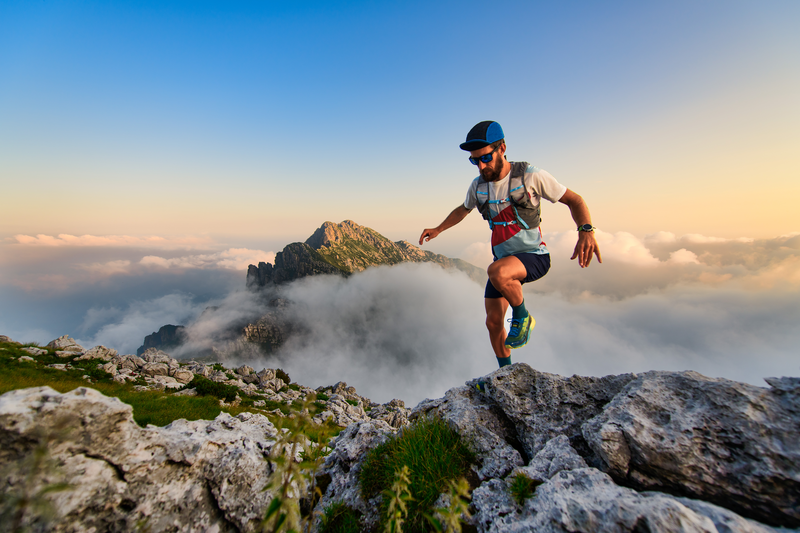
95% of researchers rate our articles as excellent or good
Learn more about the work of our research integrity team to safeguard the quality of each article we publish.
Find out more
ORIGINAL RESEARCH article
Front. Physiol. , 22 January 2025
Sec. Respiratory Physiology and Pathophysiology
Volume 15 - 2024 | https://doi.org/10.3389/fphys.2024.1509421
Background: In patients with chronic coronary syndromes (CCS), increased ventilation/carbon dioxide production (
Method: Patients with CCS, HF with reduced ejection fraction (EF < 50%), healthy controls (45+ years), and young healthy adults (<35 years) were recruited. For patients, a
Results: In patients with CCS, HF, controls, and young healthy adults, median
Conclusion: In patients with HF and/or CCS and excess ventilation, central chemoreflex VRT was reduced independently of the presence of HF. Low VRTs were related to resting excess ventilation in patients with CCS or HF; however, rapid shallow breathing at peak exercise was present only in patients with HF.
Clinical trial registration number: NCT05057884.
Chronic coronary syndromes (CCS) is the clinical presentation of coronary artery disease during stable periods, particularly those preceding or following an acute coronary syndrome (Vrints et al., 2024). CCS is the leading cause of heart failure (HF) (Ziaeian and Fonarow, 2016). HF is a clinical syndrome characterized by cardinal symptoms (e.g. breathlessness, ankle swelling, and fatigue) that may be accompanied by signs such as elevated jugular venous pressure, pulmonary crackles, and peripheral edema. It is due to a structural and/or functional abnormality of the heart, which results in elevated intra-cardiac pressures and/or inadequate cardiac output at rest and/or during exercise (McDonagh et al., 2021).
An exaggerated ventilatory response to exercise, often accompanied by early exertional dyspnea, is a hallmark in patients with chronic HF (Chua et al., 1996; Tomita et al., 2003). These observations have also been reported in patients with CCS and left ventricular dysfunction (Eser et al., 2023). Excess ventilation, often also termed ventilatory inefficiency (Neder et al., 2022), has not only been associated with reduced exercise capacity and a reduced quality of life but also with poorer prognosis in patients with HF and CCS (Ponikowski PP. et al., 2001; Arena et al., 2004; Nadruz et al., 2017; Chua et al., 1997a; Nayor et al., 2020). It is quantified by an increased
In patients with HF, impaired cardiac function may result in lung areas that are ventilated but poorly perfused (i.e., ventilation–perfusion mismatch) with
The respiratory chemoreflexes are responsible for controlling PCO2, such that tissue hydrogen ion concentration ([H+]) is constrained within viable limits for protein function. Both the central chemo-receptors, located in the medulla, and the peripheral chemoreceptors, located in the carotid bodies, respond to [H+], although they are most often modeled as responding to PCO2 (Duffin, 2005). The peripheral chemoreceptor response to [H+] is modulated by partial pressure of oxygen (PO2), whereas the central chemoreceptor response is not (Duffin, 2005). Due to this discrepancy, by administering hypercapnia under hyperoxic conditions, the peripheral chemoreceptors can be silenced, allowing the isolated measurement of central chemoreflex (Duffin, 2005). On the other hand, administering hypercapnia under hypoxic conditions triggers both central and peripheral chemoreflexes.
Increased ventilatory drive during exercise has also been found in patients with left ventricular dysfunction but without established HF (Eser et al., 2023). Chemosensitivity, however, has not been assessed so far in patients with CCS.
Most early studies have assessed peripheral chemosensitivity to hypoxia by transient inhalation of pure nitrogen (Chua et al., 1996; Ponikowski et al., 1997; Ponikowski P. et al., 2001). However, while hypoxia may occur in some patients during sleep apnea (Giannoni et al., 2019; Giannoni et al., 2008), hypoxia does not occur during exercise and is, therefore, unlikely to be related to inefficient ventilation. More recent studies have assessed respiratory chemosensitivity in patients with HF using isocapnic steady-state rebreathing to assess peripheral chemosensitivity to decreasing partial pressures of oxygen (PO2) and normoxic rebreathing to assess central chemosensitivity to increasing PCO2 (Giannoni et al., 2008; Giannoni et al., 2009) or Read’s rebreathing method (Tomita et al., 2003; Read, 1967). However, the normoxic isocapnic steady-state method used to measure central chemosensitivity has been found to not be reproducible, while normoxic rebreathing has very good reproducibility (Mannée et al., 2018). Furthermore, steady-state methods to measure peripheral chemosensitivity apply a low PO2 stimulus, with PCO2 held eucapnic or at a fixed increase in PCO2 above eucapnia. However, the choice of PCO2 markedly influences the measured peripheral chemosensitivity, and at least two tests at differing PCO2 are necessary (Keir et al., 2020a). Read’s rebreathing method has been refined by Duffin by introducing a 5-min hyperventilation period to lower the arterial PCO2. After hyperventilation and upon rebreathing, PCO2 increases while
The aims of the current study were to 1) compare central and peripheral respiratory chemoreflexes in terms of VRT and sensitivity measured by the Duffin rebreathing method in patients with inefficient ventilation and CCS to those with HF and age-matched controls; 2) assess the contribution of central and peripheral chemoreflexes to ventilatory efficiency; 3) compare resting breathing patterns in these patient groups; 4) identify age-related differences in central and peripheral chemoreflexes by comparing healthy old to healthy young volunteers.
This case–control study was conducted as a sub-study of the Breathe-HF trial (NCT05057884). Eligible patients with HF and CCS were identified and recruited during routine check-up visits at a tertiary care university cardiovascular referral center between April 2022 and April 2023. Healthy young and old volunteers were recruited by word of mouth. If they met the inclusion criteria and provided consent in writing, they were included in the study, and measurements were performed as summarized in Supplementary Figure S1. No follow-up was performed. The study was approved by the Ethics Committee of the Canton of Berne.
The study included four different groups of participants, two cardiac patient groups and two healthy control groups. Inclusion criteria for all groups were as follows: age 18–80 years, capability of performing a cardiopulmonary exercise test on a cycling ergometer, willingness to participate in a study of a total duration of 2 h, and provision of written informed consent. Exclusion criteria for all groups were as follows: currently smoking, non-cardiac conditions and comorbidities associated with hyperventilation such as pulmonary diseases, and pregnancy or lactation. Additional specific inclusion criteria for both patient groups were as follows: exertional dyspnea and
Participants of the patient and the control groups were recruited successively for the groups to be comparable with regard to age and sex. The young healthy adults group was included for comparison only and to assess the effect of age.
Body composition was assessed by bioelectrical impedance with a body composition analyzer (InBody 720, best4health gmbh, Bassersdorf, Switzerland). Weight, lean muscle mass, and body fat percentage were measured. Moreover, the body mass index (BMI) was calculated.
Central and peripheral chemosensitivity was assessed using the rebreathing protocol according to Duffin (2011). This protocol was chosen because it can directly and reliably determine the VRT, namely, the PETCO2 during hyper- and hypoxic conditions above which ventilation starts to increase, by establishing a PCO2 equilibrium between the arterial/mixed venous blood, the alveolar air, and the rebreathing bag. During hyperoxic test conditions, the peripheral chemoreflex response is reduced or eliminated, allowing quantification of the central chemoreflex only (Guluzade et al., 2023). The hypoxic test then allows measurement of the summed peripheral and central chemoreflex responses. This method also reduces the difference between end-tidal and arterial/mixed venous blood PO2 and PCO2 (Read and Leigh, 1967) and, therefore, provides the same stimulus to the central and peripheral chemoreceptors.
The prior hyperventilation of the rebreathing method by Duffin reduces PCO2 to below the central and peripheral chemoreceptor thresholds so that as PCO2 increases, the VRT can be determined. The ventilation below the VRT is a measure of the ventilation drive other than from the chemoreflexes, and since it is absent during sleep, it is therefore known as the wakefulness drive to breathe. During sleep, the VRT becomes the apnea threshold.
In contrast, other rebreathing methods extrapolate the regression line of the ventilation to PETCO2 slope to the abscissa, which creates larger errors (Jensen et al., 2010).
Patients were previously told to abstain from caffeine on the morning of the tests. The room was dimly lit and maintained at a comfortable 22°C. The rebreathing procedure was performed using the Innocor system (COSMED Nordic ApS, Odense S, Denmark) running on a Windows XP embedded operating system on an integrated computer and a pulse oximeter (NONIN, sampling frequency 100 Hz) for O2 saturation. During measurements, participants wore an EU-certified breathing mask (V2 Mask, Hans Rudolph, Shawnee, USA), as used during spiro-ergometries, covering the nose and mouth. While most rebreathing procedures mentioned in literature were carried out using a mouthpiece and nose clip, Keir et al. demonstrated the feasibility of using a breathing mask for rebreathing tests (Keir et al., 2019). The breathing mask bears the advantage of allowing the participants to breathe through the nose or mouth as desired.
Once participants were fitted with the mask, they rested in a comfortable chair for 10 min. Breath-by-breath data were collected for the following parameters during resting and rebreathing: oxygen consumption relative to body weight (
The participant then rested for 15 min without the mask, breathing room air. (Duffin and McAvoy, 1988). During this time, the bag was washed out three times and refilled to three-quarters with a hypoxic gas mixture of 4.5% O2 and 6% CO2 and balanced by N2. Hypoxic rebreathing was performed following the same protocol as before, but PETO2 was kept constant at 50 mmHg. The order of tests was kept constant for all participants to avoid the effects of hypoxia on chemosensitivity that can last several hours (Mateika et al., 1985). Parameters of the 10th minute of the resting period before the hyperoxic test (always first order) were averaged for resting values. For this period, the rapid shallow breathing index (RSBI) was also calculated as BF/VT and BF/VT relative to BSA.
Data analysis was performed as described by Duffin et al. (2000). Breath-by-breath PETCO2 was plotted against time and fitted with a least-squares regression line. In order to minimize inter-breath variability, the equation for this line provided a predicted value of PETCO2, against which
Exercise capacity was assessed with a CPET on a cycle ergometer. Prior to the test, spirometry was used to determine forced vital capacity (FCV, l) and forced expiratory volume in one second (FEV1, l*min-1). Then, after sitting on the ergometer quietly for 3 min, blood pressure was measured two times, and the lowest measurement was recorded. A 3-min warm-up was followed by an individually set ramp. Volumes, flows, and gases were sampled continuously in an open spirometric system (Quark, Cosmed, Rome, Italy) and averaged over eight breaths. Measured variables included
All analyses were performed by R (R Core Team, 2021, Version 4.1.0).
CCS, HF, control, and young healthy adult groups were defined as exposures. The primary outcome was central and peripheral VRT and chemosensitivity. The secondary outcomes were rapid RSBI and PETCO2 at rest.
Baseline characteristics were tested between groups by Kruskal–Wallis tests followed by post hoc testing adjusted for multiple testing by Benjamini–Hochberg correction. Categorical variables were tested by Fisher’s exact tests. Associations between variables were assessed by linear regression. Statistical significance for all tests was set at a p-value <0.05.
Each group included 15 participants (Supplementary Figure S1). Of 53 patients with CCS qualifying for the study (32.8% of screened patients had
There was mask leakage in one control during the hyperoxic test and in another control in the hypoxic test, leaving the results of only 14 subjects in this group for data analysis. During the hypoxic test, there was mask leakage in two patients with HF and four patients with CCS. Furthermore, one patient with HF and two patients with CCS stopped the hypoxic tests after 3–5 breaths, which did not allow the determination of VRT and slope. Therefore, only 12 patients were included for data analysis of the hypoxic test. A typical example of the sampled data of the two tests in one patient with HF and one age- and sex-matched control is shown in Supplementary Figure S2.
The HF and CCS patient groups had significantly reduced median hyperoxic test VRTs compared to controls (p = 0.004 and 0.01, respectively, Figure 1A; Table 2), but the hypoxic test VRTs did not differ (Figure 1B; Table 2). Young healthy adults had higher median VRTs than controls for both hyper- and hypoxic tests, which were not quite significant (p = 0.06 and p = 0.07, respectively).
Figure 1. Boxplots of the four study groups showing PETCO2 at hyperoxic VRT (A), PETCO2 at hypoxic VRT (B), PETCO2 at rest (C), and RSBI (D). VRTs were determined from rebreathing and RSBI and PETCO2 from resting measurement before rebreathing. VRT of the hyperoxic test was significant, and VRT of hypoxic test by trend reduced in both patient groups compared to that in controls. PETCO2 was significantly reduced in both patient groups compared to controls. RSBI was increased compared to controls only in patients with HF. * p-value<0.05, ** p-value<0.01; CHF, chronic heart failure; CCS, chronic coronary syndrome; PCO2, end-tidal carbon dioxide pressure; VRT, ventilatory response threshold; RSBI, rapid shallow breathing index; PETCO2, end-tidal carbon dioxide pressure.
In all groups, hypoxic test slopes were significantly higher than hyperoxic test slopes (all p < 0.004, Table 2). However, hyperoxic and hypoxic test slopes (sensitivity;
Linear inverse relationships between
Figure 2. Within-group linear regressions between
There was a significant positive linear correlation between hyperoxic and hypoxic VRT in the pooled sample (r2 = 0.68, p < 0.0001) and within each group (all p ≤ 0.05; Supplementary Figure S3A). Correlations between hyper- and hypoxic slopes were significant and positive for the pooled sample (r2 = 0.24; p < 0.0001) and within all groups (p < 0.006) except the CCS group (p = 0.201; Supplementary Figure S3B). PETCO2 at rest was positively related to hyperoxic and hypoxic test VRTs within all groups except for the hyperoxic VRT of the young healthy adults group (Figures 3A,B).
Figure 3. Within-group linear regressions between resting PETCO2 and hyper (A) and hypoxic VRT (B). There were strong positive within-group relationships in all groups except young healthy adults. These relationships indicate that a reduced VRT is connected to hyperventilation at rest. PCO2, end-tidal carbon dioxide pressure; VRT, ventilatory response threshold.
Resting parameters of the two patient groups were comparable to those of the control subjects except for PETCO2, which was lower in both patient groups (Figure 1C), and BF and RSBI (Figure 1D), which were higher in the HF group compared to that in the controls and patients with CCS (Supplementary Table S1). Based on the inclusion criteria for both patient groups, they had significantly higher
The present study selected patients with chronic HFrEF/HFmrEF or CCS who presented with exercise excess ventilation and compared their central and peripheral chemosensitivity and resting breathing pattern to that in age-matched controls and young healthy subjects. This is the first study that included two cohorts of well-phenotyped patients with HF (with reduced ejection fraction) and CCS and simultaneously assessed breathing efficiency at rest with standardized tests as well as at exercise with CPET-based measurements. Furthermore, the inclusion of both sexes and a young healthy group allowed a direct comparison with healthy subjects without ventilatory inefficiency and the effect of age on the measured parameters.
Key findings of the study were: 1) patients with CCS and HF had lower hyperoxic rebreathing test VRTs compared to control and young healthy subjects. 2) Central and peripheral chemosensitivity quantified by
A lower VRT in patients with HF has previously been found in patients with univentricular heart using both transient hypoxia and Read rebreathing tests (Chua et al., 1997b). Reduced VRT reflects excess ventilation (e.g. altitude hypoxia (Frost et al., 2024)), which in this case likely results from high or normal anion gap metabolic acidosis and reduced bicarbonate (Fenves and Emmett, 2021; Ren and Robbins, 1999). The VRT of the hyperoxic test depends on the buffering capacity of the brain fluid, and the VRT of the hypoxic tests depends on the buffering capacity of the blood (Duffin, 2005). PCO2 of the brain fluid and blood are correlated during rebreathing (Carr et al., 2023). This is likely the reason for the close association between hyper- and hypoxic VRTs (Supplementary Figure S3). Electrolyte derangement such as by hyponatremia, hypocalcemia, hypokalemia, hypomagnesemia, and hypophosphatemia are common in patients with HF, as documented by several studies (Milionis et al., 2002; Urso et al., 2015; Dei Cas et al., 1995). Importantly, central chemosensitivity has been found to be significantly reduced in patients with HF after intravenous iron infusion (Caravita et al., 2022). The fact that patients with CCS and HF had comparable values of reduced ventilatory efficiency, reduced VRT, and reduced PETCO2 at rest suggests that they had the same degree of excess ventilation, although with a different breathing pattern where only patients with HF developed a rapid shallow breathing pattern. This indicates that the HF-related congestion of the lung is likely responsible for the increased
Furthermore, hyperoxic and hypoxic rebreathing test
The age-related decrease in VRT for both hyperoxic and hypoxic rebreathing tests observed in our control and young healthy groups corresponds to findings of earlier studies (García-Río et al., 2007). Garcia-Rio and colleagues found the VRTs of both hyperoxic hypercapnic and isocapnic hypoxic stimulation in elderly subjects to be at a lower PETCO2 than in young healthy subjects (García-Río et al., 2007). However, they found that the hyperoxic hypercapnic VRT increased again after age 75.
Our findings of comparable VE/PETCO2 slopes of the hyper- and hypoxic rebreathing between our patient and healthy groups are in contrast to those of some previous studies that have reported increased central chemosensitivity to hypercapnia (Giannoni et al., 2008; Giannoni et al., 2009; Giannoni et al., 2022; Narkiewicz et al., 1999) and peripheral chemosensitivity to hypoxia in heart failure (Ponikowski et al., 1997; Giannoni et al., 2008; Giannoni et al., 2009; Giannoni et al., 2022; Chua et al., 1997c). Tomita et al. (2003) found increased chemosensitivity in patients with acute myocardial infarction using Read hyperoxic rebreathing tests, and peripheral chemosensitivity measured using the Duffin isoxic rebreathing method has been found to be associated with cardiovascular risk in a Chinese population (Dai et al., 2024).
We found no differences between hyperoxic and hypoxic slopes (sensitivity) between young healthy adults and controls, which is in agreement with previous findings. Increased chemosensitivity with increasing age was found during sleep (Chowdhuri et al., 2015), but another study found no differences between healthy younger and older men with transient hypoxia by rebreathing of pure nitrogen by another study (Paleczny et al., 2014).
Our study is the first to show that in patients with inefficient ventilation, resting PETCO2 is lower than that in controls regardless of left ventricular ejection fraction. While excess ventilation with exercise has been well-studied, resting excess ventilation has rarely been investigated. Resting PaCO2 <31 mmHg has been found to be associated with increased all-cause mortality (Kato et al., 2021). We have recently shown that increased resting breathing frequency was associated with major adverse events in patients with left ventricular dysfunction (Eser et al., 2023). The association of a lower resting PaCO2 with VRT or chemosensitivity has not been investigated, although in patients with HF, it has been found that Cheyenne Stokes respiration was associated with a lower resting PaCO2 (Naughton et al., 1993). It has been suggested that low PaCO2 in patients with HF may be a respiratory manifestation of elevated left ventricular filling pressures (Lorenzi-Filho et al., 2002). However, since we found the same relationships between VRT and resting PETCO2 in all groups (with healthy control groups and patients with CCS not having elevated left ventricular filling pressures), our data suggest that there may be a neurological or hormonal rather than a circulatory cause for the resting excess ventilation in patients with HF and CCS.
As in a recent validation study (Jensen et al., 2010), we found the Duffin hyperoxic and hypoxic rebreathing tests to be feasible in healthy controls but less feasible in patients with CCS or HF. One problem, particularly during the hypoxic test, was that after hyperventilation and the subsequent three breaths for equilibration, many patients took a break from breathing for a few seconds to recover from hyperventilation. When breathing resumed, they had already surpassed their VRT and stopped the test after only a few more breaths. The reduction in breaths reduced the quality of VRT and
Measurements of chemoreflexes are time-consuming, technically challenging, and uncomfortable for patients. The measurement of resting ventilation, however, is relatively easy in comparison, with the only requirement that patients need to sit calmly for 5 min and the face masks need to be tight. The close relationship between resting PETCO2 and hyper- and hypoxic VRTs indicates a deficit in buffering capacity of the blood in patients with HF or CCS and low PETCO2. Our previous findings from a study on patients with left ventricular dysfunction indicating higher incidence of major adverse cardiac events in patients with lower PETCO2 (Eser et al., 2023) indicate that resting ventilation may be an under-recognized and valuable prognostic marker.
The present study shows that 1) there was no difference between patients with HF and patients with CCS regarding VRT and chemosensitivity, and in both patient groups, VRT was lower compared to that in controls, while chemosensitivity was only slightly and insignificantly heightened in patients; 2) neither VRT nor chemosensitivity was associated with the degree of inefficient ventilation within any of the groups; 3) both patient groups had excess ventilation at rest; 4) neither VRT nor chemosensitivity was associated with age. The absence of differences regarding VRT and chemosensitivity between the two patient groups challenges the notion that inefficient ventilation and excessive ventilatory drive are due to circulatory weakness. On the other hand, the presence of rapid shallow breathing at rest found in patients with HF only was likely due to lung congestion.
The raw data supporting the conclusions of this article will be made available by the authors, without undue reservation.
The studies involving humans were approved by Ethikkommission des Kantons Bern. The studies were conducted in accordance with the local legislation and institutional requirements. The participants provided their written informed consent to participate in this study. Written informed consent was obtained from the individual(s) for the publication of any potentially identifiable images or data included in this article.
PE: conceptualization, data curation, formal analysis, investigation, methodology, project administration, resources, supervision, validation, visualization, writing–original draft, and writing–review and editing. DK: conceptualization, methodology, and writing–review and editing. PC: data curation, project administration, and writing–review and editing. AK: formal analysis, investigation, methodology, project administration, visualization, and writing–review and editing. LS: project administration, visualization, and writing–review and editing. SH: conceptualization, data curation, methodology, project administration, visualization, and writing–review and editing. JD: methodology, software, and writing–review and editing. MW: conceptualization, funding acquisition, investigation, methodology, supervision, and writing–review and editing.
The author(s) declare that no financial support was received for the research, authorship, and/or publication of this article.
The authors declare that the research was conducted in the absence of any commercial or financial relationships that could be construed as a potential conflict of interest.
The authors declare that no generative AI was used in the creation of this manuscript.
All claims expressed in this article are solely those of the authors and do not necessarily represent those of their affiliated organizations, or those of the publisher, the editors and the reviewers. Any product that may be evaluated in this article, or claim that may be made by its manufacturer, is not guaranteed or endorsed by the publisher.
The Supplementary Material for this article can be found online at: https://www.frontiersin.org/articles/10.3389/fphys.2024.1509421/full#supplementary-material
BMI, body mass index; BF, breathing frequency; CCS, chronic coronary syndromes; CO2, carbon dioxide; CPET, cardiopulmonary exercise test; HF, heart failure; HFpEF, heart failure with preserved ejection fraction; HFmrEF, heart failure with mildly reduced ejection fraction; HFrEF, heart failure with reduced ejection fraction; HR, heart rate; O2, oxygen; PaCO2, arterial partial pressure of carbon dioxide; PaO2, arterial partial pressure of oxygen; PETCO2, end-tidal partial pressure of carbon dioxide; PETO2, end-tidal partial pressure of oxygen; RPE, rate of perceived exertion; RSBI, rapid shallow breathing index; SpO2, oxygen saturation; VD, pulmonary dead space;
Agostoni P., Guazzi M. (2017). Exercise ventilatory inefficiency in heart failure: some fresh news into the roadmap of heart failure with preserved ejection fraction phenotyping. Eur. J. heart Fail. 19 (12), 1686–1689. doi:10.1002/ejhf.940
Aimo A., Saccaro L. F., Borrelli C., Fabiani I., Gentile F., Passino C., et al. (2021). The ergoreflex: how the skeletal muscle modulates ventilation and cardiovascular function in health and disease. Eur. J. heart Fail. 23 (9), 1458–1467. doi:10.1002/ejhf.2298
Arena R., Myers J., Aslam S. S., Varughese E. B., Peberdy M. A. (2004). Peak VO2 and VE/VCO2 slope in patients with heart failure: a prognostic comparison. Am. heart J. 147 (2), 354–360. doi:10.1016/j.ahj.2003.07.014
Boulet L. M., Tymko M. M., Jamieson A. N., Ainslie P. N., Skow R. J., Day T. A. (2016). Influence of prior hyperventilation duration on respiratory chemosensitivity and cerebrovascular reactivity during modified hyperoxic rebreathing. Exp. Physiol. 101 (7), 821–835. doi:10.1113/EP085706
Caravita S., Faini A., Vignati C., Pelucchi S., Salvioni E., Cattadori G., et al. (2022). Intravenous iron therapy improves the hypercapnic ventilatory response and sleep disordered breathing in chronic heart failure. Eur. J. heart Fail. 24 (10), 1940–1949. doi:10.1002/ejhf.2628
Carr J., Day T. A., Ainslie P. N., Hoiland R. L. (2023). The jugular venous-to-arterial PCO2 difference during rebreathing and end-tidal forcing: relationship with cerebral perfusion. J. physiology 601 (19), 4251–4262. doi:10.1113/JP284449
Chowdhuri S., Pranathiageswaran S., Franco-Elizondo R., Jayakar A., Hosni A., Nair A., et al. (2015). Effect of age on long-term facilitation and chemosensitivity during NREM sleep. J. Appl. Physiol. (1985) 119 (10), 1088–1096. doi:10.1152/japplphysiol.00030.2015
Chua T. P., Clark A. L., Amadi A. A., Coats A. J. (1996). Relation between chemosensitivity and the ventilatory response to exercise in chronic heart failure. J. Am. Coll. Cardiol. 27 (3), 650–657. doi:10.1016/0735-1097(95)00523-4
Chua T. P., Iserin L., Somerville J., Coats A. J. (1997b). Effects of chronic hypoxemia on chemosensitivity in patients with univentricular heart. J. Am. Coll. Cardiol. 30 (7), 1827–1834. doi:10.1016/s0735-1097(97)00360-4
Chua T. P., Ponikowski P., Harrington D., Anker S. D., Webb-Peploe K., Clark A. L., et al. (1997a). Clinical correlates and prognostic significance of the ventilatory response to exercise in chronic heart failure. J. Am. Coll. Cardiol. 29 (7), 1585–1590. doi:10.1016/s0735-1097(97)00078-8
Chua T. P., Ponikowski P., Webb-Peploe K., Harrington D., Anker S. D., Piepoli M., et al. (1997c). Clinical characteristics of chronic heart failure patients with an augmented peripheral chemoreflex. Eur. heart J. 18 (3), 480–486. doi:10.1093/oxfordjournals.eurheartj.a015269
Cross T. J., Kim C. H., Johnson B. D., Lalande S. (2020). The interactions between respiratory and cardiovascular systems in systolic heart failure. J. Appl. Physiol. (1985) 128 (1), 214–224. doi:10.1152/japplphysiol.00113.2019
Dai L., Guo J., Hui X., Wang X., Luo J., Huang R., et al. (2024). The potential interaction between chemosensitivity and the development of cardiovascular disease in obstructive sleep apnea. Sleep. Med. 114, 266–271. doi:10.1016/j.sleep.2024.01.010
Dei Cas L., Metra M., Leier C. V. (1995). Electrolyte disturbances in chronic heart failure: metabolic and clinical aspects. Clin. Cardiol. 18 (7), 370–376. doi:10.1002/clc.4960180704
Dubé B.-P., Agostoni P., Laveneziana P. (2016). Exertional dyspnoea in chronic heart failure: the role of the lung and respiratory mechanical factors. Eur. Respir. Rev. 25 (141), 317–332. doi:10.1183/16000617.0048-2016
Duffin J. (2005). Role of acid-base balance in the chemoreflex control of breathing. J. Appl. Physiol. (1985) 99 (6), 2255–2265. doi:10.1152/japplphysiol.00640.2005
Duffin J. (2007). Measuring the ventilatory response to hypoxia. J. physiology 584 (Pt 1), 285–293. doi:10.1113/jphysiol.2007.138883
Duffin J. (2011). Measuring the respiratory chemoreflexes in humans. Respir. Physiol. Neurobiol. 177 (2), 71–79. doi:10.1016/j.resp.2011.04.009
Duffin J., McAvoy G. V. (1988). The peripheral-chemoreceptor threshold to carbon dioxide in man. J. physiology 406, 15–26. doi:10.1113/jphysiol.1988.sp017365
Duffin J., Mohan R. M., Vasiliou P., Stephenson R., Mahamed S. (2000). A model of the chemoreflex control of breathing in humans: model parameters measurement. Respir. Physiol. 120 (1), 13–26. doi:10.1016/s0034-5687(00)00095-5
Eser P., Marcin T., Prescott E., Prins L. F., Kolkman E., Bruins W., et al. (2023). Breathing pattern and pulmonary gas exchange in elderly patients with and without left ventricular dysfunction-modification with exercise-based cardiac rehabilitation and prognostic value. Front. Cardiovasc Med. 10, 1219589. doi:10.3389/fcvm.2023.1219589
Fenves A. Z., Emmett M. (2021). Approach to patients with high anion gap metabolic acidosis: Core curriculum 2021. Am. J. Kidney Dis. 78 (4), 590–600. doi:10.1053/j.ajkd.2021.02.341
Frost S., Pham K., Puvvula N., Oeung B., Heinrich E. C. (2024). Changes in hypoxic and hypercapnic ventilatory responses at high altitude measured using rebreathing methods. J. Appl. Physiol., 2024. doi:10.1152/japplphysiol.00128.2024
García-Río F., Villamor A., Gómez-Mendieta A., Lores V., Rojo B., Ramírez T., et al. (2007). The progressive effects of ageing on chemosensitivity in healthy subjects. Respir. Med. 101 (10), 2192–2198. doi:10.1016/j.rmed.2007.04.015
Giannoni A., Emdin M., Bramanti F., Iudice G., Francis D. P., Barsotti A., et al. (2009). Combined increased chemosensitivity to hypoxia and hypercapnia as a prognosticator in heart failure. J. Am. Coll. Cardiol. 53 (21), 1975–1980. doi:10.1016/j.jacc.2009.02.030
Giannoni A., Emdin M., Poletti R., Bramanti F., Prontera C., Piepoli M., et al. (2008). Clinical significance of chemosensitivity in chronic heart failure: influence on neurohormonal derangement, Cheyne-Stokes respiration and arrhythmias. Clin. Sci. Lond. 114 (7), 489–497. doi:10.1042/CS20070292
Giannoni A., Gentile F., Buoncristiani F., Borrelli C., Sciarrone P., Spiesshoefer J., et al. (2022). Chemoreflex and baroreflex sensitivity hold a strong prognostic value in chronic heart failure. JACC Heart Fail 10, 662–676. doi:10.1016/j.jchf.2022.02.006
Giannoni A., Gentile F., Navari A., Borrelli C., Mirizzi G., Catapano G., et al. (2019). Contribution of the lung to the genesis of Cheyne-Stokes respiration in heart failure: plant gain beyond chemoreflex gain and circulation time. J. Am. Heart Assoc. 8 (13), e012419. doi:10.1161/JAHA.119.012419
Guluzade N. A., Huggard J. D., Duffin J., Keir D. A. (2023). A test of the interaction between central and peripheral respiratory chemoreflexes in humans. J. physiology 601 (20), 4591–4609. doi:10.1113/JP284772
Jensen D., Mask G., Tschakovsky M. E. (2010). Variability of the ventilatory response to Duffin's modified hyperoxic and hypoxic rebreathing procedure in healthy awake humans. Respir. Physiol. Neurobiol. 170 (2), 185–197. doi:10.1016/j.resp.2009.12.007
Jensen D., Wolfe L. A., O'Donnell D. E., Davies G. A. (1985)2005). Chemoreflex control of breathing during wakefulness in healthy men and women. J. Appl. Physiol. 98 (3), 822–828. doi:10.1152/japplphysiol.01208.2003
Kato T., Kasai T., Suda S., Sato A., Ishiwata S., Yatsu S., et al. (2021). Prognostic effects of arterial carbon dioxide levels in patients hospitalized into the cardiac intensive care unit for acute heart failure. Eur. Heart J. Acute Cardiovasc Care 10 (5), 497–502. doi:10.1093/ehjacc/zuab001
Keir D. A., Duffin J., Badrov M. B., Alba A. C., Floras J. S. (2020b). Hypercapnia during wakefulness attenuates ventricular ectopy: observations in a young man with heart failure with reduced ejection fraction. Circ. Heart Fail. 13 (6), e006837. doi:10.1161/CIRCHEARTFAILURE.119.006837
Keir D. A., Duffin J., Floras J. S. (2020a). Measuring peripheral chemoreflex hypersensitivity in heart failure. Front. physiology 11, 595486. doi:10.3389/fphys.2020.595486
Keir D. A., Duffin J., Millar P. J., Floras J. S. (2019). Simultaneous assessment of central and peripheral chemoreflex regulation of muscle sympathetic nerve activity and ventilation in healthy young men. J. physiology 597 (13), 3281–3296. doi:10.1113/JP277691
Lorenzi-Filho G., Azevedo E. R., Parker J. D., Bradley T. D. (2002). Relationship of carbon dioxide tension in arterial blood to pulmonary wedge pressure in heart failure. Eur. Respir. J. 19 (1), 37–40. doi:10.1183/09031936.02.00214502
Mannée D. C., Fabius T. M., Wagenaar M., Eijsvogel M. M. M., de Jongh F. H. C. (2018). Reproducibility of hypercapnic ventilatory response measurements with steady-state and rebreathing methods. ERJ Open Res. 4 (1), 00141-2017. doi:10.1183/23120541.00141-2017
Marcin T., Eser P., Prescott E., Prins L. F., Kolkman E., Bruins W., et al. (2020). Training intensity and improvements in exercise capacity in elderly patients undergoing European cardiac rehabilitation - the EU-CaRE multicenter cohort study. PloS one 15 (11), e0242503. doi:10.1371/journal.pone.0242503
Mateika J. H., Mendello C., Obeid D., Badr M. S. (1985)2004). Peripheral chemoreflex responsiveness is increased at elevated levels of carbon dioxide after episodic hypoxia in awake humans. J. Appl. Physiol. 96 (3), 1197–1196. doi:10.1152/japplphysiol.00573.2003
McDonagh T. A., Metra M., Adamo M., Gardner R. S., Baumbach A., Böhm M., et al. (2021). 2021 ESC Guidelines for the diagnosis and treatment of acute and chronic heart failure. Eur. heart J. 42 (36), 3599–3726. doi:10.1093/eurheartj/ehab368
Milionis H. J., Alexandrides G. E., Liberopoulos E. N., Bairaktari E. T., Goudevenos J., Elisaf M. S. (2002). Hypomagnesemia and concurrent acid-base and electrolyte abnormalities in patients with congestive heart failure. Eur. J. heart Fail. 4 (2), 167–173. doi:10.1016/s1388-9842(01)00234-3
Mohan R. M., Amara C. E., Cunningham D. A., Duffin J. (1999). Measuring central-chemoreflex sensitivity in man: rebreathing and steady-state methods compared. Respir. Physiol. 115 (1), 23–33. doi:10.1016/s0034-5687(99)00003-1
Nadruz W., West E., Sengelov M., Santos M., Groarke J. D., Forman D. E., et al. (2017). Prognostic value of cardiopulmonary exercise testing in heart failure with reduced, midrange, and preserved ejection fraction. J. Am. Heart Assoc. 6 (11), e006000. doi:10.1161/JAHA.117.006000
Narkiewicz K., Pesek C. A., van de Borne P. J., Kato M., Somers V. K. (1999). Enhanced sympathetic and ventilatory responses to central chemoreflex activation in heart failure. Circulation 100 (3), 262–267. doi:10.1161/01.cir.100.3.262
Naughton M., Benard D., Tam A., Rutherford R., Bradley T. D. (1993). Role of hyperventilation in the pathogenesis of central sleep apneas in patients with congestive heart failure. Am. Rev. Respir. Dis. 148 (2), 330–338. doi:10.1164/ajrccm/148.2.330
Nayor M., Xanthakis V., Tanguay M., Blodgett J. B., Shah R. V., Schoenike M., et al. (2020). Clinical and hemodynamic associations and prognostic implications of ventilatory efficiency in patients with preserved left ventricular systolic function. Circ. Heart Fail. 13 (5), e006729. doi:10.1161/CIRCHEARTFAILURE.119.006729
Neder J. A., Phillips D. B., O'Donnell D. E., Dempsey J. A. (2022). Excess ventilation and exertional dyspnoea in heart failure and pulmonary hypertension. Eur. Respir. J. 60 (5), 2200144. doi:10.1183/13993003.00144-2022
Paleczny B., Niewiński P., Rydlewska A., Piepoli M. F., Borodulin-Nadzieja L., Jankowska E. A., et al. (2014). Age-related reflex responses from peripheral and central chemoreceptors in healthy men. Clin. Auton. Res. 24 (6), 285–296. doi:10.1007/s10286-014-0263-9
Piepoli M., Clark A. L., Volterrani M., Adamopoulos S., Sleight P., Coats A. J. (1996). Contribution of muscle afferents to the hemodynamic, autonomic, and ventilatory responses to exercise in patients with chronic heart failure: effects of physical training. Circulation 93 (5), 940–952. doi:10.1161/01.cir.93.5.940
Piepoli M., Ponikowski P., Clark A. L., Banasiak W., Capucci A., Coats A. J. S. (1999). A neural link to explain the “muscle hypothesis” of exercise intolerance in chronic heart failure. Am. heart J. 137 (6), 1050–1056. doi:10.1016/s0002-8703(99)70361-3
Ponikowski P., Chua T. P., Anker S. D., Francis D. P., Doehner W., Banasiak W., et al. (2001b). Peripheral chemoreceptor hypersensitivity: an ominous sign in patients with chronic heart failure. Circulation 104 (5), 544–549. doi:10.1161/hc3101.093699
Ponikowski P., Chua T. P., Piepoli M., Ondusova D., Webb-Peploe K., Harrington D., et al. (1997). Augmented peripheral chemosensitivity as a potential input to baroreflex impairment and autonomic imbalance in chronic heart failure. Circulation 96 (8), 2586–2594. doi:10.1161/01.cir.96.8.2586
Ponikowski P. P., Chua T. P., Francis D. P., Capucci A., Coats A. J., Piepoli M. F. (2001a). Muscle ergoreceptor overactivity reflects deterioration in clinical status and cardiorespiratory reflex control in chronic heart failure. Circulation 104 (19), 2324–2330. doi:10.1161/hc4401.098491
Read D. J. (1967). A clinical method for assessing the ventilatory response to carbon dioxide. Australas. Ann. Med. 16 (1), 20–32. doi:10.1111/imj.1967.16.1.20
Read D. J., Leigh J. (1967). Blood-brain tissue Pco2 relationships and ventilation during rebreathing. J. Appl. Physiol. 23 (1), 53–70. doi:10.1152/jappl.1967.23.1.53
Ren X., Robbins P. A. (1999). Ventilatory responses to hypercapnia and hypoxia after 6 h passive hyperventilation in humans. J. physiology 514 (Pt 3), 885–894. doi:10.1111/j.1469-7793.1999.885ad.x
Scott A. C., Davies L. C., Coats A. J., Piepoli M. (2002). Relationship of skeletal muscle metaboreceptors in the upper and lower limbs with the respiratory control in patients with heart failure. Clin. Sci. Lond. 102 (1), 23–30. doi:10.1042/cs20010077
Tomita T., Takaki H., Hara Y., Sakamaki F., Satoh T., Takagi S., et al. (2003). Attenuation of hypercapnic carbon dioxide chemosensitivity after postinfarction exercise training: possible contribution to the improvement in exercise hyperventilation. Heart 89 (4), 404–410. doi:10.1136/heart.89.4.404
Urso C., Brucculeri S., Caimi G. (2015). Acid-base and electrolyte abnormalities in heart failure: pathophysiology and implications. Heart Fail. Rev. 20 (4), 493–503. doi:10.1007/s10741-015-9482-y
Vrints C., Andreotti F., Koskinas K. C., Rossello X., Adamo M., Ainslie J., et al. (2024). 2024 ESC Guidelines for the management of chronic coronary syndromes: developed by the task force for the management of chronic coronary syndromes of the European Society of Cardiology (ESC) Endorsed by the European Association for Cardio-Thoracic Surgery (EACTS). Eur. heart J. 45 (36), 3415–3537. doi:10.1093/eurheartj/ehae177
Wang M. C., Corbridge T. C., McCrimmon D. R., Walter J. M. (2020). Teaching an intuitive derivation of the clinical alveolar equations: mass balance as a fundamental physiological principle. Adv. Physiol. Educ. 44 (2), 145–152. doi:10.1152/advan.00064.2019
Weatherald J., Sattler C., Garcia G., Laveneziana P. (2018). Ventilatory response to exercise in cardiopulmonary disease: the role of chemosensitivity and dead space. Eur. Respir. J. 51 (2), 1700860. doi:10.1183/13993003.00860-2017
Keywords: inefficient ventilation, central chemosensitivity, cardiopulmonary exercise testing, resting ventilation, chronic coronary syndrome
Citation: Eser P, Käesermann D, Calamai P, Kalberer A, Stütz L, Huber S, Duffin J and Wilhelm M (2025) Excess ventilation and chemosensitivity in patients with inefficient ventilation and chronic coronary syndrome or heart failure: a case–control study. Front. Physiol. 15:1509421. doi: 10.3389/fphys.2024.1509421
Received: 10 October 2024; Accepted: 31 December 2024;
Published: 22 January 2025.
Edited by:
Francesco Gentile, Institute of Life Sciences, ItalyReviewed by:
Sabrina Montuoro, Health Science Interdisciplinary Center, ItalyCopyright © 2025 Eser, Käesermann, Calamai, Kalberer, Stütz, Huber, Duffin and Wilhelm. This is an open-access article distributed under the terms of the Creative Commons Attribution License (CC BY). The use, distribution or reproduction in other forums is permitted, provided the original author(s) and the copyright owner(s) are credited and that the original publication in this journal is cited, in accordance with accepted academic practice. No use, distribution or reproduction is permitted which does not comply with these terms.
*Correspondence: Prisca Eser, cHJpc2NhLmVzZXJAaW5zZWwuY2g=
Disclaimer: All claims expressed in this article are solely those of the authors and do not necessarily represent those of their affiliated organizations, or those of the publisher, the editors and the reviewers. Any product that may be evaluated in this article or claim that may be made by its manufacturer is not guaranteed or endorsed by the publisher.
Research integrity at Frontiers
Learn more about the work of our research integrity team to safeguard the quality of each article we publish.