Moreover, E-cadherin mRNA abundance was significantly higher in EM + CP group compared to NC and EM groups but similar to CP group. No significant differences were observed in mRNA abundance of CLDN3, ZO1, OCLN, and JAM-A among the treatment groups on d 21.
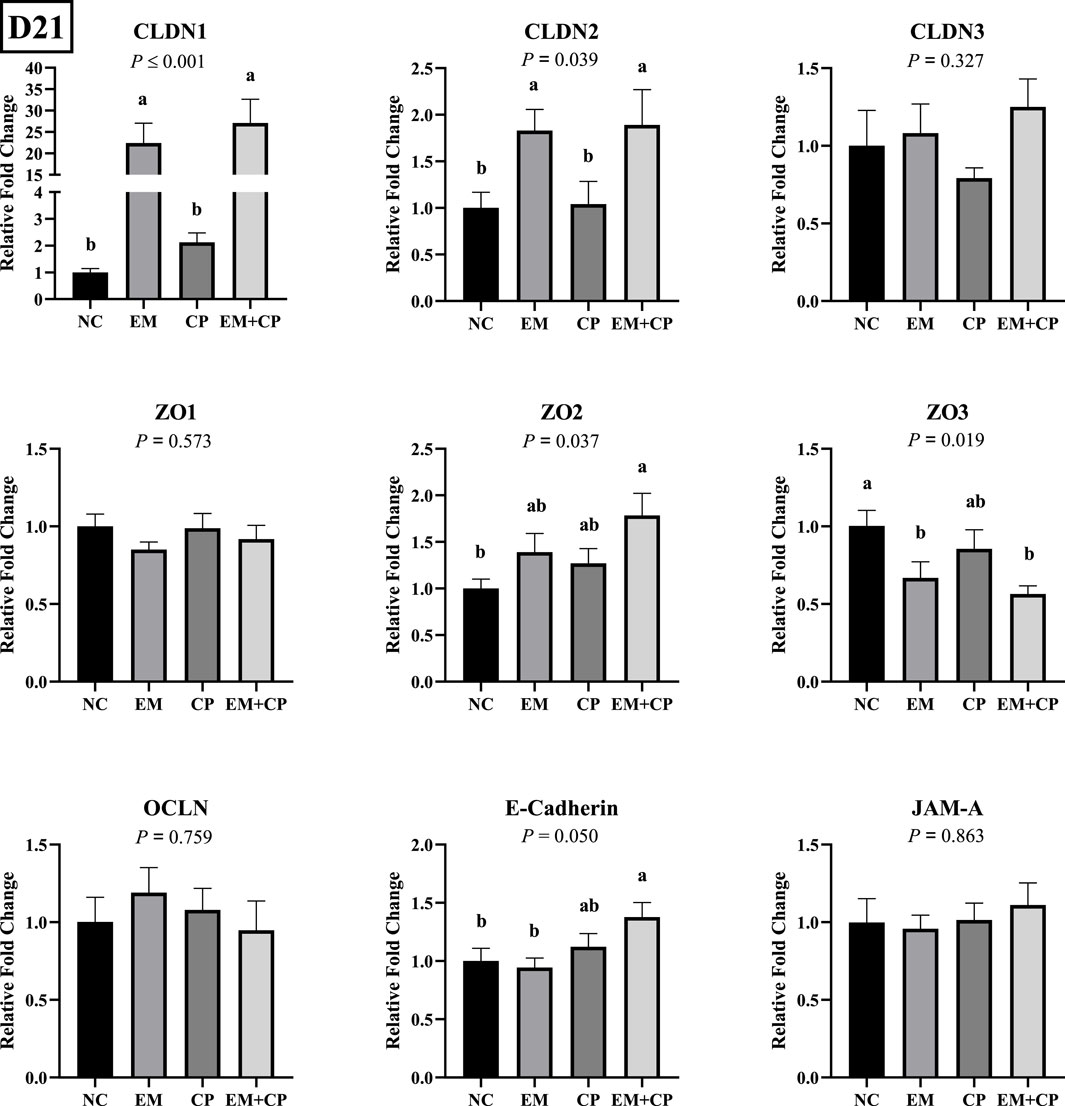
Figure 3. Relative mRNA abundance of TJ protein-related genes on d 21 in the ileum of birds challenged with E. maxima, C. perfringens, or both. The relative mRNA abundance of these targets was determined by qPCR and analyzed by 2−ΔΔCt method using NC group as a calibrator. Data are presented as mean ± SEM (n = 7 birds/group for NC, CP, and EM + CP and n = 6 birds/group for EM). (a,b)Bars with different letters are significantly different (p ≤ 0.05).
The relative mRNA abundances of GLP2, OLFM4, and MUC2 in the ileum of the birds challenged with EM, CP, or EM + CP are shown in Figure 4. GLP2 (p ≤ 0.001) and MUC2 (p = 0.003) mRNA abundance were significantly lower in the EM and EM + CP groups compared to the NC and CP groups. OLFM4 mRNA abundance was significantly greater in EM and EM + CP groups compared to NC group (p ≤ 0.001). However, CP birds had similar levels of OLFM4 mRNA compared to NC and EM birds. As shown in Figure 5, mRNA abundances of IL-1β, IFNγ, and IL-22 were significantly higher in EM and EM + CP groups compared to NC and CP groups in the ileum on d 21 (p ≤ 0.001).
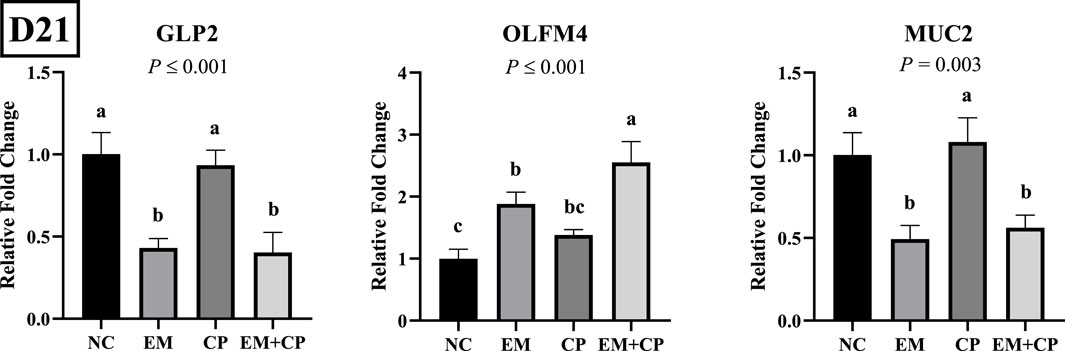
Figure 4. Relative mRNA abundance of GLP2, OLFM4, and MUC2 on d 21 in the ileum of birds challenged with E. maxima, C. perfringens, or both. The relative mRNA abundance of these targets was determined by qPCR and analyzed by 2−ΔΔCt method using NC group as a calibrator. Data are presented as mean ± SEM (n = 7 birds/group for NC, CP, and EM + CP and n = 6 birds/group for EM). (a–c)Bars with different letters are significantly different (p ≤ 0.05).
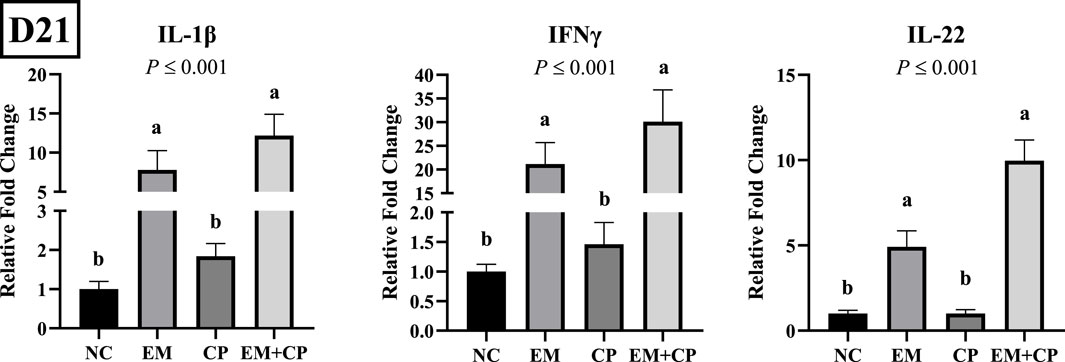
Figure 5. Relative mRNA abundance of IL-1β, IFNγ, and IL-22 on d 21 in the ileum of birds challenged with E. maxima, C. perfringens, or both. The relative mRNA abundance of these targets was determined by qPCR and analyzed by 2−ΔΔCt method using NC group as a calibrator. Data are presented as mean ± SEM (n = 7 birds/group for NC, CP, and EM + CP and n = 6 birds/group for EM). (a,b)Bars with different letters are significantly different (p ≤ 0.05).
3.5 mRNA abundance of gut integrity and immune response-related genes in ileum on d 28
CLDN3 mRNA level was significantly lower in the EM + CP group compared to the NC group. However, EM and CP groups exhibited similar CLDN3 mRNA abundance compared to NC and EM + CP groups. CLDN1, CLDN2, ZO1, ZO2, ZO3, OCLN, E-cadherin, and JAM-A, did not show statistically significant differences in mRNA abundance among the treatment groups on d 28 (Figure 6).
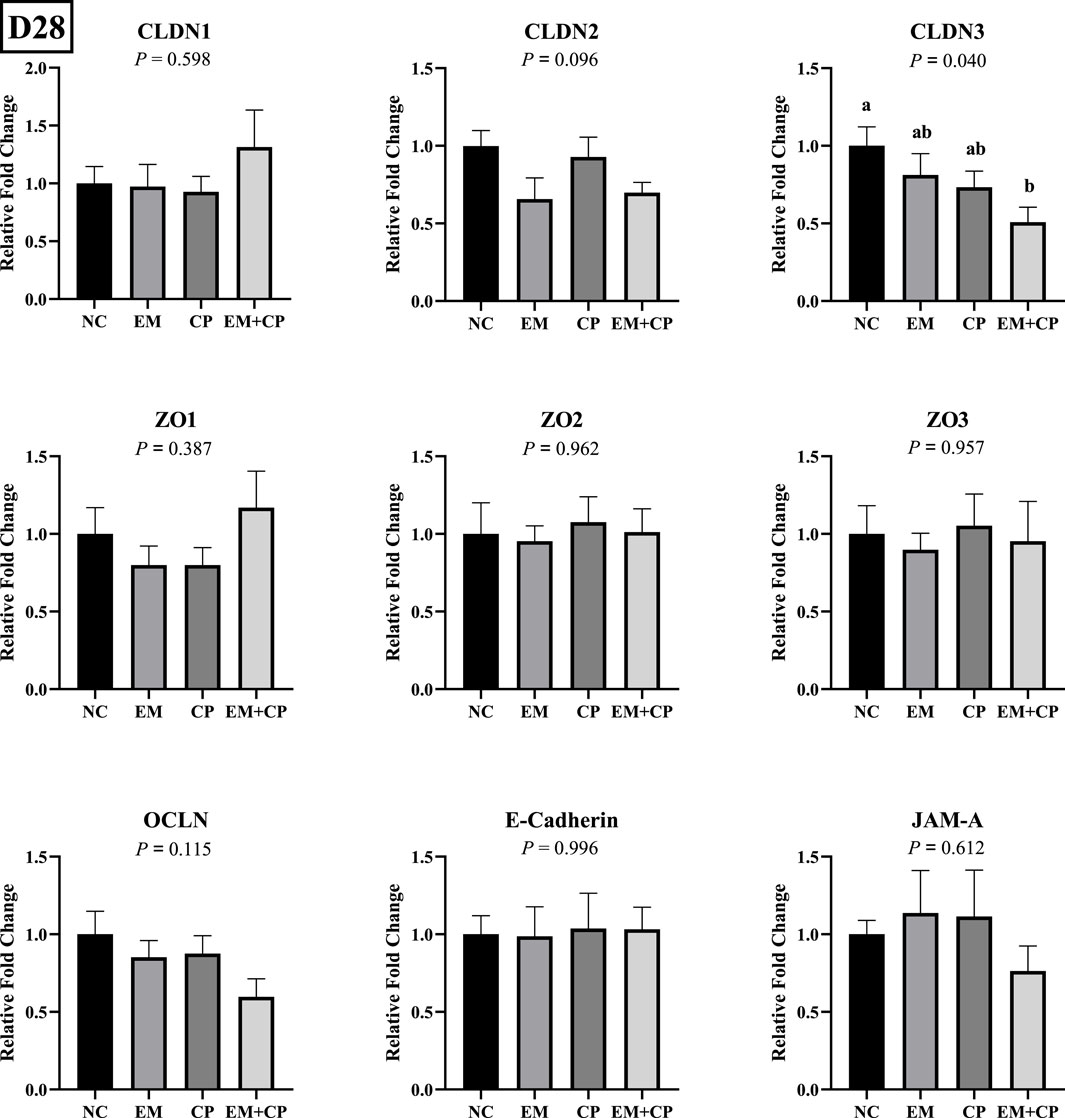
Figure 6. Relative mRNA abundance of TJ protein-related genes on d 28 in the ileum of birds challenged with E. maxima, C. perfringens, or both. The relative mRNA abundance of these targets was determined by qPCR and analyzed by 2−ΔΔCt method using NC group as a calibrator. Data are presented as mean ± SEM (n = 7 birds/group for NC, CP, and EM + CP and n = 6 birds/group for EM). (a,b)Bars with different letters are significantly different (p ≤ 0.05).
The mRNA abundance of genes associated with gut health, including GLP2, OLFM4, and MUC2, did not exhibit significant differences across the treatment groups on d 28 (Figure 7). Similarly, the mRNA abundance levels of IL-1β, IFNγ, and IL-22 showed no statistically significant differences among the treatment groups on d 28 (Figure 8).
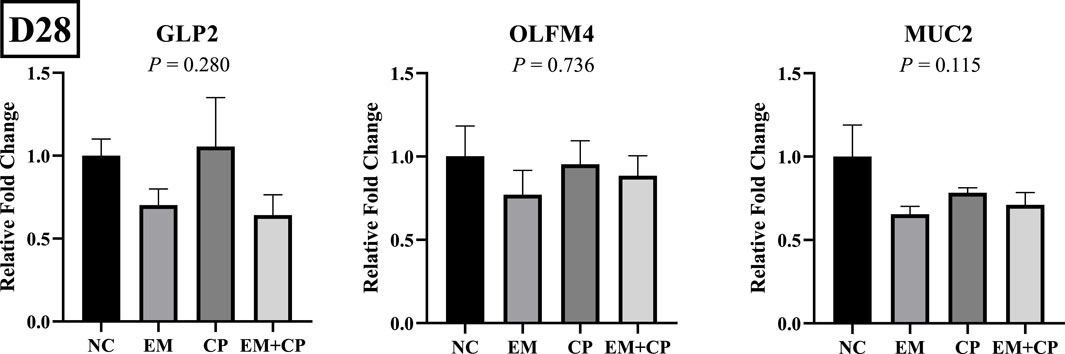
Figure 7. Relative mRNA abundance of GLP2, OLFM4, and MUC2 on d 28 in the ileum of birds challenged with E. maxima, C. perfringens, or both. The relative mRNA abundance of these targets was determined by qPCR and analyzed by 2−ΔΔCt method using NC group as a calibrator. Data are presented as mean ± SEM (n = 7 birds/group for NC, CP, and EM + CP and n = 6 birds/group for EM).
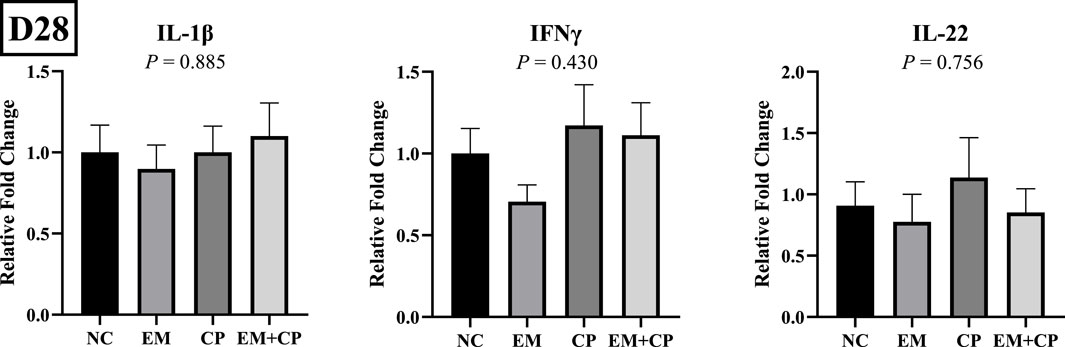
Figure 8. Relative mRNA abundance of IL-1β, IFNγ, and IL-22 on d 28 in the ileum of birds challenged with E. maxima, C. perfringens, or both. The relative mRNA abundance of these targets was determined by qPCR and analyzed by 2−ΔΔCt method using NC group as a calibrator. Data are presented as mean ± SEM (n = 7 birds/group for NC, CP, and EM + CP and n = 6 birds/group for EM).
4 Discussion
Impaired intestinal integrity caused by various factors in broilers is directly associated with reduced growth performance. Especially after the ban on the use of subtherapeutic antibiotics in diets, maintaining optimal intestinal health has become a fundamental goal in the industry for preventing diseases, supporting immune function, and ensuring optimum digestion and nutrient absorption. Accurate measurement of IP with reliable markers is one of the key strategies for assessing gut health and could help to implement effective interventions to enhance overall performance and health. Therefore, the current study investigated the use of iohexol as an IP marker in broilers challenged with E. maxima, C. perfringens, or both during both peak challenge and recovery periods.
Coccidiosis and NE challenges compromise the integrity of intestinal epithelial lining, leading to triggered inflammatory response and increased IP, which further results in impaired growth performance (Calik et al., 2019; Emami et al., 2019; Hansen et al., 2021; Blue et al., 2023; Froebel et al., 2024). In the current study, we successfully established subclinical E. maxima and NE challenge models to examine the TJ disruption and assess the efficacy of iohexol as an external IP marker during both peak challenge and recovery periods. According to our findings, birds challenged with E. maxima alone or with E. maxima and C. perfringens together showed reduced growth performance and exhibited significantly greater intestinal lesions compared to other treatments on d 21. Besides, birds in the CP group showed similar growth performance with NC birds during the challenge period. This was somewhat an expected outcome since C. perfringens needs several predisposing factors such as coccidiosis, intestinal dysbiosis, or poor-quality/poorly balanced diets to induce NE (Emami and Dalloul, 2021). In line with the performance and lesion score results, birds in EM and EM + CP groups exhibited significantly higher serum iohexol levels compared to NC and CP groups on d 21. Consistent with our observations, a recent study demonstrated that birds challenged with different strains of Eimeria spp. exhibited significantly greater serum iohexol levels on d 20 and d 21 (Rysman et al., 2023). Our findings align with previous studies in humans, horses, dogs, and rats, where iohexol effectively reflected changes in IP, supporting its role in assessing intestinal integrity under various conditions (Klenner et al., 2009; Gerova et al., 2011; Frias et al., 2012; Forsgård et al., 2016; McGilloway et al., 2023). Moreover, the current study also is the first to measure serum iohexol levels after the recovery phase (d 28) to improve our understanding and efficacy of iohexol as an IP marker. Although the EM and EM + CP groups showed slightly higher serum iohexol levels compared to the NC and CP groups, these results indicate a possible recovery of intestinal integrity over time in the challenged groups.
Epithelial cells regulate selective permeability through two main routes: the transcellular and paracellular pathways. The transcellular pathway, mediated by transporters and channels, manages nutrient absorption, while the paracellular pathway allows transport between cells, controlled by tight and adherence junctions (Suzuki, 2013). Disruption of TJ proteins result in a loss of barrier function, increasing permeability via the paracellular pathway (Berkes et al., 2003; Horowitz et al., 2023). When intestinal barrier integrity is compromised, the permeability of TJs increases, allowing external markers to pass through the paracellular pathway, where they enter the bloodstream and can be quantified in serum as a measure of IP (Schoultz and Keita, 2020). The paracellular route used by ions, water and larger hydrophilic compounds from ∼400–600 Da up to 10–20 kDa and that cannot cross transcellularly (Vanuytsel et al., 2021). Most studies on paracellular transport identify two distinct pathways regulated by TJs: (1) the pore pathway, which is a high-capacity, charge-selective route that permits the passage of small ions and uncharged molecules, and (2) the leak pathway, a low-capacity route that allows larger ions and macromolecules to pass, regardless of their charge (Vanuytsel et al., 2021; Horowitz et al., 2023). The pore pathway is primarily controlled by CLDN proteins, which form selective pores that regulate ion permeability. In contrast, the leak pathway is governed by OCLN and ZO proteins, which manage the transport of larger molecules, including proteins and peptides (Watson, 2015).
In the present study, significant alterations were observed in the mRNA abundance of key TJ proteins (CLDN1, CLDN2, and ZO3), pro-inflammatory cytokines (IL-1β, IFNγ, and IL-22), and gut health markers (GLP2, OLFM4, and MUC2) in the EM and EM + CP treatment groups compared to the NC and CP groups on d 21. CLDN1 plays a crucial role in maintaining selective permeability by regulating the passage of ions, water, and small molecules while preventing the leakage of harmful substances such as toxins and pathogens from the gut lumen into the underlying tissues (Lu et al., 2013). Interestingly, the current study revealed significantly higher CLDN1 mRNA abundance in the EM and EM + CP groups compared to the NC and CP groups, despite its role as a pore-sealing TJ protein. Due to its physiological role as a pore-sealing TJ-protein, the increase observed in the EM and EM + CP treatments is somewhat unexpected. However, greater CLDN1 mRNA abundance in the small intestine of broilers under Eimeria spp. and NE challenge conditions has been documented (Bortoluzzi et al., 2019; Gharib-Naseri et al., 2021; Goo et al., 2023). The elevation of pro-inflammatory cytokines observed in this study may contribute to the induction of CLDN1 mRNA expression, aligning with the findings of Pope et al. (2014). Additionally, this increase may be associated with the anti-apoptotic effects of CLDN1, providing protective benefits in challenged birds (Akasaka et al., 2010; Gharib-Naseri et al., 2021). Therefore, increased CLDN1 expression does not necessarily indicate enhanced TJ function, as its regulation may reflect a compensatory response to epithelial damage rather than a direct improvement in barrier integrity. Unlike CLDN1, CLDN2 is a pore-forming TJ protein and increased levels would directly lead to hyperpermeability and worsen barrier function (Yoseph et al., 2016; Oami et al., 2024). In vivo and in vitro studies have implicated IL-1β, IL-6, IL-13, IL-22, and TNFα as potential enhancers of CLDN2 (Heller et al., 2005; Mankertz et al., 2009; Suzuki et al., 2011; Tsai et al., 2017; Raju et al., 2020). Additionally, ZO3, a critical scaffold protein that links transmembrane TJ proteins (such as claudins) to the actin cytoskeleton, showed reduced mRNA abundance in response to EM and EM + CP challenges. At the molecular level, the first pore is mainly regulated by CLDNs and the latter by the OCLN and the ZO family (Vanuytsel et al., 2021). We did not observe a noticeable difference in OCLN and ZO1 in mRNA abundance during the peak infection. This lack of change is believed to be related to the subclinical challenge model used in the current study, which is consistent with previous findings (Emami et al., 2019; Emami et al., 2020; Goo et al., 2023). Observed changes in TJ proteins and pro-inflammatory cytokines were accompanied by a decrease in GLP2 and MUC2 mRNA abundance, along with an increase in OLFM4 abundance in both the EM and EM + CP groups compared to NC group. GLP2 promotes growth and function of the intestinal epithelium and improves the intestinal barrier function (Abdalqadir and Adeli, 2022). A previous study reported a significant negative association between plasma GLP2 and intestinal permeability (Cani et al., 2009). Decreased MUC2 levels are often attributed to goblet cell damage induced by intestinal challenges (Gharib-Naseri et al., 2021). Moreover, elevated CLDN1 mRNA level has been suggested to activate Notch signaling, which inhibits goblet cell differentiation and further contributes to reduced mucin production (Pope et al., 2014). OLFM4 is a stem cell marker and plays a crucial role in maintaining gut homeostasis and regenerating damaged tissue (Kinstler et al., 2024). Increased mRNA level of OLFM4 during peak infection, particularly in EM + CP treatment, is likely related to the promotion of intestinal epithelial cell regeneration in response to the increased intestinal inflammation (Xing et al., 2024). Therefore, all these observed changes in the ileum clearly show the disruptions of intestinal integrity and increase in IP during peak infection. However, during the recovery period, the mRNA levels of both TJ proteins (except CLDN3), pro-inflammatory cytokines, and intestinal health-related markers had largely returned to baseline levels, with no significant differences observed between the various treatment groups.
The impact of intestinal challenges such as Eimeria spp. or NE on intestinal TJ proteins, pro-inflammatory cytokines, and gut health markers has been extensively investigated in previous studies. However, there are few methodologies available for assessing IP with external markers in avian species. Although there is considerably more information available on FITC-d (Liu et al., 2021), studies on iohexol as an IP marker in broilers remain limited. It is known that a reliable IP marker should exhibit low absorption through the intact intestinal barrier and increased absorption through inflamed intestinal mucosa (Schoultz and Keita, 2020). In the current study, we observed a significant elevation in serum iohexol levels during the peak challenge period, which subsequently returned to near baseline levels during the recovery phase. Additionally, an IP marker should be small, non-metabolizable, water-soluble, has low protein binding, be chemically stable, and detectable by reliable methods. The radiographic contrast agent iohexol, with a molecular weight of 821 Da, is significantly smaller than FITC-d (4 kDa), a commonly used intestinal marker in broilers. The smaller molecular weight of the iohexol could be advantageous in evaluating the paracellular permeability in subclinical challenge models or during the early phase of the Eimeria spp. or NE challenges (Rysman et al., 2023). Moreover, detection methods for iohexol, such as HPLC or mass spectrometry, may enable more accurate and quantitative measurements. However, further comparative studies are needed to confirm these advantages and determine its broader applicability in broilers.
Overall, we observed an increase in serum iohexol levels during peak infection, along with significant changes in performance parameters, lesion scores, mRNA abundance of key TJ proteins, pro-inflammatory cytokines, and gut health markers in EM and EM + CP birds compared to NC and CP birds. During the recovery period, the lack of noticeable differences in these parameters likely reflects a recovery of intestinal integrity and barrier function. In line with our current findings, we conclude that iohexol can be effectively used as an IP marker to assess alterations in intestinal integrity in broilers.
Data availability statement
The original data presented in the study are included in this article, further inquiries can be directed to the corresponding author.
Ethics statement
The animal study was approved by UGA Institutional Animal Care and Use Committee. The study was conducted in accordance with the local legislation and institutional requirements.
Author contributions
AC: Conceptualization, Data curation, Formal Analysis, Investigation, Methodology, Visualization, Writing–original draft, Writing–review and editing. AN: Data curation, Formal Analysis, Writing–review and editing. BD: Data curation, Formal Analysis, Writing–review and editing. CB: Data curation, Formal Analysis, Writing–review and editing. DF: Data curation, Formal Analysis, Writing–review and editing. RD: Conceptualization, Data curation, Formal Analysis, Funding acquisition, Investigation, Methodology, Project administration, Resources, Supervision, Validation, Visualization, Writing–review and editing.
Funding
The author(s) declare that financial support was received for the research, authorship, and/or publication of this article. This research was funded in part by a cooperative agreement (# 58-6040-2-016) from the USDA Agricultural Research Service.
Acknowledgments
Ali Calik was a recipient of a fellowship from the Scientific and Technological Research Council of Türkiye (TUBITAK). E. maxima oocysts were kindly provided by Dr. Lorraine Fuller at the UGA Department of Poultry Science.
Conflict of interest
The authors declare that the research was conducted in the absence of any commercial or financial relationships that could be construed as a potential conflict of interest.
Generative AI statement
The author(s) declare that no Generative AI was used in the creation of this manuscript.
Publisher’s note
All claims expressed in this article are solely those of the authors and do not necessarily represent those of their affiliated organizations, or those of the publisher, the editors and the reviewers. Any product that may be evaluated in this article, or claim that may be made by its manufacturer, is not guaranteed or endorsed by the publisher.
References
Abdalqadir N., Adeli K. (2022). GLP-1 and GLP-2 orchestrate intestine integrity, gut microbiota, and immune system crosstalk. Microorganisms 10 (10), 2061. doi:10.3390/microorganisms10102061
Akasaka H., Sato F., Morohashi S., Wu Y., Liu Y., Kondo J., et al. (2010). Anti-apoptotic effect of claudin-1 in tamoxifen-treated human breast cancer MCF-7 cells. BMC Cancer 10, 548. doi:10.1186/1471-2407-10-548
Andersen R., Laerum F. (1995). Intestinal permeability measurements—a new application for water-soluble contrast media? Acta Radiol. 36 (399), 247–252. doi:10.1177/0284185195036s39930
Berkes J., Viswanathan V., Savkovic S., Hecht G. (2003). Intestinal epithelial responses to enteric pathogens: effects on the tight junction barrier, ion transport, and inflammation. Gut 52 (3), 439–451. doi:10.1136/gut.52.3.439
Blue C. E., Emami N. K., White M. B., Cantley S., Dalloul R. A. (2023). Inclusion of quillaja saponin clarity Q manages growth performance, immune response, and nutrient transport of broilers during subclinical necrotic enteritis. Microorganisms 11 (8), 1894. doi:10.3390/microorganisms11081894
Bortoluzzi C., Vieira B., Lumpkins B., Mathis G., King W., Graugnard D., et al. (2019). Can dietary zinc diminish the impact of necrotic enteritis on growth performance of broiler chickens by modulating the intestinal immune-system and microbiota? Poult. Sci. 98 (8), 3181–3193. doi:10.3382/ps/pez045
Calik A., Omara I. I., White M. B., Evans N. P., Karnezos T. P., Dalloul R. A. (2019). Dietary non-drug feed additive as an alternative for antibiotic growth promoters for broilers during a necrotic enteritis challenge. Microorganisms 7 (8), 257. doi:10.3390/microorganisms7080257
Camilleri M. (2019). Leaky gut: mechanisms, measurement and clinical implications in humans. Gut 68 (8), 1516–1526. doi:10.1136/gutjnl-2019-318427
Cani P. D., Possemiers S., Van de Wiele T., Guiot Y., Everard A., Rottier O., et al. (2009). Changes in gut microbiota control inflammation in obese mice through a mechanism involving GLP-2-driven improvement of gut permeability. Gut 58 (8), 1091–1103. doi:10.1136/gut.2008.165886
Carrier P., Destere A., Giguet B., Debette-Gratien M., Essig M., Monchaud C., et al. (2022). Iohexol plasma and urinary concentrations in cirrhotic patients: a pilot study. World J. Hepatol. 14 (8), 1621–1632. doi:10.4254/wjh.v14.i8.1621
Dhondt L., Croubels S., De Cock P., De Paepe P., De Baere S., Devreese M. (2019). Development and validation of an ultra-high performance liquid chromatography–tandem mass spectrometry method for the simultaneous determination of iohexol, p-aminohippuric acid and creatinine in porcine and broiler chicken plasma. J. Chromatogr. B 1117, 77–85. doi:10.1016/j.jchromb.2019.04.017
Emami N. K., Calik A., White M. B., Kimminau E. A., Dalloul R. A. (2020). Effect of probiotics and multi-component feed additives on microbiota, gut barrier and immune responses in broiler chickens during subclinical necrotic enteritis. Front. Vet. Sci. 7, 572142. doi:10.3389/fvets.2020.572142
Emami N. K., Calik A., White M. B., Young M., Dalloul R. A. (2019). Necrotic enteritis in broiler chickens: the role of tight junctions and mucosal immune responses in alleviating the effect of the disease. Microorganisms 7 (8), 231. doi:10.3390/microorganisms7080231
Emami N. K., Dalloul R. A. (2021). Centennial Review: recent developments in host-pathogen interactions during necrotic enteritis in poultry. Poult. Sci. 100 (9), 101330. doi:10.1016/j.psj.2021.101330
Forsgård R. A., Korpela R., Holma R., Lindén J., Frias R., Spillmann T., et al. (2016). Intestinal permeability to iohexol as an in vivo marker of chemotherapy-induced gastrointestinal toxicity in Sprague–Dawley rats. Cancer Chemother. Pharmacol. 78, 863–874. doi:10.1007/s00280-016-3150-3
Frias R., Strube K., Ternes W., Collado M. C., Spillmann T., Sankari S., et al. (2012). Comparison of 51Chromium-labeled ethylenediamine tetra-acetic acid and iohexol as blood markers for intestinal permeability testing in Beagle dogs. Vet. J. 192 (1), 123–125. doi:10.1016/j.tvjl.2011.04.024
Froebel L. E., Calik A., Emami N. K., Blue C. E., Dalloul R. A. (2024). Evaluating performance, intestinal lesions, and immunity related genes in heritage and modern broiler breeds during a necrotic enteritis challenge. Poult. Sci. 104 (104), 104339. doi:10.1016/j.psj.2024.104339
Gaspari F., Thakar S., Carrara F., Perna A., Trillini M., Aparicio M. C., et al. (2018). Safety of iohexol administration to measure glomerular filtration rate in different patient populations: a 25-year experience. Nephron 140 (1), 1–8. doi:10.1159/000489898
Gerova V. A., Stoynov S. G., Katsarov D. S., Svinarov D. A. (2011). Increased intestinal permeability in inflammatory bowel diseases assessed by iohexol test. World J. Gastroenterol. 17 (17), 2211–2215. doi:10.3748/wjg.v17.i17
Gharib-Naseri K., Kheravii S., Keerqin C., Swick R. A., Choct M., Wu S.-B. (2021). Differential expression of intestinal genes in necrotic enteritis challenged broiler chickens with 2 different Clostridium perfringens strains. Poult. Sci. 100 (3), 100886. doi:10.1016/j.psj.2020.11.063
Goo D., Choi J., Ko H., Choppa V. S. R., Liu G., Lillehoj H. S., et al. (2023). Effects of Eimeria maxima infection doses on growth performance and gut health in dual-infection model of necrotic enteritis in broiler chickens. Front. Physiol. 14, 1269398. doi:10.3389/fphys.2023.1269398
Hansen V. L., Kahl S., Proszkowiec-Weglarz M., Jiménez S. C., Vaessen S. F., Schreier L. L., et al. (2021). The effects of tributyrin supplementation on weight gain and intestinal gene expression in broiler chickens during Eimeria maxima-induced coccidiosis. Poult. Sci. 100 (4), 100984. doi:10.1016/j.psj.2021.01.007
Heller F., Florian P., Bojarski C., Richter J., Christ M., Hillenbrand B., et al. (2005). Interleukin-13 is the key effector Th2 cytokine in ulcerative colitis that affects epithelial tight junctions, apoptosis, and cell restitution. Gastroenterology 129 (2), 550–564. doi:10.1016/j.gastro.2005.05.002
Hernandez-Patlan D., Solis-Cruz B., Pontin K. P., Hernandez-Velasco X., Merino-Guzman R., Adhikari B., et al. (2019). Impact of a Bacillus direct-fed microbial on growth performance, intestinal barrier integrity, necrotic enteritis lesions, and ileal microbiota in broiler chickens using a laboratory challenge model. Front. Vet. Sci. 6, 108. doi:10.3389/fvets.2019.00108
Hofacre C., Froyman R., Gautrias B., George B., Goodwin M., Brown J. (1998). Use of Aviguard and other intestinal bioproducts in experimental Clostridium perfringens-associated necrotizing enteritis in broiler chickens. Avian Dis. 42, 579–584. doi:10.2307/1592685
Horowitz A., Chanez-Paredes S. D., Haest X., Turner J. R. (2023). Paracellular permeability and tight junction regulation in gut health and disease. Nat. Rev. Gastroenterol. Hepatol. 20 (7), 417–432. doi:10.1038/s41575-023-00766-3
Johnson J., Reid W. M. (1970). Anticoccidial drugs: lesion scoring techniques in battery and floor-pen experiments with chickens. Exp. Parasitol. 28 (1), 30–36. doi:10.1016/0014-4894(70)90063-9
Kinstler S. R., Wong E. A., Hofacre C., Lee M. D., Hoerr F. J., Jones M. K., et al. (2024). Subclinical necrotic enteritis impacts developmental Notch and Wnt signaling and intestinal morphology in the small intestine of broiler chickens. Avian Dis. 68, D-24–00018. doi:10.1637/aviandiseases-D-24-00018
Klenner S., Coenen M., Failing K., Hewicker-Trautwein M., Ternes W., Verspohl J., et al. (2009). Estimation of intestinal permeability in healthy dogs using the contrast medium iohexol. Vet. Clin. Pathol. 38 (3), 353–360. doi:10.1111/j.1939-165X.2009.00136.x
Liu J., Teng P.-Y., Kim W. K., Applegate T. J. (2021). Assay considerations for fluorescein isothiocyanate-dextran (FITC-d): an indicator of intestinal permeability in broiler chickens. Poult. Sci. 100 (7), 101202. doi:10.1016/j.psj.2021.101202
Livak K. J., Schmittgen T. D. (2001). Analysis of relative gene expression data using real-time quantitative PCR and the 2(-Delta Delta C(T)) Method. Methods 25 (4), 402–408. doi:10.1006/meth.2001.1262
Lu Z., Ding L., Lu Q., Chen Y.-H. (2013). Claudins in intestines: distribution and functional significance in health and diseases. Tissue Barriers 1 (3), e24978. doi:10.4161/tisb.24978
Mankertz J., Amasheh M., Krug S., Fromm A., Amasheh S., Hillenbrand B., et al. (2009). TNFalpha up-regulates claudin-2 expression in epithelial HT-29/B6 cells via phosphatidylinositol-3-kinase signaling. Cell Tissue Res. 336 (1), 67–77. doi:10.1007/s00441-009-0751-8
McGilloway M., Manley S., Aho A., Heeringa K. N., Lou Y., Squires E. J., et al. (2023). The combination of trailer transport and exercise increases gastrointestinal permeability and markers of systemic inflammation in horses. Equine Vet. J. 55 (5), 853–861. doi:10.1111/evj.13888
Oami T., Abtahi S., Shimazui T., Chen C.-W., Sweat Y. Y., Liang Z., et al. (2024). Claudin-2 upregulation enhances intestinal permeability, immune activation, dysbiosis, and mortality in sepsis. Proc. Natl. Acad. Sci. 121 (10), e2217877121. doi:10.1073/pnas.2217877121
Osho S., Bolek K., Saddoris-Clemons K., Humphrey B., Garcia M. (2023). Impact of a direct-fed microbial supplementation on intestinal permeability and immune response in broiler chickens during a coccidia challenge. Front. Microbiol. 14, 1283393. doi:10.3389/fmicb.2023.1283393
Pope J. L., Bhat A. A., Sharma A., Ahmad R., Krishnan M., Washington M. K., et al. (2014). Claudin-1 regulates intestinal epithelial homeostasis through the modulation of Notch-signalling. Gut 63 (4), 622–634. doi:10.1136/gutjnl-2012-304241
Raju P., Shashikanth N., Tsai P.-Y., Pongkorpsakol P., Chanez-Paredes S., Steinhagen P. R., et al. (2020). Inactivation of paracellular cation-selective claudin-2 channels attenuates immune-mediated experimental colitis in mice. J. Clin. Investig. 130 (10), 5197–5208. doi:10.1172/JCI138697
Rysman K., Eeckhaut V., Croubels S., Maertens B., Van Immerseel F. (2023). Iohexol is an intestinal permeability marker in broilers under coccidiosis challenge. Poult. Sci. 102 (6), 102690. doi:10.1016/j.psj.2023.102690
Schoultz I., Keita A. V. (2020). The intestinal barrier and current techniques for the assessment of gut permeability. Cells 9 (8), 1909. doi:10.3390/cells9081909
Seethaler B., Basrai M., Neyrinck A. M., Nazare J. A., Walter J., Delzenne N. M., et al. (2021). Biomarkers for assessment of intestinal permeability in clinical practice. Am. J. Physiol. Gastrointest. Liver Physiol. 321 (1), G11–G17. doi:10.1152/ajpgi.00113.2021
Souza G. C. d., Esteves G. F., Volpato F. A., Miotto R., Mores M. A. Z., Ibelli A. M. G., et al. (2024). Effects of varying concentrations of Eimeria challenge on the intestinal integrity of broiler chickens. Poultry 3 (1), 1–14. doi:10.3390/poultry3010001
Stroobant L., Croubels S., Dhondt L., Millecam J., De Baere S., Gasthuys E., et al. (2020). Simultaneous measurement of glomerular filtration rate, effective renal plasma flow and tubular secretion in different poultry species by single intravenous bolus of iohexol and para-aminohippuric acid. Animals 10 (6), 1027. doi:10.3390/ani10061027
Suzuki T. (2013). Regulation of intestinal epithelial permeability by tight junctions. Cell. Mol. Life Sci. 70, 631–659. doi:10.1007/s00018-012-1070-x
Suzuki T., Yoshinaga N., Tanabe S. (2011). Interleukin-6 (IL-6) regulates claudin-2 expression and tight junction permeability in intestinal epithelium. J. Biol. Chem. 286 (36), 31263–31271. doi:10.1074/jbc.M111.238147
Tsai P.-Y., Zhang B., He W.-Q., Zha J.-M., Odenwald M. A., Singh G., et al. (2017). IL-22 upregulates epithelial claudin-2 to drive diarrhea and enteric pathogen clearance. Cell Host Microbe. 21 (6), 671–681.e4. doi:10.1016/j.chom.2017.05.009
Vanuytsel T., Tack J., Farre R. (2021). The role of intestinal permeability in gastrointestinal disorders and current methods of evaluation. Front. Nutr. 8, 717925. doi:10.3389/fnut.2021.717925
Von Hendy-Willson V. E., Pressler B. M. (2011). An overview of glomerular filtration rate testing in dogs and cats. Vet. J. 188 (2), 156–165. doi:10.1016/j.tvjl.2010.05.006
Watson A. J. (2015). Claudins and barrier dysfunction in intestinal inflammation: cause or consequence? Gut 64 (10), 1501–1502. doi:10.1136/gutjnl-2014-309110
Wilhelm F. R., Krautwald-Junghanns M.-E., Ortín-Piqueras V., Junnila J., Cramer K., Forsgård R. A., et al. (2020). Iohexol-based measurement of intestinal permeability in birds. J. Exot. Pet. Med. 34, 18–23. doi:10.1053/j.jepm.2020.04.004
Xing Z., Li X., He J., Chen Y., Zhu L., Zhang X., et al. (2024). OLFM4 modulates intestinal inflammation by promoting IL-22+ ILC3 in the gut. Commun. Biol. 7 (1), 914. doi:10.1038/s42003-024-06601-y
Keywords: iohexol, intestinal permeability, necrotic enteritis, broiler, tight junction
Citation: Calik A, Niraula A, Dong B, Blue CEC, Fenster DA and Dalloul RA (2024) Iohexol-based assessment of intestinal permeability in broilers challenged with Eimeria maxima, Clostridium perfringens or both. Front. Physiol. 15:1520346. doi: 10.3389/fphys.2024.1520346
Received: 31 October 2024; Accepted: 04 December 2024;
Published: 20 December 2024.
Edited by:
Elizabeth Ruth Gilbert, Virginia Tech, United StatesReviewed by:
Servet Yalcin, Ege University, TürkiyeDawn A. Koltes, Iowa State University, United States
Copyright © 2024 Calik, Niraula, Dong, Blue, Fenster and Dalloul. This is an open-access article distributed under the terms of the Creative Commons Attribution License (CC BY). The use, distribution or reproduction in other forums is permitted, provided the original author(s) and the copyright owner(s) are credited and that the original publication in this journal is cited, in accordance with accepted academic practice. No use, distribution or reproduction is permitted which does not comply with these terms.
*Correspondence: Rami A. Dalloul, UmFtaS5EYWxsb3VsQHVnYS5lZHU=